- 1Tropical Aquaculture Research and Development Center, South China Sea Fisheries Research Institute, Chinese Academy of Fishery Sciences, Sanya, China
- 2Sanya Tropical Fisheries Research Institute, Sanya, China
- 3Key Laboratory of South China Sea Fishery Resources Exploitation and Utilization, Ministry of Agriculture and Rural Affairs, Guangzhou, China
In the study, the parasite from the yellowfin tuna (Thunnus albacares) was separated, and morphological observation and molecular identification were carried out. Our results showed that the parasite was similar to Pennella sp. Its cephalothorax was covered by spherical to spherical non-branched nipples of almost the same size, which were very similar in shape and arrangement. A pair of slightly larger, the unbranched antenna was present on the outer margin of the small papillae-covered area. The gene sequence of COX1 with a length of 1,558 bp in the mitochondria of the parasite was 100% similar to Pennella sp. (MZ934363). The mitochondrial genome had a total length of 14,620 bp. It consisted of 36 genes (12 protein-coding, 22 transfer RNAs and 2 ribosomal RNAs) and a dummy control region, but the mitochondrial genome had no ATP8 gene. Morphological observation showed that Pennella sp. was dark red, with a convex cephalothorax, with a total length of 8.42 cm, parasitic on the dorsal side of yellowfin tuna. Pennella sp. included the cephalothorax, neck, trunk, abdomen and egg belt. This study was the first report on the mitochondrial genome of Pennella sp. The results provide basic data for further identifying the parasites of Pennella genus.
Introduction
Pennella is a parasite belonging to Copepod that usually lives in large pelagic fishes (Hogans et al., 1985; Suyama et al., 2020). After metamorphosis, the female enters the host’s body surface to parasitize while the male swims freely (Hogans et al., 1985). Their bodies were straight and slender, with feathery bellies and straight egg sacs (Hogans, 2017). People usually judge the species of Pennella from the host, the size and number of antennal processes, the shape and arrangement of cephalothorax, etc. Due to the wide variety of species, there was no specific standard to determine the identity (Suyama et al., 2021). Suyama et al. conducted morphological and genetic analysis on 52 Pennella individuals from 12 final hosts, of which 29 were identified as large species, 20 as small and medium-sized species and three as small new species. The 17 of them were identified as P. balaenoptera, P. filosa, P. instructa, and P. benzi. Nine samples were identified as P. filosa. Three samples could not be determined as P. balaenoptera. The remaining 23 were unidentified species (Suyama et al., 2021). The systematic classification of these parasites is limited, and the complete genome of the parasite hasn’t been reported. Mitochondrial genome is a unique and easily accessible genetic marker of organisms (Brown et al., 1979). The complete mitochondrial genome can be used to distinguish parasites, phylogeny (Mueller et al., 2004; Lei et al., 2017), and population genetic structure, it is important to characterize the genome of the Copepod.
The hosts of Pennella are very diverse, including a variety of teleost fishes and even marine mammals (Suyama et al., 2021). The host yellowfin tuna (Thunnus albacares) in this study belongs to the mackerel family and tuna genus. Yellowfin tuna, a member of the scombroid family of tuna, has a distinct north-south migration habit, and its torpedo-like body shape enables it to move quickly to catch food. Yellowfin tuna were found in the tropical and subtropical waters of the Pacific, Indian and Atlantic oceans, and in the South China Sea and near Taiwan (Sund et al., 1981). It is a higher economic value nourishing treasure due to its high flesh quality and rich nutrition, deeply loved by people. Food safety is also the focus of people’s attention. We need to detect the species of parasites it infects to expand the understanding of fish vulnerable to parasites. Although tuna can be bred in captivity, the low survival of fish during artificial breeding continually hinders the tuna aquaculture industry. Up to the present, 80% of cultured fish were from wild juveniles, and wild fish can carry pathogens, viruses and parasites, which is not conducive to the smooth progress of subsequent breeding. Therefore, it is urgent to supplement the data on the parasites of yellowfin tuna.
In our study, we carried out morphological observation and molecular identification to determine its species. The results of this study provide a fundamental basis for identifying parasites in yellowfin tuna and other fish, expand people’s understanding of parasites, and lay a foundation for the occurrence and prevention of parasites. The complete mitochondrial genome sequence of Pennella sp. provides primary data for understanding the genomic diversity and evolution of fish copepods as well as for studying new genetic markers for population genetics and species identification.
Materials and Methods
Source of Materials
In our study, the parasite-attached yellowfin tuna was caught near Xincun Harbour, Hainan, China (E108°80’17’’, N18°50’15’’). Figure 1 showed the offshore area where this yellowfin tuna was caught. It was artificially fished and transported to the Tropical Aquaculture Research and Development Center, South China Sea Fisheries Research Institute, Chinese Academy of Fishery Sciences. The parasite-infected yellowfin tuna was found to be on the verge of death. The parasite was found in the muscles near the dorsal fin of the dying yellowfin tuna. We carefully collected it on the surface of the fish with tweezers and carefully observed it in a clean petri dish.
Morphological Observation and Whole Mitochondrial Genome Sequencing of Pennella sp.
We first measured the length of the parasite from the front end of the head to the back end of the abdomen and observed whether the abdomen had egg belts and raised tentacles on the cephalothorax. Then, we placed it under the anatomical microscope (Olympus SZ40, Japan), observed its morphological characteristics, pressed the cephalothorax, trunk and abdomen, observed and photographed. After observation, the whole parasite was quickly frozen with liquid nitrogen and stored at -80°C for standby. In order to further understand the genetic status and evolution of mitochondria, we studied and obtained the complete mitochondrial genome of Pennella sp. Genomic DNA of the parasite was extracted from muscle using Marine Animal Tissue Genomic DNA Extraction Kit (Tiangen Biochemical Technology Co., Ltd, China). DNA was processed by Shanghai Lingen Biotechnology Co., Ltd. and it was paired-end sequenced by Illumina NovaSeq 6000 sequencing technology, using SPAdes v3. 14.1 the software splices clean data. For most species, ring maps are usually used to display the basic research results of the genome in the first genome research. According to the assembled genome sequence of the sequenced sample, combined with the prediction results of the coding gene, the sample genome is displayed in a circle (Figure 3). The software used is CGView. Finally, the results were compared with the sequences reported in NCBI GenBank database(http://blast.ncbi.nlm.nih.gov/Blast.cgi), and the phylogenetic tree was constructed using Mega 5.2 software (Hall, 2005).
Results
Parasite Attachment Site
According to the observation of dead yellowfin tuna, it was found that the parasite is mainly attached to 1/2 of the base to the end of the dorsal fin of yellowfin tuna, on the back half of yellowfin tuna (Figures 2A, B). When the parasite is attached to the fish body, it entered the fish body from the chest to the body, while other parts were exposed to the water and could swing with the water flow.
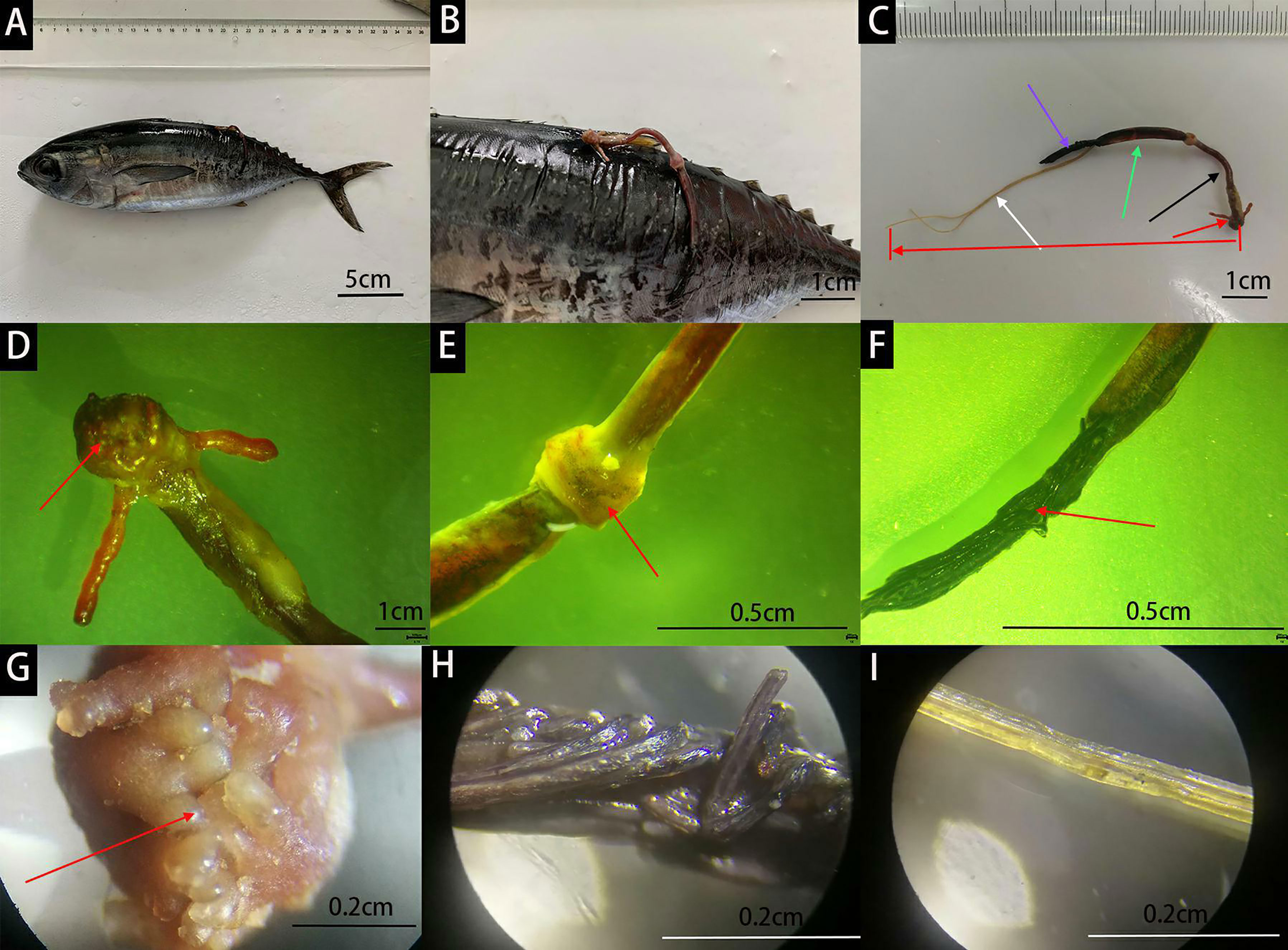
Figure 2 Infected yellowfin tuna (A). Parasites on the posterior dorsal fin (B). The red arrow represents the cephalothorax, the black arrow represents the neck, the green arrow represents the trunk, the purple arrow represents the abdomen, and the white arrow represents the egg belts (C). The head and tail of the red arrow at the bottom of the figure represent the starting and ending positions of the parasite (C). Mastoid process on the cephalothorax (D, G). The node between the neck and trunk (E) Abdomen (F, H). Yellow egg belts (I).
Parasite Appearance Description
The parasite in this study can be divided into five parts: cephalothorax, neck, trunk, abdomen and egg belt (Figure 2C). The cephalothorax was covered by several equally distributed mastoids of similar size. The heads of the mastoid were light yellow, and the roots were red (Figure 2G). There was a pair of large antennae without branches below (Figure 2D). The larger antennae were located at the lateral edge of the cephalothorax. Below the cephalothorax was the neck and trunk, between which there was a node (Figure 2E), below the trunk was the abdomen (Figure 2F), which was composed of several black strips (Figure 2H). Below the abdomen were two yellow egg belts (Figure 2I). The body length of the parasite in this study was 5.25 cm, egg band 4.49 cm, cephalothorax 0.39 cm, neck 1.79 cm, body 1.88 cm, and abdomen 1.21 cm.
Complete Mitogenome and Molecular Identification
The of the parasite was 14,620 bp in length. According to the genome circle map (Figure 3), the structure of the genome was including two rRNA genes, 12 protein-coding genes (lack of ATP8), 22 tRNA genes, two rRNA genes, a light-strand replication origin (OL), and a putative control region (CR). The overall base composition was 31.9% of A, 31.9% of T, 18% of C, and 18% of G with a slight C+G bias (36.0%) like other vertebrate mitochondrial genomes.
For the 12 protein-coding genes, 5 genes began with TTG and 5 began with ATT, while only COB and NAD3 began with ATG and ATA, respectively. Eight genes shared a stop codon TAA (NAD4L, COB, COX2, NAD1, NAD2, NAD3, NAD4 and NAD5), three have TAG (NAD6, ATP6, COX3), and COX1 has an incomplete stop codon. It had two non-coding regions, the L-strand replication origin region (350 bp) located between COX1 and COB, and the control region (449 bp) located within the COB and ATP6.
After gene sequencing, we compared the COX1 gene (1,558 bp) with NCBI database,
and found that the parasite was closely related to Pennella sp., with a similarity of 100%. We reconstructed the phylogenetic relationship between the species and other species. The phylogenetic tree was constructed by the adjacency method (Figure 4). The species of parasite samples could not be determined.
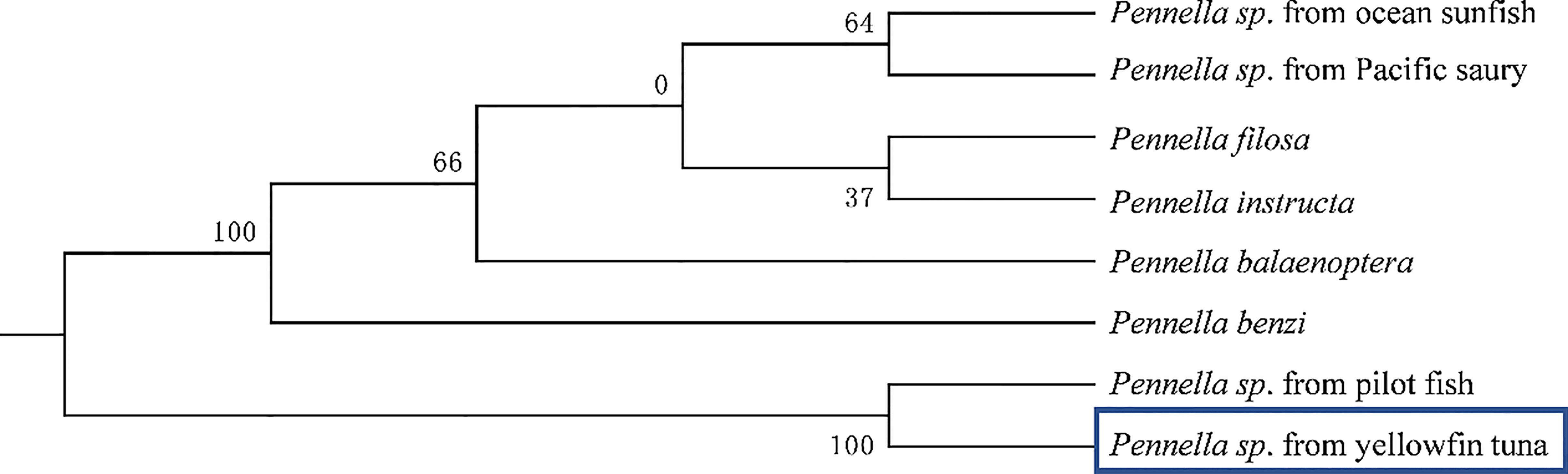
Figure 4 The phylogenetic relationship was estimated using the Maximum Likelihood method for the COX1 genes. Genbank accession numbers: Pennella sp. from pilot fish (MZ934363), Pennella benzi (LC642589), Pennella sp. from Pacific saury (LC638600), Pennella sp. from ocean sunfish (LC638579), Pennella filosa (LC642600), Pennella instructa (LC642628), Pennella balaenoptera (MG701292).
Discussion
Current studies have shown that copepods of the genus Pennella were parasites of marine aquatic organisms (e.g., cephalopods, pelagic fish, whales). Most Pennella were parasitic on marine fish (Suyama et al., 2021). They can infect fish of economic interest, including tuna and swordfish. They penetrate the muscles of the host, seriously damage the internal organs, and grow by absorbing the nutrients in the fish, resulting in fish stress response, loss of appetite, changes in swimming speed, and threatening important functions such as heart, intestine and stomach (Mugetti et al., 2021). We can see from the previous summary that Pennella sp. from North Pacific armorhead lacks oocysts due to incomplete development; Pennella sp. from Pacific sury and Pennella sp. from Japanese amberjack contain two or three pairs of large antennal processes with branches on the outer edge of the cephalothorax (Suyama et al., 2021). However, the parasite has only a pair of non-branching antennal processes and egg belts, which were not consistent with the above characteristics. It is concluded that this parasite was different from the species previously studied. By comparing this parasite with other species, it was found that the parasites in this study have similarities with Pennella sp., a parasite parasitic on pilot fish (Suyama et al., 2021). The COX1 gene of the parasite in this study was compared with the genome of Pennella sp., and the similarity of gene COX1 sequence with Pennella sp. found by predecessors was 100%, but the species was unknown. At present, there are few reports on the large parasites on the body surface of yellowfin tuna.
After gene annotation, it was found that Pennella sp. in this study had no ATP8 gene. In copepods, the complete mitochondrial genome of Eurytemora affinis, Tigriopus kingsejongenesis and Calanus sinicus contains the gene ATP8. The whole mitochondrial genome length of the above copepods was 14,900-16,700 bp, which is longer than that of the parasite (Wang et al., 2011; Choi et al., 2019; Hwang et al., 2019). Studies have reported that this gene is not found in Diphyllobothrium latum (Park et al., 2007), parasitic flatworm (Tang et al., 2017; Zhang et al., 2017), Benedenia humboldti n. sp. (Baeza et al., 2019), Cestode (Kim et al., 2007). The whole mitochondrial genome lengths of the above flatworms, tapeworms and nematodes were 13,400-14,660 bp. It can be concluded that the length of species with ATP8 gene in mitochondrial genome was longer than that without ATP8 gene. The lack of ATP8 gene was a unified feature of flatworms. It may be that the evolution process needs to adapt to the environment, or the gene ATP8 degenerates in some species (Barat et al., 2012). The specific reasons need to be further explored and studied. Whether it is also a characteristic of Pennella is still unknown and needs to be further studied.
Conclusions
Through morphological observation, Pennella sp. in this study was composed of cephalothorax, neck, trunk, abdomen and egg belt. The mitochondrial genome had a total length of 14,620 bp, including 2 rRNA genes, 22 tRNA genes and 12 protein coding genes (lack of ATP8 gene), a light-strand replication origin (OL), and a putative control region (CR). The COX1 gene with a length of 1558bp in the whole genome had a high similarity with Pennella sp. (MZ934363), which was 100%. This study provides basic data for the further development of yellowfin tuna and fills the academic gap.
Data Availability Statement
The data presented in the study are deposited in the GenBank, accession number ON161759.
Author Contributions
GY and RY: conceptualization. HL and ZF: experimental operation. HL and JH: field sampling. HL and ZF: sample determination. HL: writing – original draft preparation. ZM and SZ: writing – review and editing. All authors read and approved the final manuscript.
Funding
This work was supported by Hainan Major Science and Technology Project (ZDKJ2021011); Central Public-interest Scientific Institution Basal Research Fund, CAFS (2020TD55) and Central Public-Interest Scientific Institution Basal Research Fund South China Sea Fisheries Research Institute, CAFS (2021SD09).
Conflict of Interest
The authors declare that the research was conducted in the absence of any commercial or financial relationships that could be construed as a potential conflict of interest.
Publisher’s Note
All claims expressed in this article are solely those of the authors and do not necessarily represent those of their affiliated organizations, or those of the publisher, the editors and the reviewers. Any product that may be evaluated in this article, or claim that may be made by its manufacturer, is not guaranteed or endorsed by the publisher.
References
Baeza, J. A., Sepulveda, F. A., Gonzalez, M. T. (2019). The Complete Mitochondrial Genome and Description of a New Cryptic Species of Benedenia Diesing 1858 (Monogenea: Capsalidae), a Major Pathogen Infecting the Yellowtail Kingfish Seriola Lalandi Valenciennes in the South-East Pacific. Parasites&Vectors 12 (1), 409. doi: 10.1186/s13071-019-3711-5
Barat, A., Ali, S., Sati, J., Sivaraman, G. K. (2012). Phylogenetic Analysis of Fishes of the Subfamily Schizothoracinae (Teleostei: Cyprinidae) From Indian Himalayas Using Cytochrome B Gene. Indian J. Fisheries. 59 (1), 43–47. doi: 10.1071/MF12099
Brown, W. M., George, M., Jr., Wilson, A. C. (1979). Rapid Evolution of Animal Mitochondrial DNA. Proc. Natl. Acad. Sci. U.S.A. 76 (4), 1967–1971. doi: 10.1073/pnas.76.4.1967
Choi, B. S., Han, J., Hwang, D. S., Souissi, S., Hagiwara, A., Lee, J. S. (2019). Complete Mitochondrial Genome of the Calanoid Copepod Eurytemora Affinis (Calanoida, Temoridae). Mitochondrial. DNA Part B-Resources. 4 (2), 2731–2733. doi: 10.1080/23802359.2019.1644558
Hall, B. G. (2005). Comparison of the Accuracies of Several Phylogenetic Methods Using Protein and DNA Sequences. Mol. Biol. Evol. 22 (4), 792–802. doi: 10.1093/molbev/msi066
Hogans, W. E. (2017). Review of Pennella Oken 1816 (Copepoda: Pennellidae) With a Description of Pennella Benzi Sp. Nov., a Parasite of Escolar, Lepidocybium Flavobrunneum (Pisces) in the Northwest Atlantic Ocean. Zootaxa 4244 (1), 1–38. doi: 10.11646/zootaxa.4244.1.1
Hogans, W. E., Brattey, J., Hurlbut, T. R. (1985). Pennella Filosa and Pennella Instructa (Copepoda: Pennellidae) on Swordfish (Xiphias Gladius L.) From the Northwest Atlantic Ocean. J. Parasitol. 71 (1), 111–112. doi: 10.2307/3281987
Hwang, D. S., Choi, B. S., Lee, M. C., Han, J., Kim, S., Lee, J. S. (2019). Complete Mitochondrial Genome of the Antarctic Copepod Tigriopus Kingsejongenesis (Harpacticoida, Harpacticidae). Mitochondrial. DNA Part B-Resources. 4 (1), 1470–1471. doi: 10.1080/23802359.2019.1601042
Kim, K. H., Jeon, H. K., Park, J. K. (2007). Characterization of the Complete Mitochondrial Genome of Diphyllobothrium Nihonkaiense (Diphyllobothriidae: Cestode), and Development of Molecular Markers for Differentiating Fish Tapeworms. Mol. Cells 23 (3), 379–390. doi: 10.1016/j.cbi.2007.03.007
Lei, R. H., Frasier, C. L., Hawkins, M. T. R., Engberg, S. E., Bailey, C. A., Johnson, S. E., et al. (2017). Phylogenomic Reconstruction of Sportive Lemurs (Genus Lepilemur) Recovered From Mitogenomes With Inferences for Madagascar Biogeography. J. Heredity. 108 (2), 107–119. doi: 10.1093/jhered/esw072
Mueller, R. L., Macey, J. R., Jaekel, M., Wake, D. B., Boore, J. L. (2004). Morphological Homoplasy, Life History Evolution, and Historical Biogeography of Plethodontid Salamanders Inferred From Complete Mitochondrial Genomes. Proc. Natl. Acad. Sci. U.S. 101 (38), 13820–13825. doi: 10.1073/pnas.0405785101
Mugetti, D., Colombino, E., Menconi, V., Garibaldi, F., Mignone, W., Gustinelli, A., et al. (2021). Unusual Localization of Pennella Sp. In Swordfish (Xiphias Gladius) Hearts. Animals 11 (6), 1757. doi: 10.3390/ani11061757
Park, J. K., Kim, K. H., Kang, S., Jeon, H. K., Kim, J. H., Littlewood, D. T. J., et al. (2007). Characterization of the Mitochondrial Genome of Diphyllobothrium Latum (Cestoda: Pseudophyllidea) - Implications for the Phylogeny of Eucestodes. Parasitology 134 (5), 761–762. doi: 10.1017/S0031182007002740
Sund, P. N., Blackburn, M., Williams, F. (1981). Tunas and Their Environment in the Pacific Ocean: A Review. Oceanogr. Mar. Biol. 19, 443–512.
Suyama, S., Yanagimoto, T., Kakehi, S., Chow, S. (2020). Infection of the Pacific Saury Cololabis Saira (Brevoort 1856) (Teleostei: Beloniformes: Scomberesocidae) by Pennella Sp. (Copepoda: Siphonostomatoida: Pennellidae) South of the Subarctic Front. J. Crustacean. Biol. 40 (4), 384–389. doi: 10.1093/jcbiol/ruaa022
Suyama, S. ,. Y., Yanagimoto, T., Nakai, K., Tamura, T., Shiozaki, K., Ohshimo, S., et al. (2021). A Taxonomic Revision of Pennella Oken 1815 Based on Morphology and Genetics (Copepoda: Siphonostomatoida: Pennellidae). J. Crustacean. Biol. 41 (3), 1–12. doi: 10.1093/jcbiol/ruab040
Tang, J. F., Cai, J., Hang, Y., Lin, Z. W., Lu, Y. S., Jian, J. C. (2017). The Complete Mitochondrial Genome of a Parasitic Flatworm Senga Ophiocephalina (Cestoda: Bothriocephalidae). Mitochondrial. DNA Part B-resources. 1 (1), 917–918. doi: 10.1080/23802359.2016.1219643
Wang, M. X., Sun, S., Li, C. L., Shen, X. (2011). Distinctive Mitochondrial Genome of Calanoid Copepod Calanus Sinicus With Multiple Large non-Coding Regions and Reshuffled Gene Order: Useful Molecular Markers for Phylogenetic and Population Studies. BMC Genomics 12 (73), 73. doi: 10.1186/1471-2164-12-73
Zhang, D., Zou, H., Wu, S. G., Li, M., Jakovlic, I., Zhang, J., et al. (2017). Sequencing of the Complete Mitochondrial Genome of a Fish-Parasitic Flatworm Paratetraonchoides Inermis (Platyhelminthes: Monogenea): tRNA Gene Arrangement Reshuffling and Implications for Phylogeny. Parasites&Vectors 10, 462. doi: 10.1186/s13071-017-2404-1
Keywords: mitochondrial genome, Thunnus albacares, parasite, Pennella, copepod.
Citation: Liu H, Fu Z, Zhou S, Hu J, Yang R, Yu G and Ma Z (2022) The Complete Mitochondrial Genome of Pennella sp. Parasitizing Thunnus albacares. Front. Cell. Infect. Microbiol. 12:945152. doi: 10.3389/fcimb.2022.945152
Received: 16 May 2022; Accepted: 06 June 2022;
Published: 30 June 2022.
Edited by:
Yibin Yang, Independent Researcher, Yangtze River Fisheries Research Institute, Chinese Academy of Fishery Sciences, Wuhan, ChinaCopyright © 2022 Liu, Fu, Zhou, Hu, Yang, Yu and Ma. This is an open-access article distributed under the terms of the Creative Commons Attribution License (CC BY). The use, distribution or reproduction in other forums is permitted, provided the original author(s) and the copyright owner(s) are credited and that the original publication in this journal is cited, in accordance with accepted academic practice. No use, distribution or reproduction is permitted which does not comply with these terms.
*Correspondence: Zhenhua Ma, emhlbmh1YS5tYUBob3RtYWlsLmNvbQ==
†These authors have contributed equally to this work