- 1Department of Dermatology, The Second Affiliated Hospital of Shantou University Medical College, Shantou, China
- 2Department of Clinical Laboratory, Skin and Venereal Diseases Prevention and Control Hospital of Shantou City, Shantou, Guangdong, China
- 3Department of Cell Biology & Genetics, Shantou University Medical College, Shantou, China
- 4Research Institute of Clinical Pharmacy, Shantou University Medical College, Shantou, China
- 5Department of Dermatology, Changzheng Hospital, Second Military Medical University, Shanghai, China
- 6Department of Medical-Surgical and Experimental Sciences University of Sassari Neurology Unit, Azienza Ospedaliera Universitaria (AOU), Sassari, Italy
- 7Jiangxi Key Laboratory of Oncology (2024SSY06041), Jiangxi Cancer Hospital and Institute, The Second Affiliated Hospital of Nanchang Medical College, Nanchang, Jiangxi, China
- 8Department of Dermatology, West China Second University Hospital, Sichuan University, Chengdu, Sichuan, China
A biomarker is an important indicator of a normal physiological or pathological process, or a pharmacological response to a therapeutic intervention. This retrospective study aimed to measure blood biomarkers in wound patients, identify the microorganisms responsible for wound infections and determine their drug susceptibility patterns at a tertiary care hospital in China. The study was conducted between 2022 and 2024, including 279 patients. A total of 33 microbial species were isolated using culture techniques, identified, and analyzed for their antibiotic susceptibility. The organisms were predominantly gram-positive (50.8%), with Staphylococcus aureus (80.2%) being the most prevalent species. Among the gram-negative bacteria (41.2%), Pseudomonas aeruginosa (22.6%) was the most predominant species. Biomarkers such as white blood cells, neutrophils, lymphocytes, and erythrocyte sedimentation rate (ESR) values were higher than normal in most of microbial species associated with wound infections. The WBC value in gram-positive infections and the neutrophil and ESR values in fungal infections were statistically significantly higher than the normal range (p = 0.0002, p = 0.002, and p = 0.003, respectively). Albumin levels were high value in P. aeruginosa and K. pneumoniae (0.48 and 0.56 respectively), while lymphocytes levels were the lowest value (-0.62) in S. aureus. Resistance to at least one antibiotic was identified in 82.4% of the isolates. The prevalence of multidrug-resistant microbes in different wound infections is a significant concern in China. A health awareness campaign, coupled with improved hygiene measures, should be implemented to prevent the spread of microorganisms responsible for wound infections within the community.
1 Introduction
Skin serves as the first-line immune defense barrier against pathogen colonization. Therefore, alterations in its normal structure due to surgery or chemical, mechanical, or thermal trauma disrupt its function, leading to wounds (Prescott et al., 2017; Guan et al., 2021; Gowda et al., 2023). Regardless of the wound type, wound infections are frequently linked to patient morbidity and mortality, particularly in developing countries (Falanga et al., 2022). Treatment failure refers to increased healthcare expenses due to prolonged hospital stays for diagnostic testing, extensive antibiotic therapy, and in some cases of invasive surgery (Zuarez-Easton et al., 2017). The bacterial species most frequently responsible for wound infections include A. baumannii, P. aeruginosa, S. aureus, K. pneumoniae, and E. faecalis (Stevens, 2009; Ch’ng et al., 2019; Huszczynski et al., 2019; Puca et al., 2021). Some fungi have also been identified as causes wound infections. The entry of these organisms into the bloodstream and lymphatic system can trigger sepsis (Bilal et al., 2022).
A biomarker is measurable evidence that serves as an indicator of a normal physiological or pathological process, or a pharmacological response to a therapeutic intervention (Califf, 2018). Predictive biomarkers can predict outcomes or indicate the likelihood of therapy effectiveness. They may act as a powerful tool in tailoring therapeutic approaches for specific groups of patient populations. Diagnostic biomarkers can identify the presence of one or more factors that may influence clinical outcomes. An indicative biomarker can be utilized to determine disease development and/or therapeutic response in real time (Lindley et al., 2016).
The emergence of so-called “super-bugs,” or bacteria that are resistant to multiple drugs, is a significant public concern. Drug resistance results from the improper use of antibiotics in both humans and animals (Rahman et al., 2022). According to a 2014 WHO assessment, multidrug-resistant bacteria cause over 25,000 deaths annually in Europe and 23,000 deaths annually in the US (Diallo et al., 2020). Furthermore, approximately 50% of infections caused by K. pneumoniae, S. aureus, P. aeruginosa, and E. coli exhibit resistance to the most potent antibiotics, including third-generation cephalosporins (Diallo et al., 2020).
Large quantities of carbapenem-resistant, methicillin-resistant, and ESBL-producing bacteria have been found globally. The issue of ESBL producing microbes is particularly severe in developing countries (Iskandar et al., 2021). Therefore, bacteria that produce ESBL can resist the effects of these antibiotic classes (Nagshetty et al., 2021; Alfei and Schito, 2022). The most prevalent gram-negative bacteria producing ESBL include E. coli, K. pneumoniae, P. mirabilis, and P. aeruginosa (Oshiokhayamhe, 2023).
In this retrospective study, we identified the microbial species responsible for wound infections and analyzed their drug susceptibility patterns and determined the associated biomarker. The data provided can guide physicians in improving wound infection surveillance, prevention, and control.
2 Methods
2.1 Study site and population
This retrospective study was conducted at the Skin and Venereal Disease Prevention and Control Hospital located in Shantou City, Guangdong, China. The study period was 3 years, from January 2022 to September 2024. A total of 279 specimens (swabs, pus, tissue, and fluid) were collected from wounds by a trained nurse following standard procedures (Gjødsbøl et al., 2012). Patients with clinical signs of wound infection, such as redness, swelling, discomfort, persistent discharge, and an unpleasant odor were recorded (Gardner et al., 2001; Haesler et al., 2019). Data on patient type, age, gender, and infection sites were recorded in the wound care log database (Dong et al., 2019).
2.2 Routine laboratory protocol
2.2.1 Sample collection
Pus specimens were collected from the patients wounds in hospital wards utilizing sterile cotton swabs and fine needle syringes. Samples were taken from various parts of the body, including leg, hand, back, abdomen, foot, breast, chest, head, and neck wounds. Each sample was labeled with the patient’s information, the collection approach, and the date and time of collection. Complete information about the patient was obtained, including the infection site, symptoms, and prior antibiotic therapy. Moreover, a complete blood count (CBC: leukocytes, neutrophils, and lymphocytes) was analyzed using an Automatic blood analyzer (Sysmex XN-1000 SA-01). Erythrocyte sedimentation rate (ESR) and albumin values were measured using a sediment detection frame and BS-800 Mandry biochemical instrument, respectively.
2.2.2 Sample preparation, bacterial culture, and identification
Based on the type and location of the wound, specimens were visually assessed for consistency, color, turbidity, and the presence of blood. According to established clinical laboratory protocols, pus samples were inoculated onto blood agar, chocolate agar, MacConkey agar, nutrient agar, potato dextrose agar, Sabouraud dextrose agar, and CHROMagar-Candida medium. The VITEK 2 COMPACT system (bioMérieux, Marcy-l’Étoile, France) or the MALDI Biotyper system (Bruker Daltonics GmbH, Bremen, Germany) was used to identify the isolated bacteria and fungi according the manufacturer’s instructions.
2.2.3 Analysis of the antimicrobial susceptibility patterns of the isolated organisms
Antimicrobial susceptibility testing was conducted using the VITEK 2 system (bioMérieux, Marcy-l’Étoile, France) according to the manufacturer’s guidelines. The tested antibiotics were used against bacterial and fungal isolates. The organisms were categorized as “susceptible,” “resistant,” or “intermediate,” according to the guidelines established by the (Clinical and Laboratory Standards Institute (CLSI), 2024) (CLSI M100-ED34: 2024 performance standards for antimicrobial susceptibility testing). Multidrug-resistant (MDR) strains were defined as isolates resistance to at least one antibiotic from two or more classes.
2.2.4 Confirmation of MRSA and ESBL producers
Methicillin-resistant strains were suspected based on MIC results and confirmed using cefoxitin disc diffusion tests (Clinical and Laboratory Standards Institute (CLSI), 2007). ESBL-producing bacteria were detected among Enterobacteriaceae isolates using antibiotic discs that contained 30 μg of cefotaxime, ceftazidime, ceftriaxone, and aztreonam. According to standard guidelines, bacterial isolates with ceftazidime smaller than 22 mm and cefotaxime smaller than 27 mm were considered potential ESBL producers (Clinical and Laboratory Standards Institute (CLSI), 2007).
2.2.5 Quality control and statistical analysis
A sterility examination was conducted on all produced biochemical medium and streaked plates. The reference strains E. coli ATCC 25922 and S. aureus ATCC 25923 were used as quality controls for antibacterial susceptibility testing and biochemical assays. The strains C. albicans ATCC 10231 and C. parapsilosis ATCC 22019 were used as quality controls for antifungal susceptibility testing. The DDST was also performed and phenotypically confirmed for ESBL-producing gram-negative bacilli using E. coli ATCC 25922 as a negative control.
Data analysis was conducted using GraphPad Prism version 8.0.2. Frequencies for categorical variables were calculated. The chi-square test was used for comparisons, with a p-value of less than 0.05 considered significant. Additionally, statistical analysis of the data was performed using R 4.4.1 software. Shapiro-Wilk tests were employed to determine if the groupings followed a normal distribution. Numerical data were presented as percentages and medians (25th–75th percentiles). R 4.4.1 along with respective libraries such as ggplot2, dplyr, tidyr, corrplot, and agricolae was used for analysis. ANOVA, followed by the post-hoc Tukey test, was applied to compare the impact of microorganisms on blood biomarkers. Pearson correlation was conducted to assess the associations between microorganisms and blood biomarkers. The results were presented as a heatmap.
3 Results
3.1 Clinical characteristics of patients with wound infections
Over the 3-year period, a total of 279 patients with clinical wound infections were monitored, including 90 (32.0%) females and 189 (68.0%) males. The distribution of wound infections in males and females is shown in Figure 1. The patients had a median age of 57 years (interquartile range: 38–68 years) and were analyzed between 2022 to 2024 (Table 1). A large number of patients were recruited from the inpatient department (I and II; 234 [84.0%]), followed by the dermatology outpatient department (45 [16.0%]).
3.2 Analysis of microbial species and biomarkers
A total of 279 microbial isolates were identified, of which 142 (50.8%) were gram-positive bacteria, 115 (41.2%) were gram-negative bacteria, and 20 (7.16%) were fungi. S. aureus was the most predominant (n = 114, 40.8%), followed by P. aeruginosa (n = 26, 9.31%), K. pneumoniae (n = 19, 6.81%), E. coli (n = 14, 5.01%), S. haemolyticus (n = 13, 4.65%), and C. albicans (n = 12, 4.30%). The distribution of microorganisms per year is shown in Table 2 and Figure 2. A higher percentage of microbial isolates were identified in inpatients (n = 248, 89.5%) than in outpatients (n = 31, 10.4%) (Supplementary Table S1). The distribution of microbial species associated with biomarker in wound infections is shown in Figure 3. The leukocyte, neutrophil, and lymphocyte counts, as well as ESR values, of patients with gram-negative bacterial, gram-positive bacterial, and fungal infections were higher than the normal range (Table 3). The WBC value in gram-positive infections and neutrophil and ESR values in fungal infections were statistically significantly higher than the normal range (p = 0.0002, p = 0.002, and p = 0.003, respectively). The percentage of abnormal biomarkers leukocyte, neutrophil, and lymphocyte and ESR associated with different skin diseases is shown in Figure 4 and Table 4. Moreover, the correlation between microorganisms and biomarkers is shown in Figure 5. Albumin shows a high value in P. aeruginosa and K. pneumoniae, while lymphocytes show the lowest value in S. aureus.
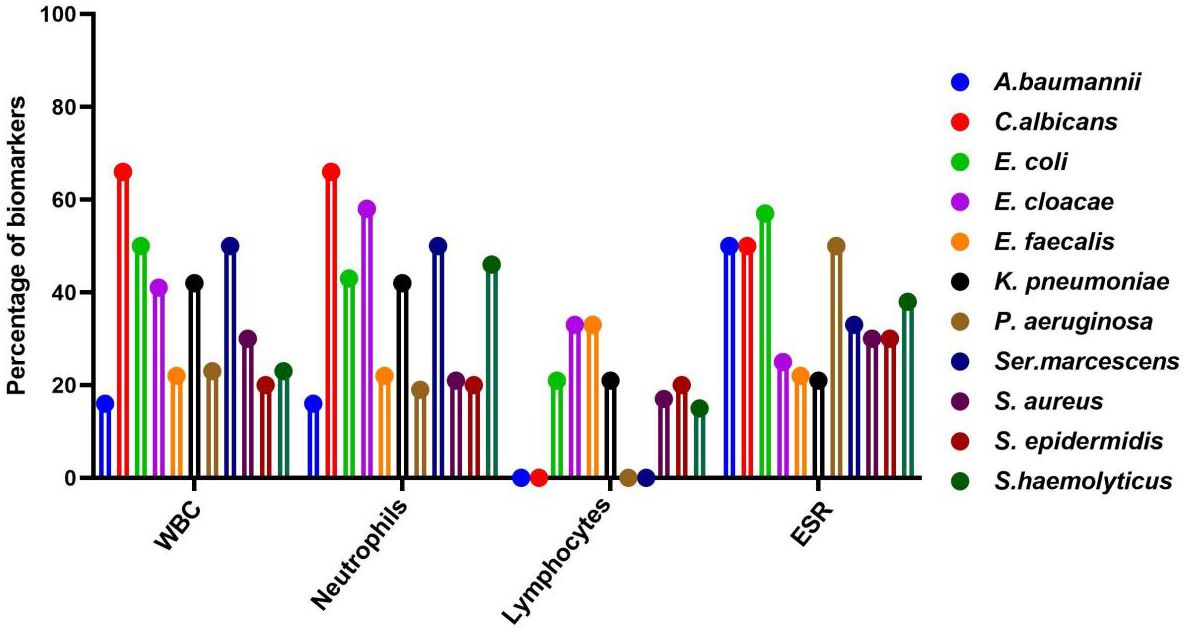
Figure 3. Distribution of microbial species associated with key biomarker abnormal range in wound infections. A, Acinetobacter; C, Candida; E. coli, Escherichia Coli; E, Entercoccus; K, klebsiella; P, Pseudomonas; and S, staphylococcus.
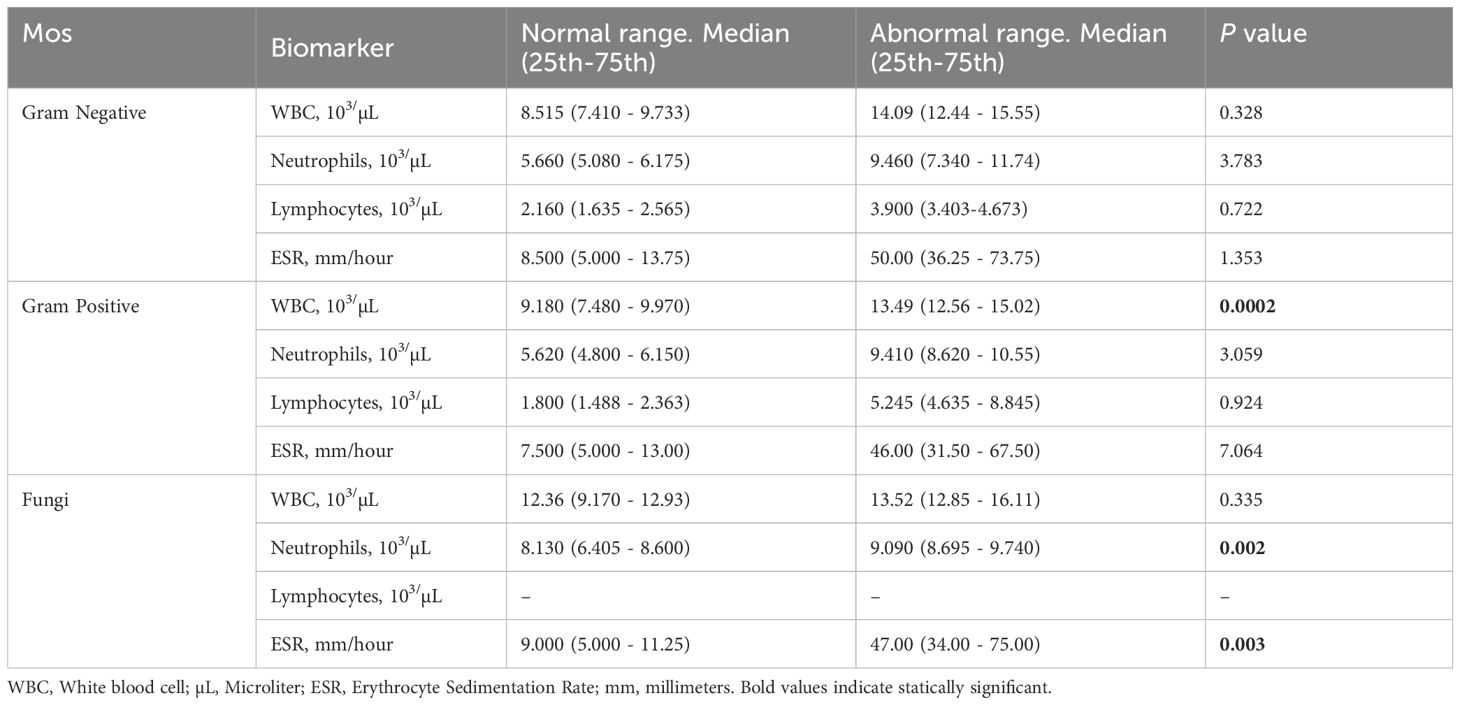
Table 3. Comparison of normal and abnormal range of laboratory measurements in patients with wound infections.
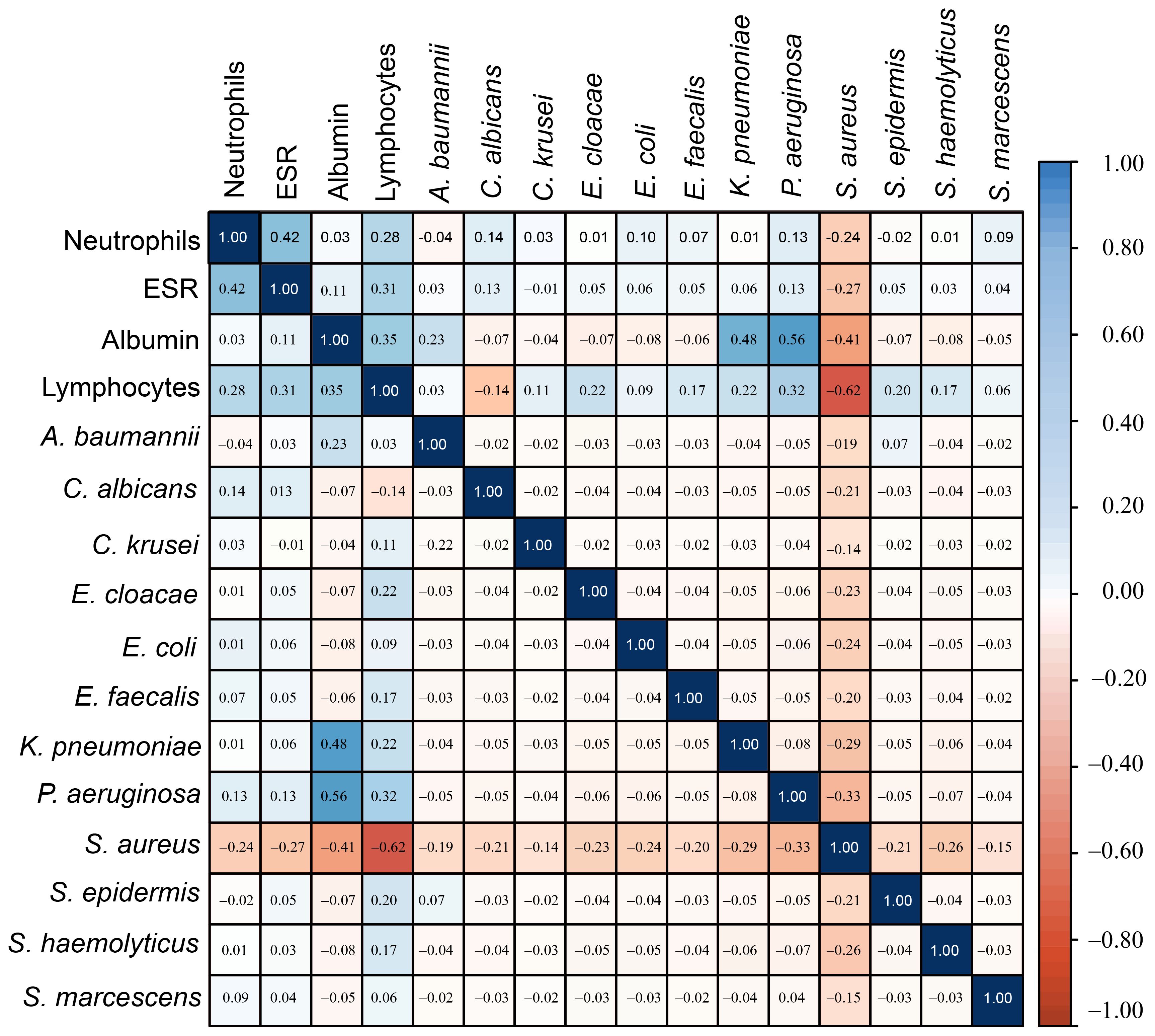
Figure 5. The correlation heatmap of microorganisms with Biomarkers showed that S. aureus had a strong correlation with lymphocytes, while K. penumoniae and P. aeruginosa showed a weak correlation with albumin.
3.3 Biomarkers associated with gram -negative bacteria
The prevalence of gram-negative and key biomarkers is shown in the Supplementary Table S2. E. coli and Ser. marcescens exhibited a high percentage (50.0%) of abnormal WBC ratios compared to normal range. Similarly, the percentage of abnormal neutrophil ratios compared to normal range was high in K. pneumoniae and Ser. marcescens. The abnormal value of lymphocytes compared with normal value was high in E. faecalis. Moreover, in E. coli and P. aeruginosa exhibited a high percentage of abnormal ESR ratios compared to the normal value. There was no statistically significant correlation between gram-negative bacteria and biomarkers such as WBC and ESR. While the correlation of neutrophils in E. cloacae and lymphocytes in E. coli, E. cloacae were identified statically significant (p < 0.05). Moreover, the correlation of A.baumannii with albumin was statistically significant (p < 0.05).
3.4 Biomarkers associated with gram- positive bacteria
The prevalence of gram-positive bacteria and key biomarkers is shown in the Supplementary Table S3. In S.aureus, the percentage (28.0%) of abnormal ratio of WBC]was high compared to the normal ratio. In S. haemolyticus, abnormal neutrophils value were detected at higher levels compared to the normal value. Similarly, the high percentage (20.0%) of abnormal lymphocytes values of compared to the normal range was found in S. epidermidis. Moreover, the abnormal ESR values were high in the S.haemolyticus and S.ludgunensis. There was no statistically significant correlation between gram-positive bacteria and biomarkers such as albumin, WBC, and ESR. However, the correlation of neutrophils in S. aureus was statistically significant (p < 0.05). Similarly, the correlation of lymphocytes was statistically significant (p < 0.05) in S. aureus and S. epidermidis.
3.5 Biomarkers associated with fungi
The distribution of fungi and key biomarkers is shown in the Supplementary Table S4. The high percentage (66.6%) of abnormal values of WBC and neutrophils compared with normal value were recorded in C.albicans and C.tropicalis. Similarly, a high percentage (50.0%) of abnormal lymphocyte values, compared with normal range, were found in C. albicans. Furthermore, a high percentage (33.3%) of abnormal lymphocyte values, compared with normal value were detected in C.tropicalis. There was no statistically significant correlation between fungi and biomarkers such as WBC, neutrophils, lymphocytes, ESR and albumins.
3.6 Antibiotic resistance patterns in wound samples from 2022 to 2024
The antibiotic resistance patterns showed that 82.4% of wound samples contained organisms resistant to at least one agent (Table 5). Of these samples, 18.6% showed resistance to only one antibiotic, 21.9% to two, 17.2% to three, 10.7% to four, 11.2% to five, and 20.0% to at least six. The assessment of resistance distribution, classified by survey year, indicated an increase in resistance from 2022 to 2024 (Table 5).
3.7 Antibacterial susceptibility
A. baumannii showed 100% susceptibility to amikacin, co-trimoxazole, rifampin, and tigecycline, while E. coli demonstrated 100% susceptibility to vancomycin and oxacillin. In contrast, all E. coli isolates were resistant to 29 tested antibiotics, with resistance rates ranging from 11.1% to 100.0%. Similarly, all E. cloacae isolates were 100% susceptible to ceftriaxone, clotrimazole, and piperacillin/tazobactam. However, E. cloacae showed high resistance to voriconazole (75.0%). K. pneumoniae exhibited high susceptibility to ertapenem (87.5%), imipenem (84.6%), and piperacillin/tazobactam (84.6%), but demonstrated 100% resistance to tetracycline. P. aeruginosa isolates were highly susceptible to moxifloxacin and tigecycline (77.7%). In contrast, all P. aeruginosa isolates were resistant to 18 tested antibiotics, with values ranging from 20.0% to 50.0%. Moreover, seven E. coli isolates (53.8%), six K. pneumoniae isolates (46.1%), and one K. oxytoca isolates (25.0%) tested positive for ESBL. Among the gram-negative MDR isolates, A. baumannii (33.3%), E. coli (78.5%), E. faecalis (16.6%), E. cloacae (60.0%), K. pneumoniae (47.3%), and P. aeruginosa (8.69%) were identified.
E. faecalis isolates exhibited highly resistant to gentamicin (80%), tetracycline (75.0%), co-trimoxazole (66.6%), and erythromycin (62.5%). All isolates of S. aureus were sensitive to 28 tested antibiotics, with susceptibility rates ranging from 51.7% to 95.0%. All staphylococcal isolates, including S. epidermidis and S. haemolyticus, were 100% susceptible to ceftriaxone, cefazolin, tigecycline, and vancomycin. In contrast, S. epidermidis and S. haemolyticus showed 100% resistance to ampicillin and oxacillin. Among the total strains, 45.5% were MRSA strains, and 50.0% were resistant to ceftazidime, followed by penicillin (43.3%), oxacillin (41.2%), clindamycin (39.3%), itraconazole (33.3%), and cefoxitin (32.8%). S. epidermidis and S. haemolyticus showed significant resistance to various antibiotics, confirming their classification as methicillin-resistant coagulase-negative staphylococci. S. epidermidis exhibited 100% resistance to oxacillin, ceftriaxone, and cefazolin and 85.71% resistance to penicillin. S. haemolyticus showed 100% resistance to oxacillin, penicillin, ceftriaxone, cefazolin, vancomycin, and tetracycline. Additionally, S. haemolyticus showed the highest proportion (100%) of gram-positive MDR isolates, followed by S. aureus (70.5%) and S. epidermidis (70.0%).
3.8 Antifungal susceptibility
C. albicans isolates were 100% susceptible to amikacin. All C. albicans isolates showed susceptibility to six other tested antibiotics, with values ranging from 50.0% to 91.6%. C. krusei isolates were 100% susceptible to six tested antibiotics and 50.0% susceptible to the only tested fluconazole. C. tropicalis isolates were 100% susceptible to five tested antibiotics but resistant to imipenem (100%) and fluconazole (33.3%). Among the fungal MDR isolates, C. tropicalis (33.3%) and C. albicans (8.33%) were identified.
4 Discussion
CBC indices, specifically leukocytes, neutrophils, and lymphocytes, are important inflammatory markers and have been gaining increasing attention. They are usually considered indicators of subclinical inflammation. Their immediate availability offers a significant advantage (Serban et al., 2024). Furthermore, inflammatory indicators are believed to be correlated with the prognosis of bacterial infections. ESR is also commonly used to assess the presence and severity of several infections in patients with wounds (Chen et al., 2024; Mahmoud et al., 2024). In our study, WBC values were significantly higher than the normal range in gram-positive bacterial infections (p = 0.0002). Similarly, neutrophil and ESR values were significantly higher than the normal range in fungal infections (p = 0.0002 and p = 0.003, respectively). However, no significant differences were found in the WBC, neutrophils, ESR, and lymphocytes ratios between normal and abnormal ranges across in microbial species, as shown in Table 4. The correlation of neutrophils in E. cloacae, and S. aureus was statistically significant (p < 0.05). Similarly, the correlation of lymphocytes was statistically significant (p < 0.05) in E. coli, E. cloacae, S. aureus and S. epidermidis. Moreover, the correlation of A.baumannii with albumin was statistically significant (p < 0.05). Albumin shows a high value in P. aeruginosa and K. pneumoniae, while lymphocytes show the lowest value in S. aureus. The results showed that albumin value increases beyond the normal range, the chances of skin infections caused by P. aeruginosa and K. pneumoniae may be increase. Similarly, the skin infections caused by S. aureus are more likely when lymphocytes decrease (Figure 5). The microorganisms showed strong correlation with biomarkers in wound infections (Siddiqui and Bernstein, 2010). The neutrophils and lymphocytes as an indicator of systemic inflammation in bacterial infections, including wound infections caused by bacteria (Rigby and Deleo, 2012). However, further research is needed to study the correlation between biomarkers and microorganisms at the molecular level. In a wound infection, the immune system involves both innate and adaptive pathways, including white blood cells, lymphocytes, and changes in ESR rate (Strbo et al., 2014; Zahorec, 2021). Neutrophils, as first responders, execute phagocytosis and secrete enzymes to eliminate infections. Inflammatory signals trigger the production of acute-phase proteins such as fibrinogen, leading to an elevated ESR as an indicator of inflammation (Gulhar et al., 2018). Adaptive immunity activates with B cells producing antibodies to neutralize infections and enhance phagocytosis, while T cells regulate the immune response and eradicate infected cells (Bonilla and Oettgen, 2010). In wound infections, the typical abnormal ratio showed changes such as elevated WBC count (>11,000 cells/µL), increased neutrophils (>70% of WBC), and decreased lymphocytes (<20% of WBC). Additionally, ESR is also elevated (>20 mm/hr, often >50 mm/hr), indicating inflammation.
Routine testing in microbiology laboratories mainly depends on culture methods to identify and isolate suspected infections from swabs, pus, or tissue biopsies. This helps in species identification and antibiotic susceptibility determination to guide treatment. A standardized method support global surveillance to mitigate the rising incidence of antibiotic resistance (Maillard et al., 2021). This retrospective analysis identified 26 microbial species from infected wounds. Gram-positive bacteria constituted a high proportion of cases (50.8%) compared to gram-negative bacteria (41.2%), aligning with previous studies (Lakhey and Bhatt, 2007; Guan et al., 2021). However, numerous previous studies have reported a higher incidence of gram-negative isolates compared to gram-positive bacteria (Du et al., 2022). This variation in results may be attributed to differences in patients’ demographic characteristics (Ghosh et al., 2022).
Our findings were consistent with other studies, which have reporting that S. aureus was predominant and highly resistant to antibiotics. S. aureus usually produces biofilms in chronic wounds, resulting in drug resistance (Parastan et al., 2020). A total of 45.5% MRSA strains were identified in this study. Our findings revealed a significant increase in the prevalence of MRSA compared to a previous survey by Haonan Guan et al (Guan et al., 2021), which reported MRSA strains resistant to vancomycin. This highlights a concerning rise in the incidence of MRSA in China. S. aureus exhibited sensitivity to oxacillin at a rate of 58.8%, but 41.18% of the variant resistant to oxacillin. This figure was lower than that reported by Haonan Guan et al. in China (Guan et al., 2021). The differences might be attributed to variations in bacterial culture methods and the geographical location of the studies. MRSA resistance to vancomycin due to genetic acquisition (vanA/B), adaptive mutations Vancomycin-Intermediate S. aureus, or biofilm-mediated tolerance (Chang et al., 2003; Holmes et al., 2012; Alav et al., 2018).
The predominant gram-negative bacteria were P. aeruginosa and E. coli, as previously reported (Guan et al., 2021; Wang et al., 2024). These bacterial species interfere with wound healing (Serra et al., 2015; Wong et al., 2015). In this study, P. aeruginosa constituted 6.81% of the most prevalent gram-negative bacteria in chronic wounds. P. aeruginosa (14.8–16.7%) was have also been reported as the predominant gram-negative bacterium in chronic wounds in earlier studies (Gadepalli et al., 2006; Wong et al., 2015). Another study demonstrated that P. aeruginosa was associated with deeper tissue layer invasion (Fazli et al., 2009). In our study, we also found that P. aeruginosa was highly resistant to antibiotics such as erythromycin, gentamicin, nitrofurantoin, and rifampin (> 30%) but showed at least > 25% resistance to vancomycin, moxifloxacin, and tigecycline. Additionally, A. baumannii demonstrated to 100% susceptibility to amikacin and tigecycline. The high susceptibility of A. baumannii to tigecycline (96.1%) and amikacin (96.4%) was also previously observed (Alhussain et al., 2021). Amikacin and tigecycline may experience minimal resistance development due to their efficient mechanism of action, limited misuse, resistance to prevalent strains, and their role in effective antimicrobial management within the study setting. Imipenem showed resistance rates below 20% for E. coli and K. pneumoniae; however, resistance increased in E. cloacae. Amikacin also showed resistance rates below 20% for E. coli and E. cloacae. Gram-negative bacteria have lowered resistance to amikacin, consistent with previously reported (Guan et al., 2021). P. aeruginosa, E. coli, and A. baumannii acquire carbapenem resistance mainly due to blaNDM, blaKPC, and OXA-type carbapenemases. Efflux pumps such as, mexAB-oprM in P. aeruginosa, adeABC in A. baumannii enhance multidrug resistance, while E. coli frequently developed plasmid-borne resistance genes like mcr-1 and blaCTX-M-15 (Alav et al., 2018; Eichenberger and Thaden, 2019; Hammoudi Halat and Ayoub Moubareck, 2020; Gauba and Rahman, 2023).
Klebsiella spp. developed significant resistance to ß-lactam antibiotics, consistent with previous studies conducted in China (Chong et al., 2018). Similarly, a review of antimicrobial resistance trends in Asia highlighted the growing threat of multidrug-resistant Klebsiella spp (Effah et al., 2020). K. pneumoniae resists β-lactams due to ESBLs (CTX-M SHV), carbapenemases (KPC and NDM), and AmpC enzymes (DHA and CMY) (Tooke et al., 2019; Yang et al., 2022). Antimicrobial resistance due to antibiotic overuse is a critical global health threat, affecting individuals of all genders and ages equally (Effah et al., 2020). The resistance of C. albicans ranged from 8.33% to 50.0%. Thus, C. albicans demonstrated a high level of antibiotic resistance, aligning with previous reports (Bilal et al., 2022).
The prevalence of the MDR profile was higher in gram-positive bacteria compared to gram-negative bacteria. The MDR rate for gram-positive bacteria was 50.3%, which aligns closely with the rates reported in previous studies in Egypt (Ahmed et al., 2023), but different from study reported in China (Guan et al., 2021). In contrast, gram-negative isolates had an overall MDR rate of 37.6%, with the highest MDR profile found in E. coli (34.3%), followed by K. pneumoniae (28.1%) and E. faecalis (7.05%). These results were lower than the findings reported by Haonan Guan et al (Guan et al., 2021). Regarding the MDR profiles of fungi, C. albicans and C. tropicalis had the lowest MDR rates (1.72%). Similar findings have been previously reported (Puca et al., 2021). However, the variation may be due to regional antibiotic prescribing practices, prolonged hospital stays enhancing exposure to nosocomial pathogens, or the lack of robust antimicrobial stewardship programs in our hospital setting. The absence of molecular characterization restricts our study from tracking transmission pathways or confirming clonal outbreaks.
The division of isolates by year is another important aspect of this study. Particularly, in 2022, the percentages of isolated gram-negative and gram-positive bacteria were similar. However, in the subsequent years 2023 and 2024, there was an increase of gram-positive isolates (approximately 57%), along with a slight decrease in the number of gram-negative bacteria. In contrast, the proportion of fungal strains showed no significant variations over time, suggesting an increased spread of gram-positive species. The main limitations of this study are as follows: 1. The presence of anaerobic and microaerophilic bacteria was not detected; 2. No data on polymicrobial infections were collected; 3. The study findings should be applied with caution due to variations in individuals and circumstances across geographic locations. However, the findings of this study’s are important for developing strategies to improve the management of wound infections in our hospital and similar settings. The biomarker analysis integrating into clinical practice, clinician can achieve early detection, targeted treatments, better prevention, and infection control measures, as results lead to better patient outcomes and diminished complications in wound infections.
5 Conclusion
In this study, the most common species causing wound infections included S. aureus and P. aeruginosa. Among the gram negative, E. coli and Ser. marcescens showed a higher percentage of abnormal WBC ratios compared to the normal range. Among gram-positive bacteria, the percentage of S.aureus with abnormal WBC ratios was higher compared with normal range. However, WBC values were significantly higher than the normal range in gram-positive bacterial infections (p = 0.0002). While neutrophils and ESR values were significantly higher than the normal range in fungal infections (p = 0.0002 and p = 0.003, respectively. Albumin shows a high value in P. aeruginosa and K. pneumoniae (0.48 and 0.56 respectively), while lymphocytes show the lowest value (-0.62) in S. aureus. The results indicated that as albumin value exceed the normal range, the chances of skin infections caused by P. aeruginosa and K. pneumoniae increases. Similarly, the skin infections caused by S. aureus also increase with a decrease in lymphocytes ratios. Overall, it is very important to further investigate the associated between key biomarkers and microbial species to effectively control and manage wound infections.
Data availability statement
The original contributions presented in the study are included in the article/Supplementary Material. Further inquiries can be directed to the corresponding authors.
Ethics statement
The studies involving humans were approved by Department of Dermatology, The Second Affiliated Hospital of Shantou University Medical College, Shantou, 515041, China. The studies were conducted in accordance with the local legislation and institutional requirements. Written informed consent for participation in this study was provided by the participants’ legal guardians/next of kin.
Author contributions
SK: Conceptualization, Methodology, Resources, Software, Writing – original draft, Writing – review & editing. BH: Methodology, Resources, Writing – review & editing. MK: Software, Validation, Writing – review & editing. MS: Formal Analysis, Validation, Writing – review & editing. LC: Formal Analysis, Project administration, Writing – review & editing. WF: Formal Analysis, Validation, Writing – review & editing. QW: Formal Analysis, Validation, Visualization, Writing – review & editing. HB: Conceptualization, Investigation, Methodology, Resources, Software, Visualization, Writing – review & editing. YZ: Funding acquisition, Project administration, Supervision, Writing – review & editing.
Funding
The author(s) declare that no financial support was received for the research and/or publication of this article.
Acknowledgments
All the authors are thankful to the second affiliated hospital of Shantou University Medical College, Shantou China for supporting this work.
Conflict of interest
The authors declare no conflict of interest. Author Contributions risk to individuals. All data will be handled confidentially per ethical and regulatory guidelines.
Generative AI statement
The author(s) declare that no Generative AI was used in the creation of this manuscript.
Publisher’s note
All claims expressed in this article are solely those of the authors and do not necessarily represent those of their affiliated organizations, or those of the publisher, the editors and the reviewers. Any product that may be evaluated in this article, or claim that may be made by its manufacturer, is not guaranteed or endorsed by the publisher.
Supplementary material
The Supplementary Material for this article can be found online at: https://www.frontiersin.org/articles/10.3389/fcimb.2025.1549470/full#supplementary-material
References
Ahmed, E. F., Rasmi, A. H., Darwish, A. M., and Gad, G. F. M. (2023). Prevalence and resistance profile of bacteria isolated from wound infections among a group of patients in upper Egypt: a descriptive cross-sectional study. BMC Res. Notes 16, 106. doi: 10.1186/s13104-023-06379-y
Alav, I., Sutton, J. M., and Rahman, K. M. (2018). Role of bacterial efflux pumps in biofilm formation. J. Antimicrob Chemother. 73, 2003–2020. doi: 10.1093/jac/dky042
Alfei, S. and Schito, A. M. (2022). β-lactam antibiotics and β-lactamase enzymes inhibitors, part 2: our limited resources. Pharmaceuticals 15, 476. doi: 10.3390/ph15040476
Alhussain, F. A., Yenugadhati, N., Al Eidan, F. A., Al Johani, S., and Badri, M. (2021). Risk factors, antimicrobial susceptibility pattern and patient outcomes of Pseudomonas aeruginosa infection: A matched case-control study. J. Infection Public Health 14, 152–157. doi: 10.1016/j.jiph.2020.11.010
Bilal, H., Shafiq, M., Hou, B., Islam, R., Khan, M. N., Khan, R. U., et al. (2022). Distribution and antifungal susceptibility pattern of Candida species from mainland China: A systematic analysis. Virulence 13, 1573–1589. doi: 10.1080/21505594.2022.2123325
Bonilla, F. A. and Oettgen, H. C. (2010). Adaptive immunity. J. Allergy Clin. Immunol. 125, S33–S40. doi: 10.1016/j.jaci.2009.09.017
Califf, R. M. (2018). Biomarker definitions and their applications. Exp. Biol. Med. (Maywood) 243, 213–221. doi: 10.1177/1535370217750088
Ch’ng, J.-H., Chong, K. K., Lam, L. N., Wong, J. J., and Kline, K. A. (2019). Biofilm-associated infection by enterococci. Nat. Rev. Microbiol. 17, 82–94. doi: 10.1038/s41579-018-0107-z
Chang, S., Sievert, D. M., Hageman, J. C., Boulton, M. L., Tenover, F. C., Downes, F. P., et al. (2003). Infection with vancomycin-resistant Staphylococcus aureus containing the vanA resistance gene. N Engl. J. Med. 348, 1342–1347. doi: 10.1056/NEJMoa025025
Chen, H., Mei, S., Zhou, Y., and Dai, J. (2024). Systematic review and meta-analysis of the diagnostic accuracy of inflammatory markers for infected diabetic foot ulcer. J. Tissue Viability 33, 598–607. doi: 10.1016/j.jtv.2024.09.007
Chong, Y., Shimoda, S., and Shimono, N. (2018). Current epidemiology, genetic evolution and clinical impact of extended-spectrum β-lactamase-producing Escherichia coli and Klebsiella pneumoniae. Infection Genet. Evol. 61, 185–188. doi: 10.1016/j.meegid.2018.04.005
Clinical and Laboratory Standards Institute (CLSI) (2007). Performance Standards for Antimicrobial Susceptibility Testing. 17th ed (Wayne, PA: Clinical and Laboratory Standards Institute).
Clinical and Laboratory Standards Institute (CLSI) (2024). Performance Standards for Antimicrobial Susceptibility Testing. 34th ed (Clinical and Laboratory Standards Institute). Available at: https://production-forum-ui.frontiersin.org/articles/1549470/files.
Diallo, O. O., Baron, S. A., Abat, C., Colson, P., Chaudet, H., and Rolain, J.-M. (2020). Antibiotic resistance surveillance systems: A review. J. Global Antimicrobial Resistance 23, 430–438. doi: 10.1016/j.jgar.2020.10.009
Dong, W., Nie, L. J., Wu, M. J., Xie, T., Liu, Y. K., Tang, J. J., et al. (2019). WoundCareLog APP - A new application to record wound diagnosis and healing. Chin J. Traumatol 22, 296–299. doi: 10.1016/j.cjtee.2019.07.003
Du, F., Ma, J., Gong, H., Bista, R., Zha, P., Ren, Y., et al. (2022). Microbial infection and antibiotic susceptibility of diabetic foot ulcer in China: literature review. Front. Endocrinol. 13, 881659. doi: 10.3389/fendo.2022.881659
Effah, C. Y., Sun, T., Liu, S., and Wu, Y. (2020). Klebsiella pneumoniae: an increasing threat to public health. Ann. Clin. Microbiol. antimicrobials 19, 1–9. doi: 10.1186/s12941-019-0343-8
Eichenberger, E. M. and Thaden, J. T. (2019). Epidemiology and mechanisms of resistance of extensively drug resistant gram-negative bacteria. Antibiotics (Basel) 8 (2), 37. doi: 10.3390/antibiotics8020037
Falanga, V., Isseroff, R. R., Soulika, A. M., Romanelli, M., Margolis, D., Kapp, S., et al. (2022). Chronic wounds. Nat. Rev. Dis. Primers 8, 50. doi: 10.1038/s41572-022-00377-3
Fazli, M., Bjarnsholt, T., Kirketerp-Møller, K., Jørgensen, B., Andersen, A. S., Krogfelt, K. A., et al. (2009). Nonrandom distribution of Pseudomonas aeruginosa and Staphylococcus aureus in chronic wounds. J. Clin. Microbiol. 47, 4084–4089. doi: 10.1128/JCM.01395-09
Gadepalli, R., Dhawan, B., Sreenivas, V., Kapil, A., Ammini, A., and Chaudhry, R. (2006). A clinico-microbiological study of diabetic foot ulcers in an Indian tertiary care hospital. Diabetes Care 29, 1727–1732. doi: 10.2337/dc06-0116
Gardner, S. E., Frantz, R. A., and Doebbeling, B. N. (2001). The validity of the clinical signs and symptoms used to identify localized chronic wound infection. Wound Repair Regener. 9, 178–186. doi: 10.1046/j.1524-475x.2001.00178.x
Gauba, A. and Rahman, K. M. (2023). Evaluation of antibiotic resistance mechanisms in gram-negative bacteria. Antibiotics (Basel) 12 (11), 1590. doi: 10.3390/antibiotics12111590
Ghosh, A. K., Unruh, M. A., Ibrahim, S., and Shapiro, M. F. (2022). Association between patient diversity in hospitals and racial/ethnic differences in patient length of stay. J. Gen. Internal Med. 37, 723–729. doi: 10.1007/s11606-021-07239-w
Gjødsbøl, K., Skindersoe, M. E., Christensen, J. J., Karlsmark, T., Jørgensen, B., Jensen, A. M., et al. (2012). No need for biopsies: comparison of three sample techniques for wound microbiota determination. Int. Wound J. 9, 295–302. doi: 10.1111/j.1742-481X.2011.00883.x
Gowda, B., Mohanto, S., Singh, A., Bhunia, A., Abdelgawad, M., Ghosh, S., et al. (2023). Nanoparticle-based therapeutic approaches for wound healing: a review of the state-of-the-art. Materials Today Chem. 27, 101319. doi: 10.1016/j.mtchem.2022.101319
Guan, H., Dong, W., Lu, Y., Jiang, M., Zhang, D., Aobuliaximu, Y., et al. (2021). Distribution and antibiotic resistance patterns of pathogenic bacteria in patients with chronic cutaneous wounds in China. Front. Med. 8, 609584. doi: 10.3389/fmed.2021.609584
Haesler, E., Swanson, T., Ousey, K., and Carville, K. (2019). Clinical indicators of wound infection and biofilm: reaching international consensus. J. Wound Care 28, s4–s12. doi: 10.12968/jowc.2019.28.Sup3b.S4
Hammoudi Halat, D. and Ayoub Moubareck, C. (2020). The current burden of carbapenemases: review of significant properties and dissemination among gram-negative bacteria. Antibiotics (Basel) 9 (4), 186. doi: 10.3390/antibiotics9040186
Holmes, N. E., Johnson, P. D., and Howden, B. P. (2012). Relationship between vancomycin-resistant Staphylococcus aureus, vancomycin-intermediate S. aureus, high vancomycin MIC, and outcome in serious S. aureus infections. J. Clin. Microbiol 50, 2548–2552. doi: 10.1128/JCM.00775-12
Huszczynski, S. M., Lam, J. S., and Khursigara, C. M. (2019). The role of Pseudomonas aeruginosa lipopolysaccharide in bacterial pathogenesis and physiology. Pathogens 9, 6. doi: 10.3390/pathogens9010006
Iskandar, K., Molinier, L., Hallit, S., Sartelli, M., Hardcastle, T. C., Haque, M., et al. (2021). Surveillance of antimicrobial resistance in low-and middle-income countries: a scattered picture. Antimicrobial Resistance Infection Control 10, 1–19. doi: 10.1186/s13756-021-00931-w
Lakhey, M. and Bhatt, C. (2007). The distribution of pathogens causing wound infection and their antibiotic susceptibility pattern. J. Nepal Health Res. Council. Available at: https://hdl.handle.net/20.500.14356/2100
Lindley, L. E., Stojadinovic, O., Pastar, I., and Tomic-Canic, M. (2016). Biology and biomarkers for wound healing. Plast Reconstr Surg. 138, 18s–28s. doi: 10.1097/PRS.0000000000002682
Mahmoud, N. N., Hamad, K., Al Shibitini, A., Juma, S., Sharifi, S., Gould, L., et al. (2024). Investigating inflammatory markers in wound healing: Understanding implications and identifying artifacts. ACS Pharmacol. Trans. Sci. 7, 18–27. doi: 10.1021/acsptsci.3c00336
Maillard, J. Y., Kampf, G., and Cooper, R. (2021). Antimicrobial stewardship of antiseptics that are pertinent to wounds: the need for a united approach. JAC Antimicrob Resist. 3, dlab027. doi: 10.1093/jacamr/dlab027
Nagshetty, K., Shilpa, B., Patil, S. A., Shivannavar, C., and Manjula, N. (2021). An overview of extended spectrum beta lactamases and metallo beta lactamases. Adv. Microbiol. 11, 37. doi: 10.4236/aim.2021.111004
Oshiokhayamhe, I. K. (2023). A review on penicillinase production and beta-lactamase activity on commonly isolated bacteria. Mathews J. Immunol. Allergy 7, 1–10. doi: 10.30654/MJIA.10020
Parastan, R., Kargar, M., Solhjoo, K., and Kafilzadeh, F. (2020). Staphylococcus aureus biofilms: Structures, antibiotic resistance, inhibition, and vaccines. Gene Rep. 20, 100739. doi: 10.1016/j.genrep.2020.100739
Prescott, S. L., Larcombe, D.-L., Logan, A. C., West, C., Burks, W., Caraballo, L., et al. (2017). The skin microbiome: impact of modern environments on skin ecology, barrier integrity, and systemic immune programming. World Allergy Organ. J. 10, 1–16. doi: 10.1186/s40413-017-0160-5
Puca, V., Marulli, R. Z., Grande, R., Vitale, I., Niro, A., Molinaro, G., et al. (2021). Microbial species isolated from infected wounds and antimicrobial resistance analysis: Data emerging from a three-years retrospective study. Antibiotics 10, 1162. doi: 10.3390/antibiotics10101162
Rahman, M. M., Alam Tumpa, M. A., Zehravi, M., Sarker, M. T., Yamin, M., Islam, M. R., et al. (2022). An overview of antimicrobial stewardship optimization: the use of antibiotics in humans and animals to prevent resistance. Antibiotics 11, 667. doi: 10.3390/antibiotics11050667
Rigby, K. M. and Deleo, F. R. (2012). Neutrophils in innate host defense against Staphylococcus aureus infections. Semin. Immunopathol 34, 237–259. doi: 10.1007/s00281-011-0295-3
Serban, D., Papanas, N., Dascalu, A. M., Kempler, P., Raz, I., Rizvi, A. A., et al. (2024). Significance of neutrophil to lymphocyte ratio (NLR) and platelet lymphocyte ratio (PLR) in diabetic foot ulcer and potential new therapeutic targets. Int. J. Lower Extremity Wounds 23, 205–216. doi: 10.1177/15347346211057742
Serra, R., Grande, R., Butrico, L., Rossi, A., Settimio, U. F., Caroleo, B., et al. (2015). Chronic wound infections: the role of Pseudomonas aeruginosa and Staphylococcus aureus. Expert Rev. anti-infective Ther. 13, 605–613. doi: 10.1586/14787210.2015.1023291
Siddiqui, A. R. and Bernstein, J. M. (2010). Chronic wound infection: facts and controversies. Clin. Dermatol. 28, 519–526. doi: 10.1016/j.clindermatol.2010.03.009
Stevens, D. L. (2009). Treatments for skin and soft-tissue and surgical site infections due to MDR Gram-positive bacteria. J. Infection 59, S32–S39. doi: 10.1016/S0163-4453(09)60006-2
Strbo, N., Yin, N., and Stojadinovic, O. (2014). Innate and adaptive immune responses in wound epithelialization. Adv. Wound Care 3, 492–501. doi: 10.1089/wound.2012.0435
Tooke, C. L., Hinchliffe, P., Bragginton, E. C., Colenso, C. K., Hirvonen, V. H., Takebayashi, Y., et al. (2019). β-lactamases and β-lactamase inhibitors in the 21st century. J. Mol. Biol. 431, 3472–3500. doi: 10.1016/j.jmb.2019.04.002
Wang, C., Niu, X., Bao, S., Shen, W., and Jiang, C. (2024). Distribution patterns and antibiotic resistance profiles of bacterial pathogens among patients with wound infections in the jiaxing region from 2021 to 2023. Infection Drug Resistance. 17, 2883–2896. doi: 10.2147/IDR.S470401
Wong, S. Y., Manikam, R., and Muniandy, S. (2015). Prevalence and antibiotic susceptibility of bacteria from acute and chronic wounds in Malaysian subjects. J. Infection Developing Countries 9, 936–944. doi: 10.3855/jidc.5882
Yang, J., Long, H., Hu, Y., Feng, Y., Mcnally, A., and Zong, Z. (2022). Klebsiella oxytoca complex: update on taxonomy, antimicrobial resistance, and virulence. Clin. Microbiol. Rev. 35, e00006–e00021. doi: 10.1128/CMR.00006-21
Zahorec, R. (2021). Neutrophil-to-lymphocyte ratio, past, present and future perspectives. Bratisl Lek Listy 122, 474–488. doi: 10.4149/BLL_2021_078
Keywords: microorganisms, wound infections, biomarkers, antibiotics resistance, China
Citation: Khan S, Hou B, Khan MN, Shafiq M, Cai L, Fang W, Wang Q, Bilal H and Zeng Y (2025) A 3-year retrospective analysis of microbial species and key biomarkers associated with wound infections in Shantou Hospital, China. Front. Cell. Infect. Microbiol. 15:1549470. doi: 10.3389/fcimb.2025.1549470
Received: 21 December 2024; Accepted: 28 April 2025;
Published: 19 May 2025.
Edited by:
Namdev Shivaji Togre, Temple University, United StatesReviewed by:
Poonam Mudgil, Charles Sturt University, AustraliaPrakash Daniel Nallathamby, University of Notre Dame, United States
Copyright © 2025 Khan, Hou, Khan, Shafiq, Cai, Fang, Wang, Bilal and Zeng. This is an open-access article distributed under the terms of the Creative Commons Attribution License (CC BY). The use, distribution or reproduction in other forums is permitted, provided the original author(s) and the copyright owner(s) are credited and that the original publication in this journal is cited, in accordance with accepted academic practice. No use, distribution or reproduction is permitted which does not comply with these terms.
*Correspondence: Hazrat Bilal, YmlsYWwubWljcm9iaW9sb2dpc3RAeWFob28uY29t; Yuebin Zeng, emVuZ195YkAxNjMuY29t
†These authors have contributed equally to this work