- 1Department of Orthopedics, Renmin Hospital of Wuhan University, Wuhan, China
- 2Renmin Hospital of Wuhan University, Wuhan, China
- 3Hubei Provincial Hospital of Traditional Chinese Medicine (TCM), Wuhan, China
The complicated connections and cross talk between the skeletal system and the immune system are attracting more attention, which is developing into the field of Osteoimmunology. In this field, cytokines that are among osteoblasts and osteoclasts play a critical role in bone remodeling, which is a pathological process in the pathogenesis and development of osteoporosis. Those cytokines include the tumor necrosis factor (TNF) family, the interleukin (IL) family, interferon (IFN), chemokines, and so on, most of which influence the bone microenvironment, osteoblasts, and osteoclasts. This review summarizes the effect of cytokines on osteoblasts and osteoclasts in bone remodeling in osteoporosis, aiming to providing the latest reference to the role of immunology in osteoporosis.
Introduction
Osteoporosis is an orthopedic disease characterized by bone mass reduction and bone tissue microstructure damage, which can increase bone fragility and the incidence of fractures (1). Osteoporosis can occur at any age, but is more common in postmenopausal women and in elderly men, indicating a close association with aging (2). Given the health impact of osteoporosis for the increasingly middle-aged and elderly global population, osteoporosis has become a chronic disease that causes a huge disease burden and great socioeconomic pressure (3, 4).
In the normal body microenvironment, there is a balance between bone formation by osteoblasts and bone resorption by osteoclasts, supporting the bone mass and bone mineral density (BMD) within the normal range. However, this balance is disrupted in osteoporosis patients and the bone resorption exceeds bone formation, initiated by the suppression of osteoblasts or the over-activation of osteoclasts, which is referred to as bone remodeling (5). In this pathological process, the immune system plays a significant role, including through immune cells, various cytokines, and signaling pathways. The cross talk between the skeletal system and the immune system forms an interdisciplinary field called Osteoimmunology (6).
In the cytokine networks and signaling pathways, the receptor of NF-κB (RANK)/RANK ligand (RANKL)/osteoprotegerin (OPG) axis plays an important role in the bone remodeling process, which has already been researched systematically (7). Although the function of other proinflammatory cytokines, such as the tumor necrosis factor (TNF) family, the interleukin (IL) family, and interferon (IFN), has already been researched quite a lot in the bone remodeling literature (8), summary work remains in need. Therefore, in this review, we will describe the roles of various cytokines in bone remodeling and their impact on the pathological process of osteoporosis, combining recent discoveries (Table 1) in order to contribute to the search for new therapeutic targets for osteoporosis.
Molecular mechanism of osteoporosis
Osteoporosis happens when the balance between bone resorption by osteoclast cells and bone formation by osteoblast cells breaks down. In the process of osteoclast differentiation and activation, osteoblast cells express RANKL and OPG, participating in modulating the differentiation of osteoclast cells. RANKL binding to RANK and lead to the activation of osteoclast differentiation via activation of downstream signaling pathways, while OPG inhibit aforementioned effect through inhibition of RANKL-RANK interaction (122) (Figure 1). In addition, osteoblast cells secrete macrophage colony-stimulating factor (M-CSF) binding to colony-stimulating factor-1 receptor (c-Fms) in osteoclast cells, which leads to the activation of phosphoinositide 3-kinase (PI3K) and growth factor receptor bound protein 2 (Grb2) and further promotes Akt and ERK signaling in osteoclast precursors or mature osteoclast cells (8). It has been widely recognized that the RANK/RANKL/OPG axis plays a critical role in the molecular mechanism of osteoporosis. When pro-inflammatory cytokines affect this axis and enhance its osteoclastogenesis to a state of decompensation, bone mass reduces and the bone tissue microstructure becomes damaged, leading to the occurrence of osteoporosis.
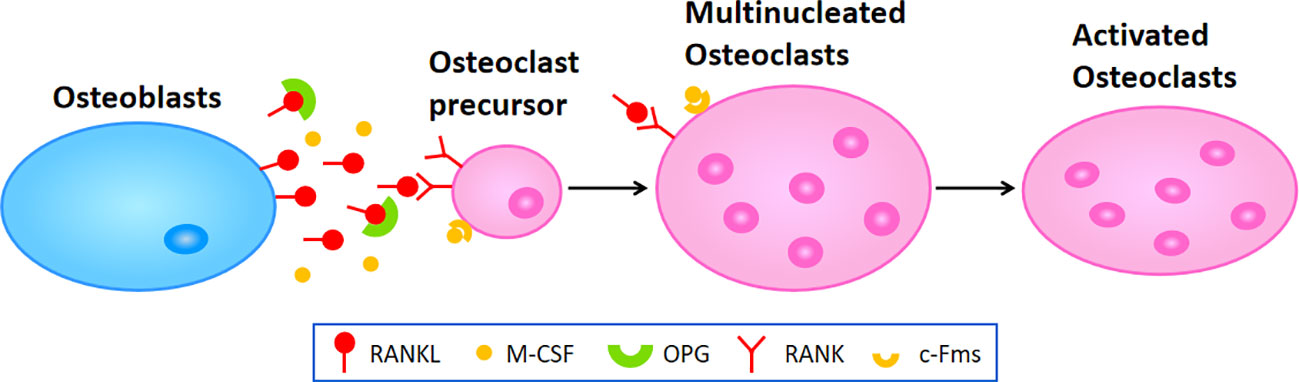
Figure 1 The molecular mechanism of osteoclastogenesis. Osteoblasts secret RANKL and M-CSF which respectively binding to RANK and c-Fms in osteoclast precursors or multinucleated osteoclasts to promote the differentiation or fusion during osteoclastogenesis, leading to the activation of osteoclasts in the end.
Tumor necrosis factor
TNF is subdivided into TNF-α and TNF-β, both of which strongly stimulate bone resorption. TNF contributes to the development of bone loss by fostering the formation of osteoclasts and inhibiting osteoblast function. TNF can stimulate osteoclast differentiation via multiple mechanisms, some of which are independent of the RANKL/RANK axis (123, 124). TNF-α stimulates stromal cells and osteoblasts and activates T cells to express the RANKL and M-CSF genes, which indirectly promote the expression of RANK in osteoclast precursors and subsequent osteoclastogenesis via M-CSF (9–11). In postmenopausal women with osteoporosis, TNF-α is closely associated with levels of RANK and estrogen. By activating NF-κB and PI3K/Akt signaling, TNF-α can promote RANKL-induced osteoclast formation in vitro in a synergistic manner (12). It may be one of the pathogenic mechanisms underlying osteoporosis after menopause.
Independent of the RANKL/RANK axis, TNF-α can directly exert biological effects. TNF-α induces the differentiation of osteoclast precursors by acting directly on their surface receptors and sequentially activating NF-κB, p50/p52, c-Fos, and nuclear factor-activated T cells c1 (NFATc1) (125, 126). TNF-α can also directly induce osteoclast precursors to express c-Fos, which produces IL-1β by interacting with bone matrix proteins and inducing osteoclast differentiation autocrine (127).
Tumor necrosis factor receptor-associated factors (TRAFs) are crucial in physiological bone remodeling and also influence TNF-induced osteoclast formation. TRAF2 (128) and TRAF5 (129) have been shown to be essential for TNF-α-induced osteoclast formation. The most important of the TRAFs is TRAF6, and mice lacking this factor develop severe osteoporosis due to inadequate osteoclast differentiation (130). However, as demonstrated in one study, RANKL might degrade the osteoclast formation inhibitor TRAF3 by inducing autophagic lysosomes, thereby stimulating TNF-induced osteoclast formation independent of TRAF6 signaling (131).
TNF has a concentration-dependent, bidirectional effect on bone formation and osteoblast function. Low concentrations of TNF stimulate mesenchymal precursor cells to differentiate into osteoblasts, whereas high concentrations of TNF inhibit osteoblast function and bone formation (13). TNF inhibits insulin-like growth factor I (IGF-I) expression in osteoblast precursor cells during the initial stages of differentiation, thereby inhibiting osteoblast differentiation (14). TNF also can inhibit the expression of RUNX2, a key factor regulating osteoblast differentiation, to suppress osteoblast differentiation (15).
Interleukins
Interleukin-1
Interleukin (IL)-1, composed of IL-1α and IL-1β, is an introductory class of cytokines that can activate osteoclasts and participate in osteoclast differentiation, multinucleation, and survival, which is also a crucial mediator of inflammatory bone loss (17). Among them, IL-1β is a decisive osteoclast factor that upregulates RANKL production and enhances its activity, and stimulates osteoclast formation (18, 19). IL-1β can indirectly promote TNF-α-induced osteoclastogenesis, induce stromal cell RANKL expression with the support of p38 MAPK, and thus directly stimulate osteoclast precursor differentiation (20). Interestingly, IL-1β has distinct effects on subpopulations of osteoclast precursors, stimulating polynucleation and bone resorption at varying rates in osteoclasts derived from the three precursors (132).
IL-1 is capable of RANKL/RANK-independent activation of osteoclast differentiation via IL-1/IL-1R1 signaling in bone marrow-derived macrophages (BMMs) by activating osteogenic marker genes such as NF-κB, JNK, p38, and ERK, as well as a microphthalmia transcription factor (MITF) to induce osteoclast differentiation. Nonetheless, this process does not stimulate c-Fos or NFATc1 expression and requires both to be at a certain baseline level (133). Not only do TNF and IL-1 induce bone resorption in osteoblasts by activating p38 MAPK in the osteoblast spectrum (21), but IL-1 also inhibits human osteoblast migration, thus impacting the fracture healing process (22).
In contrast, IL-1α can stimulate the formation of osteoclast-like cells (OLCs) by increasing the expression of M-CSF and PGE2 and decreasing the expression of OPG in osteoblasts (16).
Interleukin-3
IL-3, also known as multicolony stimulation factor (multi-CSF), is a member of the βc family due to the fact that its receptor shares the signaling subunit βc with granulocyte/macrophage colony-stimulating factor (GM-CSF) and IL-5 (134). Activated T lymphocytes, mast cells, and osteoblasts are the primary secretors (135).
Although IL-3 is predominantly regarded as an anti-osteoclastic cytokine, it actually has a role in both osteoblasts and osteoclasts.
IL-3 inhibits RANKL-induced NF-κB nuclear translocation by inhibiting IκB phosphorylation and degradation, which further inhibits RANKL-induced osteoclast differentiation by acting directly on early osteoclast precursors (23). By downregulating the expression of TNFR1 and TNFR2, IL-3 inhibits TNF-induced osteoclast differentiation (24) and bone resorption (25). All of the above-mentioned IL-3 inhibition is irreversible. IL-3 substantially inhibits c-Fms, downregulating PU.1 and c-Fos expression at the mRNA and protein levels, leading to the suppression of differentiation of blood monocytes and bone marrow cells into osteoclasts and bone resorption, thereby inhibiting bone erosion (26–28).
In the osteogenesis process, IL-3 increases osteoblast differentiation and matrix mineralization in human mesenchymal stem cells (MSCs) in a dose-dependent manner, significantly boosting the expression of osteoblast-specific genes including alkaline phosphatase, collagen type-I, osteocalcin, and osteopontin, as well as the transcription factors Runx-2 and osterix (29). Through the JAK/STAT signaling pathway, IL-3 also promotes osteoblast differentiation and BMP-2 secretion (29).
Another study demonstrated that IL-3 increased RANKL expression at both the transcriptional and translational levels without affecting OPG expression. Increased RANKL induces mononuclear osteoblasts without affecting multinuclear osteoblasts. IL-3 regulated two functional forms of RANKL by downregulating metalloproteinases, including ADAM10, ADAM17, ADAM19, and MMP3, to downregulate soluble RANKL expression and increase membrane-bound RANKL expression via the JAK2/STAT5 signaling pathway, thereby restoring the decreased RANKL/OPG ratio in adult mice (136).
Interleukin-4
IL-4 is a pleiotropic immunomodulatory cytokine produced primarily by Th2 cells, mast cells, and eosinophils (137). It regulates immune responses and has now been shown to be a potent inhibitor of osteoclastogenesis, affecting osteoclast formation and function in multiple ways, both directly and indirectly.
IL-4 reduces the nuclear translocation of NF-κB by inhibiting IκB phosphorylation in a STAT6-dependent manner, which significantly hinders the DNA-binding activity of NF-κB and directly suppresses osteoclastogenesis (30).
In addition, IL-4 can also inhibit RANKL and TNF-α-induced osteoclastogenesis by blocking the MAPK signaling pathway (31). Further studies showed that IL-4 inhibited the expression of NFATc1, a significant transcription factor for RANKL-induced osteoclast formation, via STAT6, thereby inhibiting osteoclast formation (32). In addition to inhibiting osteoclast formation, IL-4 directly inhibits the bone resorption activity of mature, differentiated osteoclasts by inhibiting the NF-κB and Ca2+ signaling pathways in a STAT6-dependent manner (33, 34). These are all direct inhibitory effects of IL-4 on osteoclastogenesis. By diminishing the production of pro-osteoclast factors such as TNF-α, IL-1, and IL-6, IL-4 also indirectly inhibits osteoclastogenesis (35). In contrast, endothelial cells of the bone vascular system secrete osteogenic cytokines and hormones to modulate bone development, remodeling, and repair. IL-4 and its closely related IL-13 can indirectly inhibit osteoclast formation by activating the STAT6 pathway to induce endothelial cells to secrete the osteoprotective hormone OPG (36).
IL-4 also has a synergistic effect with other cytokines, such as GM-CSF, in inhibiting osteoclastogenesis. In the presence of M-CSF and RANKL, monocytes differentiate into osteoclast. However in the presence of GM-CSF and IL-4, the combination of them upregulates TNF-a converting enzyme (TACE), causing M-CSF receptor shedding and monocytes are differentiating toward dendritic cells thereby disrupting osteoclastogenesis. (37).
Interleukin-6 family
Interleukin-6
The IL-6 family is a family of cytokines that share the signaling receptor subunit glycoprotein 130 kDa (gp130), including IL-6, IL-11, Oncostatin M (OSM), leukemia inhibitory factor (LIF), cardiotrophin 1 (CT-1), ciliary neurotrophic factor (CNTF), cardiotrophin-like cytokine factor 1 (CLCF1), neuropoietin (NP), IL-27, and humanin (138).
IL-6 is one of the pleiotropic cytokines that transmits signals by binding to the IL-6 receptor (IL-6R) (138). IL-6R is subdivided into the transmembrane receptor IL-6R and soluble IL-6R (sIL-6R), which exert biological effects via two distinct pathways including classic (cis) IL-6 signaling and alternative trans-signaling, respectively (139). IL-6 is produced by osteoblasts (140, 141), bone marrow stromal cells (BMSCs) (142), osteoclasts, macrophages (143), T cells (143), and neutrophils (144). Studies have indicated that IL-6 has been implicated in a number of age-related diseases, including osteoporosis, and its levels rise with age (145, 146), possibly through the P3IK/AKT, MAPK, and JAK/STAT pathways (147–149).
In the process of bone remodeling, IL-6 is primarily regarded as a pro-osteoclastic factor that promotes osteoclastic processes via direct and indirect pathways. Mouse models overexpressing IL-6 exhibit a phenotype characterized by an increase in osteoclasts and a reduction in bone trabecular volume (150). IL-6 directly stimulates osteoclast formation via a RANKL-independent mechanism, when OPG treatment does not inhibit this stimulatory effect (38), and therefore is regarded as a RANKL-independent stimulatory mechanism, although the precise mechanism was not revealed by the study. At the same time, IL-6 can indirectly stimulate osteoclastogenesis by stimulating RANKL produced by stromal and osteoblast cells (39–41) via JAK-mediated activation of STAT3 (42), resulting in an increase in the expression of osteoclast markers. Inhibition of IL-6R signaling inhibits osteoclast formation both in vitro and in vivo (151). A study showed that IL-6-deficient mice had increased bone mass, tartrate-resistant acid phosphatase (TRAP)-positive osteoclast numbers, and alkaline phosphatase activity in osteoblasts (152), indicating that IL-6 deficiency inhibited osteoclast maturation. In IL-6-deficient mice, osteoclast apoptosis was also increased (153). However, it has also been reported that IL-6 has a negative regulatory effect on osteoclast formation, and that IL-6 can act directly on osteoclast progenitors to inhibit their differentiation into osteoclasts by inhibiting the RANK signaling pathway-mediated degradation of IκB and activation of JNK (40). The differential regulation of osteoclasts by IL-6 may be attributable to the presence of varying concentrations of RANKL. IL-6/sIL-6R modulates NF-κB, ERK, and JNK signaling pathways differentially, inhibiting osteoclast formation at higher RANKL concentrations and promoting osteoclast formation at lower RANKL concentrations (43).
In addition, IL-6 contributes to the osteogenesis process. Compared with wild-type mice, IL-6 knockout mice after ovariectomy (OVX) demonstrated significant upregulation of mRNA for osteoblast-related genes, such as Runx2 and Col1a1, and downregulation of osteoclast-related genes, such as TRAP, MMP9, and CTSK (154). In a mouse model of osteoporosis, the inflammatory state of the microenvironment inhibits the osteogenic differentiation of BMSCs, and IL-6 is one of the most significant factors in this inhibition. The overactivated IL-6-STAT3 pathway inhibits β-catenin activity, and anti-IL-6 neutralizing antibodies rescue the osteoporotic phenotype in rodents (44), providing a potential therapeutic target for osteoporosis. In addition, the expression of TLR2, TLR4, IL-1, and TNF-α increases in BMSCs in response to IL-6, activating the AKT pathway and further inhibiting Setd7 expression. BMSCs, on the other hand, demonstrate a decrease in osteogenic gene expression and an increase in inflammatory gene expression (155). IL-6 inhibits osteoblast differentiation through activation of the JAK/STAT, SHP2/MEK2, and SHP2/AKT signaling pathways (45). In contrast to these findings, it has been hypothesized that the IL-6/IL-6R complex can activate the downstream STAT3 signaling pathway and promote osteogenic differentiation of bone marrow-derived mesenchymal stem cells (BM-MSCs) via an autocrine/paracrine feedback loop (156).
Interleukin-11
IL-11, also known as adipogenesis inhibitory factor (AGIF) (157), is primarily secreted by stromal cells and osteoblasts (158) and shares the co-receptor gp130 with IL-6 family members (138). Therefore, IL-11 plays a similar function to IL-6 during bone remodeling. IL-11 can not only directly stimulate osteoclast differentiation independent of RANKL (38) but can also stimulate osteoclast formation indirectly through inducing RANKL production in osteoblastic lineage cells (57). The difference is that IL-11R is expressed in the osteoblast lineage (158) and IL-11 extends the survival of osteoblast progenitor cells (58). IL-11 has pro-osteogenic effects in addition to its pro-osteoclastic effects. In vitro administration of IL-11 promotes osteoblast lineage differentiation from pluripotent progenitor cells (59). The phenotype of mice overexpressing IL-11 was characterized by increased bone formation, thickened bone cortex thickness, and enhanced bone strength (159). In contrast, mice lacking IL-11R exhibited impaired bone formation on the trabecular surface and increased adipose in the bone marrow, indicating that IL-11R signaling is essential for osteoblast differentiation (160). Various studies have shown that IL-11 also promoted the process of osteogenesis when stimulated by mechanical loading. IL-11 is upregulated to promote osteogenesis, inhibit adipogenesis (60), and suppress the expression of sclerostin (an inhibitor of osteoblast differentiation, an osteoclast gene sensitive to mechanical stress) in osteoblasts (46). This process may be mediated through the Wnt signaling pathway (161, 162) and ΔFosB (161, 163).
Oncostatin M
Oncostatin M (OSM), part of the IL-6 family, is expressed in all stages of osteoblast differentiation, including bone marrow stromal cells, stroma-producing osteoblasts, osteocytes, and bone-covered cells (46, 164). OSM expresses three receptor subunits, gp130, OSMR, and LIFR, in the osteoblast lineage, but not in osteoclasts (46). Additionally, bone macrophages can produce OSM (165–167) and bone morphogenetic proteins (BMPs) (168) to influence osteoblast differentiation. Depletion of bone macrophages can inhibit this differentiation process and is one of the mechanisms underlying diminished bone formation and bone growth in osteoporosis patients (169–171).
By stimulating RANKL transcription in stromal cells, OSM indirectly promotes osteoclast differentiation in vitro (41), but this effect is insufficient to completely support osteoclast formation in vitro (172). OSM can also promote the differentiation of stromal cells into osteoblasts rather than adipogenesis and inhibit sclerostin, an antagonist of Wnt pathway (46, 47). The two different effects mentioned above depend on the different receptors. OSM binds to gp130 first and forms a heterodimer with OSMR or LIFR, acting via LIFR to inhibit sclerostin production in stromal cell lines and osteoblasts, and acting via OSMR to stimulate RANKL production and osteoclast formation (46).
Interleukin-7
IL-7 is a member of the IL-2 family and is primarily secreted by stromal cells and osteoblasts in response to the inflammatory cytokines IL-1 or TNF-α (11). Mice that overexpress IL-7 have a phenotype characterized by decreased bone mass and increased osteoclasts (173), suggesting that IL-7 also plays a role in bone remodeling. IL-7 can indirectly promote osteoclast formation by inducing T lymphocytes to produce RANKL and TNF-α (48), whereas in nude mice lacking T lymphocytes, IL-7 failed to cause bone resorption and loss (48). Additionally, IL-7 is associated with the number of T lymphocytes. Studies have confirmed that the absence of estrogen after OVX resulted in elevated IL-7 levels, which stimulated thymus-dependent differentiation of bone marrow-derived progenitor cells and thymus-independent peripheral expansion of mature T cells, thereby upregulating T lymphocyte development and inducing bone loss (174). IL-7 secreted by osteoblasts is identified as a key cytokine for B lymphocyte differentiation, in addition to T lymphocytes (175), and is regulated in cells that overexpress osterix via the mechanistic target of rapamycin complex 1 (mTORC1) pathway (176, 177). Bone loss was caused by the IL-7-induced proliferation of B lymphocytes in wild-type rodents, while bone trabeculae and bone volume were substantially increased in IL-7R-deficient mice, indicating that the IL-7-induced increase in B lymphocyte production was also associated with bone resorption (49). Recent research suggests that IL-7/IL-7R may regulate the specific mechanisms of CTSK, NFATc1, and MMP9, as well as the phosphorylation of p38 and Akt, by activating the c-Fos/c-Jun pathway, thereby increasing the number of osteoclasts and amount of bone resorption in RANKL-stimulated macrophages (178). On the other hand, IL-7 can also directly stimulate osteoclast formation by activating STAT5 via a pathway independent of RANKL (50).
Nonetheless, other studies on IL-7 suggested a mechanism contrary to the above, and IL-7 may be a potential osteoclast formation inhibitor in vitro. One study found that IL-7 inhibited osteoclast formation in bone marrow cells from mice co-cultured with CSF-1 and RANKL (179), and another study found that IL-7-deficient mice had a significant increase in the number of osteoclasts and a significant decrease in bone trabecular bone mass compared with wild-type controls (180). The specific causes for the divergent conclusions should be investigated further.
Interleukin-8
IL-8 is a cytokine produced by osteoblasts that promotes RANKL-induced osteoclastogenesis in an autocrine manner, which can be inhibited by anti-IL-8 antibodies or IL-8 receptor inhibitors in vitro (51). Although human osteoclasts produce high levels of IL-8, the research on IL-8 is still lacking. One of the possible reasons is the lack of IL-8 equivalent in rodents, which makes it difficult to model human diseases. Therefore, finding an effective IL-8 equivalent in rodents may be the direction for future IL-8 research.
Interleukin-10
IL-10 is a type of Th2 cytokine. The levels of IL-10 were substantially lower in osteoporosis patients than in healthy individuals (181). However, IL-10 levels increased in osteoporosis patients following anti-osteoporosis treatment (182). Animal experiments in which the IL-10 expression was significantly reduced in an osteoporosis model of postmenopausal mice (183) and IL-10-deficient mice exhibited decreased bone mass, increased mechanical fragility, and inhibited bone formation (184), indicating a correlation between IL-10 levels and the development of osteoporosis.
IL-10 is produced by activated T and B lymphocytes (185) and is a direct inhibitor of both osteoclast and osteoblast formation through the RANK/RANKL/OPG axis.
IL-10 inhibits the early phases of osteoclast progenitor cell differentiation into osteoclast precursors during osteoclast differentiation (52). Specifically, IL-10 inhibits osteoclast differentiation by increasing OPG to inhibit RANKL expression and by decreasing RNAK and M-CSF expression (53). IL-10 inhibits RANK-induced osteoclast formation and inhibits calcium signaling, downstream of RANK, via TREM-2 transcriptional inhibition (54). IL-10 also inhibits NFATc1 expression and nuclear translocation by inhibiting c-Fos and c-Jun, thereby inhibiting osteoclastogenesis (55). In OVX mice with osteoporosis, the number of regulatory B (B10) cells that produce IL-10 decreased and the number of IL-17-producing Th17 cells increased compared with control mice. However, the transplantation of B10 cells reduced the number of Th17 cells and inhibited the development of osteoporosis (186), suggesting that B10 cell therapy for osteoporosis may be feasible.
For osteogenic differentiation, IL-10 inhibits bone marrow osteogenic activity by preventing bone mineralization in mouse bone marrow cells and inhibiting the synthesis of bone proteins, including alkaline phosphatase (ALP), type I collagen, and osteocalcin (56).
Interleukin-12
IL-12 is an anti-osteoclast factor that inhibits RANKL-induced osteoclastogenesis through the suppression of NFATc1 (61) or the promotion of osteoblast apoptosis via the Fas/FasL pathway (62). IL-12 also has a synergistic effect with IL-18 in the mechanism of osteoclast apoptosis induction, as will be discussed in the following section. There are not many studies on IL-12 in osteoporosis, and further exploration is still needed.
Interleukin-13
IL-13 is a Th2 anti-osteoclast cytokine which is analogous to IL-4. IL-4 and IL-13 share a specific endothelial cell surface IL-4/IL-13-receptor complex so they have common biological effects and similar downstream intracellular signaling pathways. IL-13 inhibits osteoclast formation by activating STAT6 in endothelial cells to induce OPG expression (36). By inhibiting osteoblast cyclooxygenase-2 (COX-2)-dependent prostaglandin synthesis, IL-13 and IL-4 can also inhibit bone resorption (63).
Interleukin-15
IL-15, similar to IL-7, is a member of the IL-2 super family and shares many mechanisms of action with IL-2. However, IL-2 cannot be substituted for its function in promoting the differentiation of osteoclast progenitors into osteoclast precursors (64). Additionally, IL-15 also has an indirect stimulatory effect on osteoclast formation, operating synergistically with RANKL to induce osteoclast formation primarily via activating extracellular signal-regulated kinase (ERK) to mediate this synergistic effect (65). In a co-culture environment with mouse bone marrow cells and osteoblasts, IL-15 treatment also increased caspase3 expression in NK cells in a dose-dependent manner, thereby promoting osteoblast apoptosis (66).
Interleukin-17/interleukin-25
Interleukin-17 (IL-17), a cytokine secreted by Th17 cells, is closely associated with osteoclastic effects given that Th17 cells represent a significant subpopulation of osteoclasts. In the bone marrow cells of estrogen-deficient OVX mice, both the number of Th17 cells and the level of circulating IL-17 were elevated, and increased IL-17 levels promoted the expression of pro-osteoclastic cytokines such as TNF-α, IL-6, and RNAKL in osteoblasts, thereby inducing bone loss (67). Estrogen or anti-IL-17 treatments can alleviate the symptoms of bone loss (67, 68). Administration of an anti-IL-17 neutralizing antibody can promote the regeneration of new bone in osteoporotic fractures by enhancing the activity of FOXO1 and ATF4, enhancing the expression of osteogenic markers, and decreasing the oxidative stress in the injured fraction (69). The fact that Th17 cells and IL-17 levels are associated with bone loss has also been verified in humans, where both Th17 cell frequency and IL-17 levels were higher in postmenopausal women than in premenopausal women (70). The result also correlates with reduced bone mineral density (70). IL-17 not only induces osteoclasts by elevating the expression of osteoclastic cytokines RANKL (71), TNF-α, IL-1, IL-6, and IL-8 (72, 73), but also promotes autophagy of osteoclast precursors and osteoclast formation via JNK signaling in a low dose-dependent manner (74).
However, it appears that the function of IL-17 changes with increasing concentrations. Other studies have demonstrated that high concentrations of IL-17 inhibited the differentiation of osteoclast precursors into osteoclasts (75). High concentrations of IL-17 inhibited matrix protein hydrolysis during bone resorption by downregulating the expression of histone K and MMP-9 in osteoclasts (75). In addition, IL-17 can induce proliferation, migration, motility, and osteoblast differentiation of human bone marrow-derived mesenchymal stem cells (hMSCs) in a manner dependent on ROS and MEK/ERK (76). At the same time, IL-17 induces the expression of M-CSF and RANKL on hMSCs to support the in vitro and in vivo osteoclast formation process (76).
In addition to the well-researched effects on the osteoclastic process, the role of IL-17 in osteoblast differentiation has also been examined. IL-17 has a positive effect on the early differentiation of primary osteoblasts, as well as an inhibitory effect on osteoblast calcification (77), and it inhibits osteoblast differentiation and bone regeneration processes in rodents (78). In vitro, high concentrations of IL-17 also induce osteoblast searing via the NLRP3 inflammatory vesicle pathway, triggering the release of IL-1 and RANKL and disrupting bone metabolism even further (79).
Interleukin-18
IL-18, also known as interferon-gamma-inducing factor (IGIF), is a pleiotropic pro-inflammatory cytokine with similar functions to IL-12 (187). In the case of TNF-α-induced Fas, analogous to IL-12, IL-18 can inhibit TNF-α-induced osteoclastogenesis by mediating myeloid apoptosis via Fas/FasL (80, 81). In contrast, another study demonstrated that anti-FasL antibodies were unable to completely inhibit apoptosis induced by the pathway described above (82). In the presence of TNF-α, IL-12 and IL-18 induce nitric oxide (NO) production in a synergistic manner, which also leads to apoptosis (82). Additionally, IL-18 induces the production of IFN-γ and GM-CSF in T cells, inhibiting osteoclast formation indirectly (83, 84).
IL-18 binding protein (IL-18BP) is an antagonist of IL-18 with anti-inflammatory properties. The treatment of OVX mice with IL-18BP prevented bone loss, and in women with osteoporosis, IL-18BP levels decreased while serum IL-18 levels increased (188), suggesting that IL-18BP may be used to treat postmenopausal osteoporosis.
Interleukin-19
Interleukin-19 (IL-19) is an inhibiting cytokine that belongs to the IL-10 family. By inhibiting NF-κB and p38MAPK activation and c-Fos expression, IL-19 inhibits RANKL-induced osteoclast differentiation. IL-19 also maintains the osteoclast precursor state, including monocytes and macrophages in an autocrine manner (85). In addition, IL-19 can promote the release of other pro-inflammatory cytokines such as TNF-α, IL-1β, and IL-6 while upregulating RANKL expression in synovial fibroblasts, which further promote the osteoclasts differentiation in arthritis (189). However, the research on IL-19 in osteoporosis is still needed to find more cytokines interactions and therapeutic targets.
Interleukin-20
IL-20, a member of the IL-10 family, has been shown to have higher serum concentrations in osteoporotic patients than in healthy control patients, and to be substantially upregulated in the serum of OVX mice (86). Anti-IL-20 monoclonal antibody treatments can inhibit M-CSF and RANKL-induced osteoclast differentiation in vitro (86) and may represent a potential therapy for preventing osteoporotic bone loss. In particular, IL-20 induces the expression of RANK in M-CSF-derived osteoclast precursors and promotes the transduction of osteoclastic signals such as NF-κB, TRAF6, STAT3, NFATc1, and c-Fos (86). IL-20 also induces the expression of cathepsin G in osteoclasts, thereby increasing the level of soluble RANKL (86). IL-20 can also upregulate RANKL expression in osteoblasts via an autocrine mechanism (86). Another study demonstrated that IL-20 inhibited the survival and differentiation of osteoblasts by upregulating sclerostin and downregulating osterix, RUNX2, and OPG (87).
Interleukin-23
IL-23, a member of the IL-6/IL-12 family, can participate in T-cell-mediated osteoclast formation by inducing the differentiation of naive CD4(+) T cells into Th17 cells, which secrete IL-17 for further action (88). Therefore, it is called the IL-23/IL-17 axis. In a lipopolysaccharide-induced model of inflammatory bone destruction, mice deficient in IL-17 or IL-23 exhibited significantly less bone loss (88). In addition, IL-23 has a pathway independent of IL-17 that modulates osteoclast differentiation by upregulating RANK expression in bone marrow precursor cells (89) and RANKL expression in CD4(+) T cells (90). However, the role of IL-23 on bone in vivo is also controversial, as one study discovered that IL-23 indirectly inhibited osteoclast formation in vitro in a CD4(+) T lymphocyte-dependent and dose-dependent manner (91). IL-23 can also increase bone mass in long bones by limiting resorption of immature bone formation below the growth plate (91).
Interleukin-27
IL-27, also a member of the IL-6/IL-12 family, functions via the IL-27R (IL-27R/WSX-1) and gp130 complexes. IL-27 is also a potent anti-osteoclastogenic factor that inhibits RANK downstream MAPK and NF-κB signaling pathways to eliminate RANKL-induced c-Jun and NFATc1 expressions (92). In osteoclast precursors, IL-27 downregulates TREM-2 co-stimulatory receptor expression and thereby inhibits NFATc1 action (92). In addition, IL-27 can inhibit osteoclast formation by STAT1-dependently downregulating the transcription factor c-Fos (93). In addition, another study discovered that IL-27 inhibited the secretion of RANKL and sRANKL via STAT3 in the surface of CD4(+) T cells (94). In recent years, a new study determined that IL-27 affected both osteoblasts and osteoclasts through early growth response-2 (Egr-2) and that IL-27 treatment in OVX mice led to the loss of bone trabecular structures and the preservation of cortical bone parameters (95). The reason for this is that IL-27 inhibits the differentiation of Th17 cells via the suppression of the transcription factor RORγt, activates Egr to induce IL-10-producing Tr1 cells, and inhibits osteoblast apoptosis by inducing anti-apoptotic factors such as MCL-1 via Egr-2 (95). IL-27 also inhibits osteoclastogenesis in an Egr2-dependent mechanism. It upregulates the expression of the RNAKL repressor Id2, which was also demonstrated in female patients with osteoporosis whose serum IL-27 levels were reduced along with decreased Egr2 expression (95), suggesting a potential new anti-osteoporosis treatment strategy.
Interleukin-29
IL-29, also known as interferonλ1 (IFNλ1), is a member of the IFN family along with IL-28A and IL-28B, which shares the same receptor complex (IL-28R1/IL-10R2), activates the downstream JAK-STAT signaling pathway upon binding, and transcribes numerous IFN-related genes (190). In vitro and in vivo, IL-29 derived from dendritic cells inhibits osteoclast formation and bone resorption activity (96). IL-29 inhibits RANKL-induced osteoclast formation by activating the STAT signaling pathway, blocking NF-κB activation and NFATc1 translocation, and repressing osteoclast gene expression (97).
Interleukin-32
IL-32 belongs to a class of inflammatory cytokines that elicits a variety of other cytokines, of which IL-32γ is one isoform. Age-related increases in bone formation and osteogenic capacity were observed in mice that overexpress IL-32γ (98). They were protected from OVX-induced osteoporosis more than wild-type mice, which may be mediated by the upregulation of miR-29a (98). There is also a correlation between reduced plasma IL-32γ levels and BMD in humans (98), suggesting a protective mechanism for IL-32 against bone loss.
Interleukin-33
IL-33, a member of the IL-1 family with the specific receptor orphan IL-1 receptor ST2 (IL-1R-like 1), is an osteoprotective factor that inhibits osteoclast formation by at least three mechanisms. Postmenopausal women with osteoporosis had substantially lower IL-33 levels than healthy control women (191). IL-33 interacts with its specific receptor ST2 and inhibits RANKL-induced NFATc1 expressions and nuclear translocations by regulating the expression of Blimp-1 and interferon regulatory factor-8 (IRF-8), thereby suppressing RANKL-induced osteoclast formation and osteoblast-related gene expression (99, 100). According to another study, IL-33 induced osteoclasts apoptosis by increasing the expression of pro-apoptotic molecules such as Bcl-2-associated X protein (BAX), Fas, FasL, and Fas-associated death structural domains (101). In the bone marrow culture state, IL-33 induces mRNA expression of GM-CSF, IL-4, IL-13, and IL-10 to inhibit osteoclast formation (192). The IL-33/ST2 signaling pathway on the aforementioned anti-osteoclast production, which is also associated with vitamin D (100). IL-33 inhibited TNF-induced osteoclast formation and bone resorption, as shown in a mouse model in which mice that were overexpressing TNF-α and treated with IL-33 exhibited a significant reduction in bone loss (102). Osteoprotective IL-33 induces osteoclast precursors to differentiate into CD206(+) alternatively activated macrophages (AAM) rather than osteoclasts in an autocrine manner via GM-CSF (102). In another study, mice lacking the ST2 receptor displayed typical bone formation, but increased bone resorption and decreased bone trabecular bone mass (193). Additionally, IL-33 stimulates osteoblast function, promotes matrix mineral deposition, and reduces sclerostin mRNA levels in primary osteoblasts treated with ascorbate for an extended period of time (103).
Interleukin-34
M-CSF, an essential cytokine for osteoclast formation, binds to the receptor c-Fms (CSF-1R) to exert its biological effects. Interleukin-34 (IL-34), a second ligand that can bind to c-Fms, can replace the function of M-CSF and is another crucial factor in osteoclast formation. In combination with RANKL, it can induce osteoclast differentiation and bone resorption, and systemic administration of IL-34 to mice decreased trabecular bone mass (104). Another study found that IL-34 promoted the proliferation and differentiation of bone marrow macrophages toward osteoclasts by increasing the expression of NFATc1, stimulating the expression of p-STAT3, and inhibiting the expression of Smad7 without M-CSF (105). In addition, a recent study discovered that low-dose IL-34 regulated hBMSCs osteogenesis and enhanced fracture healing in part via the PIK/AKT and ERK signaling pathways but had no effect on osteoclast formation in vitro or osteoporosis in vivo (106).
Interleukin-35
IL-35, belonging to the IL-12 family, is a novel class of anti-inflammatory and immunosuppressive factors. In bone immunology, IL-35 is a direct inhibitor of osteoclast formation, preventing TNF-induced osteoclast formation and bone resorption in vitro and inhibiting osteolysis in vivo (107). Specifically, IL-35 inhibits NFATc1, c-Fos, and TRAP through the NF-κB and MAPK pathways, which also inhibits TNF-induced osteoclast formation and promotes apoptosis through JAK1/STAT1 activation (107).
Another imbalance in osteoporosis is the imbalance between bone and adipogenesis (194). MSCs have the ability to differentiate into osteoblasts and adipocytes at the same time, which is affected by cytokines and hormone regulation in the microenvironment (195). IL-35 stimulates the proliferation of MSCs while inhibiting their apoptosis or differentiation toward adipogenic. Specifically, it increases the expression of β-catenin and Axin2, which are essential factors in the differentiation of MSCs into osteoblasts in Wnt/β-catenin-PPARγ pathway (108). IL-35 controls the equilibrium between osteogenic and lipogenic differentiation of progenitor cells, suggesting its potential application in osteoporosis and obesity intervention.
IL-35 can also be involved in RANKL and M-CSF-induced osteoclasts and angiogenesis via the Th17/IL-17 axis, exhibiting inhibitory effects in both metabolic processes commonly associated with osteoporosis (196), thereby indicating a potential therapeutic direction.
However, it has also been discovered that IL-33 stimulated the phosphorylation of relevant signaling molecules such as Syk, phospholipase Cγ2, Gab2, MAP kinases, TAK-1, and NF-κB in human CD14(+) monocytes via ST2, thereby promoting functional osteoclast formation, and also increased the expression of various osteoclast differentiation factors including TRAF6, NFATc1, c-Fos, C-Src, histone K and calcitonin receptor, and ultimately also induced bone resorption (109).
Interleukin-37
IL-37, a member of the IL-1 family, has received relatively less attention than the above cytokines. Nonetheless, its roles in inhibiting osteoclast activity and in bone resorption have been identified. IL-37 inhibits osteoclast formation and pathological bone resorption induced by lipopolysaccharide (110). IL-37 also plays a significant function in the osteogenic differentiation of MSCs, significantly increasing the expression of osteoblast specific genes to accelerate bone healing in a rat skull defect model via PI3K/AKT activation, but the specific mechanism needs further research (111).
Interferon
IFN are categorized as IFN-α, IFN-β, and IFN-γ, and each has a distinct function in bone remodeling.
IFN-α and IFN-β have inhibitory effects on RANKL-induced osteoclast formations, which can inhibit RANKL-induced osteoclastogenesis by reducing c-Fos (112, 113). A study also showed that IFN-β inhibited osteoclastogenesis by increasing NO production and the inducible nitric oxide synthase (iNOS) signaling pathway (114).
IFN-γ acts on both osteoblasts and osteoclasts (197), with osteoblasts producing modest levels of IFN-γ to stimulate the expression of osteoblast differentiation genes such as Runx2, osterix, ALP, and osteocalcin, further leading to osteoblast differentiation (115, 116). The knockdown of the IFN-γ receptor simultaneously inhibits this effect (117). IFN-γ can downregulate c-fms expression and thus counteract the effect of M-CSF on osteoclast precursors (118), as well as inhibit NFATc1 expression by stimulating TRAF6 degradation through the ubiquitin/proteasome system, which in turn inhibits downstream JNK and NF-κB (8, 197). IFN-γ can also mediate osteoclast apoptosis through the Fas/FasL signaling pathway (119). The above three pathways can inhibit the differentiation and function of osteoclast. IFN-γ also has a positive effect on osteoclastogenesis in late differentiation. It can stimulate osteoclast fusion by the expression of dendritic cell-specific transmembrane protein (DC-STAMP) through the upregulation of NFATc1 and c-Fos (120) and can promote osteoclastogenesis by CXCL10/Interferon-gamma induced protein 10 (IP-10) secretion by macrophages to stimulate RANKL and TNF-α secretion by T cells (121).
Chemokine
Numerous studies have demonstrated that chemokines influence the differentiation and function of osteoblasts and osteoclasts, regulating bone formation and resorption via autocrine or paracrine mechanisms. The current status of research on the role of various chemokines in bone remodeling has been systematically reported in this article (198). Among these chemokines, CCL2, CCL3, and CCL20 are the most researched types and we will focus on these three chemokines. CCL2 and CCL3 function as pro-osteoclastic cytokines, stimulating the osteoclastogenesis (198). In osteoporosis, CCL2 binding to its receptor C-C chemokine receptor-2 (CCR2) and then activates NF-κB and ERK1/2 signaling, which further lead to increase of RANK expression and RANKL-induced osteoclastogenesis (199). Patients with postmenopausal osteoporosis showed a significant increase in serum CCL3 compared with other groups, indicating that CCL3 may be a potential biomarker to predict disease severity of postmenopausal osteoporosis (200). CCL20 can not only stimulate the osteoclastogenesis but can also act on osteoblast differentiation (198). Other chemokines are not described in detail here.
Conclusion
The cytokines network plays a critical role in maintaining the balance of bone resorption and formation between osteoclasts and osteoblasts. Dysregulation of cytokines may result in bone diseases such as osteoporosis. Among these cytokines, TNF-α, IL-1, IL-6, IL-7, IL-8, IL-11, IL-15, IL-17, and IL-20 belong to osteoclastogenic cytokines, mainly promoting osteoclastogenesis. Meanwhile, anti-inflammatory cytokines, including IL-3, IL-4, IL-10, IL-13, IL-18, IL-19, IL-27, IL-29, IL-32, IL-33, IL-37, and IFN, function as anti-osteoclastogenic cytokines inhibiting the process of osteoclastogenesis (Figure 2). Although there has been a great deal of research focus on the effect of proinflammatory cytokines in the bone remodeling of osteoporosis, the cross talk between bone and immune system remains complex, leading to the difficulty of transformation into clinical practice. However, owing to the effort made by many scientists, some molecular-targeted drugs are in clinical trials and have achieved certain results. For example, benzydamine, a non-steroidal anti-inflammatory drug, can inhibit osteoclast differentiation and bone resorption through downregulating the expression of IL-1β (201). Oral administration of lactulose could downregulate pro-osteoclastogenic cytokines levels including TNF-α, IL-6, RANKL, and IL-17 as well as upregulate the anti-inflammatory cytokine IL-10 in OVX mice, which ameliorated estrogen deficiency-induced bone loss in these mice (202). In this review, we discussed the concrete effects of various cytokines in osteoblasts and osteoclasts during bone remodeling in osteoporosis, summarizing the current research and providing multiple therapeutic targets for further study. Therefore, we hope our review is also helpful in the development of osteoporosis research and treatment.
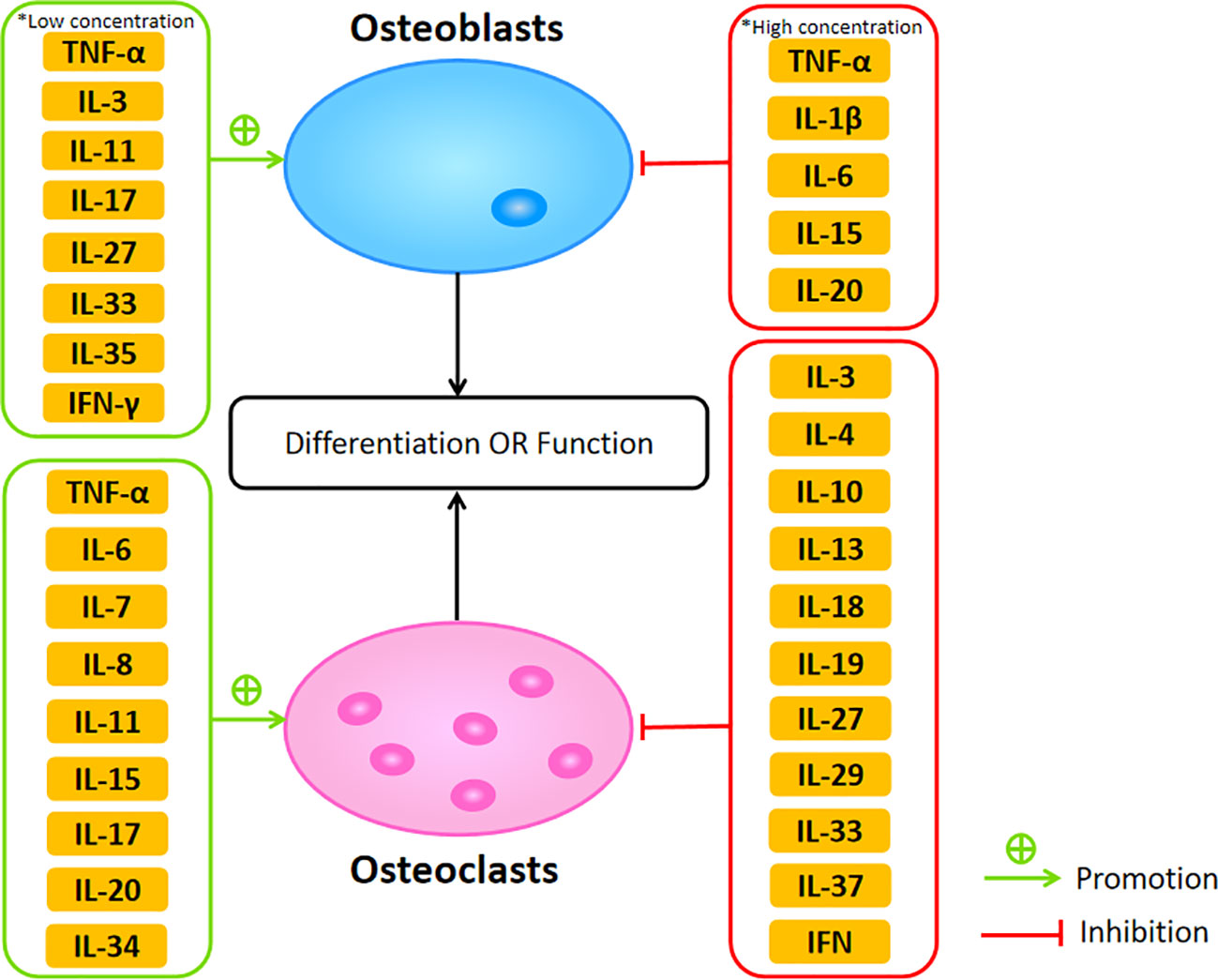
Figure 2 The promotive and suppressive effects of different cytokines on osteoblasts and osteoclasts affecting their differentiation or function.
Author contributions
JX and LY drafted and wrote the manuscript. LW revised the manuscript. FL reviewed and edited the manuscript. ZD assisted in reviewing materials and organizing manuscripts. All authors contributed to the article and approved the submitted version.
Conflict of interest
The authors declare that the research was conducted in the absence of any commercial or financial relationships that could be construed as a potential conflict of interest.
Publisher’s note
All claims expressed in this article are solely those of the authors and do not necessarily represent those of their affiliated organizations, or those of the publisher, the editors and the reviewers. Any product that may be evaluated in this article, or claim that may be made by its manufacturer, is not guaranteed or endorsed by the publisher.
References
1. Bijlsma AY, Meskers CG, Westendorp RG, Maier AB. Chronology of age-related disease definitions: osteoporosis and sarcopenia. Ageing Res Rev (2012) 11(2):320–4. doi: 10.1016/j.arr.2012.01.001
2. Seriolo B, Paolino S, Casabella A, Botticella G, Seriolo C, Molfetta L. Osteoporosis in the elderly. Aging Clin Exp Res (2013) 25 Suppl 1:S27–9. doi: 10.1007/s40520-013-0107-9
3. Sarafrazi N, Wambogo EA, Shepherd JA. Osteoporosis or low bone mass in older adults: United States, 2017-2018. NCHS Data Brief (2021) 405):1–8.
4. Si L, Winzenberg TM, Jiang Q, Chen M, Palmer AJ. Projection of osteoporosis-related fractures and costs in China: 2010-2050. Osteoporos Int (2015) 26(7):1929–37. doi: 10.1007/s00198-015-3093-2
5. Noh JY, Yang Y, Jung H. Molecular mechanisms and emerging therapeutics for osteoporosis. Int J Mol Sci (2020) 21(20):7623. doi: 10.3390/ijms21207623
6. Okamoto K, Nakashima T, Shinohara M, Negishi-Koga T, Komatsu N, Terashima A, et al. Osteoimmunology: the conceptual framework unifying the immune and skeletal systems. Physiol Rev (2017) 97(4):1295–349. doi: 10.1152/physrev.00036.2016
7. Theoleyre S, Wittrant Y, Tat SK, Fortun Y, Redini F, Heymann D. The molecular triad OPG/RANK/RANKL: involvement in the orchestration of pathophysiological bone remodeling. Cytokine Growth Factor Rev (2004) 15(6):457–75. doi: 10.1016/j.cytogfr.2004.06.004
8. Amarasekara DS, Yun H, Kim S, Lee N, Kim H, Rho J. Regulation of osteoclast differentiation by cytokine networks. Immune Netw (2018) 18(1):e8. doi: 10.4110/in.2018.18.e8
9. Kitaura H, Kimura K, Ishida M, Kohara H, Yoshimatsu M, Takano-Yamamoto T. Immunological reaction in TNF-α-mediated osteoclast formation and bone resorption in vitro and in vivo. Clin Dev Immunol (2013) 2013:181849. doi: 10.1155/2013/181849
10. Kitaura H, Zhou P, Kim HJ, Novack DV, Ross FP, Teitelbaum SL. M-CSF mediates TNF-induced inflammatory osteolysis. J Clin Invest (2005) 115(12):3418–27. doi: 10.1172/JCI26132
11. Weitzmann MN, Cenci S, Rifas L, Brown C, Pacifici R. Interleukin-7 stimulates osteoclast formation by up-regulating the T-cell production of soluble osteoclastogenic cytokines. Blood (2000) 96(5):1873–8.
12. Zha L, He L, Liang Y, Qin H, Yu B, Chang L, et al. TNF-α contributes to postmenopausal osteoporosis by synergistically promoting RANKL-induced osteoclast formation. BioMed Pharmacother (2018) 102:369–74. doi: 10.1016/j.biopha.2018.03.080
13. Osta B, Benedetti G, Miossec P. Classical and paradoxical effects of TNF-α on bone homeostasis. Front Immunol (2014) 5:48. doi: 10.3389/fimmu.2014.00048
14. Gilbert L, He X, Farmer P, Boden S, Kozlowski M, Rubin J, et al. Inhibition of osteoblast differentiation by tumor necrosis factor-alpha. Endocrinology (2000) 141(11):3956–64. doi: 10.1210/endo.141.11.7739
15. Gilbert L, He X, Farmer P, Rubin J, Drissi H, van Wijnen AJ, et al. Expression of the osteoblast differentiation factor RUNX2 (Cbfa1/AML3/Pebp2alpha a) is inhibited by tumor necrosis factor-alpha. J Biol Chem (2002) 277(4):2695–701. doi: 10.1074/jbc.M106339200
16. Tanabe N, Maeno M, Suzuki N, Fujisaki K, Tanaka H, Ogiso B, et al. IL-1 alpha stimulates the formation of osteoclast-like cells by increasing m-CSF and PGE2 production and decreasing OPG production by osteoblasts. Life Sci (2005) 77(6):615–26. doi: 10.1016/j.lfs.2004.10.079
17. Polzer K, Joosten L, Gasser J, Distler JH, Ruiz G, Baum W, et al. Interleukin-1 is essential for systemic inflammatory bone loss. Ann Rheum Dis (2010) 69(1):284–90. doi: 10.1136/ard.2008.104786
18. Nakamura I, Jimi E. Regulation of osteoclast differentiation and function by interleukin-1. Vitam Horm. (2006) 74:357–70. doi: 10.1016/S0083-6729(06)74015-8
19. Lee SK, Gardner AE, Kalinowski JF, Jastrzebski SL, Lorenzo JA. RANKL-stimulated osteoclast-like cell formation in vitro is partially dependent on endogenous interleukin-1 production. Bone (2006) 38(5):678–85. doi: 10.1016/j.bone.2005.10.011
20. Wei S, Kitaura H, Zhou P, Ross FP, Teitelbaum SL. IL-1 mediates TNF-induced osteoclastogenesis. J Clin Invest (2005) 115(2):282–90. doi: 10.1172/JCI23394
21. Kumar S, Votta BJ, Rieman DJ, Badger AM, Gowen M, Lee JC. IL-1- and TNF-induced bone resorption is mediated by p38 mitogen activated protein kinase. J Cell Physiol (2001) 187(3):294–303. doi: 10.1002/jcp.1082
22. Hengartner NE, Fiedler J, Ignatius A, Brenner RE. IL-1β inhibits human osteoblast migration. Mol Med (2013) 19(1):36–42. doi: 10.2119/molmed.2012.00058
23. Khapli SM, Mangashetti LS, Yogesha SD, Wani MR. IL-3 acts directly on osteoclast precursors and irreversibly inhibits receptor activator of NF-kappa B ligand-induced osteoclast differentiation by diverting the cells to macrophage lineage. J Immunol (2003) 171(1):142–51. doi: 10.4049/jimmunol.171.1.142
24. Yogesha SD, Khapli SM, Wani MR. Interleukin-3 and granulocyte-macrophage colony-stimulating factor inhibits tumor necrosis factor (TNF)-alpha-induced osteoclast differentiation by down-regulation of expression of TNF receptors 1 and 2. J Biol Chem (2005) 280(12):11759–69. doi: 10.1074/jbc.M410828200
25. Yogesha SD, Khapli SM, Srivastava RK, Mangaszhetti LS, Pote ST, Mishra GC, et al. IL-3 inhibits TNF-alpha-induced bone resorption and prevents inflammatory arthritis. J Immunol (2009) 182(1):361–70. doi: 10.4049/jimmunol.182.1.361
26. Oh J, Lee MS, Yeon JT, Choi SW, Kim HS, Shim H, et al. Inhibitory regulation of osteoclast differentiation by interleukin-3 via regulation of c-Fos and id protein expression. J Cell Physiol (2012) 227(5):1851–60. doi: 10.1002/jcp.22913
27. Gupta N, Barhanpurkar AP, Tomar GB, Srivastava RK, Kour S, Pote ST, et al. IL-3 inhibits human osteoclastogenesis and bone resorption through downregulation of c-Fms and diverts the cells to dendritic cell lineage. J Immunol (2010) 185(4):2261–72. doi: 10.4049/jimmunol.1000015
28. Lee J, Seong S, Kim JH, Kim K, Kim I, Jeong BC, et al. STAT5 is a key transcription factor for IL-3-mediated inhibition of RANKL-induced osteoclastogenesis. Sci Rep (2016) 6:30977. doi: 10.1038/srep30977
29. Barhanpurkar AP, Gupta N, Srivastava RK, Tomar GB, Naik SP, Joshi SR, et al. IL-3 promotes osteoblast differentiation and bone formation in human mesenchymal stem cells. Biochem Biophys Res Commun (2012) 418(4):669–75. doi: 10.1016/j.bbrc.2012.01.074
30. Abu-Amer Y. IL-4 abrogates osteoclastogenesis through STAT6-dependent inhibition of NF-kappaB. J Clin Invest (2001) 107(11):1375–85. doi: 10.1172/JCI10530
31. Wei S, Wang MW, Teitelbaum SL, Ross FP. Interleukin-4 reversibly inhibits osteoclastogenesis via inhibition of NF-kappa B and mitogen-activated protein kinase signaling. J Biol Chem (2002) 277(8):6622–30. doi: 10.1074/jbc.M104957200
32. Cheng J, Liu J, Shi Z, Xu D, Luo S, Siegal GP, et al. Interleukin-4 inhibits RANKL-induced NFATc1 expression via STAT6: a novel mechanism mediating its blockade of osteoclastogenesis. J Cell Biochem (2011) 112(11):3385–92. doi: 10.1002/jcb.23269
33. Moreno JL, Kaczmarek M, Keegan AD, Tondravi M. IL-4 suppresses osteoclast development and mature osteoclast function by a STAT6-dependent mechanism: irreversible inhibition of the differentiation program activated by RANKL. Blood (2003) 102(3):1078–86. doi: 10.1182/blood-2002-11-3437
34. Mangashetti LS, Khapli SM, Wani MR. IL-4 inhibits bone-resorbing activity of mature osteoclasts by affecting NF-kappa B and Ca2+ signaling. J Immunol (2005) 175(2):917–25. doi: 10.4049/jimmunol.175.2.917
35. te Velde AA, Huijbens RJ, Heije K, de Vries JE, Figdor CG. Interleukin-4 (IL-4) inhibits secretion of IL-1 beta, tumor necrosis factor alpha, and IL-6 by human monocytes. Blood (1990) 76(7):1392–7.
36. Stein NC, Kreutzmann C, Zimmermann SP, Niebergall U, Hellmeyer L, Goettsch C, et al. Interleukin-4 and interleukin-13 stimulate the osteoclast inhibitor osteoprotegerin by human endothelial cells through the STAT6 pathway. J Bone Miner Res (2008) 23(5):750–8. doi: 10.1359/jbmr.080203
37. Hiasa M, Abe M, Nakano A, Oda A, Amou H, Kido S, et al. GM-CSF and IL-4 induce dendritic cell differentiation and disrupt osteoclastogenesis through m-CSF receptor shedding by up-regulation of TNF-alpha converting enzyme (TACE). Blood (2009) 114(20):4517–26. doi: 10.1182/blood-2009-04-215020
38. Kudo O, Sabokbar A, Pocock A, Itonaga I, Fujikawa Y, Athanasou NA. Interleukin-6 and interleukin-11 support human osteoclast formation by a RANKL-independent mechanism. Bone (2003) 32(1):1–7. doi: 10.1016/s8756-3282(02)00915-8
39. Girasole G, Passeri G, Jilka RL, Manolagas SC. Interleukin-11: a new cytokine critical for osteoclast development. J Clin Invest (1994) 93(4):1516–24. doi: 10.1172/JCI117130
40. Yoshitake F, Itoh S, Narita H, Ishihara K, Ebisu S. Interleukin-6 directly inhibits osteoclast differentiation by suppressing receptor activator of NF-kappaB signaling pathways. J Biol Chem (2008) 283(17):11535–40. doi: 10.1074/jbc.M607999200
41. Palmqvist P, Persson E, Conaway HH, Lerner UH. IL-6, leukemia inhibitory factor, and oncostatin m stimulate bone resorption and regulate the expression of receptor activator of NF-kappa b ligand, osteoprotegerin, and receptor activator of NF-kappa b in mouse calvariae. J Immunol (2002) 169(6):3353–62. doi: 10.4049/jimmunol.169.6.3353
42. O'Brien CA, Gubrij I, Lin SC, Saylors RL, Manolagas SC. STAT3 activation in stromal/osteoblastic cells is required for induction of the receptor activator of NF-kappaB ligand and stimulation of osteoclastogenesis by gp130-utilizing cytokines or interleukin-1 but not 1,25-dihydroxyvitamin D3 or parathyroid hormone. J Biol Chem (1999) 274(27):19301–8. doi: 10.1074/jbc.274.27.19301
43. Feng W, Liu H, Luo T, Liu D, Du J, Sun J, et al. Combination of IL-6 and sIL-6R differentially regulate varying levels of RANKL-induced osteoclastogenesis through NF-κB, ERK and JNK signaling pathways. Sci Rep (2017) 7:41411. doi: 10.1038/srep41411
44. Li X, Zhou ZY, Zhang YY, Yang HL. IL-6 contributes to the defective osteogenesis of bone marrow stromal cells from the vertebral body of the glucocorticoid-induced osteoporotic mouse. PLoS One (2016) 11(4):e0154677. doi: 10.1371/journal.pone.0154677
45. Kaneshiro S, Ebina K, Shi K, Higuchi C, Hirao M, Okamoto M, et al. IL-6 negatively regulates osteoblast differentiation through the SHP2/MEK2 and SHP2/Akt2 pathways in vitro. J Bone Miner Metab (2014) 32(4):378–92. doi: 10.1007/s00774-013-0514-1
46. Walker EC, McGregor NE, Poulton IJ, Solano M, Pompolo S, Fernandes TJ, et al. Oncostatin m promotes bone formation independently of resorption when signaling through leukemia inhibitory factor receptor in mice. J Clin Invest (2010) 120(2):582–92. doi: 10.1172/JCI40568
47. Johnson RW, Brennan HJ, Vrahnas C, Poulton IJ, McGregor NE, Standal T, et al. The primary function of gp130 signaling in osteoblasts is to maintain bone formation and strength, rather than promote osteoclast formation. J Bone Miner Res (2014) 29(6):1492–505. doi: 10.1002/jbmr.2159
48. Toraldo G, Roggia C, Qian WP, Pacifici R, Weitzmann MN. IL-7 induces bone loss in vivo by induction of receptor activator of nuclear factor kappa b ligand and tumor necrosis factor alpha from T cells. Proc Natl Acad Sci U S A (2003) 100(1):125–30. doi: 10.1073/pnas.0136772100
49. Miyaura C, Onoe Y, Inada M, Maki K, Ikuta K, Ito M, et al. Increased B-lymphopoiesis by interleukin 7 induces bone loss in mice with intact ovarian function: similarity to estrogen deficiency. Proc Natl Acad Sci U S A (1997) 94(17):9360–5. doi: 10.1073/pnas.94.17.9360
50. Kim JH, Sim JH, Lee S, Seol MA, Ye SK, Shin HM, et al. Interleukin-7 induces osteoclast formation via STAT5, independent of receptor activator of NF-kappaB ligand. Front Immunol (2017) 8:1376.
51. Kopesky P, Tiedemann K, Alkekhia D, Zechner C, Millard B, Schoeberl B, et al. Autocrine signaling is a key regulatory element during osteoclastogenesis. Biol Open (2014) 3(8):767–76. doi: 10.1242/bio.20148128
52. Xu LX, Kukita T, Kukita A, Otsuka T, Niho Y, Iijima T. Interleukin-10 selectively inhibits osteoclastogenesis by inhibiting differentiation of osteoclast progenitors into preosteoclast-like cells in rat bone marrow culture system. J Cell Physiol (1995) 165(3):624–9. doi: 10.1002/jcp.1041650321
53. Liu D, Yao S, Wise GE. Effect of interleukin-10 on gene expression of osteoclastogenic regulatory molecules in the rat dental follicle. Eur J Oral Sci (2006) 114(1):42–9. doi: 10.1111/j.1600-0722.2006.00283.x
54. Park-Min KH, Ji JD, Antoniv T, Reid AC, Silver RB, Humphrey MB, et al. IL-10 suppresses calcium-mediated costimulation of receptor activator NF-kappa b signaling during human osteoclast differentiation by inhibiting TREM-2 expression. J Immunol (2009) 183(4):2444–55. doi: 10.4049/jimmunol.0804165
55. Mohamed SG, Sugiyama E, Shinoda K, Taki H, Hounoki H, Abdel-Aziz HO, et al. Interleukin-10 inhibits RANKL-mediated expression of NFATc1 in part via suppression of c-fos and c-jun in RAW264.7 cells and mouse bone marrow cells. Bone (2007) 41(4):592–602. doi: 10.1016/j.bone.2007.05.016
56. Van Vlasselaer P, Borremans B, Van Den Heuvel R, Van Gorp U, de Waal Malefyt R. Interleukin-10 inhibits the osteogenic activity of mouse bone marrow. Blood (1993) 82(8):2361–70.
57. Tamura T, Udagawa N, Takahashi N, Miyaura C, Tanaka S, Yamada Y, et al. Soluble interleukin-6 receptor triggers osteoclast formation by interleukin 6. Proc Natl Acad Sci U S A (1993) 90(24):11924–8. doi: 10.1073/pnas.90.24.11924
58. McCoy EM, Hong H, Pruitt HC, Feng X. IL-11 produced by breast cancer cells augments osteoclastogenesis by sustaining the pool of osteoclast progenitor cells. BMC Cancer (2013) 13:16. doi: 10.1186/1471-2407-13-16
59. Taguchi Y, Yamamoto M, Yamate T, Lin SC, Mocharla H, DeTogni P, et al. Interleukin-6-type cytokines stimulate mesenchymal progenitor differentiation toward the osteoblastic lineage. Proc Assoc Am Physicians (1998) 110(6):559–74.
60. Dong B, Hiasa M, Higa Y, Ohnishi Y, Endo I, Kondo T, et al. Osteoblast/osteocyte-derived interleukin-11 regulates osteogenesis and systemic adipogenesis. Nat Commun (2022) 13(1):7194. doi: 10.1038/s41467-022-34869-3
61. Amcheslavsky A, Bar-Shavit Z. Interleukin (IL)-12 mediates the anti-osteoclastogenic activity of CpG-oligodeoxynucleotides. J Cell Physiol (2006) 207(1):244–50. doi: 10.1002/jcp.20563
62. Nagata N, Kitaura H, Yoshida N, Nakayama K. Inhibition of RANKL-induced osteoclast formation in mouse bone marrow cells by IL-12: involvement of IFN-gamma possibly induced from non-T cell population. Bone (2003) 33(4):721–32. doi: 10.1016/s8756-3282(03)00213-8
63. Onoe Y, Miyaura C, Kaminakayashiki T, Nagai Y, Noguchi K, Chen QR, et al. IL-13 and IL-4 inhibit bone resorption by suppressing cyclooxygenase-2-dependent prostaglandin synthesis in osteoblasts. J Immunol (1996) 156(2):758–64.
64. Ogata Y, Kukita A, Kukita T, Komine M, Miyahara A, Miyazaki S, et al. A novel role of IL-15 in the development of osteoclasts: inability to replace its activity with IL-2. J Immunol (1999) 162(5):2754–60.
65. Okabe I, Kikuchi T, Mogi M, Takeda H, Aino M, Kamiya Y, et al. IL-15 and RANKL play a synergistically important role in osteoclastogenesis. J Cell Biochem (2017) 118(4):739–47. doi: 10.1002/jcb.25726
66. Takeda H, Kikuchi T, Soboku K, Okabe I, Mizutani H, Mitani A, et al. Effect of IL-15 and natural killer cells on osteoclasts and osteoblasts in a mouse coculture. Inflammation (2014) 37(3):657–69. doi: 10.1007/s10753-013-9782-0
67. Tyagi AM, Srivastava K, Mansoori MN, Trivedi R, Chattopadhyay N, Singh D. Estrogen deficiency induces the differentiation of IL-17 secreting Th17 cells: a new candidate in the pathogenesis of osteoporosis. PloS One (2012) 7(9):e44552. doi: 10.1371/journal.pone.0044552
68. Tyagi AM, Mansoori MN, Srivastava K, Khan MP, Kureel J, Dixit M, et al. Enhanced immunoprotective effects by anti-IL-17 antibody translates to improved skeletal parameters under estrogen deficiency compared with anti-RANKL and anti-TNF-α antibodies. J Bone Miner Res (2014) 29(9):1981–92. doi: 10.1002/jbmr.2228
69. Dixit M, Singh KB, Prakash R, Singh D. Functional block of IL-17 cytokine promotes bone healing by augmenting FOXO1 and ATF4 activity in cortical bone defect model. Osteoporos Int (2017) 28(7):2207–20. doi: 10.1007/s00198-017-4012-5
70. Bhadricha H, Patel V, Singh AK, Savardekar L, Patil A, Surve S, et al. Increased frequency of Th17 cells and IL-17 levels are associated with low bone mineral density in postmenopausal women. Sci Rep (2021) 11(1):16155. doi: 10.1038/s41598-021-95640-0
71. Lubberts E, van den Bersselaar L, Oppers-Walgreen B, Schwarzenberger P, Coenen-de Roo CJ, Kolls JK, et al. IL-17 promotes bone erosion in murine collagen-induced arthritis through loss of the receptor activator of NF-kappa b ligand/osteoprotegerin balance. J Immunol (2003) 170(5):2655–62. doi: 10.4049/jimmunol.170.5.2655
72. Hwang SY, Kim JY, Kim KW, Park MK, Moon Y, Kim WU, et al. IL-17 induces production of IL-6 and IL-8 in rheumatoid arthritis synovial fibroblasts via NF-kappaB- and PI3-kinase/Akt-dependent pathways. Arthritis Res Ther (2004) 6(2):R120–8. doi: 10.1186/ar1038
73. Jovanovic DV, Di Battista JA, Martel-Pelletier J, Jolicoeur FC, He Y, Zhang M, et al. IL-17 stimulates the production and expression of proinflammatory cytokines, IL-beta and TNF-alpha, by human macrophages. J Immunol (1998) 160(7):3513–21.
74. Ke D, Fu X, Xue Y, Wu H, Zhang Y, Chen X, et al. IL-17A regulates the autophagic activity of osteoclast precursors through RANKL-JNK1 signaling during osteoclastogenesis in vitro. Biochem Biophys Res Commun (2018) 497(3):890–6. doi: 10.1016/j.bbrc.2018.02.164
75. Kitami S, Tanaka H, Kawato T, Tanabe N, Katono-Tani T, Zhang F, et al. IL-17A suppresses the expression of bone resorption-related proteinases and osteoclast differentiation via IL-17RA or IL-17RC receptors in RAW264.7 cells. Biochimie (2010) 92(4):398–404. doi: 10.1016/j.biochi.2009.12.011
76. Huang H, Kim HJ, Chang EJ, Lee ZH, Hwang SJ, Kim HM, et al. IL-17 stimulates the proliferation and differentiation of human mesenchymal stem cells: implications for bone remodeling. Cell Death Differ (2009) 16(10):1332–43. doi: 10.1038/cdd.2009.74
77. Wang Z, Tan J, Lei L, Sun W, Wu Y, Ding P, et al. The positive effects of secreting cytokines IL-17 and IFN-γ on the early-stage differentiation and negative effects on the calcification of primary osteoblasts in vitro. Int Immunopharmacol (2018) 57:1–10. doi: 10.1016/j.intimp.2018.02.002
78. Kim YG, Park JW, Lee JM, Suh JY, Lee JK, Chang BS, et al. IL-17 inhibits osteoblast differentiation and bone regeneration in rat. Arch Oral Biol (2014) 59(9):897–905. doi: 10.1016/j.archoralbio.2014.05.009
79. Lei L, Sun J, Han J, Jiang X, Wang Z, Chen L. Interleukin-17 induces pyroptosis in osteoblasts through the NLRP3 inflammasome pathway in vitro. Int Immunopharmacol (2021) 96:107781. doi: 10.1016/j.intimp.2021.107781
80. Kitaura H, Tatamiya M, Nagata N, Fujimura Y, Eguchi T, Yoshida N, et al. IL-18 induces apoptosis of adherent bone marrow cells in TNF-alpha mediated osteoclast formation in synergy with IL-12. Immunol Lett (2006) 107(1):22–31. doi: 10.1016/j.imlet.2006.06.005
81. Morita Y, Kitaura H, Yoshimatsu M, Fujimura Y, Kohara H, Eguchi T, et al. IL-18 inhibits TNF-alpha-induced osteoclastogenesis possibly via a T cell-independent mechanism in synergy with IL-12 in vivo. Calcif Tissue Int (2010) 86(3):242–8. doi: 10.1007/s00223-010-9335-6
82. Kitaura H, Fujimura Y, Yoshimatsu M, Kohara H, Morita Y, Aonuma T, et al. IL-12- and IL-18-mediated, nitric oxide-induced apoptosis in TNF-α-mediated osteoclastogenesis of bone marrow cells. Calcif Tissue Int (2011) 89(1):65–73. doi: 10.1007/s00223-011-9494-0
83. Horwood NJ, Udagawa N, Elliott J, Grail D, Okamura H, Kurimoto M, et al. Interleukin 18 inhibits osteoclast formation via T cell production of granulocyte macrophage colony-stimulating factor. J Clin Invest (1998) 101(3):595–603. doi: 10.1172/JCI1333
84. Udagawa N, Horwood NJ, Elliott J, Mackay A, Owens J, Okamura H, et al. Interleukin-18 (interferon-gamma-inducing factor) is produced by osteoblasts and acts via granulocyte/macrophage colony-stimulating factor and not via interferon-gamma to inhibit osteoclast formation. J Exp Med (1997) 185(6):1005–12. doi: 10.1084/jem.185.6.1005
85. Tsubaki M, Takeda T, Matsuda T, Yamamoto Y, Higashinaka A, Yamamoto K, et al. Interleukin 19 suppresses RANKL-induced osteoclastogenesis via the inhibition of NF-κB and p38MAPK activation and c-fos expression in RAW264.7 cells. Cytokine (2021) 144:155591. doi: 10.1016/j.cyto.2021.155591
86. Hsu YH, Chen WY, Chan CH, Wu CH, Sun ZJ, Chang MS. Anti-IL-20 monoclonal antibody inhibits the differentiation of osteoclasts and protects against osteoporotic bone loss. J Exp Med (2011) 208(9):1849–61. doi: 10.1084/jem.20102234
87. Hsu YH, Chiu YS, Chen WY, Huang KY, Jou IM, Wu PT, et al. Anti-IL-20 monoclonal antibody promotes bone fracture healing through regulating IL-20-mediated osteoblastogenesis. Sci Rep (2016) 6:24339. doi: 10.1038/srep24339
88. Sato K, Suematsu A, Okamoto K, Yamaguchi A, Morishita Y, Kadono Y, et al. Th17 functions as an osteoclastogenic helper T cell subset that links T cell activation and bone destruction. J Exp Med (2006) 203(12):2673–82. doi: 10.1084/jem.20061775
89. Chen L, Wei XQ, Evans B, Jiang W, Aeschlimann D. IL-23 promotes osteoclast formation by up-regulation of receptor activator of NF-kappaB (RANK) expression in myeloid precursor cells. Eur J Immunol (2008) 38(10):2845–54. doi: 10.1002/eji.200838192
90. Ju JH, Cho ML, Moon YM, Oh HJ, Park JS, Jhun JY, et al. IL-23 induces receptor activator of NF-kappaB ligand expression on CD4+ T cells and promotes osteoclastogenesis in an autoimmune arthritis model. J Immunol (2008) 181(2):1507–18. doi: 10.4049/jimmunol.181.2.1507
91. Quinn JM, Sims NA, Saleh H, Mirosa D, Thompson K, Bouralexis S, et al. IL-23 inhibits osteoclastogenesis indirectly through lymphocytes and is required for the maintenance of bone mass in mice. J Immunol (2008) 181(8):5720–9. doi: 10.4049/jimmunol.181.8.5720
92. Kalliolias GD, Zhao B, Triantafyllopoulou A, Park-Min KH, Ivashkiv LB. Interleukin-27 inhibits human osteoclastogenesis by abrogating RANKL-mediated induction of nuclear factor of activated T cells c1 and suppressing proximal RANK signaling. Arthritis Rheumatol (2010) 62(2):402–13. doi: 10.1002/art.27200
93. Furukawa M, Takaishi H, Takito J, Yoda M, Sakai S, Hikata T, et al. IL-27 abrogates receptor activator of NF-kappa b ligand-mediated osteoclastogenesis of human granulocyte-macrophage colony-forming unit cells through STAT1-dependent inhibition of c-fos. J Immunol (2009) 183(4):2397–406. doi: 10.4049/jimmunol.0802091
94. Kamiya S, Okumura M, Chiba Y, Fukawa T, Nakamura C, Nimura N, et al. IL-27 suppresses RANKL expression in CD4+ T cells in part through STAT3. Immunol Lett (2011) 138(1):47–53. doi: 10.1016/j.imlet.2011.02.022
95. Shukla P, Mansoori MN, Kakaji M, Shukla M, Gupta SK, Singh D. Interleukin 27 (IL-27) alleviates bone loss in estrogen-deficient conditions by induction of early growth response-2 gene. J Biol Chem (2017) 292(11):4686–99. doi: 10.1074/jbc.M116.764779
96. Chen Y, Wang Y, Tang R, Yang J, Dou C, Dong Y, et al. Dendritic cells-derived interferon-λ1 ameliorated inflammatory bone destruction through inhibiting osteoclastogenesis. Cell Death Dis (2020) 11(6):414. doi: 10.1038/s41419-020-2612-z
97. Peng Q, Luo A, Zhou Z, Xuan W, Qiu M, Wu Q, et al. Interleukin 29 inhibits RANKL-induced osteoclastogenesis via activation of JNK and STAT, and inhibition of NF-κB and NFATc1. Cytokine (2019) 113:144–54. doi: 10.1016/j.cyto.2018.06.032
98. Lee EJ, Kim SM, Choi B, Kim EY, Chung YH, Lee EJ, et al. Interleukin-32 gamma stimulates bone formation by increasing miR-29a in osteoblastic cells and prevents the development of osteoporosis. Sci Rep (2017) 7:40240. doi: 10.1038/srep40240
99. Kiyomiya H, Ariyoshi W, Okinaga T, Kaneuji T, Mitsugi S, Sakurai T, et al. IL-33 inhibits RANKL-induced osteoclast formation through the regulation of blimp-1 and IRF-8 expression. Biochem Biophys Res Commun (2015) 460(2):320–6. doi: 10.1016/j.bbrc.2015.03.033
100. De Martinis M, Ginaldi L, Sirufo MM, Bassino EM, De Pietro F, Pioggia G, et al. IL-33/Vitamin d crosstalk in psoriasis-associated osteoporosis. Front Immunol (2021) 11:604055:604055. doi: 10.3389/fimmu.2020.604055
101. Lima IL, Macari S, Madeira MF, Rodrigues LF, Colavite PM, Garlet GP, et al. Osteoprotective effects of IL-33/ST2 link to osteoclast apoptosis. Am J Pathol (2015) 185(12):3338–48. doi: 10.1016/j.ajpath.2015.08.013
102. Ohori F, Kitaura H, Ogawa S, Shen WR, Qi J, Noguchi T, et al. IL-33 inhibits TNF-α-Induced osteoclastogenesis and bone resorption. Int J Mol Sci (2020) 21(3):1130. doi: 10.3390/ijms21031130
103. Schulze J, Bickert T, Beil FT, Zaiss MM, Albers J, Wintges K, et al. Interleukin-33 is expressed in differentiated osteoblasts and blocks osteoclast formation from bone marrow precursor cells. J Bone Miner Res (2011) 26(4):704–17. doi: 10.1002/jbmr.269
104. Chen Z, Buki K, Vääräniemi J, Gu G, Väänänen HK. The critical role of IL-34 in osteoclastogenesis. PloS One (2011) 6(4):e18689. doi: 10.1371/journal.pone.0018689
105. Cheng X, Wan QL, Li ZB. AG490 suppresses interleukin-34-mediated osteoclastogenesis in mice bone marrow macrophages. Cell Biol Int (2017) 41(6):659–68. doi: 10.1002/cbin.10771
106. Xu J, Fu L, Bai J, Zhong H, Kuang Z, Zhou C, et al. Low-dose IL-34 has no effect on osteoclastogenesis but promotes osteogenesis of hBMSCs partly via activation of the PI3K/AKT and ERK signaling pathways. Stem Cell Res Ther (2021) 12(1):268. doi: 10.1186/s13287-021-02263-3
107. Peng M, Wang Y, Qiang L, Xu Y, Li C, Li T, et al. Interleukin-35 inhibits TNF-α-Induced osteoclastogenesis and promotes apoptosis via shifting the activation from TNF receptor-associated death domain (TRADD)-TRAF2 to TRADD-Fas-Associated death domain by JAK1/STAT1. Front Immunol (2018) 9:1417:1417. doi: 10.3389/fimmu.2018.01417
108. Li Y, Wang X, Lu J. Interleukin-35 promote osteogenesis and inhibit adipogenesis: role of wnt/β-catenin and PPARγ signaling pathways. Inflammation (2023) 46(2):522–33. doi: 10.1007/s10753-022-01749-3
109. Mun SH, Ko NY, Kim HS, Kim JW, Kim DK, Kim AR, et al. Interleukin-33 stimulates formation of functional osteoclasts from human CD14(+) monocytes. Cell Mol Life Sci (2010) 67(22):3883–92. doi: 10.1007/s00018-010-0410-y
110. Saeed J, Kitaura H, Kimura K, Ishida M, Sugisawa H, Ochi Y, et al. IL-37 inhibits lipopolysaccharide-induced osteoclast formation and bone resorption in vivo. Immunol Lett (2016) 175:8–15. doi: 10.1016/j.imlet.2016.04.004
111. Ye C, Zhang W, Hang K, Chen M, Hou W, Chen J, et al. Extracellular IL-37 promotes osteogenic differentiation of human bone marrow mesenchymal stem cells via activation of the PI3K/AKT signaling pathway. Cell Death Dis (2019) 10(10):753. doi: 10.1038/s41419-019-1904-7
112. Avnet S, Cenni E, Perut F, Granchi D, Brandi ML, Giunti A, et al. Interferon-alpha inhibits in vitro osteoclast differentiation and renal cell carcinoma-induced angiogenesis. Int J Oncol (2007) 30(2):469–76.
113. Takayanagi H, Sato K, Takaoka A, Taniguchi T. Interplay between interferon and other cytokine systems in bone metabolism. Immunol Rev (2005) 208:181–93. doi: 10.1111/j.0105-2896.2005.00337.x
114. Zheng H, Yu X, Collin-Osdoby P, Osdoby P. RANKL stimulates inducible nitric-oxide synthase expression and nitric oxide production in developing osteoclasts. an autocrine negative feedback mechanism triggered by RANKL-induced interferon-beta via NF-kappaB that restrains osteoclastogenesis and bone resorption. J Biol Chem (2006) 281(23):15809–20. doi: 10.1074/jbc.M513225200
115. Gowen M, MacDonald BR, Russell RG. Actions of recombinant human gamma-interferon and tumor necrosis factor alpha on the proliferation and osteoblastic characteristics of human trabecular bone cells in vitro. Arthritis Rheumatol (1988) 31(12):1500–7. doi: 10.1002/art.1780311206
116. Maruhashi T, Kaifu T, Yabe R, Seno A, Chung SH, Fujikado N, et al. DCIR maintains bone homeostasis by regulating IFN-γ production in T cells. J Immunol (2015) 194(12):5681–91. doi: 10.4049/jimmunol.1500273
117. Duque G, Huang DC, Dion N, Macoritto M, Rivas D, Li W, et al. Interferon-γ plays a role in bone formation in vivo and rescues osteoporosis in ovariectomized mice. J Bone Miner Res (2011) 26(7):1472–83. doi: 10.1002/jbmr.350
118. Ji JD, Park-Min KH, Shen Z, Fajardo RJ, Goldring SR, McHugh KP, et al. Inhibition of RANK expression and osteoclastogenesis by TLRs and IFN-gamma in human osteoclast precursors. J Immunol (2009) 183(11):7223–33. doi: 10.4049/jimmunol.0900072
119. Kohara H, Kitaura H, Fujimura Y, Yoshimatsu M, Morita Y, Eguchi T, et al. IFN-γ directly inhibits TNF-α-induced osteoclastogenesis in vitro and in vivo and induces apoptosis mediated by Fas/Fas ligand interactions. Immunol Lett (2011) 137(1-2):53–61. doi: 10.1016/j.imlet.2011.02.017
120. Kim JW, Lee MS, Lee CH, Kim HY, Chae SU, Kwak HB, et al. Effect of interferon-γ on the fusion of mononuclear osteoclasts into bone-resorbing osteoclasts. BMB Rep (2012) 45(5):281–6. doi: 10.5483/bmbrep.2012.45.5.281
121. Kwak HB, Ha H, Kim HN, Lee JH, Kim HS, Lee S, et al. Reciprocal cross-talk between RANKL and interferon-gamma-inducible protein 10 is responsible for bone-erosive experimental arthritis. Arthritis Rheumatol (2008) 58(5):1332–42. doi: 10.1002/art.23372
122. Pietschmann P, Mechtcheriakova D, Meshcheryakova A, Föger-Samwald U, Ellinger I. Immunology of osteoporosis: a mini-review. Gerontology (2016) 62(2):128–37. doi: 10.1159/000431091
123. Suda T, Kobayashi K, Jimi E, Udagawa N, Takahashi N. The molecular basis of osteoclast differentiation and activation. Novartis Found Symp (2001) 232:235–47. doi: 10.1002/0470846658.ch16
124. Kobayashi K, Takahashi N, Jimi E, Udagawa N, Takami M, Kotake S, et al. Tumor necrosis factor alpha stimulates osteoclast differentiation by a mechanism independent of the ODF/RANKL-RANK interaction. J Exp Med (2000) 191(2):275–86. doi: 10.1084/jem.191.2.275
125. Yamashita T, Yao Z, Li F, Zhang Q, Badell IR, Schwarz EM, et al. NF-kappaB p50 and p52 regulate receptor activator of NF-kappaB ligand (RANKL) and tumor necrosis factor-induced osteoclast precursor differentiation by activating c-fos and NFATc1. J Biol Chem (2007) 282(25):18245–53. doi: 10.1074/jbc.M610701200
126. Matsuo K, Galson DL, Zhao C, Peng L, Laplace C, Wang KZ, et al. Nuclear factor of activated T-cells (NFAT) rescues osteoclastogenesis in precursors lacking c-fos. J Biol Chem (2004) 279(25):26475–80. doi: 10.1074/jbc.M313973200
127. Yao Z, Xing L, Qin C, Schwarz EM, Boyce BF. Osteoclast precursor interaction with bone matrix induces osteoclast formation directly by an interleukin-1-mediated autocrine mechanism. J Biol Chem (2008) 283(15):9917–24. doi: 10.1074/jbc.M706415200
128. Kanazawa K, Kudo A. TRAF2 is essential for TNF-alpha-induced osteoclastogenesis. J Bone Miner Res (2005) 20(5):840–7. doi: 10.1359/JBMR.041225
129. Kanazawa K, Azuma Y, Nakano H, Kudo A. TRAF5 functions in both RANKL- and TNFalpha-induced osteoclastogenesis. J Bone Miner Res (2003) 18(3):443–50. doi: 10.1359/jbmr.2003.18.3.443
130. Mizukami J, Takaesu G, Akatsuka H, Sakurai H, Ninomiya-Tsuji J, Matsumoto K, et al. Receptor activator of NF-kappaB ligand (RANKL) activates TAK1 mitogen-activated protein kinase kinase kinase through a signaling complex containing RANK, TAB2, and TRAF6. Mol Cell Biol (2002) 22(4):992–1000. doi: 10.1128/MCB.22.4.992-1000.2002
131. Yao Z, Lei W, Duan R, Li Y, Luo L, Boyce BF. RANKL cytokine enhances TNF-induced osteoclastogenesis independently of TNF receptor associated factor (TRAF) 6 by degrading TRAF3 in osteoclast precursors. J Biol Chem (2017) 292(24):10169–79. doi: 10.1074/jbc.M116.771816
132. Cao Y, Jansen ID, Sprangers S, Stap J, Leenen PJ, Everts V, et al. IL-1β differently stimulates proliferation and multinucleation of distinct mouse bone marrow osteoclast precursor subsets. J Leukoc Biol (2016) 100(3):513–23. doi: 10.1189/jlb.1A1215-543R
133. Kim JH, Jin HM, Kim K, Song I, Youn BU, Matsuo K, et al. The mechanism of osteoclast differentiation induced by IL-1. J Immunol (2009) 183(3):1862–70. doi: 10.4049/jimmunol.0803007
134. Hercus TR, Dhagat U, Kan WL, Broughton SE, Nero TL, Perugini M, et al. Signalling by the βc family of cytokines. Cytokine Growth Factor Rev (2013) 24(3):189–201. doi: 10.1016/j.cytogfr.2013.03.002
135. Soysa NS, Alles N. The role of IL-3 in bone. J Cell Biochem (2019) 120(5):6851–9. doi: 10.1002/jcb.27956
136. Singh K, Piprode V, Mhaske ST, Barhanpurkar-Naik A, Wani MR. IL-3 differentially regulates membrane and soluble RANKL in osteoblasts through metalloproteases and the JAK2/STAT5 pathway and improves the RANKL/OPG ratio in adult mice. J Immunol (2018) 200(2):595–606. doi: 10.4049/jimmunol.1601528
137. Nelms K, Keegan AD, Zamorano J, Ryan JJ, Paul WE. The IL-4 receptor: signaling mechanisms and biologic functions. Annu Rev Immunol (1999) 17:701–38. doi: 10.1146/annurev.immunol.17.1.701
138. Rose-John S. Interleukin-6 family cytokines. Cold Spring Harb Perspect Biol (2018) 10(2):a028415. doi: 10.1101/cshperspect.a028415
139. Scheller J, Garbers C, Rose-John S. Interleukin-6: from basic biology to selective blockade of pro-inflammatory activities. Semin Immunol (2014) 26(1):2–12. doi: 10.1016/j.smim.2013.11.002
140. Feyen JH, Elford P, Di Padova FE, Trechsel U. Interleukin-6 is produced by bone and modulated by parathyroid hormone. J Bone Miner Res (1989) 4(4):633–8. doi: 10.1002/jbmr.5650040422
141. Löwik CW, van der Pluijm G, Bloys H, Hoekman K, Bijvoet OL, Aarden LA, et al. Parathyroid hormone (PTH) and PTH-like protein (PLP) stimulate interleukin-6 production by osteogenic cells: a possible role of interleukin-6 in osteoclastogenesis. Biochem Biophys Res Commun (1989) 162(3):1546–52. doi: 10.1016/0006-291x(89)90851-6
142. Girasole G, Jilka RL, Passeri G, Boswell S, Boder G, Williams DC, et al. 17 beta-estradiol inhibits interleukin-6 production by bone marrow-derived stromal cells and osteoblasts in vitro: a potential mechanism for the antiosteoporotic effect of estrogens. J Clin Invest (1992) 89(3):883–91. doi: 10.1172/JCI115668
143. Briso EM, Dienz O, Rincon M. Cutting edge: soluble IL-6R is produced by IL-6R ectodomain shedding in activated CD4 T cells. J Immunol (2008) 180(11):7102–6. doi: 10.4049/jimmunol.180.11.7102
144. Modur V, Li Y, Zimmerman GA, Prescott SM, McIntyre TM. Retrograde inflammatory signaling from neutrophils to endothelial cells by soluble interleukin-6 receptor alpha. J Clin Invest (1997) 100(11):2752–6. doi: 10.1172/JCI119821
145. Khosla S, Atkinson EJ, Dunstan CR, O'Fallon WM. Effect of estrogen versus testosterone on circulating osteoprotegerin and other cytokine levels in normal elderly men. J Clin Endocrinol Metab (2002) 87(4):1550–4. doi: 10.1210/jcem.87.4.8397
146. Koshihara Y, Suematsu A, Feng D, Okawara R, Ishibashi H, Yamamoto S. Osteoclastogenic potential of bone marrow cells increases with age in elderly women with fracture. Mech Ageing Dev (2002) 123(10):1321–31. doi: 10.1016/s0047-6374(02)00071-4
147. Bonda TA, Dziemidowicz M, Cieslinska M, Tarasiuk E, Wawrusiewicz-Kurylonek N, Bialuk I, et al. Interleukin 6 knockout inhibits aging-related accumulation of p53 in the mouse myocardium. J Gerontol A Biol Sci Med Sci (2019) 74(2):176–82. doi: 10.1093/gerona/gly105
148. Tyrrell DJ, Blin MG, Song J, Wood SC, Zhang M, Beard DA, et al. Age-associated mitochondrial dysfunction accelerates atherogenesis. Circ Res (2020) 126(3):298–314. doi: 10.1161/CIRCRESAHA.119.315644
149. Wang M, Shah AM. Age-associated pro-inflammatory remodeling and functional phenotype in the heart and large arteries. J Mol Cell Cardiol (2015) 83:101–11. doi: 10.1016/j.yjmcc.2015.02.004
150. De Benedetti F, Rucci N, Del Fattore A, Peruzzi B, Paro R, Longo M, et al. Impaired skeletal development in interleukin-6-transgenic mice: a model for the impact of chronic inflammation on the growing skeletal system. Arthritis Rheumatol (2006) 54(11):3551–63. doi: 10.1002/art.22175
151. Axmann R, Böhm C, Krönke G, Zwerina J, Smolen J, Schett G. Inhibition of interleukin-6 receptor directly blocks osteoclast formation in vitro and in vivo. Arthritis Rheumatol (2009) 60(9):2747–56. doi: 10.1002/art.24781
152. Lazzaro L, Tonkin BA, Poulton IJ, McGregor NE, Ferlin W, Sims NA. IL-6 trans-signalling mediates trabecular, but not cortical, bone loss after ovariectomy. Bone (2018) 112:120–7. doi: 10.1016/j.bone.2018.04.015
153. Liu H, Feng W, Yimin, Cui J, Lv S, Hasegawa T, et al. Histological evidence of increased osteoclast cell number and asymmetric bone resorption activity in the tibiae of interleukin-6-Deficient mice. J Histochem Cytochem (2014) 62(8):556–64. doi: 10.1369/0022155414537830
154. Zhu S, He H, Gao C, Luo G, Xie Y, Wang H, et al. Ovariectomy-induced bone loss in TNFα and IL6 gene knockout mice is regulated by different mechanisms. J Mol Endocrinol (2018) 60(3):185–98. doi: 10.1530/JME-17-0218
155. Wang J, Chen J, Zhang B, Jia X. IL-6 regulates the bone metabolism and inflammatory microenvironment in aging mice by inhibiting Setd7. Acta Histochem (2021) 123(5):151718. doi: 10.1016/j.acthis.2021.151718
156. Xie Z, Tang S, Ye G, Wang P, Li J, Liu W, et al. Interleukin-6/interleukin-6 receptor complex promotes osteogenic differentiation of bone marrow-derived mesenchymal stem cells. Stem Cell Res Ther (2018) 9(1):13. doi: 10.1186/s13287-017-0766-0
157. Kawashima I, Ohsumi J, Mita-Honjo K, Shimoda-Takano K, Ishikawa H, Sakakibara S, et al. Molecular cloning of cDNA encoding adipogenesis inhibitory factor and identity with interleukin-11. FEBS Lett (1991) 283(2):199–202. doi: 10.1016/0014-5793(91)80587-s
158. Romas E, Udagawa N, Zhou H, Tamura T, Saito M, Taga T, et al. The role of gp130-mediated signals in osteoclast development: regulation of interleukin 11 production by osteoblasts and distribution of its receptor in bone marrow cultures. J Exp Med (1996) 183(6):2581–91. doi: 10.1084/jem.183.6.2581
159. Takeuchi Y, Watanabe S, Ishii G, Takeda S, Nakayama K, Fukumoto S, et al. Interleukin-11 as a stimulatory factor for bone formation prevents bone loss with advancing age in mice. J Biol Chem (2002) 277(50):49011–8. doi: 10.1074/jbc.M207804200
160. Sims NA, Jenkins BJ, Nakamura A, Quinn JM, Li R, Gillespie MT, et al. Interleukin-11 receptor signaling is required for normal bone remodeling. J Bone Miner Res (2005) 20(7):1093–102. doi: 10.1359/JBMR.050209
161. Kido S, Kuriwaka-Kido R, Imamura T, Ito Y, Inoue D, Matsumoto T. Mechanical stress induces interleukin-11 expression to stimulate osteoblast differentiation. Bone (2009) 45(6):1125–32. doi: 10.1016/j.bone.2009.07.087
162. Koyama Y, Mitsui N, Suzuki N, Yanagisawa M, Sanuki R, Isokawa K, et al. Effect of compressive force on the expression of inflammatory cytokines and their receptors in osteoblastic saos-2 cells. Arch Oral Biol (2008) 53(5):488–96. doi: 10.1016/j.archoralbio.2007.12.004
163. Kido S, Kuriwaka-Kido R, Umino-Miyatani Y, Endo I, Inoue D, Taniguchi H, et al. Mechanical stress activates smad pathway through PKCδ to enhance interleukin-11 gene transcription in osteoblasts. PloS One (2010) 5(9):e13090. doi: 10.1371/journal.pone.0013090
164. Lisignoli G, Piacentini A, Toneguzzi S, Grassi F, Cocchini B, Ferruzzi A, et al. Osteoblasts and stromal cells isolated from femora in rheumatoid arthritis (RA) and osteoarthritis (OA) patients express IL-11, leukaemia inhibitory factor and oncostatin m. Clin Exp Immunol (2000) 119(2):346–53. doi: 10.1046/j.1365-2249.2000.01114.x
165. Guihard P, Danger Y, Brounais B, David E, Brion R, Delecrin J, et al. Induction of osteogenesis in mesenchymal stem cells by activated monocytes/macrophages depends on oncostatin m signaling. Stem Cells (2012) 30(4):762–72. doi: 10.1002/stem.1040
166. Fernandes TJ, Hodge JM, Singh PP, Eeles DG, Collier FM, Holten I, et al. Cord blood-derived macrophage-lineage cells rapidly stimulate osteoblastic maturation in mesenchymal stem cells in a glycoprotein-130 dependent manner. PloS One (2013) 8(9):e73266. doi: 10.1371/journal.pone.0073266
167. Nicolaidou V, Wong MM, Redpath AN, Ersek A, Baban DF, Williams LM, et al. Monocytes induce STAT3 activation in human mesenchymal stem cells to promote osteoblast formation. PloS One (2012) 7(7):e39871. doi: 10.1371/journal.pone.0039871
168. Champagne CM, Takebe J, Offenbacher S, Cooper LF. Macrophage cell lines produce osteoinductive signals that include bone morphogenetic protein-2. Bone (2002) 30(1):26–31. doi: 10.1016/s8756-3282(01)00638-x
169. Chang MK, Raggatt LJ, Alexander KA, Kuliwaba JS, Fazzalari NL, Schroder K, et al. Osteal tissue macrophages are intercalated throughout human and mouse bone lining tissues and regulate osteoblast function in vitro and in vivo. J Immunol (2008) 181(2):1232–44. doi: 10.4049/jimmunol.181.2.1232
170. Cho SW, Soki FN, Koh AJ, Eber MR, Entezami P, Park SI, et al. Osteal macrophages support physiologic skeletal remodeling and anabolic actions of parathyroid hormone in bone. Proc Natl Acad Sci U S A (2014) 111(4):1545–50. doi: 10.1073/pnas.1315153111
171. Vi L, Baht GS, Whetstone H, Ng A, Wei Q, Poon R, et al. Macrophages promote osteoblastic differentiation in-vivo: implications in fracture repair and bone homeostasis. J Bone Miner Res (2015) 30(6):1090–102. doi: 10.1002/jbmr.2422
172. McGregor NE, Murat M, Elango J, Poulton IJ, Walker EC, Crimeen-Irwin B, et al. IL-6 exhibits both cis- and trans-signaling in osteocytes and osteoblasts, but only trans-signaling promotes bone formation and osteoclastogenesis. J Biol Chem (2019) 294(19):7850–63. doi: 10.1074/jbc.RA119.008074
173. Salopek D, Grcević D, Katavić V, Kovacić N, Lukić IK, Marusić A. Increased bone resorption and osteopenia are a part of the lymphoproliferative phenotype of mice with systemic over-expression of interleukin-7 gene driven by MHC class II promoter. Immunol Lett (2008) 121(2):134–9. doi: 10.1016/j.imlet.2008.10.002
174. Ryan MR, Shepherd R, Leavey JK, Gao Y, Grassi F, Schnell FJ, et al. An IL-7-dependent rebound in thymic T cell output contributes to the bone loss induced by estrogen deficiency. Proc Natl Acad Sci U S A (2005) 102(46):16735–40. doi: 10.1073/pnas.0505168102
175. Miller JP, Izon D, DeMuth W, Gerstein R, Bhandoola A, Allman D. The earliest step in b lineage differentiation from common lymphoid progenitors is critically dependent upon interleukin 7. J Exp Med (2002) 196(5):705–11. doi: 10.1084/jem.20020784
176. Wang Y, Xiao M, Tao C, Chen J, Wang Z, Yang J, et al. Inactivation of mTORC1 signaling in osterix-expressing cells impairs b-cell differentiation. J Bone Miner Res (2018) 33(4):732–42. doi: 10.1002/jbmr.3352
177. Martin SK, Fitter S, El Khawanky N, Grose RH, Walkley CR, Purton LE, et al. mTORC1 plays an important role in osteoblastic regulation of b-lymphopoiesis. Sci Rep (2018) 8(1):14501. doi: 10.1038/s41598-018-32858-5
178. Zhao JJ, Wu ZF, Yu YH, Wang L, Cheng L. Effects of interleukin-7/interleukin-7 receptor on RANKL-mediated osteoclast differentiation and ovariectomy-induced bone loss by regulating c-Fos/c-Jun pathway. J Cell Physiol (2018) 233(9):7182–94. doi: 10.1002/jcp.26548
179. Lee SK, Kalinowski JF, Jastrzebski SL, Puddington L, Lorenzo JA. Interleukin-7 is a direct inhibitor of in vitro osteoclastogenesis. Endocrinology (2003) 144(8):3524–31. doi: 10.1210/en.2002-221057
180. Lee SK, Kalinowski JF, Jacquin C, Adams DJ, Gronowicz G, Lorenzo JA. Interleukin-7 influences osteoclast function in vivo but is not a critical factor in ovariectomy-induced bone loss. J Bone Miner Res (2006) 21(5):695–702. doi: 10.1359/jbmr.060117
181. Ma X, Zhu X, He X, Yi X, Jin A. The wnt pathway regulator expression levels and their relationship to bone metabolism in thoracolumbar osteoporotic vertebral compression fracture patients. Am J Transl Res (2021) 13(5):4812–8.
182. Talaat RM, Sidek A, Mosalem A, Kholief A. Effect of bisphosphonates treatment on cytokine imbalance between TH17 and treg in osteoporosis. Inflammopharmacology (2015) 23(2-3):119–25. doi: 10.1007/s10787-015-0233-4
183. Sapra L, Bhardwaj A, Mishra PK, Garg B, Verma B, Mishra GC, et al. Regulatory b cells (Bregs) inhibit osteoclastogenesis and play a potential role in ameliorating ovariectomy-induced bone loss. Front Immunol (2021) 12:691081:691081. doi: 10.3389/fimmu.2021.691081
184. Dresner-Pollak R, Gelb N, Rachmilewitz D, Karmeli F, Weinreb M. Interleukin 10-deficient mice develop osteopenia, decreased bone formation, and mechanical fragility of long bones. Gastroenterology (2004) 127(3):792–801. doi: 10.1053/j.gastro.2004.06.013
185. Moore KW, de Waal Malefyt R, Coffman RL, O'Garra A. Interleukin-10 and the interleukin-10 receptor. Annu Rev Immunol (2001) 19:683–765. doi: 10.1146/annurev.immunol.19.1.683
186. Wang Y, Zhang W, Lim SM, Xu L, Jin JO. Interleukin-10-Producing b cells help suppress ovariectomy-mediated osteoporosis. Immune Netw (2020) 20(6):e50. doi: 10.4110/in.2020.20.e50
187. Okamura H, Tsutsi H, Komatsu T, Yutsudo M, Hakura A, Tanimoto T, et al. Cloning of a new cytokine that induces IFN-gamma production by T cells. Nature (1995) 378(6552):88–91. doi: 10.1038/378088a0
188. Mansoori MN, Shukla P, Kakaji M, Tyagi AM, Srivastava K, Shukla M, et al. IL-18BP is decreased in osteoporotic women: prevents inflammasome mediated IL-18 activation and reduces Th17 differentiation. Sci Rep (2016) 6:33680. doi: 10.1038/srep33680
189. Hsu YH, Hsieh PP, Chang MS. Interleukin-19 blockade attenuates collagen-induced arthritis in rats. Rheumatol (Oxford) (2012) 51(3):434–42. doi: 10.1093/rheumatology/ker127
190. Li Q, Kawamura K, Tada Y, Shimada H, Hiroshima K, Tagawa M. Novel type III interferons produce anti-tumor effects through multiple functions. Front Biosci (Landmark Ed) (2013) 18(3):909–18. doi: 10.2741/4152
191. Ginaldi L, De Martinis M, Saitta S, Sirufo MM, Mannucci C, Casciaro M, et al. Interleukin-33 serum levels in postmenopausal women with osteoporosis. Sci Rep (2019) 9(1):3786. doi: 10.1038/s41598-019-40212-6
192. Saleh H, Eeles D, Hodge JM, Nicholson GC, Gu R, Pompolo S, et al. Interleukin-33, a target of parathyroid hormone and oncostatin m, increases osteoblastic matrix mineral deposition and inhibits osteoclast formation in vitro. Endocrinology (2011) 152(5):1911–22. doi: 10.1210/en.2010-1268
193. Zaiss MM, Kurowska-Stolarska M, Böhm C, Gary R, Scholtysek C, Stolarski B, et al. IL-33 shifts the balance from osteoclast to alternatively activated macrophage differentiation and protects from TNF-alpha-mediated bone loss. J Immunol (2011) 186(11):6097–105. doi: 10.4049/jimmunol.1003487
194. Li J, Chen X, Lu L, Yu X. The relationship between bone marrow adipose tissue and bone metabolism in postmenopausal osteoporosis. Cytokine Growth Factor Rev (2020) 52:88–98. doi: 10.1016/j.cytogfr.2020.02.003
195. Tencerova M, Kassem M. The bone marrow-derived stromal cells: commitment and regulation of adipogenesis. Front Endocrinol (Lausanne) (2016) 7:127. doi: 10.3389/fendo.2016.00127
196. Zhang H, Li Y, Yuan L, Yao L, Yang J, Xia L, et al. Interleukin-35 is involved in Angiogenesis/Bone remodeling coupling through T helper 17/Interleukin-17 axis. Front Endocrinol (Lausanne) (2021) 12:642676:642676. doi: 10.3389/fendo.2021.642676
197. Xiong Q, Zhang L, Ge W, Tang P. The roles of interferons in osteoclasts and osteoclastogenesis. Joint Bone Spine (2016) 83(3):276–81. doi: 10.1016/j.jbspin.2015.07.010
198. Brylka LJ, Schinke T. Chemokines in physiological and pathological bone remodeling. Front Immunol (2019) 10:2182:2182. doi: 10.3389/fimmu.2019.02182
199. Binder NB, Niederreiter B, Hoffmann O, Stange R, Pap T, Stulnig TM, et al. Estrogen-dependent and c-c chemokine receptor-2-dependent pathways determine osteoclast behavior in osteoporosis. Nat Med (2009) 15(4):417–24. doi: 10.1038/nm.1945
200. Wan H, Qian TY, Hu XJ, Huang CY, Yao WF. Correlation of serum CCL3/MIP-1α levels with disease severity in postmenopausal osteoporotic females. Balkan Med J (2018) 35(4):320–5. doi: 10.4274/balkanmedj.2017.1165
201. Son HS, Lee J, Lee HI, Kim N, Jo YJ, Lee GR, et al. Benzydamine inhibits osteoclast differentiation and bone resorption via down-regulation of interleukin-1 β expression. Acta Pharm Sin B (2020) 10(3):462–74. doi: 10.1016/j.apsb.2019.11.004
Keywords: osteoporosis, cytokine, osteoblasts, osteoclasts, bone remodeling
Citation: Xu J, Yu L, Liu F, Wan L and Deng Z (2023) The effect of cytokines on osteoblasts and osteoclasts in bone remodeling in osteoporosis: a review. Front. Immunol. 14:1222129. doi: 10.3389/fimmu.2023.1222129
Received: 13 May 2023; Accepted: 12 June 2023;
Published: 05 July 2023.
Edited by:
Yufeng Yao, Huazhong University of Science and Technology, ChinaCopyright © 2023 Xu, Yu, Liu, Wan and Deng. This is an open-access article distributed under the terms of the Creative Commons Attribution License (CC BY). The use, distribution or reproduction in other forums is permitted, provided the original author(s) and the copyright owner(s) are credited and that the original publication in this journal is cited, in accordance with accepted academic practice. No use, distribution or reproduction is permitted which does not comply with these terms.
*Correspondence: Feng Liu, cm0wMDIyODhAd2h1LmVkdS5jbg==
†These authors contributed equally to this work and share first authorship