- Department of Diabetes, Endocrinology and Clinical Immunology, School of Medicine, Hyogo Medical University, Nishinomiya, Hyogo, Japan
Background: Although excessive daytime napping has been shown to be involved in diabetes occurrence, its impact on insulin secretion and sensitivity has not been elucidated. It is speculated that excessive napping disrupts the sleep-wake rhythm and increases sympathetic nerve activity during the day, resulting in decreased insulin sensitivity, which may be a mechanism leading to development of diabetes. We previously conducted a cross-sectional study that showed an association of autonomic dysfunction with decreased insulin sensitivity, though involvement of autonomic function in the association between napping and insulin sensitivity remained unclear. Furthermore, the effects of napping used to supplement to short nighttime sleep on insulin secretion and sensitivity are also unknown. In the present cross-sectional study, we examined the relationships of daytime nap duration and autonomic function with insulin secretion and sensitivity in 436 subjects enrolled in the Hyogo Sleep Cardio-Autonomic Atherosclerosis (HSCAA) Cohort Study who underwent a 75-g oral glucose tolerance test (75-g OGTT), after excluding those already diagnosed with diabetes.
Methods: Daytime nap duration was objectively measured using actigraphy, with the subjects divided into the short (≤1 hour) and long (>1 hour) nap groups. Insulin secretion and sensitivity were determined using 75-g OGTT findings. Standard deviation of normal to normal R-R interval (SDNN), a measure of autonomic function, was also determined based on heart rate variability. Subgroup analysis was performed for the associations of napping with insulin secretion and sensitivity, with the results stratified by nighttime sleep duration of less or greater than six hours.
Results: Subjects in the long nap group exhibited lower insulin sensitivity parameters (QUICKI: β=-0.135, p<0.01; Matsuda index: β=-0.119, p<0.05) independent of other clinical factors. In contrast, no associations with insulin secretion were found in either group. Furthermore, the association of long nap duration with insulin sensitivity was not confounded by SDNN. Specific subgroup analyses revealed more prominent associations of long nap habit with lower insulin sensitivity in subjects with a short nighttime sleep time (β=-0.137, p<0.05).
Conclusion: Long daytime nap duration may be a potential risk factor for decreased insulin sensitivity.
Introduction
Diabetes mellitus is a multifactorial disease caused by multiple genetic factors leading to decreased insulin secretion and sensitivity, in addition to lifestyle factors such as overeating and lack of exercise (1, 2). In recent years, attention has focused on the central nervous system, including the hypothalamus, brainstem, and dopamine reward system, which are involved in sleep and the autonomic nervous system, and have effects on insulin secretion and cardiovascular function shown in pancreatic β cells (3–7). Clinically, heart rate variability coefficient, an indicator of autonomic function, is a predictive factor for development of diabetes (8), while sleep duration and sleep quality, assessed by questionnaire, were also found to be associated with its development (9–11). Although nighttime sleep duration is associated with obesity, there are reports that fragmented sleep due to excessive daytime napping is more associated with obesity (12), drawing attention to the health effects of napping as well as nighttime sleep.
Previous studies have shown that an appropriate daytime napping habit improves work performance and mental health (13–15). On the other hand, excessive napping increased health risks such as the mental effects and cardiovascular disease have also been reported (16–20). As for the relationship with glucose metabolism, excessive daytime napping has been implicated in development of diabetes in a number of reported cases. A meta-analysis of 10 studies (four cross-sectional and six longitudinal cohort studies) found that napping for longer than one hour per day was associated with both the prevalence and incidence of diabetes, with a 31% increased risk of developing diabetes during the follow-up period. In contrast, no such association was found for those who took short naps of less than one hour (21). A cohort study of 2,620 subjects aged 60 years and older also found that those who took longer daytime naps (more than one hour per day) had a higher risk of developing diabetes compared with those who did not nap (22). It is speculated that excessive napping disrupts the sleep-wake rhythm (23) and increases sympathetic nerve activity during the day, resulting in decreased insulin sensitivity, which may be a mechanism leading to development of diabetes (10, 24). However, though some studies have noted that naps of 30 minutes or more significantly increase insulin resistance as compared to individuals who do not nap (25), others have found no clinically significant differences (26), thus the effects of daytime naps on insulin secretion and sensitivity remain unclear.
Autonomic dysfunction has been shown to occur even in the pre-diabetic stage (27–29), suggesting its possible contribution to development of diabetes. Furthermore, we previously reported findings of a cross-sectional study indicating that autonomic dysfunction is associated with decreased insulin sensitivity (30). For determining cardiac modulation, heart rate variability (HRV) is commonly used as a non-invasive procedure, as it is known to be a versatile marker of autonomic function (31, 32) and has been shown to be lower in patients with diabetes (8). Nevertheless, the mutual impact of nap duration and autonomic nervous function on insulin secretion and sensitivity in the pre-diabetic phase remains unresolved.
The Hyogo Sleep Cardio-Autonomic Atherosclerosis (HSCAA) cohort study was initiated in October 2010 with the aim to analyze quantitative changes in sleep duration, sleep quality, and apnea, including daytime napping, and their impact on autonomic function on metabolism and atherosclerosis. Based on quantitative assessments of nap time and autonomic function in 436 subjects who agreed to undergo a 75-g oral glucose tolerance test (75-g OGTT), and examinations of their interrelationship with insulin secretion and sensitivity, the present study aimed to explore the possibility that nap time and autonomic function are related to diabetes onset, whether napping as a supplement to short nighttime sleep is associated with insulin secretion and sensitivity, thus providing important information to help with early intervention for its prevention.
Materials and methods
Study population
All subjects gave written informed consent to participate in the study according to a protocol approved by the Ethics Committee of Hyogo College of Medicine (approval no. 2351). The HSCAA study enrolled patients under treatment at the Department of Diabetes, Endocrinology and Metabolism, Hyogo Medical University Hospital (Hyogo, Japan) who had at least one cardiovascular risk factor, such as diabetes, hypertension, dyslipidemia, chronic kidney disease, history of cardiovascular events, obesity, or smoking habit, as well as sleep or autonomic nervous system function, or glucose metabolism disturbance (33, 34). The present cohort study aimed to investigate the relationship of sleep with autonomic function and glucose metabolism, metabolic syndrome, and atherosclerosis. This cross-sectional study was conducted as part of the HSCAA study and did not include patients taking sleeping pills. There were 1155 patients between the ages of 20 and 90 years enrolled in the HSCAA Study from October 2010 to December 2021. Those diagnosed with and treated for diabetes mellitus or HbA1c >6.5%, malignant neoplasms, overt endocrine disease, or renal failure were excluded, while there were also 154 missing baseline data, including monitor malfunctions. Thus, 436 patients able to undergo actigraph, active tracer, and 75-g OGTT examinations were included in this study (Figure 1).
Assessment of classic cardiovascular risk factors
The medical history of each subject was obtained by interview to determine self-reported smoking and drinking habits, and history of cardiovascular events. Drinker was defined as consumption of alcoholic drinks at least three times a week and an individual with a current smoking habit as smoker. Body height and weight were measured, and body mass index (BMI) was calculated as weight (kilograms) divided by the square of height (meters) (kg/m2). Hypertension was defined as medical treatment for hypertension or systolic blood pressure ≥140 mmHg or diastolic blood pressure ≥90 mmHg. Dyslipidemia was defined as currently receiving treatment for dyslipidemia or elevated low-density lipoprotein cholesterol (≥140 mg/dl), decreased high-density lipoprotein cholesterol (<40 mg/dl), or elevated triglyceride (≥150 mg/dl) level (26). Blood samples were collected in the early morning following an overnight fast and quickly centrifuged to obtain plasma. Whole blood was used for hemoglobin A1c, EDTA plasma for glucose and lipids, and serum for other biochemical measurements.
Biochemical parameters, 75-g OGTT, and calculation
For blood sample examinations, whole blood was used to measure hemoglobin A1c, and in EDTA-plasma was used for glucose (Glu) and insulin (IRI). Glucose was measured with a glucose oxidase method and insulin a radio immunometric assay (RIA-BEAD II; Dinabot, Tokyo, Japan). The patients fasted for at least 12 hours after dinner the previous day, then plasma samples for glucose (mmol/L) and insulin (pmol/L) measurements were collected at 0, 30, 60, 90, and 120 minutes after glucose loading, and 75-g OGTT was performed. The results were used to classify abnormal blood glucose levels [normal glucose tolerance (NGT), impaired glucose tolerance (IGT), diabetic type], and assess insulin secretion and sensitivity. Patients with fasting a plasma glucose level ≤109 mg/dl or two-hour plasma glucose ≤139 mg/dl were classified as NGT, fasting plasma glucose ≥110 mg/dl or two-hour plasma glucose from 140 to 199 mg/dl as IGT, and two-hour plasma glucose ≥200 mg/dl as DMtype. For estimation of insulin secretion and sensitivity, the following indices were calculated. Secretion: insulinogenic index = (IRI30 - IRI0)/(Glu30 - FPG) (35), corrected insulin response (CIR) = [(100 × IRI30)/(Glu30) × (Glu30 - 3.89)], disposition index = insulinogenic index × quantitative insulin sensitivity check index (QUICKI). Sensitivity: QUICKI = 1/(log IRI0 + log FPG) (36), BIGTT - sensitivity index (SI) = exp[4.9 - (0.00402 × IRI0) - (0.000556 × IRI30) - (0.00127 × IRI120) - (0.152 × FPG) - (0.00871 × Glu30) - (0.0373 × Glu120) - (0.145 × gender) - (0.0376 × BMI)] (37), Matsuda index = 10,000/[sqrt (FPG × IRI0 × mean PG × mean IRI)] (38).
Determination of sleep conditions
Based on recent advances in technology, actigraphy is commonly used for diagnosis of sleep problems and findings demonstrating its effectiveness have been presented (30). Because a polysomnography device is complicated to fit and examinations with it are difficult to perform with a large number of subjects, the present study used findings for quantitative analyses of sleep duration and sleep quality obtained with an actigraph device (Ambulatory Monitoring, Inc., Ardley, NY, USA) (24, 32). The actigraph was attached to the wrist of the non-dominant arm and used twice for 24 consecutive hours. An actigraph converts signals generated by an accelerometer and collects them with frequency noted in hertz, then sums the values according to a user-specified time sampling interval termed an epoch and records them as activity counts. The approximate cutoff values for activity count are 0 to 1.5 for sedentary, 1.5 to 3 for light physical activity, 3 to 6 for moderate physical activity, and 6 or more for vigorous physical activity (39). Nap time was calculated as the sum of sleep time during waking hours. Wake-up time was set as the time when continuous activity was shown to be initiated on the actigraph. As used in several previous studies, we set a threshold of 1 hour for nap time, which has been shown to be associated with the risk of developing diabetes (21, 22, 40, 41), napping time was used to divide the patients into two groups; short (≤1 hour) and long (>1 hour). The activity mean is the average of the activity counts detected by the accelerometer during one minute, and the activity index is the percentage of the number of epochs where the activity count is greater than zero over the length of the measurement time period. Nocturnal activity index is a measure of sleep quality and calculated as movement of the whole body during sleep hours, with higher values indicating lower sleep quality (32, 33). Apnea-hypopnea index (AHI) was determined using an apnomonitor (SAS-2100, Teijin, Tokyo, Japan) (34).
Assessment of autonomic nervous function
For assessment of cardiac autonomic function, HRV was measured using an active tracer device (AC-301A®, Arm Electronics, Tokyo, Japan) and the MemCalc Chiram 3 system, version 2.0 (Suwa Trust, Tokyo, Japan), with the standard deviation of the NN (RR) interval (SDNN), noted in previous reports as a noninvasive method (24, 25, 34–36), used as an index. Subjects wore an actigraph twice for 24 consecutive hours.
Statistical analysis
Non-repeated t-tests and chi-square tests (categorical variables) were utilized to compare variables, including insulin secretion and sensitivity, between the short and long nap groups, as appropriate. Multiple regression analysis was performed to determine whether there was an independent relationship between the variables considered. Associations of age (under and over 50 years), gender, BMI (under and over 25 kg/m2), current smoker, alcohol consumption, hypertension, dyslipidemia, and HbA1c (under and over 5.8%) with disposition index, QUICKI, and Matsuda index were analyzed. For multivariate linear regression analyses, the covariates included age, gender, body mass index, current smoker, alcohol consumption, hypertension, dyslipidemia, and HbA1c. Multivariate linear regression analyses of factors associated with disposition index were performed using QUICKI and Matsuda index, with Model 1 including nap duration, Model 2 nocturnal sleep duration, Model 3 SDNN, Model 4 nap duration and nocturnal sleep duration, Model 5 nap duration and SDNN, and Model 6 nap duration, nocturnal sleep duration, and SDNN, in addition to the other covariates previously noted. Naptime and daytime physical activity were not included in the covariates, as simultaneous entry was deemed difficult due to multicollinearity.
The present patients were divided into two groups, those whose sleeping time was less than and more than six hours (22, 24), with subgroup analysis conducted to examine the relationship between Matsuda index, a measure of insulin sensitivity, and nap time. For multivariate linear regression analysis of factors associated with Matsuda index of patients with short and long nocturnal sleep duration, Model 1 included nap duration, Model 2 SDNN, and Model 3 nap duration and SDNN, in addition to the other covariates previously noted. Daytime nap time, nocturnal sleep time, insulin secretion/sensitivity index, and SDNN were natural log transformed (ln) to normalize the skewed distribution. All statistical analyses were performed using JMP® Pro, version 15.2.0 (SAS Institute, Cary, NC, USA). The reported p values are two-tailed and were considered to be statistically significant at <0.05.
Results
Comparisons of baseline characteristics for the subjects categorized by daytime napping duration are shown in Table 1. Those in the long nap group exhibited higher BMI and prevalence for alcohol consumption. In contrast, age, gender, BMI, HbA1c, AHI, and diabetes type shown by 75-g OGTT findings were not different between the groups. There are differences in clinical characteristics between people included in this analysis compared to those excluded (Supplementary Table).
Comparisons of insulin secretion (insulinogenic index, CIR, disposition index) and sensitivity (QUICKI, BIGTT- SI, Matsuda index), calculated using a 75-g OGTT, between the groups are presented in Figure 2. Insulin sensitivity values, such as QUICKI (p<0.01), BIGTT- SI (p<0.05), and Matsuda index (p<0.05), for the long nap group were lower, while parameters for insulin secretion were not different. For autonomic function, SDNN for the long nap group was lower (p<0.01), while LF/HF and HF were not different (Figure 3). Additionally, sleep duration was shorter (p<0.01) and daytime activity lower (p<0.05) for the long nap group, while nocturnal activity index was not different (Figure 4).
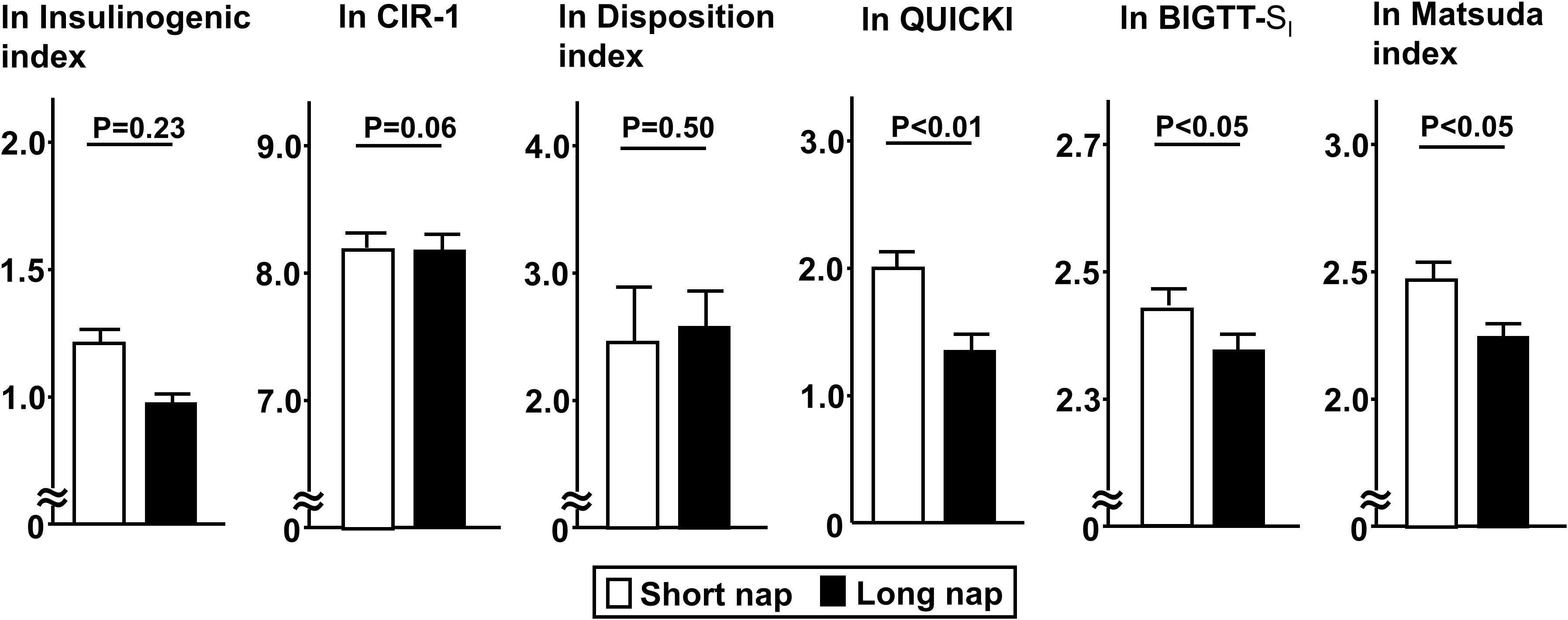
Figure 2 Comparisons of indices for insulin secretion and sensitivity between short and long nap groups. Parameters for insulin secretion [insulinogenic index, corrected insulin response (CIR), disposition index] and insulin sensitivity (QUICKI, BIGTT- SI, Matsuda index) were calculated based on plasma glucose and insulin levels determined at 0, 30, 60, 90, 120 minutes with a 75-g oral glucose tolerance test. These parameters were natural logarithm transformed to achieve a normal distribution. Each column shows the mean ± standard error. P values were determined using Student’s t-test.
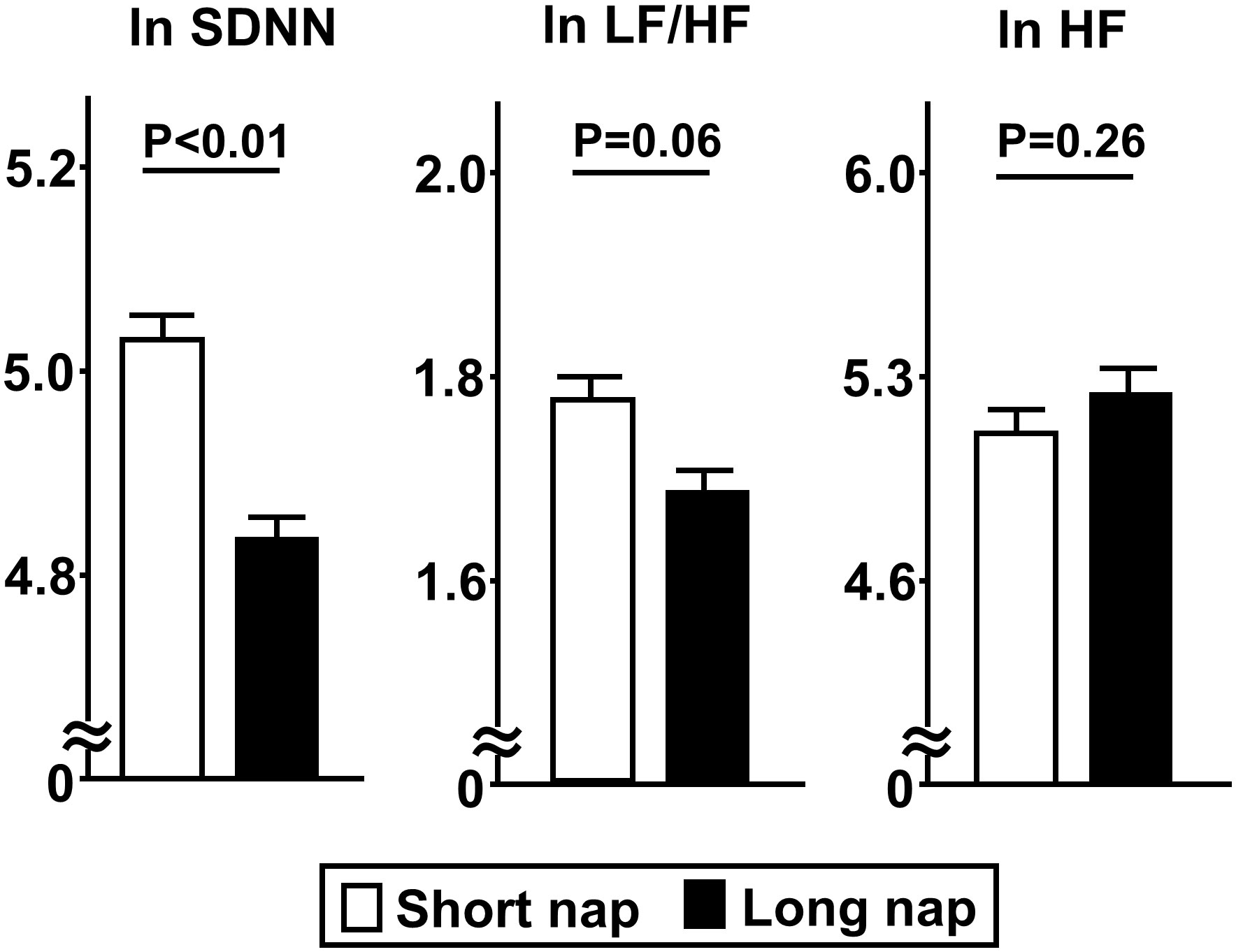
Figure 3 Comparisons of indices for HRV between short and long nap groups. As parameters for HRV, SDNN [standard deviation of NN (RR)), LF/HF (low-frequency domain/ high-frequency domain), and HF (high-frequency domain) were determined. These parameters were natural logarithm transformed to achieve a normal distribution. Each column shows the mean ± standard error. P values were determined using Student’s t-test.
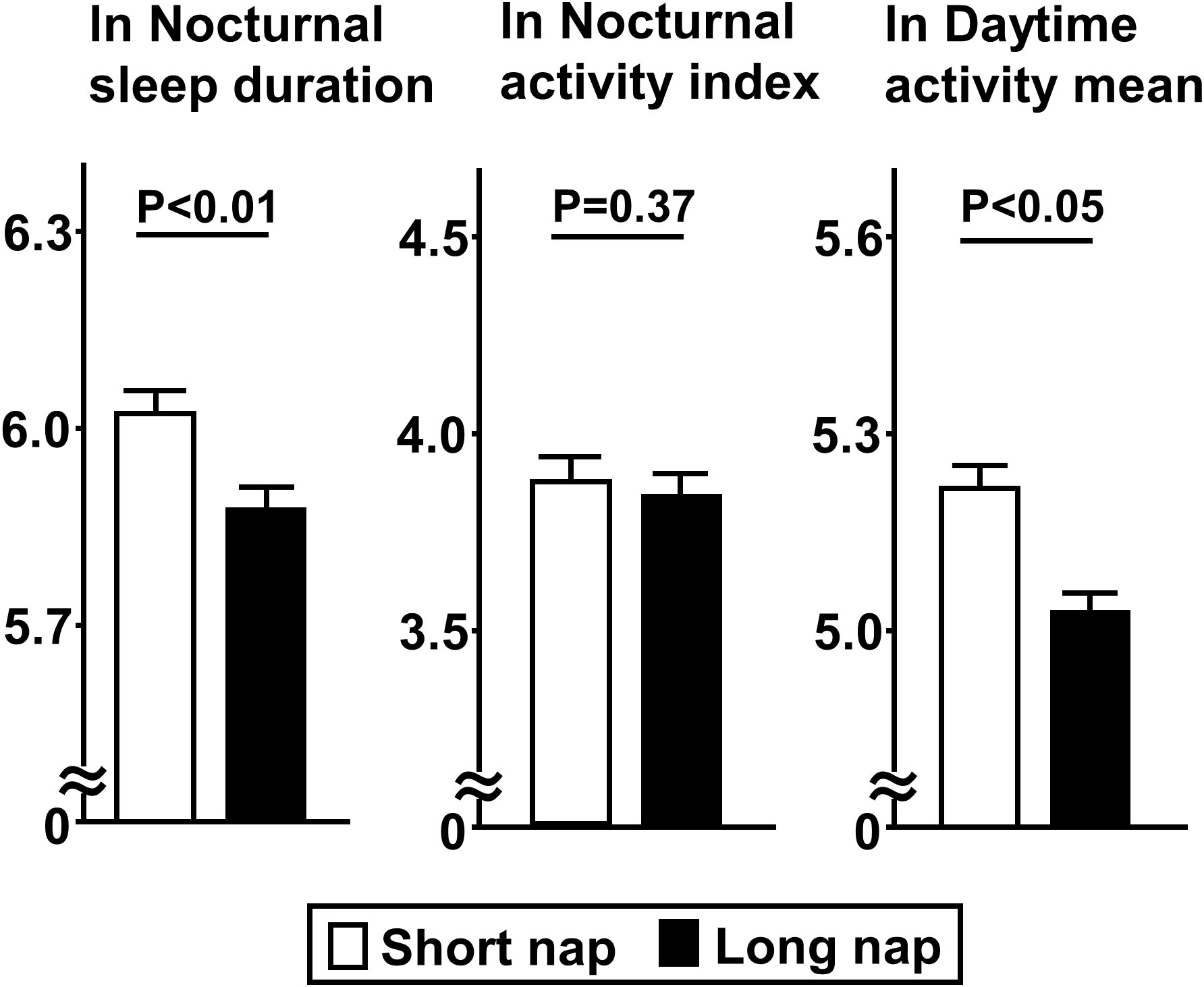
Figure 4 Comparisons of indices for sleep duration and quality between short and long nap groups. Parameters for sleep duration, quality, and daytime activity were determined. These parameters were natural logarithm transformed to achieve a normal distribution. Each column shows the mean ± standard error. P values were determined using Student’s t-test.
Next, the relationships of disposition index for insulin secretion, and QUICKI and Matsuda index for insulin sensitivity with other clinical factors were examined (Table 2). Disposition index values were lower for subjects with advanced age (>50 years), alcohol consumption, hypertension, and high HbA1c, while QUICKI values were lower for subjects with advanced age, high BMI, current smoking habit, dyslipidemia, and high HbA1c. On the other hand, Matsuda index values were higher for subjects with advanced age, high BMI, current smoking habit, and dyslipidemia.
Multiple linear regression analysis was performed to further examine whether the associations of nap time, sleep duration, and SDNN with body mass index, QUICKI, and Matsuda index were independent of potential clinical confounders (Table 3). The adjusted R2 for models 1-6 were all between 0.13 and 0.26, which were considered reasonable as goodness of fit. Those results showed that long nap duration remained inversely associated with QUICKI (β=-0.135, p<0.01) and Matsuda index (β=-0.119, p<0.05) (Model 1), while SDNN showed a positive association with QUICKI (β=0.118, p<0.05) (Model 3). Furthermore, even after addition of sleep duration (Model 4), SDNN (Model 5), as well as both (Model 6) to Model 1, long nap duration remained inversely associated with QUICKI (β=-0.127, p<0.05) and Matsuda index (β=-0.123, p<0.05). On the other hand, nap duration was not associated with insulin secretion or sensitivity (Model 2).
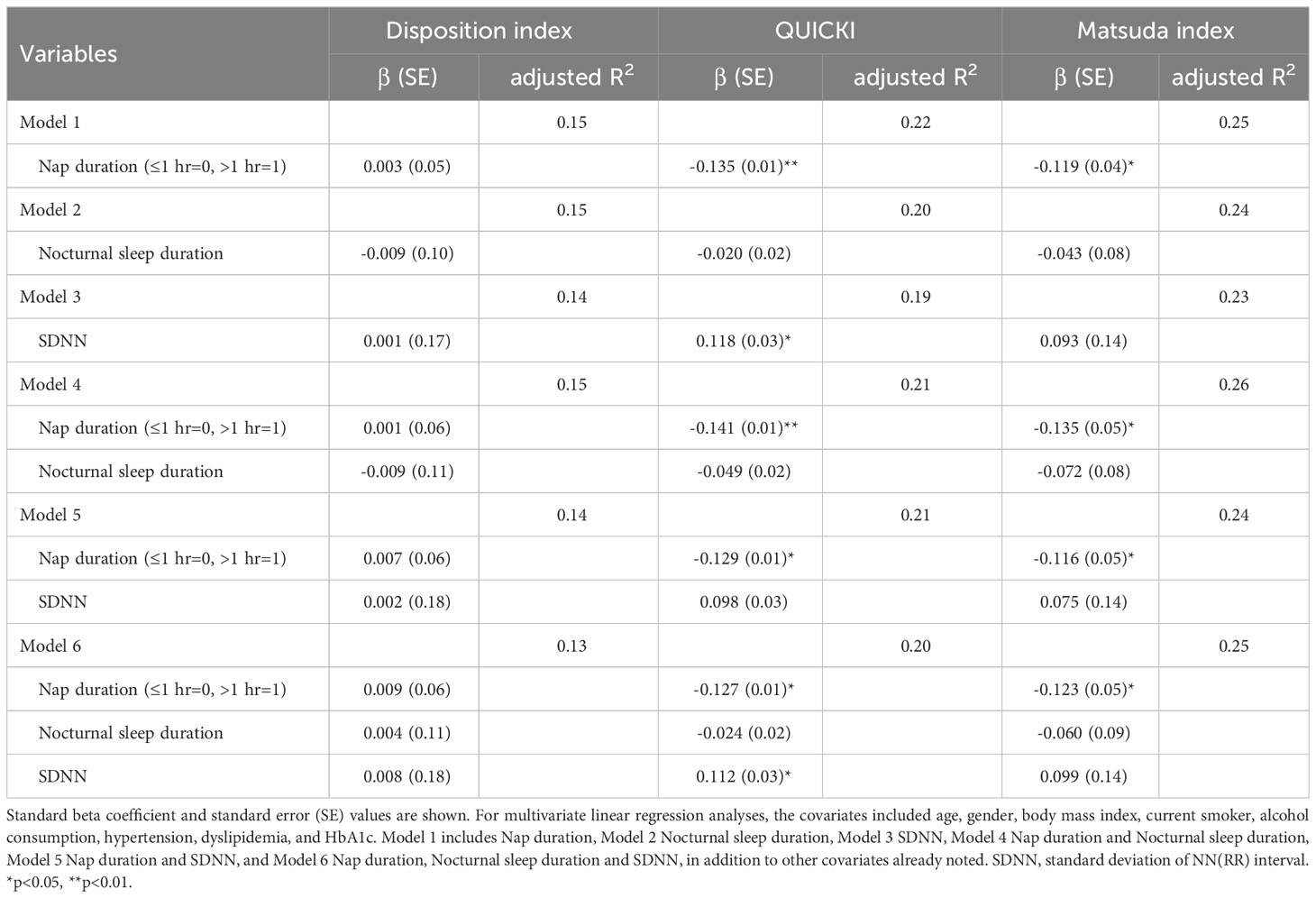
Table 3 Multivariate linear regression analysis of factors associated with disposition index, QUICKI, and Matsuda index.
Finally, specific subgroup analyses showed more prominent associations of long nap duration with lower Matsuda index value in subjects with a short sleep duration (≤6 hours) (β=-0.137, p<0.05, adjusted R2=0.23), while that was not a factor in those with a long sleep duration (>6 hours) (β=-0.156, p=0.06, adjusted R2=0.27) (Table 4).
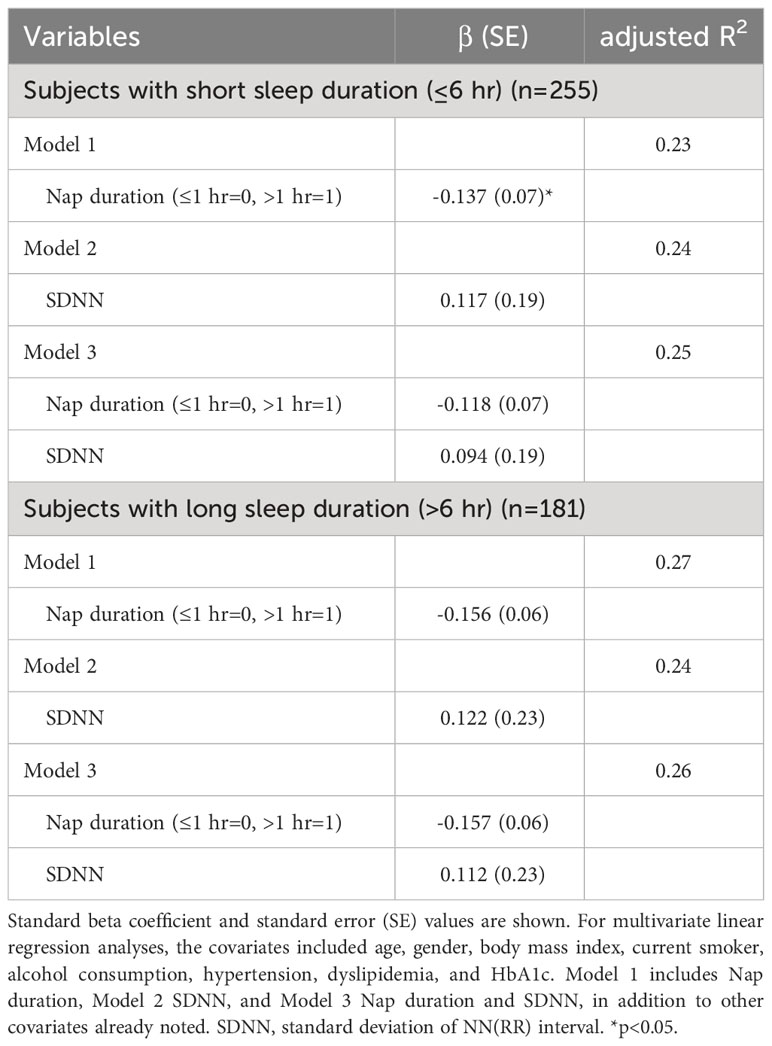
Table 4 Multivariate linear regression analysis of factors associated with Matsuda index in short- and long-time nocturnal sleep duration groups.
Discussion
Results obtained in the present study showed that a nap time of greater than one hour was independently associated with decreased insulin sensitivity related to autonomic dysfunction. Moreover, specific subgroup analyses revealed a more prominent association of long nap time with lower insulin sensitivity in individuals with a nighttime sleep duration of six hours or less.
A previous report noted that shorter (six hours) and longer (eight hours) sleep durations were associated with incident diabetes mellitus (22). Additionally, recent studies have shown that excessive napping for more than one hour increases the risk for development of diabetes, in contrast to a shorter nap duration (21, 22, 40, 41). Previously reported ORs for diabetes onset were 1.2395% (CI 1.18 .1.29) for individuals who nap for one hour or less and 1.55 (95% CI 1.45.1.66) for those who nap for more than one hour, as compared to those who do not nap (40). This is considered to be due to decreased sleep duration and quality caused by a longer nap duration (42–44).
Another report speculated that long naps during the day may affect the sleep-wake cycle and noted that disruption of the circadian rhythm is an environmental risk factor predisposing to type 2 diabetes, as fragmented sleep induces sympathetic stimulation during the day, a mechanism possibly related to insulin sensitivity (24). Thus, misalignment of the circadian rhythm results in reduced insulin sensitivity due to sympathetic and other influences. Our previous study found that autonomic function is associated with decreased insulin sensitivity (30). Initially, we speculated that a prolonged nap duration would thus be shown to be associated with decreased insulin sensitivity via decreased autonomic function, and the present results showed that autonomic function was lower in the long as compared with the short group. However, multivariate analysis revealed that nap duration was independently associated with decreased insulin sensitivity. This may be because several factors other than autonomic function are involved in the association of long nap duration with development of diabetes.
Excessive napping may also decrease daytime activity and calorie consumption (45), leading to obesity (46), increased insulin resistance, and increased risk of developing diabetes. The present long nap group had lower daytime activity and higher BMI. Although nap time was associated with drinkers in this study, the results of multiple regression analysis with alcohol consumption as a covariate, which showed that excessive napping was independently associated with insulin sensitivity, did not mean that napping was associated with reduced insulin sensitivity via alcohol consumption. Although napping as a complement to short nighttime sleep may be refreshing, naps should total no more than one hour each day. Many previous reports have used self-reported questionnaires (Pittsburgh Sleep Quality Index) to assess sleep and nap duration (40, 47–49), while objectively obtained quantitative data based on actigraph results were used in the present investigation. Thus, the results may be different as compared to self-reported and polysomnographic assessments. It is conceivable that use of self-reported questionnaires indicate shorter nap durations, thus leading to underestimation. In a study in which polysomnography and actigraph were simultaneously used to assess nap duration, that determined by actigraph findings was correlated with polysomnography results (50). Thus, use of an actigraph to determine actual nap duration is considered to be reasonable.
Napping may mitigate adverse health effects in individuals who sleep for a short time at night. In the present study, excessive napping was associated with decreased insulin sensitivity only in subjects with less than six nighttime sleep hours, suggesting that a nap of one hour or more may contribute to increased risk of diabetes development, even when naps are used to compensate for short nighttime sleep. Another study found that daytime napping is a symptom of obstructive sleep apnea (51), which induces oxygen deprivation, and also causes elevated catecholamines and cortisol, resulting in increased insulin resistance and contributing to impaired glucose tolerance (40, 52). However, the present study found no difference in AHI between the long and short groups. This may be due to the fact that few of the subjects were affected by moderate or severe sleep apnea.
This study has several limitations. First, the results may not be generalizable for all patients with varying glycemic status. Second, due to the cross-sectional design, a causal relationship could not be determined. To avoid loss of statistical power, subjects who were first diagnosed as diabetic based on glucose tolerance testing were not excluded. Third, data for subgroups based on different levels of glucose intolerance (NGT, IGT, DM types) were not analyzed so as to avoid statistical power loss. Fourth, sleep assessment with actigraphy was performed during hospitalization, thus actual sleep behavior during daily life could not be assessed. The results during hospitalization may not be exactly the same as napping habits in daily life, but we believe that daily habits may be reflected in how patients spend their time during hospitalization. Fifth, polysomnography, considered as the gold standard for evaluating sleep, was not used in this study and the possibility that the results may differ from those obtained with that method cannot be ruled out. Finally, the present results did not reveal any potential mechanisms involved in the association of excessive napping with autonomic function or insulin sensitivity. Leptin is known to be involved in both sleep and autonomic function, and considered as a potential candidate factor for napping, autonomic function, and insulin sensitivity. In clinical studies, plasma leptin levels have been shown to be associated with insulin sensitivity in both healthy and obese women, as well as patients with type 2 diabetes. Nevertheless, the present study is the first to report an association of long nap duration with increased insulin sensitivity. We believe that these results provide important information to better understand the pathophysiological significance of napping as a factor related to abnormal blood glucose levels.
In conclusion, in patients without apparent diabetes, napping for a long period of time was found to be independently associated with decreased insulin sensitivity from autonomic dysfunction. Additionally, a prominent association of long nap duration with lower insulin sensitivity was observed in patients with a short nighttime sleep period.
Data availability statement
The raw data supporting the conclusions of this article will be made available by the authors, without undue reservation.
Ethics statement
The studies involving humans were approved by Department of Diabetes, Endocrinology and Clinical Immunology, Hyogo Medical University. The studies were conducted in accordance with the local legislation and institutional requirements. The participants provided their written informed consent to participate in this study.
Author contributions
MK-H, MK and HK: conceptualization, methodology, software use. MK-H, MK, AMo, AMi, KK-H, AK: data curation. MK-H and MK: writing - original draft preparation. KK, YK, and TS: supervision. MK: validation. MK and HK: writing - reviewing and editing. All authors contributed to the article and approved the submitted version.
Funding
This work was supported by a Hyogo Medical University Diversity Grant for Research Promotion under the MEXT Funds for the Development of Human Resources in Science and Technology, and the Initiative for Realizing Diversity in the Research Environment (Characteristic-Compatible Type).
Acknowledgments
The authors are grateful for the excellent technical assistance of Tomoe Ushitani and Ai Matsumoto. We also wish to thank the other investigators and staff, as well as the participants of the Hyogo Sleep Cardio-Autonomic Atherosclerosis study for their valuable contributions.
Conflict of interest
The authors declare that the research was conducted in the absence of any commercial or financial relationships that could be construed as a potential conflict of interest.
Publisher’s note
All claims expressed in this article are solely those of the authors and do not necessarily represent those of their affiliated organizations, or those of the publisher, the editors and the reviewers. Any product that may be evaluated in this article, or claim that may be made by its manufacturer, is not guaranteed or endorsed by the publisher.
Supplementary material
The Supplementary Material for this article can be found online at: https://www.frontiersin.org/articles/10.3389/fendo.2023.1211705/full#supplementary-material
References
1. Bellou V, Belbasis L, Tzoulaki I, Evangelou E. Risk factors for type 2 diabetes mellitus: An exposure-wide umbrella review of meta-analyses. PloS One (2018) 13:e0194127. doi: 10.1371/journal.pone.0194127
2. DeForest N, Majithia AR. Genetics of type 2 diabetes: implications from large-scale studies. Curr Diabetes Rep (2022) 22:227–35. doi: 10.1007/s11892-022-01462-3
3. Morton GJ, Cummings DE, Baskin DG, Barsh GS, Schwartz MW. Central nervous system control of food intake and body weight. Nature (2006) 443:289–95. doi: 10.1038/nature05026
4. Jais A, Bruning JC. Hypothalamic inflammation in obesity and metabolic disease. J Clin Invest (2017) 127:24–32. doi: 10.1172/JCI88878
5. Sohn JW, Harris LE, Berglund ED, Liu T, Vong L, Lowell BB, et al. Melanocortin 4 receptors reciprocally regulate sympathetic and parasympathetic preganglionic neurons. Cell (2013) 152:612–9. doi: 10.1016/j.cell.2012.12.022
6. Kozuka C, Kaname T, Shimizu-Okabe C, Takayama C, Tsutsui M, Matsushita M, et al. Impact of brown rice-specific gamma-oryzanol on epigenetic modulation of dopamine D2 receptors in brain striatum in high-fat-diet-induced obesity in mice. Diabetologia (2017) 60:1502–11. doi: 10.1007/s00125-017-4305-4
7. Simonds SE, Pryor JT, Ravussin E, Greenway FL, Dileone R, Allen AM, et al. Leptin mediates the increase in blood pressure associated with obesity. Cell (2014) 159:1404–16. doi: 10.1016/j.cell.2014.10.058
8. Carnethon MR, Golden SH, Folsom AR, Haskell W, Liao D. Prospective investigation of autonomic nervous system function and the development of type 2 diabetes: the Atherosclerosis Risk In Communities study, 1987-1998. Circulation (2003) 107:2190–5. doi: 10.1161/01.CIR.0000066324.74807.95
9. Knutson KL, Ryden AM, Mander BA, Van Cauter E. Role of sleep duration and quality in the risk and severity of type 2 diabetes mellitus. Arch Intern Med (2006) 166:1768–74. doi: 10.1001/archinte.166.16.1768
10. Cappuccio FP, D'Elia L, Strazzullo P, Miller MA. Quantity and quality of sleep and incidence of type 2 diabetes: a systematic review and meta-analysis. Diabetes Care (2010) 33:414–20. doi: 10.2337/dc09-1124
11. Shan Z, Ma H, Xie M, Yan P, Guo Y, Bao W, et al. Sleep duration and risk of type 2 diabetes: a meta-analysis of prospective studies. Diabetes Care (2015) 38:529–37. doi: 10.2337/dc14-2073
12. van den Berg JF, Knvistingh Neven A, Tulen JH, Hofman A, Witteman JC, Miedema HM, et al. Actigraphic sleep duration and fragmentation are related to obesity in the elderly: the Rotterdam Study. Int J Obes (Lond) (2008) 32:1083–90. doi: 10.1038/ijo.2008.57
13. Lau EY, Wong ML, Lau KN, Hui FW, Tseng CH. Rapid-eye-movement-sleep (REM) associated enhancement of working memory performance after a daytime nap. PloS One (2015) 10:e0125752. doi: 10.1371/journal.pone.0125752
14. Milner CE, Cote KA. Benefits of napping in healthy adults: impact of nap length, time of day, age, and experience with napping. J Sleep Res (2009) 18:272–81. doi: 10.1111/j.1365-2869.2008.00718.x
15. Dhand R, Sohal H. Good sleep, bad sleep! The role of daytime naps in healthy adults. Curr Opin Pulm Med (2006) 12:379–82. doi: 10.1097/01.mcp.0000245703.92311.d0
16. Yuan S, Levin MG, Titova OE, Chen J, Sun Y, Million Veteran Program VA, et al. daytime napping, and risk of peripheral artery disease: multinational cohort and Mendelian randomization studies. Eur Heart J Open (2023) 3:oead008. doi: 10.1093/ehjopen/oead008
17. Li L, Zhang Q, Zhu L, Zeng G, Huang H, Zhuge J, et al. Daytime naps and depression risk: A meta-analysis of observational studies. Front Psychol (2022) 13:1051128. doi: 10.3389/fpsyg.2022.1051128
18. Wang Z, Yang W, Li X, Qi X, Pan KY, Xu W. Association of sleep duration, napping, and sleep patterns with risk of cardiovascular diseases: A nationwide twin study. J Am Heart Assoc (2022) 11:e025969. doi: 10.1161/JAHA.122.025969
19. Mo T, Wang Y, Gao H, Li W, Zhou L, Yuan Y, et al. Sleep duration, midday napping, and serum homocysteine levels: A gene-environment interaction study. Nutrients (2023) 15. doi: 10.3390/nu15010210
20. Yamada T, Hara K, Shojima N, Yamauchi T, Kadowaki T. Daytime napping and the risk of cardiovascular disease and all-cause mortality: A prospective study and dose-response meta-analysis. Sleep (2015) 38:1945–53. doi: 10.5665/sleep.5246
21. Guo VY, Cao B, Wong CKH, Yu EYT. The association between daytime napping and risk of diabetes: a systematic review and meta-analysis of observational studies. Sleep Med (2017) 37:105–12. doi: 10.1016/j.sleep.2017.01.018
22. Lin L, Lu C, Chen W, Guo VY. Daytime napping and nighttime sleep duration with incident diabetes mellitus: A cohort study in Chinese older adults. Int J Environ Res Public Health (2021) 18. doi: 10.3390/ijerph18095012
23. Feinberg I, Maloney T, March JD. Precise conservation of NREM period 1 (NREMP1) delta across naps and nocturnal sleep: implications for REM latency and NREM/REM alternation. Sleep (1992) 15:400–3. doi: 10.1093/sleep/15.5.400
24. Tasali E, Leproult R, Spiegel K. Reduced sleep duration or quality: relationships with insulin resistance and type 2 diabetes. Prog Cardiovasc Dis (2009) 51:381–91. doi: 10.1016/j.pcad.2008.10.002
25. Li X, Pang X, Zhang Q, Qu Q, Hou Z, Liu Z, et al. Long-term single and joint effects of excessive daytime napping on the HOMA-IR index and glycosylated hemoglobin: A prospective cohort study. Med (Baltimore) (2016) 95:e2734. doi: 10.1097/MD.0000000000002734
26. Baoying H, Hongjie C, Changsheng Q, Peijian W, Qingfei L, Yinghua L, et al. Association of napping and night-time sleep with impaired glucose regulation, insulin resistance and glycated hemoglobin in Chinese middle-aged adults with no diabetes: a cross-sectional study. BMJ Open (2014) 4:e004419. doi: 10.1136/bmjopen-2013-004419
27. Pfeifer MA, Weinberg CR, Cook DL, Reenan A, Halter JB, Ensinck JW, et al. Autonomic neural dysfunction in recently diagnosed diabetic subjects. Diabetes Care (1984) 7:447–53. doi: 10.2337/diacare.7.5.447
28. Poliakova N, Despres JP, Bergeron J, Almeras N, Tremblay A, Poirier P. Influence of obesity indices, metabolic parameters and age on cardiac autonomic function in abdominally obese men. Metabolism (2012) 61:1270–9. doi: 10.1016/j.metabol.2012.02.006
29. Kurajoh M, Koyama H, Kadoya M, Naka M, Miyoshi A, Kanzaki A, et al. Plasma leptin level is associated with cardiac autonomic dysfunction in patients with type 2 diabetes: HSCAA study. Cardiovasc Diabetol (2015) 14:117. doi: 10.1186/s12933-015-0280-6
30. Kakutani-Hatayama M, Kadoya M, Morimoto A, Miyoshi A, Kosaka-Hamamoto K, Kusunoki Y, et al. Associations of sleep quality, sleep apnea and autonomic function with insulin secretion and sensitivity: HSCAA study. Metabol Open (2020) 6:100033. doi: 10.1016/j.metop.2020.100033
31. Malik M, Bigger JT, Camm AJ, Kleiger RE, Malliani A, Moss AJ. Heart rate variability. Standards of measurement, physiological interpretation, and clinical use. Task Force of the European Society of Cardiology and the North American Society of Pacing and Electrophysiology. Eur Heart J (1996) 17:354–81. doi: 10.1093/oxfordjournals.eurheartj.a014868
32. Bellavere F, Balzani I, De Masi G, Carraro M, Carenza P, Cobelli C, et al. Power spectral analysis of heart-rate variations improves assessment of diabetic cardiac autonomic neuropathy. Diabetes (1992) 41:633–40. doi: 10.2337/diab.41.5.633
33. Kadoya M, Koyama H, Kurajoh M, Kanzaki A, Kakutani-Hatayama M, Okazaki H, et al. Sleep, cardiac autonomic function, and carotid atherosclerosis in patients with cardiovascular risks: HSCAA study. Atherosclerosis (2015) 238:409–14. doi: 10.1016/j.atherosclerosis.2014.12.032
34. Kidawara Y, Kadoya M, Morimoto A, Daimon T, Kakutani-Hatayama M, Kosaka-Hamamoto K, et al. Sleep apnea and physical movement during sleep, but not sleep duration, are independently associated with progression of left ventricular diastolic dysfunction: prospective Hyogo sleep cardio-autonomic atherosclerosis cohort study. J Am Heart Assoc (2022) 11:e024948. doi: 10.1161/JAHA.121.024948
35. Seltzer HS, Allen EW, Herron AL Jr., Brennan MT. Insulin secretion in response to glycemic stimulus: relation of delayed initial release to carbohydrate intolerance in mild diabetes mellitus. J Clin Invest (1967) 46:323–35. doi: 10.1172/JCI105534
36. Katz A, Nambi SS, Mather K, Baron AD, Follmann DA, Sullivan G, et al. Quantitative insulin sensitivity check index: a simple, accurate method for assessing insulin sensitivity in humans. J Clin Endocrinol Metab (2000) 85:2402–10. doi: 10.1210/jcem.85.7.6661
37. Hansen T, Drivsholm T, Urhammer SA, Palacios RT, Volund A, Borch-Johnsen K, et al. The BIGTT test: a novel test for simultaneous measurement of pancreatic beta-cell function, insulin sensitivity, and glucose tolerance. Diabetes Care (2007) 30:257–62. doi: 10.2337/dc06-1240
38. Matsuda M, DeFronzo RA. Insulin sensitivity indices obtained from oral glucose tolerance testing: comparison with the euglycemic insulin clamp. Diabetes Care (1999) 22:1462–70. doi: 10.2337/diacare.22.9.1462
39. Freedson PS, Melanson E, Sirard J. Calibration of the computer science and applications, inc. accelerometer. Med Sci Sports Exerc (1998) 30:777–81. doi: 10.1097/00005768-199805000-00021
40. Xu Q, Song Y, Hollenbeck A, Blair A, Schatzkin A, Chen H. Day napping and short night sleeping are associated with higher risk of diabetes in older adults. Diabetes Care (2010) 33:78–83. doi: 10.2337/dc09-1143
41. Yamada T, Shojima N, Yamauchi T, Kadowaki T. J-curve relation between daytime nap duration and type 2 diabetes or metabolic syndrome: A dose-response meta-analysis. Sci Rep (2016) 6:38075. doi: 10.1038/srep38075
42. Dautovich ND, McCrae CS, Rowe M. Subjective and objective napping and sleep in older adults: are evening naps "bad" for nighttime sleep? J Am Geriatr Soc (2008) 56:1681–6. doi: 10.1111/j.1532-5415.2008.01822.x
43. Yoon IY, Kripke DF, Youngstedt SD, Elliott JA. Actigraphy suggests age-related differences in napping and nocturnal sleep. J Sleep Res (2003) 12:87–93. doi: 10.1046/j.1365-2869.2003.00345.x
44. Yoon IY, Kripke DF, Elliott JA, Youngstedt SD, Rex KM, Hauger RL. Age-related changes of circadian rhythms and sleep-wake cycles. J Am Geriatr Soc (2003) 51:1085–91. doi: 10.1046/j.1532-5415.2003.51356.x
45. Leng Y, Cappuccio FP, Surtees PG, Luben R, Brayne C, Khaw KT. Daytime napping, sleep duration and increased 8-year risk of type 2 diabetes in a British population. Nutr Metab Cardiovasc Dis (2016) 26:996–1003. doi: 10.1016/j.numecd.2016.06.006
46. Patel SR, Hayes AL, Blackwell T, Evans DS, Ancoli-Israel S, Wing YK, et al. Study of Osteoporotic Fractures Research, The association between sleep patterns and obesity in older adults. Int J Obes (Lond) (2014) 38:1159–64. M. Osteoporotic Fractures in, and XXXG. doi: 10.1038/ijo.2014.13
47. Renko AK, Hiltunen L, Laakso M, Rajala U, Keinanen-Kiukaanniemi S. The relationship of glucose tolerance to sleep disorders and daytime sleepiness. Diabetes Res Clin Pract (2005) 67:84–91. doi: 10.1016/j.diabres.2004.06.003
48. Lindberg E, Berne C, Franklin KA, Svensson M, Janson C. Snoring and daytime sleepiness as risk factors for hypertension and diabetes in women–a population-based study. Respir Med (2007) 101:1283–90. doi: 10.1016/j.rmed.2006.10.015
49. Hublin C, Lehtovirta M, Partinen M, Koskenvuo M, Kaprio J. Napping and the risk of type 2 diabetes: a population-based prospective study. Sleep Med (2016) 17:144–8. doi: 10.1016/j.sleep.2015.11.004
50. Kanady JC, Drummond SP, Mednick SC. Actigraphic assessment of a polysomnographic-recorded nap: a validation study. J Sleep Res (2011) 20:214–22. doi: 10.1111/j.1365-2869.2010.00858.x
51. Masa JF, Rubio M, Perez P, Mota M, de Cos JS, Montserrat JM. Association between habitual naps and sleep apnea. Sleep (2006) 29:1463–8. doi: 10.1093/sleep/29.11.1463
Keywords: daytime nap, insulin sensitivity, actigraphy, heart rate variability, glucose intolerance
Citation: Kakutani-Hatayama M, Kadoya M, Morimoto A, Miyoshi A, Kosaka-Hamamoto K, Kanzaki A, Konishi K, Kusunoki Y, Syoji T and Koyama H (2023) Excessive daytime napping independently associated with decreased insulin sensitivity in cross-sectional study – Hyogo Sleep Cardio-Autonomic Atherosclerosis cohort study. Front. Endocrinol. 14:1211705. doi: 10.3389/fendo.2023.1211705
Received: 25 April 2023; Accepted: 17 October 2023;
Published: 03 November 2023.
Edited by:
Mohammed Al-Abri, Sultan Qaboos University, OmanReviewed by:
Marquis Samuel Hawkins, University of Pittsburgh, United StatesRakhmat Ari Wibowo, Gadjah Mada University, Indonesia
Copyright © 2023 Kakutani-Hatayama, Kadoya, Morimoto, Miyoshi, Kosaka-Hamamoto, Kanzaki, Konishi, Kusunoki, Syoji and Koyama. This is an open-access article distributed under the terms of the Creative Commons Attribution License (CC BY). The use, distribution or reproduction in other forums is permitted, provided the original author(s) and the copyright owner(s) are credited and that the original publication in this journal is cited, in accordance with accepted academic practice. No use, distribution or reproduction is permitted which does not comply with these terms.
*Correspondence: Manabu Kadoya, bWthZG95YUBoeW8tbWVkLmFjLmpw