- 1Department of Dermatovenereology, Tianjin Medical University General Hospital/Tianjin Institute of Sexually Transmitted Disease, Tianjin, China
- 2Shanghai Institute of Virology, Shanghai Jiao Tong University School of Medicine, Shanghai, China
- 3Department of Obstetrics and Gynecology, Hunan Provincial Maternal and Child Health Care Hospital, Changsha, Hunan, China
- 4Department of Microbiology, Immunology and Molecular Genetics, University of Texas Health Science Center at San Antonio, Texas, TX, United States
Introduction: Chlamydia trachomatis (CT) is a major sexually transmitted pathogen with severe complications. Using Chlamydia muridarum (CM) as a model, this study evaluates the attenuated mutant Chlamydia muridarum (CM-pGP3S) as a novel rectal vaccine to protect against genital tract infection and pathology.
Methods: Female C57BL/6 mice were rectally immunized with low (1×103), middle (1×105), or high (1×107) doses of CM-pGP3S. Mice were challenged intravaginally with wild-type Chlamydia muridarum 63 days post-immunization. Protection was assessed via genital Chlamydia shedding, hydrosalpinx incidence (gross/histopathology), serum IgG, fecal IgA, and T cell responses. Gut microbiota stability was analyzed using qPCR.
Results: CM-pGP3S immunization significantly reduced CM-WT genital shedding duration (3–7 days vs. 21 days in controls, p < 0.01) and hydrosalpinx incidence (0% vs. 80% in controls, p < 0.01). Elevated systemic and mucosal immunity were observed, including higher serum IgG (1:100–1:1600 dilutions, p < 0.05–0.01) and fecal IgA (p < 0.05–0.01). CD4+ and CD8+ T cells exhibited increased IFN-γ (p < 0.01), while CD8+ T cells showed elevated TNF-α and IL-2 (p < 0.05). No colitis or significant gut microbiota disruption occurred post-immunization.
Discussion: Rectal CM-pGP3S vaccination induces robust transmucosal immunity, protecting against genital Chlamydia infection and pathology without gastrointestinal adverse effects. This highlights its potential as a safe and effective mucosal vaccine strategy to combat CT genital tract infections.
1 Introduction
Chlamydia trachomatis (CT) genital infection is a prevalent sexually transmitted disease globally (Workowski et al., 2021). A systematic review of 100 studies estimated CT’s global prevalence at approximately 3.8% among women aged 15 to 49 years in 2016 (Hu et al., 2021). The clinical symptoms of CT genital infection are often mild and insidious, with 70-95% of men and 45-96% of women being asymptomatic (Dielissen et al., 2013). Chronic CT infection, however, can lead to severe complications, including epididymitis, prostatitis, and proctitis in men, and cervicitis, urethritis, pelvic inflammatory disease, and ectopic pregnancy in women. In severe cases, it can result in infertility (Mohseni et al., 2025). Treatment failures are common due to factors such as inappropriate dosage, improper drug selection, and insufficient treatment duration, complicating CT management (Kong and Hocking, 2015). Although much progress has been made in understanding CT infection, developing an effective vaccine remains one of the most promising approaches for CT prevention and control (Poston et al., 2019).
Research on Chlamydia vaccines has gained attention since the 1950s, leading to the development of Deoxyribonucleic acid (DNA), subunit, and live attenuated vaccines (McIntosh, 2020). Vaccine delivery systems, novel adjuvants, and delivery methods have also played key auxiliary roles in Chlamydia vaccine research (Borges et al., 2022). A protective vaccine targeting the major outer membrane protein of Chlamydia has entered early clinical trials, but no Chlamydia vaccine has yet been approved for human use, making further research essential (de la Maza et al., 2021). Previous vaccines have primarily used vaginal or intramuscular administration, which can cause discomfort and inconvenience for patients and healthcare providers. Mucosal immunization, which leverages the common mucosal immune system to elicit immune responses at various mucosal sites, presents a practical alternative. This approach can stimulate Immunoglobulin (Ig) A production to block pathogen invasion (Li et al., 2020). The intestinal mucosa, a primary site for foreign pathogen entry, is particularly suited for vaccine delivery (Azegami et al., 2014). This method is straightforward and safe, bypassing stringent vaccination standards and facilitating implementation (Li et al., 2020). Since CT is often detected in the digestive tract after genital tract infection in females (Zhong, 2018; Dirks et al., 2019), gastrointestinal vaccination against Chlamydia may represent a convenient and effective new transmucosal vaccination strategy (Morrison et al., 2020).
While the medical significance of CT in the human digestive tract remains unclear, studies have shown that Chlamydia muridarum (CM) can survive and colonize the mouse digestive tract, thereby reducing genital CM infection and upper genital tract pathology (Wang et al., 2018; Zhong, 2018). CM causes urogenital infections in mice, mirroring human CT infections, making the CM mouse genital tract model widely used for studying CT pathogenesis and immune protection (Lundy et al., 2021). Previous research has demonstrated that administering CM-wild-type (CM-WT) orally in the upper digestive tract can provide substantial transmucosal protection, reducing the severity and duration of CM genital infection and related hydrosalpinx pathology (Wang et al., 2018). Colonization of CM-WT in the upper digestive tract did not result in significant intestinal pathology, noticeable epithelial inflammation, or major shifts in gut flora (Yeruva et al., 2013; Ma et al., 2020). To enhance vaccine safety, an attenuated CM strain lacking plasmid-encoded Glycoprotein 3 (with a premature stop codon in the pgp3 gene, termed CM-pGP3S) has been evaluated in mice (Hou et al., 2018). Oral administration of CM-pGP3S successfully induced robust transmucosal immunity against CM-WT genital infection and pathogenicity (Zhou et al., 2022).
However, it remains uncertain whether oral administration is the optimal route. Rectal administration may offer superior protection. Several reasons could account for rectal immunization being superior to oral immunization. Oral vaccines may be inactivated by gastric acid or digestive enzymes, and PGP3S-CM cannot be found in the digestive tract when degraded (Zhang et al., 2019), so the immune effect may be poor. Rectal administration can bypass the degradation of the upper gastrointestinal tract and improve the stability of the vaccine. Two studies showed the results of a medium-dose oral vaccine (Wang et al., 2018; Zhou et al., 2022), but the present study investigated whether a low-dose CM-pGP3S rectal vaccine could induce a strong immune protection effect in the vagina. Furthermore, it has been reported that humoral and cellular immunity can be induced by rectal inoculation (Pais et al., 2017; Varotto-Boccazzi et al., 2023).
This study explores whether rectal inoculation with the CM-pGP3S vaccine could generate transmucosal immune responses that protect mice against subsequent CM-WT genital infection and associated pathology. Our findings suggest that CM-pGP3S may be developed into a safe rectal vaccine to induce protective immunity in the female genital tract.
2 Materials and methods
2.1 Animals
All animals were housed in a pathogen-free environment and provided food ad libitum. A total of 80 six-week-old female C57BL/6 mice obtained from Beijing Charles River Laboratories were used in this study. Although it is known that this strain of mice may clear infection faster and show a lower baseline pathological level, the model is identical to that of C57BL/6 mice in previous studies using CM oral vaccine (Wang et al., 2018; Zhou et al., 2022). The mice were maintained under specific pathogen-free (SPF) conditions at Tianjin Medical University General Hospital’s animal facility, with controlled temperature (22 ± 1°C) and relative humidity (55 ± 5%). They were allowed a 7-day acclimation period before the experiment.
All procedures for the care and use of animals were approved by the Ethics Committee of Tianjin Medical University General Hospital (IRB2022-DWFL-129), and all institutional and governmental regulations regarding the ethical use of animals were followed. All immunizations, challenges, and surgery were performed under 2% isoflurane, and all efforts were made to minimize suffering.
2.2 Cell culture
HeLa cells were provided by the Tianjin Institute of Sexually Transmitted Diseases. CM and rabbit anti-CM serum antibodies were kindly donated by Professor Guangming Zhong from the Microbial Immunology Department of the University of Texas Health Medical Center in San Antonio.
2.3 Chlamydia organism vaccines
The vaccine candidate used in this investigation consisted of CM with a premature stop codon in the pGP3 gene to create CM-pGP3S, as previously detailed (Hou et al., 2018). CM strains, including CM-WT and CM-pGP3S, were generously provided by Dr. Guangming Zhong’s lab at the University of Texas Health Science Center in San Antonio, USA. These strains had been previously titrated on HeLa cell monolayers, purified via renografin gradients, and stored at -80°C. All aliquots of the purified Elementary Bodies (EBs) were stored at -80°C until use.
2.4 Mice rectal immunization and vaginal challenge infection
Purified CM EBs were used to inoculate 6-week-old female C57BL/6J mice (Beijing Charles River Laboratories) rectally (as the immunization route) or intravaginally (as the challenge infection route) as previously described (Wang et al., 2018; Zhou et al., 2022). The EBs and RBs of Chlamydia were purified from cell cultures by density gradient centrifugation using Gastrografin® and K-36 buffer. The steps involved centrifuging a partially purified EBs/RBs sample in a 20% Gastrografin gradient, followed by further separation in a 34%, 44%, and 54% Gastrografin discontinuous gradient, and finally collecting an EBs-enriched fraction from the 44%/54% interface (Klasinc et al., 2021). Then, 50 μL of the coated solution containing 1×105 inclusion forming units (IFUs) CM-EBs was added to each well of the ELISA plate. Live CM-EBs were used for packet encapsulation. Three doses of CM-pGP3S (1×10³, 1×105, and 1×107 IFUs) were administered rectally. Subsequently, mice were challenged intravaginally with 2×105 IFUs of CM-WT to assess CM-pGP3S protection, in line with prior studies (Wang et al., 2018; Zhou et al., 2022). To evaluate long-term protection, an intravaginal challenge was conducted 63 days post-rectal CM-pGP3S inoculation. The mice’s health status and infection levels were monitored by enumerating Chlamydia IFUs from vaginal and/or rectal swabs using indirect immunofluorescence. The mean number of IFUs at each time point was calculated. At the end of the experiment, mice were deeply anesthetized, and blood, feces, and the entire genital tract were collected for further research.
2.5 Titration of live Chlamydia organisms
To quantify live Chlamydia organisms in vaginal or rectal swabs, each swab was soaked in 0.5 mL of sucrose-phosphate-glutamate (SPG) buffer, vortexed with glass beads, and the released Chlamydia organisms in the supernatant were titrated on HeLa cell monolayers in duplicate. The infected cultures were processed for immunofluorescence assay as described (Liu et al., 2014). Inclusions were counted in five random fields per coverslip under a fluorescence microscope. For coverslips with fewer than one IFU per field, the entire coverslip was counted. The total number of IFUs per swab was calculated based on the mean IFUs per view, the ratio of the view area to that of the well, the dilution factor, and inoculation volumes. When possible, a mean IFUs/swab value was derived from serially diluted duplicate samples for each swab. The total IFUs/swab count was converted to log10, which was used to calculate the mean and standard deviation across mice of the same group at each time point.
2.6 Immunofluorescence assay
Briefly, infected HeLa cells grown on coverslips were fixed with 4% paraformaldehyde for 30 minutes at room temperature, followed by permeabilization with 2% (wt/vol) saponin (Sigma) for 60 minutes, then blocking. A 1:2000 dilution of rabbit anti-CM serum antibody was added and incubated at 37°C for 1 hour. Next, a 1:200 dilution of secondary fluorescent Fluorescein Isothiocyanate (FITC)-labeled goat anti-rabbit IgG antibody and a 1:1000 dilution of Hoechst blue fluorescent dye were added. Rabbit antibodies against CM, along with a secondary antibody conjugated with Cyanine Dyes (Cy) 2 (green; Abnova), were used to mark Chlamydia inclusions. Immunofluorescence images were obtained using a confocal laser scanning microscope (Carl Zeiss Microscopy GmbH-Axio Imager A2, Germany).
2.7 Gross and histopathological analysis of the genital tract after CM-pGP3S rectal inoculation
After the CM-WT intravaginal challenge, mice were euthanized to assess genital tract pathology, focusing on upper genital tract hydrosalpinx. Prior to removing the genital tract tissues, a gross examination was performed to detect oviduct hydrosalpinx or related abnormalities. The genital tract tissues were isolated, placed on a blue background, and imaged. Oviduct hydrosalpinx was visually scored based on dilation size using a scoring system as previously described (Conrad et al., 2016). Mice with hydrosalpinx on either side of the oviducts were classified as hydrosalpinx positive, and the severity of hydrosalpinx was scored as follows (Conrad et al., 2016): 0, no oviduct dilation (no hydrosalpinx); 1, hydrosalpinx visible only under a stereoscope; 2, hydrosalpinx visible to the naked eye but smaller than the ovary; 3, hydrosalpinx equal to the ovary in size; 4, hydrosalpinx larger than the ovary. Scores from both oviducts in each mouse were combined as the total pathology score. Hydrosalpinx incidence and severity scores were statistically analyzed between mice infected with CM-WT. Hydrosalpinx incidence was calculated as the number of mice with a bilateral score of 1 or higher divided by the total number of mice in the group.
2.8 Hematoxylin and eosin staining
For histological pathology observation, isolated genital tract tissues were fixed in 10% neutral formalin, embedded in paraffin, and sectioned longitudinally. Efforts were made to include the cervix, uterine horns, and oviducts, as well as luminal structures, in each section. The sections were stained with Hematoxylin and Eosin (H&E) as previously described (Conrad et al., 2016). H&E-stained sections were examined under a microscope for inflammation severity and pathology, following modified, established schemes. The severity of oviduct inflammatory infiltration was semi-quantitatively scored based on the following criteria (Conrad et al., 2016): 0, no significant inflammatory infiltration; 1, infiltration at one focus; 2, infiltration at two to four foci; 3, infiltration at more than four foci; 4, confluent infiltration. Images of each mouse’s oviduct dilation and inflammatory infiltration were taken under a 10X objective lens.
2.9 Gross and histopathological analysis of the lower digestive tract after CM-pGP3S rectal inoculation
On day 63 post-CM-pGP3S rectal immunization, mice in the high-dose group (1×107) were euthanized to evaluate inflammation in the lower digestive tract. The tissues were isolated, laid on a blue background for imaging, and measured for length to assess potential shortening due to inflammation. Histopathological analysis of these tissues was conducted as described above.
2.10 Quantitative PCR
Fecal samples were collected from female C57BL/6J mice on various days after rectal immunization, with or without CM-pGP3S. Baseline fecal samples were collected from all mice prior to inoculation and designated as day 0 samples. Samples were frozen in liquid nitrogen and stored at -80°C until DNA extraction. Total genomic DNA was extracted from each sample (150 mg) using a QIAamp DNA stool mini kit (Qiagen Inc., Germantown, MD) per the manufacturer’s instructions. The DNA purity was assessed by determining absorbance ratios at 260 nm/280 nm. Real-time quantitative Polymerase Chain Reaction (qPCR) using a SYBR green-based method with iTaq universal SYBR green super mix 500 (Bio-Rad) quantified bacterial 16S ribosomal Ribonucleic Acid (rRNA) genes. The primers used were identical to those in previous studies (Wang et al., 2018). PCR conditions were 95°C for 15 s and 60°C for 30 s, repeated for 45 cycles. The number of PCR cycles required for a given amplicon level was used to calculate the relative abundance of the targeted template.
2.11 Enzyme-linked immunosorbent assays
Levels of CM-specific antibodies (IgG and IgA) in sera and fecal samples collected 63 days post-high-dose CM-pGP3S rectal inoculation (1×107) were measured by standard ELISA procedures (Wang et al., 2018). A 50 µL coating solution containing activated CM EBs (1×105) in a 50 mM carbonate/bicarbonate buffer was added to each well of a 96-well ELISA plate, followed by overnight incubation at 4°C. Plates were washed five times with phosphate-buffered saline (PBS) containing 0.05% Tween-20 for 3 minutes each. Plates were blocked with PBS containing 5% skim milk and incubated at room temperature for 2 hours. Serum samples collected from the tail vein were serially diluted 1:100 in PBS and incubated at 4°C for 2 hours. Fecal samples were resuspended in PBS to a final concentration of 1 mg/µL, centrifuged at 13000 rpm for 5 minutes, and supernatants were applied to 96-well plates with or without 2-fold serial dilutions. Horseradish peroxidase-labeled goat anti-mouse IgG (1:500) and IgA (1:1000) antibodies (Dingguo Biotechnology Co.) were added and incubated at 37°C for 1 hour. Absorbance Optical Density (OD) at 405 nm was measured using the 2,2’-Azinobis-(3-ethylbenzthiazoline-6-sulfonic acid) diammonium salt (ABTS) substrate.
2.12 Flow cytometry
Flow cytometry was used to monitor changes in T-cell inflammatory factors in mice. Dendritic cells were exposed to CM overnight based on a previously described method (Lu and Zhong, 1999). Dendritic cells were mixed with CM at an infection ratio of 10:1 (MOI=10) to allow full contact of EBs with dendritic cells, and the mixed suspension was then added into 24-well plates and incubated overnight at 37°C without centrifugation. Because centrifugation may interfere with the natural phagocytosis function of dendritic cells or pathogen structure, direct mixing is closer to the process of antigen uptake under physiological conditions. A high MOI (e.g., MOI=10) ensured that the EBs were in full contact with the dendritic cells without the need for additional centrifugation steps. Spleen cells from CM-pGP3S (1×107) rectally inoculated mice were collected and incubate with EBs-pulsed dendritic cells to be used to present CM antigens to T cells overnight. After overnight in vitro antigen re-stimulation (10–14 hours), the Golgi stop protein transport inhibitor was added to the culture at a final concentration of 25 µg/mL for 6 hours. To identify T cell subsets and detect intracellular inflammatory factors, lymphocytes cultured in vitro were blocked with rat anti-mouse Fc receptor (Cluster of Differentiation (CD) 16/CD32) antibodies. Cells were then labeled with anti-CD4 and anti-CD8 antibodies, followed by antibodies against Tumor Necrosis Factor (TNF) -α, Interferon (IFN) -γ, Interleukin (IL) -5, and IL-2. After incubation, cells stained with specific antibody combinations were counted using Laser-based Sorting and Research Instrument II (LSRII) flow cytometry (BD Bioscience), as previously described. Data analysis was conducted using FlowJo software (FlowJo, LLC).
2.13 Statistical analysis
All statistical analyses were performed using Statistical Package for the Social Sciences (SPSS) Statistics 27 software (IBM Corp., USA). One-way analysis of variance (ANOVA) was used for comparisons among three or more groups. Differences between the two groups were compared using an unpaired Student’s t-test. Differences were considered statistically significant at p < 0.05.
3 Results
3.1 Effect of vaginal infection of CM-WT and CM-pGP3S on genital tract pathology
Mice were infected vaginally with CM-WT and CM-pGP3S at 2×105 IFUs. After 63 days, 80% of mice infected with CM-WT exhibited severe oviduct hydrosalpinx, with a gross tubal score of 4.40 ± 2.96. However, no hydrosalpinx was induced in mice infected with CM-pGP3S (p < 0.01) (Figure 1A). Tubal histopathology, verified by microscopy, showed severe chronic inflammatory cell infiltration in oviduct tissue of CM-WT-infected mice (inflammatory score 4.00 ± 2.55), while no inflammatory cell infiltration was evident in CM-pGP3S-treated mice (p < 0.01) (Figure 1B). There was a statistically significant difference in the scores of hydrosalpinx and oviduct inflammatory between the two group (p < 0.01) (Figure 1C). These findings align with the gross pathology results, indicating that CM-pGP3S caused no pathological changes in the genital tract compared to CM-WT.
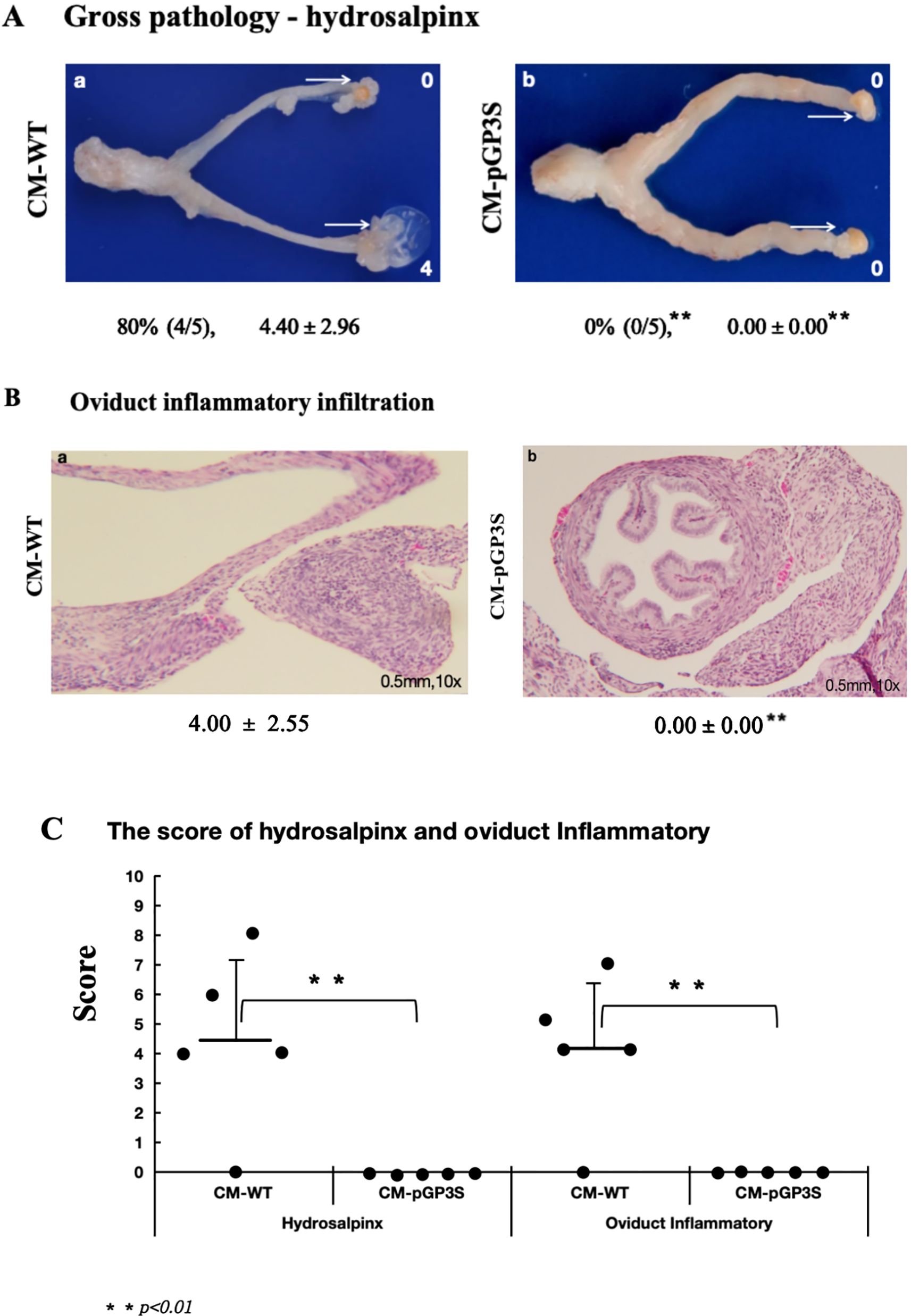
Figure 1. Effect of vaginal infection of CM-WT and CM-pGP3S on genital tract pathology. (A, B) C57BL/6J mice were infected vaginally with CM-WT (n=5) and CM-pGP3S (n=5) at 2×10^5 IFUs (Inclusion Forming Units). All mice were sacrificed after 63 days, and gross pathology and histopathological analyses of the genital tract were performed. (C) CM-WT induced significant hydrosalpinx and inflammatory changes in oviduct tissue under a 10x objective lens, while CM-pGP3S did not cause any pathological changes. The difference was statistically significant (**p<0.01).
3.2 CM-pGP3S rectal immunization induces transmucosal protection against vaginal challenge infection by CM-WT
Rectal immunization with varying doses of CM-pGP3S in the gastrointestinal tract reduced CM-WT infection in the genital tract following vaginal challenge. CM-WT infections were monitored by the weekly collection of vaginal-cervical swabs from individual mice, with Chlamydia IFUs enumerated. Rectal immunization with low (1×103), middle (1×105), and high (1×107) doses of CM-pGP3S significantly reduced the intensity and duration of CM-WT genital shedding (Figure 2A). Immunized mice challenged vaginally with CM-WT exhibited genital infection for only 3 to 7 days, compared to 21 days in the control group (non-immunized mice), with substantial reductions in genital shedding (p < 0.01). By day 14 post-challenge, CM-WT organisms were undetectable in immunized mice, whereas control mice exhibited persistent shedding until day 21 (Figure 2A).
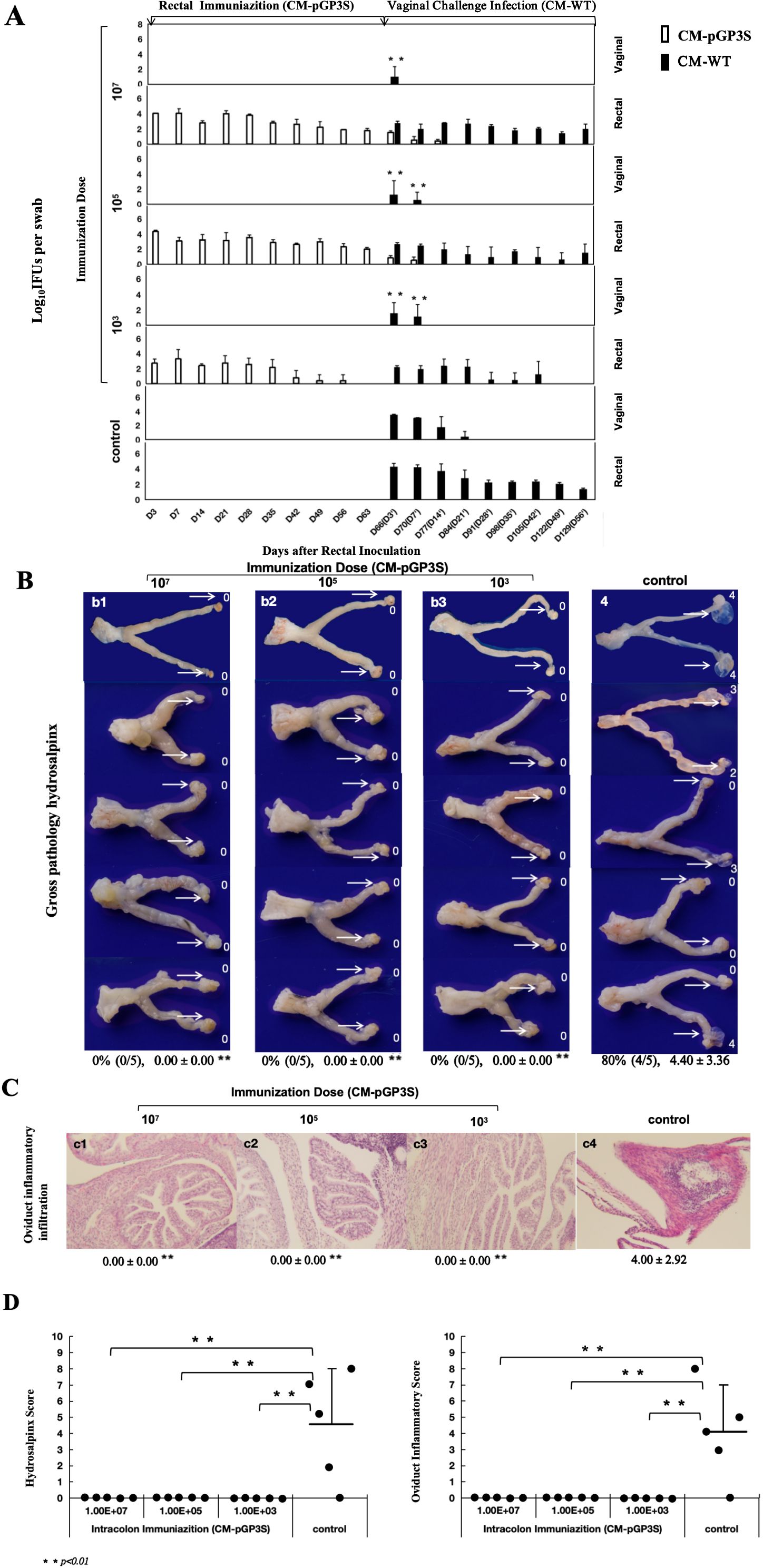
Figure 2. Effect of rectal immunization with attenuated CM-pGP3S vaccine on infectivity and pathogenicity against CM-WT vaginal challenge. (A) Four groups of C57BL/6J mice (n=5 per group) were monitored for live Chlamydia burdens in the genital tract. Vaginal and rectal swabs were collected on days 3, 7, and weekly thereafter following immunization and challenge infection. Results, expressed as Log10 IFUs per swab, showed a significant reduction in IFU shedding in CM-pGP3S-immunized mice compared to controls on days 3 and 7 (p<0.01). (B) Macroscopic and pathological images of the entire genital tract in both control and immunized groups were analyzed. Severely dilated oviducts, easily identified in the control group, had a hydrosalpinx incidence of 80% (4/5) with an average score of 4.40 ± 3.36 (a4). In contrast, CM-pGP3S-immunized mice at low (1×10^3, a1), middle (1×10^5, a2), and high doses (1×10^7, a3) did not develop hydrosalpinx following a 56-day CM-WT vaginal challenge (0/5). Histological examination with H&E staining under a 10x objective lens showed inflammatory infiltration in the oviduct lumen and tissue (b1-b4). Both hydrosalpinx and inflammatory scores were semi-quantitatively assessed as described in the materials and methods. The three immunized groups showed significantly reduced oviduct dilation (c1: **p<0.01) and inflammatory infiltration in the upper genital tract (c2: **p<0.01).
Further evaluation of genital pathology was performed at both macroscopic (Figure 2B-a) and microscopic levels (Figure 2C). Vaginal challenge with CM-WT induced significant hydrosalpinx in 80% of the control mice, with a gross tubal score of 4.40 ± 3.36, whereas no hydrosalpinx cases were detected in the CM-pGP3S group. To validate these findings, genital tissues were stained with H&E, showing clear oviduct dilation and inflammatory infiltration in control mice with hydrosalpinx. The oviduct inflammatory score for the control group was 4.00 ± 2.92. In contrast, rectally immunized mice in the low, middle, and high-dose groups had no inflammatory score, indicating significant protection from oviduct hydrosalpinx and inflammatory infiltration induced by CM-WT vaginal challenge (Figure 2D).
3.3 The lower digestive tract immunized with CM-pGP3S is non-pathogenic
The impact of rectal CM-pGP3S vaccination on the digestive tract was evaluated through macroscopic observation, pathology, and gut microbiota analysis. After 63 days, the colons were examined carefully. No significant differences were observed in the gross appearance (Figure 3A-a) or length of the colon between mice that received Chlamydia rectal inoculation and control mice (Figure 3A-b, p > 0.05), indicating that CM-pGP3S did not cause colitis in the digestive tract.
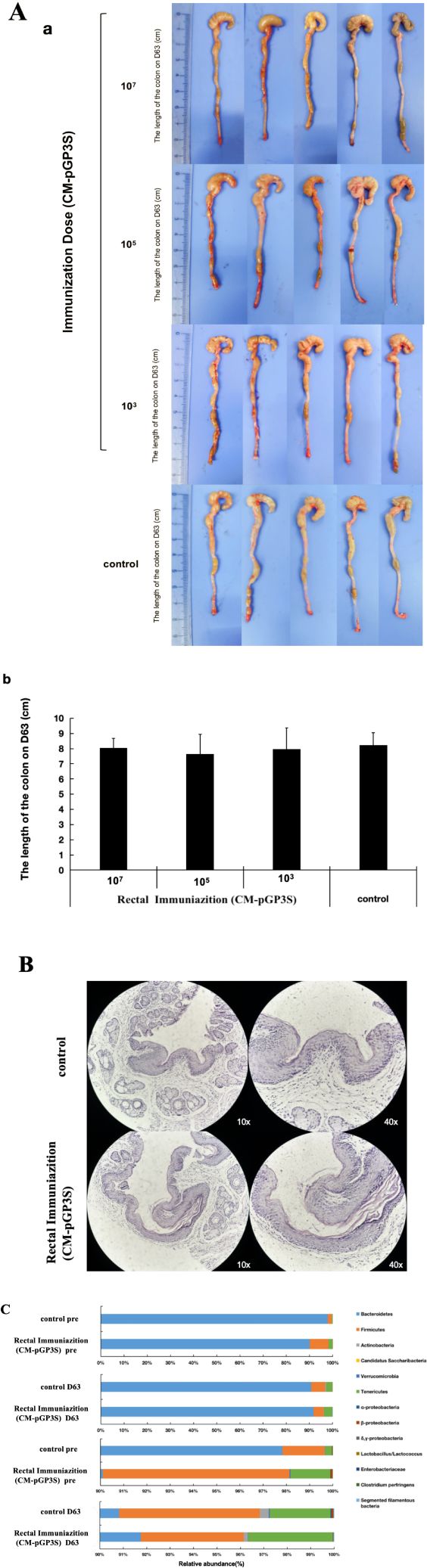
Figure 3. Effects of rectal immunization with attenuated CM-pGP3S vaccine on lower digestive tract. (A) Macroscopic images of the lower digestive tract in the three CM-pGP3S rectal immunization groups and the control group (n=5). No significant differences were observed in gross appearance (a) or colon length (b) across low (1×10^3), middle (1×10^5), high dose (1×10^7), and control groups on day 63. (B) Pathological biopsies of colonic epithelial tissue showed no significant differences between the high-dose group and controls after 63 days (n=5). (C) Analysis of intestinal flora in high-dose (n=5) and control (n=5) groups revealed stable increases in the phylum Tenericutes in all groups on day 63.
Mice that received a high dose (1×107) of CM-pGP3S underwent pathological examination, which showed that the epithelial tissue structure remained intact. Adjacent sections stained with H&E demonstrated no significant inflammatory infiltration in colon tissue between immunized and control mice (Figure 3B). We further assessed the effects of CM-pGP3S on intestinal flora (Figure 3C). Fecal samples from C57BL/6J mice that underwent high-dose CM-pGP3S rectal inoculation and control mice were analyzed by qPCR for seven major bacterial phyla, including four subclasses of the phylum Proteobacteria. Compared with the control group, the proportion of Verrucomicrobia and β-Proteobacteria in the CM-pGP3S inoculation group showed a slight decrease (Table 1, p < 0.05) on day 63. Upon closely examining the less abundant phyla, we found that the phylum Tenericutes in all groups showed a stable increase, irrespective of CM-pGP3S rectal immunization on day 63. These time-dependent changes in the intestinal flora were not significantly affected by lower digestive tract CM-pGP3S.
3.4 The humoral and cellular immunity of CM-pGP3S rectal immunization
We used CM-pGP3S to induce transmucosal immunoprotection to elicit gastrointestinal mucosal immune responses, promoting lymphocyte proliferation. As mucosal tissue contains mucosal lymphoid tissue, which produces IgA to prevent pathogen invasion, CM-pGP3S rectal immunization increased serum-specific IgG and intestinal fecal IgA levels. Recognized epitopes were displayed on the surface of CM EBs coated on ELISA plates. IgG levels were significantly higher than in the immunized group after high-dose (1×107) CM-pGP3S rectal immunization on day 63 from 1:100 to 1:1600 serum dilution, with 1:100 and 1:400 dilutions showing the highest difference (p < 0.01) and 1:200, 1:800, and 1:1600 dilutions also showing significant differences (Figure 4A, p < 0.05). Fecal IgA levels increased after high-dose (1×107) CM-pGP3S rectal immunization on day 63 from neat to 1:2 fecal dilution (Figure 4A, p < 0.01, p < 0.05). Analysis of spleen cells from mice immunized with CM-pGP3S (1×107) on day 63 via flow cytometry showed increased expression of cytokines by CD4+ and CD8+ T cells, particularly IFN-γ, a key player in mucosal immunity (Figure 4B, p < 0.01). The number of TNF-α and IL-2 expressing CD8+ T cells was also significantly higher than in the control group (Figure 4B, p < 0.05). These findings suggest that CM-pGP3S rectal immunization can stimulate the production of humoral and cellular immunity.
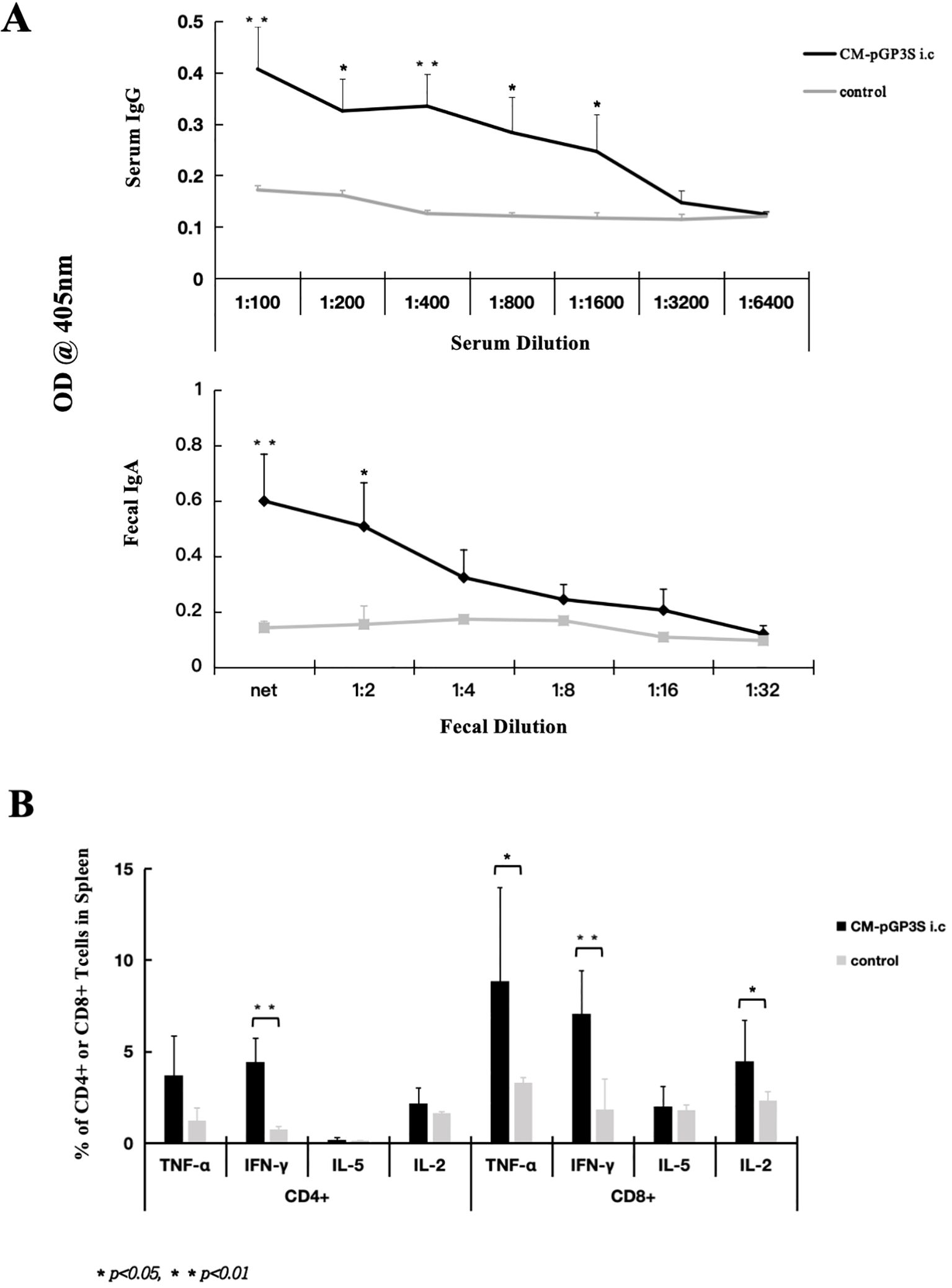
Figure 4. CM-pGP3S rectal immunization induces humoral and cellular immune responses. (A) Serum and fecal samples from mice on day 63 post-CM-pGP3S rectal immunization were tested for IgG and fecal IgA levels. The vaccine significantly increased serum IgG and intestinal IgA levels. Serum IgG levels at 1:100 and 1:400 dilutions were significantly higher than in controls (**p<0.01), with additional differences at 1:200, 1:800, and 1:1600 dilutions (p<0.05). Fecal IgA levels also increased compared to controls at net to 1:2 fecal dilutions (**p<0.01, *p<0.05). (B) Cytokine analysis of spleen CD4+ and CD8+ T cells on day 63 post-rectal immunization with CM-pGP3S showed increased IFN-γ levels in both T cell subsets, indicating a role in mucosal immunity (**p<0.01). TNF-α and IL-2 expression in CD8+ T cells was significantly higher than in controls (*p<0.05).
4 Discussion
Genital tract CT infection is a common sexually transmitted disease, often leading to hydrosalpinx and other serious complications (Hocking et al., 2023). Despite extensive efforts, no effective Chlamydia vaccine has been approved for clinical use (Olivares-Zavaleta et al., 2014; Qu et al., 2015; Jiang et al., 2017; Schmidt et al., 2017). Recent studies suggest that a Chlamydia digestive tract vaccine might be a promising approach. The presence of Chlamydia in the digestive tract could provide immune protection against reproductive tract infections, reducing the duration and intensity of Chlamydia genital tract infection and preventing complications such as oviduct hydrosalpinx (Hou et al., 2018; Wang et al., 2018). We used CM-pGP3S as a live attenuated vaccine for rectal inoculation, with protection against upper genital tract pathology assessed through infertility and tubal inflammation markers. The results indicate that rectal immunization induces cross-reactive immune responses in the lower digestive tract, reducing the length and intensity of CM-WT genital shedding and preventing CM-WT–induced hydrosalpinx. These findings highlight the potential of rectal immunization as a novel route for inducing protective immunity in the female genital tract.
In this study, a CM infection model was used to evaluate CT’s effects on genital tract infection invasiveness. Previous findings suggest that oral CM inoculation through the mucosal membrane induces resistance to subsequent CM vaginal challenges (Wang et al., 2018). Transmucosal protection is rapidly induced and can persist long-term. The vaccine inoculation route plays a crucial role in immune response induction, effectively preventing oviduct and uterine inflammation through humoral immunity. A suitable route of administration could induce immune responses in both mucosal and systemic tissues (Mayr et al., 2012). Although CM-WT colonization of the digestive tract does not cause intestinal mucosa inflammation or other pathological changes (Zhong, 2018), using the pathogenic wild-type CM-WT as a vaccine is not safe. Evidence shows that CT can directly infect the rectum, potentially causing severe proctitis or rectal colitis and increasing the risk of CT rectal transmission to the genital tract (O’Farrell et al., 2008; White, 2009). Recent studies indicate that the deficient CM-pGP3S strain does not cause hydrosalpinx or other genital tract pathologies (Hou et al., 2018). Therefore, the use of non-pathogenic attenuated CM-pGP3S as a digestive tract vaccine for intestinal mucosal immunity could enhance safety and help reduce persistent Chlamydia genital infections and irreversible pathologies like oviduct hydrosalpinx. Currently, an oral attenuated CM-pGP3S vaccine has been developed to induce mucosal immunity, potentially preventing genital Chlamydia infection and related pathogenicity (Zhou et al., 2022).
This study further explored rectal immunization with CM-pGP3S targeting the digestive tract. When CM-pGP3S was administered rectally, no pathogenicity was observed in the upper tract, indicating that CM-pGP3S is safe for the digestive system. Rectal immunization with CM-pGP3S induced robust transmucosal protection against CM-WT vaginal challenge. These findings support previous results, suggesting that the CM-pGP3S rectal vaccine provides a significant immune effect, resulting in a notable reduction in both the frequency and duration of Chlamydia infections following CM-WT vaginal challenge. Even at a low dose, CM-pGP3S conferred substantial protection. Rectal inoculation of wild-type CM (non-attenuated strain) in animal models can induce immune protection, but it may cause intestinal inflammation or spread to the vagina to cause reproductive tract infection (Zhong, 2018), while the attenuated strain CM-PGP3S in this study is non-pathogenic to avoid this risk. In addition, the study attempted to apply a low-dose attenuated vaccine to rectal inoculation, and the CM-pGP3S were cleared on the 63rd day after inoculation, which had no pathogenic effect on mice and could induce an immune protection effect in the vagina. After CM-WT infection, the persistent rectal infection of CM-WT disappeared on D49, thus laying the foundation for subsequent research on a low-dose attenuated vaccine.
Protective effects on the genital tract were confirmed through both macroscopic and microscopic pathological examinations. Notably, the CM-pGP3S rectal vaccine-induced protective immunity in the female genital tract, offering strong defense against Chlamydia-related genital diseases and presenting a novel approach to Chlamydia vaccine development targeting upper genital tract pathology. Despite prolonged colonization in the digestive tract, CM-pGP3S did not exhibit any significant pathology. This study thoroughly examined both lower digestive tract tissues and intestinal flora. Macroscopically, no signs of colitis were observed, and microscopically, CM-pGP3S did not induce significant inflammatory infiltration in intestinal epithelial cells nor cause major alterations in gut microbiota flora.
Using attenuated CM-pGP3S for rectal inoculation protected mice from Chlamydia genital infection and lesions in the upper genital tract. Rectal inoculation is appealing for immunization against genital Chlamydia infection as it provides transmucosal immunity through common immune effectors against mucosal pathogens such as CT. Additionally, the rectal approach avoids some adverse effects associated with traditional injectable immunizations and is considered a convenient mucosal immune strategy against microbial pathogens (Tsai et al., 2023).
This study also demonstrated that CM-pGP3S rectal immunization significantly increased serum IgG and fecal IgA, indicating strong systemic and localized intestinal antibody responses, consistent with previous research on CM-WT oral vaccines (Wang et al., 2018; Shillova et al., 2020). Although some studies have suggested that IgG antibodies may be involved in Chlamydia immunoprotection through neutralization or opsonophagy (Quigley and Timms, 2021), other data (Mathias et al., 2014) have not observed a significant association between IgG and infection protection. Therefore, the specific role of IgG in primary Chlamydia infection still needs to be further studied and may be influenced by the host immune background or antibody subtype specificity. Mucosal immunoglobulin A (IgA) has been demonstrated to perform critical immunosurveillance functions in gastrointestinal homeostasis, particularly through its regulation of commensal microbiota composition and prevention of pathogenic bacterial translocation (Crank et al., 2019).
Immunologic evaluation showed that rectal CM-pGP3S immunization induced a cellular immune response characterized by high levels of Chlamydia-specific CD4+ and CD8+ T cells that secreted IFN-γ, with increased TNF-α and IL-2 levels in CD8+ T cells. CD4+ T cells have been shown in both human and animal studies to be vital in CT immunity (Murthy et al., 2007). Studies have highlighted antibodies’ central role in CM-induced immunity, with effector cell activation in genital tract tissues dependent on CD4+ T cells (Farris et al., 2010). IFN-γ from CD4+ T cells activates these effector cells, promoting antibody-mediated anti-Chlamydia immunity (Naglak et al., 2016). CD8+ T cells, known as cytotoxic T lymphocytes, resist various pathogens, including different organisms (St Paul and Ohashi, 2020). The elevated TNF-α, IFN-γ, and IL-2 levels in CD8+ T cells observed in this study suggest that these inflammatory factors play essential roles in the immune response and defense against pathogen infections elicited by CM-pGP3S rectal immunization.
Our study demonstrated that rectal inoculation induces immune responses and mediates CM clearance in the reproductive system, indicating cooperative mucosal interactions between the rectum and reproductive tract, acting as sites for mucosal induction and effect. Within the mucosal immune system, vaccine-induced immunity has distinct functions in preventing Chlamydia infection sequelae, such as infertility and upper genital tract inflammation. Collectively, these results highlight rectal delivery as a viable approach for eliciting mucosal pathway-mediated protection in the female reproductive tract.
There are many studies that used live, live-attenuated, or killed Chlamydial EBs delivered via the intranasal route, effectively demonstrating notable protection correlates, such as heightened serum and vaginal Chlamydia-specific IgG/IgA, reduced time-to-clearance, reduced burdens, and subsequently reduced pathology. Indeed, intranasal EB immunization provides near complete protection, while intramuscular EB provides partial protection (Phillips et al., 2019; Zuo et al., 2021; Poston, 2024). The protective effect was found to be associated with reduced TNF-α response by antigen-specific CD8+ T cells, which mediate this inhibition. It suggests that intranasal immunity may mitigate pathology by modulating T-cell responses but requires a specific form of antigen (such as live EB) (Murthy et al., 2024). Poston et al (Poston et al., 2025). used intranasal CPAF plus ADU-S100 adjuvant to induce a CD4+ T cell response and reduce bacterial shedding and duration of infection. It suggests that intranasal immunization combined with specific adjuvants can effectively enhance mucosal immunity and shorten clearance time. Still, the present study chose to use the rectal route for several reasons. More targeted: rectal immunization more directly simulates the natural infection path and induces a local immune response in the rectal and vaginal areas (Zhu et al., 2012). In addition, the rectal area is larger than the intranasal area, allowing for more effective contact of the vaccine with the mucosa, and intranasal immunization may be more focused on local immunity of upper respiratory tract mucosa. Safety: intranasal immunization may cause local inflammation of the respiratory tract, while rectal immunization, as shown in this study, did not show significant intestinal pathology or flora disturbance in mice models. Compliance: Mucosal immunization is more acceptable to patients than intramuscular injection (Neutra and Kozlowski, 2006; Parez et al., 2006; Song et al., 2024). Furthermore, a mucosal Chlamydia vaccine may offer better protection at the mucosal surfaces where the infection occurs, while intramuscular vaccines focus on systemic immunity (Stary et al., 2015; de la Maza et al., 2021).
Notably, CM-pGP3S in the gastrointestinal tract induced transmucosal immunity that protected mice from subsequent genital CM-WT infection. However, CM-WT self-inoculation between the female reproductive tract and anorectum may lead to persistent CM infection in the rectal mucosa, thus prolonging the infection cycle and increasing the risk of chronic inflammation or secondary complications. Therefore, future studies should be devoted to exploring the dual effects of shortening rectal mucosal colonization time and improving reproductive tract immune protection by the development research of attenuated CM vaccines. In this study, only serum IgG, fecal IgA, and spleen CD4+ and CD8+ T cells of the high-dose immunization group (1×107 IFUs) and the control group were detected on the 63rd day of immunization. WT-CT detection after vaginal infection was not performed. It will be investigated in future studies.
In conclusion, this study demonstrated that rectal immunization induces immune effectors that facilitate Chlamydia clearance in the genital tract, reflecting cooperation between rectal and genital mucosae as mucosal inductive and effector sites within the common mucosal immune system. Our findings underscore the potential of a rectal vaccine as an effective mucosal route for inducing protective immunity in the female genital tract.
Data availability statement
The original contributions presented in the study are included in the article/Supplementary Material. Further inquiries can be directed to the corresponding author.
Ethics statement
All procedures for the care and use of animals were approved by the Ethics Committee of Tianjin Medical University General Hospital (IRB2022-DWFL-129), and all institutional and governmental regulations regarding the ethical use of animals were followed.
Author contributions
JM: Conceptualization, Data curation, Formal analysis, Writing – original draft, Writing – review & editing. TZ: Conceptualization, Formal analysis, Writing – original draft, Writing – review & editing. QT: Conceptualization, Writing – original draft, Writing – review & editing. MX: Formal analysis, Writing – original draft, Writing – review & editing. LH: Formal analysis, Writing – original draft, Writing – review & editing. QL: Writing – original draft, Writing – review & editing. GZ: Writing – original draft, Writing – review & editing. YL: Writing – original draft, Writing – review & editing.
Funding
The author(s) declare that financial support was received for the research and/or publication of this article. This study was funded by the Tianjin Key Medical Discipline (Specialty) Construction Project (No: TJYXZDXK-057B). The National Natural Science Foundation of China General Program (32470204).
Conflict of interest
The authors declare that the research was conducted in the absence of any commercial or financial relationships that could be construed as a potential conflict of interest.
Generative AI statement
The author(s) declare that no Generative AI was used in the creation of this manuscript.
Publisher’s note
All claims expressed in this article are solely those of the authors and do not necessarily represent those of their affiliated organizations, or those of the publisher, the editors and the reviewers. Any product that may be evaluated in this article, or claim that may be made by its manufacturer, is not guaranteed or endorsed by the publisher.
References
Azegami, T., Yuki, Y., Kiyono, H. (2014). Challenges in mucosal vaccines for the control of infectious diseases. Int. Immunol. 26, 517–528. doi: 10.1093/intimm/dxu063
Borges, A. H., Follmann, F., Dietrich, J. (2022). Chlamydia trachomatis vaccine development - a view on the current challenges and how to move forward. Expert Rev. Vaccines 21, 1555–1567. doi: 10.1080/14760584.2022.2117694
Conrad, T. A., Gong, S., Yang, Z., Matulich, P., Keck, J., Beltrami, N., et al. (2016). The chromosome-encoded hypothetical protein TC0668 is an upper genital tract pathogenicity factor of chlamydia muridarum. Infect. Immun. 84, 467–479. doi: 10.1128/IAI.01171-15
Crank, M. C., Ruckwardt, T. J., Chen, M., Morabito, K. M., Phung, E., Costner, P. J., et al. (2019). A proof of concept for structure-based vaccine design targeting RSV in humans. Science 365, 505–509. doi: 10.1126/science.aav9033
de la Maza, L. M., Darville, T. L., Pal, S. (2021). Chlamydia trachomatis vaccines for genital infections: where are we and how far is there to go? Expert Rev. Vaccines 20, 421–435. doi: 10.1080/14760584.2021.1899817
Dielissen, P. W., Teunissen, D. A., Lagro-Janssen, A. L. (2013). Chlamydia prevalence in the general population: is there a sex difference? A systematic review. BMC Infect. Dis. 13, 534. doi: 10.1186/1471-2334-13-534
Dirks, J., van Liere, G., Hoebe, C., Wolffs, P., Dukers-Muijrers, N. (2019). Genital and anal Chlamydia trachomatis bacterial load in concurrently infected women: a cross-sectional study. Sex Transm. Infect. 95, 317–321. doi: 10.1136/sextrans-2018-053678
Farris, C. M., Morrison, S. G., Morrison, R. P. (2010). CD4+ T cells and antibody are required for optimal major outer membrane protein vaccine-induced immunity to Chlamydia muridarum genital infection. Infect. Immun. 78, 4374–4383. doi: 10.1128/IAI.00622-10
Hocking, J. S., Geisler, W. M., Kong, F. Y. S. (2023). Update on the epidemiology, screening, and management of chlamydia trachomatis infection. Infect. Dis. Clin. North Am. 37, 267–288. doi: 10.1016/j.idc.2023.02.007
Hou, S., Yue, L., Xu, R., Zhu, C., Shan, S., Wang, H., et al. (2018). Chlamydia muridarum plasmid induces mouse oviduct pathology by promoting chlamydial survival and ascending infection and triggering host inflammation. Eur. J. Dermatol. 28, 628–636. doi: 10.1684/ejd.2018.3399
Hu, H., Zhou, Y., Shi, L., Lu, J., Zhang, Z., Xu, X., et al. (2021). High prevalence of Chlamydia trachomatis infection among women attending STD and gynecology clinics in Jiangsu province, China: A cross-sectional survey. Med. (Baltimore) 100, e27599. doi: 10.1097/MD.0000000000027599
Jiang, P., Cai, Y., Chen, J., Ye, X., Mao, S., Zhu, S., et al. (2017). Evaluation of tandem Chlamydia trachomatis MOMP multi-epitopes vaccine in BALB/c mice model. Vaccine 35, 3096–3103. doi: 10.1016/j.vaccine.2017.04.031
Klasinc, R., Reiter, M., Digruber, A., Tschulenk, W., Walter, I., Kirschner, A., et al. (2021). A novel flow cytometric approach for the quantification and quality control of chlamydia trachomatis preparations. Pathogens 10, 5. doi: 10.3390/pathogens10121617
Kong, F. Y., Hocking, J. S. (2015). Treatment challenges for urogenital and anorectal Chlamydia trachomatis. BMC Infect. Dis. 15, 293. doi: 10.1186/s12879-015-1030-9
Li, M., Wang, Y., Sun, Y., Cui, H., Zhu, S. J., Qiu, H. J. (2020). Mucosal vaccines: Strategies and challenges. Immunol. Lett. 217, 116–125. doi: 10.1016/j.imlet.2019.10.013
Liu, Y., Huang, Y., Yang, Z., Sun, Y., Gong, S., Hou, S., et al. (2014). Plasmid-encoded Pgp3 is a major virulence factor for Chlamydia muridarum to induce hydrosalpinx in mice. Infect. Immun. 82, 5327–5335. doi: 10.1128/IAI.02576-14
Lu, H., Zhong, G. (1999). Interleukin-12 production is required for chlamydial antigen-pulsed dendritic cells to induce protection against live Chlamydia trachomatis infection. Infect. Immun. 67, 1763–1769. doi: 10.1128/IAI.67.4.1763-1769.1999
Lundy, S. R., Abney, K., Ellerson, D., Igietseme, J. U., Carroll, D., Eko, F. O., et al. (2021). MiR-378b modulates chlamydia-induced upper genital tract pathology. Pathogens 10, 4. doi: 10.3390/pathogens10050566
Ma, J., He, C., Huo, Z., Xu, Y., Arulanandam, B., Liu, Q., et al. (2020). The cryptic plasmid improves chlamydia fitness in different regions of the gastrointestinal tract. Infect. Immun. 88, 4. doi: 10.1128/IAI.00860-19
Mathias, A., Pais, B., Favre, L., Benyacoub, J., Corthesy, B. (2014). Role of secretory IgA in the mucosal sensing of commensal bacteria. Gut Microbes 5, 688–695. doi: 10.4161/19490976.2014.983763
Mayr, U. B., Kudela, P., Atrasheuskaya, A., Bukin, E., Ignatyev, G., Lubitz, W. (2012). Rectal single dose immunization of mice with Escherichia coli O157:H7 bacterial ghosts induces efficient humoral and cellular immune responses and protects against the lethal heterologous challenge. Microb. Biotechnol. 5, 283–294. doi: 10.1111/j.1751-7915.2011.00316.x
McIntosh, E. D. G. (2020). Development of vaccines against the sexually transmitted infections gonorrhoea, syphilis, Chlamydia, herpes simplex virus, human immunodeficiency virus and Zika virus. Ther. Adv. Vaccines Immunother. 8, 2515135520923887. doi: 10.1177/2515135520923887
Mohseni, M., Sung, S., Takov, V. (2025). “Chlamydia,” in StatPearls (Treasure Island (FL): StatPearls Publishing).
Morrison, S. G., Giebel, A. M., Toh, E., Banerjee, A., Nelson, D. E., Morrison, R. P. (2020). A Genital Infection-Attenuated Chlamydia muridarum Mutant Infects the Gastrointestinal Tract and Protects against Genital Tract Challenge. mBio 11, 3. doi: 10.1128/mBio.02770-20
Murthy, A. K., Chambers, J. P., Meier, P. A., Zhong, G., Arulanandam, B. P. (2007). Intranasal vaccination with a secreted chlamydial protein enhances resolution of genital Chlamydia muridarum infection, protects against oviduct pathology, and is highly dependent upon endogenous gamma interferon production. Infect. Immun. 75, 666–676. doi: 10.1128/IAI.01280-06
Murthy, A. K., Wright-McAfee, E., Warda, K., Moy, L. N., Bui, N., Musunuri, T., et al. (2024). Protective anti-Chlamydial vaccine regimen-induced CD4+ T cell response mediates early inhibition of pathogenic CD8+ T cell response following genital challenge. Pathog. Dis. 82, 13. doi: 10.1093/femspd/ftae008
Naglak, E. K., Morrison, S. G., Morrison, R. P. (2016). IFNgamma is required for optimal antibody-mediated immunity against genital chlamydia infection. Infect. Immun. 84, 3232–3242. doi: 10.1128/IAI.00749-16
Neutra, M. R., Kozlowski, P. A. (2006). Mucosal vaccines: the promise and the challenge. Nat. Rev. Immunol. 6, 148–158. doi: 10.1038/nri1777
O’Farrell, N., Morison, L., Moodley, P., Pillay, K., Vanmali, T., Quigley, M., et al. (2008). Genital ulcers and concomitant complaints in men attending a sexually transmitted infections clinic: implications for sexually transmitted infections management. Sex Transm. Dis. 35, 545–549. doi: 10.1097/OLQ.0b013e31816a4f2e
Olivares-Zavaleta, N., Whitmire, W. M., Kari, L., Sturdevant, G. L., Caldwell, H. D. (2014). CD8+ T cells define an unexpected role in live-attenuated vaccine protective immunity against Chlamydia trachomatis infection in macaques. J. Immunol. 192, 4648–4654. doi: 10.4049/jimmunol.1400120
Pais, R., Omosun, Y., He, Q., Blas-MaChado, U., Black, C., Igietseme, J. U., et al. (2017). Rectal administration of a Chlamydial subunit vaccine protects against genital infection and upper reproductive tract pathology in mice. PloS One 12, e0178537. doi: 10.1371/journal.pone.0178537
Parez, N., Fourgeux, C., Mohamed, A., Dubuquoy, C., Pillot, M., Dehee, A., et al. (2006). Rectal immunization with rotavirus virus-like particles induces systemic and mucosal humoral immune responses and protects mice against rotavirus infection. J. Virol. 80, 1752–1761. doi: 10.1128/jvi.80.4.1752-1761.2006
Phillips, S., Quigley, B. L., Timms, P. (2019). Seventy years of chlamydia vaccine research - limitations of the past and directions for the future. Front. Microbiol. 10. doi: 10.3389/fmicb.2019.00070
Poston, T. B. (2024). Advances in vaccine development for Chlamydia trachomatis. Pathog. Dis. 82, ftae017. doi: 10.1093/femspd/ftae017
Poston, T. B., Girardi, J., Kim, M., Zwarycz, P., Polson, A. G., Yount, K. S., et al. (2025). Intranasal immunization with CPAF combined with ADU-S100 induces an effector CD4 T cell response and reduces bacterial burden following intravaginal infection with Chlamydia muridarum. Vaccine 43, 126526. doi: 10.1016/j.vaccine.2024.126526
Poston, T. B., Gottlieb, S. L., Darville, T. (2019). Status of vaccine research and development of vaccines for Chlamydia trachomatis infection. Vaccine 37, 7289–7294. doi: 10.1016/j.vaccine.2017.01.023
Qu, Y., Frazer, L. C., O’Connell, C. M., Tarantal, A. F., Andrews, C. W., Jr., O’Connor, S. L., et al. (2015). Comparable genital tract infection, pathology, and immunity in rhesus macaques inoculated with wild-type or plasmid-deficient chlamydia trachomatis serovar D. Infect. Immun. 83, 4056–4067. doi: 10.1128/IAI.00841-15
Quigley, B. L., Timms, P. (2021). The koala immune response to chlamydial infection and vaccine development-advancing our immunological understanding. Anim. (Basel) 11, 12. doi: 10.3390/ani11020380
Schmidt, S. T., Neustrup, M. A., Harloff-Helleberg, S., Korsholm, K. S., Rades, T., Andersen, P., et al. (2017). Systematic investigation of the role of surfactant composition and choice of oil: design of a nanoemulsion-based adjuvant inducing concomitant humoral and CD4(+) T-cell responses. Pharm. Res. 34, 1716–1727. doi: 10.1007/s11095-017-2180-9
Shillova, N., Howe, S. E., Hyseni, B., Ridgell, D., Fisher, D. J., Konjufca, V. (2020). Chlamydia-Specific IgA Secretion in the Female Reproductive Tract Induced via Per-Oral Immunization Confers Protection against Primary Chlamydia Challenge. Infect. Immun. 89, 12. doi: 10.1128/IAI.00413-20
Song, Y., Mehl, F., Zeichner, S. L. (2024). Vaccine strategies to elicit mucosal immunity. Vaccines (Basel) 12 (2), 191. doi: 10.3390/vaccines12020191
Stary, G., Olive, A., Radovic-Moreno, A. F., Gondek, D., Alvarez, D., Basto, P. A., et al. (2015). VACCINES. A mucosal vaccine against Chlamydia trachomatis generates two waves of protective memory T cells. Science 348, aaa8205. doi: 10.1126/science.aaa8205
St Paul, M., Ohashi, P. S. (2020). The roles of CD8(+) T cell subsets in antitumor immunity. Trends Cell Biol. 30, 695–704. doi: 10.1016/j.tcb.2020.06.003
Tsai, C. J. Y., Loh, J. M. S., Fujihashi, K., Kiyono, H. (2023). Mucosal vaccination: onward and upward. Expert Rev. Vaccines 22, 885–899. doi: 10.1080/14760584.2023.2268724
Varotto-Boccazzi, I., Epis, S., Cattaneo, G. M., Guerrini, N., Manenti, A., Rubolini, D., et al. (2023). Rectal Administration of Leishmania Cells Elicits a Specific, Th1-Associated IgG2a Response in Mice: New Perspectives for Mucosal Vaccination against Leishmaniasis, after the Repurposing of a Study on an Anti-Viral Vaccine Candidate. Trop. Med. Infect. Dis. 8, 4. doi: 10.3390/tropicalmed8080406
Wang, L., Zhu, C., Zhang, T., Tian, Q., Zhang, N., Morrison, S., et al. (2018). Non-pathogenic colonization with chlamydia in the gastrointestinal tract as oral vaccination for inducing transmucosal protection. Infect. Immun. 86, 4. doi: 10.1128/IAI.00630-17
White, J. A. (2009). Manifestations and management of lymphogranuloma venereum. Curr. Opin. Infect. Dis. 22, 57–66. doi: 10.1097/QCO.0b013e328320a8ae
Workowski, K. A., Bachmann, L. H., Chan, P. A., Johnston, C. M., Muzny, C. A., Park, I., et al. (2021). Sexually transmitted infections treatment guidelines, 2021. MMWR Recomm. Rep. 70, 1–187. doi: 10.15585/mmwr.rr7004a1
Yeruva, L., Spencer, N., Bowlin, A. K., Wang, Y., Rank, R. G. (2013). Chlamydial infection of the gastrointestinal tract: a reservoir for persistent infection. Pathog. Dis. 68, 88–95. doi: 10.1111/2049-632X.12052
Zhang, T., Huo, Z., Ma, J., He, C., Zhong, G. (2019). The plasmid-encoded pGP3 promotes chlamydia evasion of acidic barriers in both stomach and vagina. Infect. Immun. 87, 4. doi: 10.1128/IAI.00844-18
Zhong, G. (2018). Chlamydia spreading from the genital tract to the gastrointestinal tract - A two-hit hypothesis. Trends Microbiol. 26, 611–623. doi: 10.1016/j.tim.2017.12.002
Zhou, Z., Tian, Q., Wang, L., Zhong, G. (2022). Chlamydia deficient in plasmid-encoded glycoprotein 3 (pGP3) as an attenuated live oral vaccine. Infect. Immun. 90, e0047221. doi: 10.1128/IAI.00472-21
Zhu, Q., Talton, J., Zhang, G., Cunningham, T., Wang, Z., Waters, R. C., et al. (2012). Large intestine–targeted, nanoparticle-releasing oral vaccine to control genitorectal viral infection. Nat. Med. 18, 1291–1296. doi: 10.1038/nm.2866
Zuo, Z., Zou, Y., Li, Q., Guo, Y., Zhang, T., Wu, J., et al. (2021). Intranasal immunization with inactivated chlamydial elementary bodies formulated in VCG-chitosan nanoparticles induces robust immunity against intranasal Chlamydia psittaci challenge. Sci. Rep. 11, 10389. doi: 10.1038/s41598-021-89940-8
Keywords: Chlamydia muridarum, attenuated rectal vaccine, pGP3S, mucosal immunity, rectal immunization, genital tract infection
Citation: Ma J, Zhang T, Tian Q, Xiao M, Han L, Liu Q, Zhong G and Liu Y (2025) Chlamydia muridarum mutant CM-pGP3S as a novel attenuated live rectal vaccine protects against genital tract infection. Front. Cell. Infect. Microbiol. 15:1550455. doi: 10.3389/fcimb.2025.1550455
Received: 01 January 2025; Accepted: 14 April 2025;
Published: 13 May 2025.
Edited by:
Francis O. Eko, Morehouse School of Medicine, United StatesReviewed by:
George W. Liechti, Uniformed Services University of the Health Sciences, United StatesYongzheng Wu, Institut Pasteur, France
Copyright © 2025 Ma, Zhang, Tian, Xiao, Han, Liu, Zhong and Liu. This is an open-access article distributed under the terms of the Creative Commons Attribution License (CC BY). The use, distribution or reproduction in other forums is permitted, provided the original author(s) and the copyright owner(s) are credited and that the original publication in this journal is cited, in accordance with accepted academic practice. No use, distribution or reproduction is permitted which does not comply with these terms.
*Correspondence: Yuanjun Liu, bGl1eXVhbmp1bjE5ODBAMTI2LmNvbQ==