- 1Department of Nephrology, Radboud Institute for Molecular Life Sciences, Radboud University Medical Center, Nijmegen, Netherlands
- 2Department of Internal Medicine and Radboud Center for Infectious Diseases, Radboud University Medical Center, Nijmegen, Netherlands
- 3Department of Intensive Care Medicine, Radboud University Medical Center, Nijmegen, Netherlands
- 4Deparment of Immunology and Metabolism, Life & Medical Sciences Institute, University of Bonn, Bonn, Germany
- 5Biomedical Engineering and Imaging Institute, Icahn School of Medicine at Mount Sinai, New York, NY, United States
Reports suggest a role of endothelial dysfunction and loss of endothelial barrier function in COVID-19. It is well established that the endothelial glycocalyx-degrading enzyme heparanase contributes to vascular leakage and inflammation. Low molecular weight heparins (LMWH) serve as an inhibitor of heparanase. We hypothesize that heparanase contributes to the pathogenesis of COVID-19, and that heparanase may be inhibited by LMWH. To test this hypothesis, heparanase activity and heparan sulfate levels were measured in plasma of healthy controls (n = 10) and COVID-19 patients (n = 48). Plasma heparanase activity and heparan sulfate levels were significantly elevated in COVID-19 patients. Heparanase activity was associated with disease severity including the need for intensive care, lactate dehydrogenase levels, and creatinine levels. Use of prophylactic LMWH in non-ICU patients was associated with a reduced heparanase activity. Since there is no other clinically applied heparanase inhibitor currently available, therapeutic treatment of COVID-19 patients with low molecular weight heparins should be explored.
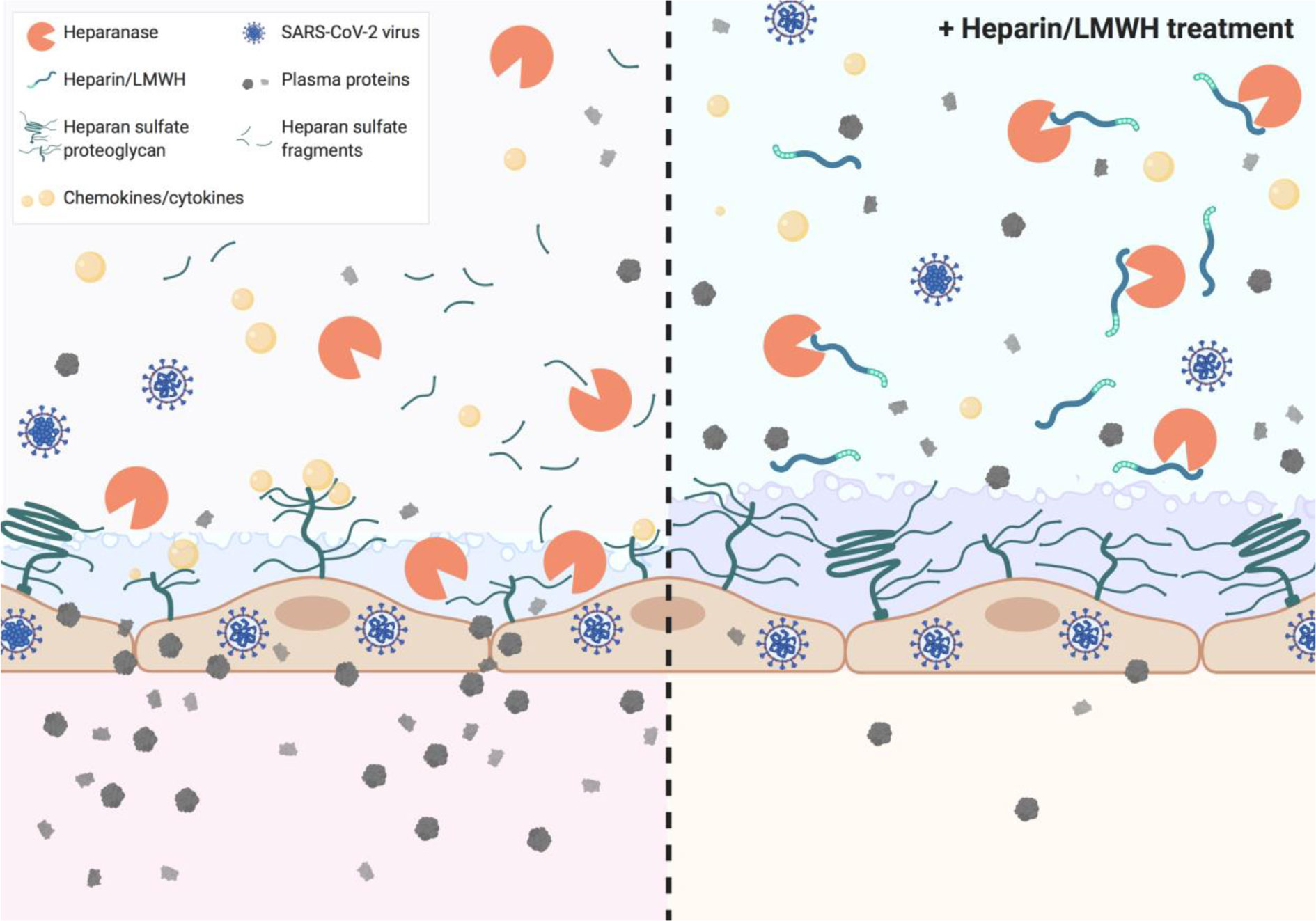
Graphical Abstract Heparin and low molecular weight heparins (LMWH) inhibit heparanase activity which is associated with disease severity in COVID-19.
Introduction
The coronavirus disease-2019 (COVID-19) pandemic is caused by the severe acute respiratory syndrome coronavirus 2 (SARS-CoV-2) (1). Severe COVID-19 usually manifests as pneumonitis or acute respiratory distress syndrome (ARDS) (2, 3). Studies showed that upon hospital admission 59% of COVID-19 patients had proteinuria (4), and 22% of the non-ventilated patients and 90% of the ventilated patients developed acute kidney injury (AKI) (5, 6).
Endothelial barrier function is crucial in the regulation of fluid and protein extravasation, particularly in the lungs (7, 8) and in the kidneys (9, 10). An important role for endothelial cell dysfunction in the pathogenesis of the complications of COVID-19 has been proposed by several studies (11, 12). As pulmonary edema occurs when fluid leaks into alveoli, dysfunction of the endothelium is likely to contribute to pulmonary edema in COVID-19. Furthermore, it has been well established that proteinuria occurs when the endothelial barrier function in the glomerulus is compromised (9, 10, 13).
Endothelial cells are covered with a thick layer of negatively charged glycosaminoglycans (GAGs), termed the glycocalyx. Heparan sulfate (HS) is the predominant sulfated GAG in the glycocalyx. HS contributes to the endothelial charge-dependent barrier function due to its negative charge (14). Degradation of HS by heparanase (HPSE), the only known mammalian HS-degrading enzyme, disrupts the endothelial glycocalyx and subsequent loss of endothelial barrier function, as observed in ARDS and proteinuric kidney diseases (7, 15–17). In addition to compromising barrier function, HPSE generates a pro-inflammatory glycocalyx that promotes the binding of chemokines, cytokines, and leukocytes to the endothelial cell surface (18). Inhibition of HPSE and/or HPSE deficiency is beneficial in experimental lung and kidney diseases (7, 15–17, 19). Notably, heparins and low molecular weight heparins (LMWH) that have been suggested to be beneficial for COVID-19 patients (20), are potent inhibitors of HPSE activity (21, 22).
Taken together, we hypothesize that increased HPSE activity is one of the driving forces in severe COVID-19 manifestations and that HPSE may be inhibited by the use of LMWH in COVID-19.
Methods
Human Samples, Demographics, and Baseline Characteristics
This study was performed according to the latest version of the declaration of Helsinki and guidelines for good clinical practice. The local independent ethical committee approved the study protocol (CMO 2020-6344, CMO 2020-6359, CMO 2016-2923). All patients admitted to the Radboud University Medical Center (Radboudumc) with a PCR-proven SARS-CoV-2 infection was asked for informed consent for participation in this study. After obtaining informed consent, ethylenediaminetetraacetic acid (EDTA) blood was collected and centrifuged for 10 min at 2,954 xg at room temperature (RT), plasma was collected and stored at −80°C for later analysis. For most patients in the non-ICU group, the blood samples collected between day 2 and 4 upon hospitalization were selected for this study except for the patients in the LMWH- group for whom the sample had to be collected immediately upon hospital admission prior to initiation of any standard medical intervention. Demographic data, medical history, and clinical laboratory measurements were collected from the medical file and processed in encoded form in electronic case report forms using Castor electronic data capture (Castor EDC, Amsterdam, Netherlands).
HPSE Activity Assay
The activity of HPSE in EDTA plasma was determined by an in-house developed activity assay, which was optimized by the use of recombinant active human HPSE (Bio-techne, Abingdon, United Kingdom, Cat#7570-GH-005). In detail, Nunc maxisorp flat bottom 96 plates (Thermo scientific, Breda, Netherlands) were coated with 10 ug/ml heparan sulfate from bovine kidney (HSBK) (Sigma-Aldrich, Zwijndrecht, Netherlands) in optimized HS coating buffer, overnight in a humidified chamber at RT. Subsequently, plates were washed with 0.05% PBS-Tween 20 (Sigma-Aldrich) (PBST) and blocked for minimal 2 h with 1% bacto-gelatin (Difco laboratories, Detroit, Michigan, USA) in PBS at RT. Plates were washed with PBST, followed by a final washing step with PBS prior to 2 h incubation at 37°C with 4 times diluted plasma sample in HPSE buffer. Samples were randomly distributed over plates. The HPSE buffer consisted of 50 mM citric acid-sodium citrate (Merck, Zwijndrecht, Netherlands) buffer supplemented with 50 mM NaCl (Merck), 1 mM CaCl2 (Sigma-Aldrich), and 1 mM DTT (Sigma-Aldrich) at final pH 5.0. Next, plates were washed with PBST and incubated with primary mouse anti-rat IgM HS antibody JM403 (Amsbio, Abingdon, United Kingdom, cat. no. #370730-S, RRID: AB_10890960, 1 μg/ml in PBST) for 1 h at RT. Subsequently, plates were washed with PBST and incubated with secondary goat anti-mouse IgM HRP antibody (Southern Biotech, Uden, Netherlands, cat. no. #1020-05, RRID: AB_2794201, dilution 1:10000 in PBST) for 1 h at RT. Finally, plates were washed with PBST and 3,3’,5,5’-tetramethylbenzidine (TMB) substrate (Invitrogen, Breda, Netherlands) was added and reaction was stopped by addition of 2 M sulfuric acid, and absorbance was measured at 450 nm. The HPSE activity in plasma was related to a standard curve of recombinant human HPSE in healthy control EDTA plasma.
For the in vitro HPSE inhibition experiment with dalteparin (Pfizer, Capelle a/d Ijssel, Netherlands, Fragmin 12,500 IU/0.5 ml), the HPSE activity was determined using the HPSE activity assay as outlined above. For inhibition studies 0–1 IU/ml dalteparin was used with a constant amount of 150 ng/ml recombinant human HPSE.
HS Competition Assay
HS in EDTA plasma samples was quantified by a previously described HS competition assay (23, 24). Importantly, this assay is specific to HS, therefore the measurement is not affected by the presence of LMWH use. Plates were coated with HSBK and blocked with bacto-gelatin as outlined for the HPSE activity assay. Uncoated plates, blocked with bacto-gelatin, were washed with PBST. The plasma samples were four times diluted in PBST containing primary mouse anti-rat IgM HS antibody JM403 (1.3 μg/ml) and incubated for 1 h at RT. Samples were randomly distributed over plates. Subsequently, the samples were transferred from the uncoated plates to the HSBK-coated plates and incubated for 1 h at RT. Plates were washed with PBST and incubated with secondary goat anti-mouse IgM HRP antibody for 1 h at RT. Plates were developed and measured as outlined for the HPSE activity assay. The amount of HS detected in plasma is expressed in arbitrary units since HS from bovine kidney was coated and used to prepare the standard curve.
IL-6 Measurements
IL-6 concentration was measured in plasma of COVID-19 patients using commercial ELISA kits for human IL-6 (Bio-techne, Abingdon, United Kingdom) according to manufacturer’s instruction.
Statistical Analysis
Values are expressed as mean ± SEM. D’Agostino & Pearson normality test was performed to test for normality of data. Significance was determined by Fisher’s exact test to compare categorical variables, by Student’s t-test or Mann Whitney test to compare two groups and by Kruskal-Wallis test followed by Dunn’s test to compare more than two groups using GraphPad Prism V.8.4.2 (La Jolla, USA). P values less than 0.05 were considered as statistically significant.
Results
Demographics and Baseline Characteristics of COVID-19 Patients
Plasma was collected from 48 PCR-confirmed COVID-19 patients admitted to the ICU (n = 14) or to designated COVID-19 clinical wards (n = 34). More men than women were included (Table 1). All ICU patients received LMWH as part of standard ICU treatment. The non-ICU patients were further aggregated in those receiving prophylactic LMWH (LMWH+) (in general dalteparin 5,000 IU subcutaneously once daily) (n = 17) and those receiving either alternative anticoagulation (n = 8; vitamin K antagonist n = 6, direct oral anticoagulant n = 2) or patients for whom the sample collection was performed before initiation of any standard medical intervention (LMWH−) (n = 9). Hospital stay duration was significantly different between ICU and non-ICU groups as well as between LMWH− and LMWH+ groups, while number of deaths was not significantly different between the different subgroups. Notably, ICU patients had a significantly higher C-reactive protein concentration than non-ICU patients (Table 2). The LMWH+ group had significantly higher median D-dimer concentrations compared to LMWH− group, whereas the concentrations of the inflammatory markers C-reactive protein and serum ferritin were similar between LMWH+ and LMWH−.
Plasma HPSE Activity Is Elevated in COVID-19 Patients
Several (experimental) disease models have shown that increased HPSE activity can lead to endothelial barrier dysfunction, which may be involved in the development of ARDS and proteinuria/AKI (7, 25, 26). Measurement of plasma HPSE activity levels in COVID-19 patients and healthy controls revealed that HPSE activity was significantly elevated in COVID-19 patients compared to healthy controls (Figure 1A). In line with the increased HPSE activity, HS plasma levels were also significantly elevated in COVID-19 patients compared to healthy controls (Figure 1B). Similarly, we detected significantly elevated IL-6 levels in COVID-19 patients compared to healthy controls (Figure 1C). Overall, these results suggest that SARS-CoV-2 infection is associated with an increase in the activity of HPSE in plasma and an increase in plasma HS and IL-6 levels.
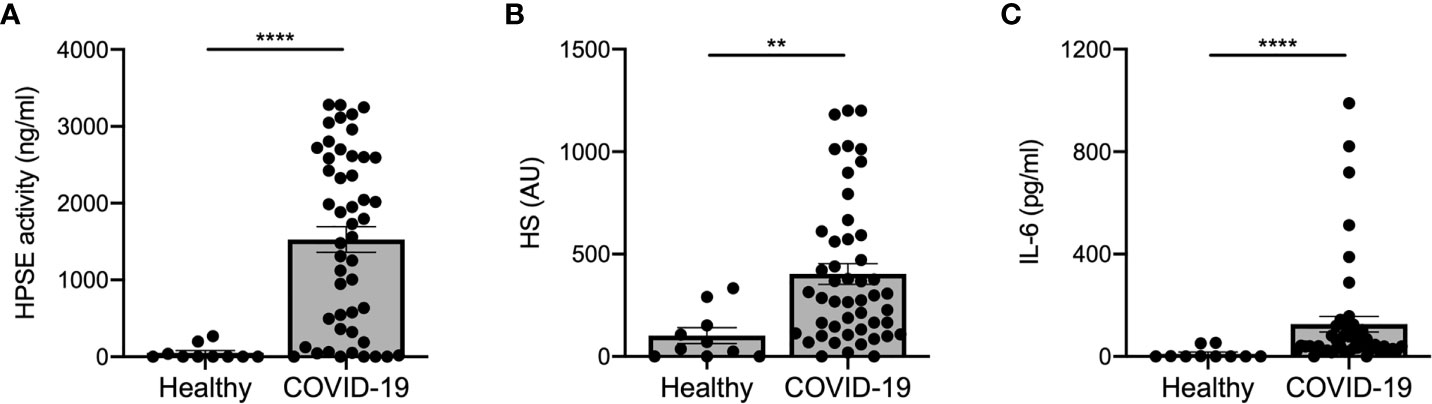
Figure 1 COVID-19 patients display increased HPSE activity, and elevated levels of heparan sulfate and IL-6 in plasma. (A) HPSE activity was increased in plasma of COVID-19 patients compared to healthy controls. HPSE activity was measured using an in-house developed ELISA with a specific anti-HS antibody. (B) HS levels were increased in plasma of COVID-19 patients compared to healthy controls. HS levels were measured by an in-house developed competition ELISA using a specific anti-HS antibody. (C) IL-6 levels were increased in plasma of COVID-19 patients compared to healthy controls. IL-6 levels were measured using a commercial IL-6 ELISA. Data were presented as mean ± SEM and tested for normal distribution with D’Agostino & Pearson omnibus normality test and statistical differences were calculated using Mann Whitney test (n = 10 healthy; n = 48 COVID-19; **p < 0.01; ****p < 0.0001). HPSE, heparanase; HS, heparan sulfate; Healthy, healthy controls; COVID-19, coronavirus disease-19 patients; AU, arbitrary units.
HPSE Activity Associates With COVID-19 Disease Severity
Next, we investigated whether HPSE activity levels were associated with COVID-19 disease severity. Plasma HPSE activity was significantly increased in both non-ICU and ICU patients compared to healthy controls, and HPSE levels in ICU patients, who all received mechanical ventilation, were higher than in non-ICU patients (Figure 2A). Moreover, HS (Figure 2B) and IL-6 levels (Figure 2C) in plasma were also higher in both non-ICU and ICU patients compared to healthy controls. Finally, plasma HPSE activity was significantly higher in patients with elevated LDH values (Figure 2D), and in patients with elevated serum creatinine values (Figure 2E). These findings reveal that patients with severe COVID-19 disease display higher plasma HPSE activity levels than patients with moderate COVID-19 disease.
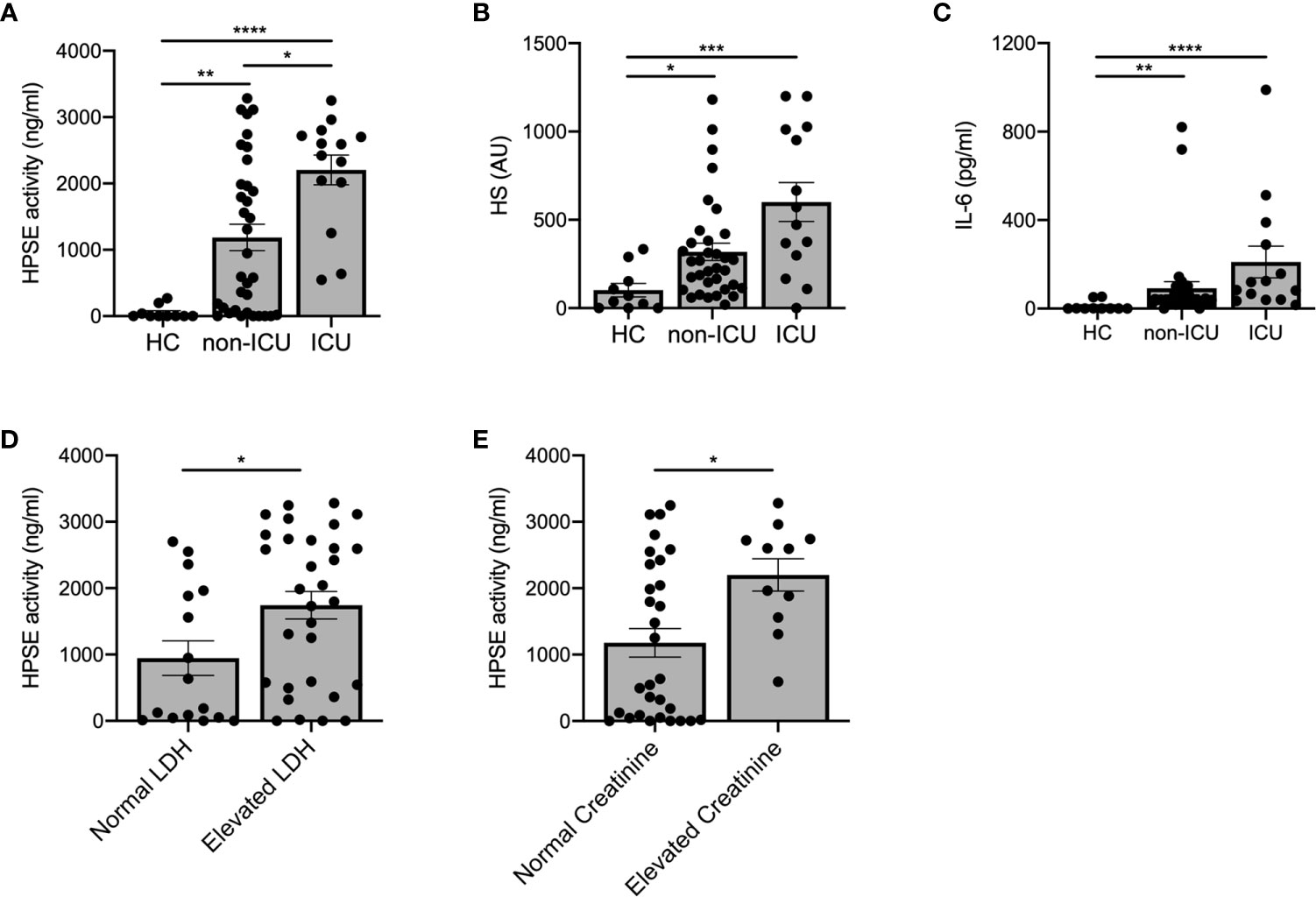
Figure 2 Increased plasma HPSE activity associates with COVID-19 disease severity. (A) Plasma HPSE activity was significantly higher in ICU and non-ICU patients compared to healthy controls, and higher in ICU patients compared to non-ICU patients (n = 10 healthy; n = 34 non-ICU; n = 14 ICU). (B) HS plasma levels were significantly increased in plasma of ICU and non-ICU patients compared to healthy controls (n = 10 healthy; n = 34 non-ICU; n = 14 ICU). (C) IL-6 plasma levels were significantly increased in plasma of ICU and non-ICU patients compared to healthy controls (n = 10 healthy; n = 34 non-ICU; n = 14 ICU). (D) HPSE activity was significantly higher in plasma of patients with elevated LDH (>280 U/L) values compared to patients with normal LDH levels (n = 15 normal LDH; n = 26 elevated LDH). (E) HPSE activity was significantly higher in plasma of patients with elevated creatinine (>110 µmol/L for men and >90 µmol/L for women) values compared to patients with normal creatinine values (n = 30 normal creatinine; n = 11 elevated creatinine; patients with history of renal disease were excluded from this analysis). HPSE activity was measured using an in-house developed ELISA with a specific anti-HS antibody and HS plasma levels were measured using an in-house developed competition ELISA with a specific anti-HS antibody. Data were presented as mean ± SEM and tested for normal distribution with D’Agostino & Pearson omnibus normality test and statistical differences were calculated using Kruskal Wallis test followed by Dunn’s multiple comparison test, unpaired one-tailed Student’s t-test or unpaired one-tailed Mann Whitney test (*p < 0.05; **p < 0.01; ***p < 0.001; ****p < 0.0001). HPSE, heparanase; HS, heparan sulfate; LDH, lactate dehydrogenase; Healthy, healthy controls; non-ICU, COVID-19 patients in normal hospital ward; ICU, COVID-19 patients in ICU; AU, arbitrary units.
Use of LMWH Is Associated With Lower HPSE Activity in Plasma of COVID-19 Patients
Prophylactic treatment with LMWH is recommended for patients hospitalized with COVID-19 (27), whereas some experts recommend higher doses for critically ill patients (28). As LMWH inhibits HPSE activity and it is known that HPSE deficiency reduces expression of various cytokines (15), we analyzed the effect of prophylactic LMWH on HPSE activity, HS levels and IL-6 levels in plasma of COVID-19 patients. Markedly, non-ICU patients who received LMWH displayed significantly lower plasma HPSE activity compared to non-ICU patients without LMWH prophylaxis (Figure 3A), whereas no statistically significant differences could be observed on HS levels (Figure 3B) or IL-6 levels (Figure 3C) between non-ICU patients with or without LMWH prophylaxis. According to literature, a single injection of 5,000 units dalteparin would result in an estimated concentration of around 0.37 U/ml in vivo (29). We found a dose dependent inhibition of recombinant HPSE at concentrations between 0.0025 and 0.05 U/ml and full inhibition starting from 0.25 U/ml dalteparin in vitro (Figure 3D). These data suggest that the applied prophylactic LMWH dose is already effective in inhibition of HPSE activity within plasma of moderately diseased, while HPSE activity remained high in severely ill, COVID-19 patients.
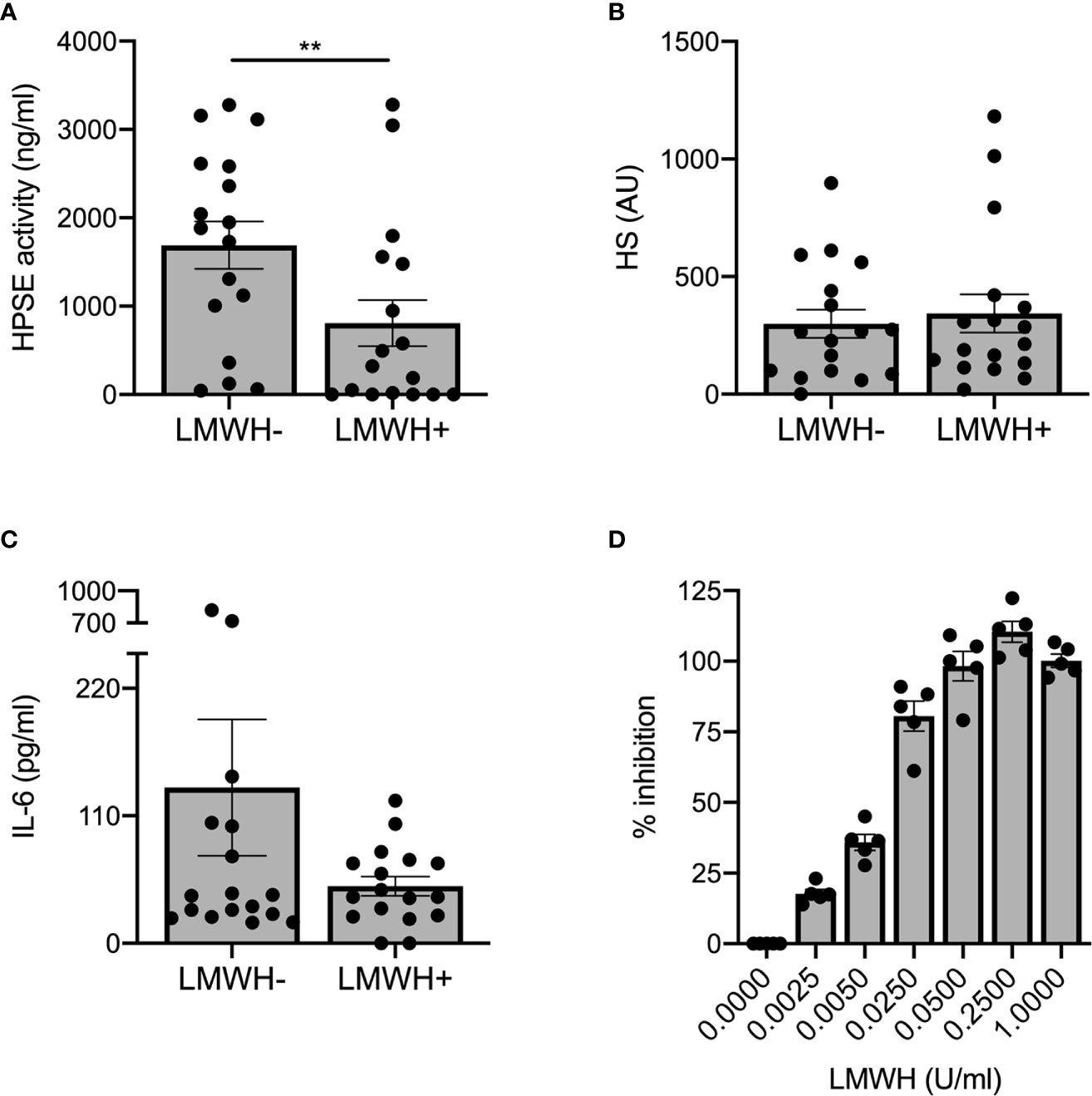
Figure 3 LMWH reduces plasma HPSE activity, but not plasma HS or IL-6 levels in moderately diseased COVID-19 patients. (A) LMWH reduces HPSE activity in plasma of non-ICU patients with COVID-19, which was measured using in-house developed HPSE activity assay (n = 17 for both groups, **p < 0.01). (B) LMWH does not reduce HS levels in plasma of non-ICU patients with COVID-19, which was measured with an in-house developed competition ELISA with a specific anti-HS antibody. (C) LMWH does not reduce IL-6 levels in plasma of non-ICU patients with COVID-19, which was measured using an in-house developed competition ELISA with a specific anti-HS antibody. Data were presented as mean ± SEM and tested for normal distribution with D’Agostino & Pearson omnibus normality test and statistical difference was calculated using unpaired one-tailed Mann Whitney test. (D) LMWH inhibits recombinant HPSE activity in vitro in a dose-dependent manner. HPSE activity was measured using an in-house developed ELISA with a specific anti-HS antibody (n = 5). HPSE, heparanase; HS, heparan sulfate; LMWH, low molecular weight heparin.
Discussion
COVID-19 appears to be a disease that leads to endothelial dysfunction and disruption of the endothelial barrier, which may underly development of ARDS and proteinuria/AKI (11, 30). Here, we report increased HPSE activity and HS levels in plasma of COVID-19 patients, which were also associated with severity of the disease.
Several mechanisms are currently proposed to explain pulmonary edema and ARDS in COVID-19. One suggested mechanism focuses on the kallikrein/kinin system, which is involved in the local inflammation and vascular leakage in the lung (31, 32). Over-activation of the bradykinin pathway may occur due to consumption of angiotensin converting enzyme-2 (ACE2) during viral entry (32). Interestingly, endothelial cell surface GAGs, such as HS, regulate activation of bradykinin pathways whereas degradation of HS by bacterial heparinases promotes proteolytic bradykinin generation (33). Therefore, increased plasma HPSE activity in COVID-19 patients could contribute to activation of the bradykinin pathway, and subsequently vascular leakage and local inflammation. The renin-angiotensin system also could be involved in endothelial dysfunction in COVID-19 patients (34). Increased angiotensin II levels have been reported in COVID-19 patients (35). Angiotensin II induces vasoconstriction, inflammation, fibrosis, and proliferation, which in turn can cause thrombosis, ARDS, and AKI. Importantly, we have previously shown that Angiotensin II is a potent inducer of HPSE expression (36, 37). Moreover, it is feasible that endothelin-1, one of the downstream mediators activated by angiotensin II (38, 39) is also increased in COVID-19 and it is known that endothelin-1 can induce HPSE expression as well (40).
Besides the role of HPSE in compromising the endothelial glycocalyx, HPSE and HS fragments play an important role in inflammation (41). HPSE can activate macrophages, resulting in secretion of MCP-1, TNF-α, and IL-1β, independent of HS-degrading activity (42). Released HS fragments also induce a pro-inflammatory response by binding to TLR2 and TLR4 (42, 43). Moreover, cleavage of HS by HPSE releases HS bound molecules, such as chemokines and cytokines, thereby promoting inflammation (44). Furthermore, cells exposed to HPSE show an enhanced response to pro-inflammatory cytokines like IFN-γ (17, 45, 46). Interestingly, cytokines such as IL-1β, IL-6, TNF-α, and MCP-1 appear to be elevated in COVID-19 patients (47–49) and also can induce HPSE expression (15). These data suggest the formation of a HPSE-mediated positive feed forward loop for inflammation in COVID-19. Notably, HPSE appears to have a direct effect in shaping the cytokine milieu, since HPSE deficiency reduces expression of a wide range of cytokines including TNF-α, IL-6, IFN-γ in experimental models (15).
Potential beneficial effects of prophylactic as well as therapeutic doses of LMWH in COVID-19 patients have been reported (50–53). Our data reveal that prophylactic doses of LMWH is associated with reduced HPSE activity in moderately diseased COVID-19 patients while this reduction of HPSE activity with LMWH prophylaxis was not accompanied with a reduction in HS or IL-6 plasma levels. Moreover, HPSE activity remained high in COVID-19 patients in ICU, all of whom received prophylactic LMWH as part of standard ICU treatment regimen. Therefore, therapeutic LMWH dose instead of prophylactic dose might be required to further inhibit HPSE both in non-ICU and ICU COVID-19 patients. This further reduction of HPSE activity might eventually lead to additional beneficial effect, such as reduced HS and IL-6 plasma levels. In addition to inhibition of HPSE, LMWH has other non-anticoagulant functions that may be beneficial for patients with COVID-19, such as neutralization of chemokines/cytokines, interference with leukocyte trafficking, neutralization of extracellular cytotoxic histones, neutralization of high molecular weight kinogen, and reduction of viral entry (33, 54–57).
In summary, this cross-sectional study shows that HPSE activity and HS levels are significantly elevated in plasma of COVID-19 patients, which is associated with the severity of COVID-19. Targeting of HPSE activity could be beneficial for the clinical outcome of COVID-19 patients, since it is well established that increased HPSE activity compromises the endothelial glycocalyx and contributes to a pro-inflammatory cytokine milieu. Considering the fact that no specific clinically approved heparanase inhibitors are currently available, prospective studies evaluating the clinical outcome of COVID-19 patients treated with therapeutic doses of LMWH are urgently needed.
Author’s Note
This manuscript has been released as a preprint at medRxiv (58).
Data Availability Statement
All datasets presented in this study are included in the article/supplementary material.
Ethics Statement
The studies involving human participants were reviewed and approved by the Local Independent Ethical Committee, Radboud University Medical Center, Nijmegen, Netherlands. The patients/participants provided their written informed consent to participate in this study.
Author Contributions
BB, CY, QdM, and JvdV designed the experiments, analyzed data, and wrote the manuscript. BB, CY, and MdG performed the experiments. AdN, IG, NAFJ, LABJ, MGN, and FLvdV were co-investigators on CMO 2020-6344, which provided COVID-19 patient samples. BB, CY, IJ, NR, RD, and JvdV were co-investigators on CMO 2020-6359, which provided COVID-19 patient samples. MK and PP were co-investigators on CMO 2016-2923, which facilitated COVID-19 ICU patient sampling. MLM-H created the graphical abstract and wrote the manuscript. TN and LH helped with analysis of the clinical data. JvdV has full access to all the data in the study and takes responsibility for the integrity of the data. All authors contributed to the article and approved the submitted version. BB and CY share first authorship and AdN and IG are co-second authors, listed in alphabetical order.
Funding
This study was financially supported by the Radboud University Medical Center PhD fellow program and consortium grant LSHM16058-SGF (GLYCOTREAT; a collaborative project financed by the PPP allowance made available by Top Sector Life Sciences & Health to the Dutch Kidney Foundation to stimulate public-private partnerships) coordinated by JvdV. MGN was supported by an ERC Advanced grant (#833247) and a Spinoza Grant of the Netherlands Organization for Scientific Research. The graphical abstract was created with BioRender.com.
Conflict of Interest
The authors declare that the research was conducted in the absence of any commercial or financial relationships that could be construed as a potential conflict of interest.
References
1. Gordon DE, Jang GM, Bouhaddou M, Xu J, Obernier K, White KM, et al. A SARS-CoV-2 protein interaction map reveals targets for drug repurposing. Nature (2020). doi: 10.1038/s41586-020-2286-9
2. Wu C, Chen X, Cai Y, Ja X, Zhou X, Xu S, et al. Risk Factors Associated With Acute Respiratory Distress Syndrome and Death in Patients With Coronavirus Disease 2019 Pneumonia in Wuhan, China. JAMA Internal Med (2020) 180(7):934–43. doi: 10.1001/jamainternmed.2020.0994
3. Lake MA. What we know so far: COVID-19 current clinical knowledge and research. Clin Med (Lond) (2020) 20(2):124–7. doi: 10.7861/clinmed.2019-coron
4. Li Z, Wu M, Yao J, Guo J, Liao X, Song S, et al. Caution on Kidney Dysfunctions of COVID-19 Patients. medRxiv (2020). doi: 10.1101/2020.02.08.20021212
5. Cheng Y, Luo R, Wang K, Zhang M, Wang Z, Dong L, et al. Kidney disease is associated with in-hospital death of patients with COVID-19. Kidney Int (2020) 97(5):829–38. doi: 10.1101/2020.02.18.20023242
6. Hirsch JS, Ng JH, Ross DW, Sharma P, Shah HH, Barnett RL, et al. Acute kidney injury in patients hospitalized with COVID-19. Kidney Int (2020) 98(1):209–18. doi: 10.1016/j.kint.2020.05.006
7. Schmidt EP, Yang Y, Janssen WJ, Gandjeva A, Perez MJ, Barthel L, et al. The pulmonary endothelial glycocalyx regulates neutrophil adhesion and lung injury during experimental sepsis. Nat Med (2012) 18(8):1217–23. doi: 10.1038/nm.2843
8. Haeger SM, Yang Y, Schmidt EP. Heparan Sulfate in the Developing, Healthy, and Injured Lung. Am J Respir Cell Mol Biol (2016) 55(1):5–11. doi: 10.1165/rcmb.2016-0043TR
9. Garsen M, Rops AL, Rabelink TJ, Berden JH, van der Vlag J. The role of heparanase and the endothelial glycocalyx in the development of proteinuria. Nephrol Dial Transplant (2014) 29(1):49–55. doi: 10.1093/ndt/gft410
10. Haraldsson B, Nyström J, Deen WM. Properties of the Glomerular Barrier and Mechanisms of Proteinuria. Physiol Rev (2008) 88(2):451–87. doi: 10.1152/physrev.00055.2006
11. Varga Z, Flammer AJ, Steiger P, Haberecker M, Andermatt R, Zinkernagel AS, et al. Endothelial cell infection and endotheliitis in COVID-19. Lancet (2020) 395(10234):1417–8. doi: 10.1016/S0140-6736(20)30937-5
12. Sardu C, Gambardella J, Morelli M, Wang X, Marfella R, Santulli G. Is COVID-19 an endothelial disease? Clinical and basic evidence. Preprints (2020) 2020040204. doi: 10.20944/preprints202004.0204.v1
13. Salmon AH, Satchell SC. Endothelial glycocalyx dysfunction in disease: albuminuria and increased microvascular permeability. J Pathol (2012) 226(4):562–74. doi: 10.1002/path.3964
14. Goldberg R, Meirovitz A, Hirshoren N, Bulvik R, Binder A, Rubinstein AM, et al. Versatile role of heparanase in inflammation. Matrix Biol (2013) 32(5):234–40. doi: 10.1016/j.matbio.2013.02.008
15. Garsen M, Benner M, Dijkman HB, van Kuppevelt TH, Li JP, Rabelink TJ, et al. Heparanase Is Essential for the Development of Acute Experimental Glomerulonephritis. Am J Pathol (2016) 186(4):805–15. doi: 10.1016/j.ajpath.2015.12.008
16. Gil N, Goldberg R, Neuman T, Garsen M, Zcharia E, Rubinstein AM, et al. Heparanase is essential for the development of diabetic nephropathy in mice. Diabetes (2012) 61(1):208–16. doi: 10.2337/db11-1024
17. Kiyan Y, Tkachuk S, Kurselis K, Shushakova N, Stahl K, Dawodu D, et al. Heparanase-2 protects from LPS-mediated endothelial injury by inhibiting TLR4 signalling. Sci Rep (2019) 9(1):13591. doi: 10.1038/s41598-019-50068-5
18. McDonald KK, Cooper S, Danielzak L, Leask RL. Glycocalyx Degradation Induces a Proinflammatory Phenotype and Increased Leukocyte Adhesion in Cultured Endothelial Cells under Flow. PloS One (2016) 11(12):e0167576. doi: 10.1371/journal.pone.0167576
19. Vlag J, Buijsers B. The Glomerular Endothelium in Diabetic Nephropathy: Role of Heparanase: Pathophysiology and Clinical Aspects. Eds. Roelofs, Joris J., Vogt, Liffert, Springer (2019) pp. 153–70.
20. Shi C, Wang C, Wang H, Yang C, Cai F, Zeng F, et al. The potential of low molecular weight heparin to mitigate cytokine storm in severe covid-19 patients: a retrospective clinical study. medRxiv (2020). doi: 10.1101/2020.03.28.20046144
21. Zetser A, Levy-Adam F, Kaplan V, Gingis-Velitski S, Bashenko Y, Schubert S, et al. Processing and activation of latent heparanase occurs in lysosomes. J Cell Sci (2004) 117:2249–58. doi: 10.1242/jcs.01068
22. Bar-Ner M, Eldor A, Wasserman L, Matzner Y, Cohen IR, Fuks Z, et al. Inhibition of heparanase-mediated degradation of extracellular matrix heparan sulfate by non-anticoagulant heparin species. Blood (1987) 70(2):551–7. doi: 10.1182/blood.V70.2.551.551
23. Rops AL, van den Hoven MJ, Baselmans MM, Lensen JF, Wijnhoven TJ, van den Heuvel LP, et al. Heparan sulfate domains on cultured activated glomerular endothelial cells mediate leukocyte trafficking. Kidney Int (2008) 73(1):52–62. doi: 10.1038/sj.ki.5002573
24. Cohen-Mazor M, Sela S, Mazor R, Ilan N, Vlodavsky I, Rops AL, et al. Are primed polymorphonuclear leukocytes contributors to the high heparanase levels in hemodialysis patients? Am J Physiol Heart Circ Physiol (2008) 294(2):H651–8. doi: 10.1152/ajpheart.00952.2007
25. LaRiviere WB, Schmidt EP. The Pulmonary Endothelial Glycocalyx in ARDS: A Critical Role for Heparan Sulfate. Curr Top Membr (2018) 82:33–52. doi: 10.1016/bs.ctm.2018.08.005
26. Chowdhury MS, Rathod J, Gernsheimer J. A Rapid Systematic Review of Clinical Trials Utilizing Chloroquine and Hydroxychloroquine as a Treatment for COVID-19. Acad Emerg Med (2020) 27(6):493–504. doi: 10.1111/acem.14005
27. Thachil J, Tang N, Gando S, Falanga A, Cattaneo M, Levi M, et al. ISTH interim guidance on recognition and management of coagulopathy in COVID-19. J Thromb Haemost (2020) 18(5):1023–6. doi: 10.1111/jth.14810
28. Klok FA, Kruip M, van der Meer NJM, Arbous MS, Gommers D, Kant KM, et al. Incidence of thrombotic complications in critically ill ICU patients with COVID-19. Thromb Res (2020) 191:145–7. doi: 10.1016/j.thromres.2020.04.041
29. Yee JY, Duffull SB. The effect of body weight on dalteparin pharmacokinetics. A preliminary study. Eur J Clin Pharmacol (2000) 56(4):293–7. doi: 10.1007/s002280000141
30. Sardu C, Gambardella J, Morelli MB, Wang X, Marfella R, Santulli G. Hypertension, Thrombosis, Kidney Failure, and Diabetes: Is COVID-19 an Endothelial Disease? A Comprehensive Evaluation of Clinical and Basic Evidence. J Clin Med (2020) 9(5):1417. doi: 10.3390/jcm9051417
31. Sodhi CP, Wohlford-Lenane C, Yamaguchi Y, Prindle T, Fulton WB, Wang S, et al. Attenuation of pulmonary ACE2 activity impairs inactivation of des-Arg(9) bradykinin/BKB1R axis and facilitates LPS-induced neutrophil infiltration. Am J Physiol Lung Cell Mol Physiol (2018) 314(1):L17–l31. doi: 10.1152/ajplung.00498.2016
32. van de Veerdonk F, Netea M, Deuren M, Hoogen F, Mast Q, Bruggemann R, et al. Kinins and Cytokines in COVID-19: A Comprehensive Pathophysiological Approach. Preprints (2020) 2020040023. doi: 10.20944/preprints202004.0023.v1
33. Renne T, Schuh K, Muller-Esterl W. Local bradykinin formation is controlled by glycosaminoglycans. J Immunol (2005) 175(5):3377–85. doi: 10.4049/jimmunol.175.5.3377
34. Guo J, Huang Z, Lin L, Lv J. Coronavirus Disease 2019 (COVID‐19) and Cardiovascular Disease: A Viewpoint on the Potential Influence of Angiotensin‐Converting Enzyme Inhibitors/Angiotensin Receptor Blockers on Onset and Severity of Severe Acute Respiratory Syndrome Coronavirus 2 Infection. J Am Heart Assoc (2020) 9(7):e016219. doi: 10.1161/JAHA.120.016219
35. Liu Y, Yang Y, Zhang C, Huang F, Wang F, Yuan J, et al. Clinical and biochemical indexes from 2019-nCoV infected patients linked to viral loads and lung injury. Sci China Life Sci (2020) 63(3):364–74. doi: 10.1007/s11427-020-1643-8
36. Kramer A, van den Hoven M, Rops A, Wijnhoven T, van den Heuvel L, Lensen J, et al. Induction of glomerular heparanase expression in rats with adriamycin nephropathy is regulated by reactive oxygen species and the renin-angiotensin system. J Am Soc Nephrol (2006) 17(9):2513–20. doi: 10.1681/ASN.2006020184
37. van den Hoven MJ, Waanders F, Rops AL, Kramer AB, van Goor H, Berden JH, et al. Regulation of glomerular heparanase expression by aldosterone, angiotensin II and reactive oxygen species. Nephrol Dial Transplant (2009) 24(9):2637–45. doi: 10.1093/ndt/gfp182
38. Lin YJ, Kwok CF, Juan CC, Hsu YP, Shih KC, Chen CC, et al. Angiotensin II enhances endothelin-1-induced vasoconstriction through upregulating endothelin type A receptor. Biochem Biophys Res Commun (2014) 451(2):263–9. doi: 10.1016/j.bbrc.2014.07.119
39. Hong HJ, Chan P, Liu JC, Juan SH, Huang MT, Lin JG, et al. Angiotensin II induces endothelin-1 gene expression via extracellular signal-regulated kinase pathway in rat aortic smooth muscle cells. Cardiovasc Res (2004) 61(1):159–68. doi: 10.1016/j.cardiores.2003.10.019
40. Garsen M, Lenoir O, Rops ALWMM, Dijkman HB, Willemsen B, van Kuppevelt TH, et al. Endothelin-1 Induces Proteinuria by Heparanase-Mediated Disruption of the Glomerular Glycocalyx. J Am Soc Nephrol JASN (2016) 27(12):3545–51. doi: 10.1681/ASN.2015091070
41. Rabelink TJ, van den Berg BM, Garsen M, Wang G, Elkin M, van der Vlag J. Heparanase: roles in cell survival, extracellular matrix remodelling and the development of kidney disease. Nat Rev Nephrol (2017) 13(4):201–12. doi: 10.1038/nrneph.2017.6
42. Blich M, Golan A, Arvatz G, Sebbag A, Shafat I, Sabo E, et al. Macrophage activation by heparanase is mediated by TLR-2 and TLR-4 and associates with plaque progression. Arterioscler Thromb Vasc Biol (2013) 33(2):e56–65. doi: 10.1161/ATVBAHA.112.254961
43. Goodall KJ, Poon IK, Phipps S, Hulett MD. Soluble heparan sulfate fragments generated by heparanase trigger the release of pro-inflammatory cytokines through TLR-4. PloS One (2014) 9(10):e109596. doi: 10.1371/journal.pone.0109596
44. Gordts Philip LSM, Foley Erin M, Lawrence R, Sinha R, Lameda-Diaz C, Deng L, et al. Reducing Macrophage Proteoglycan Sulfation Increases Atherosclerosis and Obesity through Enhanced Type I Interferon Signaling. Cell Metab (2014) 20(5):813–26. doi: 10.1016/j.cmet.2014.09.016
45. Goldberg R, Rubinstein AM, Gil N, Hermano E, Li JP, van der Vlag J, et al. Role of heparanase-driven inflammatory cascade in pathogenesis of diabetic nephropathy. Diabetes (2014) 63(12):4302–13. doi: 10.2337/db14-0001
46. Goldberg R, Sonnenblick A, Hermano E, Hamburger T, Meirovitz A, Peretz T, et al. Heparanase augments insulin receptor signaling in breast carcinoma. Oncotarget (2017) 8(12):19403–12. doi: 10.18632/oncotarget.14292
47. Coperchini F, Chiovato L, Croce L, Magri F, Rotondi M. The Cytokine storm in COVID-19: An overview of the involvement of the chemokine/chemokine-receptor system. Cytokine Growth Factor Rev (2020) 53:25–32. doi: 10.1016/j.cytogfr.2020.05.003
48. Huang C, Wang Y, Li X, Ren L, Zhao J, Hu Y, et al. Clinical features of patients infected with 2019 novel coronavirus in Wuhan, China. Lancet (2020) 395(10223):497–506. doi: 10.1016/S0140-6736(20)30183-5
49. Boels MGS, Koudijs A, Avramut MC, Sol WMPJ, Wang G, van Oeveren-Rietdijk AM, et al. Systemic Monocyte Chemotactic Protein-1 Inhibition Modifies Renal Macrophages and Restores Glomerular Endothelial Glycocalyx and Barrier Function in Diabetic Nephropathy. Am J Pathol (2017) 187(11):2430–40. doi: 10.1016/j.ajpath.2017.07.020
50. Tang N, Bai H, Chen X, Gong J, Li D, Sun Z. Anticoagulant treatment is associated with decreased mortality in severe coronavirus disease 2019 patients with coagulopathy. J Thromb Haemost (2020) 18(5):1094–9. doi: 10.1111/jth.14817
51. Negri E, Piloto B, Morinaga L, Jardim C, Lamy S, Ferreira M, et al. Heparin therapy improving hypoxia in COVID-19 patients - a case series. medRxiv (2020). doi: 10.1101/2020.04.15.20067017
52. Shi C, Wang C, Wang H, Yang C, Cai F, Zeng F, et al. Clinical observations of low molecular weight heparin in relieving inflammation in COVID-19 patients: A retrospective cohort study. medRxiv (2020). doi: 10.1111/cts.12880
53. Thachil J. The versatile heparin in COVID-19. J Thromb Haemost (2020) 18(5):1020–2. doi: 10.1111/jth.14821
54. Buijsers B, Yanginlar C, Maciej-Hulme ML, de Mast Q, van der Vlag J. Beneficial non-anticoagulant mechanisms underlying heparin treatment of COVID-19 patients. EBioMedicine (2020) 59:102969. doi: 10.1016/j.ebiom.2020.102969
55. Ludwig RJ. Therapeutic use of heparin beyond anticoagulation. Curr Drug Discovery Technol (2009) 6(4):281–9. doi: 10.2174/157016309789869001
56. Kim S, Jin W, Sood A, Montgomery D, Grant O, Fuster M, et al. Glycosaminoglycan binding motif at S1/S2 proteolytic cleavage site on spike glycoprotein may facilitate novel coronavirus (SARS-CoV-2) host cell entry. bioRxiv (2020). doi: 10.1101/2020.04.14.041459
57. Wang F, Zhang N, Li B, Liu L, Ding L, Wang Y, et al. Heparin defends against the toxicity of circulating histones in sepsis. Front Biosci (Landmark Ed) (2015) 20:1259–70. doi: 10.2741/4370
Keywords: inflammation, heparanase, LMWH (low molecular weight heparin), vascular leakage, glycocalyx damage, COVID-19
Citation: Buijsers B, Yanginlar C, de Nooijer A, Grondman I, Maciej-Hulme ML, Jonkman I, Janssen NAF, Rother N, de Graaf M, Pickkers P, Kox M, Joosten LAB, Nijenhuis T, Netea MG, Hilbrands L, van de Veerdonk FL, Duivenvoorden R, de Mast Q and van der Vlag J (2020) Increased Plasma Heparanase Activity in COVID-19 Patients. Front. Immunol. 11:575047. doi: 10.3389/fimmu.2020.575047
Received: 22 June 2020; Accepted: 14 September 2020;
Published: 06 October 2020.
Edited by:
Christoph Thiemermann, Queen Mary University of London, United KingdomReviewed by:
Fausto Chiazza, University of Eastern Piedmont, ItalyGareth S. D. Purvis, University of Oxford, United Kingdom
Michael Rutzler, ApoGlyx, Sweden
Copyright © 2020 Buijsers, Yanginlar, de Nooijer, Grondman, Maciej-Hulme, Jonkman, Janssen, Rother, de Graaf, Pickkers, Kox, Joosten, Nijenhuis, Netea, Hilbrands, van de Veerdonk, Duivenvoorden, de Mast and van der Vlag. This is an open-access article distributed under the terms of the Creative Commons Attribution License (CC BY). The use, distribution or reproduction in other forums is permitted, provided the original author(s) and the copyright owner(s) are credited and that the original publication in this journal is cited, in accordance with accepted academic practice. No use, distribution or reproduction is permitted which does not comply with these terms.
*Correspondence: Johan van der Vlag, Sm9oYW4udmFuZGVyVmxhZ0ByYWRib3VkdW1jLm5s; orcid.org/0000-0001-7843-5918
†These authors share first authorship
‡These authors share last authorship