- 1Graduate School of Beijing, University of Chinese Medicine, Beijing, China
- 2Department of Oncology, Dongfang Hospital, Beijing University of Chinese Medicine, Beijing, China
Postoperative delayed gastric emptying is a prevalent complication following surgical procedures, imposing heavy physical and financial burdens on patients. However, current treatment options remain suboptimal. In recent years, an increasing number of studies have highlighted that the gut microbiota and its metabolites are closely associated with postoperative complications. Various factors can disrupt the gut microbiome after surgery. This review discusses the potential mechanisms by which the gut microbiota and their metabolites may contribute to the pathogenesis of postoperative delayed gastric emptying. However, the current knowledge base is limited in terms of fully understanding the exact mechanisms involved. It is therefore evident that further research is required to fully elucidate the role of the gut microbiome in postoperative delayed gastric emptying, with the aim of uncovering new possibilities for preventive measures and therapeutic treatments.
1 Introduction
Postoperative delayed gastric emptying (DGE), also known as postoperative gastroparesis syndrome, is defined as delayed gastric emptying in the absence of mechanical obstruction, which is clinically manifested by nausea, vomiting, early satiety, postprandial fullness, and epigastric pain (Camilleri and Sanders, 2022; Camilleri et al., 2022). It is a common complication following gastrointestinal surgery (Eshuis et al., 2015; Takatsuki et al., 2021; Baia et al., 2022) and can also manifest after non-abdominal procedures, with varying incidence among different surgical specialties. Statistically, approximately 7%-59% of patients may experience DGE after pancreaticoduodenectomy (Parmar et al., 2013; Mirrielees et al., 2020; Li et al., 2021), while the estimated incidence after gastrectomy ranges from 6% to 23% (Zhu et al., 2018). Postoperative DGE can increase the length of stay (Smits et al., 2022), reduce the quality of life, increase the economic burden, and may have a negative impact on oncological outcomes (Futagawa et al., 2017; Dominguez et al., 2023). Restoring gastrointestinal function is crucial for rapid recovery in postoperative patients. Unfortunately, there is currently a lack of ideal clinical therapeutic options for postoperative DGE, emphasizing the desperate necessity for the development of safer and more efficient treatments.
The pathogenesis of postoperative DGE remains poorly deconvoluted. Currently, it is thought to be multifactorial, involving nerve damage, gastric smooth muscle lesions, interstitial cells of Cajal (ICCs) damage, and other unknown elements. Recently, there has been an emerging awareness that the gut microbiota and their metabolites may be significant conductors in postoperative recovery (Liu et al., 2023; Yu et al., 2023; Zhang et al., 2023). It has been observed that gastrointestinal procedures can change the composition and structure of the intestinal flora, leading to postoperative intestinal microbial dysbiosis (Guyton and Alverdy, 2017), which may contribute to the development of postoperative gastrointestinal complications (Li et al., 2020; Gibiino et al., 2022; Zheng et al., 2023; Zhang et al., 2024). An early study in 1967 showed that germ-free mice had significantly lower gastric emptying and intestinal transit rates compared to conventional mice, suggesting a significant relationship between intestinal flora and gastrointestinal motility (Abrams and Bishop, 1967; Waclawiková et al., 2022). In addition, differences in the structure of the intestinal flora between Wistar rats, which are characterized by gastroparesis, and SD rats have been observed (Dalziel et al., 2017). Moreover, it is reported that duodenal flora is associated with DGE (Shanahan et al., 2023). These results suggest that dysbiosis of the gut microbiome may be closely related to the pathogenesis of DGE (Mandarino et al., 2023). Previous literature investigated the mechanisms of intestinal flora and their metabolites in postoperative ileus (Ma et al., 2023). Although postoperative acute gastroparesis may be a part of the ileus syndrome, dysmotility may not manifest throughout the gastrointestinal tract due to the diversity between regions of the alimentary tract (Sanders et al., 2012). The present article focuses on the potential pathogenic mechanisms of intestinal flora in postoperative DGE, trying to inspire viable and rational therapeutic strategies.
2 Physiology of gastric emptying and general pathogenesis of postoperative delayed gastric emptying
Gastric emptying and its regulation involve a complex series of sequential processes that encompass the central nervous system (CNS), the autonomic nervous system (ANS), the enteric nervous system (ENS), smooth muscle cells, ICCs, and gastrointestinal hormones, among others (Liu et al., 2021b). Gastric motility is primarily regulated by extrinsic innervation from the sympathetic and parasympathetic nervous systems, with intrinsic innervation providing local control. Current understanding suggests that sympathetic nerves have a limited role in regulating gastrointestinal motility, while parasympathetic nerves (especially the vagus nerves) dominating excitatory effects on gastrointestinal motility. In addition, endocrine regulation, which is mainly achieved by gastrointestinal hormones, is another significant form of regulation (Sanger and Lee, 2008). These hormones, also known as brain-gut peptides, dominantly including gastrin, motilin, Ghrelin, cholecystokinin, vasoactive intestinal peptide, glucagon-like peptide-1 (GLP-1), etc. Among them, ghrelin and motilin agonists are being investigated as potential targets for the treatment of gastroparesis (Sanger and Furness, 2016). Any dysfunction of these components can result in gastric motility disorders.
The research dilemma in postoperative DGE is partly due to the unclear pathogenesis, necessitating further exploration. Current understanding suggests that DGE is a neuromuscular dysfunction disorder potentially linked to various factors, such as autonomic and enteric neuropathy, smooth muscle dysfunction, abnormal duodenal antral coordination, gastric dysrhythmia, gastrointestinal hormones imbalances, etc (Sullivan et al., 2020; Abell et al., 2021; Gilbert et al., 2023). Firstly, surgical procedures may lead to anatomic modifications that can cause regional abnormalities in the motility patterns of the fundus, body, antrum, and pylorus, ultimately resulting in DGE. Additionally, intraoperative damage to the vagus nerve is a significant factor in postoperative DGE (Ye et al., 2022). Also, surgical stress can lead to sympathetic activation and decreased parasympathetic excitability, further contributing to the slowing of gastric emptying (Nguyen et al., 2020). On the other hand, ENS is essential for coordinating gastrointestinal motility. Reduced density of intestinal neurons in the gastric mucosa has been demonstrated in patients with gastroparesis and in animal models (Kedar et al., 2011; Baker et al., 2020). Gastrointestinal hormones are involved in the regulation of gastrointestinal motility after surgery (Stengel and Taché, 2014). Research has shown that perioperative serum levels of gastrin, motilin, and substance P are positively associated with early flatulence, while negatively correlated with cholecystokinin and somatostatin levels (Chen et al., 2022). There is ample evidence that loss or structural abnormalities of ICCs are implicated in the pathogenesis of delayed gastric emptying (Grover et al., 2019; Liu et al., 2021a). In patients with gastroparesis, a reduction in the number of ICCs in biopsy tissue specimens from the jejunum, colon, and antrum has been reported (Chikkamenahalli et al., 2020). Besides, ischemic injury-induced cell damage is recognized as a mechanism underlying postoperative gastroparesis (Vélez and Kuo, 2021).
3 Various factors influence intestinal microbiota after surgery
Intestinal microbiota refers to the microorganisms that colonize and survive in the gastrointestinal tract in a complex and delicate balance, including trillions of bacteria, archaea, fungi, protozoa, and viruses, with bacteria being the most prevalent (D’Argenio and Salvatore, 2015). Intestinal bacteria consists of nine phyla, of which Bacillota and Bacteroidota are the dominant ones. Emerging delect studies have demonstrated that surgical interventions can significantly shift the abundance and function of the gut flora and their metabolites (Guyton and Alverdy, 2017; Shao et al., 2017; Koliarakis et al., 2020; Gibiino et al., 2022). In 2021, a systematic review encompassing 14 studies for the first time highlighted substantial alterations in the gut microbiome following surgical procedures (Ferrie et al., 2021). Subsequently, another meta-analysis, which included 33 gastrointestinal surgeries, further confirmed the alterations in gut microbiome composition following surgical procedures (Tarazi et al., 2022).
The alterations in the structure and function of the gut microbiota postsurgery are influenced by various factors, such as the type of operation and the methods of digestive tract reconstruction (Guyton and Alverdy, 2017; Yao et al., 2022; Tsigalou et al., 2023). For instance, patients with gastric cancer who underwent radical distal gastrectomy exhibited a notable rise in Escherichia/Shigella, Akkermansia, Dialister, and Prevotella, while a significant decline in bacteria such as Klebsiella, Streptococcus, Phascolarctobacterium, and Bifidobacterium after surgery compared to the preoperative period (Liang et al., 2019). Another study showed that the diversity and abundance of the gut microbiota in gastric cancer patients after gastrectomy were higher than in the normal control group, with elevated levels of oral microorganisms, aerobic bacteria, and facultative anaerobes (Erawijantari et al., 2020). Gastrectomy leads to a reduction in gastric acid secretion and damage to the gastric mucosal barrier, allowing oral bacteria to survive and eventually colonize the intestine (Maksimaityte et al., 2021). A pilot study found that the relative abundance of Pseudomonadota in colorectal cancer patients after surgery was significantly higher in comparison to pre-surgery levels. Additionally, the genus Klebsiella exhibited a higher proportion than that observed prior to surgery (Cong et al., 2018). A case-control study of patients with colorectal cancer revealed significant alterations in the gut microbiota following surgery, including a decrease in obligate anaerobes, an increase in pathogenic bacteria, and a reduction in short-chain fatty acids (Ohigashi et al., 2013). In a recent study, Fang and colleagues revealed a significant increase in the prevalence of Klebsiella and Lachnoclostridium in the intestinal microbiota of patients after pancreaticoduodenectomy (Fang et al., 2024). It is evident that there is no consensus regarding the impact of surgical procedures on the microbiological structure of the patient’s gut. Table 1 provides a summary of several studies on the changes in the gut microbiota in patients following different types of surgery.
For another, perioperative medications could be a significant cause of changes in the composition and abundance of the gut microbiota (Liufu et al., 2020). Surgical bowel preparations, such as intestinal mechanical preparations, and oral or intravenous antibiotics, can significantly impact the gut microbiota composition following surgery (Omar Al-Hassi et al., 2018; Nalluri-Butz et al., 2022). As evidenced by a 31-fold decrease in total microbial load after the bowel cleaning. However, this change was mostly recovered within a period of 14 days (Jalanka et al., 2015). Multiple studies have demonstrated that antibiotics, commonly prescribed in the perioperative period to prevent infections, are capable of disrupting the balance of the intestinal microflora by reducing the abundance and diversity of microbiota (Duan et al., 2022; Xue et al., 2023). Moreover, intraoperative fluid therapy regimens and postoperative application of proton pump inhibitors can also alter the perioperative gut flora characteristics of patients (Jackson et al., 2016; Lu et al., 2022). Interestingly, postoperative use of proton pump inhibitors (PPIs) has been linked to a significantly higher incidence of delayed gastric emptying after pancreaticoduodenectomy (Panni et al., 2023). But it is unclear to what extent the gut microbiota contributes to the adverse effects associated with PPIs use (Macke et al., 2020). Exposure to anesthetics can lead to significant and lasting changes in gut microbiome diversity (Serbanescu et al., 2019; Wang et al., 2019; Minerbi and Shen, 2022). Opioid medications are the mainstay for controlling perioperative pain. Treatment with opioids, such as morphine, has been shown to have selective effects on the gut microbiome, primarily leading to an increase in potentially harmful bacteria and a decrease in potentially beneficial bacteria (Taboun and Sadeghi, 2023).
Besides, intraoperative mechanical injury is another important factor that affects the intestinal flora. Gastric venous stasis can lead to gastric ischemia and impaired gastric emptying (Loos et al., 2022; Stoop et al., 2023). Preserving the correct gastric vasculature may help reduce gastric venous congestion and ischemia, thereby maintaining gastric motility (Marchegiani et al., 2023). Intraoperative ischemia, hypoxia, and ischemia-reperfusion injury can induce changes in the intestinal flora (Li et al., 2022). The intestinal mucosa is particularly sensitive to ischemia and hypoxia. Tian et al. observed that systemic hypovolemia-induced intestinal ischemia-reperfusion injury disturbed the intestinal microbiome in mice, resulting in a decrease in Bacteroidota and an increase in Pseudomonadota (Tian et al., 2016). Collectively, a growing body of evidence has demonstrated that manifold confounders during the perioperative period may contribute to the changes of the gut microbiota (Figure 1). However, these changes are quite heterogeneous across different studies.
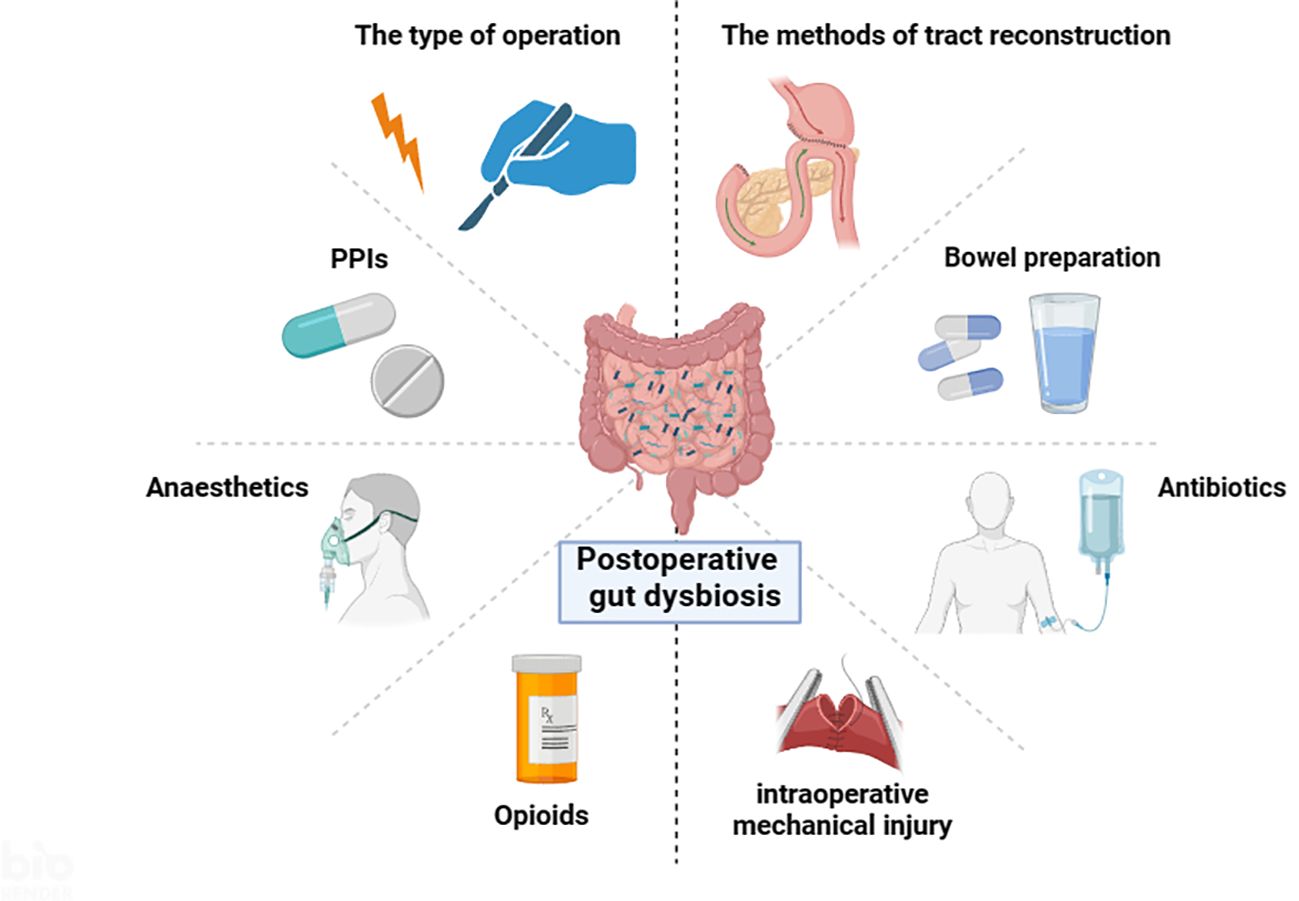
Figure 1. Multiple factors influence the intestinal microbiota during the perioperative period (By BioRender.com).
4 Potential abnormal gastrointestinal microbiota associated with delayed gastric emptying
In recent years, clinical and fundamental scientific studies have highlighted the role of gut microbiota and their metabolites in postoperative complications (Schmitt et al., 2019; Lederer et al., 2021). For instance, in a prospective cohort study conducted by Shogan and colleagues, which included 101 patients undergoing colorectal surgery, analysis of fecal samples collected on the day of surgery and postoperative day 2 showed an increase in the abundance of Bacteroidota, Parabacteroides, and Ruminococcus in patients who developed postoperative intestinal obstruction (Shogan et al., 2020). This suggests that alterations in the intestinal flora may be a contributing factor in the development of postoperative intestinal obstruction. Nevertheless, few studies have directly examined the gut microbiota changes in postoperative DGE to address the connection between postoperative DGE and gut flora characteristics. An analysis of the intestinal flora in 14 patients who underwent gastrectomy and Billroth II reconstruction for gastric cancer revealed a notable decrease in both α-diversity and β-diversity of the intestinal flora, and these changes in intestinal microorganisms were closely associated with gastrointestinal symptoms (Bausys et al., 2022). The duodenum has been identified as a crucial component in the pathogenesis of upper gastrointestinal diseases. Shanahan et al. conducted an analysis of the mucosa-associated flora through duodenal biopsy. They demonstrated a negative correlation between the relative abundance of Veillonella spp. and gastric emptying time (Shanahan et al., 2023). Lung transplant recipients with DGE have reduced microbial diversity in gastric fluid compared to those with normal gastric emptying (Lötstedt et al., 2021). These findings suggest that gut microorganisms may play a potential role in the initiation and progression of gastroparesis (Mandarino et al., 2023), which warrants further investigation. It is noteworthy that most current studies are based on samples of gut flora from feces, while the small bowel and mucosal microbes may need more focus.
5 Possible mechanisms of gut dysbiosis affecting postoperative gastric emptying
As mentioned above, surgical procedures can lead to profound microbial alterations. Because of the vital role of gut microbiota in host physiology, it is reasonable to hypothesize that the imbalance within the microbiota may interact with other pathophysiological mechanisms to contribute to the initiation, development, or exacerbation of postoperative DGE. Recent research has demonstrated that 12α-hydroxylated bile acids have the ability to regulate gastric emptying in mice (Higuchi et al., 2020). Nonetheless, the specific mechanisms linking these microbiota alterations to gastric motility in the postoperative period remain largely unknown. Several speculative mechanisms are proposed below (Figure 2).
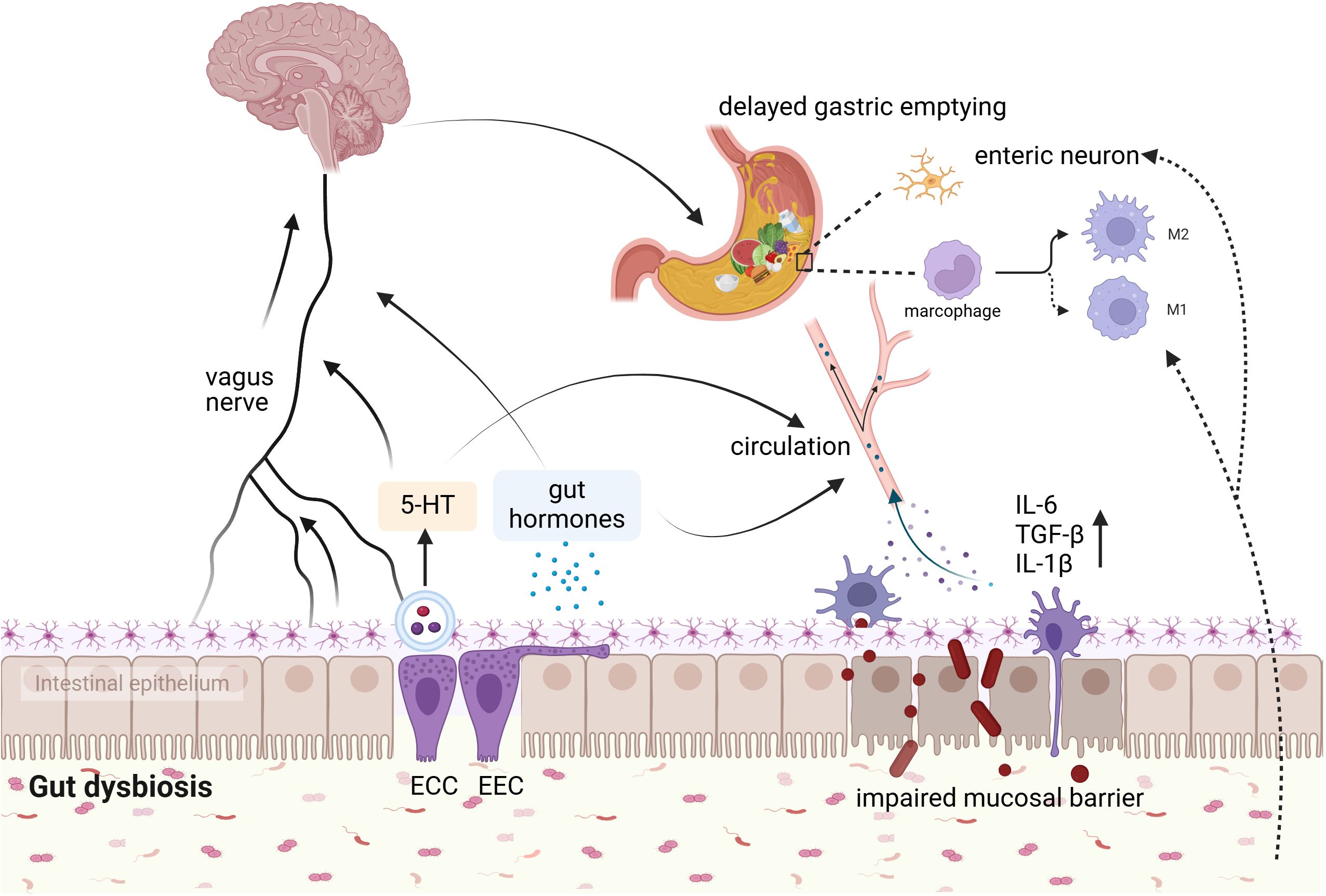
Figure 2. Potential mechanism of association between gut microbiota and postoperative DGE. The interaction between gut microbes and postoperative DGE may involve hormone secretion, 5-HT, gut mucosal barrier, enteric nerves systems, and muscularis macrophages. ECC, enterochromaffin cells; EEC, enteroendocrine cells (By BioRender.com).
5.1 Intestinal microbiota and gastrointestinal hormones, 5-hydroxytryptamine
Brain peptides play a crucial role in the treatment of gastrointestinal motility disorders (Van den Houte et al., 2020; Mori et al., 2022). Abnormal levels of gastrointestinal hormones are one of the underlying mechanisms of postoperative gastrointestinal dysfunction (Wen et al., 2016), underscoring the importance of targeting these peptides for treatment. Falkén Y et al. demonstrated that a 3-hour intravenous infusion of ghrelin can accelerate gastric emptying rates in patients who have undergone colorectal surgery (Falkén et al., 2013).
Available evidence suggests that gut flora and their metabolites can modulate the release of gut hormones in many different ways (Neuman et al., 2015; Fukui et al., 2018; Sun et al., 2020), and the most well-studied are GLP-1 and peptide YY(PYY). Research has shown that serum levels of GLP-1 are significantly elevated in rats with gut microbiota dysbiosis induced by broad-spectrum antibiotics (Lai et al., 2024). GLP-1 and PYY are both predominantly produced by intestinal enteroendocrine L-cells, slow stomach emptying (Mori et al., 2022). Studies have shown that the microbiota can modulate the number and intestinal expression of the enteroendocrine L-cells population, thus regulating the secretion of GLP and PYY (Greiner and Bäckhed, 2016; Covasa et al., 2019). Further, research shows that Enterococcus faecalis Metalloprotease GelE is able to degrade GLP-1, which enhances insights into the specific host-microbiome intricate interactions (LeValley et al., 2020). Ghrelin stimulates the gastric emptying rate (Murray et al., 2005; Levin et al., 2006). A number of microbes and metabolites have been found seems to be positively or negatively associated with Ghrelin circulating levels (Schalla and Stengel, 2020). In addition to Ghrelin secretion, the intestinal microbiota can also affect Ghrelin signaling processes. It has been documented that the gut microbiome impacts growth hormone-releasing peptidergic signaling by influencing metabolites at the Ghrelin receptor level (Schalla and Stengel, 2020; Leeuwendaal et al., 2021). Research has shown that microbial metabolites such as butyrate, propionate, acetate, and lactate, as well as bacterial strain supernatants, influence the activation of a complex signal transduction cascade by growth hormone secretagogue receptor-1a in response to Ghrelin (Torres-Fuentes et al., 2019).
5-hydroxytryptamine (5-HT), also known as serotonin, plays a crucial role as a gastrointestinal neurotransmitter in regulating gastrointestinal motility. Agonists of the 5-HT3, 5-HT4, and 5-HT4 receptor have shown effectiveness in relieving symptoms in patients with gastroparesis, making them a significant pharmacological target of this condition (Abell et al., 2023; Naing et al., 2023). Approximately 90% of 5-HT in humans is synthesized by enterochromaffin cells (Banskota et al., 2019). In enterochromaffin cell-specific Tph1CreERT2/+ transgenic mouse, which enterochromaffin cells were depleted, showed a reduction in 5-HT levels, thus leading to delayed gastric emptying. Furthermore, patients with idiopathic gastroparesis demonstrated reduced 5-HT in gastric sinus EC cells and serum, which was inversely associated with delayed gastric emptying (Wei et al., 2021). This study confirms the significant role of 5-HT in the pathogenesis of gastroparesis for the first time (Wei et al., 2021). The gut microbiota and its metabolites can influence 5-HT synthesis and secretion directly or indirectly (Reigstad et al., 2015; Bhattarai et al., 2017; Yang et al., 2017). Clostridium butyricum could upregulate 5-HT levels in plasma, intestine by stimulating 5-HT secretion (Mandić et al., 2019). Bifidobacterium dentis increases acetate concentration in the mouse intestine, stimulates 5-HT secretion from enterochromaffin cells, and upregulates the expression of intestinal 5-HT receptors and 5-HT transporter proteins (Bonaz et al., 2018).
5.2 Intestinal microbiota and the central nervous system, enteric nervous system
It is well known that gastric motility is considerably innervated by the extrinsic neural signals originating from the dorsal vagal complex in the brainstem (Browning and Travagli, 2014). The pacemaker neurons in the dorsal motor nucleus of the vagus nerve are influenced by inputs from the nearby nucleus tractus solitarius and higher centers, including projections from the paraventricular nucleus of the hypothalamus (Wang et al., 2023). Studies suggest that disruptions in the innervation between the brain and gut, through the vagus nerve or enteric nervous system, are common in individuals with gastroparesis (Maksimaityte et al., 2021). Animal experiments have shown that abdominal surgery can trigger the release of corticotropin-releasing factor (CRF), which in turn slows gastric emptying. And the paraventricular nucleus of the hypothalamus and the dorsal vagal complex are key areas that respond to CRF-induced delays in gastric emptying (Wang et al., 2011).
The bidirectional intercommunication between the CNS, ANS, and ENS occurs through the brain-gut axis, with the vagus nerves and brain-gut peptides playing crucial roles in maintaining its normal function, while the gut microbiota acts as key mediators of brain-gut interactions, closely influencing gastrointestinal motility regulation. The gut microbiota regulate CNS activities through neural, immune, and endocrine pathways (Cryan et al., 2019). On the one hand, certain metabolites produced by gut microbes can enter the bloodstream and travel to the associated brain regions to influence CNS functioning and actions (Sharon et al., 2016). Additionally, it has been suggested that the afferent branch of the vagus nerve can detect microbial signals that manifest as bacterial metabolites (Margolis et al., 2021). These pieces of information are transmitted upward via afferent nerves to the CNS, which receives and integrates a range of relevant information from both internal and external environments. Subsequently, the CNS sends regulatory signals through the autonomic nerve and neuroendocrine system to the gastrointestinal plexus or directly to target cells within the gastrointestinal tract (Bonaz et al., 2018; Wang et al., 2020). Surprisingly, latest research demonstrated that hypothalamic neurons can detect changes in gut microbiota structure (Gabanyi et al., 2022). Together, multiple potential pathways exit, both direct and indirect, through which altered gut microbiota can modulate the vague nerve signals (Fülling et al., 2019). However, there is no relevant research has been performed to explore whether the changes in microbiota influence the brain actions involved in gastric emptying.
Normal enteric nerve fibers play a role in gastric emptying through motor pathways that involve inhibitory and excitatory neurotransmitters. Study have shown that transgenic mice with disrupted intestinal glial cells exhibit delayed gastric emptying compared to normal mice (P<0.05) (Aubé et al., 2006). Evidence suggests that gut microbes are essential for promoting the survival and maintenance of enteric neurons. In germ free mice, there was a reduction in both nitrergic and total neurons in the distal ileum compared to control mice (Anitha et al., 2012). Nitric oxide released by enteric nerves plays a key role in regulating gastric emptying by controlling pyloric sphincter relaxation (Idrizaj et al., 2021). Physiologically, gastric emptying requires functional GLP-1 receptors and intestinal neuronal nitric oxide synthase, which need to function in an intestinal environment with beneficial microflora (Grasset et al., 2017). Gut microbiota dysbiosis can reduce GLP-1R and neuronal nitric oxide synthase expression in the ENS, leading to inhibited gastric emptying by blocking GLP-1-induced nitric oxide production through a pattern recognition receptor-dependent mechanism (Yamane and Inagaki, 2018).
5.3 Intestinal microbiota and the intestinal mucosal barrier
The intestinal mucosal barrier is comprised of biological, mechanical, immune, and chemical barriers (Yang et al., 2021). Normally, the intestinal mucosal barrier is able to segregate the intestinal luminal contents, prevent the invasion of pathogenic antigens into the submucosal tissues and circulatory system. Notably, a present clinical study reported that patients with DGE have higher serum levels of pro-inflammatory cytokines, such as interleukin-1β, and tumor necrosis factor α, in conjunction with damaged intestinal mucosal barrier integrity, compared to patients with normal gastric emptying (Greis et al., 2017). Martinez et al. found a decrease in zonulin, a protein considered a marker of the integrity of the intestinal mucosal barrier, was associated with DGE in surgical critically ill pediatric patients (Martinez et al., 2020). Moreover, a recent study reported a novel regulatory mechanism of gastric emptying in humans, that the increasing concentrations of interleukin-6 delays gastric emptying in human (Lehrskov et al., 2018).
The intestinal microbiota acts as a biological barrier to the intestinal mucosa. Abnormal intestinal flora can impair the structure and function of the intestinal mucosal barrier through a variety of mechanisms, such as regulating the intestinal mucus barrier function, and the expression of tight junction proteins (Allam-Ndoul et al., 2020; Paone and Cani, 2020). Besides, it is reported that a decrease in short-chain fatty acids can result in impaired intestinal mucosal barrier and bacterial translocation (Schreiber et al., 2024). Toll-like receptors are a group of pattern-recognition receptors that detect microbial components and trigger the production of inflammatory cytokines. The composition of the intestinal flora can affect the function of the intestinal mucosal barrier by altering the toll-like receptors signaling pathway. An animal study reported that surgery can shift gut microbiological composition, and these microbiological changes were shown to exacerbate the disruption of the intestinal barrier in aged mice (Pan et al., 2023). Increased intestinal permeability allows the translocation of toxic metabolites, pathogens, or other antigens into the bloodstream and thus increase circulating levels of molecules that contribute to the activation of inflammatory pathways and the release of pro-inflammatory cytokines, such as interleukin-6, tumor necrosis factor α, etc (König et al., 2016). It has been reported that some pro-inflammatory cytokines or mediators may decrease gastrointestinal motility (Docsa et al., 2022). Okdahl et al. found that prolonged gastric emptying in individuals with diabetes was associated with elevated serum levels of interleukin-8, and the subjective cardinal symptoms of gastroparesis were negatively correlated with interleukin-6 levels (Okdahl et al., 2023).
5.4 Intestinal microbiota and macrophages
Muscularis macrophages carry out vital functions in the regulation of gastrointestinal motility (Muller et al., 2014; Chikkamenahalli et al., 2024), which can be classified as pro-inflammatory M1 types and anti-inflammatory M2 types. Studies have established that the quantity and phenotype of macrophages present in the gastrointestinal tract are important factors in the development of gastroparesis (Cipriani et al., 2016, 2018). Specifically, Grover et al. found a heightened immunoreactivity of CD68 in patients suffering from gastroparesis through full thickness biopsies, indicating an increased presence of M1 macrophages in these patients (Grover et al., 2011). Furthermore, Grover and colleagues noted a deficiency of CD206, a marker for M2 type macrophages, in patients with diabetic gastroparesis and idiopathic gastroparesis (Grover et al., 2017). Abdominal surgery activates M1 type macrophages in the gastrointestinal plexus of rats and increases the expression of pro-inflammatory cytokines; additionally, gastric emptying is significantly and negatively correlated with MHCII/CD206+- (M1) macrophages in the gastrointestinal plexus (Yuan and Taché, 2017).
Several studies have identified that the intestinal microbiota regulates gastrointestinal peristalsis partly through influencing the interactions between muscularis macrophages and enteric neurons (Muller et al., 2014). Becker et al. demonstrated that alterations in gut microbiota contribute to changes in macrophage phenotype (Becker et al., 2018). Yang et al. conducted a study to investigate the impact of gut microbiota on the relationship between gastrointestinal motility, 5-HT expression, and macrophage abundance. They found a notable rise in the numbers of muscularis MR-positive macrophages in the upper gastrointestinal and colon of germ-free mice that received fecal transplantation compared with germ-free mice without transplantation (Yang et al., 2017).
Although surgery may directly cause impaired gastric motility through factors such as vagal injury, anatomical changes, etc., it should not be excluded that DGE could also be partially affected by microbiome alterations resulting from surgery. While intriguing, it should be pointed out that this highly theoretical hypothetical scenario nevertheless warrants further clinical and experimental studies to either support or invalidate it of action.
6 Possible therapeutic strategies based on microbiological modulation of postoperative gastric motility
Current therapeutic options for managing gut dysbiosis include probiotics, prebiotics, synbiotics, dietary interventions, fecal microbiota transplantation (FMT), and others. However, research on their application in DGE is limited. Several studies, as discussed below, have shown the efficacy of this approach in improving digestive clinical symptoms (Table 2).
In recent years, probiotics have gained attention for their role in postoperative recovery (Krezalek and Alverdy, 2016; Kotzampassi, 2022; Xiong et al., 2024). Evidence suggests that oral administration of Clostridium butyrate after gastric cancer surgery can help restore postoperative intestinal flora, improve inflammation and immunity, and thus reduce postoperative complications (Cao et al., 2022). A clinical trial was conducted to investigate the effect of probiotic compounds on postoperative recovery in patients with distal gastric cancer. The patients were randomly assigned to a probiotic group or a placebo group. The results showed that patients in the probiotic group exhibited an earlier recovery of gastrointestinal function than those in the placebo group (Xiong et al., 2024). Furthermore, a meta-analysis supported the benefits of perioperative probiotic or synbiotic supplementation in facilitating gastrointestinal recovery in postoperative patients with gastrointestinal malignancies. The supplementation was linked to a reduction in the number of days to the first solid and liquid diet, a decrease in postoperative hospital stay, and a lower occurrence of postoperative abdominal distension and intestinal obstruction (Tang et al., 2022). In patients with colorectal cancer undergoing colorectomy, the perioperative probiotic treatment benefits the fecal microbiota, improves the integrity of the gut mucosal barrier, and shortens the first defecation, but there are no statistically significant differences in the fluid and solid intake times (Liu et al., 2011). A recent study observed that the administration of autoprobiotics in the early postoperative period can decrease dyspeptic complaints after surgery for colorectal cancer (Ermolenko et al., 2024). This may involve positive alterations in the gut microbiota.
These findings suggest that probiotics regulating the intestinal flora balance appear to be a potentially promising therapeutic approach or an add-on therapy to an approved approach for postoperative DGE. However, future large-scale trials are necessary to verify the effectiveness of restoring gut flora imbalance in improving postoperative gastrointestinal dysfunction.
Furthermore, recent research has indicated the possibility of dietary interventions to restore the balance of the gut microbiota in disease. It has been proposed that modifying the gut microbiota through dietary interventions in patients with colorectal cancer may be an effective approach to improving perioperative dysbiosis and postoperative outcomes (Martínez-Montoro et al., 2022).
FMT, a method to reconstruct gut microbiota that involves the transfer of fecal material from a healthy donor to another individual to treat disease, shows a promise in intervening in gastrointestinal motility disorders (Antushevich, 2020). However, the application of FMT should be approached with caution and a comprehensive understanding of its potential benefits and risks.
7 Prospects
The postoperative restoration of gastrointestinal motility is a key issue in facilitating rapid perioperative recovery. It is possible that dysbiosis of the gut flora may contribute to the development of DGE. However, this field is still at the exploratory stage, and it has not yet been demonstrated whether these changes are responsible for the postoperative DGE outcomes and, if so, to what extent. It would be beneficial for future studies to focus on investigating the microbiota changes associated with postoperative impaired gastric motility in large patient cohorts. Furthermore, it would be advantageous to validate these findings through fecal transplantation experiments to determine the causal relationship. This would help identify specific bacterial taxa that are associated with postoperative gastroparesis. Additionally, mucosa-associated microbiomes seem to exert a more pathogenic influence compared to fecal microbes. A comprehensive understanding of the interactions between microbiota and DGE may be helpful in designing novel non-invasive diagnostic tools utilizing DGE-specific microbiota profiles and metabolites, as well as strategies for the management and prevention of this gastrointestinal disorder. Currently, evidence suggests that preoperative gut flora may serve as a predictive factor for postoperative intestinal paralysis (Jin et al., 2020), inspiring us to investigate the predictive role of altered preoperative gut flora in DGE.
Author contributions
ZW: Conceptualization, Investigation, Writing – original draft. CL: Investigation, Writing – original draft. KH: Investigation, Writing – review & editing. MZ: Investigation, Writing – original draft. ZT: Investigation, Writing – original draft. YW: Investigation, Writing – original draft. QZ: Conceptualization, Funding acquisition, Writing – review & editing. QL: Conceptualization, Writing – review & editing.
Funding
The author(s) declare financial support was received for the research, authorship, and/or publication of this article. This work was supported by the National Natural Science Foundation of China (No.82204855).
Acknowledgments
The authors thank the BioRender (biorender.com) for figures artwork.
Conflict of interest
The authors declare that the research was conducted in the absence of any commercial or financial relationships that could be construed as a potential conflict of interest.
Publisher’s note
All claims expressed in this article are solely those of the authors and do not necessarily represent those of their affiliated organizations, or those of the publisher, the editors and the reviewers. Any product that may be evaluated in this article, or claim that may be made by its manufacturer, is not guaranteed or endorsed by the publisher.
Abbreviations
DGE, delayed gastric emptying; ICCs, interstitial cells of Cajal; CNS, central nervous system; ANS, autonomic nervous system; ENS, enteric nervous system; PPIs, proton pump inhibitors; GLP-1, glucagon-like peptide-1; PYY, peptide YY; 5-HT, 5-hydroxytryptamine; CRF, corticotropin-releasing factor; FMT, fecal microbiota transplantation.
References
Abell, T. L., Kedar, A., Stocker, A., Beatty, K., McElmurray, L., Hughes, M., et al. (2021). Pathophysiology of gastroparesis syndromes includes anatomic and physiologic abnormalities. Dig Dis. Sci. 66, 1127–1141. doi: 10.1007/s10620-020-06259-6
Abell, T. L., Kuo, B., Esfandyari, T., Pfeifer, N. D., Grimaldi, M., Renzulli, C., et al. (2023). A randomized, double-blind, placebo-controlled, phase 2b study of the efficacy and safety of velusetrag in subjects with diabetic or idiopathic gastroparesis. Neurogastroenterol. Motil. 35, e14523. doi: 10.1111/nmo.14523
Abrams, G. D., Bishop, J. E. (1967). Effect of the normal microbial flora on gastrointestinal motility. Proc. Soc. Exp. Biol. Med. 126, 301–304. doi: 10.3181/00379727-126-32430
Allam-Ndoul, B., Castonguay-Paradis, S., Veilleux, A. (2020). Gut microbiota and intestinal trans-epithelial permeability. Int. J. Mol. Sci. 21, 6402. doi: 10.3390/ijms21176402
Anitha, M., Vijay-Kumar, M., Sitaraman, S. V., Gewirtz, A. T., Srinivasan, S. (2012). Gut microbial products regulate murine gastrointestinal motility via Toll-like Receptor 4 signaling. Gastroenterology 143, 1006–1016.e4. doi: 10.1053/j.gastro.2012.06.034
Antushevich, H. (2020). Fecal microbiota transplantation in disease therapy. Clinica Chimica Acta 503, 90–98. doi: 10.1016/j.cca.2019.12.010
Aubé, A., Cabarrocas, J., Bauer, J., Philippe, D., Aubert, P., Doulay, F., et al. (2006). Changes in enteric neurone phenotype and intestinal functions in a transgenic mouse model of enteric glia disruption. Gut 55, 630–637. doi: 10.1136/gut.2005.067595
Baia, M., Conti, L., Pasquali, S., Sarre-Lazcano, C., Abatini, C., Cioffi, S. P. B., et al. (2022). Delayed gastric emptying after multivisceral resection for retroperitoneal sarcoma. Ann. Surg. Oncol. 29, 3264–3270. doi: 10.1245/s10434-021-11154-z
Baker, C., Ahmed, M., Cheng, K., Arciero, E., Bhave, S., Ho, W. L. N., et al. (2020). Hypoganglionosis in the gastric antrum causes delayed gastric emptying. Neurogastroenterol. Motil. 32, e13766. doi: 10.1111/nmo.13766
Banskota, S., Ghia, J.-E., Khan, W. I. (2019). Serotonin in the gut: Blessing or a curse. Biochimie 161, 56–64. doi: 10.1016/j.biochi.2018.06.008
Bausys, A., Horvath, A., Sabaliauskaite, R., Jarmalaite, S., Schuetz, B., Stiegler, P., et al. (2022). Intestinal dysbiosis after subtotal gastrectomy with Billroth II anastomosis for gastric cancer. Eur. J. Surg. Oncol. 48, e172–e173. doi: 10.1016/j.ejso.2021.12.357
Becker, L., Spear, E. T., Sinha, S. R., Haileselassie, Y., Habtezion, A. (2018). Age-related changes in gut microbiota alter phenotype of muscularis macrophages and disrupt gastrointestinal motility. Cell Mol. Gastroenterol. Hepatol. 7, 243–245.e2. doi: 10.1016/j.jcmgh.2018.09.001
Bhattarai, Y., Schmidt, B. A., Linden, D. R., Larson, E. D., Grover, M., Beyder, A., et al. (2017). Human-derived gut microbiota modulates colonic secretion in mice by regulating 5-HT3 receptor expression via acetate production. Am. J. Physiol. Gastrointest Liver Physiol. 313, G80–G87. doi: 10.1152/ajpgi.00448.2016
Bonaz, B., Bazin, T., Pellissier, S. (2018). The vagus nerve at the interface of the microbiota-gut-brain axis. Front. Neurosci. 12. doi: 10.3389/fnins.2018.00049
Browning, K. N., Travagli, R. A. (2014). Central nervous system control of gastrointestinal motility and secretion and modulation of gastrointestinal functions. Compr. Physiol. 4, 1339–1368. doi: 10.1002/cphy.c130055
Camilleri, M., Kuo, B., Nguyen, L., Vaughn, V. M., Petrey, J., Greer, K., et al. (2022). ACG clinical guideline: gastroparesis. Am. J. Gastroenterol. 117, 1197–1220. doi: 10.14309/ajg.0000000000001874
Camilleri, M., Sanders, K. M. (2022). Gastroparesis. Gastroenterology 162, 68–87.e1. doi: 10.1053/j.gastro.2021.10.028
Cao, W., Zheng, C., Xu, X., Jin, R., Huang, F., Shi, M., et al. (2022). Clostridium butyricum potentially improves inflammation and immunity through alteration of the microbiota and metabolism of gastric cancer patients after gastrectomy. Front. Immunol. 13. doi: 10.3389/fimmu.2022.1076245
Chen, X., Wang, P., Leng, C., Sun, H., Liu, X., Zhang, R., et al. (2022). Early oral feeding after esophagectomy accelerated gut function recovery by regulating brain-gut peptide secretion. Surgery 172, 919–925. doi: 10.1016/j.surg.2022.04.041
Chikkamenahalli, L. L., Jessen, E., Bernard, C. E., Ip, W. K. E., Breen-Lyles, M., Cipriani, G., et al. (2024). Single cell atlas of human gastric muscle immune cells and macrophage-driven changes in idiopathic gastroparesis. iScience 27, 108991. doi: 10.1016/j.isci.2024.108991
Chikkamenahalli, L. L., Pasricha, P. J., Farrugia, G., Grover, M. (2020). Gastric Biopsies in Gastroparesis: Insights into Gastric Neuromuscular Disorders to aid treatment. Gastroenterol. Clin. North Am. 49, 557–570. doi: 10.1016/j.gtc.2020.04.009
Cipriani, G., Gibbons, S. J., Kashyap, P. C., Farrugia, G. (2016). Intrinsic gastrointestinal macrophages: their phenotype and role in gastrointestinal motility. Cell Mol. Gastroenterol. Hepatol. 2, 120–130.e1. doi: 10.1016/j.jcmgh.2016.01.003
Cipriani, G., Gibbons, S. J., Miller, K. E., Yang, D. S., Terhaar, M. L., Eisenman, S. T., et al. (2018). Change in populations of macrophages promotes development of delayed gastric emptying in mice. Gastroenterology 154, 2122–2136.e12. doi: 10.1053/j.gastro.2018.02.027
Cong, J., Zhu, H., Liu, D., Li, T., Zhang, C., Zhu, J., et al. (2018). A pilot study: changes of gut microbiota in post-surgery colorectal cancer patients. Front. Microbiol. 9. doi: 10.3389/fmicb.2018.02777
Covasa, M., Stephens, R. W., Toderean, R., Cobuz, C. (2019). Intestinal sensing by gut microbiota: targeting gut peptides. Front. Endocrinol. 10. doi: 10.3389/fendo.2019.00082
Cryan, J. F., O’Riordan, K. J., Cowan, C. S. M., Sandhu, K. V., Bastiaanssen, T. F. S., Boehme, M., et al. (2019). The microbiota-gut-brain axis. Physiol. Rev. 99, 1877–2013. doi: 10.1152/physrev.00018.2018
D’Argenio, V., Salvatore, F. (2015). The role of the gut microbiome in the healthy adult status. Clinica Chimica Acta 451, 97–102. doi: 10.1016/j.cca.2015.01.003
Dalziel, J. E., Fraser, K., Young, W., McKenzie, C. M., Bassett, S. A., Roy, N. C. (2017). Gastroparesis and lipid metabolism-associated dysbiosis in Wistar-Kyoto rats. Am. J. Physiol. Gastrointest Liver Physiol. 313, G62–G72. doi: 10.1152/ajpgi.00008.2017
Docsa, T., Sipos, A., Cox, C. S., Uray, K. (2022). The role of inflammatory mediators in the development of gastrointestinal motility disorders. Int. J. Mol. Sci. 23, 6917. doi: 10.3390/ijms23136917
Dominguez, O. H., Grigorian, A., Wolf, R. F., Imagawa, D. K., Nahmias, J. T., Jutric, Z. (2023). Delayed gastric emptying is associated with increased risk of mortality in patients undergoing pancreaticoduodenectomy for pancreatic adenocarcinoma. Updates Surg. 75, 523–530. doi: 10.1007/s13304-022-01404-4
Duan, H., Yu, L., Tian, F., Zhai, Q., Fan, L., Chen, W. (2022). Antibiotic-induced gut dysbiosis and barrier disruption and the potential protective strategies. Crit. Rev. Food Sci. Nutr. 62, 1427–1452. doi: 10.1080/10408398.2020.1843396
Erawijantari, P. P., Mizutani, S., Shiroma, H., Shiba, S., Nakajima, T., Sakamoto, T., et al. (2020). Influence of gastrectomy for gastric cancer treatment on faecal microbiome and metabolome profiles. Gut 69, 1404–1415. doi: 10.1136/gutjnl-2019-319188
Ermolenko, E., Baryshnikova, N., Alekhina, G., Zakharenko, A., Ten, O., Kashchenko, V., et al. (2024). Autoprobiotics in the treatment of patients with colorectal cancer in the early postoperative period. Microorganisms 12, 980. doi: 10.3390/microorganisms12050980
Eshuis, W. J., de Bree, K., Sprangers, M. A. G., Bennink, R. J., van Gulik, T. M., Busch, O. R. C., et al. (2015). Gastric emptying and quality of life after pancreatoduodenectomy with retrocolic or antecolic gastroenteric anastomosis. Br. J. Surg. 102, 1123–1132. doi: 10.1002/bjs.9812
Falkén, Y., Webb, D.-L., Abraham-Nordling, M., Kressner, U., Hellström, P. M., Näslund, E. (2013). Intravenous ghrelin accelerates postoperative gastric emptying and time to first bowel movement in humans. Neurogastroenterol Motil. 25, 474–480. doi: 10.1111/nmo.12098
Fang, J., Xiao, C., Qi, Y., Hong, W., Wang, M. (2024). Influence of pancreaticoduodenectomy for periampullary carcinoma on intestinal microbiome and metabolites. Heliyon 10, e24393. doi: 10.1016/j.heliyon.2024.e24393
Ferrie, S., Webster, A., Wu, B., Tan, C., Carey, S. (2021). Gastrointestinal surgery and the gut microbiome: a systematic literature review. Eur. J. Clin. Nutr. 75, 12–25. doi: 10.1038/s41430-020-0681-9
Fukui, H., Xu, X., Miwa, H. (2018). Role of gut microbiota-gut hormone axis in the pathophysiology of functional gastrointestinal disorders. J. Neurogastroenterol. Motil. 24, 367–386. doi: 10.5056/jnm18071
Fülling, C., Dinan, T. G., Cryan, J. F. (2019). Gut microbe to brain signaling: what happens in vagus. Neuron 101, 998–1002. doi: 10.1016/j.neuron.2019.02.008
Futagawa, Y., Kanehira, M., Furukawa, K., Kitamura, H., Yoshida, S., Usuba, T., et al. (2017). Impact of delayed gastric emptying after pancreaticoduodenectomy on survival. J. Hepato-Biliary-Pancreatic Sci. 24, 466–474. doi: 10.1002/jhbp.482
Gabanyi, I., Lepousez, G., Wheeler, R., Vieites-Prado, A., Nissant, A., Chevalier, G., et al. (2022). Bacterial sensing via neuronal Nod2 regulates appetite and body temperature. Science 376, eabj3986. doi: 10.1126/science.abj3986
Gibiino, G., Binda, C., Cristofaro, L., Sbrancia, M., Coluccio, C., Petraroli, C., et al. (2022). Dysbiosis and gastrointestinal surgery: current insights and future research. Biomedicines 10, 2532. doi: 10.3390/biomedicines10102532
Gilbert, R. J., Siamwala, J. H., Kumar, V., Thompson, C. C., Shikora, S. A. (2023). Reconsideration of the gastroparetic syndrome. Curr. Gastroenterol. Rep. 25, 75–90. doi: 10.1007/s11894-023-00865-w
Grasset, E., Puel, A., Charpentier, J., Collet, X., Christensen, J. E., Tercé, F., et al. (2017). A specific gut microbiota dysbiosis of type 2 diabetic mice induces GLP-1 resistance through an enteric NO-dependent and gut-brain axis mechanism. Cell Metab. 25, 1075–1090.e5. doi: 10.1016/j.cmet.2017.04.013
Greiner, T. U., Bäckhed, F. (2016). Microbial regulation of GLP-1 and L-cell biology. Mol. Metab. 5, 753–758. doi: 10.1016/j.molmet.2016.05.012
Greis, C., Rasuly, Z., Janosi, R. A., Kordelas, L., Beelen, D. W., Liebregts, T. (2017). Intestinal T lymphocyte homing is associated with gastric emptying and epithelial barrier function in critically ill: a prospective observational study. Crit. Care 21, 70. doi: 10.1186/s13054-017-1654-9
Grover, M., Bernard, C. E., Pasricha, P. J., Parkman, H. P., Gibbons, S. J., Tonascia, J., et al. (2017). Diabetic and idiopathic gastroparesis is associated with loss of CD206-positive macrophages in the gastric antrum. Neurogastroenterol. Motil. 29, e13018. doi: 10.1111/nmo.13018
Grover, M., Farrugia, G., Lurken, M. S., Bernard, C. E., Faussone-Pellegrini, M. S., Smyrk, T. C., et al. (2011). Cellular changes in diabetic and idiopathic gastroparesis. Gastroenterology 140, 1575–1585.e8. doi: 10.1053/j.gastro.2011.01.046
Grover, M., Farrugia, G., Stanghellini, V. (2019). Gastroparesis: A turning point in understanding and treatment. Gut 68, 2238–2250. doi: 10.1136/gutjnl-2019-318712
Guyton, K., Alverdy, J. C. (2017). The gut microbiota and gastrointestinal surgery. Nat. Rev. Gastroenterol. Hepatol. 14, 43–54. doi: 10.1038/nrgastro.2016.139
Higuchi, S., Ahmad, T. R., Argueta, D. A., Perez, P. A., Zhao, C., Schwartz, G. J., et al. (2020). Bile acid composition regulates GPR119-dependent intestinal lipid sensing and food intake regulation in mice. Gut 69, 1620–1628. doi: 10.1136/gutjnl-2019-319693
Idrizaj, E., Traini, C., Vannucchi, M. G., Baccari, M. C. (2021). Nitric oxide: from gastric motility to gastric dysmotility. Int. J. Mol. Sci. 22, 9990. doi: 10.3390/ijms22189990
Jackson, M. A., Goodrich, J. K., Maxan, M.-E., Freedberg, D. E., Abrams, J. A., Poole, A. C., et al. (2016). Proton pump inhibitors alter the composition of the gut microbiota. Gut 65, 749–756. doi: 10.1136/gutjnl-2015-310861
Jalanka, J., Salonen, A., Salojärvi, J., Ritari, J., Immonen, O., Marciani, L., et al. (2015). Effects of bowel cleansing on the intestinal microbiota. Gut 64, 1562–1568. doi: 10.1136/gutjnl-2014-307240
Jin, Y., Geng, R., Liu, Y., Liu, L., Jin, X., Zhao, F., et al. (2020). Prediction of postoperative ileus in patients with colorectal cancer by preoperative gut microbiota. Front. Oncol. 10. doi: 10.3389/fonc.2020.526009
Kedar, A., Nikitina, Y., Selim, M., Wendelschafer-Crabb, G., Taylor, M., Henry, O., et al. (2011). Enteric plexus inflammation and associated mucosal nerve fiber density in patients with gastroparesis: 112. Off. J. Am. Coll. Gastroenterol. 106, S45. doi: 10.14309/00000434-201110002-00112
Koliarakis, I., Athanasakis, E., Sgantzos, M., Mariolis-Sapsakos, T., Xynos, E., Chrysos, E., et al. (2020). Intestinal microbiota in colorectal cancer surgery. Cancers 12, 3011. doi: 10.3390/cancers12103011
König, J., Wells, J., Cani, P. D., García-Ródenas, C. L., MacDonald, T., Mercenier, A., et al. (2016). Human intestinal barrier function in health and disease. Clin. Trans. Gastroenterol. 7, e196. doi: 10.1038/ctg.2016.54
Kotzampassi, K. (2022). Why give my surgical patients probiotics. Nutrients 14, 4389. doi: 10.3390/nu14204389
Krezalek, M. A., Alverdy, J. C. (2016). The role of the microbiota in surgical recovery. Curr. Opin. Clin. Nutr. Metab. Care 19, 347. doi: 10.1097/MCO.0000000000000299
Lai, T.-T., Tsai, Y.-H., Liou, C.-W., Fan, C.-H., Hou, Y.-T., Yao, T.-H., et al. (2024). The gut microbiota modulate locomotion via vagus-dependent glucagon-like peptide-1 signaling. NPJ Biofilms Microbiomes 10, 2. doi: 10.1038/s41522-024-00477-w
Lederer, A.-K., Chikhladze, S., Kohnert, E., Huber, R., Müller, A. (2021). Current insights: the impact of gut microbiota on postoperative complications in visceral surgery—A narrative review. Diagnostics 11, 2099. doi: 10.3390/diagnostics11112099
Leeuwendaal, N. K., Cryan, J. F., Schellekens, H. (2021). Gut peptides and the microbiome: focus on ghrelin. Curr. Opin. Endocrinol. Diabetes Obes. 28, 243–252. doi: 10.1097/MED.0000000000000616
Lehrskov, L. L., Lyngbaek, M. P., Soederlund, L., Legaard, G. E., Ehses, J. A., Heywood, S. E., et al. (2018). Interleukin-6 delays gastric emptying in humans with direct effects on glycemic control. Cell Metab. 27, 1201–1211.e3. doi: 10.1016/j.cmet.2018.04.008
LeValley, S. L., Tomaro-Duchesneau, C., Britton, R. A. (2020). Degradation of the incretin hormone glucagon-like peptide-1 (GLP-1) by enterococcus faecalis metalloprotease gelE. mSphere 5, e00585–e00519. doi: 10.1128/mSphere.00585-19
Levin, F., Edholm, T., Schmidt, P. T., Grybäck, P., Jacobsson, H., Degerblad, M., et al. (2006). Ghrelin stimulates gastric emptying and hunger in normal-weight humans. J. Clin. Endocrinol. Metab. 91, 3296–3302. doi: 10.1210/jc.2005-2638
Li, X., Qin, T., Zhu, F., Wang, M., Dang, C., He, L., et al. (2021). Clinical efficacy of the preservation of the hepatic branch of the vagus nerve on delayed gastric emptying after laparoscopic pancreaticoduodenectomy. J. Gastrointest Surg. 25, 2172–2183. doi: 10.1007/s11605-021-05024-y
Li, Y., Wang, Y., Shi, F., Zhang, X., Zhang, Y., Bi, K., et al. (2022). Phospholipid metabolites of the gut microbiota promote hypoxia-induced intestinal injury via CD1d-dependent γδ T cells. Gut Microbes 14, 2096994. doi: 10.1080/19490976.2022.2096994
Li, G. Q., Zhang, T., Yang, W. G., Zhong, H.-L., Xiao, P., Liu, L.-W., et al. (2020). Gut microbiota patterns associated with somatostatin in patients undergoing pancreaticoduodenectomy: a prospective study. Cell Death Discovery 6, 1–14. doi: 10.1038/s41420-020-00329-4
Liang, W., Yang, Y., Wang, H., Wang, H., Yu, X., Lu, Y., et al. (2019). Gut microbiota shifts in patients with gastric cancer in perioperative period. Medicine 98, e16626. doi: 10.1097/MD.0000000000016626
Liu, B., Dong, J., Wang, S., Yu, H., Li, Z., Sun, P., et al. (2021a). Helicobacter pylori causes delayed gastric emptying by decreasing interstitial cells of Cajal. Exp. Ther. Med. 22, 1–7. doi: 10.3892/etm.2021.10095
Liu, W., Jin, Y., Wilde, P. J., Hou, Y., Wang, Y., Han, J. (2021b). Mechanisms, physiology, and recent research progress of gastric emptying. Crit. Rev. Food Sci. Nutr. 61, 2742–2755. doi: 10.1080/10408398.2020.1784841
Liu, Z., Li, N., Dang, Q., Liu, L., Wang, L., Li, H., et al. (2023). Exploring the roles of intestinal flora in enhanced recovery after surgery. iScience 26, 105959. doi: 10.1016/j.isci.2023.105959
Liu, Z., Qin, H., Yang, Z., Xia, Y., Liu, W., Yang, J., et al. (2011). Randomised clinical trial: the effects of perioperative probiotic treatment on barrier function and post-operative infectious complications in colorectal cancer surgery – a double-blind study. Alimentary Pharmacol. Ther. 33, 50–63. doi: 10.1111/j.1365-2036.2010.04492.x
Liufu, N., Liu, L., Shen, S., Jiang, Z., Dong, Y., Wang, Y., et al. (2020). Anesthesia and surgery induce age-dependent changes in behaviors and microbiota. Aging (Albany NY) 12, 1965–1986. doi: 10.18632/aging.v12i2
Loos, M., Mehrabi, A., Ramouz, A., Contin, P., Strobel, O., Müller-Stich, B. P., et al. (2022). Gastric venous congestion after total pancreatectomy is frequent and dangerous. Ann. Surg. 276, e896. doi: 10.1097/SLA.0000000000004847
Lötstedt, B., Boyer, D., Visner, G., Freiberger, D., Lurie, M., Kane, M., et al. (2021). The impact of gastrointestinal dysmotility on the aerodigestive microbiome of pediatric lung transplant recipients. J. Heart Lung Transplant. 40, 210–219. doi: 10.1016/j.healun.2020.11.013
Lu, X., Wang, Y., Luo, Y., Yu, B. (2022). Influence of different regimens of volumetric therapy on perioperative intestinal flora in the surgical patients with pancreas tumor, a randomized controlled trial study. BMC Anesthesiol 22, 162. doi: 10.1186/s12871-022-01693-7
Ma, T., Xue, X., Tian, H., Zhou, X., Wang, J., Zhao, Z., et al. (2023). Effect of the gut microbiota and their metabolites on postoperative intestinal motility and its underlying mechanisms. J. Transl. Med. 21, 349. doi: 10.1186/s12967-023-04215-2
Macke, L., Schulz, C., Koletzko, L., Malfertheiner, P. (2020). Systematic review: the effects of proton pump inhibitors on the microbiome of the digestive tract—evidence from next-generation sequencing studies. Alimentary Pharmacol. Ther. 51, 505–526. doi: 10.1111/apt.15604
Maksimaityte, V., Bausys, A., Kryzauskas, M., Luksta, M., Stundiene, I., Bickaite, K., et al. (2021). Gastrectomy impact on the gut microbiome in patients with gastric cancer: A comprehensive review. World J. Gastrointest Surg. 13, 678–688. doi: 10.4240/wjgs.v13.i7.678
Mandarino, F. V., Sinagra, E., Barchi, A., Verga, M. C., Brinch, D., Raimondo, D., et al. (2023). Gastroparesis: the complex interplay with microbiota and the role of exogenous infections in the pathogenesis of the disease. Microorganisms 11, 1122. doi: 10.3390/microorganisms11051122
Mandić, A. D., Woting, A., Jaenicke, T., Sander, A., Sabrowski, W., Rolle-Kampcyk, U., et al. (2019). Clostridium ramosum regulates enterochromaffin cell development and serotonin release. Sci. Rep. 9, 1177. doi: 10.1038/s41598-018-38018-z
Marchegiani, G., Di Gioia, A., Giuliani, T., Lovo, M., Vico, E., Cereda, M., et al. (2023). Delayed gastric emptying after pancreatoduodenectomy: One complication, two different entities. Surgery 173, 1240–1247. doi: 10.1016/j.surg.2022.12.013
Margolis, K. G., Cryan, J. F., Mayer, E. A. (2021). The microbiota-gut-brain axis: from motility to mood. Gastroenterology 160, 1486–1501. doi: 10.1053/j.gastro.2020.10.066
Martinez, E. E., Zurakowski, D., Pereira, L., Freire, R., Emans, J. B., Nurko, S., et al. (2020). Interleukin-10 and zonulin are associated with postoperative delayed gastric emptying in critically ill surgical pediatric patients: A prospective pilot study. J. Parenteral Enteral Nutr. 44, 1407–1416. doi: 10.1002/jpen.1874
Martínez-Montoro, J. I., Martínez-Sánchez, M. A., Balaguer-Román, A., Gil-Martínez, J., Mesa-López, M. J., Egea-Valenzuela, J., et al. (2022). Dietary modulation of gut microbiota in patients with colorectal cancer undergoing surgery: A review. Int. J. Surg. 104, 106751. doi: 10.1016/j.ijsu.2022.106751
Minerbi, A., Shen, S. (2022). Gut Microbiome in anesthesiology and pain medicine: a narrative review. Anesthesiology 137, 93–108. doi: 10.1097/ALN.0000000000004204
Mirrielees, J. A., Weber, S. M., Abbott, D. E., Greenberg, C. C., Minter, R. M., Scarborough, J. E. (2020). Pancreatic fistula and delayed gastric emptying are the highest-impact complications after whipple. J. Surg. Res. 250, 80–87. doi: 10.1016/j.jss.2019.12.041
Mori, H., Verbeure, W., Schol, J., Carbone, F., Tack, J. (2022). Gastrointestinal hormones and regulation of gastric emptying. Curr. Opin. Endocrinology Diabetes Obes. 29, 191. doi: 10.1097/MED.0000000000000707
Muller, P. A., Koscsó, B., Rajani, G. M., Stevanovic, K., Berres, M.-L., Hashimoto, D., et al. (2014). Crosstalk between muscularis macrophages and enteric neurons regulates gastrointestinal motility. Cell 158, 300–313. doi: 10.1016/j.cell.2014.04.050
Murray, C. D. R., Martin, N. M., Patterson, M., Taylor, S. A., Ghatei, M. A., Kamm, M. A., et al. (2005). Ghrelin enhances gastric emptying in diabetic gastroparesis: a double blind, placebo controlled, crossover study. Gut 54, 1693–1698. doi: 10.1136/gut.2005.069088
Naing, L. Y., Heckroth, M., Mathur, P., Abell, T. L. (2023). Gastroparesis syndromes: emerging drug targets and potential therapeutic opportunities. Expert Opin. Investigational Drugs 32, 245–262. doi: 10.1080/13543784.2023.2186222
Nalluri-Butz, H., Bobel, M. C., Nugent, J., Boatman, S., Emanuelson, R., Melton-Meaux, G., et al. (2022). A pilot study demonstrating the impact of surgical bowel preparation on intestinal microbiota composition following colon and rectal surgery. Sci. Rep. 12, 10559. doi: 10.1038/s41598-022-14819-1
Neuman, H., Debelius, J. W., Knight, R., Koren, O. (2015). Microbial endocrinology: the interplay between the microbiota and the endocrine system. FEMS Microbiol. Rev. 39, 509–521. doi: 10.1093/femsre/fuu010
Nguyen, L., Wilson, L. A., Miriel, L., Pasricha, P. J., Kuo, B., Hasler, W. L., et al. (2020). Autonomic function in gastroparesis and chronic unexplained nausea and vomiting: Relationship with etiology, gastric emptying, and symptom severity. Neurogastroenterol Motil. 32, e13810. doi: 10.1111/nmo.13810
Ohigashi, S., Sudo, K., Kobayashi, D., Takahashi, T., Nomoto, K., Onodera, H. (2013). Significant changes in the intestinal environment after surgery in patients with colorectal cancer. J. Gastrointestinal Surg. 17, 1657–1664. doi: 10.1007/s11605-013-2270-x
Okdahl, T., Wegeberg, A.-M., Jensen, A. B. H., Jensen, S. T., Andersen, H. R. P., Størling, J., et al. (2023). Systemic cytokine expression in diabetes is associated with prolonged gastrointestinal transit times and cardinal gastroparesis symptoms. Biomedicines 11, 1027. doi: 10.3390/biomedicines11041027
Omar Al-Hassi, H., Ng, O., Brookes, M. (2018). Tumour-associated and non-tumour-associated microbiota in colorectal cancer. Gut 67, 395. doi: 10.1136/gutjnl-2017-314219
Pan, C., Zhang, H., Zhang, L., Chen, L., Xu, L., Xu, N., et al. (2023). Surgery-induced gut microbial dysbiosis promotes cognitive impairment via regulation of intestinal function and the metabolite palmitic amide. Microbiome 11, 248. doi: 10.1186/s40168-023-01689-6
Panni, U., Srivastava, R., Bewley, A., Williams, G. A., Fields, R. C., Sanford, D. E., et al. (2023). Postoperative Proton Pump Inhibitors are associated with a significantly higher rate of delayed gastric emptying after pancreatoduodenectomy. HPB (Oxford) 25, 659–666. doi: 10.1016/j.hpb.2023.02.015
Paone, P., Cani, P. D. (2020). Mucus barrier, mucins and gut microbiota: the expected slimy partners? Gut 69, 2232–2243. doi: 10.1136/gutjnl-2020-322260
Parmar, A. D., Sheffield, K. M., Vargas, G. M., Pitt, H. A., Kilbane, E. M., Hall, B. L., et al. (2013). Factors associated with delayed gastric emptying after pancreaticoduodenectomy. HPB (Oxford) 15, 763–772. doi: 10.1111/hpb.12129
Reigstad, C. S., Salmonson, C. E., Rainey, J. F., Szurszewski, J. H., Linden, D. R., Sonnenburg, J. L., et al. (2015). Gut microbes promote colonic serotonin production through an effect of short-chain fatty acids on enterochromaffin cells. FASEB J. 29, 1395–1403. doi: 10.1096/fj.14-259598
Sanders, K. M., Koh, S. D., Ro, S., Ward, S. M. (2012). Regulation of gastrointestinal motility—insights from smooth muscle biology. Nat. Rev. Gastroenterol. Hepatol. 9, 633–645. doi: 10.1038/nrgastro.2012.168
Sanger, G. J., Furness, J. B. (2016). Ghrelin and motilin receptors as drug targets for gastrointestinal disorders. Nat. Rev. Gastroenterol. Hepatol. 13, 38–48. doi: 10.1038/nrgastro.2015.163
Sanger, Gj, Lee, K. (2008). Hormones of the gut-brain axis as targets for the treatment of upper gastrointestinal disorders. Nat. Rev. Drug Discovery 7, 241–254. doi: 10.1038/nrd2444
Schalla, M. A., Stengel, A. (2020). Effects of microbiome changes on endocrine ghrelin signaling – A systematic review. Peptides 133, 170388. doi: 10.1016/j.peptides.2020.170388
Schmitt, F. C. F., Brenner, T., Uhle, F., Loesch, S., Hackert, T., Ulrich, A., et al. (2019). Gut microbiome patterns correlate with higher postoperative complication rates after pancreatic surgery. BMC Microbiol. 19, 42. doi: 10.1186/s12866-019-1399-5
Schreiber, F., Balas, I., Robinson, M. J., Bakdash, G. (2024). Border control: the role of the microbiome in regulating epithelial barrier function. Cells 13, 477. doi: 10.3390/cells13060477
Serbanescu, M. A., Mathena, R. P., Xu, J., Santiago-Rodriguez, T., Hartsell, T. L., Cano, R. J., et al. (2019). General anesthesia alters the diversity and composition of the intestinal microbiota in mice. Anesth. Analgesia 129, e126. doi: 10.1213/ANE.0000000000003938
Shanahan, E. R., Kang, S., Staudacher, H., Shah, A., Do, A., Burns, G., et al. (2023). Alterations to the duodenal microbiota are linked to gastric emptying and symptoms in functional dyspepsia. Gut 72, 929–938. doi: 10.1136/gutjnl-2021-326158
Shao, Y., Ding, R., Xu, B., Hua, R., Shen, Q., He, K., et al. (2017). Alterations of gut microbiota after roux-en-Y gastric bypass and sleeve gastrectomy in sprague-dawley rats. Obes. Surg. 27, 295–302. doi: 10.1007/s11695-016-2297-7
Sharon, G., Sampson, T. R., Geschwind, D. H., Mazmanian, S. K. (2016). The central nervous system and the gut microbiome. Cell 167, 915–932. doi: 10.1016/j.cell.2016.10.027
Shogan, B. D., Chen, J., Duchalais, E., Collins, D., Chang, M., Krull, K., et al. (2020). Alterations of the rectal microbiome are associated with the development of postoperative ileus in patients undergoing colorectal surgery. J. Gastrointest Surg. 24, 1663–1672. doi: 10.1007/s11605-020-04593-8
Smits, F. J., Verweij, M. E., Daamen, L. A., van Werkhoven, C. H., Goense, L., Besselink, M. G., et al. (2022). Impact of complications after pancreatoduodenectomy on mortality, organ failure, hospital stay, and readmission: analysis of a nationwide audit. Ann. Surg. 275, e222. doi: 10.1097/SLA.0000000000003835
Stengel, A., Taché, Y. (2014). Brain peptides and the modulation of postoperative gastric ileus. Curr. Opin. Pharmacol. 19, 31–37. doi: 10.1016/j.coph.2014.06.006
Stoop, T. F., von Gohren, A., Engstrand, J., Sparrelid, E., Gilg, S., Del Chiaro, M., et al. (2023). Risk factors, management, and outcome of gastric venous congestion after total pancreatectomy: an underestimated complication requiring standardized identification, grading, and management. Ann. Surg. Oncol. 30, 7700–7711. doi: 10.1245/s10434-023-13847-z
Sullivan, A., Temperley, L., Ruban, A. (2020). Pathophysiology, aetiology and treatment of gastroparesis. Dig Dis. Sci. 65, 1615–1631. doi: 10.1007/s10620-020-06287-2
Sun, L.-J., Li, J.-N., Nie, Y.-Z. (2020). Gut hormones in microbiota-gut-brain cross-talk. Chin. Med. J. (Engl) 133, 826–833. doi: 10.1097/CM9.0000000000000706
Taboun, Z. S., Sadeghi, J. (2023). The bidirectional relationship between opioids and the gut microbiome: Implications for opioid tolerance and clinical interventions. Int. Immunopharmacol. 125, 111142. doi: 10.1016/j.intimp.2023.111142
Takatsuki, M., Hidaka, M., Natsuda, K., Adachi, T., Ono, S., Hamada, T., et al. (2021). Prevention of delayed gastric emptying after living donor left hepatectomy. Asian J. Surg. 44, 1274–1277. doi: 10.1016/j.asjsur.2021.02.006
Tang, G., Huang, W., Tao, J., Wei, Z. (2022). Prophylactic effects of probiotics or synbiotics on postoperative ileus after gastrointestinal cancer surgery: A meta-analysis of randomized controlled trials. PloS One 17, e0264759. doi: 10.1371/journal.pone.0264759
Tarazi, M., Jamel, S., Mullish, B. H., Markar, S. R., Hanna, G. B. (2022). Impact of gastrointestinal surgery upon the gut microbiome: A systematic review. Surgery 171, 1331–1340. doi: 10.1016/j.surg.2021.10.014
Tian, F., Gao, X., Zhang, L., Wang, X., Wan, X., Jiang, T., et al. (2016). Effects of n-3 PUFAs on Intestinal Mucosa Innate Immunity and Intestinal Microbiota in Mice after Hemorrhagic Shock Resuscitation. Nutrients 8, 609. doi: 10.3390/nu8100609
Torres-Fuentes, C., Golubeva, A. V., Zhdanov, A. V., Wallace, S., Arboleya, S., Papkovsky, D. B., et al. (2019). Short-chain fatty acids and microbiota metabolites attenuate ghrelin receptor signaling. FASEB J. 33, 13546–13559. doi: 10.1096/fj.201901433R
Tsigalou, C., Paraschaki, A., Bragazzi, N. L., Aftzoglou, K., Stavropoulou, E., Tsakris, Z., et al. (2023). Alterations of gut microbiome following gastrointestinal surgical procedures and their potential complications. Front. Cell Infect. Microbiol. 13. doi: 10.3389/fcimb.2023.1191126
Van den Houte, K., Scarpellini, E., Verbeure, W., Mori, H., Schol, J., Masuy, I., et al. (2020). The role of GI peptides in functional dyspepsia and gastroparesis: A systematic review. Front. Psychiatry 11. doi: 10.3389/fpsyt.2020.00172
Vélez, C. D., Kuo, B. (2021). “Chapter 9 - Gastroparesis and the brain-gut axis,” in Gastroparesis. Eds. McCallum, R. W., Parkman, H. P. (New York: Academic Press), 95–107. doi: 10.1016/B978-0-12-818586-5.00009-0
Waclawiková, B., Codutti, A., Alim, K., El Aidy, S. (2022). Gut microbiota-motility interregulation: insights from in vivo, ex vivo and in silico studies. Gut Microbes 14, 1997296. doi: 10.1080/19490976.2021.1997296
Wang, X., Chen, X., Wang, G., Cai, R., Wang, H., Wang, H., et al. (2023). A neural circuit for gastric motility disorders driven by gastric dilation in mice. Front. Neurosci. 17. doi: 10.3389/fnins.2023.1069198
Wang, L., Goebel-Stengel, M., Stengel, A., Wu, S. V., Ohning, G., Taché, Y. (2011). Comparison of CRF-immunoreactive neurons distribution in mouse and rat brains and selective induction of Fos in rat hypothalamic CRF neurons by abdominal surgery. Brain Res. 1415, 34–46. doi: 10.1016/j.brainres.2011.07.024
Wang, L., Yang, X., Wu, H. (2019). Juvenile rats show altered gut microbiota after exposure to isoflurane as neonates. Neurochem. Res. 44, 776–786. doi: 10.1007/s11064-018-02707-y
Wang, S. Z., Yu, Y. J., Adeli, K. (2020). Role of gut microbiota in neuroendocrine regulation of carbohydrate and lipid metabolism via the microbiota-gut-brain-liver axis. Microorganisms 8, 527. doi: 10.3390/microorganisms8040527
Wei, L., Singh, R., Ha, S. E., Martin, A. M., Jones, L. A., Jin, B., et al. (2021). Serotonin deficiency is associated with delayed gastric emptying. Gastroenterology 160, 2451–2466.e19. doi: 10.1053/j.gastro.2021.02.060
Wen, S.-L., Feng, X., Chen, Z.-Q., Xiao, J., Zhang, W.-X. (2016). Effect of XiangBin granules on post-operative gastrointestinal function and brain-gut peptides after transabdominal gynecological surgery. Eur. J. Obstetrics Gynecology Reprod. Biol. 205, 1–6. doi: 10.1016/j.ejogrb.2016.07.496
Xiong, H., He, Z., Wei, Y., Li, Q., Xiao, Q., Yang, L., et al. (2024). Probiotic compounds enhanced recovery after surgery for patients with distal gastric cancer: A prospective, controlled clinical trial. Ann. Surg. Oncol. 31, 5240–5251. doi: 10.1245/s10434-024-15394-7
Xue, L., Ding, Y., Qin, Q., Liu, L., Ding, X., Zhou, Y., et al. (2023). Assessment of the impact of intravenous antibiotics treatment on gut microbiota in patients: Clinical data from pre-and post-cardiac surgery. Front. Cell. Infection Microbiol. 12. doi: 10.3389/fcimb.2022.1043971
Yamane, S., Inagaki, N. (2018). Regulation of glucagon-like peptide-1 sensitivity by gut microbiota dysbiosis. J. Diabetes Invest. 9, 262–264. doi: 10.1111/jdi.12762
Yang, M., Fukui, H., Eda, H., Kitayama, Y., Hara, K., Kodani, M., et al. (2017). Involvement of gut microbiota in the association between gastrointestinal motility and 5−HT expression/M2 macrophage abundance in the gastrointestinal tract. Mol. Med. Rep. 16, 3482–3488. doi: 10.3892/mmr.2017.6955
Yang, S., Liang, X., Song, J., Li, C., Liu, A., Luo, Y., et al. (2021). A novel therapeutic approach for inflammatory bowel disease by exosomes derived from human umbilical cord mesenchymal stem cells to repair intestinal barrier via TSG-6. Stem Cell Res. Ther. 12, 315. doi: 10.1186/s13287-021-02404-8
Yao, Y., Sun, S., Gu, J., Ni, H., Zhong, K., Xu, Q., et al. (2022). Roux-en-Y reconstruction alleviates radical gastrectomy-induced colitis via down-regulation of the butyrate/NLRP3 signaling pathway. eBioMedicine 86, 104347. doi: 10.1016/j.ebiom.2022.104347
Ye, Y., Yin, Y., Huh, S. Y., Almansa, C., Bennett, D., Camilleri, M. (2022). Epidemiology, etiology, and treatment of gastroparesis: real-world evidence from a large US national claims database. Gastroenterology 162, 109–121.e5. doi: 10.1053/j.gastro.2021.09.064
Yu, J., Zhu, P., Shi, L., Gao, N., Li, Y., Shu, C., et al. (2023). Bifidobacterium longum promotes postoperative liver function recovery in patients with hepatocellular carcinoma. Cell Host Microbe S1931-3128, 00461–00464. doi: 10.1016/j.chom.2023.11.011
Yuan, P.-Q., Taché, Y. (2017). Abdominal surgery induced gastric ileus and activation of M1-like macrophages in the gastric myenteric plexus: prevention by central vagal activation in rats. Am. J. Physiol. Gastrointest Liver Physiol. 313, G320–G329. doi: 10.1152/ajpgi.00121.2017
Zhang, Y., Baldyga, K., Dong, Y., Song, W., Villanueva, M., Deng, H., et al. (2023). The association between gut microbiota and postoperative delirium in patients. Transl. Psychiatry 13, 1–7. doi: 10.1038/s41398-023-02450-1
Zhang, Q. L., Zhou, S. J., Chen, X. H., Chen, Q. (2024). Changes of intestinal flora and the effect on intestinal function in infants with ventricular septal defect after cardiopulmonary bypass surgery. Curr. Problems Cardiol. 49, 102111. doi: 10.1016/j.cpcardiol.2023.102111
Zheng, Z., Hu, Y., Tang, J., Xu, W., Zhu, W., Zhang, W. (2023). The implication of gut microbiota in recovery from gastrointestinal surgery. Front. Cell. Infection Microbiol. 13. doi: 10.3389/fcimb.2023.1110787
Keywords: postoperative delayed gastric emptying, gut microbiota, postoperative complications, gastric emptying, gastroparesis
Citation: Wang Z, Liu C, Hu K, Zuo M, Tian Z, Wei Y, Zhou Q and Li Q (2024) Postoperative delayed gastric emptying: may gut microbiota play a role? Front. Cell. Infect. Microbiol. 14:1449530. doi: 10.3389/fcimb.2024.1449530
Received: 15 June 2024; Accepted: 22 July 2024;
Published: 13 August 2024.
Edited by:
Ann-Kathrin Lederer, Johannes Gutenberg University Mainz, GermanyReviewed by:
Francesco Vito Mandarino, Vita-Salute San Raffaele University, ItalyMohamed Tarek Badr, Freiburg University Medical Center, Germany
Copyright © 2024 Wang, Liu, Hu, Zuo, Tian, Wei, Zhou and Li. This is an open-access article distributed under the terms of the Creative Commons Attribution License (CC BY). The use, distribution or reproduction in other forums is permitted, provided the original author(s) and the copyright owner(s) are credited and that the original publication in this journal is cited, in accordance with accepted academic practice. No use, distribution or reproduction is permitted which does not comply with these terms.
*Correspondence: Quanwang Li, cXVhbndhbmdsaUAxMjYuY29t; Qin Zhou, cWluemkwNDI0QDEyNi5jb20=