- 1Department of Geriatrics, Nanjing Central Hospital, Nanjing, China
- 2Department of General Medicine, Zhongda Hospital, School of Medicine, Southeast University, Nanjing, China
- 3Department of Geriatrics, Zhongda Hospital, School of Medicine, Southeast University, Nanjing, China
- 4Department of Cardiology, Yangpu Hospital, Tongji University School of Medicine, Shanghai, China
Retinol-binding protein 4 (RBP4) has emerged as a critical adipokine involved in the pathophysiology of metabolic and cardiovascular diseases. Beyond its classical role in retinol transport, RBP4 influences insulin resistance, inflammation, lipid metabolism, mitochondrial function, and cellular apoptosis in both skeletal and cardiac muscles. Elevated levels of RBP4 are associated with obesity, type 2 mellitus diabetes, and cardiovascular diseases, making it a potential biomarker and therapeutic target. This comprehensive review elucidates the molecular mechanisms by which RBP4 affects skeletal and cardiac muscle physiology. We discuss its clinical implications as a biomarker for disease risk and progression, explore therapeutic strategies targeting RBP4, and highlight future research directions. Understanding the multifaceted roles of RBP4 could pave the way for novel interventions against metabolic and cardiovascular disorders.
1 Introduction
Retinol-binding protein 4 (RBP4) is a 21-kDa secreted protein belonging to the lipocalin family, primarily synthesized in the liver and adipose tissue, serving as the main transport protein for retinol (vitamin A alcohol) in the bloodstream (Kanai et al., 1968; Peterson, 1971; Blaner, 1989). Beyond its traditional role, RBP4 functions as an adipokine involved in regulating glucose metabolism and insulin sensitivity (Yang et al., 2005; Graham et al., 2006). Elevated RBP4 levels have been observed in insulin-resistant states such as obesity and type 2 diabetes mellitus (T2DM), implicating it in the development of metabolic syndrome (Klöting et al., 2007; Kovacs et al., 2007; Olsen and Blomhoff, 2020; Flores-Cortez et al., 2022). Skeletal muscle, accounting for approximately 80% of insulin-stimulated glucose disposal, is critical for glucose uptake and utilization (DeFronzo and Tripathy, 2009). Cardiac muscle relies on efficient energy metabolism for continuous contractile activity. Dysregulation of RBP4 affects both muscle types, leading to insulin resistance in skeletal muscle and contributing to cardiac remodeling, hypertrophy, and dysfunction (Nono and Blüher, 2021; Li et al., 2024; Ji et al., 2022; Schiborn et al., 2022). Recent studies highlight RBP4’s role in promoting cardiomyocyte injury and pyroptosis post-myocardial infarction and its association with adverse cardiovascular events (Zhang et al., 2021; Zhou et al., 2021; Qian et al., 2022; Chen et al., 2021). Understanding RBP4’s mechanisms in these tissues is crucial for developing targeted therapies for metabolic and cardiovascular diseases. The study of RBP4 has evolved significantly since its initial characterization, incorporating insights from various disciplines, including endocrinology, immunology, and molecular biology. This interdisciplinary approach has been crucial in unraveling the complex roles of RBP4 in metabolic and cardiovascular health. As we delve into the molecular mechanisms and clinical implications of RBP4, it is important to consider both the advancements and the challenges in this field of research.
2 Molecular mechanisms of RBP4 in skeletal muscle
RBP4 affects skeletal muscle through a complex network of molecular interactions, influencing insulin signaling, inflammation, lipid metabolism, and muscle physiology. These mechanisms are intricately intertwined, creating a multifaceted impact on muscle function and metabolic health. The following sections will explore each of these aspects in detail, highlighting the latest research findings and their implications for muscle biology.
2.1 Impairment of insulin signaling
RBP4 impairs insulin signaling by interfering with insulin receptor substrate (IRS) proteins. Elevated RBP4 levels reduce tyrosine phosphorylation of IRS-1, diminishing its ability to activate downstream signaling molecules like phosphatidylinositol 3-kinase (PI3K) and protein kinase B (Akt) (Craig et al., 2007; Janke et al., 2006). This results in decreased glucose transporter type 4 (GLUT4) translocation to the plasma membrane, reducing glucose uptake (Suh et al., 2010). RBP4 may induce suppression of cytokine signaling 3, which binds to IRS-1 and inhibits its activation (Norseen et al., 2012). Additionally, RBP4 activates c-Jun N-terminal kinase (JNK), leading to serine phosphorylation of IRS-1, further impairing insulin signaling (Sabio and Davis, 2010). Downregulation of GLUT4 expression involves inhibition of myocyte enhancer factor 2 and GLUT4 enhancer factor, key transcription factors regulating GLUT4 gene expression (Zorzano et al., 2005; Handschin and Spiegelman, 2008). Moreover, RBP4 affects insulin signaling by activating the retinol transport receptor STRA6, leading to downstream effects on JAK2/STAT3 signaling pathways (Huang et al., 2021; Perumalsamy et al., 2021), promoting gene expression that interferes with insulin action.
2.2 Promotion of inflammation
Chronic low-grade inflammation is a hallmark of insulin resistance. RBP4 stimulates pro-inflammatory cytokines such as tumor necrosis factor-alpha (TNF-α), interleukin-6 (IL-6), and interleukin-1β (IL-1β) in skeletal muscle cells and infiltrating macrophages (Moraes-Vieira et al., 2014; Hotamisligil, 2006). These cytokines activate inhibitory kinases such as IκB kinase (IKK) and JNK, which phosphorylate IRS-1 on serine residues, impairing insulin signaling (Nono and Blüher, 2021). RBP4 binds to toll-like receptor 4 on muscle cells, triggering nuclear factor kappa-light-chain-enhancer of activated B cells (NF-κB) and mitogen-activated protein kinases (MAPKs) pathways, promoting inflammatory gene transcription and contributing to insulin resistance (Flores-Cortez et al., 2022). This suggests RBP4 acts as a pro-inflammatory mediator, linking metabolic stress to inflammatory pathways. Numerous clinical studies have demonstrated strong correlations between elevated RBP4 levels and increased inflammatory markers, including high-sensitivity C-reactive protein (hsCRP), TNF-α, and IL-6 (Bujak and Frangogiannis, 2007a). Importantly, longitudinal interventional studies have demonstrated that reductions in RBP4 levels through exercise, dietary modifications, or combined interventions are accompanied by parallel improvements in metabolic parameters, suggesting a causal relationship rather than a mere association (Flores-Cortez et al., 2022; Ghorbanian et al., 2023a; Ghorbanian and Saberi, 2021). For instance, Ghorbanian et al. (2022) demonstrated that a 12-week aerobic exercise intervention significantly reduced serum RBP4 levels by approximately 11% (P = 0.001) in men with metabolic syndrome, while a combined intervention with ketogenic diet produced an even more pronounced reduction of 23.1% (P = 0.020). These reductions in RBP4 levels were significantly correlated with improvements in insulin resistance markers, as evidenced by decreased HOMA-IR values (P = 0.001). Notably, when comparing intervention groups, both ketogenic diet alone (P = 0.041) and combined exercise-diet intervention (P = 0.017) resulted in significantly lower RBP4 levels compared to controls, highlighting the potential therapeutic value of targeting RBP4 through lifestyle interventions (Ghorbanian et al., 2023a). RBP4 also activates antigen-presenting cells, leading to adipose tissue inflammation and systemic insulin resistance (Schiborn et al., 2022), and the muscle-adipose tissue crosstalk mediated by RBP4 exacerbates metabolic dysfunction.
2.3 Alteration of lipid metabolism
Altered lipid metabolism contributes to insulin resistance. RBP4 suppresses peroxisome proliferator-activated receptor alpha (PPARα) and its target genes involved in fatty acid β-oxidation, like carnitine palmitoyltransferase I (Amengual et al., 2008; Carrasco et al., 2023). This leads to the accumulation of intramyocellular lipids and intermediates like diacylglycerol and ceramides, activating protein kinase C isoforms that phosphorylate IRS-1 on serine residues, inhibiting insulin signaling (Itani et al., 2002). RBP4 upregulates sterol regulatory element-binding protein 1c, enhancing lipogenic enzyme expression and contributing to lipid accumulation and insulin resistance (Eberlé et al., 2004). Additionally, RBP4 may interfere with adipose triglyceride lipase and hormone-sensitive lipase, which are crucial for lipid mobilization, disrupting lipid homeostasis (Steinhoff et al., 2024) and exacerbating ectopic lipid deposition in skeletal muscle.
2.4 Impact on mitochondrial function and oxidative stress
RBP4 decreases peroxisome proliferator-activated receptor gamma coactivator 1-alpha (PGC-1α), a regulator of mitochondrial biogenesis (Patti et al., 2003), leading to reduced mitochondrial content, decreased oxidative capacity, and energy production. Mitochondrial dysfunction results in the overproduction of reactive oxygen species (ROS) (Anderson et al., 2009), causing oxidative damage to cellular components and activating stress kinases such as JNK and IKK, further impairing insulin signaling (Houstis et al., 2006). RBP4 may impair mitochondrial dynamics by affecting fusion and fission balance, leading to fragmented mitochondria and reduced adenosine triphosphate (ATP) production (Jheng et al., 2012), exacerbating metabolic dysfunction in skeletal muscle.
2.5 Influence on muscle fiber type and composition
RBP4 influences muscle fiber type distribution by promoting a shift from oxidative type I fibers to glycolytic type II fibers (Schiaffino and Reggiani, 2011). Glycolytic fibers are less efficient in glucose oxidation and more susceptible to insulin resistance. RBP4 may downregulate PGC-1α and affect transcription factors involved in fiber type specification (Lin et al., 2005), reducing oxidative capacity and impairing endurance, which may affect muscle performance and contribute to fatigue in metabolic disorders. The shift in muscle fiber type composition induced by RBP4 not only affects metabolic properties but also has significant implications for muscle strength and endurance. Type I fibers, which are more oxidative, are crucial for sustained, low-intensity activities, while type II fibers are essential for high-intensity, short-duration activities. The RBP4-induced shift toward type II fibers may contribute to reduced endurance capacity and increased fatigability, potentially exacerbating the physical limitations often observed in metabolic disorders (Schiaffino and Reggiani, 2011; Lin et al., 2005). This interplay between RBP4 and muscle fiber type adds another layer to our understanding of how metabolic dysregulation can impact overall physical function and quality of life.
2.6 Modulation of autophagy
RBP4 impairs autophagy by activating the mechanistic target of rapamycin complex 1, inhibiting autophagy initiation (Mizushima and Komatsu, 2011). Impaired autophagy leads to the accumulation of dysfunctional mitochondria and proteins, contributing to insulin resistance, oxidative stress, and inflammation, further impairing metabolic function. Enhancing autophagy may represent a therapeutic strategy to mitigate RBP4-induced metabolic dysfunction.
2.7 Induction of muscle atrophy and pyroptosis
RBP4 promotes muscle-specific ubiquitin ligases, such as atrogin-1 and muscle RING-finger protein-1, increasing protein degradation (Bodine et al., 2001). Zhang et al. demonstrated that RBP4 exacerbates denervation-induced muscle atrophy via the STRA6-dependent pathway (Zhang et al., 2024). RBP4 induces pyroptosis in muscle cells by interacting with NOD-, LRR- and pyrin domain-containing protein 3 (NLRP3) inflammasomes (Zhang et al., 2021; Zhang et al., 2024), contributing to muscle cell loss and atrophy. Pyroptosis amplifies inflammatory responses, leading to further tissue damage and functional impairment.
To clearly illustrate the multiple pathways through which RBP4 affects skeletal muscle, Figure 1 provides a schematic representation of the molecular mechanisms by which RBP4 mediates insulin resistance in skeletal muscle cells.
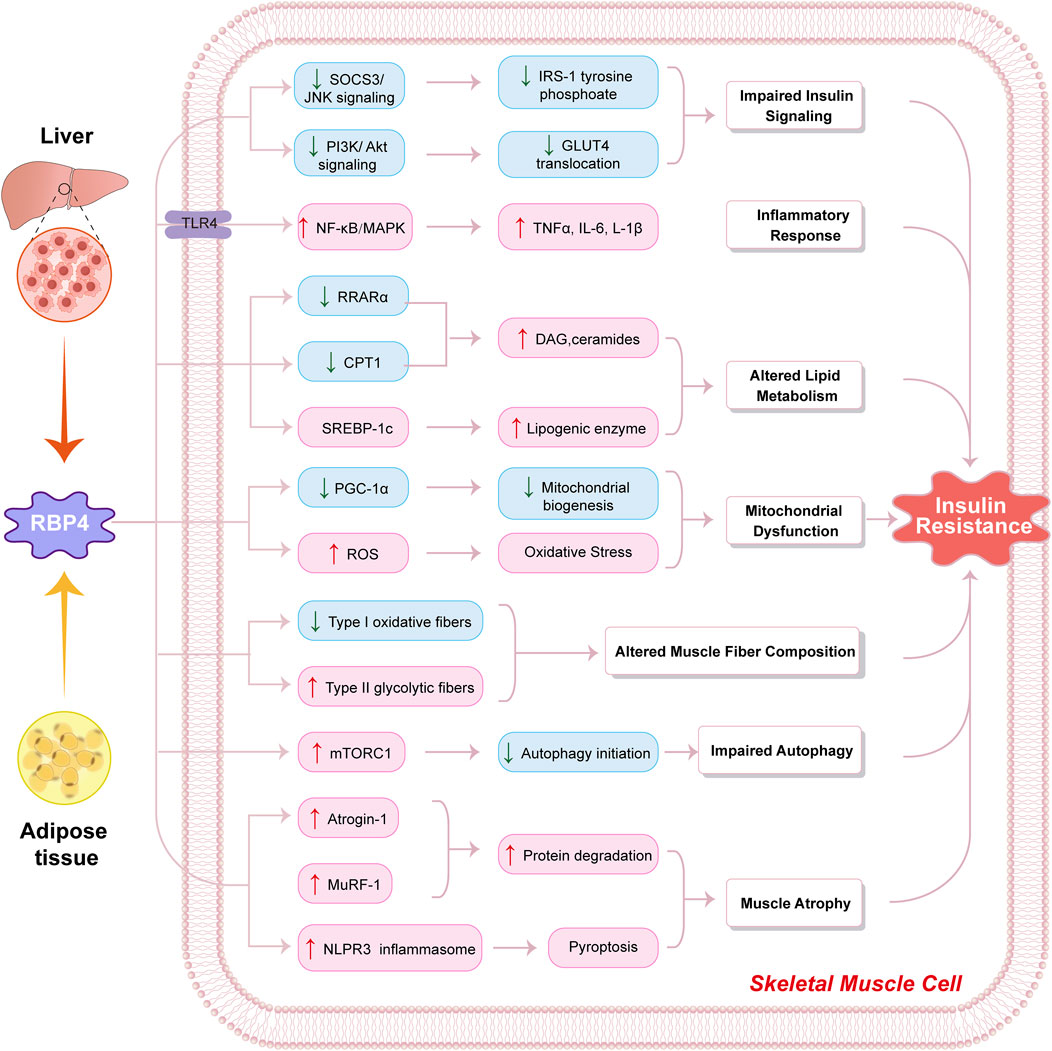
Figure 1. Molecular mechanisms by which RBP4 influences skeletal muscle metabolism. Schematic illustration of how retinol-binding protein 4 (RBP4) derived from liver and adipose tissue induces insulin resistance in skeletal muscle cells through multiple pathways. RBP4 triggers: (1) Impaired insulin signaling via decreased SOCS3/JNK and PI3K/Akt signaling, leading to reduced IRS-1 tyrosine phosphorylation and GLUT4 translocation; (2) Inflammatory response through TLR4-mediated activation of NF-κB/MAPK pathway, resulting in increased production of inflammatory cytokines (TNFα, IL-6, L-1β); (3) Altered lipid metabolism through downregulation of RRARα and CPT1, and activation of SREBP-1c, leading to increased DAG/ceramides and lipogenic enzyme levels; (4) Mitochondrial dysfunction via decreased PGC-1α-mediated mitochondrial biogenesis and increased ROS production causing oxidative stress; (5) Altered muscle fiber composition showing decreased Type I oxidative fibers and increased Type II glycolytic fibers; (6) Impaired autophagy through mTORC1 activation and reduced autophagy initiation; and (7) Muscle atrophy via upregulation of Atrogin-1, MuRF-1, and NLPR3 inflammasome activation leading to increased protein degradation and pyroptosis. These molecular alterations collectively contribute to the development of insulin resistance in skeletal muscle cells. Blue boxes indicate decreased expression/activity; pink boxes indicate increased expression/activity. Arrows indicate activation/induction; lines indicate inhibition.
3 Molecular mechanisms of RBP4 in cardiac muscle
In cardiac muscle, RBP4 plays a crucial role in various pathophysiological processes, including cardiac remodeling, fibrosis, oxidative stress, and energy metabolism. The effects of RBP4 on the heart are multifaceted, involving complex signaling cascades and interactions with various cellular components. Understanding these mechanisms is essential for developing targeted therapies for cardiovascular diseases associated with metabolic dysfunction.
3.1 Promotion of cardiac hypertrophy
RBP4 promotes cardiomyocyte hypertrophy through activation of the MAPK/ERK pathway, stimulating ERK1/2 phosphorylation and enhancing hypertrophic gene transcription (Liu et al., 2021; Gao et al., 2016), increasing expression of atrial natriuretic factor and brain natriuretic peptide. RBP4 stimulates the PI3K/Akt/mTOR pathway, leading to increased protein synthesis and cell growth (Molkentin, 2004). RBP4 may increase intracellular calcium levels, activating calcineurin, which dephosphorylates the nuclear factor of activated T-cells, promoting hypertrophic gene expression (Wilkins and Molkentin, 2004). Additionally, RBP4 can modulate microRNA expression in cardiomyocytes, influencing gene networks associated with hypertrophy and fibrosis (Zhao et al., 2021), adding an epigenetic layer to its impact on cardiac remodeling.
3.1.1 Induction of cardiac fibrosis
RBP4 increases transforming growth factor-beta one and connective tissue growth factor expression in cardiac fibroblasts (Bujak and Frangogiannis, 2007b), stimulating fibroblast activation and collagen synthesis. RBP4 alters matrix metalloproteinase activity, disrupting the balance between matrix degradation and synthesis (Lindsey et al., 2016), and may influence the phenotypic transformation of vascular smooth muscle cells under high-glucose conditions via the RhoA/ROCK1 pathway (Zhou et al., 2023), contributing to vascular remodeling and stiffness. The resulting fibrosis impairs myocardial compliance and contributes to diastolic dysfunction.
3.2 Enhancement of oxidative stress and apoptosis
RBP4 enhances ROS production by activating NADPH oxidase components (Takac et al., 2011) and impairing electron transport chain function (Madamanchi and Runge, 2007), leading to excess ROS. Elevated ROS levels induce oxidative damage and activate apoptotic pathways in cardiomyocytes. RBP4 modulates Bcl-2 family proteins, decreasing anti-apoptotic Bcl-2 and increasing pro-apoptotic Bax, leading to caspase activation (Whelan et al., 2012; Elmore, 2007). The intrinsic apoptotic pathway culminates in cardiomyocyte death, contributing to cardiac dysfunction and remodeling.
3.3 Impairment of endothelial function
RBP4 reduces endothelial nitric oxide synthase expression and activity, decreasing nitric oxide (NO) production (Kim et al., 2006), leading to vasoconstriction and impaired vasodilation. RBP4 increases adhesion molecules and chemokine expression on endothelial cells (Gimbrone and García-Cardeña, 2016), facilitating leukocyte adhesion and contributing to atherogenesis. Elevated RBP4 levels are associated with increased arterial stiffness (Armeni et al., 2021), underscoring its role in vascular aging and stiffness.
3.3.1 Disruption of calcium homeostasis
RBP4 affects sarcoplasmic reticulum Ca2+-ATPase, decreasing its expression and impairing calcium reuptake (Bers, 2002), leading to diastolic calcium overload and systolic dysfunction. It alters the ryanodine receptor function, affecting calcium release during excitation-contraction coupling (Hegyi et al., 2019). Disrupted calcium homeostasis activates pathways promoting hypertrophic gene expression (Wilkins and Molkentin, 2004; Sag et al., 2009), contributing to cardiac dysfunction.
3.3.1.1 Alteration of cardiac energy metabolism
RBP4 decreases PPARα activity, reducing fatty acid oxidation gene expression (Finck and Kelly, 2006) and shifting substrate utilization toward glucose metabolism. Under stress conditions, reliance on glucose metabolism is less efficient and may exacerbate energy deficits. RBP4 impairs mitochondrial biogenesis and function, reducing ATP production and contributing to energy deficits in the myocardium (Lesnefsky et al., 2001), impairing contractile function, and promoting pathological remodeling.
3.3.2 Induction of cardiomyocyte pyroptosis
RBP4 induces pyroptosis in cardiomyocytes by interacting with the NLRP3 inflammasome, leading to gasdermin D cleavage (Zhang et al., 2021; Wang et al., 2019), resulting in inflammatory cell death and releasing pro-inflammatory cytokines such as IL-1β and IL-18. This contributes to cardiac injury post-myocardial infarction, amplifies inflammation, exacerbates tissue damage, and impairs cardiac repair mechanisms. The diverse effects of RBP4 on cardiac muscle highlight the complex interplay between metabolic dysfunction and cardiovascular health. The ability of RBP4 to induce both hypertrophy and cell death (through apoptosis and pyroptosis) underscores the delicate balance in cardiac physiology and the potential for RBP4 to tip this balance toward pathological outcomes. Moreover, the impact of RBP4 on cardiac energy metabolism suggests a potential link between systemic metabolic disorders and heart failure, a connection that warrants further investigation (Finck and Kelly, 2006; Lesnefsky et al., 2001; Wang et al., 2019).
Figure 2 comprehensively depicts the molecular mechanisms through which RBP4 induces cardiac dysfunction via its multifaceted effects on endothelial cells, cardiac fibroblasts, and cardiomyocytes.
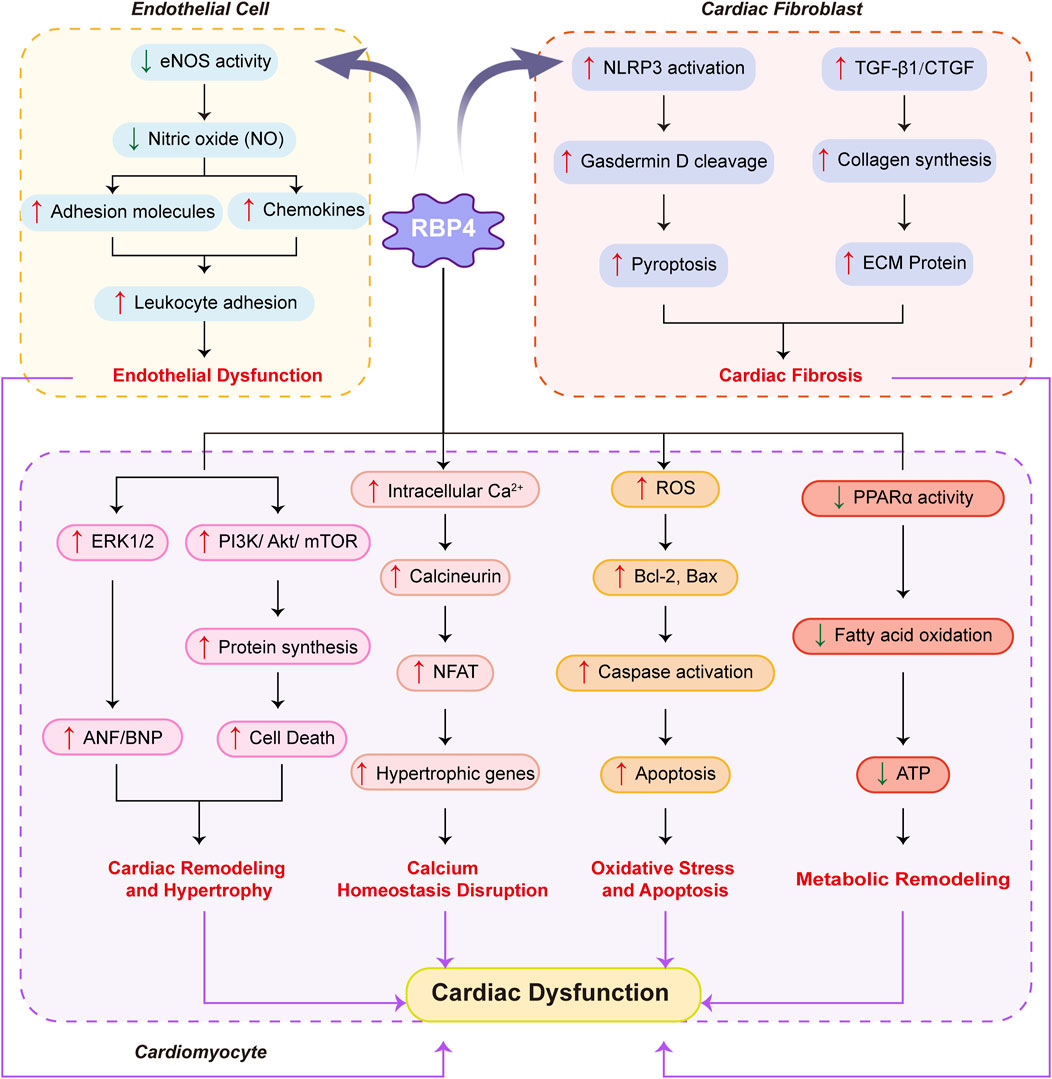
Figure 2. Molecular mechanisms by which RBP4 affects cardiac muscle.Schematic representation of how RBP4 mediates cardiac dysfunction through its effects on endothelial cells, cardiac fibroblasts, and cardiomyocytes. In endothelial cells, RBP4 decreases eNOS activity and NO production, leading to increased adhesion molecules and chemokines, resulting in enhanced leukocyte adhesion and endothelial dysfunction. In cardiac fibroblasts, RBP4 activates NLRP3 inflammasome and increases TGF-β1/CTGF expression, promoting gasdermin D cleavage, pyroptosis, collagen synthesis, and ECM protein production, ultimately causing cardiac fibrosis. In cardiomyocytes, RBP4 triggers four major pathological pathways: (1) Cardiac remodeling and hypertrophy through activation of ERK1/2 and PI3K/Akt/mTOR signaling, leading to increased ANF/BNP expression and protein synthesis; (2) Calcium homeostasis disruption via increased intracellular Ca2+ and calcineurin/NFAT signaling, inducing hypertrophic gene expression; (3) Oxidative stress and apoptosis through elevated ROS production, Bcl-2/Bax dysregulation, and caspase activation; and (4) Metabolic remodeling via decreased PPARα activity, resulting in reduced fatty acid oxidation and ATP production. These pathological changes collectively contribute to cardiac dysfunction. Blue arrows indicate decreased expression/activity; red arrows indicate increased expression/activity. Solid arrows represent direct effects; dashed lines indicate regulatory relationships.
4 Clinical implications
Elevated serum RBP4 levels are associated with insulin resistance and T2DM, correlating with impaired glucose tolerance and hyperinsulinemia (Takebayashi et al., 2007; Cho et al., 2006). Meta-analyses confirm the association between high RBP4 levels and increased T2DM risk (Tan et al., 2024; Zabetian-Targhi et al., 2015). In obesity and metabolic syndrome, RBP4 levels correlate with markers of metabolic dysregulation (Nono and Blüher, 2021; Dadej et al., 2022). Beyond statistical associations, multiple lines of evidence establish RBP4 as an independent prognostic risk factor for cardiovascular disease. Prospective cohort studies with multivariate adjustment for traditional risk factors demonstrate that elevated RBP4 levels independently predict future adverse cardiovascular events with hazard ratios ranging from 1.80 to 3.26 for intermediate and high RBP4-based scores, respectively (Qian et al., 2022; Chen et al., 2021). Ye et al. (2023) developed and validated an RBP4-based multimarker score that significantly improved risk prediction accuracy beyond established risk factors (net reclassification improvement of 0.24, 95% CI, 0.15–0.34; P < 0.001). Mechanistically, the direct pathophysiological effects of RBP4 on vascular inflammation, endothelial dysfunction, and cardiac remodeling provide strong biological plausibility for its causal role in cardiovascular pathogenesis, as evidenced by interventional studies showing that modulation of RBP4 levels directly affects cardiovascular outcomes (Ji et al., 2022; Schiborn et al., 2022; Li et al., 2020). High RBP4 levels predict coronary artery disease (Qian et al., 2022; Perumalsamy et al., 2021), correlate with atherosclerosis severity (Chen et al., 2021; Ye et al., 2023), and are associated with cardiac remodeling in heart failure (Li et al., 2020; Christou et al., 2023). RBP4 levels are increased in gestational diabetes mellitus (Wu et al., 2022; Leca et al., 2024), non-alcoholic fatty liver disease (NAFLD) (Huang and Xu, 2022; Hu et al., 2023), and may be linked to neurodegenerative diseases such as amyotrophic lateral sclerosis (Yosry et al., 2022). Additionally, RBP4 levels are associated with carotid intima-media thickness, suggesting a role in early atherosclerosis (Rychter et al., 2024), and serve as a potential biomarker for sarcopenia in older adults, correlating with changes in lean mass (Chang et al., 2023).
Pharmacological interventions targeting RBP4 include fenretinide, a synthetic retinoid reducing RBP4 synthesis and secretion, thereby improving insulin sensitivity (Napoli, 2012); thiazolidinediones decreasing RBP4 levels by activating PPARγ (Cho et al., 2006); and DPP-4 inhibitors that may reduce RBP4 levels and exert anti-inflammatory effects (Kim et al., 2015). The development of RBP4 antagonists, such as small molecules or antibodies inhibiting RBP4 function, shows promise in preclinical studies (Kim and Priefer, 2021; Oluwamodupe and Adeleye, 2023). Targeting the RBP4-STRA6 signaling pathway offers therapeutic potential in mitigating insulin resistance and β-cell dysfunction (Huang et al., 2021; Perumalsamy et al., 2021). Lifestyle modifications, including dietary interventions and exercise, reduce RBP4 levels and improve metabolic parameters (Dadej et al., 2022; Ghorbanian et al., 2023b). High-intensity interval training decreases RBP4 levels and improves insulin sensitivity in metabolic syndrome (Ghorbanian and Saberi, 2023a), and weight loss through bariatric surgery also reduces RBP4 levels and improves metabolic outcomes (Hany et al., 2022).
Measuring RBP4 levels aids in identifying individuals at risk for metabolic and cardiovascular diseases, with changes in RBP4 levels reflecting intervention effectiveness. Combining RBP4 with other biomarkers improves prognostic accuracy for adverse cardiovascular events (Flores-Cortez et al., 2022; Ye et al., 2023), and an RBP4-based multimarker score has been proposed as a prognostic tool for patients with acute coronary syndrome (Ye et al., 2023). RBP4 could be integrated into multimarker panels for risk stratification and monitoring disease progression. While the potential of RBP4 as a biomarker is promising, it is important to note the challenges in its clinical application. The lack of standardized measurement methods and established reference ranges across different populations presents a significant hurdle (Takebayashi et al., 2007; Cho et al., 2006). Additionally, the specificity of RBP4 as a marker for particular diseases or conditions remains a subject of debate, given its involvement in multiple physiological and pathological processes (Tan et al., 2024; Zabetian-Targhi et al., 2015). Future research should focus on developing and validating robust, standardized assays for RBP4 quantification and establishing population-specific reference values to enhance its clinical utility.
5 Conclusion
RBP4 significantly impacts skeletal and cardiac muscle physiology through diverse molecular mechanisms. Its involvement in insulin resistance, inflammation, lipid metabolism, mitochondrial dysfunction, and apoptosis underscores its importance in metabolic and cardiovascular diseases. RBP4 serves as a valuable biomarker and a promising therapeutic target. Integrating findings from extensive research enhances our understanding and highlights potential interventions. Future research focusing on unraveling its complex interactions and developing targeted therapies holds great promise for improving patient outcomes. As our understanding of RBP4 continues to evolve, this protein sits at the intersection of multiple physiological and pathological processes. The complexity of RBP4’s actions underscores the need for a systems biology approach to fully elucidate its roles and potential as a therapeutic target. While significant progress has been made, many questions remain unanswered, particularly regarding the long-term effects of RBP4 modulation and its interactions with other metabolic regulators. Future research will undoubtedly continue to unravel the intricate web of RBP4’s actions, potentially revolutionizing our approach to metabolic and cardiovascular diseases.
6 Future directions
Future research should focus on three key areas while maintaining comprehensive investigation. First, elucidating molecular mechanisms through identifying specific receptors beyond STRA6 (Huang et al., 2021; Zhang et al., 2024), and characterizing downstream signaling pathways. Advanced technologies like CRISPR-Cas9 will be valuable for understanding tissue-specific RBP4 functions and potential off-target effects of therapies (Rychter et al., 2024; Yang et al., 2021). Second, Developing RBP4-targeted therapeutics including small-molecule antagonists and antibodies (Kim and Priefer, 2021; Oluwamodupe and Adeleye, 2023), while exploring synergistic effects with existing treatments. The RBP4-STRA6 signaling pathway offers particular promise (Huang et al., 2021; Perumalsamy et al., 2021). Third, integrating RBP4 assessment into personalized medicine by identifying disease-associated RBP4 gene polymorphisms (Rychter et al., 2024; Yang et al., 2021). and incorporating RBP4 into risk prediction models for patient stratification. Additional exploration of RBP4’s role in neurodegenerative disorders (Yosry et al., 2022), immune regulation (Yang et al., 2021), and its interplay with other adipokines in epicardial adipose tissue (Christou et al., 2023) may reveal novel intervention targets, as could investigation of its effects on vascular smooth muscle cell phenotypic transformation (Zhou et al., 2023).
Author contributions
KZ: Conceptualization, Investigation, Methodology, Project administration, Resources, Software, Writing – original draft, Writing – review and editing. LW: Data curation, Formal Analysis, Investigation, Project administration, Resources, Writing – original draft. WG: Investigation, Software, Supervision, Visualization, Writing – review and editing. RG: Formal Analysis, Funding acquisition, Supervision, Validation, Writing – review and editing.
Funding
The author(s) declare that financial support was received for the research and/or publication of this article. This work was supported by a grant from the Foundation of China (No. 82300400 to KZ) and the foundation of Yangpu District Health Commission (No. YPM202415 to RG).
Conflict of interest
The authors declare that the research was conducted in the absence of any commercial or financial relationships that could be construed as a potential conflict of interest.
Generative AI statement
The author(s) declare that no Generative AI was used in the creation of this manuscript.
Publisher’s note
All claims expressed in this article are solely those of the authors and do not necessarily represent those of their affiliated organizations, or those of the publisher, the editors and the reviewers. Any product that may be evaluated in this article, or claim that may be made by its manufacturer, is not guaranteed or endorsed by the publisher.
References
Amengual, J., Ribot, J., Bonet, M. L., and Palou, A. (2008). Retinoic acid treatment increases lipid oxidation capacity in skeletal muscle of mice. Obes. (Silver Spring) 16 (3), 585–591. doi:10.1038/oby.2007.104
Anderson, E. J., Lustig, M. E., Boyle, K. E., Woodlief, T. L., Kane, D. A., Lin, C. T., et al. (2009). Mitochondrial H2O2 emission and cellular redox state link excess fat intake to insulin resistance in both rodents and humans. J. Clin. Invest. 119 (3), 573–581. doi:10.1172/JCI37048
Armeni, E., Chondrou, A., Nigdelis, M., Augoulea, A., Rizos, D., Kaparos, G., et al. (2021). Serum levels of retinol binding protein-4 are associated with the arterial stiffness in early postmenopausal women. Maturitas 152, 79. doi:10.1016/j.maturitas.2021.08.038
Bers, D. M. (2002). Cardiac excitation-contraction coupling. Nature 415 (6868), 198–205. doi:10.1038/415198a
Blaner, W. S. (1989). Retinol-binding protein: the serum transport protein for vitamin A. Endocr. Rev. 10 (3), 308–316. doi:10.1210/edrv-10-3-308
Bodine, S. C., Latres, E., Baumhueter, S., Lai, V. K., Nunez, L., Clarke, B. A., et al. (2001). Identification of ubiquitin ligases required for skeletal muscle atrophy. Science 294 (5547), 1704–1708. doi:10.1126/science.1065874
Bujak, M., and Frangogiannis, N. G. (2007a). The role of TGF-β signaling in myocardial infarction and cardiac remodeling. Cardiovasc Res. 74 (2), 184–195. doi:10.1016/j.cardiores.2006.10.002
Bujak, M., and Frangogiannis, N. G. (2007b). The role of TGF-β signaling in myocardial infarction and cardiac remodeling. Cardiovasc Res. 74 (2), 184–195. doi:10.1016/j.cardiores.2006.10.002
Chang, C. L., Li, Y. R., Wang, Z. Y., Li, M. L., Jia, K. Y., Sun, H. X., et al. (2023). Serum retinol binding protein 4 as a potential biomarker for sarcopenia in older adults. J. Gerontol. A Biol. Sci. Med. Sci. 78 (1), 34–41. doi:10.1093/gerona/glac151
Chen, H., Zhang, J., Lai, J., Zhou, Y., Lin, X., Deng, G., et al. (2021). Circulating retinol binding protein 4 levels in coronary artery disease: a systematic review and meta-analysis. Lipids Health Dis. 20 (1), 89. doi:10.1186/s12944-021-01516-7
Cho, Y. M., Youn, B. S., Lee, H., Lee, N., Min, S. S., Kwak, S. H., et al. (2006). Plasma retinol-binding protein-4 concentrations are elevated in human subjects with impaired glucose tolerance and type 2 diabetes. Diabetes Care 29 (11), 2457–2461. doi:10.2337/dc06-0360
Christou, G. A., Andriopoulou, C. E., Liakopoulou, A., Tsape, E., Apostolakis, E., Tselepis, A. D., et al. (2023). Unraveling the role of resistin, retinol-binding protein 4 and adiponectin produced by epicardial adipose tissue in cardiac structure and function: evidence of a paracrine effect. Horm. (Athens) 22 (2), 321–330. doi:10.1007/s42000-023-00447-5
Carrasco, A. G., Izquierdo-Lahuerta, A., Valverde, A. M., Ni, L., Flores-Salguero, E., Coward, R. J., et al. (2023). The protective role of peroxisome proliferator-activated receptor gamma in lipotoxic podocytes. Biochim. Biophys. Acta. Mol. Cell. Biol. Lipids. 868 (7), 159329. doi:10.1016/j.bbalip.2023.159329
Craig, R. L., Chu, W. S., and Elbein, S. C. (2007). Retinol binding protein 4 as a candidate gene for type 2 diabetes and prediabetic intermediate traits. Mol. Genet. Metab. 90 (3), 338–344. doi:10.1016/j.ymgme.2006.11.003
Dadej, D., Szczepanek-Parulska, E., and Ruchała, M. (2022). Interplay between fatty acid binding protein 4, fetuin-A, retinol binding protein 4, and thyroid function in metabolic dysregulation. Metabolites 12 (4), 300. doi:10.3390/metabo12040300
DeFronzo, R. A., and Tripathy, D. (2009). Skeletal muscle insulin resistance is the primary defect in type 2 diabetes. Diabetes Care 32 (Suppl. 2), S157–S163. doi:10.2337/dc09-S302
Eberlé, D., Hegarty, B., Bossard, P., Ferré, P., and Foufelle, F. (2004). SREBP transcription factors: master regulators of lipid homeostasis. Biochimie 86 (11), 839–848. doi:10.1016/j.biochi.2004.09.018
Elmore, S. (2007). Apoptosis: a review of programmed cell death. Toxicol. Pathol. 35 (4), 495–516. doi:10.1080/01926230701320337
Finck, B. N., and Kelly, D. P. (2006). PGC-1 coactivators: inducible regulators of energy metabolism in health and disease. J. Clin. Invest. 116 (3), 615–622. doi:10.1172/JCI27794
Flores-Cortez, Y. A., et al. (2022). Interplay of retinol binding protein 4 with obesity and associated chronic alterations (Review). Mol. Med. Rep. 26 (1), 244. doi:10.3892/mmr.2022.12760
Gao, W., Wang, H., Zhang, L., Cao, Y., Bao, J. Z., Liu, Z. X., et al. (2016). Retinol-Binding Protein 4 Induces Cardiomyocyte Hypertrophy by Activating TLR4/MyD88 Pathway. Endocrinology 157 (6), 2282–2293. doi:10.1210/en.2015-2022
Gorbanian, B., and Saberi, Y. (2021). Changes in Fetuin-B and RBP4 During A Course of High-Intensity Interval Training in Women with Nonalcoholic Fatty Liver. J. Babol. Univ. Med. Sci. 23 (1), 398–404. doi:10.22088/jbums.23.1.398
Ghorbanian, B., Wong, A., and Iranpour, A. (2023a). The effect of dietary carbohydrate restriction and aerobic exercise on retinol binding protein 4 (RBP4) and fatty acid binding protein 5 (FABP5) in middle-aged men with metabolic syndrome. Br. J. Nutr. 130 (4), 553–563. doi:10.1017/S0007114522003580
Ghorbanian, B., Wong, A., and Iranpour, A. (2023b). The effect of dietary carbohydrate restriction and aerobic exercise on retinol binding protein 4 (RBP4) and fatty acid binding protein 5 (FABP5) in middle-aged men with metabolic syndrome. Br. J. Nutr. 130 (4), 553–563. doi:10.1017/S0007114522003580
Gimbrone, M. A. Jr, and García-Cardeña, G. (2016). Endothelial cell dysfunction and the pathobiology of atherosclerosis. Circ. Res. 118 (4), 620–636. doi:10.1161/CIRCRESAHA.115.306301
Graham, T. E., Yang, Q., Blüher, M., Hammarstedt, A., Ciaraldi, T. P., Henry, R. R., et al. (2006). Retinol-binding protein 4 and insulin resistance in lean, obese, and diabetic subjects. N. Engl. J. Med. 354 (24), 2552–2563. doi:10.1056/NEJMoa054862
Hegyi, B., Bers, D. M., and Bossuyt, J. (2019). CaMKII signaling in heart diseases: Emerging role in diabetic cardiomyopathy. J. Mol. Cell. Cardiol. 127, 246–259. doi:10.1016/j.yjmcc.2019.01.001
Handschin, C., and Spiegelman, B. M. (2008). The role of exercise and PGC1alpha in inflammation and chronic disease. Nature 454 (7203), 463–469. doi:10.1038/nature07206
Hany, M., Demerdash, H. M., Agayby, A. S., Ibrahim, M., and Torensma, B. (2022). Can leptin/ghrelin ratio and retinol-binding protein 4 predict improved insulin resistance in patients with obesity undergoing sleeve gastrectomy? Obes. Surg. 32 (12), 3942–3950. doi:10.1007/s11695-022-06296-2
Hotamisligil, G. S. (2006). Inflammation and metabolic disorders. Nature 444 (7121), 860–867. doi:10.1038/nature05485
Houstis, N., Rosen, E. D., and Lander, E. S. (2006). Reactive oxygen species have a causal role in multiple forms of insulin resistance. Nature 440 (7086), 944–948. doi:10.1038/nature04634
Hu, R., Yang, X., He, X., and Song, G. (2023). The relationship between NAFLD and retinol-binding protein 4—an updated systematic review and meta-analysis. Lipids Health Dis. 22 (1), 8. doi:10.1186/s12944-022-01771-2
Huang, H., and Xu, C. (2022). Retinol-binding protein-4 and nonalcoholic fatty liver disease. Chin. Med. J. Engl. 135 (10), 1182–1189. doi:10.1097/CM9.0000000000002135
Huang, R., Bai, X., Li, X., Wang, X., and Zhao, L. (2021). Retinol-binding protein 4 activates STRA6, provoking pancreatic β-cell dysfunction in type 2 diabetes. Diabetes 70 (2), 449–463. doi:10.2337/db19-1241
Itani, S. I., Ruderman, N. B., Schmieder, F., and Boden, G. (2002). Lipid-induced insulin resistance in human muscle is associated with changes in diacylglycerol, protein kinase C, and IkappaB-alpha. Diabetes 51 (7), 2005–2011. doi:10.2337/diabetes.51.7.2005
Janke, J., Engeli, S., Boschmann, M., Adams, F., Böhnke, J., Luft, F. C., et al. (2006). Retinol-binding protein 4 in human obesity. Diabetes 55 (10), 2805–2810. doi:10.2337/db06-0616
Jheng, H. F., Tsai, P. J., Guo, S. M., Kuo, L. H., Chang, C. S., Su, I. J., et al. (2012). Mitochondrial fission contributes to mitochondrial dysfunction and insulin resistance in skeletal muscle. Mol. Cell Biol. 32 (2), 309–319. doi:10.1128/MCB.05603-11
Ji, Y., Song, J., Su, T., and Gu, X. (2022). Adipokine retinol binding protein 4 and cardiovascular diseases. Front. Physiol. 13, 856298. doi:10.3389/fphys.2022.856298
Kanai, M., Raz, A., and Goodman, D. S. (1968). Retinol-binding protein: the transport protein for vitamin A in human plasma. J. Clin. Invest. 47 (9), 2025–2044. doi:10.1172/JCI105889
Kim, S. C., Schneeweiss, S., Glynn, R. J., Doherty, M., Goldfine, A. B., Solomon, D. H., et al. (2015). Dipeptidyl peptidase-4 inhibitors in type 2 diabetes may reduce the risk of autoimmune diseases: a population-based cohort study. Ann. Rheum. Dis. 74 (11), 1968–1975. doi:10.1136/annrheumdis-2014-205216
Kim, J. A., Montagnani, M., Koh, K. K., and Quon, M. J. (2006). Reciprocal relationships between insulin resistance and endothelial dysfunction: molecular and pathophysiological mechanisms. Circulation 113 (15), 1888–1904. doi:10.1161/CIRCULATIONAHA.105.563213
Kim, N., and Priefer, R. (2021). Retinol binding protein 4 antagonists and protein synthesis inhibitors: potential for therapeutic development. Eur. J. Med. Chem. 226, 113856. doi:10.1016/j.ejmech.2021.113856
Klöting, N., Graham, T. E., Berndt, J., Kralisch, S., Kovacs, P., Wason, C. J., et al. (2007). Serum retinol-binding protein is more highly expressed in visceral than in subcutaneous adipose tissue and is a marker of intra-abdominal fat mass. Cell Metab. 6 (1), 79–87. doi:10.1016/j.cmet.2007.06.002
Kovacs, P., Geyer, M., Berndt, J., Kl\xF6ting, N., Graham, T. E., B\xF6ttcher, Y., et al. (2007). Effects of genetic variation in the human retinol binding protein-4 gene (RBP4) on insulin resistance and fat depot-specific mRNA expression. Diabetes 56 (12), 3095–100. doi:10.2337/db06-1647
Leca, B. M., Kite, C., Lagojda, L., Davasgaium, A., Dallaway, A., Chatha, K. K., et al. (2024). Retinol-binding protein 4 (RBP4) circulating levels and gestational diabetes mellitus: a systematic review and meta-analysis. Front. Public Health 12, 1348970. doi:10.3389/fpubh.2024.1348970
Li, X. Z., Zhang, K. Z., Yan, J. J., Wang, L., Wang, Y., Shen, X. Y., et al. (2020). Serum retinol-binding protein 4 as a predictor of cardiovascular events in elderly patients with chronic heart failure. ESC Heart Fail 7 (2), 542–550. doi:10.1002/ehf2.12591
Liu, C., Zhou, X. R., Ye, M. Y., Xu, X. Q., Zhang, Y. W., Liu, H., et al. (2021). RBP4 is associated with insulin resistance in Hyperuricemia-induced rats and patients with Hyperuricemia. Front. Endocrinol. (Lausanne). 12, 653819–8471. doi:10.3389/fendo.2021.653819
Li, Z., Lv, F., Wen, X., Guo, C., Li, L., Cai, X., et al. (2024). Dapagliflozin treatment and cardiovascular outcome in RBP4/TTRVal30Met (transthyretin cardiac amyloidosis) mice. ESC. Heart. Fail. 11 (1), 179–188. doi:10.1002/ehf2.14567
Lesnefsky, E. J., Moghaddas, S., Tandler, B., Kerner, J., and Hoppel, C. L. (2001). Mitochondrial dysfunction in cardiac disease: ischemia–reperfusion, aging, and heart failure. J. Mol. Cell Cardiol. 33 (6), 1065–1089. doi:10.1006/jmcc.2001.1378
Lin, J., Handschin, C., and Spiegelman, B. M. (2005). Metabolic control through the PGC-1 family of transcription coactivators. Cell Metab. 1 (6), 361–370. doi:10.1016/j.cmet.2005.05.004
Lindsey, M. L., Iyer, R. P., Jung, M., DeLeon-Pennell, K. Y., and Ma, Y. (2016). Matrix metalloproteinases as input and output signals for post-myocardial infarction remodeling. J. Mol. Cell Cardiol. 91, 134–140. doi:10.1016/j.yjmcc.2015.12.018
Madamanchi, N. R., and Runge, M. S. (2007). Mitochondrial dysfunction in atherosclerosis. Circ. Res. 100 (4), 460–473. doi:10.1161/01.RES.0000258450.44413.96
Mizushima, N., and Komatsu, M. (2011). Autophagy: renovation of cells and tissues. Cell 147 (4), 728–741. doi:10.1016/j.cell.2011.10.026
Molkentin, J. D. (2004). Calcineurin-NFAT signaling regulates the cardiac hypertrophic response in coordination with the MAPKs. Cardiovasc Res. 63 (3), 467–475. doi:10.1016/j.cardiores.2004.01.021
Moraes-Vieira, P. M., Yore, M. M., Dwyer, P. M., Syed, I., Aryal, P., and Kahn, B. B. (2014). RBP4 activates antigen-presenting cells, leading to adipose tissue inflammation and systemic insulin resistance. Cell Metab. 19 (3), 512–526. doi:10.1016/j.cmet.2014.01.018
Napoli, J. L. (2012). Physiological insights into all-trans-retinoic acid biosynthesis. Biochim. Biophys. Acta 1821 (1), 152–167. doi:10.1016/j.bbalip.2011.05.004
Norseen, J., Hosooka, T., Hammarstedt, A., Yore, M. M., Kant, S., Aryal, P., et al. (2012). Retinol-binding protein 4 inhibits insulin signaling in adipocytes by inducing proinflammatory cytokines in macrophages through a c-Jun N-terminal kinase- and toll-like receptor 4-dependent and retinol-independent mechanism. Mol. Cell. Biol. 32 (10), 2010–2019. doi:10.1128/MCB.06193-11
Nono, N. P. A., and Blüher, M. (2021). Retinol-binding protein 4 in obesity and metabolic dysfunctions. Mol. Cell Endocrinol. 531, 111312. doi:10.1016/j.mce.2021.111312
Olsen, T., and Blomhoff, R. (2020). Retinol, retinoic acid, and retinol-binding protein 4 are differentially associated with cardiovascular disease, type 2 diabetes, and obesity: an overview of human studies. Adv. Nutr. 11 (3), 644–666. doi:10.1093/advances/nmz131
Oluwamodupe, C., and Adeleye, A. O. (2023). Targeting retinol-binding protein 4 (RBP4) in the management of cardiometabolic diseases. Cardiovasc Toxicol. 23 (9-10), 285–294. doi:10.1007/s12012-023-09803-8
Patti, M. E., Butte, A. J., Crunkhorn, S., Cusi, K., Berria, R., Kashyap, S., et al. (2003). Coordinated reduction of genes of oxidative metabolism in humans with insulin resistance and diabetes: potential role of PGC1 and NRF1. Proc. Natl. Acad. Sci. U. S. A. 100 (14), 8466–8471. doi:10.1073/pnas.1032913100
Perumalsamy, S., Ahmad, W. A., and Huri, H. Z. (2021). Retinol-binding protein-4—a predictor of insulin resistance and the severity of coronary artery disease in type 2 diabetes patients with coronary artery disease. Biol. (Basel) 10 (9), 858. doi:10.3390/biology10090858
Peterson, P. A. (1971). Characteristics of a vitamin A-transporting protein complex occurring in human serum. J. Biol. Chem. 246 (1), 34–43. doi:10.1016/S0021-9258(18)62528-1
Qian, K., Yan, X., Xu, C., Fang, Y., and Ma, M. (2022). Association between circulating retinol-binding protein 4 and adverse cardiovascular events in stable coronary artery disease. Front. Cardiovasc Med. 9, 829347. doi:10.3389/fcvm.2022.829347
Rychter, A. M., Skrzypczak-Zielińska, M., Naskręt, D., Michalak, M., Zawada, A., Walczak, M., et al. (2024). Are variants of the RBP4 gene associated with serum retinol-binding protein 4 concentrations and carotid intima-media thickness values in women with obesity? Gene 893, 147909. doi:10.1016/j.gene.2023.147909
Sabio, G., and Davis, R. J. (2010). cJun NH2-terminal kinase 1 (JNK1): roles in metabolic regulation of insulin resistance. Trends Biochem. Sci. 35 (9), 490–496. doi:10.1016/j.tibs.2010.04.004
Sag, C. M., Wadsack, D. P., Khabbazzadeh, S., Abesser, M., Grefe, C., Neumann, K., et al. (2009). Calcium/calmodulin-dependent protein kinase II contributes to cardiac arrhythmogenesis in heart failure. Circ. Heart Fail 2 (6), 664–675. doi:10.1161/CIRCHEARTFAILURE.109.865279
Schiaffino, S., and Reggiani, C. (2011). Fiber types in mammalian skeletal muscles. Physiol. Rev. 91 (4), 1447–1531. doi:10.1152/physrev.00031.2010
Schiborn, C., Weber, D., Grune, T., Biemann, R., Jäger, S., Neu, N., et al. (2022). Retinol and retinol-binding protein 4 levels and cardiometabolic disease risk. Circ. Res. 131 (7), 637–649. doi:10.1161/CIRCRESAHA.122.321295
Suh, J. B., Kim, S. M., Cho, G. J., Choi, K. M., Han, J. H., Taek Geun, H., et al. (2010). Elevated serum retinol-binding protein 4 is associated with insulin resistance in older women. Metabolism 59 (1), 118–122. doi:10.1016/j.metabol.2009.06.025
Steinhoff, J. S., Wagner, C., Dähnhardt, H. E., Košić, K., Meng, Y., Taschler, U., et al. (2024). Adipocyte HSL is required for maintaining circulating vitamin A and RBP4 levels during fasting. EMBO Rep. 25 (7), 2878–2895. doi:10.1038/s44319-024-00158-x
Takac, I., Schröder, K., Zhang, L., Lardy, B., Anilkumar, N., Lambeth, J. D., et al. (2011). The E-loop is involved in hydrogen peroxide formation by the NADPH oxidase Nox4. J. Biol. Chem. 286 (15), 13304–13313. doi:10.1074/jbc.M110.192138
Takebayashi, K., Suetsugu, M., Wakabayashi, S., Aso, Y., and Inukai, T. (2007). Retinol binding protein-4 levels and clinical features of type 2 diabetes patients. J. Clin. Endocrinol. Metab. 92 (7), 2712–2719. doi:10.1210/jc.2006-1249
Tan, X., Zhang, H., Liu, L., Yu, Z., Liu, X., Cui, L., et al. (2024). The association between retinol-binding protein 4 and risk of type 2 diabetes: a systematic review and meta-analysis. Int. J. Environ. Health Res. 34 (2), 1053–1063. doi:10.1080/09603123.2023.2196396
Wang, J., Feng, Y., Huo, H., Zhang, X., Yue, J., Zhang, W., et al. (2019). NLRP3 inflammasome mediates angiotensin II-induced islet β-cell apoptosis. Acta Biochim. Biophys. Sin. (Shanghai) 51 (5), 501–508. doi:10.1093/abbs/gmz032
Whelan, R. S., Konstantinidis, K., Wei, A. C., Chen, Y., Reyna, D. E., Jha, S., et al. (2012). Bax regulates primary necrosis through mitochondrial dynamics. Proc. Natl. Acad. Sci. U. S. A. 109 (17), 6566–6571. doi:10.1073/pnas.1201608109
Wilkins, B. J., and Molkentin, J. D. (2004). Calcium–calcineurin signaling in the regulation of cardiac hypertrophy. Biochem. Biophys. Res. Commun. 322 (4), 1178–1191. doi:10.1016/j.bbrc.2004.07.121
Wu, P., Wang, Y., Ye, Y., Yang, X., Lu, Q., Liu, Y., et al. (2022). Serum retinol-binding protein 4 levels and risk of gestational diabetes mellitus: a nested case-control study in Chinese women and an updated meta-analysis. Diabetes Metab. Res. Rev. 38 (2), e3496. doi:10.1002/dmrr.3496
Yang, M., Weng, H., Pei, Q., Jing, F., and Yi, Q. (2021). The relationship between retinol-binding protein 4 and markers of inflammation and thrombogenesis in children with Kawasaki disease. Mediat. Inflamm. 2021, 7029514. doi:10.1155/2021/7029514
Yang, Q., Graham, T. E., Mody, N., Preitner, F., Peroni, O. D., Zabolotny, J. M., et al. (2005). Serum retinol binding protein 4 contributes to insulin resistance in obesity and type 2 diabetes. Nature 436 (7049), 356–362. doi:10.1038/nature03711
Ye, B., Zhao, Q., Fan, J., Li, X., Shan, C., Liu, F., et al. (2023). RBP4-based multimarker score: a prognostic tool for adverse cardiovascular events in acute coronary syndrome patients. J. Clin. Endocrinol. Metab. 108 (12), 3111–3121. doi:10.1210/clinem/dgad389
Yosry, A., et al. (2022). Serum level of retinol-binding protein 4 in relation to amyotrophic lateral sclerosis among Egyptian patients. Ain Shams Med. J. 73 (3), 531–541. doi:10.21608/asmj.2022.270224
Zabetian-Targhi, F., Mahmoudi, M. J., Rezaei, N., and Mahmoudi, M. (2015). Retinol binding protein 4 in relation to diet, inflammation, immunity, and cardiovascular diseases. Adv. Nutr. 6 (6), 748–762. doi:10.3945/an.115.008292
Zhang, K. Z., Li, J. W., Xu, J. S., Shen, Z. K., Lin, Y. S., Zhao, C., et al. (2024). RBP4 promotes denervation-induced muscle atrophy through STRA6-dependent pathway. J. Cachexia Sarcopenia Muscle 15 (4), 1601–1615. doi:10.1002/jcsm.13518
Zhang, K. Z., Shen, X. Y., Wang, M., Wang, L., Sun, H. X., Li, X. Z., et al. (2021). Retinol-binding protein 4 promotes cardiac injury after myocardial infarction via inducing cardiomyocyte pyroptosis through an interaction with NLRP3. J. Am. Heart Assoc. 10 (22), e022011. doi:10.1161/JAHA.121.022011
Zhao, Y., Rao, J., Qiu, T., Li, C., and Zhou, X. (2021). The effect of RBP4 on microRNA expression profiles in porcine granulosa cells. Anim. (Basel) 11 (5), 1391. doi:10.3390/ani11051391
Zhou, W., Ye, S. D., and Wang, W. (2021). Elevated retinol binding protein 4 levels are associated with atherosclerosis in diabetic rats via JAK2/STAT3 signaling pathway. World J. Diabetes 12 (4), 466–479. doi:10.4239/wjd.v12.i4.466
Zhou, W., Yuan, X., Li, J., Wang, W., and Ye, S. (2023). Retinol binding protein 4 promotes the phenotypic transformation of vascular smooth muscle cells under high glucose condition via modulating RhoA/ROCK1 pathway. Transl. Res. 259, 13–27. doi:10.1016/j.trsl.2023.03.004
Keywords: retinol-binding protein 4, skeletal muscle, cardiac muscle, insulin resistance, inflammation, cardiovascular disease, molecular mechanisms, adipokine
Citation: Zhang K, Wang L, Gao W and Guo R (2025) Retinol-binding protein 4 in skeletal and cardiac muscle: molecular mechanisms, clinical implications, and future perspectives. Front. Cell Dev. Biol. 13:1587165. doi: 10.3389/fcell.2025.1587165
Received: 04 March 2025; Accepted: 24 March 2025;
Published: 10 April 2025.
Edited by:
Georgios A. Christou, University of Ioannina, GreeceReviewed by:
Dimitris Kiortsis, University of Ioannina, GreeceCopyright © 2025 Zhang, Wang, Gao and Guo. This is an open-access article distributed under the terms of the Creative Commons Attribution License (CC BY). The use, distribution or reproduction in other forums is permitted, provided the original author(s) and the copyright owner(s) are credited and that the original publication in this journal is cited, in accordance with accepted academic practice. No use, distribution or reproduction is permitted which does not comply with these terms.
*Correspondence: Wei Gao, ZHJ3ZWlnMTk4NEBvdXRsb29rLmNvbQ==; Rong Guo, MDlfZG9jdG9yZ3VvQHRvbmdqaS5lZHUuY24=