- 1Department of Gastroenterology, West China Hospital, Sichuan University, Chengdu, Sichuan, China
- 2Department of Urology, Beijing Chaoyang Hospital, Capital Medical University, Beijing, China
- 3Technology Research Institute of Shuxi Condiments of Sichuan Cuisine Co. LTD, Chengdu, Sichuan, China
The gut microbiome has been considered to play an important role in inflammatory bowel disease (IBD). Our previous study reported that tacrolimus-altered gut microbiota elicited immunoregulatory effects in both colonic mucosa and circulation, contributing to an increased allograft survival rate in mice. Here, we aimed to observe the changes in the tacrolimus-induced microbiome in a dextran sulfate sodium (DSS)-induced colitis mouse model and explore the possibility and efficacy of combination therapy with tacrolimus and the microbiome on colitis. Mice were divided into the control, DSS, tacrolimus monotherapy and tacrolimus plus Lactobacillus plantarum 550 (Lacto)-treated groups. The body weight, stool consistency, hematochezia and survival of mice were observed daily. Total RNA from colonic mucosa was extracted and subjected to transcriptome sequencing. Cecal contents were collected and the 16S rRNA sequencing was performed to characterize the gut microbiome and the ultrahigh- performance liquid chromatography-MS/MS (UHPLC-MS/MS) was used for targeted quantification of bile acids. The results confirmed that tacrolimus significantly ameliorated DSS-induced colitis in mice. Beneficial alterations of the gut microbiome characterized by a remarkable expansion of the genus Lactobacillus were induced by tacrolimus treatment. Oral supplementation with Lacto further improved the tacrolimus-mediated suppression of body weight loss in colitis, while the survival time of mice was further prolonged and the inflammation of colonic mucosa was obviously relieved. The immune and inflammation-related signaling pathways, including IFN-γ and IFN-α response, allograft rejection, IL2 STAT5 signaling and the inflammatory response pathways, were further downregulated in the tacrolimus plus Lacto cotreatment group. Cotreatment also improved the diversity of the gut microbiome and rescued the concentration of taurochenodeoxycholic acid (TCDCA) in colitis. The latter was positively correlated with the abundance of Lactobacillus but negatively related to the disease activity index score. Overall, our results indicated that Lactobacillus plantarum promoted the therapeutic effect of tacrolimus in experimental colitis, offering a promising strategy to combine tacrolimus and Lactobacillus in the treatment of colitis patients.
Highlights
1. Tacrolimus induced beneficial alterations in the gut microbiome in DSS-induced colitis, characterized by a remarkable expansion of the genus_Lactobacillus.
2. Compared with tacrolimus monotherapy, oral supplementation with Lactobacillus plantarum further ameliorated colitis and inhibited the proinflammatory signaling pathways in mice.
3. The combination of tacrolimus and Lactobacillus plantarum improved the diversity and composition of the gut microbiome as well as bile acid metabolism in colitis mice.
1 Introduction
Inflammatory bowel disease (IBD), encompassing Crohn’s disease (CD) and ulcerative colitis (UC), is a group of chronic and relapsing inflammatory disorders that causes intestinal lesions and even extraintestinal manifestations (Wright et al., 2018). In the past decade, the incidence of IBD has significantly increased in newly industrialized countries or urbanized countries in Asia (Ng et al., 2017; Ng et al., 2019). This leads IBD to be a public health challenge worldwide. Considerable evidence suggests that the pathogenesis of IBD is characterized by an inappropriate and persistent mucosal immune response, caused by genetic and environmental factors (Caruso et al., 2020). The gut microbiome has been considered as a pivotal cause of the occurrence and development of IBD (Honda and Littman, 2012; Caruso et al., 2020). Dysbiosis in IBD manifests as a decrease in commensal bacterial diversity and alterations in the gut microbiota composition (Honda and Littman, 2012; Caruso et al., 2020). The finding that the genetically susceptible mice fail to develop colitis under germ-free conditions, and the effectiveness of antibiotics and probiotics in IBD therapy further support the important role of the gut microbiome. (Podolsky, 2002; Caruso et al., 2020; Lee and Chang, 2021).
Tacrolimus (Tacro), a commonly used immunosuppressant in the transplantation area, is also recommended in the treatment of refractory IBD patients with impressive efficacy (Baumgart et al., 2006; Rodríguez-Lago et al., 2020). With the advantages of high oral bioavailability and the potent inhibitory effects on activated T-cells(Fellermann et al., 2002; Komaki et al., 2016), FK has been selectively utilized to induce remission in corticosteroid-refractory UC and refractory fistulizing perianal CD in recent years (Rodríguez-Lago et al., 2020; Gordon et al., 2022). However, due to the relatively narrow therapeutic window and dose-dependent side effects such as neurotoxicity, nephrotoxicity, metabolic disorders and opportunistic infection (Baumgart et al., 2006), it is recommended that tacrolimus be used as a bridging strategy or for short-term induction of remission in IBD (Rodríguez-Lago et al., 2020). Further studies are needed to shed light on the strategy that reduces the side effects and enhances the efficacy of tacrolimus in IBD.
Conventionally, tacrolimus exerts its immunosuppressive functions by binding to the FK506-binding protein to inhibit calcineurin phosphatase and block the transcription and secretion of cytokines, particularly interleukin (IL)-2, which leads to the suppression of T lymphocyte activation and proliferation (Thomson et al., 1995; Chow and Leong, 2007). In recent years, studies by our group and other groups have reported that tacrolimus alters the composition and bacterial taxa of the gut microbiota (Jiang et al., 2018; Zhang et al., 2018). We have demonstrated a significant expansion of Allobaculum, Bacteroides and Lactobacillus in tacrolimus-treated mice (Zhang et al., 2018). Innovatively, our study reported that the tacrolimus-induced microbiota elicited immunoregulatory effects in both colonic mucosa and circulation, causing a significantly increased proportion of CD4+CD25hiFoxP3+ regulatory T cells in peripheral blood mononuclear cells, mesenteric lymph nodes and colonic mucosa (Zhang et al., 2018). This suppressive function of tacrolimus–induced gut microbiota was further confirmed in a skin transplant mouse model. Furthermore, our study confirmed that tacrolimus-induced disorders of glucose metabolism were partially associated with the gut microbiota, and oral supplementation with butyrate might prevent and reverse tacrolimus-induced hyperglycemia in mice (Jiao et al., 2020). These data raise the possibility of combining immunosuppressive agents and the gut microbiome for the treatment of immune diseases. However, to our knowledge, the composition and function of the tacrolimus-induced microbiome in the colitis model and the underlying mechanism remain unclear.
In this study, we evaluated the role of tacrolimus and its impact on the gut microbiota, immune response and bile acid profiles in dextran sulfate sodium (DSS)-induced colitis mouse model. Furthermore, the combination of tacrolimus and the gut microbiome might be considered as a prospective strategy for the treatment of colitis patients in the clinic.
2 Materials and methods
2.1 Animal studies
The animal experiments were conducted in two different centers (West China Hospital and Chaoyang Hospital) under the same conditions to confirm the reliability of the results. Six-week-old male C57BL/6 mice were obtained from Beijing Vital River Laboratory Animal Technology Corporation Ltd. and the Experimental Animal Center of Sichuan University. All animals were housed in specific pathogen-free (SPF) conditions under constant temperature and humidity with a 12 h light-dark cycle. Standard diet and sterile water were supplied ad libitum during the initial 7-day adaptation period. All animal procedures were approved by the Animal Ethics Committees of Beijing Chao-Yang Hospital and the Animal Ethics Committee of West China Hospital of Sichuan University (Ref. No. 20211277A).
2.2 Dextran sulfate sodium-induced colitis model and treatment
Mice were randomly divided into different groups of 6-10 animals each before the start of the experiment. Colitis was induced by adding 2.5% (w/v) dextran sulfate sodium (DSS, M.W: 36000-50000, MP Biomedicals, Canada) to the drinking water for 7 days, then replaced with sterile water. Tacrolimus (Tacro, Astellas Ireland Corporation Ltd, Shenyang, China) at different dosages (0.1 mg/kg, 1 mg/kg or 10 mg/kg) was administered via oral gavage in sterile water once a day and maintained until the end of experiments. Lactobacillus plantarum 550 (Lacto) in powder form was isolated from pickle and provided as a gift from Technology Research Institute of Shuxi Condiments of Sichuan Cuisine Corporation Ltd (Chengdu, China), 1×108 CFU in 200 μL sterile water/mouse/day was given daily via oral gavage 3 days before DSS administration and maintained until the end of experiments. Mice in the control group were administered an equal volume of sterile water by gavage. Body weight and water consumption were recorded daily. The blood in the stool was detected by Pyramidon semiquantitative assay using a Fecal Occult Blood test kit (BA2020B, BaSO, Zhuhai, China) and recorded as “-”, “+”, “++”, “+++”, or “++++”. The disease activity index (DAI) was evaluated by scoring the body weight loss (%), stool consistency and blood in feces as described in the literature(Jang et al., 2019), with minor modifications (Supplementary Table 1). Mice were sacrificed at the end of the treatment, and the colon lengths were measured. Cecal contents were collected, snap frozen and kept at -80 °C for 16S rRNA sequencing and targeted bile acid metabolomics assays. Colonic mucosa was isolated and frozen until transcriptomics analysis. Sections of the proximal and distal colon were fixed in 4% paraformaldehyde and stained with hematoxylin and eosin (H&E). Histological scores were calculated according to the criteria described in the literature (a score from 0 to 4 was given for each colon segment [proximal or distal] based on the severity of inflammation, and a combined score [proximal and distal] provided a total colonic histological score per mouse) (Liu et al., 2017).
2.3 16S rRNA amplicon sequencing
The total genomic DNA was extracted from cecal contents of mice using the TGuide S96 Magnetic Soil and Stool DNA Kit (TIANGEN Biotech Corporation Ltd, Beijing, China) according to the manufacturer’s instructions. The full-length 16S rRNA gene was amplified with universal primers (27F: 5’-AGRGTTTGATYNTGGCTCAG-3’; 1492R:5’-TASGGHTACCTTGTTASGACTT-3’) under the following conditions: 95 °C for 2 min; 25 cycles of 98 °C for 10 s, 55 °C for 30 s, and 72 °C for 90 s; and a final step at 72°C for 2 min. The purified PCR products were sequenced on the PacBio platform by Biomarker Technologies Corporation (Beijing, China). The circular consensus sequencing (CCS) reads were generated from the corrected original subreads by SMRT Link (version 8.0) following the setting parameters: minPasses ≥ 5 and minPredictedAccuracy ≥ 0.9. After barcode and primer identification followed by chimera removal, high-quality reads were obtained and clustered as operational taxonomic units (OTUs) with similarity over 97% using USEARCH (version 10.0). Species annotation and taxonomic and diversity analyses were conducted with QIIME2 (version 2020.06). Chao1 and Shannon indexes were used to reflect alpha diversity, while nonmetric multidimensional scaling (NMDS) analysis was carried out to show the dissimilarity of beta diversity. The vegan package in R language was applied for ANOSIM analysis to measure the significant differences in beta diversity between groups. Linear discriminant analysis effect size (LEfSe) analysis with LDA score > 3 and statistical analysis of metagenomic profiles (STAMP) were performed to identify the significantly different bacteria between groups at the genus level.
2.4 Bile acid targeted metabolomic analysis
The high-throughput targeted quantification of bile acids in mice cecal contents was performed at Biomarker Technologies Corporation (Beijing, China) by UHPLC-MS/MS. Aliquots (25 mg) from 35 samples were weighed and added 1000 μL of precooled extract solution containing 0.1% formic acid and isotopically labeled internal standard mixture (acetonitrile-methanol-water, 2:2:1) was added per tube. After vortexing for 30 s, the mixture was homogenized at 35 Hz for 4 min, followed by sonication for 5 min in an ice-water bath. The latter 9-min circle was repeated three times, and then the tubes were incubated at -40°C for 1 h and centrifuged at 12000 rpm and 4°C for 15 min. The resulting supernatants were collected for UHPLC-MS/MS analysis.
An UHPLC System (Vanquish, ThermoFisher Scientific) equipped with a Waters ACQUITY UPLC BEH C18 column (150 *2.1 mm, 1.7 μm, Waters) was used for chromatographic separation. Mobile phase A included 1 mmol/L ammonium acetate and 0.1% acetic acid in water, and the mobile phase B was acetonitrile. The column temperature was set at 50°C, while the autosampler temperature was maintained at 4°C. The injection volume was 1 μL. Mass spectrometry in parallel reaction monitoring (PRM) mode was carried out by a Q Exactive HFX mass spectrometer (Thermo Fisher Scientific). The ion source parameters were as follows: spray voltage = +3500/-3100 V, sheath gas (N2) flow rate = 40, aux gas (N2) flow rate = 15, sweep gas (N2) flow rate = 0, aux gas (N2) temperature = 350°C, capillary temperature = 320°C.
A total of 39 bile acids were identified in this study (detailed in Supplementary Table 2). The calibration standard solutions were diluted stepwise with a dilution factor of 2 before being subjected to UHPLC-PRM-MS analysis. The final concentration (cF, nmol/L) of the samples measured was obtained by multiplying the directly calculated concentration (cC, nmol/L) output from the system by the dilution factor. The targeted metabolite concentration (cM, nmol/kg) in the tissue samples was calculated according to the following formula (VF: final volume of sample; m: sample mass):
2.5 Transcriptome analysis
Total RNA from mice colonic mucosa was extracted using the mirVana miRNA Isolation Kit (Ambion) following the manufacturer’s protocol. RNA integrity was assessed by an Agilent 2100 Bioanalyzer (Agilent Technologies, Santa Clara, CA, USA). Samples with an RNA integrity number (RIN) ≥ 7 were subjected to the subsequent analysis. The TruSeq Stranded mRNA LT Sample Prep Kit (Illumina, San Diego, CA, USA) was used to construct the libraries. Transcriptome sequencing and analysis were conducted by OE Biotech Corporation Ltd. (Shanghai, China).
The libraries were sequenced on the Illumina HiSeq X Ten platform. Approximately 50 megabytes of raw reads for each sample were generated. Raw data were preprocessed using Trimmomatic. After removing the reads containing poly N and the low-quality reads, clean reads were obtained and mapped to the mouse genome (GRCm38.p6) using HISAT2. The fragments per kilobase of exon model per million mapped fragments (FPKM) value of each gene was calculated using Cufflinks, and the read counts of each gene were obtained by HTSeq-count. Differentially expressed genes (DEGs) were identified using the DESeq (2012) R package, followed by gene set enrichment analysis (GSEA). P value < 0.05 and fold change > 2 or fold change < 0.5 were set as the thresholds for significantly differential expression. To demonstrate the expression pattern of genes in different groups and samples, hierarchical cluster analysis of DEGs was performed. R software based on the hypergeometric distribution was employed for Gene Ontology (GO) enrichment and Kyoto Encyclopedia of Genes and Genomes (KEGG) pathway enrichment analysis of DEGs. Immune infiltration analysis was performed using Immune Cell Abundance Identifier (ImmuCellAI).
2.6 Statistical analysis
Data are presented as the mean ± standard error of the mean (SEM). The GraphPad Prism (version 8.0; GraphPad Software, San Diego, CA, USA) was utilized for statistical analyses. Normality of distribution was confirmed using the Shapiro-Wilk test, and differences among groups were assessed using the Student’s t-test or two-way ANOVA when appropriate, with the Tukey’s post-hoc test. The log-rank test was used to compare survival rates. P < 0.05 was considered statistically significant.
3 Results
3.1 Tacrolimus ameliorated colitis in a DSS mouse model
Compared to the control mice, body weight loss, increased DAI scores and decreased survival rates were significantly observed in mice orally treated with 2.5% DSS for 7 days (Figures 1A, B, E). The dosage of tacrolimus (10 mg/kg) was determined by our previous study, which is the highest tolerated dose that most closely resembled the serum concentration in humans (Zhang et al., 2018). No significant difference in body weight, DAI scores, survival rate or colon length was observed in the tacrolimus and control groups, supporting the safety of tacrolimus at this dosage. Body weight, DAI scores and survival rates in the DSS colitis model were significantly (P<0.0001, P<0.0001, P<0.01, respectively) ameliorated by tacrolimus treatment (Figures 1A, B, E). Furthermore, colitis manifestations, including the shortened length, disruption of crypt-villus structure, goblet cell loss and immune cell infiltration, as determined by morphological changes and pathological scores were significantly (P<0.01) ameliorated in the DSS + Tacro group compared to the DSS group (Figures 1C, D, F, G). All these results indicated that tacrolimus ameliorated DSS-induced colitis in both clinical manifestation and pathological changes.
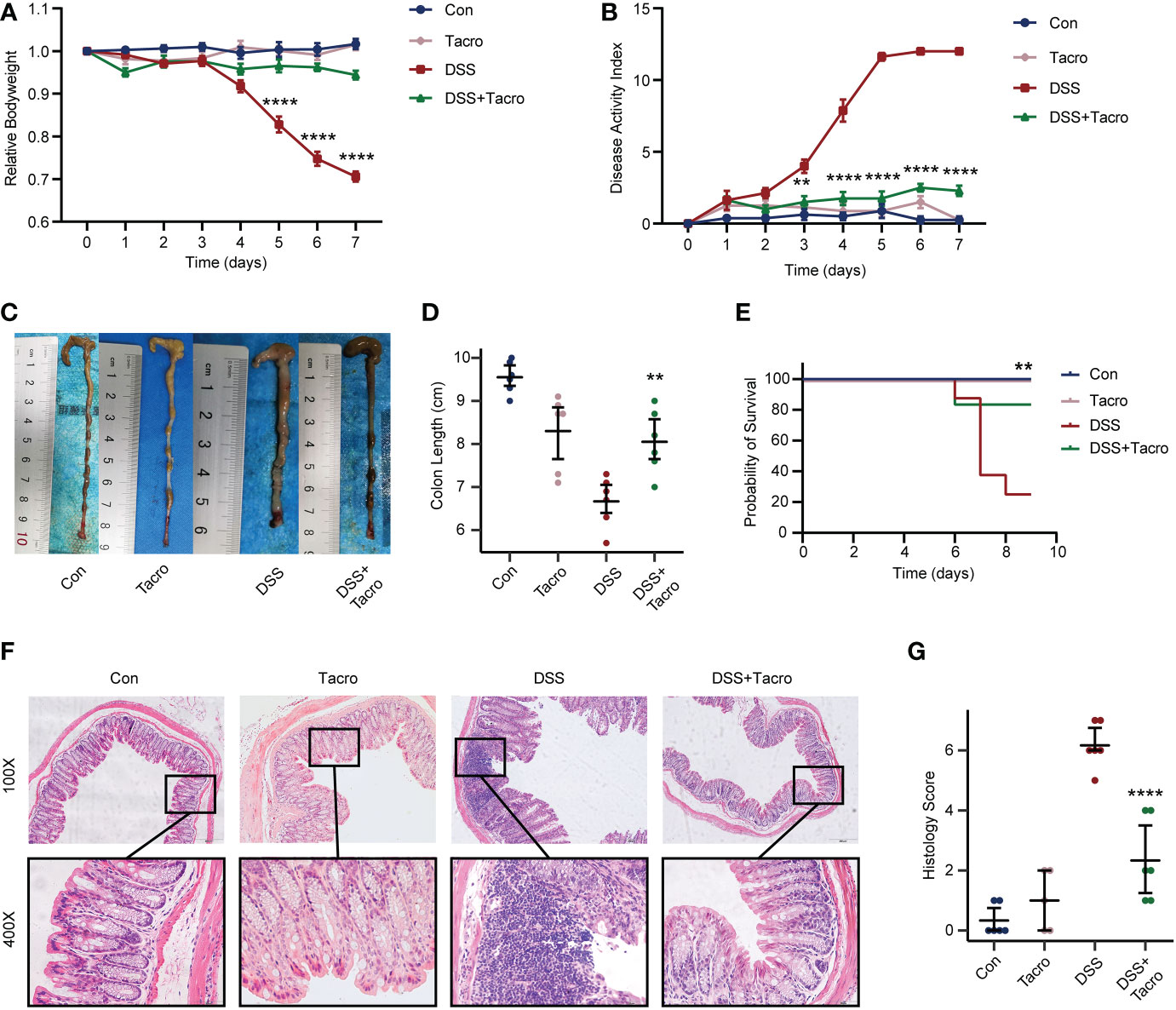
Figure 1 Tacrolimus alleviated DSS-induced colitis in mice. (A, B) Body weight changes and DAI scores of mice in different treatment groups. n=8 per group. (C, D) Gross view and length of colon. n=6 per group. (E) Survival rate of mice during the experiments. (F) Representative hematoxylin and eosin-stained images (100× and 400×) of colon sections. Scale bars, 200 μm (above) and 50 μm (below). (G) Histological scores of proximal and distal colons. n=6 per group. Data are presented as the mean ± SEM. **P < 0.01 and ****P < 0.0001. Significance reported for comparison of the DSS + Tacro and DSS groups. DAI, disease activity index; Con, control; DSS, dextran sulfate sodium; Tacro, 10 mg/kg tacrolimus.
Lower dosages of tacrolimus (0.1 mg/kg and 1 mg/kg) were also used in the acute DSS colitis model in our study. Tacrolimus at these lower dosages attenuated the increased DAI scores and improved survival in colitis (Supplementary Figures 1B, E), whereas no significant differences in weight change and colon length were observed in the low-dose DSS + Tacro group compared to the DSS group (Supplementary Figures 1A, C, D). These data also supported the effectiveness of tacrolimus in the DSS mouse model. For the consistency and effectiveness of the study, tacrolimus was used at a dosage of 10 mg/kg in the following study.
3.2 Tacrolimus induced beneficial alterations of the gut microbiome in DSS-induced colitis, characterized by a remarkable expansion of the genus Lactobacillus
To investigate the effect of tacrolimus on the diversity and composition of the gut microbiome in DSS-treated mice, 16S rRNA amplicon sequencing of DNA extracted from the cecal contents of mice was performed. The α-diversity of gut microbiota was not significantly different among the Control, Tacro, DSS and DSS + Tacro groups, manifested as the Chao1 index or Shannon index in Figure 2A.
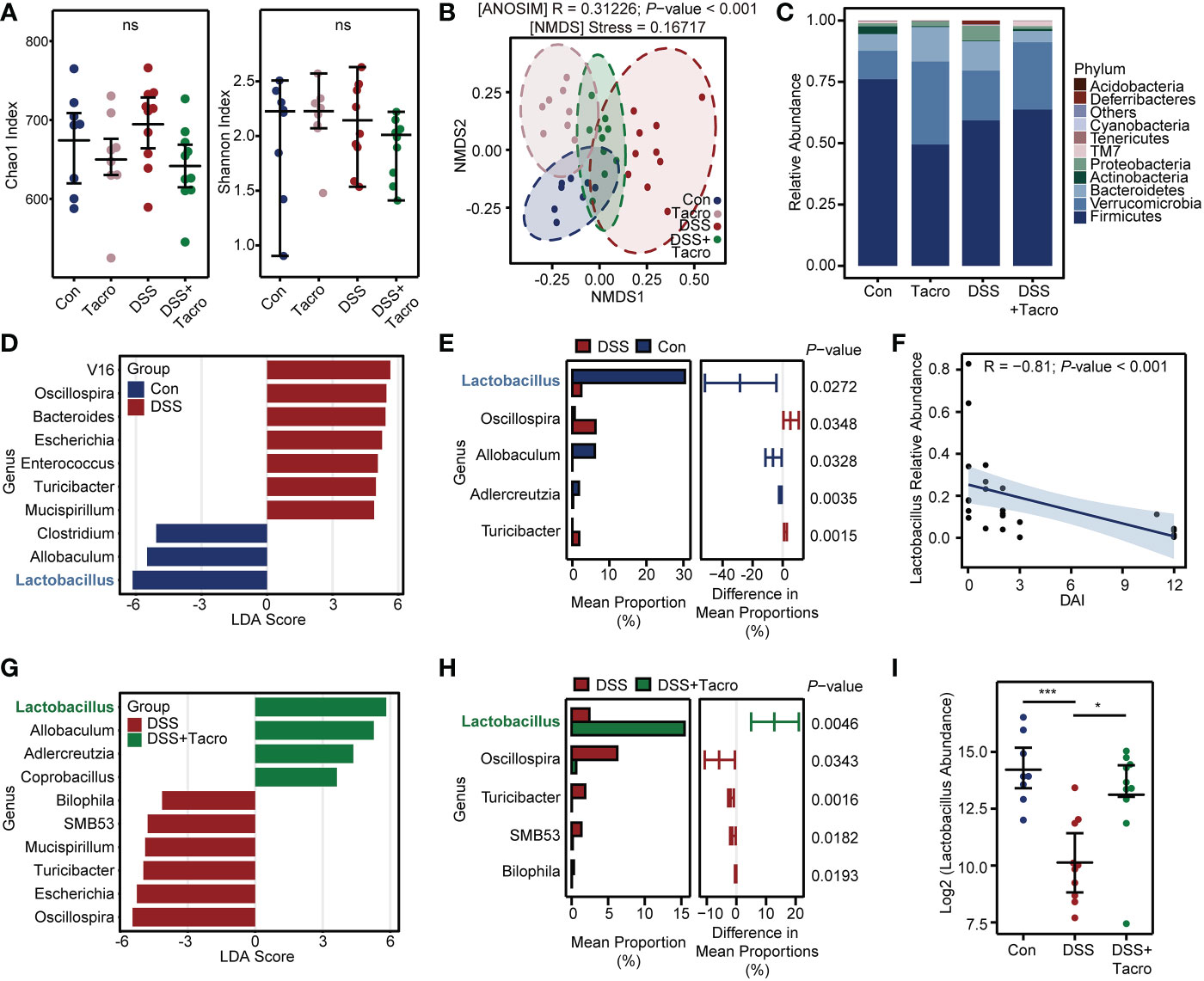
Figure 2 Tacrolimus triggered a significant increase in the genus Lactobacillus in colitis. (A) The microbial α-diversity in the cecal contents of mice. (B) β-diversity analysis based on the NMDS and ANOSIM methods. (C) Relative abundance of gut bacterial composition at the phylum level. (D) The LDA scores between the control and DSS-treated groups at the genus level. The threshold was set as 3. (E) The significantly different bacteria between the control and DSS-treated groups evaluated by STAMP. (F) Correlation analysis between Lactobacillus relative abundance and DAI score. A total of 28 samples from the Con, DSS and DSS + Tacro groups were included in this analysis. (G) The LDA scores of the DSS + Tacro group when compared with the DSS group at the genus level. (H) Significantly different genera between the DSS and DSS + Tacro groups evaluated by STAMP. (I) Lactobacillus abundance in log 2 scale among different treatment groups. Data are presented as the mean ± SEM. *P < 0.05, ***P < 0.001, ns, no significance; Con, control; DSS, dextran sulfate sodium; Tacro, 10 mg/kg tacrolimus; NMDS, nonmetric multidimensional scaling; LDA, linear discriminant analysis; STAMP, statistical analysis of metagenomic profile; DAI, disease activity index.
According to the β-diversity of the gut microbiota, NMDS analysis was used, and there was significant (P<0.001) separation of microbial communities among the control, DSS and DSS + Tacro groups. The DSS group was located distant from the control group, while the position of the DSS + Tacro group was closer to the control group than to the DSS group (Figure 2B). Consistent with a previous study (Shin et al., 2015), DSS administration resulted in an obvious increase in the relative abundance of pylum_Proteobacteria and Deferribacteres and a decrease in the Firmicutes/Bacteroidetes ratio. All these changes were reversed by tacrolimus treatment (Figure 2C). LEfSe is a commonly used method to identify potential biomarkers with significant differences among groups. In our study, the abundances of the genera_Oscillospira, Bacteroides, Escherichia, Enterococcus, Turicibacter and Mucispirillum were significantly enriched, whereas the abundances of the genera_Lactobacillus, Allobaculum and Clostridium were significantly decreased in the DSS group compared to the normal control group (Figure 2D). Among the bacteria that differed significantly between the control and DSS groups at the genus level, Lactobacillus occupied the largest mean proportion, approximately 30% (Figure 2E). Correlation analysis showed that the DAI scores of colitis were negatively correlated with the abundance of the genus_Lactobacillus, as shown in Figure 2F (R=-0.81; P < 0.001) and Supplementary Figure 2A (R=-0.76; P < 0.001).
In the DSS + Tacro group, LEfSe analysis found that the increased abundance of the genera_Oscillospira, Escherichia, Turicibacter, Mucispirillum, SMB53, Bilophila as well as the decreased abundance of the genera_Lactobacillus and Allobaculum in the colitis model were significantly reversed by tacrolimus treatment (Figure 2G). Tacrolimus remarkably (P < 0.05) restored the abundance of the genera_ Lactobacillus, Oscillospira, Turicibacter, etc. (Figures 2H, I). Among these significant changes in the microbiota, the abundance of the genus_Lactobacillus showed the most significant changes, with the highest proportion (approximately 15%) in the DSS + Tacro group (Figure 2H). To validate our findings, we performed the same research in another center and confirmed similar trends, both showing a decrease in the genus_Lactobacillus in the DSS group and a restoration of its abundance when treated with tacrolimus (Supplementary Figures 2A, B). This strengthened our confidence in the findings, although the composition of gut microbiota is not similar in two centers.
3.3 Lactobacillus plantarum 550 (Lacto) further ameliorated colitis combined with tacrolimus treatment
Lacto, a strain of the genus Lactobacillus plantarum, was recently isolated from pickle of Sichuan cuisine by our group and determined by 16S ribosomal DNA identification. Body weight changes in the DSS + 1Tacro (1 mg/kg) group and the DSS + Lacto group were not significantly different from those in the DSS group, whereas the combination of Lacto in the DSS + 1Tacro + Lacto group maintained the body weight at a higher level, even closer to that in the DSS + 10Tacro (10 mg/kg) group (Figure 3A). Moreover, Lacto further prevented body weight loss in DSS + 10Tacro mice (Figure 3A) compared to monotherapy with tacrolimus in the DSS model (P < 0.05). As determined by DAI, the combination of Lacto with either tacrolimus at doses of 1 mg/kg or 10 mg/kg significantly (P < 0.01 and P < 0.0001, respectively) decreased DAI scores compared to the DSS group (Figure 3B). Colon length, which is negatively related to the inflammation of colitis (Liu et al., 2017), was measured, and a significantly increased length was reported in the DSS + 10Tacro (P < 0.01) as well as the DSS + Tacro + Lacto groups (P < 0.05, P < 0.01, respectively), with the most increased length in the DSS + 10 Tacro + Lacto group (Figures 3C, D). Survival rates were significantly (P < 0.01) increased in the DSS + 10Tacro and DSS + 10Tacro + Lacto groups, with the latter group showing better survival (Figure 3E). Compared to the DSS group, cotreatment with tacrolimus and Lacto obviously (P < 0.05 and P < 0.01, respectively) relieved the inflammation of the colonic mucosa, as determined by the histological scores (Figures 3F, G). Overall, Lacto, combined with tacrolimus, further ameliorated colitis in a mouse model in clinical and histological manifestations.
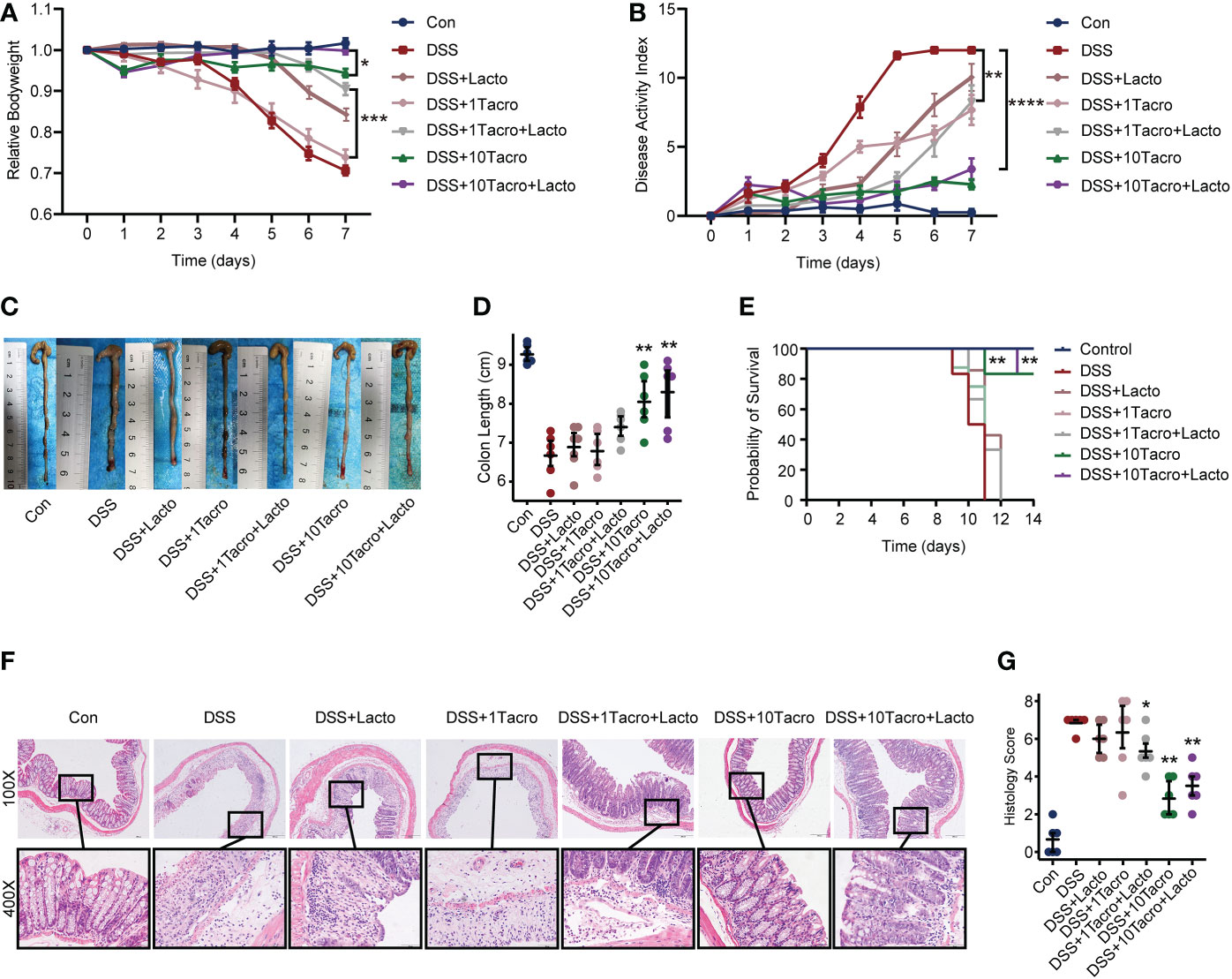
Figure 3 The combination of tacrolimus and Lactobacillus plantarum 550 achieved better remission in colitis. (A, B) Body weight changes and DAI scores of mice in different treatment groups. n=8 per group. (C, D) Gross view and length of colons. n=6 per group. (E) Survival rate of mice during the experiments. (F) Representative hematoxylin and eosin-stained images (100× and 400×) of colon sections. Scale bars, 200 μm (above) and 50 μm (below). (G) Histological scores of proximal and distal colons. n=6 per group. Data are presented as the mean ± SEM. *P < 0.05, **P < 0.01, ***P < 0.001 and ****P < 0.0001. The significance reported in (B), (D), (E), and (G) is for comparison all to the DSS group. DAI, disease activity index; Con, control; DSS, dextran sulfate sodium; 1Tacro, 1 mg/kg tacrolimus; 10Tacro, 10 mg/kg tacrolimus; Lacto, Lactobacillus plantarum 550.
3.4 The combination of tacrolimus and lacto significantly inhibited the proinflammatory signaling pathways
RNA sequencing data revealed that at the transcriptional level, the immune and inflammation-related signaling pathways, including the allograft rejection, IFN-γ response, IFN-α response, IL6 JAK STAT3 signaling, inflammatory response, TNFα signaling via NF-κB, complement and IL2 STAT5 signaling were significantly upregulated after DSS administration (Figure 4A). Most of these pathways, including the allograft rejection, TNFα signaling via NF-κB, IL6 JAK STAT3 signaling, inflammatory response and complement were significantly downregulated by tacrolimus (Figure 4B). When tacrolimus was combined with Lacto, the signaling pathways, including IFN-γ and IFN-α response, allograft rejection, IL2 STAT5 signaling and the inflammatory response, were further downregulated (Figure 4C), implying that the combined therapy of tacrolimus and Lacto further inhibited the inflammatory response in the colonic mucosa of colitis mice compared to the tacrolimus monotherapy group. The results of the gene set variation analysis are visualized in Figure 4D. The range of colors indicates the relative abundance of the signaling pathways mentioned above for each sample.
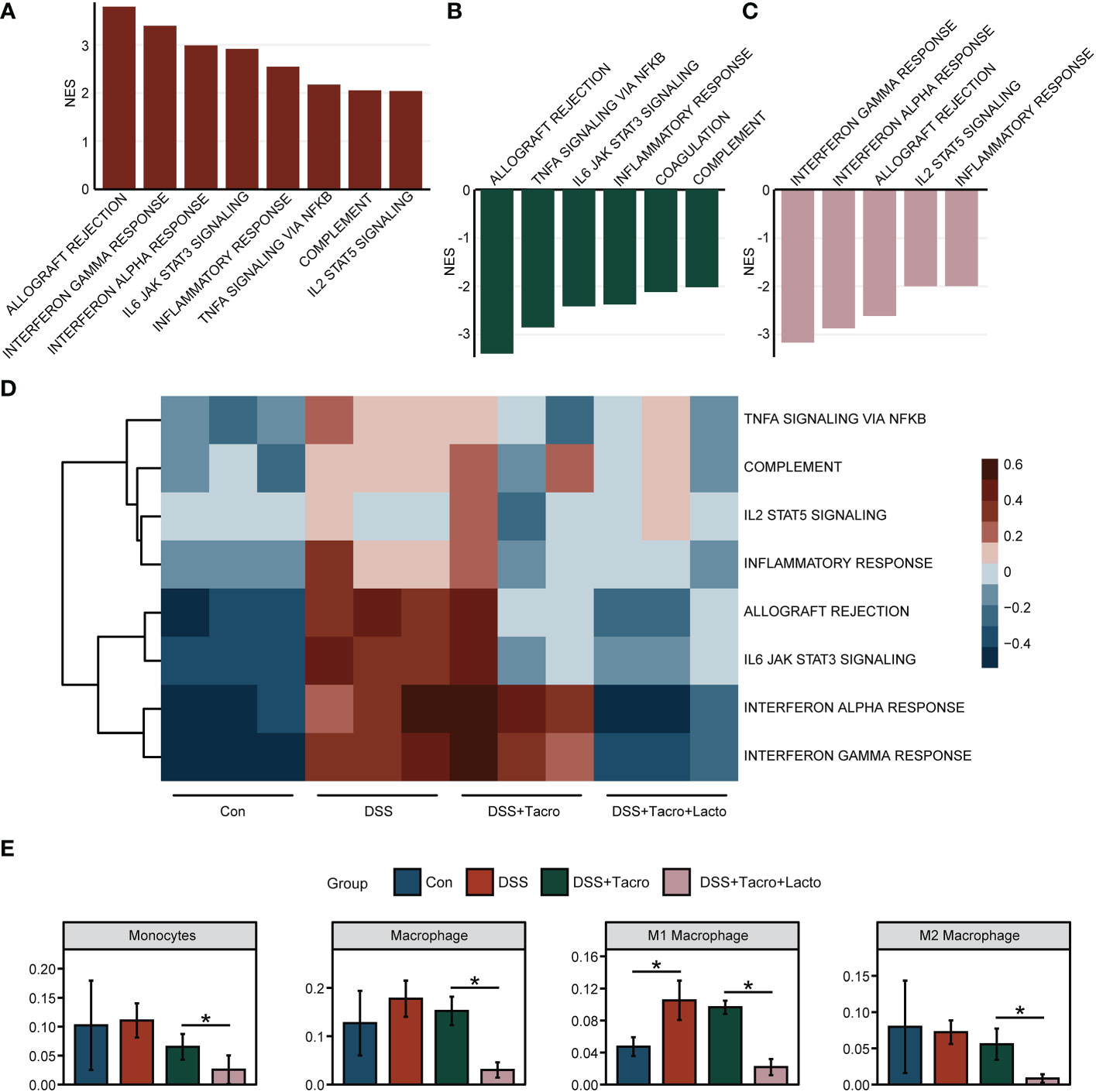
Figure 4 Lactobacillus plantarum 550 supplementation modulated intestinal mucosa inflammation at the transcriptional level. (A–C) Gene set enrichment analysis. NES values greater than 2 were demonstrated. The results represent pathways modulated in the (A) DSS group versus Con group, (B) DSS + Tacro group versus DSS group and (C) DSS + Tacro + Lacto group versus DSS + Tacro group. (D) Results of gene set variation analysis for selected biological processes in all groups. (E) The profile of infiltrating immune cells in the Con, DSS, DSS + Tacro and DSS + Tacro + Lacto groups. Data are presented as the mean ± SEM. *P < 0.05. NES, normalized enrichment score; Con, control; DSS, dextran sulfate sodium; Tacro, 10 mg/kg tacrolimus; Lacto, Lactobacillus plantarum 550. n=3 per group.
To demonstrate the profile of immune cells in the colonic mucosa, we analyzed the immune cell infiltration levels based on transcriptome sequencing data. As shown in Figure 4E, the proportion of monocytes and macrophages, especially the M1 subtypes, was elevated in the DSS group, while the M2 subtypes were slightly reduced with DSS intervention, in accordance with published reports overviewing the opposing roles of M1 and M2 macrophages in DSS-induced colitis (Lin et al., 2014). To a certain extent, tacrolimus suppressed the increase in the proportion of monocytes and macrophages, whereas coadministration of tacrolimus and Lacto significantly (P < 0.05) inhibited the expansion of these innate immune cells (Figure 4E) but not other immune cells, including dendritic cells (DCs), type 1 conventional dendritic cells (cDC1), type 2 conventional dendritic cells (cDC2), plasmacytoid dendritic cells (pDCs), B cells, memory B cells, B1 cells, follicular B cells, germinal center B cells, CD8+ cytotoxic T cells (Tc), naïve CD8+ T cells, Tγδ cells (Tgd), mast cells, M2 macrophages, NK cells, basophils, granulocytes and eosinophils (Supplementary Figure 3). Altogether, our results suggested that the downregulation of inflammatory signaling pathways may be one of the possible mechanisms accounting for the alleviation of colonic mucosal inflammation after Lacto supplementation.
3.5 Combination of tacrolimus and Lacto improved the diversity of the gut microbiome and changed the bile acids profiles in colitis
Next, to explore whether the effect of Lacto supplementation on colitis remission had some relationship with alterations in the gut microbiome, similar to that previously observed in the tacrolimus-treated group (Zhang et al., 2018; Jiao et al., 2020), we compared the results of 16S rRNA sequencing in the presence and absence of Lacto. Increased α-diversity of the gut microbiome was observed in the combination treatment group compared to the tacrolimus-treated group, as reflected by the Chao1 and Shannon indexes (Figure 5A). The results from NMDS and ANOSIM analysis clearly indicated that the bacterial communities of these two groups differed from each other (Figure 5B). In contrast with tacrolimus treatment, the relative abundance of the pathogenic bacteria Proteobacteria was further reduced, while the abundance of Firmicutes and Bacteroidetes, the two major phyla composing the gut microbiota community in healthy humans, was better maintained by Lacto supplementation (Figure 5C). However, Lacto alone did not significantly affect the bacterial diversity or cause beneficial changes in the composition of gut microbiota in colitis (Figures 5D–F).
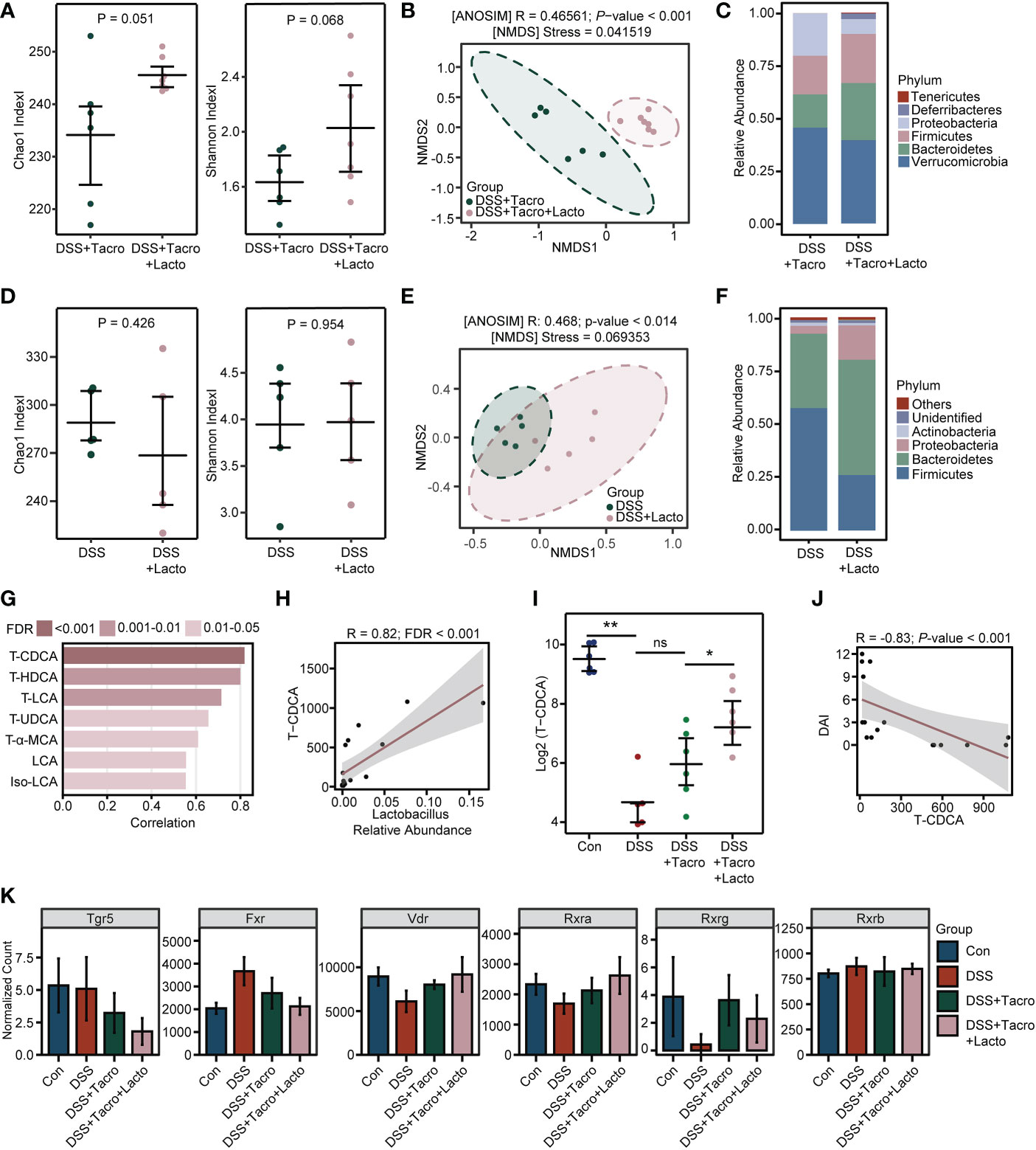
Figure 5 Tacrolimus coadministered with Lactobacillus plantarum 550 changed the diversity of the gut microbiome and bile acid metabolism in colitis. (A, D) Gut microbial α-diversity determined by Chao1 and Shannon indexes. (B, E) β-diversity analysis based on the NMDS and ANOSIM methods. (C, F) Relative abundance of gut bacterial composition at the phylum level. (G, H) Correlation analysis between bile acid concentrations and Lactobacillus relative abundance. (I) Relative concentration of TCDCA on a log 2 scale. (J) Correlation analysis between DAI score and TCDCA concentration. (K) The normalized expression levels of bile acid receptors among all groups. Data are presented as the mean ± SEM. *P < 0.05, **P < 0.01, ns, no significance. NMDS, nonmetric multidimensional scaling; FDR, false discovery rate; DAI, disease activity index; Con, control; DSS, dextran sulfate sodium; Tacro, 10 mg/kg tacrolimus; Lacto, Lactobacillus plantarum 550; TCDCA, taurochenodeoxycholic acid; Tgr5, Takeda G protein-coupled receptor 5; Fxr, farnesoid X receptor; Vdr, vitamin D (1,25- dihydroxyvitamin D3) receptor; Rxra, retinoid X receptor alpha; Rxrg, retinoid X receptor gamma; Rxrb, retinoid X receptor beta.
Bile acid metabolism is a biological process in which specific intestinal floras are actively involved (Wahlström et al., 2016). Recently, gut microbiome-derived bile acids have been reported to play a critical role in IBD patients and experimental colitis(Lavelle and Sokol, 2020; Sinha et al., 2020; Thomas et al., 2022). Considering the significant changes in diversity and composition after the combination of tacrolimus and Lacto, bile acids were also detected in our study through high-throughput sequencing. Among all the bile acids analyzed in the Con, DSS and DSS + Tacro groups, the cecal taurochenodeoxycholic acid (TCDCA) level was most strongly correlated with the relative abundance of the genus_Lactobacillus (Figure 5G). Furthermore, a significant positive correlation (R=0.82; FDR<0.001) between the TCDCA concentration and the abundance of genus_Lactobacillus was observed in our experiments (Figure 5H).
The TCDCA levels that remarkably (P < 0.01) declined after DSS intervention were not restored by tacrolimus treatment. However, the combined treatment of tacrolimus plus Lacto drastically (P < 0.05) increased the TCDCA concentrations in comparison with tacrolimus treatment (Figure 5I). In addition, the cecal TCDCA concentrations of mice were negatively (R=-0.83; P<0.001) correlated with the DAI score (Figure 5J), suggesting an association between this bile acid and colitis severity.
Bile acids can function as signaling molecules to activate their receptors, thereby affecting intestinal inflammation (Perino et al., 2021; Yang et al., 2021). As presented by our transcriptome analysis, DSS administration perturbed the expression of the bile acid-sensing receptors, including G-protein-coupled bile acid receptor 1 (Gpbar1, TGR5), farnesoid X receptor (FXR), vitamin D (1,25-dihydroxyvitamin D3) receptor (VDR), and retinoid X receptor (RXR), to various degrees, all of which were mitigated somewhat by tacrolimus treatment except TGR5, and this effect was amplified further by coadministration of Lacto (Figure 5K). Collectively, our findings demonstrated that the combination of tacrolimus and Lacto improved the diversity and composition of the gut microbiome as well as bile acid metabolism in colitis mice, which possibly contributed to the alleviation of intestinal inflammation. The detailed mechanisms remain to be further explored.
4 Discussion
In this study, we confirmed that tacrolimus ameliorated DSS-induced colitis in a mouse model. Beneficial alterations of the gut microbiome characterized by a remarkable expansion of the genus Lactobacillus were observed after tacrolimus treatment. Oral supplementation with one of the species in this genus (Lactobacillus plantarum 550, Lacto) further ameliorated colitis. Compared to the monotherapy of tacrolimus, the combination of Lacto and tacrolimus further downregulated the proinflammatory signaling pathways while significantly inhibiting the proportion of monocytes and macrophages, especially the M1 subtypes in the colon. During this process, the diversity of the gut microbiome as well as bile acid metabolism was significantly changed, which may contribute to the remission of colitis.
Recently, tacrolimus has been recommended for the treatment of refractory IBD, showing efficacy in both human and animal colitis (Baumgart et al., 2006; Yoshino et al., 2010). In addition to the traditional calcineurin-dependent inhibitory effect on T cells, several studies have reported that the mechanism of tacrolimus-ameliorated colitis might be related to the suppression of activated macrophages (Yoshino et al., 2010; Cai et al., 2021), the restriction of dendritic cell migration and the subsequent differentiation of CD4+ T cells to Th1 and Th17 cells (Regmi et al., 2019), as well as protection against apoptosis-mediated intestinal epithelial injury (Satake et al., 2022). In accordance with previous research, we found a significant alleviation of DSS-induced colitis by tacrolimus treatment. Signaling pathways, including the IFN-γ response, TNFα signaling via NF-κB, IL6 JAK STAT3 signaling and inflammatory response pathways upregulated after DSS administration were significantly inhibited by tacrolimus.
The gut microbiome plays an important role in the regulation of the immune response (Shi et al., 2017). Except for the immunosuppressive effect of tacrolimus itself, previous studies by our group and other groups have reported that tacrolimus changes the diversity and composition of the gut microbiome, resulting in the regulation of graft rejection (Jiang et al., 2018; Zhang et al., 2018), glucose metabolism (Bhat et al., 2017; Jiao et al., 2020), infections (Tourret et al., 2017) and endothelial function (Toral et al., 2018). In this study, the changes in the tacrolimus-induced microbiome in a colitis model were first described, which was characterized by a significant increase in the abundance of Lactobacillus followed by Allobaculum at the genus level. This is consistent with our prior studies showing the abundant genera Lactobacillus and Allobaculum after tacrolimus treatment in normal mice (Zhang et al., 2018; Jiao et al., 2020). Allobaculum was favored as an indicator of defective immune responses (Dimitriu et al., 2013).
The genus Lactobacillus was commonly reported to be decreased in IBD and experimental colitis (Sartor, 2004; Wang et al., 2020), which was also confirmed in our present study. And restoration or supplementation of this probiotic was associated with the alleviation of intestinal inflammation (Oliva et al., 2012; Wang et al., 2020). Based on our previous research proposing that tacrolimus plus gut microbiota achieved an increased allograft survival rate (Zhang et al., 2018), this study confirmed that the combination of tacrolimus and Lactobacillus strains played a positive role in colitis. Since we observed that the colonic contents were inadequate for the detection of two-omics, especially in the condition of colitis, the cecal content was used following the protocol as previous studies (Wong et al., 2022). There are certain limitations to do this since the most representative alterations in the gut microbiome are in the area where inflammation occurs. However, the efficacy of combination therapy confirmed the decreased abundance of the genus_Lactobacillus in DSS-induced colitis model.
Efficacy of tacrolimus combined with Lactobacillus spp. has been demonstrated in lupus-prone mice (Kim et al., 2021), graft-versus-host disease (Beak et al., 2022), and adult-type atopic dermatitis (Moroi et al., 2011), which acted by modulating the balance of Treg cells and Th17 cells. Here, we chose Lacto, one strain recently isolated from pickle by our group in Sichuan cuisine and proven to generate short-chain fatty acids (butyric acid, acetic acid, propionic acid, etc.) in preliminary experiments as a supplement for tacrolimus treatment. In recent years, some strains from Lactobacillus plantarum were reported to attenuate colitis in mice by restoring the disturbed gut microbiota, affecting intestinal barrier functions and immunity-related gene expression(Sun et al., 2020; Wu et al., 2022). Our results showed better remission of colitis in the combination treatment group than in the tacrolimus monotherapy group, accompanied by further downregulation of inflammatory signaling pathways. As we showed in Figure 3A, the combination of tacrolimus and Lacto, at both lower dosage (1 mg/kg) and higher dosage (10 mg/kg) promoted the therapeutic effect of colitis in mice, compared to the monotherapy of tacrolimus. Especially, the efficacy of tacrolimus (1mg/kg) combined with Lacto was close to the tacrolimus (10mg/kg) monotherapy. All of these results have shown that the combination therapy may reduce the dosage of tacrolimus for the treatment of colitis, thereby reducing dose-related side effects. Furthermore, by analyzing transcriptome sequencing data, we first reported that tacrolimus combined with Lacto treatment decreased the proportion of both colonic monocytes and macrophages in colitis but not dendritic cells (DC), type 1 conventional dendritic cells (cDC1), type 2 conventional dendritic cells (cDC2), plasmacytoid dendritic cells (pDC), B cells, memory B cells, B1 cells, follicular B cells, germinal center B cells, CD8+ cytotoxic T cells (Tc), naïve CD8+ T cells, Tγδ cells (Tgd), mast cells, M2 macrophages, NK cells, basophils, granulocytes and eosinophils. Our data demonstrated that macrophages and the proinflammatory signaling pathway play a vital role in the amelioration of colitis induced by tacrolimus combined with Lacto.
Theoretically, an improved composition of the gut microbiome and bile acid metabolism might help explain the mechanism of amelioration after combination therapy. A recent study has shown that one strain from Lactobacillus casei ameliorated DSS-induced colitis in mice by increasing taurine-conjugated bile acids, and the activation of FXR by the increased TCDCA might exhibit anti-inflammatory effects (Wong et al., 2022). This possibly resulted from the activation of FXR shifting the polarization of macrophages toward the anti-inflammatory M2 phenotype, which promoted IL-10 secretion and inhibited IFN-γ production (Fiorucci et al., 2018). Published evidence also supports that colonic VDR signaling upregulated by 1,25−dihydroxyvitamin D (1,25(OH)2D3) or microbial metabolites (bile acids, butyrate, etc.) contributed to the restoration of macrophage subtype balance, thus ameliorating colitis (Zhu et al., 2019; Battistini et al., 2020). Moreover, the nuclear receptor RXR can form heterodimers with VDR or the peroxisome proliferator–activated receptor γ (PPAR γ) (Kiss et al., 2013), which is highly expressed in the colon and can be activated by butyrate (Desreumaux et al., 2001; Litvak et al., 2018). Either VDR/RXR or RXR/PPAR γ heterodimer activation was proved to protect against colonic inflammation (Desreumaux et al., 2001; Zhu et al., 2019). In our study, the observed increase in TCDCA concentration after combination treatment might not be attributed to a direct metabolic effect of the genus Lactobacillus on TCDCA, since this probiotic is mainly involved in the deconjugation, 7α−dehydroxylation and esterification of bile acids(Jia et al., 2018). Instead, this might result from complex factors such as regulation of enterohepatic circulation or bacterial interactions that deserve further research. Based on our results and the studies mentioned above, we speculated that the combination treatment of tacrolimus and Lacto induced beneficial alterations in the gut microbiome as well as the derived bile acid profile, which led to an upregulated expression of bile acid receptors (FXR, VDR, RXR) in colonic macrophages, thereby restoring the balance of the macrophage M1/M2 subtype and inhibiting the release of proinflammatory cytokine. As a result, the inflammatory signaling pathways were downregulated, and colonic inflammation was relieved. Additional experiments in vitro that correlating TCDCA, intestinal inflammation and Lactobacillus plantarum should be conducted to explore the mechanism underlying the efficacy of the combination therapy in colitis in the future.
5 Conclusion
The narrow therapeutic window of tacrolimus requires a promising treatment strategy for IBD that reduces the side effects while enhancing its efficacy. In this study, we confirmed the efficacy of tacrolimus treatment and innovatively demonstrated alterations in the tacrolimus-induced gut microbiome in a colitis model. Lactobacillus plantarum promotes the therapeutic effect of tacrolimus in colitis, possibly resulting from alterations in the gut microbiome and bile acid profile, which lead to the maintenance of macrophage M1/M2 subtype balance. Our findings offer a prospective strategy to combine tacrolimus and Lactobacillus for the treatment of colitis patients.
Data availability statement
The raw data of the 16S rRNA sequencing in this study is publicly available in the SRA database, accession number PRJNA940074.
Ethics statement
The animal study was reviewed and approved by Animal Ethics Committees of Beijing Chao-Yang Hospital and the Animal Ethics Committee of West China Hospital of Sichuan University (Ref. No. 20211277A).
Author contributions
WL and DZ contributed equally to this paper. WL performed the experiments and drafted the manuscript. DZ performed part of the experiment, analyzed the data, drew the figures and helped to revise the manuscript. TH, YL and LS provided ideas for writing and helped with the experiments and revision of the manuscript. ZL and XP isolated the Lactobacillus plantarum 550 and completed pre-experiments for strain performance testing. LL and YW designed and supervised this study. All authors contributed to the article and agree to be accountable for the content of the work.
Funding
This study was supported by The General Program of National Natural Science Foundation of China (NSFC) (81970463) and the Sichuan Regional Innovation Cooperation Project (2022YFQ0053).
Acknowledgments
We thank Technology Research Institute of Shuxi Condiments of Sichuan Cuisine Corporation Ltd. (Chengdu, China) for their contribution of the probiotic used in this study.
Conflict of interest
ZL and XP are employed by Technology Research Institute of Shuxi Condiments of Sichuan Cuisine Co. LTD.
The remaining authors declare that the research was conducted in the absence of any commercial or financial relationships that could be construed as a potential conflict of interest.
Publisher’s note
All claims expressed in this article are solely those of the authors and do not necessarily represent those of their affiliated organizations, or those of the publisher, the editors and the reviewers. Any product that may be evaluated in this article, or claim that may be made by its manufacturer, is not guaranteed or endorsed by the publisher.
Supplementary material
The Supplementary Material for this article can be found online at: https://www.frontiersin.org/articles/10.3389/fcimb.2023.1130820/full#supplementary-material
References
Battistini, C., Ballan, R., Herkenhoff, M. E., Saad, S. M. I., Sun, J. (2020). Vitamin d modulates intestinal microbiota in inflammatory bowel diseases. Int. J. Mol. Sci. 22 (1):362. doi: 10.3390/ijms22010362
Baumgart, D. C., Pintoffl, J. P., Sturm, A., Wiedenmann, B., Dignass, A. U. (2006). Tacrolimus is safe and effective in patients with severe steroid-refractory or steroid-dependent inflammatory bowel disease–a long-term follow-up. Am. J. Gastroenterol. 101 (5), 1048–1056. doi: 10.1111/j.1572-0241.2006.00524.x
Beak, J. A., Park, M. J., Kim, S. Y., Jhun, J., Woo, J. S., Choi, J. W., et al. (2022). FK506 and lactobacillus acidophilus ameliorate acute graft-versus-host disease by modulating the T helper 17/regulatory T-cell balance. J. Transl. Med. 20 (1), 104. doi: 10.1186/s12967-022-03303-z
Bhat, M., Pasini, E., Copeland, J., Angeli, M., Husain, S., Kumar, D., et al. (2017). Impact of immunosuppression on the metagenomic composition of the intestinal microbiome: a systems biology approach to post-transplant diabetes. Sci. Rep. 7 (1), 10277. doi: 10.1038/s41598-017-10471-2
Cai, X., Wang, X., He, M., Wang, Y., Lan, M., Zhao, Y., et al. (2021). Colon-targeted delivery of tacrolimus using pH-responsive polymeric nanoparticles for murine colitis therapy. Int. J. Pharm. 606, 120836. doi: 10.1016/j.ijpharm.2021.120836
Caruso, R., Lo, B. C., Núñez, G. (2020). Host-microbiota interactions in inflammatory bowel disease. Nat. Rev. Immunol. 20 (7), 411–426. doi: 10.1038/s41577-019-0268-7
Chow, D. K., Leong, R. W. (2007). The use of tacrolimus in the treatment of inflammatory bowel disease. Expert Opin. Drug Saf. 6 (5), 479–485. doi: 10.1517/14740338.6.5.479
Desreumaux, P., Dubuquoy, L., Nutten, S., Peuchmaur, M., Englaro, W., Schoonjans, K., et al. (2001). Attenuation of colon inflammation through activators of the retinoid X receptor (RXR)/peroxisome proliferator-activated receptor gamma (PPARgamma) heterodimer. a basis for new therapeutic strategies. J. Exp. Med. 193 (7), 827–838. doi: 10.1084/jem.193.7.827
Dimitriu, P. A., Boyce, G., Samarakoon, A., Hartmann, M., Johnson, P., Mohn, W. W. (2013). Temporal stability of the mouse gut microbiota in relation to innate and adaptive immunity. Environ. Microbiol. Rep. 5 (2), 200–210. doi: 10.1111/j.1758-2229.2012.00393.x
Fellermann, K., Tanko, Z., Herrlinger, K. R., Witthoeft, T., Homann, N., Bruening, A., et al. (2002). Response of refractory colitis to intravenous or oral tacrolimus (FK506). Inflammation Bowel Dis. 8 (5), 317–324. doi: 10.1097/00054725-200209000-00002
Fiorucci, S., Biagioli, M., Zampella, A., Distrutti, E. (2018). Bile acids activated receptors regulate innate immunity. Front. Immunol. 9, 1853. doi: 10.3389/fimmu.2018.01853
Gordon, M., Sinopoulou, V., Akobeng, A. K., Pana, M., Gasiea, R., Moran, G. W. (2022). Tacrolimus (FK506) for induction of remission in corticosteroid-refractory ulcerative colitis. Cochrane Database Syst. Rev. 4 (4), Cd007216. doi: 10.1002/14651858.CD007216.pub2
Honda, K., Littman, D. R. (2012). The microbiome in infectious disease and inflammation. Annu. Rev. Immunol. 30, 759–795. doi: 10.1146/annurev-immunol-020711-074937
Jang, Y. J., Kim, W. K., Han, D. H., Lee, K., Ko, G. (2019). Lactobacillus fermentum species ameliorate dextran sulfate sodium-induced colitis by regulating the immune response and altering gut microbiota. Gut Microbes 10 (6), 696–711. doi: 10.1080/19490976.2019.1589281
Jia, W., Xie, G., Jia, W. (2018). Bile acid-microbiota crosstalk in gastrointestinal inflammation and carcinogenesis. Nat. Rev. Gastroenterol. Hepatol. 15 (2), 111–128. doi: 10.1038/nrgastro.2017.119
Jiang, J. W., Ren, Z. G., Lu, H. F., Zhang, H., Li, A., Cui, G. Y., et al. (2018). Optimal immunosuppressor induces stable gut microbiota after liver transplantation. World J. Gastroenterol. 24 (34), 3871–3883. doi: 10.3748/wjg.v24.i34.3871
Jiao, W., Zhang, Z., Xu, Y., Gong, L., Zhang, W., Tang, H., et al. (2020). Butyric acid normalizes hyperglycemia caused by the tacrolimus-induced gut microbiota. Am. J. Transplant. 20 (9), 2413–2424. doi: 10.1111/ajt.15880
Kim, D. S., Park, Y., Choi, J. W., Park, S. H., Cho, M. L., Kwok, S. K. (2021). Lactobacillus acidophilus supplementation exerts a synergistic effect on tacrolimus efficacy by modulating Th17/Treg balance in lupus-prone mice via the SIGNR3 pathway. Front. Immunol. 12, 696074. doi: 10.3389/fimmu.2021.696074
Kiss, M., Czimmerer, Z., Nagy, L. (2013). The role of lipid-activated nuclear receptors in shaping macrophage and dendritic cell function: From physiology to pathology. J. Allergy Clin. Immunol. 132 (2), 264–286. doi: 10.1016/j.jaci.2013.05.044
Komaki, Y., Komaki, F., Ido, A., Sakuraba, A. (2016). Efficacy and safety of tacrolimus therapy for active ulcerative colitis; a systematic review and meta-analysis. J. Crohns Colitis 10 (4), 484–494. doi: 10.1093/ecco-jcc/jjv221
Lavelle, A., Sokol, H. (2020). Gut microbiota-derived metabolites as key actors in inflammatory bowel disease. Nat. Rev. Gastroenterol. Hepatol. 17 (4), 223–237. doi: 10.1038/s41575-019-0258-z
Lee, M., Chang, E. B. (2021). Inflammatory bowel diseases (IBD) and the microbiome-searching the crime scene for clues. Gastroenterology 160 (2), 524–537. doi: 10.1053/j.gastro.2020.09.056
Lin, Y., Yang, X., Yue, W., Xu, X., Li, B., Zou, L., et al. (2014). Chemerin aggravates DSS-induced colitis by suppressing M2 macrophage polarization. Cell Mol. Immunol. 11 (4), 355–366. doi: 10.1038/cmi.2014.15
Litvak, Y., Byndloss, M. X., Bäumler, A. J. (2018). Colonocyte metabolism shapes the gut microbiota. Science 362 (6418):eaat9076. doi: 10.1126/science.aat9076
Liu, L., Dong, Y., Ye, M., Jin, S., Yang, J., Joosse, M. E., et al. (2017). The pathogenic role of NLRP3 inflammasome activation in inflammatory bowel diseases of both mice and humans. J. Crohns Colitis 11 (6), 737–750. doi: 10.1093/ecco-jcc/jjw219
Moroi, M., Uchi, S., Nakamura, K., Sato, S., Shimizu, N., Fujii, M., et al. (2011). Beneficial effect of a diet containing heat-killed lactobacillus paracasei K71 on adult type atopic dermatitis. J. Dermatol. 38 (2), 131–139. doi: 10.1111/j.1346-8138.2010.00939.x
Ng, S. C., Kaplan, G. G., Tang, W., Banerjee, R., Adigopula, B., Underwood, F. E., et al. (2019). Population density and risk of inflammatory bowel disease: A prospective population-based study in 13 countries or regions in Asia-pacific. Am. J. Gastroenterol. 114 (1), 107–115. doi: 10.1038/s41395-018-0233-2
Ng, S. C., Shi, H. Y., Hamidi, N., Underwood, F. E., Tang, W., Benchimol, E. I., et al. (2017). Worldwide incidence and prevalence of inflammatory bowel disease in the 21st century: a systematic review of population-based studies. Lancet 390 (10114), 2769–2778. doi: 10.1016/S0140-6736(17)32448-0
Oliva, S., Di Nardo, G., Ferrari, F., Mallardo, S., Rossi, P., Patrizi, G., et al. (2012). Randomised clinical trial: the effectiveness of lactobacillus reuteri ATCC 55730 rectal enema in children with active distal ulcerative colitis. Aliment Pharmacol. Ther. 35 (3), 327–334. doi: 10.1111/j.1365-2036.2011.04939.x
Perino, A., Demagny, H., Velazquez-Villegas, L., Schoonjans, K. (2021). Molecular physiology of bile acid signaling in health, disease, and aging. Physiol. Rev. 101 (2), 683–731. doi: 10.1152/physrev.00049.2019
Podolsky, D. K. (2002). Inflammatory bowel disease. N Engl. J. Med. 347 (6), 417–429. doi: 10.1056/NEJMra020831
Regmi, S., Pathak, S., Nepal, M. R., Shrestha, P., Park, J., Kim, J. O., et al. (2019). Inflammation-triggered local drug release ameliorates colitis by inhibiting dendritic cell migration and Th1/Th17 differentiation. J. Control Release 316, 138–149. doi: 10.1016/j.jconrel.2019.11.001
Rodríguez-Lago, I., Castro-Poceiro, J., Fernández-Clotet, A., Mesonero, F., López-Sanromán, A., López-García, A., et al. (2020). Tacrolimus induces short-term but not long-term clinical response in inflammatory bowel disease. Aliment Pharmacol. Ther. 51 (9), 870–879. doi: 10.1111/apt.15687
Sartor, R. B. (2004). Therapeutic manipulation of the enteric microflora in inflammatory bowel diseases: Antibiotics, probiotics, and prebiotics. Gastroenterology 126 (6), 1620–1633. doi: 10.1053/j.gastro.2004.03.024
Satake, M., Sakuraba, H., Hiraga, H., Yoshida, S., Akemoto, Y., Ota, S., et al. (2022). Tacrolimus up-regulates expression of TGFβ receptor type II via ERK, providing protection against intestinal epithelial injury. In Vivo 36 (4), 1684–1693. doi: 10.21873/invivo.12880
Shi, N., Li, N., Duan, X., Niu, H. (2017). Interaction between the gut microbiome and mucosal immune system. Mil Med. Res. 4, 14. doi: 10.1186/s40779-017-0122-9
Shin, N. R., Whon, T. W., Bae, J. W. (2015). Proteobacteria: Microbial signature of dysbiosis in gut microbiota. Trends Biotechnol. 33 (9), 496–503. doi: 10.1016/j.tibtech.2015.06.011
Sinha, S. R., Haileselassie, Y., Nguyen, L. P., Tropini, C., Wang, M., Becker, L. S., et al. (2020). Dysbiosis-induced secondary bile acid deficiency promotes intestinal inflammation. Cell Host Microbe 27 (4), 659–670.e655. doi: 10.1016/j.chom.2020.01.021
Sun, M., Liu, Y., Song, Y., Gao, Y., Zhao, F., Luo, Y., et al. (2020). The ameliorative effect of lactobacillus plantarum-12 on DSS-induced murine colitis. Food Funct. 11 (6), 5205–5222. doi: 10.1039/D0FO00007H
Thomas, J. P., Modos, D., Rushbrook, S. M., Powell, N., Korcsmaros, T. (2022). The emerging role of bile acids in the pathogenesis of inflammatory bowel disease. Front. Immunol. 13, 829525. doi: 10.3389/fimmu.2022.829525
Thomson, A. W., Bonham, C. A., Zeevi, A. (1995). Mode of action of tacrolimus (FK506): molecular and cellular mechanisms. Ther. Drug Monit 17 (6), 584–591. doi: 10.1097/00007691-199512000-00007
Toral, M., Romero, M., Rodríguez-Nogales, A., Jiménez, R., Robles-Vera, I., Algieri, F., et al. (2018). Lactobacillus fermentum improves tacrolimus-induced hypertension by restoring vascular redox state and improving eNOS coupling. Mol. Nutr. Food Res. 62 (14), e1800033. doi: 10.1002/mnfr.201800033
Tourret, J., Willing, B. P., Dion, S., MacPherson, J., Denamur, E., Finlay, B. B. (2017). Immunosuppressive treatment alters secretion of ileal antimicrobial peptides and gut microbiota, and favors subsequent colonization by uropathogenic escherichia coli. Transplantation 101 (1), 74–82. doi: 10.1097/TP.0000000000001492
Wahlström, A., Sayin, S. I., Marschall, H. U., Bäckhed, F. (2016). Intestinal crosstalk between bile acids and microbiota and its impact on host metabolism. Cell Metab. 24 (1), 41–50. doi: 10.1016/j.cmet.2016.05.005
Wang, M. X., Lin, L., Chen, Y. D., Zhong, Y. P., Lin, Y. X., Li, P., et al. (2020). Evodiamine has therapeutic efficacy in ulcerative colitis by increasing lactobacillus acidophilus levels and acetate production. Pharmacol. Res. 159, 104978. doi: 10.1016/j.phrs.2020.104978
Wong, W. Y., Chan, B. D., Sham, T. T., Lee, M. M., Chan, C. O., Chau, C. T., et al. (2022). Lactobacillus casei strain shirota ameliorates dextran sulfate sodium-induced colitis in mice by increasing taurine-conjugated bile acids and inhibiting NF-κB signaling via stabilization of IκBα. Front. Nutr. 9, 816836. doi: 10.3389/fnut.2022.816836
Wright, E. K., Ding, N. S., Niewiadomski, O. (2018). Management of inflammatory bowel disease. Med. J. Aust. 209 (7), 318–323. doi: 10.5694/mja17.01001
Wu, Y., Jha, R., Li, A., Liu, H., Zhang, Z., Zhang, C., et al. (2022). Probiotics (Lactobacillus plantarum HNU082) supplementation relieves ulcerative colitis by affecting intestinal barrier functions, immunity-related gene expression, gut microbiota, and metabolic pathways in mice. Microbiol. Spectr. 10 (6), e0165122. doi: 10.1128/spectrum.01651-22
Yang, M., Gu, Y., Li, L., Liu, T., Song, X., Sun, Y., et al. (2021). Bile acid-gut microbiota axis in inflammatory bowel disease: From bench to bedside. Nutrients 13 (9):3143. doi: 10.3390/nu13093143
Yoshino, T., Nakase, H., Honzawa, Y., Matsumura, K., Yamamoto, S., Takeda, Y., et al. (2010). Immunosuppressive effects of tacrolimus on macrophages ameliorate experimental colitis. Inflammation Bowel Dis. 16 (12), 2022–2033. doi: 10.1002/ibd.21318
Zhang, Z., Liu, L., Tang, H., Jiao, W., Zeng, S., Xu, Y., et al. (2018). Immunosuppressive effect of the gut microbiome altered by high-dose tacrolimus in mice. Am. J. Transplant. 18 (7), 1646–1656. doi: 10.1111/ajt.14661
Keywords: colitis, tacrolimus, gut microbiome, Lactobacillus plantarum, bile acid
Citation: Lv W, Zhang D, He T, Liu Y, Shao L, Lv Z, Pu X, Wang Y and Liu L (2023) Combination of Lactobacillus plantarum improves the effects of tacrolimus on colitis in a mouse model. Front. Cell. Infect. Microbiol. 13:1130820. doi: 10.3389/fcimb.2023.1130820
Received: 23 December 2022; Accepted: 06 February 2023;
Published: 13 March 2023.
Edited by:
Xiaoyan Wang, Department of Gastroenterology, Central South University, ChinaReviewed by:
Juan Francisco Burgueño, Independent Researcher, Gothenburg, SwedenRen Mao, The First Affiliated Hospital of Sun Yat-sen University, Guangzhou, China
Hailong Cao, Tianjin Medical University General Hospital, China
Copyright © 2023 Lv, Zhang, He, Liu, Shao, Lv, Pu, Wang and Liu. This is an open-access article distributed under the terms of the Creative Commons Attribution License (CC BY). The use, distribution or reproduction in other forums is permitted, provided the original author(s) and the copyright owner(s) are credited and that the original publication in this journal is cited, in accordance with accepted academic practice. No use, distribution or reproduction is permitted which does not comply with these terms.
*Correspondence: Yufang Wang, d2FuZ3l1ZmFuZzA0QDEyNi5jb20=; Ling Liu, bGluZ3ppcHVycGxlQDE2My5jb20=
†These authors have contributed equally to this work and share first authorship
‡ORCID: Ling Liu, orcid.org/0000-0001-6413-708X