- 1Department of Pharmacy, Shaoxing Hospital of Traditional Chinese Medicine Affiliated to Zhejiang Chinese Medical University, Shaoxing, Zhejiang, China
- 2Department of Clinical Laboratory, Shaoxing Hospital of Traditional Chinese Medicine Affiliated to Zhejiang Chinese Medical University, Shaoxing, Zhejiang, China
- 3Department of Respiratory Medicine, Shaoxing Hospital of Traditional Chinese Medicine Affiliated to Zhejiang Chinese Medical University, Shaoxing, Zhejiang, China
- 4Pharmacy Department of Chinese Medicine, Shaoxing Hospital of Traditional Chinese Medicine Affiliated to Zhejiang Chinese Medical University, Shaoxing, Zhejiang, China
- 5Department of Pharmacy, Quzhou Maternal and Child Health Care Hospital, Quzhou, China
- 6Department of Clinical Laboratory, Zhejiang Hospital, Hangzhou, Zhejiang, China
Objectives: To characterize a rare ST852 Klebsiella quasipneumoniae strain co-producing NDM-1 and KPC-2 isolated from a clinical patient.
Methods: Minimum inhibitory concentrations (MICs) were measured using a VITEK 2 compact system and broth microdilution. Conjugation experiments were conducted using film matings. Whole genome sequencing (WGS) was performed using Illumina and Nanopore platforms. Antimicrobial resistance determinants were identified using the ABRicate program in the ResFinder database. Insertion sequences (ISs) were identified using ISFinder. Bacterial virulence factors were identified using a virulence factor database (VFDB). Genome function annotation and classification were further analyzed using the Kyoto Encyclopedia of Genes and Genomes (KEGG) and Cluster of Orthologous Groups (COG) databases. Capsular polysaccharides (KL) and lipooligosaccharides (OCL) were tested using Kleborate with the Kaptive. Multilocus sequence typing (MLST) and replicon types were identified using the Center for Genomic Epidemiology website. Prophage region analysis was performed using PHASTEST software. Conjugation-related elements were predicted using oriTfinder. The plasmid structure was visualized using Circos and similar plasmids in the public database were tracked using BacWGSTdb. A global phylogeny for the ST852 K. quasipneumoniae isolates was further performed.
Results: K. quasipneumoniae KPSY isolate was identified as ST852, with KL18 and O3/O3a. It has an extensive drug-resistant (XDR) profile. WGS analysis revealed that it contained one circular chromosome and three plasmids. The results of the COG and KEGG functional classifications showed that most of the functions were associated with metabolism. pKPSY-2 is a 239,226-bp IncU plasmid carrying the carbapenem resistance gene blaNDM-1. pKPSY-3 is a smaller plasmid belonging to the IncN-type conjugative plasmid with blaKPC-2. Importantly, oriT sequence, the T4SS region, T4CP, and relaxase were identified. Tracking of the blaKPC-2 plasmids showed they were identified in different species in different countries, including E. coli, Leclercia sp., Pantoea sp., and E. hormaechei. Global analysis data showed 13 ST852 strains were mainly isolated from China (84.62%, 11/13), and the remaining isolates were collected from Switzerland.
Conclusions: This is the first study to identify an ST852 NDM-1-KPC-2 coproducing K. quasipneumoniae clinical isolate. Surveillance is warranted, and early detection of this high-risk clone in the clinic is recommended to avoid its extensive spread.
Introduction
Carbapenem-resistant Enterobacteriaceae (CRE) are known to cause a variety of important nosocomial infections associated with high mortality rates and are a global public health threat to patients in hospital settings (Hala et al., 2019). The treatment of CRE is considered a tremendous challenge by the US Centers for Disease Control and Prevention (CDC) (Gu et al., 2018). A majority of CRE-associated genes have been identified, including the New Delhi Metallo-beta-lactamases (NDMs) and Klebsiella pneumoniae carbapenemases (KPCs) (Krapp et al., 2018; Bar-Yoseph et al., 2019).
Klebsiella pneumoniae (KP) was initially classified into three closely related phylogroups. Based on genome sequencing, they are classified into three distinct species: K. pneumoniae, K. quasipneumoniae, and K. variicola (Holt et al., 2015). Their distinction was possible through the comparison of core genomes and not through conventional multi-locus sequence typing (MLST) and capsule genotyping (Long et al., 2017). Importantly, all three species cause human infections (Holt et al., 2015).
Because of the significant overlap in their biochemical profiles, phenotypic testing using traditional microbiological assays is incapable of accurately differentiating K. pneumoniae, K. quasipneumoniae, and K. variicola (Sakai and Maesaki, 2022). Among these, K. quasipneumoniae was reported as an emerging pathogen in 2004 that could cause bloodstream infections (BSIs) in healthy individuals (Dong et al., 2023). Comparative genomics is crucial for understanding the relationships and distinctions between these organisms, facilitating accurate and timely diagnosis and clinical management (Long et al., 2017).
Here, a rare ST852 K. quasipneumoniae clinical isolate co-harboring blaNDM-1 and blaKPC-2 was isolated from wound secretion in 2022 in China and its complete genetic characteristics were studied. To the best of our knowledge, this is the first study to identify an ST852 NDM-1-KPC-2 coproducing K. quasipneumoniae clinical isolate. Importantly, the combination of Illumina and MinION whole-genome sequencing (WGS) approaches provides complete insight into the genomic structural features of the important resistance plasmids found in rare K. quasipneumoniae isolates of clinical origin.
Materials and methods
Bacterial isolation and identification
K. quasipneumoniae KPSY isolate was collected from a teaching hospital in China during routine diagnostic analysis of wound secretion of a 42-year-old male patient from the intensive care unit (ICU) in 2022 in Zhejiang province, China. Isolate identification at the species level was conducted using matrix-assisted laser desorption ionization time-of-flight mass spectrometry (MALDI-TOF MS; Bruker Daltonics GmbH, Bremen, Germany) and confirmed using the fIDBAC database (http://fbac.dmicrobe.cn/home/).
Antimicrobial susceptibility testing
Minimum inhibitory concentrations (MICs) were measured using the VITEK 2 compact system: amikacin, aztreonam, ertapenem, imipenem, meropenem, levofloxacin, ciprofloxacin, ceftazidime, ceftriaxone, cefazolin, cefepime, cefoxitin, gentamicin, tobramycin, and piperacillin. The MIC of colistin was determined using the broth microdilution method. Experimental data were interpreted according to the recommendations of the Clinical and Laboratory Standards Institute (CLSI) 2021 guidelines.
Hypermucoviscous phenotype determination
The string test was performed based on our previous study (Tian et al., 2022). In brief, strain was cultured on a sheep blood agar plate and then performed overnight culture at 37°C followed by streaking an inoculation loop through a colony next day. If a string of > 5 mm was positive, the strain was considered hypermucoviscous.
Plasmids conjugation assays via film mating
To determine the transferability of blaKPC-2 or blaNDM-1 positive plasmids, conjugation experiments using E. coli EC600 (rifampin-resistant) as the recipient strain were conducted using the film-mating method (Yang et al., 2021; Tian et al., 2022). Transconjugants were screened on Mueller–Hinton agar plates containing rifampin (150 μg/mL) and meropenem (2 μg/mL). The identities of the putative transconjugants were confirmed using PCR using specific primers and MALDI-TOF MS. Experiments were independently performed three times.
Short-read and long-read whole genome sequencing
Short-read illumina sequencing
Genomic DNA was extracted using a Qiagen Mini kit (Qiagen, Hilden, Germany), in accordance with the manufacturer’s recommendations. The quality and quantity of the DNA were assessed using a NanoDrop 2000 (Thermo Scientific, USA) and a Qubit 4.0 fluorometer (Invitrogen, USA). Libraries were prepared using the TruePrepTM DNA Library Prep Kit V2 (Vazyme). Individual libraries were assessed with the QIAxcel Advanced Automatic Nucleic Acid Analyzer using a high-resolution gel cartridge (Qiagen, Germany) and then quantified using qPCR using the KAPA SYBR FAST qPCR Kit Kapa KK4610 (KAPA Biosystems, Wilmington, MA, U.S.A.). Paired-end sequencing was performed using an Illumina HiSeq X Ten platform (Illumina, San Diego, CA, USA).
Long-read Oxford Nanopore sequencing
Long-read sequencing was performed for the strain. DNA was extracted using the Gentra® Puregene® Yeast/Bact. kit (Qiagen, Germany), according to the manufacturer’s protocol. Oxford Nanopore sequencing libraries were prepared using the SQU-LSK109 Ligation Sequencing Kit in conjunction with the PCR-Free ONT EXP-NBD104 Native Barcode Expansion Kit (Oxford Nanopore Technologies, UK). DNA was processed without the optional shearing steps to select long reads. After quantification of the individual libraries using Qubit and normalization of library concentrations, the libraries were sequenced using the GridION X5 platform (Oxford Nanopore Technologies, UK).
Bioinformatic analyses for the genomes
Hybrid assembly of the short and long reads of Illumina and MinION was constructed using Unicycler v0.4.8 (Wick et al., 2017). Genome annotation was performed using the National Center for Biotechnology Information (NCBI) Prokaryotic Genome Annotation Pipeline (PGAP) (http://www.ncbi.nlm.nih.gov/genome/annotation_prok/ ) (Tatusova et al., 2016) and Prokka (Seemann, 2014). Genome sequence function annotation and classification were further analyzed using the Kyoto Encclopedia of Genes and Genomes (KEGG) and Cluster of Orthologous Groups (COG) databases. Antimicrobial resistance genes were identified using ABRicate program (https://github.com/tseemann/abricate) using the ResFinder database and the Center for Genomic Epidemiology website (https://genomicepidemiology.org/). Virulence factors were identified using the virulence factor database (VFDB, http://www.mgc.ac.cn/VFs/) (Liu et al., 2022). Capsular polysaccharide (K locus) and lipooligosaccharide (OC locus) were analyzed using Kleborate with the command line of kleborate, ASSEMBLIES-k (Lam et al., 2021, Lam et al., 2022). Multilocus sequence typing (MLST) and replicon types were identified using the Center for Genomic Epidemiology website (https://genomicepidemiology.org/). Prophage region analysis of the genome was conducted with PHASTEST (PHAge Search Tool with the Enhanced Sequence Translation) web server (https://phastest.ca/).
For the analysis of blaKPC-2- and blaNDM-1-positive plasmids, conjugation transfer elements, including the origin site of DNA transfer (oriT), type IV secretion system (T4SS) region, type IV coupling protein (T4CP), and relaxase, were predicted using oriTfinder with default parameter settings (Li et al., 2018). The insertion sequences (ISs) were identified using ISfinder (Siguier et al., 2006). Plasmid structure was visualized using DNAplotter (Carver et al., 2009) and Circos (Krzywinski et al., 2009). Similar plasmids in public databases were tracked using the BacWGSTdb (http://bacdb.org/BacWGSTdb) (Ruan et al., 2020; Feng et al., 2021). Plasmids comparisons were conducted using Proksee (https://proksee.ca/projects/new). Default parameters were used for all software packages.
Global analyses for ST852 K. quasipneumoniae isolates
To construct a global phylogeny for the ST852 K. quasipneumoniae isolates, we downloaded 2,639 genome sequences from the National Center for Biotechnology Information (NCBI) database (search conducted on 1.4.2025). All genome sequences were analyzed by mlst v2.19.0 command and ABRicate v1.0.1 (Liu et al., 2021). Quality control was performed using FastANI v1.33 and QUAST v5.0.2 (Gurevich et al., 2013). As a result, the obtained ST852 genomes were further sent to the further epidemiology and genetic relationship analysis. Snippy v4.4.5 (https://github.com/tseemann/snippy) was used to generate a core genome alignment. Phylogenies were constructed using FastTree (Price et al., 2009). The resulting tree was annotated and visualized using iTOL v7 (https://itol.embl.de/).
Results
MICs and antimicrobial resistance genes profile
The antimicrobial susceptibility testing data revealed K. quasipneumoniae KPSY clinical isolate possessed an extensively drug-resistant (XDR) profile, with the imipenem, meropenem, and ertapenem MICs > 8 μg/mL (Table 1). Furthermore, K. quasipneumoniae KPSY strain was resistant to many antimicrobial agents, including amikacin, aztreonam, levofloxacin, ciprofloxacin, ceftazidime, ceftriaxone, cefazolin, cefepime, cefoxitin, gentamicin, tobramycin, and piperacillin (Table 1). However, it was still susceptible to colistin (< 0.125 μg/mL).
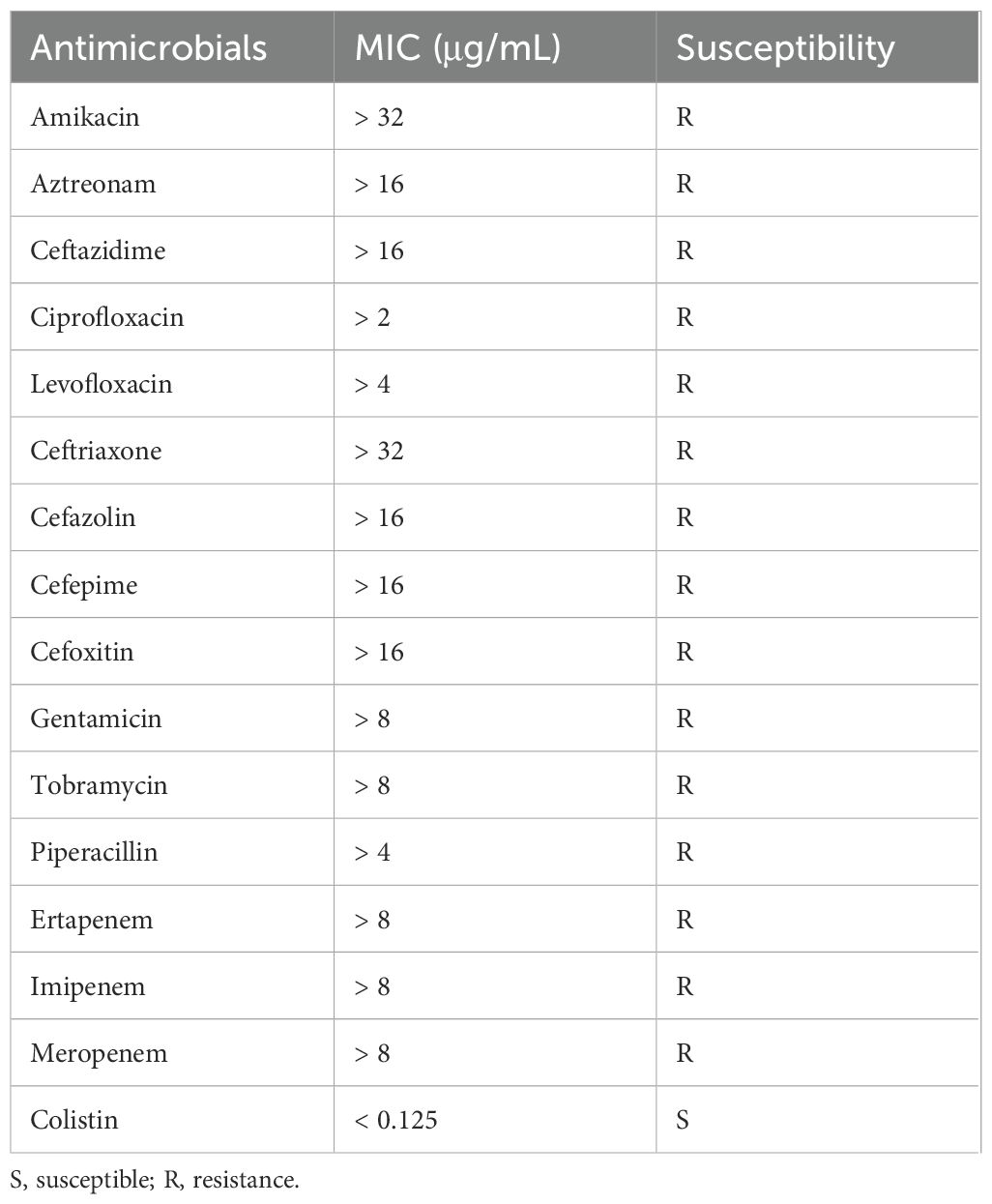
Table 1. Minimal inhibitory concentrations (MICs) to different antimicrobial agents of K. quasipneumoniae KPSY.
Analysis of the genome of K. quasipneumoniae KPSY clinical isolate revealed that, in addition to co-harboring chromosomal blaOKP-B-2, fosA, oqxA and oqxB, a series of genes conferring resistance to β-lactams (blaNDM-1, blaKPC-2), aminoglycosides (aac(6’)-Ib3, aadA16, aadA1), sulfonamides (sul1), trimethoprim/sulfamethoxazole (dfrA27), quinolones (qnrS1), rifampicin (ARR-3), chloramphenicol (catB3), phenicol (cmlA1), fosfomycin (fosE) and bleomycin (ble-MBL) (Table 2).
Virulence factors in K. quasipneumoniae KPSY isolate
There are many virulence factors in the K. quasipneumoniae KPSY isolate, including iron-enterobactin transporter-related protein genes (fepABCDG), siderophore esterase (iroE), type 1 fimbrial (fimABCDEFGHI), type 3 fimbriae (mrkABCDFHIJ), and many others (tli1, vasE/tssK, vasE/tssK, vasE/tssK, clpV/tssH, dotU/tssL and vipB/tssC). However, the result of Kleborate showed that the virulence score is 0. Consistent with the Kleborate data, no genes encoding yersiniabactin, colibactin, aerobactin, salmochelin, RmpADC and RmpA2 were found in our strain. Moreover, the string test experiment is negative.
Multilocus sequence typing, KL and OCL
Based on the K. pneumoniae MLST scheme, K. quasipneumoniae KPSY isolates were typed as ST852 (gapA-18, infB-22, mdh-18, pgi-22, phoE-139, rpoB-69, tonB-179). Kleborate showed that K. quasipneumoniae KPSY strain was KL18, with 96.32% nucleotide identity and 99.93% coverage. The O locus was subjected to O3/O3a, matching 98.52% and 99.77% nucleotide identity.
Chromosome and plasmids characterization of the KPSY strain
The hybrid assembly showed that K. quasipneumoniae KPSY strain had a 5,185,420-bp size circular chromosome (Table 2). The blaOKP-B-2 gene was identified on this chromosome. Moreover, no amino acid mutations in GyrA (S83F) or ParC (S80I), which confer resistance to fluoroquinolones, were found.
Three plasmids were identified in our K. quasipneumoniae KPSY strain, namely pKPSY-1 to pKPSY-3, with sizes between 58,981-bp to 342,682-bp and GC contents ranging from 47% to 52% (Table 2). pKPSY-1 is an IncFIB(K)-type plasmid and no resistance was detected. The pKPSY-2 and pKPSY-3 plasmids have different multidrug resistance (MDR) plasmids harboring various important resistance genes. pKPSY-2 is an IncU plasmid carrying the carbapenem-resistance gene blaNDM-1. pKPSY-3 is the smaller plasmid, which belonged to IncN-typed plasmid. It possesses six antimicrobial resistance (AMR) genes: blaKPC-2, aadA1, cmlA1, fosE, aac(6’)-Ib3, and sul1.
Function classification of COG and KEGG
The results of the COG and KEGG functional classification showed that the majority of the functions were associated with metabolism, including lipid transport and metabolism, amino acid metabolism, and nucleotide metabolism (Figures 1A, B). In addition, the functions of cell motility, replication, and repair were classified using both the COG and KEGG databases (Figures 1A, B).
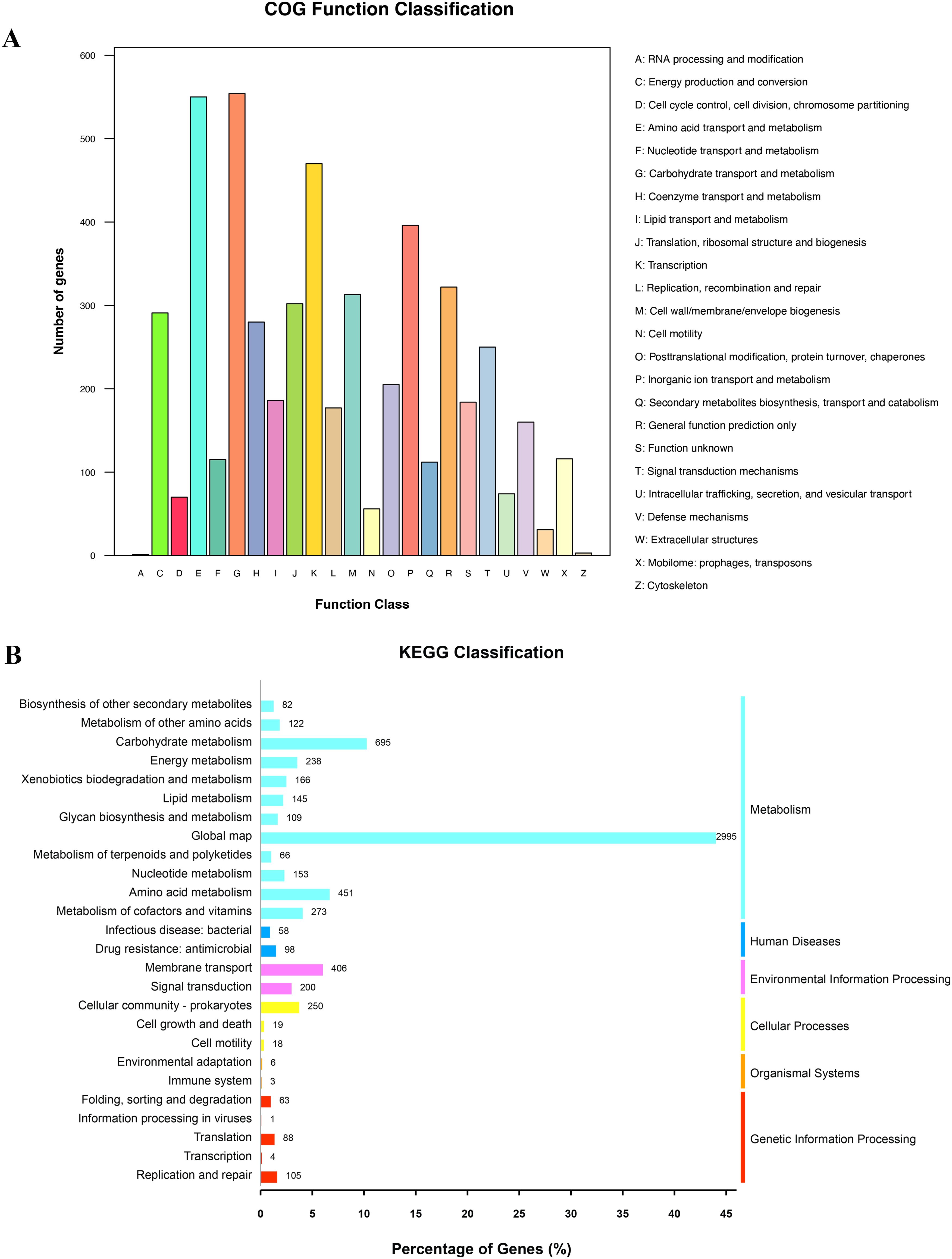
Figure 1. Counts of subsystem based on COG and KEGG annotation. (A) COG function annotation and classification. (B) KEGG classification.
Prophage regions in the genome
Prophage regions were predicted using the PHASTEST tool, and the results showed one intact, one questionable, and four incomplete regions on the chromosome of the K. quasipneumoniae KPSY strain (Table 3). Region 1 in the chromosome was in length of 37.9 kb. It was predicted to be intact because of a score > 90 and a protein number of 43. In addition, Region 3 was classified as questionable with a score of 80. Among the three plasmids, only one intact prophage region was found in pKPSY-1 (protein number: 29). The majority of the prophage regions reported in the plasmids were incomplete owing to their low scores (Table 3).
Genetic features of IncU blaNDM-1-positive plamid pKPSY-2
K. quasipneumoniae KPSY clinical isolate harbored a 239,226-bp plasmid, pKPSY-2, with an IncU replicon. The structure of the plasmid is shown in Figure 2A. Downstream of blaNDM-1 is the bleomycin resistance-related gene ble-MBL. However, ISAba125 was not found upstream of blaNDM-1. Moreover, one class 1 integron with the intI1-aac(6’)-Ib3-ARR-3-dfrA27-aadA16-sul1 genetic array was identified in the pKPSY-2 plasmid Figure 2B. In addition, many mobile genetic elements (MGEs), including IS26, IS5, ISEC33, and ISKpn19, were detected. Two copies of IS26 were detected in the pKPSY-2 plasmid. One copy of IS26 was flanked by an 8-bp direct repeat (DR) sequence (ACTTCGAG and GAAGCAGA). Another copy of IS26 was flanked by different 8-bp DR sequences (CGCTGGAC and TTTTCGAG). Additionally, the results of oriTfinder showed that no oriT, T4SS region, T4CP, or relaxase were found in this plasmid. Mating assays were performed to analyze the transfer ability of blaNDM-1; however, the results showed blaNDM-1 failed to transfer to the recipient strain.
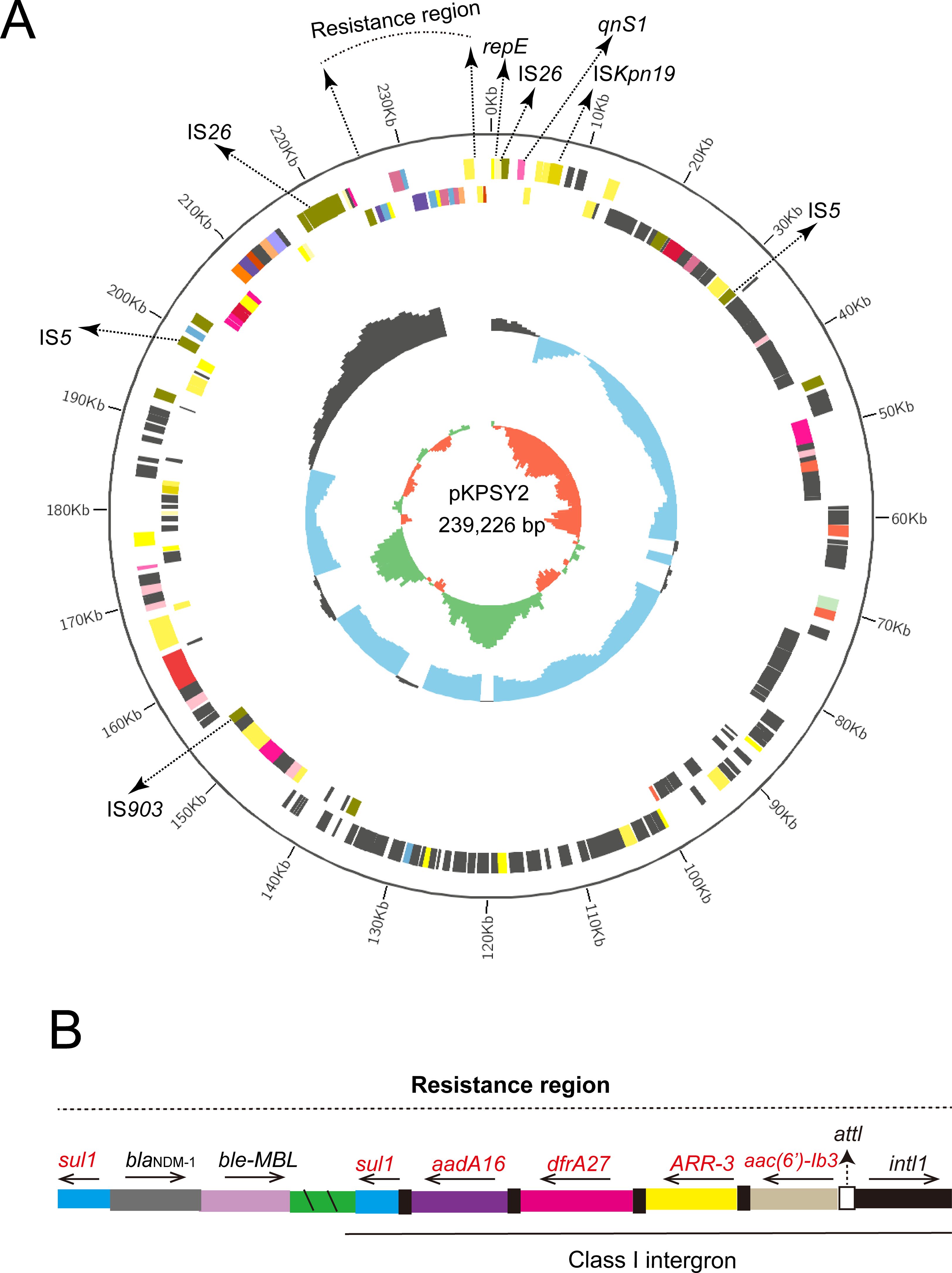
Figure 2. Circular map of pKPSY-2 and the genetic structure of the blaNDM-1. (A) Circular map of pKPSY-2 plasmid. (B) Structure of the resistance region containing blaNDM-1 gene and class I intergron.
Characterization of the KPC-2-producing plasmid pKPSY-3
Based on bioinformatic analysis, blaKPC-2 carbapenem gene was located in a 58,981-bp plasmid, designated pKPSY-3, with an IncN-type replicon. pKPSY-3 had an average GC content of 52% and comprised different regions, including the T4SS and class 1 integron regions (Figure 3A). The resistance genes array of intI1-fosE-aac(6’)-Ib3-cmlA1-aadA1-sul1 was identified in the pKPSY-3 plasmid (Figure 3A). The genetic context of blaKPC-2 is ISKpn27-blaKPC-2-ISKpn6. Importantly, oriT sequence, the T4SS region, T4CP, and relaxase were identified in the plasmid. The inverted repeat (IR) and conserved nick regions of the oriT sequence are shown in Figure 3B. Conjugation experiments demonstrated that it could be transferred to the recipient strain.
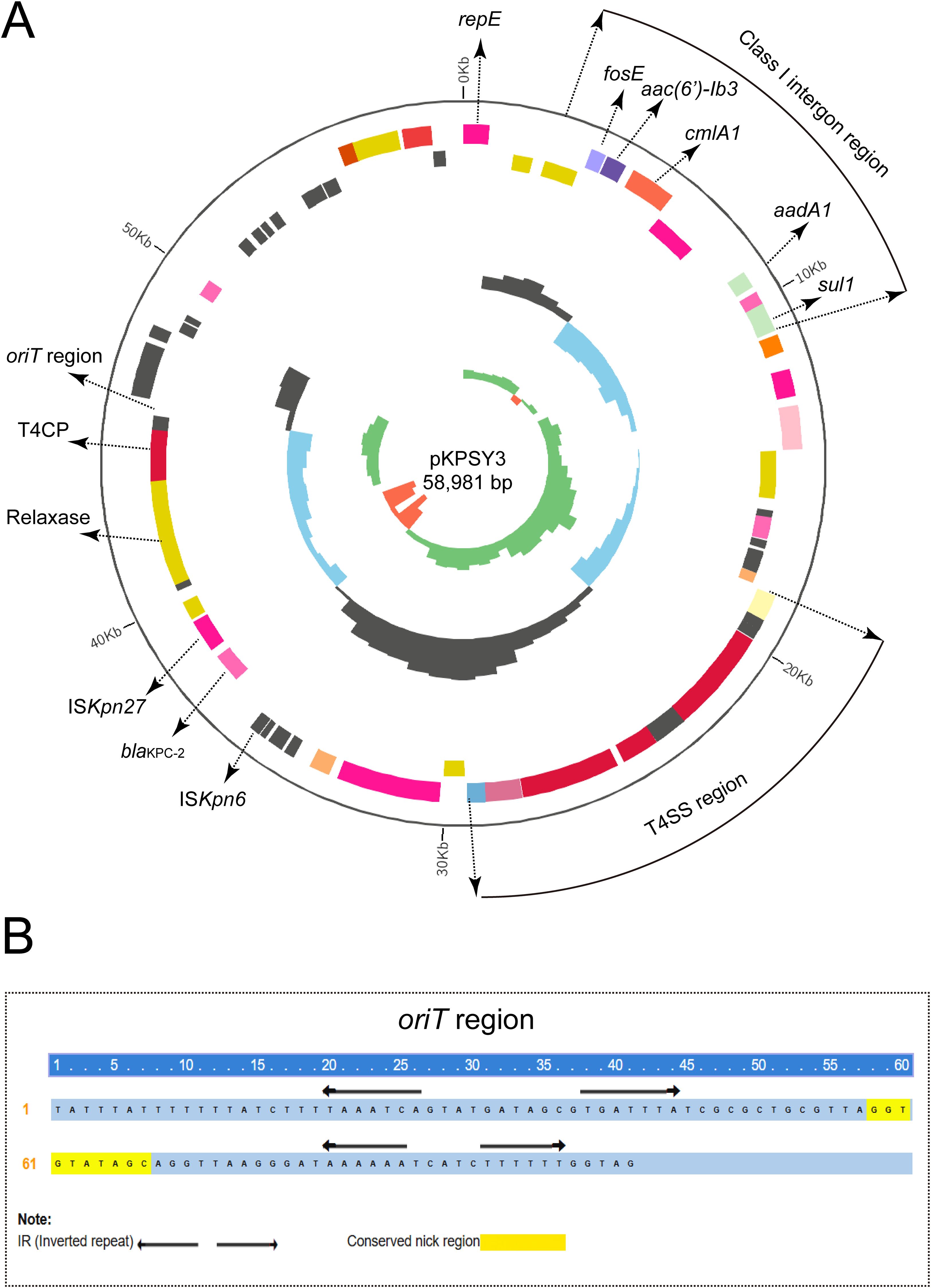
Figure 3. Circular map of pKPSY-3 and the genetic structure of the blaKPC-2. (A) Circular map of pKPSY-3 plasmid. The T4SS region and class I intergron were labelled. The oriT, T4CP and relaxase were also shown. (B) Sequence of the oriT region. IR and the conserved nick region were shown.
Track of similar plasmids in public database
Comparative genomic analysis was performed using the BacWGSTdb server to analyze similar plasmids from different countries. Information on similar plasmids is shown in Table 4. As a result, data showed all blaNDM-1-harboring plasmids belong to IncN-typed replicon. The size ranges from 49,215-bp to 63,046-bp and is found in different species, including K. pneumoniae, Escherichia coli and Enterobacter cloacae. The size was smaller than that of the pKPSY-2 plasmid and the replicon varied. Plasmids comparisons showed the plasmid backbone is completely different and only the blaNDM-1-related region is similar (Figure 4A).
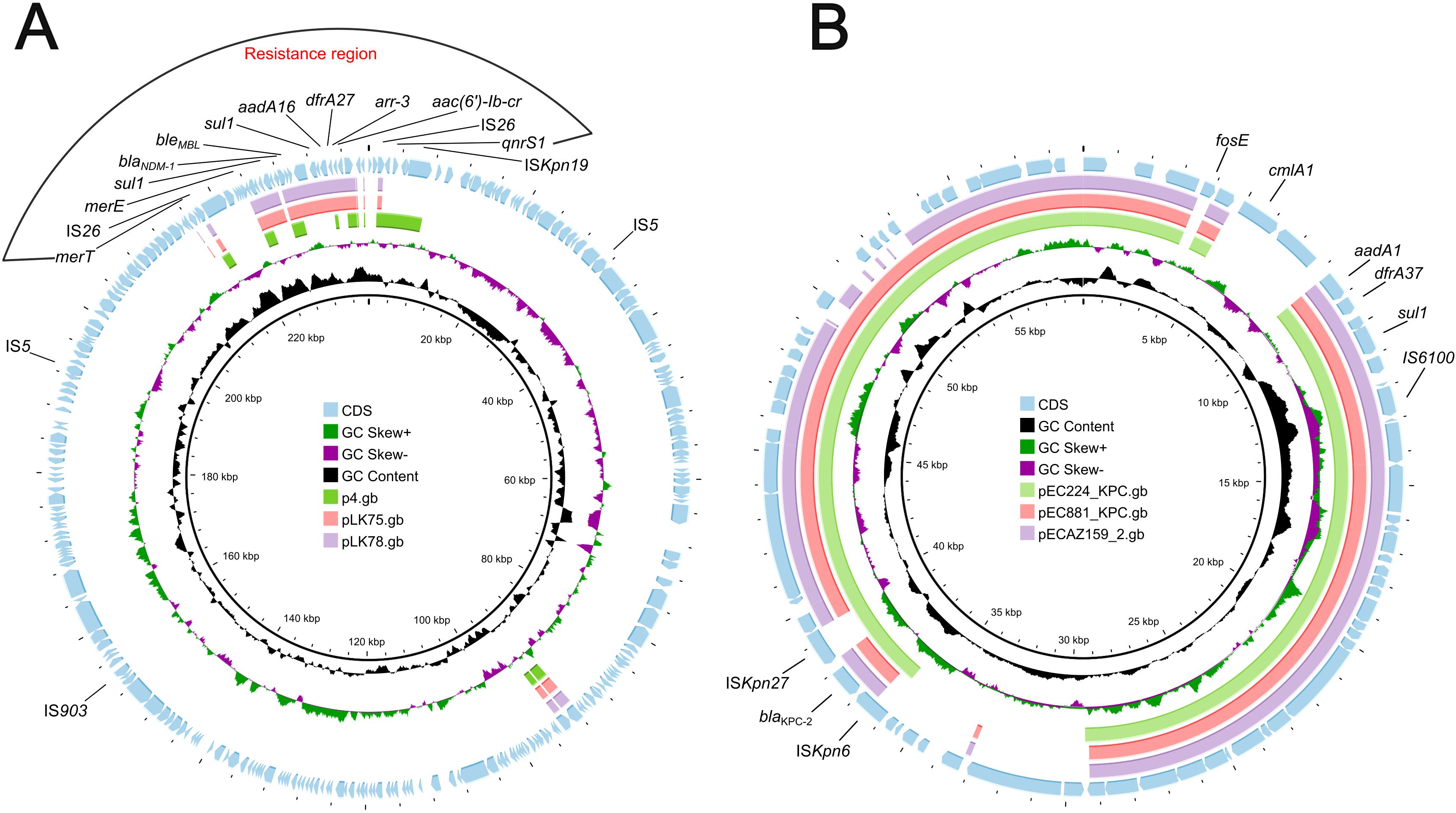
Figure 4. Plasmids comparison of blaNDM-1-harboring and blaKPC-2-positive plasmids. (A) blaNDM-1-harboring plasmids comparison using Proksee. pKPSY-2 was used as reference plasmid. Plasmids p4, pLK75 and pLK78 were compared with pKPSY-2. (B) blaKPC-2-positive plasmids comparison. pKPSY-3 was used as reference plasmid. Plasmids pEC224_KPC, pEC881_KPC and pECA159_2 were compared with pKPSY-3.
Tracking of the blaKPC-2 plasmids identified them in different species, including E. coli, Leclercia sp., Pantoea sp., and E. hormaechei. The size was similar to that of the pKPSY-3 plasmid identified in this study, and the replicon was identical (IncN). However, blaKPC-2-positive plasmids have been found in various countries such as Colombia, Vietnam, Ecuador, and the USA, suggesting that transmission occurred worldwide. Plasmids comparisons revealed that blaKPC-2-positive plasmids possessed huge similarity (Figure 4B). However, the genetic environment of blaKPC-2 is various among them (Figure 4B).
Comparative genomics analysis of global ST852 clone
To analyze the genetic characteristics of the ST852 clone from a global view, comparative genomics analysis of 13 global K. quasipneumoniae strains was performed. Data showed 13 ST852 strains were mainly isolated from China (84.62%, 11/13), and the remaining isolates were collected from Switzerland (Figure 5). Carbapenemase genes analysis showed 7 strains only harbored blaNDM-1 gene and two isolates carried blaKPC-2 and blaIMP-4, simultaneously. Co-carriage of blaNDM-1 and blaKPC-2 has so far only been seen in our strain in China in 2022 (Figure 5). In addition, a relatively close genetic relationship with isolates recovered from China in 2023 was observed (Figure 5).
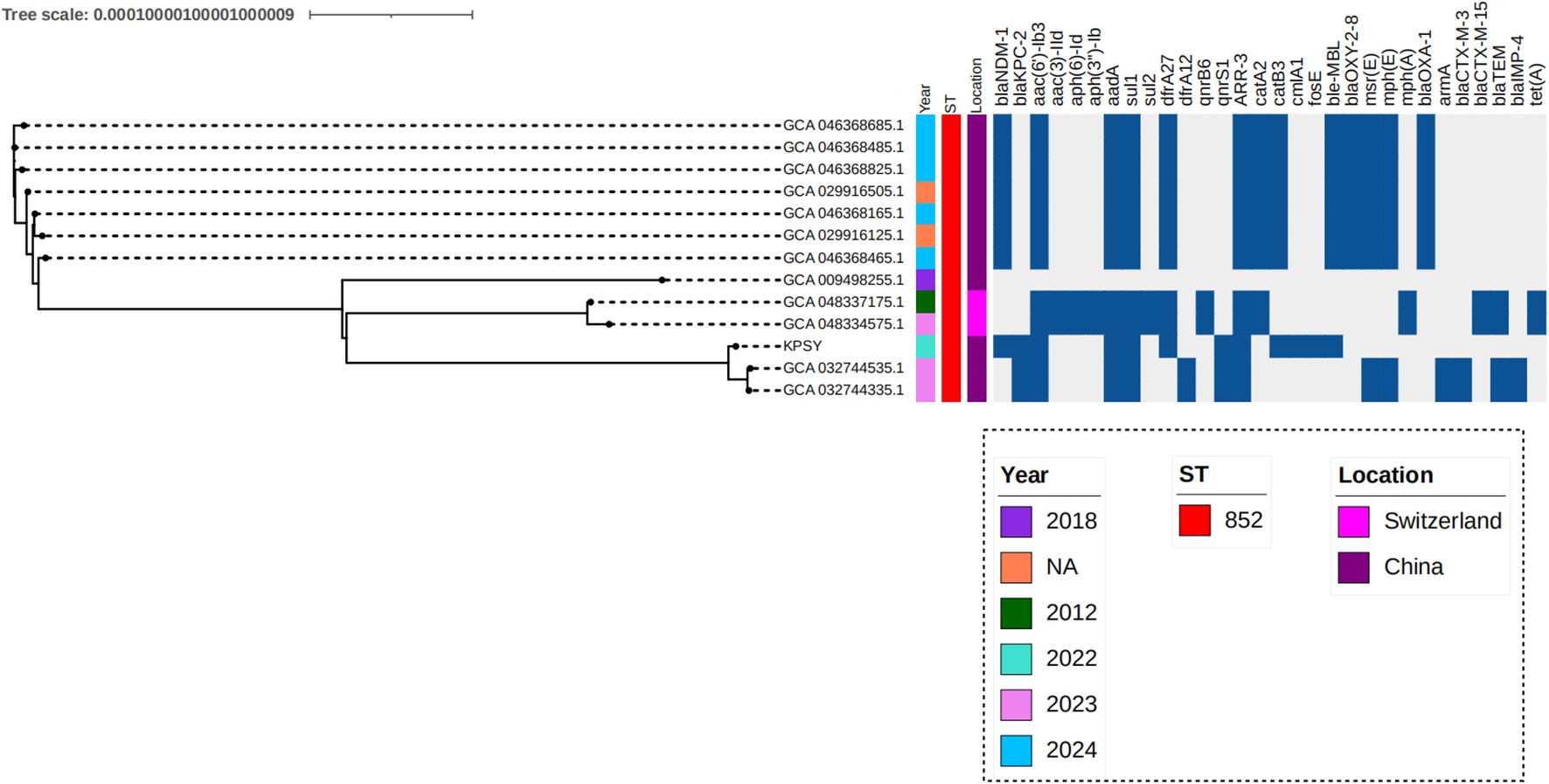
Figure 5. Phylogenetic analysis of ST852 K. quasipneumoniae strains. Phylogenetic analysis was conducted the tree was visualized with iTOL v7. Isolates name, location, collection date, ST type and resistance genes are shown.
Discussion
Nosocomial outbreaks caused by carbapenem-resistant Enterobacteriaceae (CRE) strains are rapidly emerging worldwide and are a cause of concern (Ernst et al., 2020). Among the clinical strains of CRE, K. quasipneumoniae is considered an important nosocomial pathogen that has not been comprehensively studied. The emergence of XDR K. quasipneumoniae, specifically carbapenem-resistant isolates, presents a significant challenge for clinical treatment (Ma et al., 2024).
Mobile genetic elements (MGEs), including ISs, integrons (Ins), and transposons (Tns), play particularly important roles in the transfer of resistance genes among the same or different species (Gorbunova et al., 2021). Dong et al. characterized blaKPC-2- and blaIMP-4-carrying conjugative IncHI5 hybrid plasmids in a clinical ST852-KL18 K. quasipneumoniae strain (Dong et al., 2023). Based on our strain data, we found that the ST and KL types were identical. However, the plasmids (blaKPC-2- and blaNDM-1-carrying plasmids) were different, including the plasmid size (> 200 Kb and 58 Kb), plasmid structure (with and without oriT, T4SS region, T4CP, relaxase) and the resistance genes context (flanked by ISKpn27-ISKpn6 for blaKPC-2 and no ISs were found in the upstream and downstream for blaKPC-2). Thus, these data suggested that diverse resistance plasmids were existed in K. quasipneumoniae. One interesting finding in this study is that blaKPC-2-positive plasmids were observed in different species in different countries, suggesting that the IncU-typed plasmid has likely spread globally. Indeed, we found blaKPC-2-positive pKPSY3 plasmid is a transferable plasmid containing oriT, T4SS, T4CP, and relaxase-related genes, further emphasizing its importance in the dissemination of the carbapenem resistance gene.
Moreover, genetic environment analysis of many gene cassettes revealed a complex class 1 integron in blaKPC-2 and blaNDM-1-carrying plasmids in the present study. Integrons can capture gene cassettes via attl and attC, which can contribute to the resistance to different antimicrobial agents and lead to a global resistance crisis (Ghaly et al., 2021). Many studies have shown that IS26 plays an important role in the spread of resistance genes in gram-negative bacteria (Harmer et al., 2014; Hua et al., 2020). A crucial feature of the IS26 genetic element is its ability to cointegrate molecules comprising distinct DNA segments (Hua et al., 2020). Here, we found two copies of IS26 in the large pKPSY2 plasmid flanked by various 8-bp target site duplications (TSD) sequences. When IS26 relocates, a short sequence (8-bp) at the site they move to (the target site) is duplicated and this appears as one 8-bp direct duplication, named TSD, flanking the IS26 in its new location (Harmer and Hall, 2024). The same 8-bp sequences could be used as an insertion marker. However, considering the 8-bp different TSD sequences in this study, we thought we are failed to find the direct evidence to indicate this large pKPSY-2 plasmid was formed by cointegration.
It is well-documented that the OCL and KL gene clusters in bacteria are responsible for the biosynthesis of the outer core of lipooligosaccharides and the capsule (Kenyon and Hall, 2022). These are potentially useful epidemiological markers that play key roles in vaccine development (Wyres et al., 2020). In the current study, KL18 had a high identity but was only reported in one strain coharboring blaKPC-2 and blaIMP-4 in China. However, this clone has been suggested to be rare.
However, this study has some limitations, such as the need to assess the virulence level of this strain using insect larval or murine models. In addition, the transfer frequency of the blaKPC-2-positive plasmid was measured by conjugation. Finally, the fitness cost of transconjugants containing the blaKPC-2 plasmid was tested using the growth curve method.
Conclusion
In summary, this is the first study to report the complete genome characteristics of the rare KL18-O3/O3a ST852 K. quasipneumoniae strain co-carrying blaNDM-1 and blaKPC-2. Furthermore, it promoted carbapenem resistance via conjugation. Tracking of blaKPC-2-harboring plasmids identified them in different species in different countries, suggesting the possible spread of this IncU-type plasmid worldwide. Therefore, XDR K. quasipneumoniae and its different resistance plasmids are a public health concern and require further surveillance to avoid their extensive spread in healthcare settings.
Data availability statement
The datasets presented in this study can be found in online repositories. The names of the repository/repositories and accession number(s) can be found below: The genomes of K. quasipneumoniae KPSY strain have been deposited in GenBank under the BioProject: PRJNA1202470.
Ethics statement
The studies involving humans were approved by Shaoxing Hospital of Traditional Chinese Medicine Affiliated to Zhejiang Chinese Medical University local ethics committees (No. 2024-011). The studies were conducted in accordance with the local legislation and institutional requirements. The participants provided their written informed consent to participate in this study.
Author contributions
CT: Writing – original draft, Writing – review & editing. YiZ: Writing – review & editing. SD: Writing – review & editing. ZY: Writing – review & editing. JC: Writing – review & editing. HX: Writing – review & editing. YF: Writing – review & editing. YeZ: Writing – review & editing.
Funding
The author(s) declare that financial support was received for the research and/or publication of this article. This work was supported by the Shaoxing Science and Technology Program (2024A14018), Medical Scientific Research Foundation of Zhejiang Province (2023KY438), Medical and Health Technology Projects of Zhejiang Province, China (2023KY1270), Chinese medicine science foundation of Zhejiang Province, China (2024ZR180, 2023ZL729), Shaoxing Health Science and Technology Project, China (2023SKY058, 2024SKY059), National traditional Chinese medicine characteristic technology inheritance talent training program (T20234832005).
Conflict of interest
The authors declare that the research was conducted in the absence of any commercial or financial relationships that could be construed as a potential conflict of interest.
Generative AI statement
The author(s) declare that no Generative AI was used in the creation of this manuscript.
Publisher’s note
All claims expressed in this article are solely those of the authors and do not necessarily represent those of their affiliated organizations, or those of the publisher, the editors and the reviewers. Any product that may be evaluated in this article, or claim that may be made by its manufacturer, is not guaranteed or endorsed by the publisher.
References
Bar-Yoseph, H., Cohen, N., Korytny, A., Andrawus, E. R., Even Dar, R., Geffen, Y., et al. (2019). Risk factors for mortality among carbapenem-resistant enterobacteriaceae carriers with focus on immunosuppression. J. Infect. 78, 101–105. doi: 10.1016/j.jinf.2018.10.003
Carver, T., Thomson, N., Bleasby, A., Berriman, M., Parkhill, J. (2009). DNAPlotter: circular and linear interactive genome visualization. Bioinformatics 25, 119–120. doi: 10.1093/bioinformatics/btn578
Dong, H., Liu, Z., Wu, Z., Zhang, T., Xia, Z., Zhao, Y., et al. (2023). Characterization of a Conjugative Hybrid Plasmid Coharboring bla(KPC-2) and bla(IMP-4) in a Klebsiella quasipneumoniae Clinical Isolate. Microbiol Spectr. 11, e0261622. doi: 10.1128/spectrum.02616-22
Ernst, C. M., Braxton, J. R., Rodriguez-Osorio, C. A., Zagieboylo, A. P., Li, L., Pironti, A., et al. (2020). Adaptive evolution of virulence and persistence in carbapenem-resistant Klebsiella pneumoniae. Nat. Med. 26, 705–711. doi: 10.1038/s41591-020-0825-4
Feng, Y., Zou, S., Chen, H., Yu, Y., Ruan, Z. (2021). BacWGSTdb 2.0: a one-stop repository for bacterial whole-genome sequence typing and source tracking. Nucleic Acids Res. 49, D644–D650. doi: 10.1093/nar/gkaa821
Ghaly, T. M., Tetu, S. G., Gillings, M. R. (2021). Predicting the taxonomic and environmental sources of integron gene cassettes using structural and sequence homology of attC sites. Commun. Biol. 4, 946. doi: 10.1038/s42003-021-02489-0
Gorbunova, V., Seluanov, A., Mita, P., Mckerrow, W., Fenyo, D., Boeke, J. D., et al. (2021). The role of retrotransposable elements in ageing and age-associated diseases. Nature 596, 43–53. doi: 10.1038/s41586-021-03542-y
Gu, D., Dong, N., Zheng, Z., Lin, D., Huang, M., Wang, L., et al. (2018). A fatal outbreak of ST11 carbapenem-resistant hypervirulent Klebsiella pneumoniae in a Chinese hospital: a molecular epidemiological study. Lancet Infect. Dis. 18, 37–46. doi: 10.1016/S1473-3099(17)30489-9
Gurevich, A., Saveliev, V., Vyahhi, N., Tesler, G. (2013). QUAST: quality assessment tool for genome assemblies. Bioinformatics 29, 1072–1075. doi: 10.1093/bioinformatics/btt086
Hala, S., Antony, C. P., Alshehri, M., Althaqafi, A. O., Alsaedi, A., Mufti, A., et al. (2019). First report of Klebsiella quasipneumoniae harboring bla(KPC-2) in Saudi Arabia. Antimicrob Resist. Infect. Control 8, 203. doi: 10.1186/s13756-019-0653-9
Harmer, C. J., Hall, R. M. (2024). IS26 and the IS26 family: versatile resistance gene movers and genome reorganizers. Microbiol Mol. Biol. Rev. 88, e0011922. doi: 10.1128/mmbr.00119-22
Harmer, C. J., Moran, R. A., Hall, R. M. (2014). Movement of IS26-associated antibiotic resistance genes occurs via a translocatable unit that includes a single IS26 and preferentially inserts adjacent to another IS26. mBio 5, e01801–e01814. doi: 10.1128/mBio.01801-14
Holt, K. E., Wertheim, H., Zadoks, R. N., Baker, S., Whitehouse, C. A., Dance, D., et al. (2015). Genomic analysis of diversity, population structure, virulence, and antimicrobial resistance in Klebsiella pneumoniae, an urgent threat to public health. Proc. Natl. Acad. Sci. U S A 112, E3574–E3581. doi: 10.1073/pnas.1501049112
Hua, X., Zhang, L., Moran, R. A., Xu, Q., Sun, L., Van Schaik, W., et al. (2020). Cointegration as a mechanism for the evolution of a KPC-producing multidrug resistance plasmid in Proteus mirabilis. Emerg Microbes Infect. 9, 1206–1218. doi: 10.1080/22221751.2020.1773322
Kenyon, J. J., Hall, R. M. (2022). Updated analysis of the surface carbohydrate gene clusters in a diverse panel of acinetobacter baumannii isolates. Antimicrob Agents Chemother. 66, e0180721. doi: 10.1128/AAC.01807-21
Krapp, F., Ozer, E. A., Qi, C., Hauser, A. R. (2018). Case report of an extensively drug-resistant klebsiella pneumoniae infection with genomic characterization of the strain and review of similar cases in the United States. Open Forum Infect. Dis. 5, ofy074. doi: 10.1093/ofid/ofy074
Krzywinski, M., Schein, J., Birol, I., Connors, J., Gascoyne, R., Horsman, D., et al. (2009). Circos: an information aesthetic for comparative genomics. Genome Res. 19, 1639–1645. doi: 10.1101/gr.092759.109
Lam, M. M. C., Wick, R. R., Judd, L. M., Holt, K. E., Wyres, K. L. (2022). Kaptive 2.0: updated capsule and lipopolysaccharide locus typing for the Klebsiella pneumoniae species complex. Microb Genom 8. doi: 10.1099/mgen.0.000800
Lam, M. M. C., Wick, R. R., Watts, S. C., Cerdeira, L. T., Wyres, K. L., Holt, K. E. (2021). A genomic surveillance framework and genotyping tool for Klebsiella pneumoniae and its related species complex. Nat. Commun. 12.4188 doi: 10.1038/s41467-021-24448-3
Li, X., Xie, Y., Liu, M., Tai, C., Sun, J., Deng, Z., et al. (2018). oriTfinder: a web-based tool for the identification of origin of transfers in DNA sequences of bacterial mobile genetic elements. Nucleic Acids Res. 46, W229–W234. doi: 10.1093/nar/gky352
Liu, H., Moran, R. A., Chen, Y., Doughty, E. L., Hua, X., Jiang, Y., et al. (2021). Transferable Acinetobacter baumannii plasmid pDETAB2 encodes OXA-58 and NDM-1 and represents a new class of antibiotic resistance plasmids. J. Antimicrob Chemother. 76, 1130–1134. doi: 10.1093/jac/dkab005
Liu, B., Zheng, D., Zhou, S., Chen, L., Yang, J. (2022). VFDB 2022: a general classification scheme for bacterial virulence factors. Nucleic Acids Res. 50, D912–D917. doi: 10.1093/nar/gkab1107
Long, S. W., Linson, S. E., Ojeda Saavedra, M., Cantu, C., Davis, J. J., Brettin, T., et al. (2017). Whole-Genome Sequencing of Human Clinical Klebsiella pneumoniae Isolates Reveals Misidentification and Misunderstandings of Klebsiella pneumoniae, Klebsiella variicola, and Klebsiella quasipneumoniae. mSphere 2. doi: 10.1128/mSphereDirect.00290-17
Ma, Z., Qian, C., Yao, Z., Tang, M., Chen, K., Zhao, D., et al. (2024). Coexistence of plasmid-mediated tmexCD2-toprJ2, bla(IMP-4), and bla(NDM-1) in Klebsiella quasipneumoniae. Microbiol Spectr. 12, e0387423. doi: 10.1128/spectrum.03874-23
Price, M. N., Dehal, P. S., Arkin, A. P. (2009). FastTree: computing large minimum evolution trees with profiles instead of a distance matrix. Mol. Biol. Evol. 26, 1641–1650. doi: 10.1093/molbev/msp077
Ruan, Z., Yu, Y., Feng, Y. (2020). The global dissemination of bacterial infections necessitates the study of reverse genomic epidemiology. Brief Bioinform. 21, 741–750. doi: 10.1093/bib/bbz010
Sakai, J., Maesaki, S. (2022). Prevalence, molecular characterization, and drug susceptibility of extended-spectrum beta-lactamase-producing Klebsiella pneumoniae, K. quasipneumoniae and K. variicola in Japan. Microb Pathog 169, 105663. doi: 10.1016/j.micpath.2022.105663
Seemann, T. (2014). Prokka: rapid prokaryotic genome annotation. Bioinformatics 30, 2068–2069. doi: 10.1093/bioinformatics/btu153
Siguier, P., Perochon, J., Lestrade, L., Mahillon, J., Chandler, M. (2006). ISfinder: the reference centre for bacterial insertion sequences. Nucleic Acids Res. 34, D32–D36. doi: 10.1093/nar/gkj014
Tatusova, T., Dicuccio, M., Badretdin, A., Chetvernin, V., Nawrocki, E. P., Zaslavsky, L., et al. (2016). NCBI prokaryotic genome annotation pipeline. Nucleic Acids Res. 44, 6614–6624. doi: 10.1093/nar/gkw569
Tian, C., Xing, M., Zhao, Y., Fan, X., Bai, Y., Fu, L., et al. (2022). Whole genome sequencing of OXA-232-producing wzi93-KL112-O1 carbapenem-resistant Klebsiella pneumoniae in human bloodstream infection co-harboring chromosomal ISEcp1-based bla (CTX-M-15) and one rmpA2-associated virulence plasmid. Front. Cell Infect. Microbiol 12, 984479. doi: 10.3389/fcimb.2022.984479
Wick, R. R., Judd, L. M., Gorrie, C. L., Holt, K. E. (2017). Unicycler: Resolving bacterial genome assemblies from short and long sequencing reads. PloS Comput. Biol. 13, e1005595. doi: 10.1371/journal.pcbi.1005595
Wyres, K. L., Cahill, S. M., Holt, K. E., Hall, R. M., Kenyon, J. J. (2020). Identification of Acinetobacter baumannii loci for capsular polysaccharide (KL) and lipooligosaccharide outer core (OCL) synthesis in genome assemblies using curated reference databases compatible with Kaptive. Microb Genom 6. doi: 10.1099/mgen.0.000339
Keywords: K. quasipneumoniae, WGS, ST852, NDM-1, KPC-2, plasmid structure
Citation: Tian C, Zhao Y, Dong S, You Z, Chen J, Xu H, Fang Y and Zhang Y (2025) Emergence and characterization of a ST852 Klebsiella quasipneumoniae clinical isolate coharboring blaNDM-1 and blaKPC-2 in China. Front. Cell. Infect. Microbiol. 15:1564277. doi: 10.3389/fcimb.2025.1564277
Received: 21 January 2025; Accepted: 21 April 2025;
Published: 09 May 2025.
Edited by:
Jinxin Zhao, Monash University, AustraliaReviewed by:
Vittoria Mattioni Marchetti, University of Pavia, ItalyAbid Ali, Texas A and M University, United States
Copyright © 2025 Tian, Zhao, Dong, You, Chen, Xu, Fang and Zhang. This is an open-access article distributed under the terms of the Creative Commons Attribution License (CC BY). The use, distribution or reproduction in other forums is permitted, provided the original author(s) and the copyright owner(s) are credited and that the original publication in this journal is cited, in accordance with accepted academic practice. No use, distribution or reproduction is permitted which does not comply with these terms.
*Correspondence: Yapei Zhang, aHBienlwQDE2My5jb20=; Yuejuan Fang, MTUxNjgzMjY3MTRAMTYzLmNvbQ==