- School of Education, University of California, Riverside, Riverside, CA, United States
Teachers’ cognition and affect can influence their pedagogical choices, which in turn can shape the development of their students’ spatial skills. Here we examined the relations between primary teachers’ spatial cognition, affect, and their inclination for choosing teaching practices, such as models and diagrams, that engage and facilitate spatial learning. Seventy-seven K-6 teachers completed measures of spatial skills, spatial anxiety, spatial habits of mind, and a measure of preferences for spatial pedagogy. Additionally, we assessed and controlled for their general reasoning and general anxiety. Results revealed primary teachers’ spatial skills were positively associated with their spatial habits of mind and their preference for using spatial strategies for teaching science content. Yet, their pedagogical preferences for teaching math content varied with their teaching experience. Teachers’ spatial skills and spatial anxiety were not related. These findings have implications for how to leverage teacher education programs to bolster primary STEM learning and outcomes.
1 Introduction
Teachers’ skills, characteristics, and attitudes shape students’ skills, knowledge, and interests through their pedagogical choices (e.g., Otumfuor and Carr, 2017; Yang et al., 2020). The strategies and instructional techniques a teacher employs to convey formal learning content not only influences how well students learn the information, but also acquire crucial associated skill sets. For instance, teachers utilizing inquiry-based learning for teaching science also facilitate the development of students’ critical thinking skills (e.g., Duran and Dökme, 2016), and teachers who incorporate peer-to-peer learning into math lessons bolster students’ collaboration and communication skills (e.g., Klang et al., 2021; Sofroniou and Poutos, 2016). While critical thinking, collaboration, and communication skills are formally acknowledged and are emphasized as fundamental aspects of pre-university education in nations around the globe (e.g., Ananiadou and Claro, 2009), research indicates that teachers’ pedagogical decisions also shape other less recognized skill sets, such as spatial skills (e.g., Gilligan-Lee et al., 2022), which are critical to students’ academic achievement (Atit et al., 2020a; Burke et al., 2020).
Spatial skills are the ability to visualize and reason about the static (non-moving) and dynamic (moving) spatial relations within (e.g., shape and size of an object, the folding and unfolding of an object) and between objects (e.g., the distance between two or more stationary or moving objects). This skill set plays a critical role in daily activities (e.g., Newcombe and Shipley, 2015; Uttal et al., 2013) such as when picturing the number of shoes you can fit into your carry-on suitcase for your upcoming trip or when planning the most efficient path to the store by visualizing two possible routes to drive your car there. Additionally, strong spatial skills are important for students’ learning of science, technology, engineering, and mathematics (STEM) disciplines (e.g., reasoning about fractions, molecules, geologic formations, and how propellers work on an engine) across all educational levels (e.g., Atit et al., 2021; Hodgkiss et al., 2018; Kinach, 2012; Mix et al., 2017).
Longitudinal studies reveal that spatial skills established early in education are fundamental to concurrent STEM achievement (e.g., Hodgkiss et al., 2018; Mix et al., 2016), and predictive of STEM attainment in subsequent years (e.g., Hanline et al., 2010; Li and Geary, 2013). Primary students’ spatial skills are positively related to their math and science achievement assessed in the same year (Hodgkiss et al., 2018; Mix et al., 2016) and to math achievement the following year (Geer et al., 2019). Despite their importance, mathematics and science continue to be challenging subjects for students in many nations (OECD, 2023). Given the strong connection between proficiency in STEM subjects and opportunities for equity and social mobility (e.g., Hoskins and Barker, 2020; Wolniak et al., 2008), identifying how to facilitate the development of students’ STEM content understanding by bolstering their early spatial skills is a critical area of research.
Fortunately, evidence indicates spatial skills are malleable and can be improved with exposure and practice. A meta-analysis synthesizing the findings from 217 studies revealed that spatial skills can be enhanced through training and repetition, and that the effects of training are not limited to the task at hand. The learning gains acquired through training on a specific kind of task (e.g., mental rotation—an intrinsic dynamic spatial task) were found to transfer to other spatial activities within the same category (e.g., paper folding—another intrinsic-dynamic spatial task), as well as transfer to different tasks in different categories (e.g., water level task—an extrinsic static spatial task; Uttal et al., 2013).
While benefiting from deliberate practice, the improvement of spatial skills is not dependent on systematic training and repetition. Informal experiences engaging in spatial tasks, such as playing with construction toys and video games, can also enhance students’ spatial skills (e.g., Bediou et al., 2018; Jirout and Newcombe, 2015; Levine et al., 2012). The frequency with which four- to seven-year-old children played with puzzles, blocks, and board games was related to their performance on the Block Design subtest of the Wechsler Preschool and Primary Scale of Intelligence–Fourth Edition, while other types of activities were not related to Block Design score (Jirout and Newcombe, 2015). Similarly, a recent meta-analysis examining the effects of playing video games on cognition established that individuals who have greater experience playing action video games (e.g., Call of Duty, Halo) have stronger spatial skills than those who report little to no action video game experience (Bediou et al., 2018).
Moreover, engaging in spatial tasks can improve students’ STEM learning and outcomes (Hawes et al., 2022; Miller and Halpern, 2013). Hawes et al. (2022) found a positive effect of training spatial skills on mathematics performance relative to control participants ages three to twenty. Similarly, Miller and Halpern (2013) showed that training spatial skills in undergraduate students improved their introductory physics exam scores relative to control students. However, one limitation of the research on the effects of spatial training on STEM learning and reasoning is that the activities used to bolster students’ spatial skills are often aimed at improving isolated skills independent of teacher pedagogical delivery and STEM lesson contexts. For instance, the spatial training implemented by Miller and Halpern (2013) was completing 12 h of spatial workbook exercises, such as creating orthographic and isometric drawings. Thus, the impact of bolstering students’ spatial skills through domain-specific classroom activities (e.g., drawing a diagram of the water cycle) is not well understood. Few studies have examined spatial learning when embedded as a formal part of curriculum planning. Thus, while the importance of spatial skills is well-documented, their integration into teaching practices remains unexplored.
Spatial learning, how students learn and acquire spatial skills, is not a separate formal aspect of the pre-university educational curriculum in most nations (e.g., Gilligan-Lee et al., 2022). Yet, teachers play a substantive role in the development of students’ spatial skills. Students with primary teachers who report high anxiety for completing spatial tasks (i.e., spatial anxiety) show less positive growth in their spatial skills than students of teachers with low spatial anxiety across a school year (Gunderson et al., 2013). Spatial anxiety refers to the feelings of tension or stress that arise when an individual is required to perform tasks involving spatial thinking or spatial reasoning (i.e., spatial tasks; Atit and Rocha, 2021). Examples of spatial tasks include activities such as reading maps, visualizing how two-dimensional furniture assembly instructions relate to the assembly of three-dimensional furniture pieces, interpreting diagrams, or solving problems that require mental manipulation of shapes and figures. Individuals with high spatial anxiety may avoid or struggle to complete spatial tasks (Geer et al., 2024).
Moreover, teachers’ spatial skills and affect have been linked to their use of instructional methods (i.e., spatial pedagogical practices), such as gestures, models, and diagrams, that support students’ spatial learning (e.g., Atit and Rocha, 2021; Gilligan-Lee et al., 2023). Spatial pedagogical practices explicitly engage students’ spatial skills when learning course content. Studies have found that K-12 teachers with high spatial anxiety report more regularly implementing spatial pedagogical activities during informal learning periods in their classrooms than during formal curriculum (Gilligan-Lee et al., 2023). K-12 teachers with strong spatial skills were also observed using a greater number of spatial pedagogical practices when teaching geometry (Otumfuor and Carr, 2017). Additionally, K-12 teachers’ spatial skills have been positively linked with their reported frequency of implementing spatial pedagogical practices into their classroom lessons (Atit and Rocha, 2021).
One factor unexamined in teachers is their spatial habits of mind (SHOM). Kim and Bednarz (2013) defined SHOM as “an internalized thinking process that uses spatial ways of thinking, such as the appreciation of spatial concepts and reasoning and the spatial representation of ideas (e.g., visualization)” (pg. 165). Where basic spatial skills can be task-specific and procedural, SHOM are dispositional, reflecting how someone habitually thinks and reasons over time. Thus, SHOM represents a deeper cognitive orientation toward using spatial tools and perspectives to understand and solve problems. Individuals espousing greater SHOM have a greater proclivity to adopt cognitive methods that use spatial ways of thinking to carry out every day and professional activities (Kim and Bednarz, 2013). For example, teachers with greater SHOM may use mental visualization to plan a classroom layout where all of the students will have an unobstructed view of the teacher. Moreover, engaging in spatially intensive curricular activities, such as using geographical information systems, can enhance one’s SHOM (e.g., Cortes et al., 2022). Learning about primary teachers’ SHOM may provide further insight into their instructional decisions.
In addition to teachers’ cognition and affect, formal teacher training may also shape their pedagogical choices. In the United States, teachers who have the credentials to teach at the primary educational level (i.e., multiple subject teaching credential) have undergone distinct training from teachers who have a credential to teach at the secondary level (i.e., single subject teaching credential) (Commission on Teacher Credentialing, 2016). Yet, despite differences in their formal training, Rocha et al. (2022) found that teachers’ spatial skills and affect (i.e., spatial anxiety), as well as their reported frequency of using spatial teaching practices, did not vary by teacher type (primary versus secondary). However, their study only explored if teachers’ use of spatial pedagogy varied based on their formal certification and did not examine the context of when that training occurred.
In the United States, the most recent substantive changes in science and mathematics curricular standards occurred with the implementation of the Next Generation Science Standards [(NGSS), National Research Council, 2013] and the Common Core State Standards in Mathematics [(CCSS-Math) as part of the Common Core State Standards Initiative, 2010] in 2013. Compared to their policy predecessor, the No Child Left Behind Act of 2001 (NCLB, 2002), the NGSS and CCSS-Math implicitly challenged K-12 teachers to incorporate spatial thinking by emphasizing pedagogy that asked students to engage in practices such as analyzing patterns, data, and relationships between scales and objects in space, etc (LaDue et al., 2015; Moore et al., 2015; Pruitt, 2014). For example, an NGSS standard for fourth-grade students is to analyze and interpret data from maps to describe patterns of Earth’s features (National Research Council, 2013). Studies on teacher preparation detail how educational reforms at the state and national levels result in curriculum-aligned reformations in teacher education programs (e.g., ByBee, 2014; Merritt et al., 2018). Yet, the effect of these changes on teachers’ cognition, affect, and pedagogical choices are unknown.
Building on prior research (Atit et al., 2018; Rocha et al., 2022), this study aims to answer three research questions. First, we ask if primary teachers’ spatial cognition, affect, and preferences for spatial pedagogical practices when teaching mathematics and science differ based on whether or not they began teaching before or after recent educational reforms? Informed by studies showing that educational reforms result in curriculum-aligned reformations in teacher education programs (e.g., ByBee, 2014), that spatial cognitive and affective characteristics are malleable and improve with exposure and engagement in spatial tasks (e.g., Cortes et al., 2022; Kim and Bednarz, 2013; Uttal et al., 2013), we expect primary teachers who began teaching prior to the implementation of NGSS and CCSS-Math to have weaker spatial skills and SHOM, higher spatial anxiety, and show a lower inclination for implementing spatial instructional strategies during mathematics and science instruction.
Second, we ask what are the relations between primary teachers’ spatial skills, spatial anxiety, and SHOM? Based on studies suggesting that strong SHOM are a result of sustained engagement in spatially-demanding tasks (Cortes et al., 2022; Kim and Bednarz, 2013) and are indicative of achieving spatial literacy (National Research Council, 2006), we hypothesize that primary teachers’ spatial skills will be positively associated with SHOM, while spatial anxiety will be negatively associated with SHOM.
Third, we ask how are primary teachers’ spatial cognition and affect related to their preferences for using spatial pedagogical practices for teaching mathematics and science content? Researchers have found that teachers’ spatial skills are positively associated with their reported use of spatial instructional practices in the classroom. However, spatial anxiety is not significantly related to teachers’ reported use of spatial instruction (Atit and Rocha, 2021). Habits of mind are associated with a consistent pattern in thinking which can influence one’s decision making and problem solving approaches (Costa and Kallick, 2008; Cuoco et al., 1996). In line with this research, we hypothesize that primary teachers’ spatial skills and SHOM will be positively associated with their inclination for implementing spatial teaching practices in science and mathematics curriculum, but spatial anxiety will not be a significant explanatory factor.
2 Materials and methods
2.1 Participants
Participants of this study were eighty-two primary school pre-service and in-service teachers certified in the state of California in the United States. California had adopted NGSS and CCSS-Math for pre-undergraduate mathematics and science education by 2013 (California Association of Science Educators, 2024; California Department of Education, 2024). Data from five participants were excluded due to failure to follow study instructions. Thus, data from 77 participants (male = 4, female = 72, unreported = 1) were examined. An a priori power analysis estimated that for an alpha of 0.05, 77 participants provided 80% power to detect a medium effect size (f2 = 0.15) for a linear multiple regression for a fixed model examining for R2 increase, with three tested predictors and eight total predictors. Teachers were recruited through social media, state-wide educator conferences, and professional networks. Informed consent was conducted before their participation. Participants were compensated with a $50 e-gift card. The sample predominantly consisted of teachers pursuing or having earned their credentials for teaching multiple subjects (82%), see Table 1. Reported teaching experience ranged from preservice teachers (students enrolled in a teacher education program who are engaged in fieldwork teaching in a classroom) to teachers with more than 20 years of experience (see Figure 1).
2.2 Measures
2.2.1 Spatial Skills and Spatial Anxiety Assessment
This measure was adapted from Gagnier et al. (2021) and consisted of 34 items assessing participants’ spatial skills and spatial anxiety. Seventeen spatial skills items were selected from psychometric assessments of mental rotation, perspective-taking, visualizing cross-sections, paper folding, disembedding, and reasoning about isometric projections to directly measure participants’ spatial skills. For each item, participants had to solve the spatial problem and select the correct answer choice from the response options provided. Participants’ spatial skill score on the assessment was the sum of the number of items solved correctly.
To measure spatial anxiety, after each spatial skills item, participants answered the following question: “How anxious do you feel when asked to solve this problem?” Response options included (0) not at all, (1) slightly, (2) moderately, (3) very, or (4) extremely. Using Gagnier et al. (2021) scoring method, scores were computed for both parts of the measure (i.e., spatial skills and spatial anxiety) separately. Cronbach’s α indicated an acceptable internal reliability for the spatial skills (α = 0.73) and spatial anxiety (α = 0.91) components.
2.2.2 Spatial Habits of Mind Inventory-Revised (SHOMI-R)
This measure was adapted from Kim and Bednarz (2013) for general use by revising or removing any domain-specific items, with the aim of increasing the applicability of the measure to a general audience which includes teachers. For example, “I have difficulty in explaining spatial concepts such as scale and map projection to my friends” was modified to “I have difficulty in explaining spatial concepts such as patterns, arrangements, or location of objects to my friends.” Response options included “strongly disagree,” “disagree,” “neutral,” “agree,” and “strongly agree.” In the revised version, the following four subscales were retained from the original measure: (1) pattern recognition, (2) spatial recognition, (3) visualization, and (4) spatial concept usage. The SHOMI-R includes 23 items and has excellent internal reliability (α = 0.92).
2.2.3 Measure of preference for spatial pedagogy (MPSP)
The MPSP is a researcher-created instrument assessing primary teachers’ inclination for using “more spatial” versus “less spatial” instructional tools in specified math and science teaching contexts. Creation of this measure was informed by the California Frameworks for Mathematics and Science from the California State Board of Education (2015, 2016). In each of the seven items, participants are provided a hypothetical teaching scenario followed by four potential methods of teaching it. Scenarios varied in how much they engage students’ spatial thinking. For each strategy, participants were asked to rate their preference from “not rely on it at all” to “rely on it heavily.”
An example item includes:
“To teach students about mathematical equivalence and the meaning of the ‘equal’ (=) sign, I would use…”
1. vocabulary prompting to emphasize the definition of “equal”
2. a drawing/sketch of what each side of an equation represents (i.e., drawing four stars plus five stars being equal to three stars plus six stars)
3. a physical scale to show equal manipulative units on each side creating a balance
4. a mnemonic or song that helps students to remember that both sides of the equation have to be equal.
Participants’ score on each item is the sum of their ratings for each method of teaching. “More spatial” methods were scored from 1 (not rely on it at all) to 4 (rely on it heavily). Responses for “less spatial” methods were reverse coded. Participants received three different scores on this measure: (1) overall MPSP, (2) math pedagogy, and (3) science pedagogy. For all three score types, teachers with higher scores can be said to display a greater preference for implementing spatial pedagogical practices. The full MPSP with its two subscales, how it was created, and more detailed scoring information is provided in Supplementary Appendix A.
2.2.4 International Cognitive Ability Resource (ICAR)–verbal reasoning and matrix reasoning
The verbal reasoning and matrix reasoning from the International Cognitive Ability Resource Project (ICAR; Condon and Revelle, 2014) were used to assess general reasoning (Kyllonen and Christal, 1990).
2.2.4.1 Verbal reasoning
The verbal reasoning measure consisted of 16 multiple-choice items assessing participants’ logic, vocabulary, and general knowledge. An example item includes “If the day after tomorrow is two days before Thursday, then what day is it today?” Participants were asked to choose the correct answer from the following: (a) Friday, (b) Monday, (c) Wednesday, (d) Saturday, (e) Tuesday, and (f) Sunday. Scores were calculated as the total number of correct responses (The International Cognitive Ability Resource Team, 2014). The measure had acceptable reliability in our sample (α = 0.69).
2.2.4.2 Matrix reasoning
Matrix reasoning, a measure of fluid reasoning, consists of 11 multiple-choice items similar to Raven’s Progressive Matrices. In each item, participants were presented with a 3 × 3 array of geometric shapes where one of the nine shapes is missing. The instructions asked participants to respond by selecting one of the six presented geometric shapes to best complete the array. Participants’ score on the assessment was calculated from the sum of the number of correct responses (The International Cognitive Ability Resource Team, 2014). Using Cronbach’s alpha, the measure had acceptable reliability in our sample (α = 0.70).
2.2.5 Trait Anxiety Inventory
The Trait Anxiety Inventory (Spielberger et al., 1970) is a 20-item measure assessing general anxiety. For each item, participants were asked to select the response that corresponds to how frequently they experience general feelings of anxiety. An example item is, “I worry too much over something that really doesn’t matter.” Response options ranged from (0) almost never to (3) almost always. Participants’ score on the measure was the sum of all of their responses. Higher total scores indicate higher levels of general anxiety. Cronbach’s α indicated excellent internal reliability (α = 0.93).
2.2.6 Demographics and background questionnaire
This researcher-created questionnaire included items asking about participants’ demographic and professional background, such as about prior teaching experience and training, race, gender, educational attainment, and the current grade they teach. Teachers’ responses to years of reported teaching experience were used to classify teachers into two categories. Teachers who identified as preservice, or reported less than 10 years of experience were designated as post-reform teachers as they would have received their teaching credentials after 2013. Teachers who reported more than 10 years of teaching experience, receiving teaching credentials prior to 2013, were designated as pre-reform teachers. The full Demographics and Background Questionnaire is provided in Supplementary Appendix B.
2.3 Procedure
This study was administered online using Qualtrics. After providing consent, participants completed the measures in the following order: Spatial Skills and Spatial Anxiety Assessment, SHOMI-R, MPSP, ICAR verbal and matrix reasoning, and the Trait Anxiety Inventory. The Demographics and Background Questionnaire was administered last to minimize potential effects of stereotype threat (e.g., Spencer et al., 1999; Steele and Aronson, 1995).
3 Results
Analyses were conducted using R version 4.4.0 (R Core Team, 2023). Preliminary analyses were conducted to assess the normality of the data examined and to identify associations between variables. The descriptive statistics, skewness, kurtosis, and results from the Kolmogorov–Smirnov test of normality for the Spatial Skills and Spatial Anxiety Assessment, SHOMI-R, MPSP, ICAR verbal reasoning, ICAR matrix reasoning, and Trait Anxiety Inventory are provided in Table 1 in Supplementary Appendix C. Results of the Kolmogorov–Smirnov tests revealed that the data do not follow a normal distribution. Thus, Spearman’s correlations between all measures, teaching experience, and teachers’ educational level were conducted and are presented in Table 2 in Supplementary Appendix C. Spearman’s correlations are non-parametric tests used to assess the strength of the relation between two variables when the data do not follow a normal distribution (e.g., Myers and Sirois, 2006).
Following Cohen’s (1988) conventions, correlational analyses suggest that primary teachers with stronger spatial skills showed stronger SHOM (r = 0.40), greater preference for implementing spatial pedagogical practices (r = 0.23), especially for science content (r = 0.23), and had less spatial anxiety (r = −0.27). Additionally, teachers with stronger SHOM had greater preference for implementing spatial pedagogical practices (r = 0.23), especially for mathematics content (r = 0.24), and had less spatial anxiety (r = −0.33).
3.1 Do spatial cognition, affect, and pedagogical preferences differ between pre-reform and post-reform teachers?
To identify if primary teachers’ spatial skills, SHOM, spatial anxiety, and preferences for implementing spatial pedagogical practices varied between pre-reform and post-reform teachers, Mann–Whitney U tests were conducted for each measure (see Table 2). Results indicate that there was no difference between pre-reform and post-reform teachers except for on the Math Pedagogy subtest. Pre-reform teachers reported greater spatial pedagogical preferences for teaching mathematics concepts than post-reform teachers. Regression analyses, shown Tables 3, 4 in Supplementary Appendix C, reveal that these results remain unchanged even after accounting for teachers’ general anxiety and general reasoning skills. To ensure that the results are not occluded by general reasoning skills or general anxiety, we control for teachers’ matrix reasoning, verbal reasoning, and Trait Anxiety Inventory scores in all subsequent analyses.
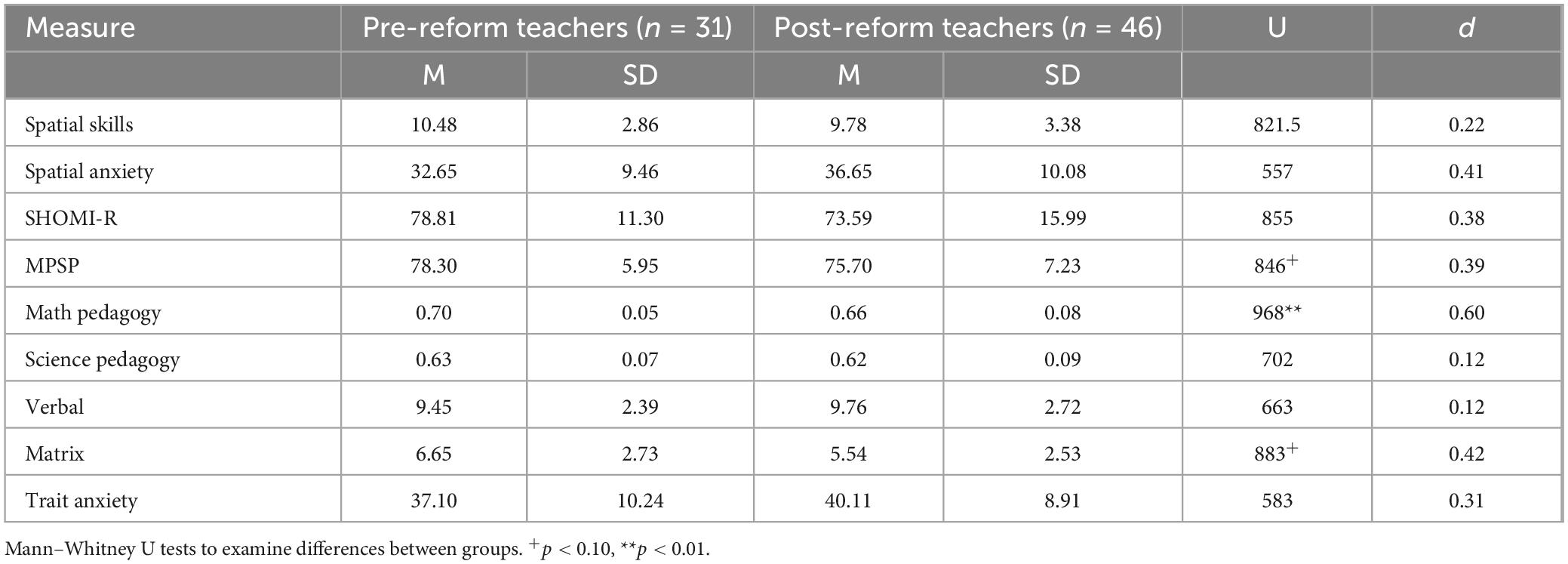
Table 2. Results of Mann–Whitney U tests examining differences between pre and post-reform primary teachers.
3.2 What are the relations between primary teachers’ spatial skills, spatial anxiety, and SHOM?
To examine the relations between teachers’ spatial skills, spatial anxiety, and SHOM, two additional regression models were conducted. Model 1 examined if teachers’ spatial skills score was related to their spatial anxiety score and Model 2 examined if teachers’ spatial skills and spatial anxiety were associated with their SHOM. The results, presented in Table 3, indicate that there was no relation between spatial skills and spatial anxiety (Model 1). However, spatial skills were positively related to SHOMI-R score, but spatial anxiety was not associated with SHOMI-R (Model 2). These results suggest that after accounting for teachers’ general reasoning skills and general anxiety, primary teachers with stronger spatial skills also demonstrate greater SHOM. Yet, their spatial skills are not associated with their spatial anxiety, and spatial anxiety is not associated with SHOM.
3.3 Do teachers’ spatial skills, spatial anxiety, and SHOM relate to their preferences for spatial pedagogical practices?
To understand if and how teachers’ spatial skills, spatial anxiety, and SHOM were related to their preferences for using spatial pedagogical practices, three additional regression models were conducted and compared. As prior analyses revealed that teachers’ pedagogical preferences vary by when they started teaching, we also controlled for teacher category (i.e., pre-reform versus post-reform) in these analyses. Results, shown in Table 4, revealed that primary teachers’ spatial skills, spatial anxiety, and SHOMI-R score do not relate to their overall preferences for implementing spatial pedagogical practices (Model 3) or specifically their preferences when teaching math (Model 4). However, primary teachers’ spatial skills are positively related to their preferences for using spatial pedagogical practices when teaching science (Model 5). Teachers with stronger spatial skills showed a greater preference for using spatial pedagogy for teaching science, even after accounting for their general reasoning, general anxiety, and when they started teaching.
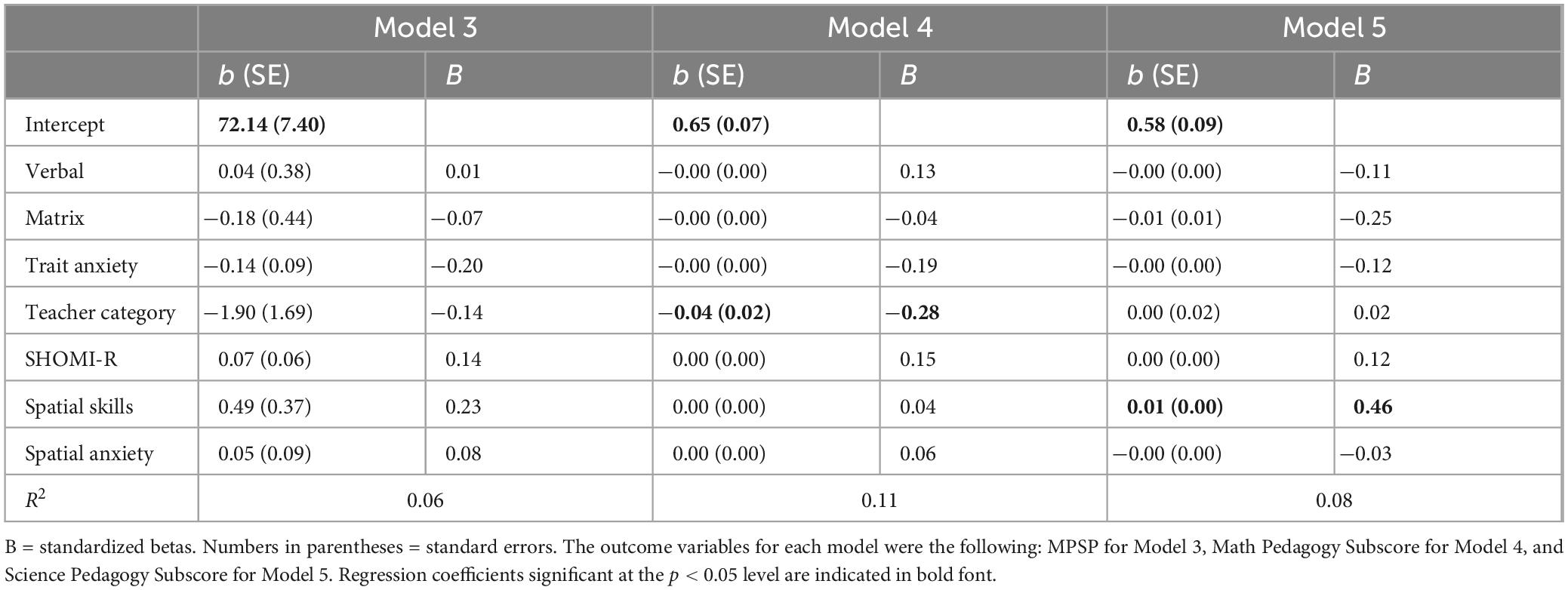
Table 4. Regressions examining the relations between teachers’ spatial cognition, affect, and spatial pedagogical preferences.
4 Discussion
Teachers play a critical role in shaping students’ learning and outcomes (e.g., Ball et al., 2005; Perera and John, 2020). This study examined the links between primary teachers’ spatial cognition, affect, and their inclination for choosing teaching practices that bolster students’ spatial skills during math and science instruction. Additionally, this study investigated whether primary teachers’ spatial cognition, affect, and preferences for using spatial pedagogical practices during STEM content varied based on their teaching start date–before or after educational reforms that emphasized spatial thinking (e.g., Pruitt, 2014; Moore et al., 2015).
Findings revealed that after accounting for their general reasoning and general anxiety, primary teachers’ spatial skills were positively associated with their SHOM. Additionally, teachers who began teaching before the most recent national educational reforms for pre-undergraduate mathematics and science showed a greater preference for including spatial pedagogy when teaching mathematics than teachers who began teaching after the reforms. Our study also found that primary teachers’ preferences for implementing spatial pedagogical practices when teaching science did not vary based on their training time point. However, they did vary based on teachers’ spatial skills. Teachers’ with stronger spatial skills showed a greater preference for using spatial teaching strategies in science regardless of educational training emphasis.
To contextualize these findings, it is important to consider how prior studies have examined the relationship between spatial skills and affective factors; indicating that pre-undergraduate teachers’ spatial anxiety is linked to their spatial skills (e.g., Atit and Rocha, 2021; Rocha et al., 2022). Yet, after accounting for general reasoning and anxiety, we found no relation between primary teachers’ spatial skills and spatial anxiety. Specifically, Atit and Rocha (2021) found that pre-undergraduate teachers’ spatial skills were negatively associated with their spatial anxiety for mental manipulation tasks. Rocha et al. (2022) replicated this finding after accounting for teachers’ general reasoning and anxiety. Differences in measurement tools may explain why our findings diverged from those of prior research (Atit and Rocha, 2021; Rocha et al., 2022). Both Atit and Rocha (2021) and Rocha et al. (2022) used mental rotation to assess teachers’ spatial skills and used the Spatial Anxiety Scale (Lyons et al., 2018) to assess teachers’ spatial anxiety.
The present study was built on the methodology used by Gagnier et al. (2021) and measured spatial skills utilizing a battery of 17 items assessing a variety of spatial skills. To measure teachers’ spatial anxiety, we asked teachers to rate their level of anxiety after completing each spatial problem. Research indicates that there is more than one kind of spatial skill (e.g., Newcombe and Shipley, 2015) and that the relation between spatial skills and spatial anxiety varies by the type of spatial task being assessed (Geer et al., 2024). Additionally, studies have found that problem-specific affective judgments may be more accurate than global and generic assessments of affective states (e.g., math anxiety, academic self-efficacy; Stankov et al., 2017). However, much of this research has been conducted outside of the field of spatial cognition. The inclusion of multiple spatial skill types and the use of problem-specific affective judgments to assess spatial anxiety may have obscured the relationship between spatial skills and spatial anxiety. Future studies should examine if and how the relation between spatial skills and spatial anxiety varies based on task and measurement characteristics.
Spatial skills and SHOM have both been recognized as aspects of spatial cognition essential to STEM reasoning and practice (e.g., Atit et al., 2020b; Jant et al., 2020). However, little research has examined the links between them. We found a positive relation between spatial skills and SHOM in primary teachers. Peterson et al. (2020) also found a positive relation between adolescents’ spatial skills and their SHOM. Moreover, they found that adolescents’ current participation in spatial activities predicted their current spatial skills, but their engagement in spatial activities during childhood predicted their later SHOM. In our research, after accounting for teachers’ general reasoning and general anxiety, we found no relation between teachers’ SHOM and their preferences for using spatial pedagogy. Instead, teachers’ spatial skills were positively linked to their pedagogical choices when teaching science (although not math). These findings suggest that early engagement in spatial tasks supports SHOM development. However, spatial skills appear to play a stronger role in teachers’ current preferences for spatial activities (in this case, during science instruction). To inform how to support the development of these factors, subsequent research should aim to identify how they are causally and longitudinally linked.
4.1 Primary teachers’ preferences for using spatial pedagogical practices when teaching mathematics versus science
Primary school science instruction often relies on textbooks or other educational resources (e.g., Appleton, 2002; Tobin, 1994) due to insufficient teacher preparation for effective science instruction (Trygstad et al., 2013). Primary teachers often do not specialize in science fields and are often not provided the necessary added pedagogical training to teach science content (Shulman, 1986). Thus, they have little STEM content knowledge and STEM pedagogical knowledge, both of which are essential to teaching effectively across the multiple disciplines required by primary science curricula (e.g., Bodzin and Beerer, 2003; Zhou et al., 2023).
Additionally, mastering science content and practices heavily relies on teachers’ engaging in spatial thinking and pedagogy supporting students’ spatial skill development. Spatial skills are positively linked with science achievement across primary, secondary, and undergraduate educational levels (Atit et al., 2025). For instance, understanding patterns in weather and climate (NGSS Standard 3-ESS2-1) requires skill in recording weather data, finding patterns, and making predictions using spatial visualizations. Our study revealed that those primary teachers who have stronger spatial skills showed a greater preference for using spatial pedagogy. Thus, leveraging teacher education programs to enhance teachers’ spatial skills may have implications for enhancing and better supporting the quality of primary science instruction and student outcomes more broadly.
Lastly, primary teachers’ spatial cognition and affect did not relate to their preferences for using spatial pedagogical practices when teaching mathematics. This finding may have resulted because we did not account for teachers’ math anxiety which can negatively impact pedagogical choices in primary classrooms (Schaeffer et al., 2021). Given math anxiety is particularly prevalent among primary teachers (Ganley et al., 2019), future investigations should account for teachers’ math anxiety to further enhance our understanding of the factors that can influence spatial pedagogical choices.
4.2 Limitations
Any study of teaching and pedagogical practices naturally has limitations. One limitation of this research involves how we gathered participants’ years of teaching experience. The item requesting information about the amount of prior teaching experience on our Demographic and Background Questionnaire asked participants to choose a response capturing the most accurate five year interval (e.g., 0–5 years of experience, 6–10 years of experience). We did not collect precise data on what year participants began teaching, nor accounted for possible breaks in service. Thus, not only the length of teaching experience, but when this teaching experience precisely occurred, may have further impacted our study outcomes (e.g., pre-reform teachers report more spatial pedagogy due to more teaching experience and not only as a result of years of experience during policy reform). Future studies should ask teachers to report more detailed information, such as the year in which they started teaching and their years of active service, to provide more accurate insight into the factors shaping their pedagogical choices.
A second limitation of our research is that our measure of teachers’ spatial pedagogical preferences implemented self-report survey methodology. Our survey did not include additional in situ classroom observations, limiting our ability to provide convergent evidence of teacher stated beliefs as being demonstrated during STEM instructional practices. Adopting a mixed-methods approach would be of benefit in future studies because research examining the accuracy of teachers’ reports regarding their own pedagogical choices are mixed (Koziol and Burns, 1986; Ernst et al., 2023).
Third, data collection in our study was limited to teachers only in California to standardize credentialing experiences because teacher training requirements vary from state to state (TEACH, 2021). However, while California is a large state with a robust teaching population that has implemented both NGSS and CCSS-Math, these policy shifts have also been adopted in a number of other states. Only using California as a control variable limits the further generalizability of these initial findings to teachers in other states.
5 Conclusion
This study underlines the importance of examining primary teachers’ spatial cognition and affect to enhance students’ spatial learning. Primary teachers’ spatial skills were found to be linked to their preferences for implementing spatial pedagogy when teaching science content. Inequities in STEM learning and achievement persist and continue to hinder economic (e.g., National Academies of Sciences, Engineering and Medicine, 2016) and social progress (e.g., Garibay and Teasdale, 2019), and early spatial skill development is fundamental for students’ concurrent and future STEM outcomes (e.g., Hanline et al., 2010; Hodgkiss et al., 2018). Thus, studying factors influencing primary teachers’ instructional decisions is critical for improving STEM education and outcomes.
Data availability statement
The datasets presented in this study can be found in online repositories. The names of the repository/repositories and accession number(s) can be found below: The data examined and the data analysis scripts for this study can be found here on the Open Science Framework: https://osf.io/v3dsw/?view_only=7e5bb1892f4b409e9844ffab881e699b.
Ethics statement
The studies involving humans were approved by the Institutional Review Board at the University of California, Riverside. The studies were conducted in accordance with the local legislation and institutional requirements. The ethics committee/institutional review board waived the requirement of written informed consent for participation from the participants or the participants’ legal guardians/next of kin because the study was conducted virtually via the Qualtrics survey platform.
Author contributions
KR: Conceptualization, Data curation, Methodology, Project administration, Writing – original draft. CL: Conceptualization, Supervision, Writing – review and editing. KA: Conceptualization, Formal Analysis, Supervision, Writing – original draft, Writing – review and editing.
Funding
The authors declare that no financial support was received for the research and/or publication of this article.
Conflict of interest
The authors declare that the research was conducted in the absence of any commercial or financial relationships that could be construed as a potential conflict of interest.
Generative AI statement
The authors declare that no Generative AI was used in the creation of this manuscript.
Publisher’s note
All claims expressed in this article are solely those of the authors and do not necessarily represent those of their affiliated organizations, or those of the publisher, the editors and the reviewers. Any product that may be evaluated in this article, or claim that may be made by its manufacturer, is not guaranteed or endorsed by the publisher.
Supplementary material
The Supplementary Material for this article can be found online at: https://www.frontiersin.org/articles/10.3389/feduc.2025.1582737/full#supplementary-material
References
Ananiadou, K., and Claro, M. (2009). 21st Century Skills and Competences for New Millennium Learners in OECD Countries (OECD Education Working Papers). Paris: Organization for Economic Co-Operation and Development (OECD), doi: 10.1787/218525261154
Appleton, K. (2002). Science activities that work: Perceptions of primary school teachers. Res. Sci. Educ. 32, 393–410. doi: 10.1023/a:1020878121184
Atit, K., and Rocha, K. (2021). Examining the relations between spatial skills, spatial anxiety, and K-12 teacher practice. Mind Brain Educ. 6, 139–148. doi: 10.1111/mbe.12274
Atit, K., Miller, D. I., Newcombe, N. S., and Uttal, D. H. (2018). Teachers’ spatial skills across disciplines and education levels: Exploring nationally representative data. Arch. Sci. Psychol. 6, 130–137. doi: 10.1037/arc0000041
Atit, K., Peterson, E. G., Gilligan-Lee, K., and Hawes, Z. (2025). Relations between spatial skills and science achievement: A meta-analysis. Manuscript under revision.
Atit, K., Power, J. R., Pigott, T., Lee, J., Geer, E. A., Uttal, D. H., et al. (2021). Examining the relations between spatial skills and mathematical performance: A meta-analysis. Psychon. Bull. Rev. 29, 699–720. doi: 10.3758/s13423-021-02012-w
Atit, K., Power, J. R., Veurink, N., Uttal, D. H., Sorby, S., Panther, G., et al. (2020a). Examining the role of spatial skills and mathematics motivation on middle school mathematics achievement. Int. J. STEM Educ. 7:38. doi: 10.1186/s40594-020-00234-3
Atit, K., Uttal, D. H., and Stieff, M. (2020b). Situating space: Using a discipline-focused lens to examine spatial thinking skills. Cogn. Res. Principles Implications 5:19. doi: 10.1186/s41235-020-00210-z
Ball, D. L., Hill, H. C., and Bass, H. (2005). Knowing mathematics for teaching: Who knows mathematics well enough to teach third grade, and how can we decide? Am. Educ. 29, 14–17.
Bediou, B., Adams, D. M., Mayer, R. E., Tipton, E., Green, C. S., and Bavelier, D. (2018). Meta-analysis of action video game impact on perceptual, attentional, and cognitive skills. Psychol. Bull. 144, 77–110. doi: 10.1037/bul0000130
Bodzin, A. M., and Beerer, K. M. (2003). Promoting inquiry-based science instruction: The validation of the science teacher inquiry rubric (STIR). J. Elementary Sci. Educ. 15, 39–49. doi: 10.1007/bf03173842
Burke, C., Luu, R., Lai, A., Hsiao, V., Cheung, E., Tamashiro, D., et al. (2020). Making STEM equitable: An active learning approach to closing the achievement gap. Int. J. Active Learn. 5, 71–85. doi: 10.15294/IJAL.V5I2.23933
ByBee, R. W. (2014). NGSS and the next generation of science teachers. J. Sci. Teach. Educ. 25, 211–221. doi: 10.1007/s10972-014-9381-4
California Association of Science Educators (2024). Available online at: https://cascience.org/ngss/key-facts (accessed May 2, 2024).
California Department of Education (2024). What are the Common Core Standards?. Sacramento, CA: California Department of Education.
California State Board of Education (2015). Mathematics Frameworks for California Public Schools: Kindergarten through Grade Twelve. Sacramento, CA: California Department of Education.
California State Board of Education (2016). Science Frameworks for California Public Schools: Kindergarten through Grade Twelve. Sacramento, CA: California Department of Education.
Commission on Teacher Credentialing (2016). Available online at: https://www.ctc.ca.gov/ (accessedn May 2, 2024).
Costa, A., and Kallick, B. (2008). Learning and Leading with Habits of Mind: 16 Essential Characteristics for Success. Arlington: Association for Supervision and Curriculum Development.
Cuoco, A., Goldenberg, E. P., and Mark, J. (1996). Habits of mind: An organizing principle for mathematics curricula. J. Math. Behav. 15, 375–402.
Common Core State Standards Initiative (2010). Common Core State Standards for Mathematics. National Governors Association Center for Best Practices and the Council of Chief State School Officers. Available online at: https://corestandards.org/wp-content/uploads/2023/09/Math_Standards1.pdf
Condon, D. M., and Revelle, W. (2014). The international cognitive ability resource: Development and initial validation of a public-domain measure. Intelligence 43, 52–64. doi: 10.1016/j.intell.2014.01.004
Cortes, R. A., Peterson, E. G., Kraemer, D. J. M., Kolvoord, R. A., Uttal, D. H., Dinh, N., et al. (2022). Transfer from spatial education to verbal reasoning and prediction of transfer from learning-related neural change. Sci. Adv. 8:abo3555. doi: 10.1126/sciadv.abo3555
Duran, M., and Dökme, İ (2016). The effect of the inquiry-based learning approach on student’s critical thinking skills. Eurasia J. Math. Sci. Technol. Educ. 12, 2887–2908. doi: 10.12973/eurasia.2016.02311a
Ernst, H. M., Wittwer, J., and Voss, T. (2023). Do they know what they know? Accuracy in teacher candidates’ self-assessments and its influencing factors. Br. Educ. Res. J. 49, 649–673. doi: 10.1002/berj.3860
Gagnier, K. M., Holochwost, S. J., and Fisher, K. R. (2021). Spatial thinking in science, technology, engineering, and mathematics: Elementary teachers’ beliefs, perceptions, and self-efficacy. J. Res. Sci. Teach. 59, 95–126. doi: 10.1002/tea.21722
Ganley, C. M., Schoen, R. C., LaVenia, M., and Tazaz, A. M. (2019). The construct validation of the math anxiety scale for teachers. AERA Open 5:233285841983970. doi: 10.1177/2332858419839702
Garibay, C., and Teasdale, R. M. (2019). Equity and evaluation in informal STEM education: Equity and evaluation in informal STEM education. New Dir. Eval. 2019, 87–106. doi: 10.1002/ev.20352
Geer, E. A., Barroso, C., Conlon, R. A., Dasher, J. M., and Ganley, C. M. (2024). A meta-analytic review of the relation between spatial anxiety and spatial skills. Psychol. Bull. 150, 464–486. doi: 10.1037/bul0000420
Geer, E. A., Quinn, J. M., and Ganley, C. M. (2019). Relations between spatial skills and math performance in elementary school children: A longitudinal investigation. Dev. Psychol. 55, 637–652. doi: 10.1037/dev0000649
Gilligan-Lee, K. A., Bradbury, A., Bradley, C., Farran, E. K., Van Herwegen, J., Wyse, D., et al. (2023). Spatial thinking in practice: A snapshot of teacher’s spatial activity use in the early years’ classroom. Mind Brain Educ. 17, 107–116. doi: 10.1111/mbe.12352
Gilligan-Lee, K. A., Hawes, Z. C. K., and Mix, K. S. (2022). Spatial thinking as the missing piece in mathematics curricula. npj Sci. Learn. 7:10. doi: 10.1038/s41539-022-00128-9
Gunderson, E. A., Ramirez, G., Beilock, S. L., and Levine, S. C. (2013). Teachers’ spatial anxiety relates to 1st-and 2nd-graders’ spatial learning. Mind Brain Educ. 7, 196–199. doi: 10.1111/mbe.12027
Hanline, M. F., Milton, S., and Phelps, P. C. (2010). The relationship between preschool block play and reading and maths abilities in early elementary school: A longitudinal study of children with and without disabilities. Early Child Dev. Care 180, 1005–1017. doi: 10.1080/03004430802671171
Hawes, Z. C. K., Gilligan-Lee, K. A., and Mix, K. S. (2022). Effects of spatial training on mathematics performance: A meta-analysis. Dev. Psychol. 58, 112–137. doi: 10.1037/dev0001281
Hodgkiss, A., Gilligan, K. A., Tolmie, A. K., Thomas, M. S. C., and Farran, E. K. (2018). Spatial cognition and science achievement: The contribution of intrinsic and extrinsic spatial skills from 7 to 11 years. Br. J. Educ. Psychol. 88, 675–697. doi: 10.1111/bjep.12211
Hoskins, K., and Barker, B. (2020). STEM, Social Mobility and Equality: Avenues for Widening Access. Berlin: Springer Nature.
Jant, E. A., Uttal, D. H., Kolvoord, R., James, K., and Msall, C. (2020). Defining and measuring the influences of GIS-based instruction on students’ STEM-relevant reasoning. J. Geogr. 119, 22–31. doi: 10.1080/00221341.2019.1676819
Jirout, J. J., and Newcombe, N. S. (2015). Building blocks for developing spatial skills: Evidence from a large, representative U.S. sample. Psychol. Sci. 26, 302–310. doi: 10.1177/0956797614563338
Kim, M., and Bednarz, R. (2013). Effects of a GIS course on self-assessment of spatial habits of mind (SHOM). J. Geogr. 112, 165–177. doi: 10.1080/00221341.2012.684356
Kinach, B. M. (2012). Fostering spatial vs. metric understanding in geometry. Math. Teach. 105, 534–540. doi: 10.5951/mathteacher.105.7.0534
Klang, N., Karlsson, N., Kilborn, W., Eriksson, P., and Karlberg, M. (2021). Mathematical problem-solving through cooperative learning—the importance of peer acceptance and friendships. Front. Educ. 6:710296. doi: 10.3389/feduc.2021.710296
Koziol, S. M., and Burns, P. (1986). Teachers’ accuracy in self-reporting about instructional practices using a focused self-report inventory. J. Educ. Res. 79, 205–209. doi: 10.1080/00220671.1986.10885678
Kyllonen, P. C., and Christal, R. E. (1990). Reasoning ability is (little more than) working-memory capacity?! Intelligence 14, 389–433. doi: 10.1016/S0160-2896(05)80012-1
LaDue, N. D., Libarkin, J. C., and Thomas, S. R. (2015). Visual representations on high school biology, chemistry, earth science, and physics assessments. J. Sci. Educ. Technol. 24, 818–834. doi: 10.1007/s10956-015-9566-4
Levine, S. C., Ratliff, K. R., Huttenlocher, J., and Cannon, J. (2012). Early puzzle play: A predictor of preschoolers’ spatial transformation skill. Dev. Psychol. 48, 530–542. doi: 10.1037/a0025913
Li, Y., and Geary, D. C. (2013). Developmental gains in visuospatial memory predict gains in mathematics achievement. PLoS One 8:e70160. doi: 10.1371/journal.pone.0070160
Lyons, I. M., Ramirez, G., and Maloney, E. A. (2018). Spatial anxiety: A novel questionnaire with subscales for measuring three aspects of spatial anxiety. J. Numerical Math. 4, 526–553. doi: 10.5964/jnc.v4i3.154
Merritt, E. G., Chiu, J., Peters-Burton, E., and Bell, R. (2018). Teachers’ integration of scientific and engineering practices in primary classrooms. Res. Sci. Educ. 48, 1321–1337. doi: 10.1007/s11165-016-9604-0
Miller, D. I., and Halpern, D. F. (2013). Can spatial training improve long-term outcomes for gifted STEM undergraduates? Learn. Individ. Dif. 26, 141–152. doi: 10.1016/j.lindif.2012.03.012
Mix, K. S., Levine, S. C., Cheng, Y.-L., Young, C. J., Hambrick, D. Z., and Konstantopoulos, S. (2017). The latent structure of spatial skills and mathematics: A replication of the two-factor model. J. Cogn. Dev. 18, 465–492. doi: 10.1080/15248372.2017.1346658
Mix, K. S., Levine, S. C., Cheng, Y.-L., Young, C., Hambrick, D. Z., Ping, R., et al. (2016). Separate but correlated: The latent structure of space and mathematics across development. J. Exp. Psychol. General 145, 1206–1227. doi: 10.1037/xge0000182
Moore, T. J., Tank, K. M., Glancy, A. W., and Kersten, J. A. (2015). NGSS and the landscape of engineering in K-12 state science standards. J. Res. Sci. Teach. 52, 296–318. doi: 10.1002/tea.21199
Myers, L., and Sirois, M. J. (2006). Spearman Correlation Coefficients, Differences Between. Encyclopedia of Statistical Sciences. Hoboken, NJ: John Wiley & Sons, Inc, doi: 10.1002/0471667196.ess5050.pub2
National Academies of Sciences, Engineering and Medicine (2016). Barriers and Opportunities for 2-year and 4-year STEM Degrees: Systemic Change to Support Students’ Diverse Pathways. Washington, DC: National Academies Press, doi: 10.17226/21739
National Research Council (2006). Learning to Think Spatially. Washington, DC: The National Academies Press.
National Research Council (2013). Next Generation Science Standards: For States, by States. Washington, DC: The National Academies Press.
NCLB (2002). No Child Left Behind Act of 2001, Pub. L. No. 107-110, Stat. 1425. Available online at: https://www.congress.gov/bill/107th-congress/house-bill/1
Newcombe, N. S., and Shipley, T. F. (2015). Thinking about spatial thinking: New typology, new assessments. Studying visual spatial reason. Design Creativity 15, 179–192. doi: 10.1007/978-94-017-9297-4_10
Otumfuor, B. A., and Carr, M. (2017). Teacher spatial skills are linked to differences in geometry instruction. Br. J. Educ. Psychol. 87, 683–699. doi: 10.1111/bjep.12172
Perera, H. N., and John, J. E. (2020). Teachers’ self-efficacy beliefs for teaching math: Relations with teacher and student outcomes. Contemp. Educ. Psychol. 61:101842. doi: 10.1016/j.cedpsych.2020.101842
Peterson, E. G., Weinberger, A. B., Uttal, D. H., Kolvoord, B., and Green, A. E. (2020). Spatial activity participation in childhood and adolescence: Consistency and relations to spatial thinking in adolescence. Cogn. Res. Principles Implications 5:43. doi: 10.1186/s41235-020-00239-0
Pruitt, S. L. (2014). The next generation science standards: The features and challenges. J. Sci. Teach. Educ. 55, 145–156. doi: 10.1007/s10972-014-9385-0
R Core Team (2023). R: A Language and Environment for Statistical Computing (Version 4.4.0) [Computer software]. Vienna: R Foundation for Statistical Computing.
Rocha, K., Lussier, C. M., and Atit, K. (2022). What makes online teaching spatial? Examining the connections between K-12 teachers’ spatial skills, affect, and their use of spatial pedagogy during remote instruction. Cogn. Res. Principles Implications 7:25. doi: 10.1186/s41235-022-00377-7
Schaeffer, M. W., Rozek, C. S., Maloney, E. A., Berkowitz, T., Levine, S. C., and Beilock, S. L. (2021). Elementary school teachers’ math anxiety and students’ math learning: A large-scale replication. Dev. Sci. 24:e13080. doi: 10.1111/desc.13080
Shulman, L. S. (1986). Those who understand: Knowledge growth in teaching. Educ. Res. 15, 4–14. doi: 10.3102/0013189X015002004
Sofroniou, A., and Poutos, K. (2016). Investigating the effectiveness of group work in mathematics. Educ. Sci. 6:30. doi: 10.3390/educsci6030030
Spencer, S. J., Steele, C. M., and Quinn, D. M. (1999). Stereotype threat and women’s math performance. J. Exp. Soc. Psychol. 35, 4–28. doi: 10.1006/jesp.1998.1373
Spielberger, C. D., Gorsuch, R., and Lushene, R. (1970). The State-Trait Anxiety Inventory (STAI): Test Manual Form X. Palo Alto, CA: Consulting Psychologists Press.
Stankov, L., Morony, S., and Lee, Y. P. (2017). Confidence: The Best Non-Cognitive Predictor of Academic Achievement? Noncognitive Psychological Processes and Academic Achievement. Milton Park: Routledge, 19–38.
Steele, C. M., and Aronson, J. (1995). Stereotype threat and the intellectual test performance of African Americans. J. Pers. Soc. Psychol. 69, 797–811. doi: 10.1037//0022-3514.69.5.797
TEACH. (2021). 2021 Teacher Certification (Complete Guide). Available online at: https://www.teach.org/becoming-teacher/teaching-certification#state
The International Cognitive Ability Resource Team (2014). Available online at: https://icar-project.com/
Tobin, K. (1994). “Research on instructional strategies for teaching science,” in Handbook of Research on Science Teaching and Learning, ed. D. Gabel (New York, NY: Macmillan).
Trygstad, P. J., Smith, P., Banilower, E., Nelson, M. M., and Banilower, E. (2013). The Status of Elementary Science Education: Are We Ready for the Next Generation Science Standards?. Chapel Hill, NC: Horizon Research, Inc.
Uttal, D. H., Meadow, N. G., Tipton, E., Hand, L. L., Alden, A. R., Warren, C., et al. (2013). The malleability of spatial skills: A meta-analysis of training studies. Psychol. Bull. 139, 352–402. doi: 10.1037/a0028446
Wolniak, G. C., Seifert, T. A., Reed, E. J., and Pascarella, E. T. (2008). College majors and social mobility. Res. Soc. Stratification Mobility 26, 123–139. doi: 10.1016/j.rssm.2008.02.002
Yang, Y., Long, Y., Sun, D., Aalst, J., and Cheng, S. (2020). Fostering students’ creativity via educational robotics: An investigation of teachers’ pedagogical practices based on teacher interviews. Br. J. Educ. Technol. 51, 1826–1842. doi: 10.1111/bjet.12985
Keywords: teacher cognition, spatial skills, spatial anxiety, spatial habits of mind, primary education
Citation: Rocha K, Lussier CM and Atit K (2025) Bolstering spatial learning in the primary classroom: identifying the factors underlying primary teachers’ spatial pedagogical practices. Front. Educ. 10:1582737. doi: 10.3389/feduc.2025.1582737
Received: 28 February 2025; Accepted: 14 April 2025;
Published: 30 April 2025.
Edited by:
Lawrence Grabau, University of Kentucky, United StatesReviewed by:
Bettina Pedemonte, University of California, San Francisco, United StatesElyssa A. Geer, University of Oregon, United States
Copyright © 2025 Rocha, Lussier and Atit. This is an open-access article distributed under the terms of the Creative Commons Attribution License (CC BY). The use, distribution or reproduction in other forums is permitted, provided the original author(s) and the copyright owner(s) are credited and that the original publication in this journal is cited, in accordance with accepted academic practice. No use, distribution or reproduction is permitted which does not comply with these terms.
*Correspondence: Kinnari Atit, a2lubmFyaUB1Y3IuZWR1