- 1Department of Radiation Oncology, The Affiliated Cancer Hospital of Zhengzhou University & Henan Cancer Hospital, Zhengzhou, China
- 2Laboratory of Radiation Oncology, The Affiliated Cancer Hospital of Zhengzhou University, Zhengzhou, China
The use of immune checkpoint inhibitors (ICIs) has revolutionized cancer treatment, particularly in lung cancer. However, their use in patients with pre-existing autoimmune diseases (PADs) presents unique challenges. PADs, such as rheumatoid arthritis (RA), systemic lupus erythematosus (SLE) and psoriasis, complicate the clinical management of lung cancer due to concerns about worsening autoimmune symptoms during ICI therapy. This review summarized the safety and efficacy of ICIs in lung cancer patients with PAD, focusing on the available clinical evidence, the optimal timing of ICI initiation, and the potential predictive biomarkers for immune-related adverse events (irAEs). Future prospective studies are needed to establish definitive guidelines for the use of ICIs in this population, with a focus on identifying patients at risk, managing ICI resumption after irAE and developing new medications with durable control of both cancer and PAD.
1 Introduction
Immune checkpoints (ICs) are pivotal in maintaining immune homeostasis and self-tolerance, balancing protective immunity against overactivation of the immune system. Recently, inhibitory ICs contribute to the immune escape of cancer cells and offer several therapeutic targets. Immune checkpoint inhibitors (ICIs), which target ICs, such as cytotoxic T-lymphocyte antigen 4 (CTLA-4), programmed death protein 1 (PD-1) or programmed death protein ligand 1 (PD-L1), effectively release the brakes on ICs and restore the anti-tumor immunity (1, 2).
Meanwhile, ICIs stimulate an excessive immune response, disrupt self-tolerance, and precipitate in immune-related adverse events (irAEs) that may affect nearly any organ system. The incidence of any grade irAEs ranges from 20% to 50% of patients, with most being mild to moderate in severity. Fatal irAEs are rare, occurring in 0.3-1.3% of patients, with myocarditis being the most common cause, followed by pneumonitis. Although the exact pathophysiological mechanisms remain unclear, irAEs are generally attributed to widespread immune activation, which can attack normal organs and manifest symptoms resembling autoimmune diseases (ADs) (3).
To date, the US Food and Drug Administration (FDA) has approved various PD-1/PD-L1 inhibitors and CTLA-4 inhibitors for cancer treatment, including in lung cancer patients. However, research specifically addressing cancer patients with pre-existing autoimmune diseases (PADs) remains limited. These patients are routinely excluded from clinical trials due to heightened concerns about the risk of irAEs and PAD flares.
Lung cancer is the most prevalent malignant tumor, with 14% to 25% of patients reported to have PADs at diagnosis. With the increasing use of ICIs and the significant proportion of lung cancer patients affected by PADs, it is important to assess the safety and efficacy of these treatments in this unique subgroup. While several studies have examined ICI safety and efficacy in general cancer populations (4–6), a comprehensive synthesis of recent evidence specifically focused on lung cancer remains notably absent from the literature. This gap is particularly significant given the unique immunobiological characteristics of pulmonary malignancies and their distinct response profiles to immunotherapy. While strong evidence from prospective studies is lacking, numerous case reports and retrospective studies offer limited insights into the use of ICIs in patients with PADs.
This review synthesizes current evidence on the use of ICIs in lung cancer patients with PAD, focusing on their safety and efficacy. It also explores the optimal timing for ICI administration and evaluates potential blood-predictive markers for irAEs. The review aims to deepen the understanding of ICI use in this unique population, thereby guiding treatment strategies and future research directions.
2 Lung cancer patients with PAD: epidemiology and mechanisms
The intersection of cancer and ADs represents a significant clinical challenge, with 11-25% of cancer patients having PADs (7). In lung cancer specifically, 14-25% of patients are diagnosed with PAD before cancer diagnosis (8). The bidirectional relationship stems from shared pathogenic mechanisms: dysregulated immunity promotes both autoimmunity and carcinogenesis, while immunosuppressive therapies impair tumor surveillance (9–11). Studies confirm organ-specific cancer risks associated with autoimmune and inflammatory diseases (9, 12–16), particularly for lung cancer in systemic sclerosis (SSc) (4.2-fold risk) (17), rheumatoid arthritis (RA) (1.7-fold) (13, 18), systemic lupus erythematosus (SLE) (1.6-fold) (19). Key mechanisms include immune system dysregulation, chronic tissue inflammation and damage caused by immunity overaction, immunosuppressive treatments, and the shared predisposing factors, as summarized in Figure 1 (10–13, 20–25). Even when not associated with ADs, the tumor may coexist during either the active or stable phase of the disease (26).
2.1 Dysregulated of immune system, chronic inflammation and tissue damage
ADs create a chronically inflamed microenvironment that promotes lung carcinogenesis through multiple interconnected mechanisms (Figure 2). Dysregulated immunity disrupts self-tolerance with effector T cells (Th1/Th17), B cells, and macrophages sustaining a pro-tumorigenic cascade (27).
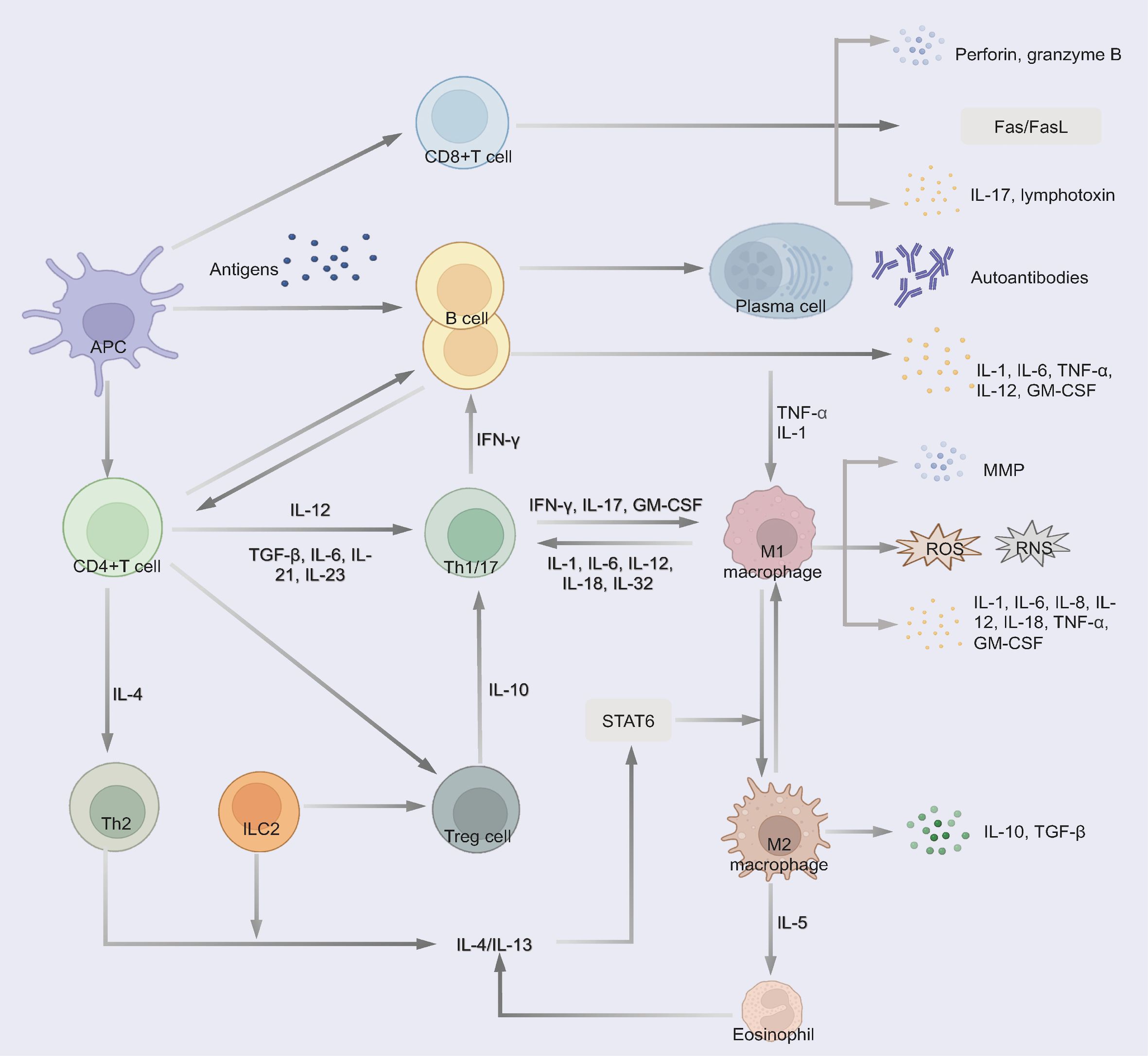
Figure 2. Immune dysregulation pathways in autoimmune diseases contributing to chronic inflammation and tissue damage. 1) CD4(+) T cells differentiate into Th1/17 cells and produce excessive pro-inflammatory cytokines. 2) Cytotoxic CD8+ T cells produce perforin and granzyme B, induce Fas/FasL-mediated cytotoxicity and the secretion of pro-inflammatory cytokines, thereby contributing to tissue damage. 3) B cells differentiate into plasma cells and secrete autoantibodies to trigger tissue damage through complement fixation and ADCC. Some B cells secrete multiple pro-inflammatory cytokines. 4) Macrophages polarize to a pro-inflammatory phenotype and release MMP, pro-inflammatory cytokines and small-molecule mediators of inflammation (such as ROS and RNS). Th2, ILC2 and eosinophil regulate the differentiation of macrophages to the M2 phenotype, which could secret anti-inflammatory cytokines. 5) Treg secret anti-inflammatory cytokines to suppress pathogenic T cells.
Th17 differentiation (driven by IL-6, TGF-β, and IL-23) and Th1 responses generate excess IL-17, IFN-γ, and TNF-α, activating macrophages and recruiting cytotoxic CD8(+) T cells and B cells (28–30). These CD8+ T cells exacerbate tissue damage via perforin/granzyme B and Fas/FasL-mediated cytotoxicity while secreting pro-inflammatory cytokines (30, 31).
B cells could contribute through autoantibodies production (via CD4+ PD1+CXCR5- cells peripheral Tph-cell) (32), triggering complement fixation and antibody-dependent cytotoxicity (ADCC) or pro-inflammatory cytokines secretion (IL-1, TNF-α) to promote inflammation, induce tissue damage, and activate oncogenic pathways (32, 33).
While macrophages release matrix metalloproteinase (MMP), pro-inflammatory cytokines (IL-1, IL-6, IL-8, etc.) and reactive oxygen species (ROS)/reactive nitrogen species (RNS) that induce DNA damage and genomic instability (34, 35). Otherwise, the induction of anti-inflammatory cytokines, such as TGF-β and IL-10, is reduced (35, 36).
M2 macrophages secrete IL-10 and TGF-β, while Th2/ILC2-derived IL-5 promotes M2 polarization, suppressing M1-mediated inflammation (33). This M1/M2 imbalance contributes to both AD pathogenesis and cancer development (37–39). Concurrently, ADs exhibit impaired Treg function, leading to defective immune regulation and uncontrolled tissue damage (39). Notably, chronic upregulation of immune checkpoints (PD-1/CTLA-4) in ADs may paradoxically facilitate immune evasion by pre-malignant cells (40, 41).
This vicious cycle of tissue damage, defective repair, and impaired surveillance creates an ideal niche for malignant transformation.
2.2 Immunosuppressive treatments
Immunosuppressive therapies play a crucial role in managing ADs but carry significant oncogenic risks. Widely used biologic agents including TNF-α inhibitors (e.g., infliximab, adalimumab) and IL-6 antagonists (e.g., tocilizumab) exert broad immunosuppressive effects that compromise critical antitumor immune mechanisms (42, 43). By inhibiting key inflammatory pathways, these treatments impair dendritic cell maturation, CD8+ T cell cytotoxicity, and NK cell surveillance - creating permissive conditions for malignant cell escape and proliferation. Clinical studies demonstrate that prolonged use (>2 years) of immunosuppressive therapies correlate with a 1.3-2.5-fold increased malignancy risk, particularly for non-melanoma skin cancers and lymphoproliferative disorders (42). This risk-benefit paradox necessitates careful monitoring of AD patients receiving chronic immunosuppressive therapy, particularly those with additional cancer risk factors such as smoking history or family predisposition (43).
2.3 Shared predisposing factors
Growing evidence highlights overlapping etiological factors between ADs and lung cancer. Genetically, shared signatures include susceptibility single nucleotide polymorphism (SNP) (rs13194781, rs1270942) linking SLE to lung carcinogenesis (44), as well as ferroptosis-related genes (FANCD2, HELLS, VLDLR) shared by RA and lung cancer (45). The immune-regulator PDE4A further demonstrates mechanistic convergence in lung cancer and multiple sclerosis (MS) (46).
Environmental and lifestyle factors synergistically promote both disease groups. Smoking, the predominant risk factor for lung cancer, concurrently activates pro-inflammatory pathways implicated in RA, SLE, SSc, and MS development (22, 47). Additional shared risks include alcohol consumption, obesity, occupational exposures (e.g., crystalline silica) (16, 18). The gut-lung axis further connects these conditions, where microbiota dysbiosis impairs immune homeostasis and facilitates IL-25-mediated recruitment of inflammatory lymphoid cells to pulmonary tissues (48–50).
Pulmonary complications of ADs substantially elevate cancer risk. Chronic inflammation in SSs and RA drives pulmonary fibrosis, a condition characterized by thickened and scarred lung tissue (13, 14), inducing hypoxia and mutagenesis that predispose to malignant transformation (23). Notably, patients with scleroderma-associated interstitial lung disease (ILD) exhibit exceptional vulnerability to lung cancer, underscoring the need for vigilant monitoring in this population (24, 25).
3 Clinical evidences for ICIs in lung cancer patients with PAD: safety and efficacy
ICIs are becoming a common and effective way to fight lung cancer through immune regulation. Up to 80% of patients treated with ICIs develop irAEs, with the first irAEs occurring 3–6 months after the start of ICI treatment (51–53). IrAEs can affect any organ but most commonly affect the skin, gastrointestinal tract, liver, lungs and endocrine glands. Although irAEs are usually mild and can be managed by clinicians, few can be life-threatening (54). The most common serious irAEs are pneumonitis and colitis, while myocarditis is the irAE with the highest mortality rate (53, 55).
Although the pathogenesis of irAEs is still not fully understood, the over-activation of immunity is very similar to ADs. IrAEs may develop through a combination of pathways involving autoreactive T cells, autoantibodies, and cytokines. Briefly, ICI therapy inhibits the combination of ICs and their gland, thus removing the inhibitory signals and causing aberrant activation of T cells, further leading to the production of autoantibodies and inflammatory cytokines, regulating immune cells and finally contributing to the development of irAEs (55, 56).
Cancer patients with PAD were thought to be at increased risk of flares or de novo irAEs following ICI treatment due to their pre-activated immune system (57) and were excluded from clinical trials. Given the increasing global incidence and prevalence of ADs worldwide, this raises important questions about the safety and efficacy of ICI treatment in these patients (58). However, their absence from clinical trials makes it difficult to consider the safety and efficacy of ICIs in this population (59).
The association of PAD with the risk of irAEs and survival has been controversial in various studies. Previous studies have shown that in patients with PAD treated with ICIs, 25-50% develop PAD flares, while approximately 16-40% develop de novo irAEs, and 24-46% discontinue ICIs due to irAEs (60, 61). Analyses of retrospective studies and the SEER database suggest that the development of flare or de novo irAEs after ICI initiation is more common in patients with PAD (62–64). A meta-analysis combining 95 studies with 23,897 patients has reported that patients with PAD were 30% more likely to report an irAE than patients without PAD (61). However, some studies have shown that there is no association between PAD and the incidence or severity of irAEs (65, 66). In terms of efficacy, some studies have even reported that patients with PAD had an increased progression-free survival (PFS) or overall survival (OS) when treated with ICIs compared to patients without PAD (63–65). In the meta-analysis, there were no statistically significant differences in cancer response to ICIs between patients with and without PAD (61).
A meta-analysis focusing on lung cancer included 250 non-small cell lung cancer (NSCLC) patients with PAD from 9 cohort studies (67). The incidence of PAD flare was 23% (95% CI: 9%-40%). PAD was associated with an increased risk of any grade and grade 3–4 de novo irAE. More discontinuations were observed in patients with PAD (10% vs. 3%). PAD was also associated with a better tumor response (complete response/partial response). However, this meta-analysis included three studies with interstitial lung disease (ILD) patients, those who may not be AD patients. Due to the small sample size and a high heterogeneity, the safety and efficacy of ICIs in lung cancer with PAD may be different from the results in all cancer populations. Without sufficient clinical evidence, it is difficult for clinicians to make decisions about how to treat this unique patient population.
To illustrate the use of ICI in lung cancer patients with PAD, we conducted a comprehensive search of MEDLINE, EMBASE, Web of Science, PubMed, and the Cochrane Library databases for studies of NSCLC patients with PAD who received ICI treatment. After removing studies with no usable data and duplicate studies, we identified 19 retrospective studies (68–87). We did not include the case reports or case series to avoid bias. Eleven studies are specific to lung cancer (68, 69, 72, 73, 75, 77, 79, 81–84, 87), and seven studies have more than one type of cancer from which we can get data on lung cancer (70, 71, 74, 76, 78, 80, 85, 86). Of these, 13 studies with 468 patients were able to provide safety data and 15 studies with 997 patients provided efficacy data. We have summarized the safety and efficacy data in Table 1.
The majority of patients had NSCLC, with only two patients having small cell lung cancer. The ICIs used in these studies include PD-1 inhibitors (nivolumab, pembrolizumab), PD-L1 inhibitors (atezolizumab, durvalumab), CTLA-4 inhibitor (ipilimumab), and the combination. In the 190 patients with detailed PAD data from seven studies (68, 70, 76, 79, 84, 86, 87), we find 50.0% of patients have rheumatological PAD, followed by endocrine PAD (18.4%), dermatological PAD (14.7%) and neurological (7.9%). RA is the most common PAD, occurring in 25.8% of patients (49/190), followed by psoriasis and thyroiditis. The grade ≥3 irAEs are colitis (n=1), diarrhea (n=1), hepatitis dysfunction (n=2), and pneumonitis (n=1).
3.1 Safety of ICIs in lung cancer patients with PAD
The rate of PAD flares was reported in 11 studies, ranging from 5.9% to 33.3%, and the incidence of grade ≥ 3 flares was 0-10.0%. The incidence of de novo irAEs of any grade in the 12 included studies ranged from 12.5% to 90.0%, with only two studies reporting an incidence greater than 50%. The incidence of grade ≥ 3 de novo irAEs was reported in 3.3%-26.0% of patients in seven studies and was less than 10.0% in 50.0% of studies. Permanent discontinuation of ICIs due to flares or de novo irAEs was reported in 6.7-38.0% of patients from six studies.
Some studies have reported that the risk and severity of irAEs in patients with PAD are similar to those in the overall population (78, 81). Higgins et al. reported that the PAD group tended to have a higher incidence of irAEs (42.9% vs. 32.7%), although not statistically significant (p = 0.130) (82). The incidence of grade ≥ 3 irAEs was reported to be unaffected by PAD (79, 87). Two studies even reported a lower incidence of grade ≥ 3 irAEs in the PAD group, although the difference was not significant (82, 83).
Any grade and grade ≥ 3 flare/de novo irAEs were not increased compared to patients without PAD. Ansel et al. showed that low-grade adrenal insufficiency was the only irAE that occurred significantly in the PAD group (p = 0.02) (84). Ardizzoni et al. found a moderate increase of respiratory or gastrointestinal AEs in patients with PAD (81). Ohe et al. reported that the incidence of grade ≥ 3 irAEs was higher in patients with a history of ILD (18.4% vs. 9.4%, p = 0.0056) or current ILD (24.6% vs. 8.9%, p < 0.0001) compared to the patients without ILD (83).
So far, based on the limited data, ICI treatment appears to be relatively safe for lung cancer patients with PAD. Larger trials could provide more details about which patients need to be monitored closely and what AEs may occur.
3.2 Efficacy of ICIs in lung cancer patients with PAD
Two multicenter studies reported that ICIs were beneficial in lung cancer patients with PAD compared with non-ICI treatments (87, 88). Among the included studies, the overall response rate (ORR) ranged from 22.0% to 71.4% in six studies, and the disease control rate (DCR) ranged from 50.0% to 96.0% in five studies. Median PFS ranged from 2.9 to 16.5 months in eight studies, and median OS ranged from 8.5 to 29.1 months in ten studies.
Ardizzoni et al. reported a slightly shorter median OS in the PAD group compared with patients without PAD (10.1 vs. 11.2 months) (81). Most studies reported that the presence of PAD did not affect survival (68, 72, 79, 88). Higgins et al. even showed that patients with PAD tended to have longer median PFS (5.5 vs. 3.5 months, p = 0.8551) and median OS (17.2 vs. 14.4 months, p = 0.991) (82). Data are still limited to answer the question of the efficacy of ICIs in lung cancer patients with PAD.
3.3 Combination therapy: ICI and chemotherapy
Asao et al. included 69 NSCLC patients with PAD and compared the safety and efficacy of ICI monotherapy and the combination with cytotoxic chemotherapy (87). PAD flares were more frequent with combination treatment than with ICI monotherapy, although the difference was not significant (35.7% vs. 22.8%, p = 0.32). There were no significant differences in the incidence of de novo irAEs between combination treatment and ICI monotherapy (combination vs. monotherapy: all-grade irAEs: 50.0% vs. 43.9%, p = 0.68; grade ≥ 3 irAEs: 21.4% vs. 24.6%, p > 0.99). PAD exacerbations were more likely when NSCLC was diagnosed within one year after the diagnosis of AD (p = 0.016). When adjusted by inverse probability weighting, the use of ICI could prolong survival in NSCLC patients with PAD (p = 0.0006).
Although the rates of flares and irAEs tended to be higher with combination therapy, the differences were not significant, supporting the feasibility of combining ICI and chemotherapy in patients with NSCLC and PAD. Physicians need to be cautious when treating patients within a short time after diagnosis of PAD.
3.4 Dual therapy: combination of PD-1 and CTLA-4 inhibitors
Reid et al. enrolled 25 lung patients treated with PD-1 inhibitors and 16 lung patients received the combination of PD-1 and CTLA-4 inhibitors (85). The dual therapy showed an increased incidence of PAD flares, and any grade/high grade de novo irAEs compared to PD-1 inhibitor alone, but was not significantly different (dual therapy vs. monotherapy: flares: 18% vs. 23%, p = 0.36; any grade de novo irAEs: 71% vs. 27%, p = 0.12; high grade de novo irAEs: 26% vs. 4%, p = 0.17).
Thus, the combination use of PD-1 and CTLA-4 inhibitors does not increase the risk of flare or de novo irAEs and could be used in lung cancer patients with PAD.
3.5 The association between irAEs and clinical outcomes
Recently, it has been suggested that irAEs may be associated with therapeutic response and may serve as survival predictor. Tison et al. found that flare/de novo irAE was associated with prolonged PFS in cancer patients with PAD treated with ICIs (p = 0.026) (73). In addition, Fukihara et al. reported that pneumonitis is associated with poor outcomes in NSCLC patients treated with PD-1 inhibitors (89). However, Cytryn et al. reported no statistically significant association between survival and the presence of irAE (70). The relationship between irAEs and clinical response has not been fully investigated. Further studies are needed to evaluate the correlation between the flares, irAEs, their severity, organ site and efficacy (90).
These studies have shown that ICIs are effective, often leading to durable responses with tolerable toxicity. Physicians should consider using ICIs in lung cancer patients with PAD. Combining an ICI with chemotherapy or using two types of ICI is also acceptable. Notably, the ongoing NCT03816345 phase Ib trial (National Cancer Institute (NCI)-sponsored) aims to enroll 300 patients with advanced cancer and PADs (RA, SLE, inflammatory bowel disease (IBD), SSc, psoriasis, etc.) to evaluate nivolumab safety and biomarkers (completion: 2026-08-31). This may provide the first high-quality data on irAE patterns in specific PADs (91). The use of ICIs in this population remains challenging and further prospective, controlled studies are needed.
4 Optimal timing of ICI treatment in lung cancer patients with PAD
To determine the optimal timing of ICI treatment, we need to balance clinical benefit and toxicity. Because of the underlying mortality associated with cancer, the short-term risk of death from cancer may outweigh the risk of worsening of AD or induction of irAEs. Patient assessment by a multidisciplinary team is required to make clinical decisions.
4.1 PAD status
We focused on the relationship between PAD status and the safety of ICI use. Patients can be divided into active status and inactive status. Leonardi et al. reported on 56 NSCLC patients with PAD who were treated with PD-1 inhibitors, and the patients with active PAD were the majority of those who experienced flares (7). Cortellini et al. showed that inactive patients had fewer irAEs and a better prognosis in cancer patients treated with PD-1 inhibitors compared to patients with active PAD (66). Two meta-analyses reported no differences in the presentation of flares/irAEs and ORR between patients with active and inactive PAD (92, 93). Khozin et al. also found there was no association between PAD status and outcomes in NSCLC patients but reported higher rates of selected AEs, including endocrine, gastrointestinal and hematological disorders, in patients with active PAD (72).
Therefore, it is generally safe to receive ICIs when PAD is well controlled. For those whose disease is not yet under control, there is a high likelihood of flares/irAEs. Disease status must be considered and carefully evaluated before starting treatment and during ICI therapy.
4.2 Baseline use of immunomodulatory medications
The management of immunomodulatory medications prior to ICI initiation requires careful consideration of both AD control and potential impacts on cancer outcomes. These agents can be categorized into three clinically relevant classes: (1) corticosteroids, (2) conventional disease-modifying antirheumatic drugs (DMARDs; e.g., methotrexate), and (3) biologics/targeted therapies (e.g., TNF inhibitors, IL-6 antagonists) (94). Current evidence suggests differential effects based on medication class.
Cytryn et al. (70) demonstrated that overall immunomodulator use at ICI initiation was not associated with increased AD flares/irAEs or survival differences, while Leonardi et al. (7) specifically found no association between immunomodulators (including both corticosteroids and steroid-sparing agents) and ICI response rates (p = 0.66). The meta-analysis found that these agents may paradoxically reduce severe irAE risk (29.4% vs 70.0%, p = 0.007) while maintaining comparable overall immunotoxicity profiles (43.2% vs 58.3%, p = 0.148) (95).
However, corticosteroid-specific analyses reveal significant concerns; Fountzilas et al. (78) reported significantly poorer PFS in patients receiving baseline corticosteroids (p = 0.003), a finding supported by two additional studies (73, 85) and confirmed in a recent pooled analysis of six clinical trials by Verheijden et al. (96), which showed reduced objective response rates (ORR: 28% vs. 42%) and shorter PFS (HR: 1.54) with corticosteroid use for treatment-related adverse events (trAEs).
Data on DMARDs and biologics remain limited but suggest intermediate effects. While they appear less detrimental than corticosteroids for ICI efficacy, their immunomodulatory mechanisms may still partially interfere with checkpoint blockade. Notably, the reduced severe irAE risk seen with immunosuppressive therapy overall may be particularly relevant for this class (95).
These findings indicate that while baseline immunomodulators—especially corticosteroids—may reduce ICI efficacy, they do not elevate flare risk and could potentially decrease severe immune-related toxicity. Consequently, clinical practice should prioritize: (1) initiating ICIs during AD quiescence, (2) minimizing pre-ICI corticosteroids to <10 mg/day prednisone-equivalent (preferably ≤7.5 mg/day for maintenance therapy (97), and (3) favoring steroid-sparing immunomodulators when continuous treatment is required. This strategy achieves optimal balance between preserving antitumor immunity and maintaining AD control.
4.3 PAD Type
Different PADs may pose different risks to patients receiving ICI treatment. Previous studies have found that flares are more common in patients with pre-existing psoriasis/psoriatic arthritis (39%), IBD (37%) and RA (36%) (63). Gutzmer et al. noted that PAD flares appeared to be more common in patients with rheumatological PAD and psoriasis (98). Alexander et al. found that patients with rheumatological PAD had a significantly higher incidence of flares than patients with non-rheumatological PAD (74). Patients with RA also have a higher incidence of all-grade and severe irAEs (65).
In our review, 50.0% of patients have rheumatological PAD, followed by endocrine PAD (18.4%), dermatological PAD (14.7%) and neurological (7.9%). RA is the most prevalent PAD, occurring in 25.8% of cases (49/190), followed by psoriasis and thyroiditis.
RA patients could be well-controlled by DMARDs, biologics, or low-dose steroids (99, 100). So, ICIs could be started in these stable patients stopped their DMARDs or controlled by low-dose. If RA flares occur post-ICI, they typically present as joint pain, swelling, or stiffness but are rarely severe or life-threatening. Flares can often be managed with corticosteroids, nonsteroidal anti-inflammatory drugs (NSAIDs), or escalation of RA therapy without requiring ICI discontinuation and did not appear to negatively impact the tumor response to immunotherapy (101, 102).
Psoriasis is often mild-to-moderate and well-controlled with topical agents, phototherapy, or systemic therapies (e.g., methotrexate, biologics) (103). ICI-induced psoriasis flares usually manifest as worsening skin plaques or new-onset psoriatic lesions, but severe cases (e.g., erythroderma, pustular psoriasis) are rare. Most flares respond to topical steroids, methotrexate, or biologics without needing ICI cessation (6, 103, 104).
Patients with thyroiditis always have stable thyroid function on hormone replacement (levothyroxine) or antithyroid medications. ICIs commonly induce hypothyroidism (more frequent) or transient hyperthyroidism, but thyroid storms or life-threatening dysfunction are rare (93). Monitoring TSH/T4 and adjusting thyroid medications usually suffice (105).
Since ICIs may be the most effective treatment option for advanced lung cancer, and even the only choice for those intolerance to chemotherapy and unsuitable to target treatment, the benefits often outweigh the risks of RA exacerbation, particularly when PADs are manageable with low-dose of medications. It is importance to individually assess the risk-benefit rather than blanket avoidance. Thus, physicians should consider the type of PAD when deciding whether to treat with ICIs, especially rheumatological PAD. Cancer patients with rheumatological PAD may require careful stabilization and possibly delayed use of ICIs.
4.4 ICI type
PAD flares were increased in patients receiving PD-1/PD-L1 inhibitors, while de novo irAEs were observed more frequently in patients receiving CTLA-4 inhibitors (95). Postow et al. reported that colitis and hypophysitis seem to be more common with CTLA-4 inhibitors, whereas pneumonitis and thyroiditis are more common with PD-1 inhibitors (51).
The risk of irAEs was lower in patients on monotherapy compared to combination therapy (85, 95). Reid et al. reported that patients with gastrointestinal PAD were more likely to experience flares with combination ICI therapy, and patients with dermatological or endocrine PAD had a lower incidence of de novo irAEs with monotherapy (85).
Therefore, physicians should be cautious when using a combination of PD-1/PD-L1 inhibitors and CTLA-1 inhibitors in patients with gastrointestinal, dermatological or endocrine PAD.
4.5 Cancer stage and progression
Patients with advanced or rapidly progressing lung cancer may need earlier ICIs to effectively control disease progression. In early, slow-progressing cases with PAD, delaying ICIs until the PAD is better controlled may be a safer approach (106).
In conclusion, the optimal timing of ICIs in lung cancer patients with PAD should be personalized based on the status and type of PAD, the use of immunoregulatory treatment, the type of ICI used, and the stage of lung cancer. It is generally recommended to start ICI treatment when PAD is stable. Minimal immunosuppression at the initiation of ICI must be achieved by stopping or minimizing the dose of medications in quiescent patients. It is important to use low-dose immunosuppression treatment to prevent flares and to avoid further delay of ICI initiation in some patients. The timing of ICI can be complicated by the high heterogeneity of PAD. Experienced multidisciplinary team discussions, involving oncologists, rheumatologists, and other specialists, are essential for optimal timing and management.
5 Predictive biomarkers of irAEs after ICI initiation
PAD flares or irAEs generally improve with the discontinuation of ICIs with or without the administration of immunosuppressive therapy. However, several case reports raise concerns about the potentially irreversible morbidity of irAEs. Prediction and early recognition are crucial to optimizing therapy, avoiding discontinuation of ICI, and preventing morbidity and mortality. Currently, there is no reliable biomarkers correlating with risk of irAEs. Considering blood as a non-invasive and convenient origin for tests. We focused on potential blood biomarkers based on cancer patients without PADs and summarized the advances.
5.1 Autoantibodies
Autoantibodies detected at the baseline and during treatment in cancer patients treated with ICI may predict the incidence of irAE (107). Sakakida et al. reported that positive anti-nuclear antibody (ANA) was associated with a higher risk of ICI-mediated colitis, but not with classic ANA-associated ADs such as SLE and scleroderma (108). Osorio et al. found that the baseline levels of anti-thyroglobulin and anti-thyroid peroxidase antibodies were higher in NSCLC patients who developed thyroiditis after the PD-1 inhibitor treatment (109). In a retrospective study of NSCLC patients treated with PD-1 inhibitors, pre-existing ANA, rheumatoid factor, anti-thyroglobulin and anti-thyroid peroxidase antibody positivity correlated with the development of irAEs, but also with clinical benefit from ICIs (110).
Another study reported that patients who experienced irAEs had a low baseline autoantibody level, which increased significantly after initiation of ICI therapy (111). This may suggest that the dynamic change may be more useful for the early recognition of irAEs.
The likely mechanism is that PD-1/PD-L1 blockade or deletion of PD-1 can led to increased B cell proliferation and antibody response to T cell-independent antigens (112), resulting in autoantibody expansion and subsequent irAE development. Monitoring the dynamic change of relevant autoantibodies at baseline will help to identify high-risk patients and detect the early onset of irAEs during ICI treatment.
5.2 Cytokines
As irAE is a result of over-activation of the immune system, baseline and dynamic changes in cytokines have been extensively studied to predict irAE. Lower baseline levels of TNF-α, IL-6, IL-8, IL-15, CXCL9, CXCL10, CXCL11, and CXCL19 and higher baseline levels of IL-17 or IL-6 are associated with a high risk of irAE (113–117). High levels of IL-17 (114) and IL-6 (115) were also found to correlate with high-grade irAEs. Baseline Ang-1 (p = 0.005) and CD40L (p = 0.006) were significantly increased in patients who developed dermatitis, compared to those who did not. Patients who developed pneumonitis had significantly elevated baseline IL-17 (p = 0.009) and trends towards decreased baseline IL-8 (p = 0.06) and IL-15 (p = 0.06). In patients who developed colitis, there was a trend towards decreased baseline GCSF (p = 0.08) (116).
Significant increases in the levels of IFN-γ, IL-6, GM-CSF, CCL5, CXCL9, and CXCL10 levels after treatment are associated with irAE (113, 115, 118, 119). Elevated levels of IL-6 are associated with severe irAEs, particularly colitis (115). IL-17 has been observed to be elevated in patients with ipilimumab-induced colitis (120). Significant downregulation of MICA, CX3-CL1 or VEGF-A is associated with dermatitis (116). Patients who developed pneumonitis had significant upregulation of MICB, IL-2, IL-17, IFN-γ and CCL4 (116).
5.3 Gene expression
Whole blood could provide gene expression information that associated with the risk of irAE. The SNP rs2910164 at baseline and during ICI therapy, which leads to reduced miR-146a expression, was associated with an increased risk of developing severe irAEs, and reduced PFS (121). Other SNPs, such as rs11743438 and rs3026321, mapped to genes that associated with inflammation and ADs and led to the development of irAEs (122). Friedlander et al. used whole-blood RNA transcript-based gene signatures and reported a 16-gene signature panel to detect severe colitis/diarrhea in patients with advanced melanoma treated with the CTLA-4 inhibitors (123). When using the next-generation sequencing technique to test the circulating tumor DNA in blood samples, the baseline expression of several immune-related genes, including CD3E, CD37, CD4, and IL-32, is associated with increased gastrointestinal irAEs. In particular, increased expression of CD177 and CEACAM1gene, two neutrophil-activation markers, were closely associated with gastrointestinal irAEs and early predictors (124). Researchers found gastric cancer patients with alterations in CEBPA, FGFR4, MET, or KMT2B gene had a greater likelihood of irAEs (p = 0.09) (125).
Since T and B lymphocytes are also important mediators of immune tolerance and play a crucial role in the occurrence of irAEs. Peripheral CD8+ T cell clonal expansion has been found to correlate with the development of severe irAEs in cancer patients treated with CTLA-4 inhibitors (126, 127).
5.4 Routine blood count
Routine blood count could provide information about immune conditions and serve as valuable indicators of irAE risk. High absolute lymphocyte count, low absolute neutrophil count, low absolute monocyte count, and low neutrophil-to-lymphocyte ratio (NLR) before and during ICI treatment are associated with irAE onset in cancer patients with ICI treatment (128). Pavan A et al. reported that low NLR and platelet-to-lymphocyte ratio (PLR) at baseline were significantly associated with the development of irAEs (129). A high absolute lymphocyte count has been associated with an increased risk of irAEs, possibly due to increased immune reactivity (130). A high level of baseline absolute eosinophil count is an indicator of ICI-associated pneumonitis (130). Increased total white blood cell count and decreased relative lymphocyte count are associated with severe irAEs or lung/gastrointestinal irAEs (131) and a significant increase of C-reactive protein is an early marker of irAE (132).
In summary, some results of the current studies are controversial, further research is needed to validate these biomarkers in large prospective trials and to develop predictive panels to stratify patients by risk in cancer patients with PAD undergoing ICI therapy.
6 Safe use of ICIs in lung cancer patients with PAD
6.1 Improving the treatment strategies
Oncologists should be familiar with the manifestations of ADs, risk factors for irAEs, and related therapeutic medications. A multidisciplinary team including rheumatologists, gastroenterologists, endocrinologists, neurologists and dermatologists is essential (56). Before ICI treatment, physicians must perform a baseline medical history, physical examination, appropriate laboratory tests, and imaging tests to assess the risk of flares/irAEs and determine the optimal timing of ICIs based on the cancer stage, PAD status (active/inactive) and type (mild/life-threatening), and the use of immunoregulatory treatments. Predictive biomarkers can be used to determine the likelihood of irAEs and the possible irAEs, and may also aid in early detection and intervention, which could reduce the duration and severity of irAEs (133).
Physicians need to discuss the symptoms and signs of possible irAEs with patients and their families. Education and communication will enable patients to recognize irAEs early and seek timely medical help, especially when patients are discharged from hospital. As there are no clear recommendations available, we recommend a treatment process for lung cancer patients with PAD is illustrated in Figure 3, the strategy may vary depending on the type of pre-existing autoimmune disease.
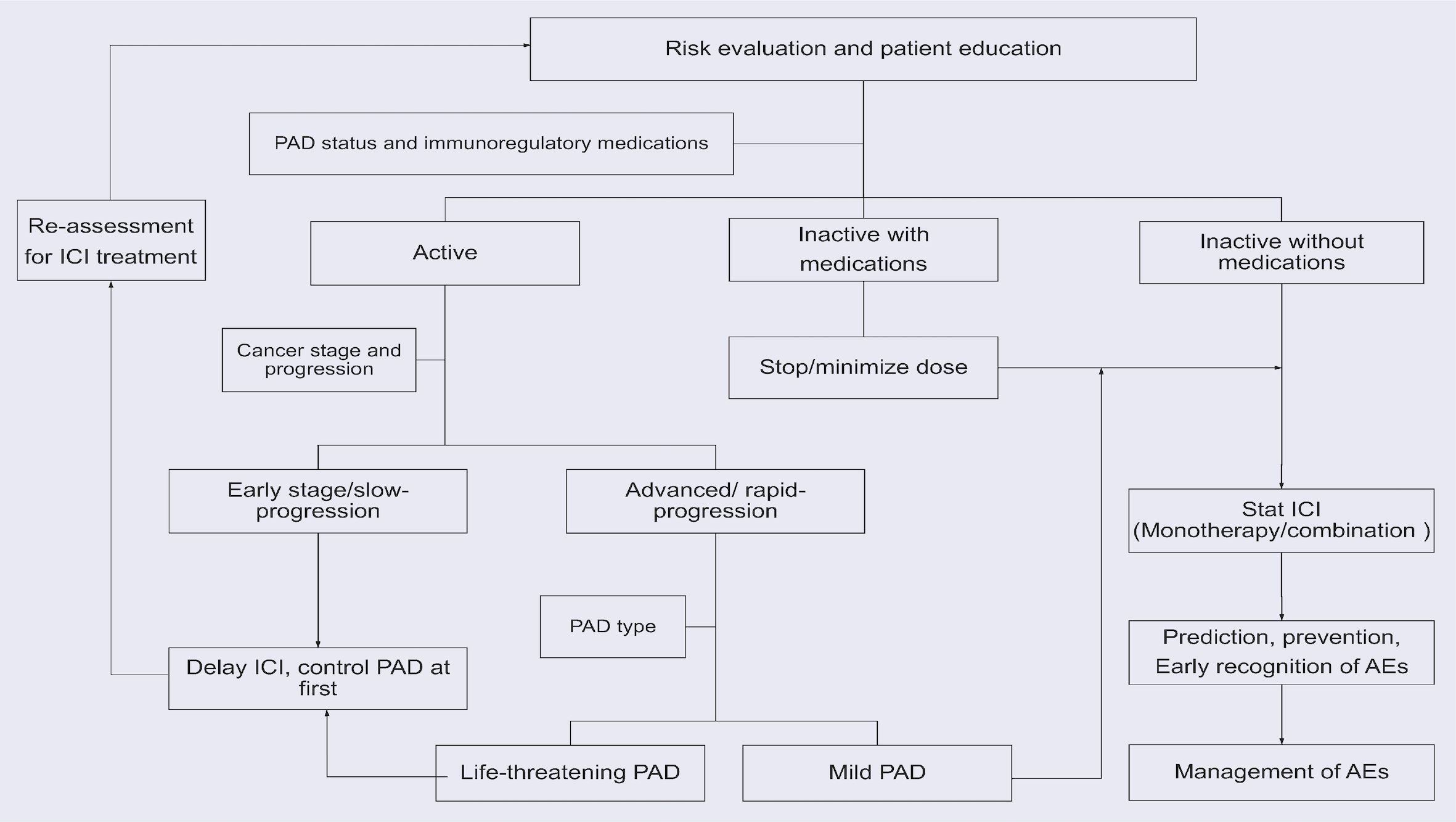
Figure 3. The recommended treatment process for lung cancer patients with PADs. The strategy may vary depending on the type of PADs.
In inactive patients, ICI can be started after discontinuing or minimizing the use of immunoregulatory therapy. The type of ICI or its combination with chemotherapy or a different type of ICI should be discussed personally.
In patients with active PAD, clinicians should weigh the potential benefits of ICIs against the possible AEs. In advanced cancer patients with rapid progression, there may be no other options other than ICIs. If the PAD is mild with acceptable possible AEs, ICI should be used with close monitoring. If the PAD is also severe with life-threatening symptoms, proper control is required before immunotherapy can be started. For active patients with early or slowly progressing cancer, it’s better to delay the use of ICIs and control the PAD at first. Then, re-evaluate the possibility of using ICIs and use ICIs if the PAD becomes inactive.
Once ICI treatment is started, it is important to conduct regular examinations. Abnormal findings on relevant tests will help in the early detection of flares/irAEs. The management of irAEs in patients treated with ICIs should always be considered. There is no specific guideline for ICI treatment in cancer patients with PAD; we refer to the recommendations of the National Comprehensive Cancer Network (NCCN) (97), the Society for Immunotherapy of Cancer (SITC) (134, 135), the American Society of Clinical Oncology (ASCO) (136, 137), and the European Society for Medical Oncology (ESMO) (138) for the irAE management recommendations. IrAEs are graded from 1 to 5 according to the severity based on the Common Terminology Criteria for Adverse Events (CTCAE). Briefly, discontinuation of ICIs is not necessary for grade 1 irAEs if close monitoring is feasible, whereas temporary withholding of ICIs and moderate systemic corticosteroids should be considered for most grade 2 toxicities. ICIs are resumed when toxicity decreases to grade 1 or symptoms resolve. For grade 3 irAEs, ICIs should be discontinued and high-dose corticosteroids should be started and tapered over 4–6 weeks. If symptoms worsen, steroid-sparing biologic immunosuppressants such as TNF-α inhibitors or IL-6 antagonists should be considered. For grade 4 irAEs, permanent discontinuation of ICIs is recommended. With proper management, most irAEs in cancer patients with PAD resolve, and only few patients discontinue using ICIs.
6.2 Managing the resumption of ICIs after flares/irAEs
When the flares/irAEs improve with treatment, the physicians will consider resuming ICI treatment. Here we summarize the previous research and try to make some suggestions.
Allouchery et al. reported 180 patients from the French pharmacovigilance database who experienced at least one grade ≥2 irAE leading to ICI discontinuation; 41.4% were lung cancer patients (139). 61.1% of patients had no grade ≥2 irAEs after ICI resumption. Among patients who experienced a recurrent irAE, 70% had their initial irAE, 25.7% had a de novo irAE, and 4.3% had both. Most patients (68.6%) required corticosteroids, and 8.6% required immunosuppressive medications. No deaths related to recurrent irAE were reported, and 76.6% of second irAEs resolved to grade 1 or lower (139).
There is a lack of data on the risk factors associated with irAE recurrence. Allouchery et al. found that initial gastrointestinal irAEs were more likely to recur (p = 0.007), whereas initial endocrine irAEs were associated with lower rates of irAE recurrence (p = 0.003) (139). Dolladille et al. reported that colitis (p = 0.01), hepatitis (p = 0.01), and pneumonitis (p = 0.01) were associated with a higher recurrence rate, whereas adrenal events were associated with a lower recurrence rate (p = 0.03) (140). However, Pollack et al. reported that colitis/diarrhea recurred less frequently than other irAEs (6% vs. 28%, p = 0.01) (141).
Abu-Sbeih et al. reported a multicenter retrospective study focusing on patients treated with ICI treatment after the onset of immune-mediated diarrhea and colitis (IMDC) (142). IMDC recurred in 34% of patients; 81% of the recurrences required corticosteroid therapy and 12% required the addition of infliximab or vedolizumab. IMDC recurred in 44% of patients who resumed CTLA-4 inhibitors and in 32% of whom resumed PD-1/L1 inhibitors. Multivariate analysis showed that risk factors significantly associated with IMDC recurrence were the initial need for immunosuppressive therapy (p = 0.019) and the longer duration of initial IMDC symptoms (p = 0.031) (142). Initial use of PD-1/L1 inhibitors was higher risk of IMDC recurrence than CTLA-4 inhibitors (p = 0.002). However, PD-1/L1 inhibitor resumption had lower risk of recurrence than CTLA-4 inhibitors resumption, regardless of the type of initial ICI (p = 0.019) (142). Pollack et al. studied 80 patients who discontinued PD-1 and CTLA-4 inhibitor combination therapy due to irAEs. Of these, 96% received corticosteroids and 21% received additional immunosuppressive treatment. All patients were resumed on PD-1 inhibitors, and patients with initial colitis or hypophysitis could safely resume PD-1 inhibitors (141). However, Allouchery et al. reported that the resumption of the same ICI treatment was associated with a lower rate of irAE recurrence (77.1% vs. 90%, p = 0.02) (139).
The reported duration before resumption ranged from 0.9 to 6.3 months (139–145). Sminonaggio et al. reported that a shorter time to the first irAE was associated with the occurrence of a second irAE (9 vs. 15 weeks, p = 0.04) (143). However, Allouchery et al. reported that the duration from ICI discontinuation to resumption and irAE severity did not predict recurrent irAEs (p = 0.53 and p = 0.40, respectively) (139).
To investigate the efficacy of resuming ICI retreatment, Santini et al. retrospectively analyzed 68 NSCLC patients with severe irAEs requiring interruption of PD-L1 inhibitor (144). Among them, 56% of patients resumed PD-L1 inhibitors, and 44% were permanently discontinued. In the retreatment cohort, 48% of patients had no irAEs, 26% had initial irAEs recurrence, and 26% had de novo irAEs. Most recurrences/de novo irAEs were mild (58% were grade 1-2) and controllable (84% resolved or reduced to grade 1). For those without an observed partial response before the irAE, PFS and OS were longer with ICI resumption. Conversely, in those who had an objective response before irAE, PFS and OS were similar with or without resumption of ICI. Therefore, patients who had no treatment response prior to irAE onset may be more need in ICI resumption. Similarly, another retrospective analysis of melanoma patients treated with nivolumab and ipilimumab showed that patients could continue to benefit from previous immunotherapy even after the discontinuation due to irAEs (145).
Overall, the retrospective design, heterogeneity of cancer type, irAE and ICI treatment, and small sample sizes of the studies make it difficult to conclude resumption after irAEs. AE recurrences are mostly low-grade and manageable, but it remains controversial whether there is any additional benefit from continuing ICI after irAE. The risk-benefit ratio of resumption should be considered personally. It is challenging but necessary to look for specific predictive risk factors for irAE recurrence.
6.3 Prevention of flares/irAEs with selective immunosuppressive medications
There are no successful strategies for preventing irAEs. Previous studies mentioned that selective antibody therapy, such as anti-IL-6, anti-CD20, and anti-TNF therapy, is effective in treating irAEs or preventing the flares and maintaining the clinical benefit of ICIs (146–148). This increased our interest in therapies that could control PAD and alleviate cancer at the same time.
Many medications for ADs also have anti-tumor activity. Janus kinase/signal transducer and activator of transcription (JAK/STAT) signaling regulates the secretion of various cytokines, which play a central role in the development of ADs and tumorigenesis (149). Inhibition of JAK/STAT pathways by JAK inhibitors alleviates ADs by decreasing cytokines secretion. Moreover, JAK inhibitors have the potential to counteract tumorigenesis by reversing drug resistance, inducing G2 arrest, and augmenting apoptosis (150).
In a phase II clinical trial, 21 treatment-naïve metastatic NSCLC patients with tumor PD-L1 ≥50% were treated with pembrolizumab and delayed itacitinib (a JAK1 inhibitor) (151). Patients received pembrolizumab until disease progression, and two cycles of itacitinib were added on cycles 3 and 4. The 12-week ORR was 62%, and the best overall response (BOR) after 12 weeks was 67%. After a median follow-up time of 27.6 months, the median PFS was 23.8 months. Response assessment at 6 weeks showed that five patients had an early response to pembrolizumab before itacitinib. In contrast, eight patients who failed to respond or progress after initial pembrolizumab were able to respond after itacitinib. Hence, patients who failed to respond to initial PD-1 inhibitor could still achieve a high response rate with the addition of a JAK inhibitor in NSCLC patients with tumor PD-L1 ≥50%. Itacitinib promoted CD8 T cell plasticity and therapeutic responses of exhausted and effector memory-like T cell clonotypes. Thus, it may enhance the efficacy of PD-1 inhibitors by altering the dynamics of T-cell differentiation.
6.4 Reduce toxicities via development of next-generation ICIs and drug-delivery system
Recent advances in protein engineering and drug delivery technologies are addressing the critical need to improve the safety profile of ICIs, particularly for cancer patients with PADs.
To enhance selectivity and minimize toxicity, protein engineered next-generation ICIs are being developed with optimized pharmacokinetics, including longer half-lives, greater stability, and more controlled activation (152). One strategy is to modify the Fc region of ICIs to reduce binding to Fcγ receptors and minimize off-target immune activation of macrophages or dendritic cells (153). For example, IgG4 isotype ICIs have lower Fc effector function than IgG1 type (154). Second, tumor-specific bispecific antibodies are designed with increased affinity to target tumor cells while sparing normal tissues. It could target ICIs specifically to tumors by conjugating to tumor antigen-binding domains (e.g., Anti-PD-1/Her2 Bispecific Antibody IBI315) (155). Third, modified immune checkpoint proteins are used to make ICIs inactive until conditionally active in the tumor microenvironment (TME). For example, CX-072 (a PD-L1 inhibitor) has protease-cleavable masking and is only activated by tumor-associated proteases (156). pH low insertion peptide (pHLIP) modified PD-L1 has pH-sensitive design and suppresses T-cell activation in the acidic TME (157). Hence, pHLIP modified ICIs could remaining less active in normal tissues and potent in the acidic TME. Selective targeting reduces off-target effects and minimizes the risk of irAEs, paving the way for safer cancer immunotherapies for cancer patients with PAD.
Otherwise, specific drug delivery systems are used for safer ICI therapy. Encapsulation of ICIs in nanoparticles, which release medications preferentially in the TME with the design of pH-, redox-, or enzyme-responsive, makes the medications tumor-targeted and reduces broad immune activation (158, 159). Cell membrane-coated nanoparticles use autologous immune cell membranes (e.g., T cell membranes) to improve tumor homing and reduce off-target effects (160).
Intriguingly, irAEs can be prevented to some extent by modifying ICIs so that they are only active within the TME, or restricting their delivery to the TME.
7 Conclusion
The increasing prevalence of PADs among lung cancer patients presents both challenges and opportunities for the use of ICIs. Our comprehensive review addresses critical gaps in the existing literature by incorporating the most recent clinical evidence (2021-2024) that was absent from previous reviews limited to pre-2021 data and predominantly melanoma-focused studies. The unique value of our work lies in several key contributions. First, we provide a mechanistic understanding of the relationship between autoimmune diseases and lung cancer, particularly examining how dysregulated immunity, chronic inflammation, and tissue damage in autoimmune conditions may contribute to oncogenesis. Second, we offer a comprehensive synthesis of clinical evidence regarding ICI safety and efficacy specifically in lung cancer patients with autoimmune comorbidities, including analysis of combination therapies with chemotherapy or dual ICIs. Third, our review advances translational research by exploring the relationship between immune-related adverse events and clinical outcomes, while discussing potential predictive biomarkers for risk stratification. We also develop a practical clinical decision framework to guide optimal timing and use of ICIs in this complex patient population. When considering ICIs, it’s important to weigh the potential benefits against the risks of exacerbating PAD or de novo irAEs. This balance may differ depending on the PAD activity, use of immunosuppressive treatments, type of PAD, ICI and cancer.
In conclusion, although ICIs present an exciting therapeutic option for lung cancer patients with PADs, their use requires individualized treatment strategies with multidisciplinary management. It is acceptable to use ICIs in patients with a low risk of irAE after informing patients of involved irAEs and monitoring them closely. Early recognition and timely intervention for irAEs can help ensure safer ICI administration. The resumption of ICI after irAEs is another clinical question that remains to be answered. Prevention of flare/irAE with selective immunosuppressive medications is an important area of future research.
Author contributions
HY: Conceptualization, Data curation, Investigation, Visualization, Writing – original draft, Writing – review & editing. YS: Data curation, Investigation, Visualization, Writing – original draft. HG: Funding acquisition, Supervision, Writing – review & editing.
Funding
The author(s) declare that financial support was received for the research and/or publication of this article. This work was supported by funding from the Major Science and Technology Projects in Henan Province (No. 221100310100).
Conflict of interest
The authors declare that the research was conducted in the absence of any commercial or financial relationships that could be construed as a potential conflict of interest.
Generative AI statement
The author(s) declare that no Generative AI was used in the creation of this manuscript.
Publisher’s note
All claims expressed in this article are solely those of the authors and do not necessarily represent those of their affiliated organizations, or those of the publisher, the editors and the reviewers. Any product that may be evaluated in this article, or claim that may be made by its manufacturer, is not guaranteed or endorsed by the publisher.
Abbreviations
AE, adverse effect; AD, autoimmune disease; ADCC, antibody-dependent cytotoxicity; ANA, anti-nuclear antibody; ASCO, American Society of Clinical Oncology; BOR, best overall response; CTCAE, Common Terminology Criteria for Adverse Events; CTLA-4, cytotoxic T-lymphocyte antigen 4; DCR, disease control rate; DMARDs, disease-modifying antirheumatic drugs; ESMO, European Society for Medical Oncology; FDA, Food and Drug Administration; IBD, inflammatory bowel disease; ICs, Immune checkpoints; ICIs, Immune checkpoint inhibitors; ILD, interstitial lung disease; IMDC, immune-mediated diarrhea and colitis; irAEs, immune-related adverse events; JAK/STAT, Janus kinase/signal transducer and activator of transcription; MMP, metalloproteinase; MS, multiple sclerosis; NA, not applicable; NCCN, National Comprehensive Cancer Network; NCI, National Cancer Institute; NLR, neutrophil-to-lymphocyte ratio; NSAIDs, nonsteroidal anti-inflammatory drugs; NSCLC, non-small cell lung cancer; ORR, overall response rate; OS, overall survival; PAD, pre-existing autoimmune disease; PFS, progression-free survival; PLR, platelet-to-lymphocyte ratio; PD-1, programmed death protein 1; PD-L1, programmed death protein ligand 1; pHLIP, pH low insertion peptide; PMR, polymyalgia rheumatica; RA, rheumatoid arthritis; RNS, reactive nitrogen species; ROS, reactive oxygen species; SITC, Society for Immunotherapy of Cancer; SLE, systemic lupus erythematosus; SNP, single nucleotide polymorphism; SSc, systemic sclerosis; TME, tumor microenvironment; trAEs, treatment-related adverse events.
References
1. Fife BT and Bluestone JA. Control of peripheral T-cell tolerance and autoimmunity via the CTLA-4 and PD-1 pathways. Immunol Rev. (2008) 224:166–82. doi: 10.1111/j.1600-065X.2008.00662.x
2. Yenyuwadee S, Aliazis K, Wang Q, Christofides A, Shah R, Patsoukis N, et al. Immune cellular components and signaling pathways in the tumor microenvironment. Semin Cancer Biol. (2022) 86:187–201. doi: 10.1016/j.semcancer.2022.08.004
3. Rose NR. Prediction and prevention of autoimmune disease in the 21st century: A review and preview. Am J Epidemiol. (2016) 183:403–6. doi: 10.1093/aje/kwv292
4. Tang H, Zhou J, and Bai C. The efficacy and safety of immune checkpoint inhibitors in patients with cancer and preexisting autoimmune disease. Front Oncol. (2021) 11:625872. doi: 10.3389/fonc.2021.625872
5. Xie W, Huang H, Xiao S, Fan Y, Deng X, and Zhang Z. Immune checkpoint inhibitors therapies in patients with cancer and preexisting autoimmune diseases: A meta-analysis of observational studies. Autoimmun Rev. (2020) 19:102687. doi: 10.1016/j.autrev.2020.102687
6. Yu Y, Zhou Y, Zhang X, Tan K, Zheng J, Li J, et al. Immune checkpoint inhibitors in the treatment of patients with cancer and preexisting psoriasis: A systematic review and meta-analysis of observational studies. Front Oncol. (2022) 12:934093. doi: 10.3389/fonc.2022.934093
7. Johnson DB, Sullivan RJ, and Menzies AM. Immune checkpoint inhibitors in challenging populations. Cancer. (2017) 123:1904–11. doi: 10.1002/cncr.30642
8. Khan SA, Pruitt SL, Xuan L, and Gerber DE. Prevalence of autoimmune disease among patients with lung cancer: implications for immunotherapy treatment options. JAMA Oncol. (2016) 2:1507–8. doi: 10.1001/jamaoncol.2016.2238
9. Franks AL and Slansky JE. Multiple associations between a broad spectrum of autoimmune diseases, chronic inflammatory diseases and cancer. Anticancer Res. (2012) 32:1119–36.
10. Giat E, Ehrenfeld M, and Shoenfeld Y. Cancer and autoimmune diseases. Autoimmun Rev. (2017) 16:1049–57. doi: 10.1016/j.autrev.2017.07.022
11. Bernatsky S, Joseph L, Boivin JF, Gordon C, Urowitz M, Gladman D, et al. The relationship between cancer and medication exposures in systemic lupus erythaematosus: a case-cohort study. Ann Rheum Dis. (2008) 67:74–9. doi: 10.1136/ard.2006.069039
12. Maria ATJ, Partouche L, Goulabchand R, Rivière S, Rozier P, Bourgier C, et al. Intriguing relationships between cancer and systemic sclerosis: role of the immune system and other contributors. Front Immunol. (2019) 9:3112. doi: 10.3389/fimmu.2018.03112
13. Wu R, Huang Y, Chen J, Huo Z, Wang R, Feng Y, et al. Rheumatoid arthritis and risk of lung cancer: Meta-analysis and Mendelian randomization study. Semin Arthritis Rheumatol. (2021) 51:565–75. doi: 10.1016/j.semarthrit.2021.03.015
14. Bin J, Bernatsky S, Gordon C, Boivin JF, Ginzler E, Gladman D, et al. Lung cancer in systemic lupus erythematosus. Lung Cancer. (2007) 56:303–6. doi: 10.1016/j.lungcan.2007.01.007
15. Handel AE, Joseph A, and Ramagopalan SV. Multiple sclerosis and lung cancer: an unexpected inverse association. QJM. (2010) 103:625–6. doi: 10.1093/qjmed/hcq071
16. Zahr ZA and Baer AN. Malignancy in myositis. Curr Rheumatol Rep. (2011) 13:208–15. doi: 10.1007/s11926-011-0169-7
17. Olesen AB, Svaerke C, Farkas DK, and Sørensen HT. Systemic sclerosis and the risk of cancer: a nationwide population-based cohort study. Br J Dermatol. (2010) 163:800–6. doi: 10.1111/j.1365-2133.2010.09861.x
18. Wang F, Palmer N, Fox K, Liao KP, Yu KH, and Kou SC. Large-scale real-world data analyses of cancer risks among patients with rheumatoid arthritis. Int J Cancer. (2023) 153:1139–50. doi: 10.1002/ijc.34606
19. Song L, Wang Y, Zhang J, Song N, Xu X, and Lu Y. The risks of cancer development in systemic lupus erythematosus (SLE) patients: a systematic review and meta-analysis. Arthritis Res Ther. (2018) 20:270. doi: 10.1186/s13075-018-1760-3
20. Nocturne G, Boudaoud S, Miceli-Richard C, Viengchareun S, Lazure T, Nititham J, et al. Germline and somatic genetic variations of TNFAIP3 in lymphoma complicating primary Sjogren’s syndrome. Blood. (2013) 122:4068–76. doi: 10.1182/blood-2013-05-503383
21. Liu H, Ma H, Li Y, and Zhao H. Advances in epigenetic modifications and cervical cancer research. Biochim Biophys Acta Rev Cancer. (2023) 1878:188894. doi: 10.1016/j.bbcan.2023.188894
22. Baka Z, Buzás E, and Nagy G. Rheumatoid arthritis and smoking: putting the pieces together. Arthritis Res Ther. (2009) 11:238. doi: 10.1186/ar2751
23. Kinoshita T and Goto T. Molecular mechanisms of pulmonary fibrogenesis and its progression to lung cancer: A review. Int J Mol Sci. (2019) 20:1461. doi: 10.3390/ijms20061461
24. Pontifex EK, Hill CL, and Roberts-Thomson P. Risk factors for lung cancer in patients with scleroderma: a nested case-control study. Ann Rheum Dis. (2007) 66:551–3. doi: 10.1136/ard.2006.056424
25. Colaci M, Giuggioli D, Sebastiani M, Manfredi A, Vacchi C, Spagnolo P, et al. Lung cancer in scleroderma: results from an Italian rheumatologic center and review of the literature. Autoimmun Rev. (2013) 12:374–9. doi: 10.1016/j.autrev.2012.06.003
26. Pantuck M, McDermott D, and Drakaki A. To treat or not to treat: Patient exclusion in immune oncology clinical trials due to preexisting autoimmune disease. Cancer. (2019) 125:3506–13. doi: 10.1002/cncr.32326
27. Cappelli LC and Shah AA. The relationships between cancer and autoimmune rheumatic diseases. Best Pract Res Clin Rheumatol. (2020) 34:101472. doi: 10.1016/j.berh.2019.101472
28. Awasthi A and Kuchroo VK. Th17 cells: from precursors to players in inflammation and infection. Int Immunol. (2009) 21:489–98. doi: 10.1093/intimm/dxp021
29. Rodríguez Murúa S, Farez MF, and Quintana FJ. The immune response in multiple sclerosis. Annu Rev Pathol. (2022) 17:121–39. doi: 10.1146/annurev-pathol-052920-040318
30. Ogura H, Murakami M, Okuyama Y, Tsuruoka M, Kitabayashi C, Kanamoto M, et al. Interleukin-17 promotes autoimmunity by triggering a positive-feedback loop via interleukin-6 induction. Immunity. (2008) 29:628–36. doi: 10.1016/j.immuni.2008.07.018
31. Golstein P and Griffiths GM. An early history of T cell-mediated cytotoxicity. Nat Rev Immunol. (2018) 18:527–35. doi: 10.1038/s41577-018-0009-3
32. Rao DA, Gurish MF, Marshall JL, Slowikowski K, Fonseka CY, Liu Y, et al. Pathologically expanded peripheral T helper cell subset drives B cells in rheumatoid arthritis. Nature. (2017) 542:110–4. doi: 10.1038/nature20810
33. Song Y, Li J, and Wu Y. Evolving understanding of autoimmune mechanisms and new therapeutic strategies of autoimmune disorders. Signal Transduct Target Ther. (2024) 9:263. doi: 10.1038/s41392-024-01952-8
34. Chen Z, Bozec A, Ramming A, and Schett G. Anti-inflammatory and immune-regulatory cytokines in rheumatoid arthritis. Nat Rev Rheumatol. (2019) 15:9–17. doi: 10.1038/s41584-018-0109-2
35. Mantovani A, Allavena P, Sica A, and Balkwill F. Cancer-related inflammation. Nature. (2008) 454:436–44. doi: 10.1038/nature07205
36. Murray PJ, Allen JE, Biswas SK, Fisher EA, Gilroy DW, Goerdt S, et al. Macrophage activation and polarization: nomenclature and experimental guidelines. Immunity. (2014) 41:14–20. doi: 10.1016/j.immuni.2014.06.008
37. Myers KV, Amend SR, and Pienta KJ. Targeting Tyro3, Axl and MerTK (TAM receptors): implications for macrophages in the tumor microenvironment. Mol Cancer. (2019) 18:94. doi: 10.1186/s12943-019-1022-2
38. Zhu X, Liang R, Lan T, Ding D, Huang S, Shao J, et al. Tumor-associated macrophage-specific CD155 contributes to M2-phenotype transition, immunosuppression, and tumor progression in colorectal cancer. J Immunother Cancer. (2022) 10:e004219. doi: 10.1136/jitc-2021-004219
39. Josefowicz SZ, Lu LF, and Rudensky AY. Regulatory T cells: mechanisms of differentiation and function. Annu Rev Immunol. (2012) 30:531–64. doi: 10.1146/annurev.Immunol.25.022106.141623
40. Rincon-Arevalo H, Wiedemann A, Stefanski AL, Lettau M, Szelinski F, Fuchs S, et al. Deep phenotyping of CD11c+ B cells in systemic autoimmunity and controls. Front Immunol. (2021) 12:635615. doi: 10.3389/fimmu.2021.635615
41. Murray-Brown W, Guo Y, Small A, Lowe K, Weedon H, Smith MD, et al. Differential expansion of T peripheral helper cells in early rheumatoid arthritis and osteoarthritis synovium. RMD Open. (2022) 8:e002563. doi: 10.1136/rmdopen-2022-002563
42. Kreher MA, Noland MMB, Konda S, Longo MI, and Valdes-Rodriguez R. Risk of melanoma and nonmelanoma skin cancer with immunosuppressants, part I: Calcineurin inhibitors, thiopurines, IMDH inhibitors, mTOR inhibitors, and corticosteroids. J Am Acad Dermatol. (2023) 88:521–30. doi: 10.1016/j.jaad.2022.11.044
43. Kreher MA, Konda S, Noland MMB, Longo MI, and Valdes-Rodriguez R. Risk of melanoma and nonmelanoma skin cancer with immunosuppressants, part II: Methotrexate, alkylating agents, biologics, and small molecule inhibitors. J Am Acad Dermatol. (2023) 88:534–42. doi: 10.1016/j.jaad.2022.11.043
44. Ladouceur A, Bernatsky S, Ramsey-Goldman R, and Clarke AE. Managing cancer risk in patients with systemic lupus erythematous. Expert Rev Clin Immunol. (2018) 14:793–802. doi: 10.1080/1744666X.2018.1519394
45. Cai B, Huang Y, Liu D, You Y, Chen N, Jie L, et al. Identification of the ferroptosis-related gene signature and the associated regulation axis in lung cancer and rheumatoid arthritis. Genes Immun. (2024) 25:367–80. doi: 10.1038/s41435-024-00287-2
46. Yang J, Hu X, Wang Y, Liu W, Zhang M, Zhang A, et al. Identification of the shared gene signatures and molecular mechanisms between multiple sclerosis and non-small cell lung cancer. Front Immunol. (2023) 14:1180449. doi: 10.3389/fimmu.2023.1180449
47. Bernatsky S, Ramsey-Goldman R, Petri M, Urowitz MB, Gladman DD, Fortin PR, et al. Smoking is the most significant modifiable lung cancer risk factor in systemic lupus erythematosus. J Rheumatol. (2018) 45:393–6. doi: 10.3899/jrheum.170652
48. Ma PJ, Wang MM, and Wang Y. Gut microbiota: A new insight into lung diseases. BioMed Pharmacother. (2022) 155:113810. doi: 10.1016/j.biopha.2022.113810
49. Georgiou K, Marinov B, Farooqi AA, and Gazouli M. Gut microbiota in lung cancer: where do we stand? Int J Mol Sci. (2021) 22:10429. doi: 10.3390/ijms221910429
50. Correale J, Hohlfeld R, and Baranzini SE. The role of the gut microbiota in multiple sclerosis. Nat Rev Neurol. (2022) 18:544–58. doi: 10.1038/s41582-022-00697-8
51. Postow MA, Sidlow R, and Hellmann MD. Immune-related adverse events associated with immune checkpoint blockade. New Engl J Med. (2018) 378:158–68. doi: 10.1056/nejmra1703481
52. Michot JM, Bigenwald C, Champiat S, Collins M, Carbonnel F, Postel-Vinay S, et al. Immune-related adverse events with immune checkpoint blockade: a comprehensive review. Eur J Cancer. (2016) 54:139–48. doi: 10.1016/j.ejca.2015.11.016
53. Xing P, Zhang F, Wang G, Xu Y, Li C, Wang S, et al. Incidence rates of immune-related adverse events and their correlation with response in advanced solid tumours treated with NIVO or NIVO+IPI: a systematic review and meta-analysis. J Immunother Cancer. (2019) 7:341. doi: 10.1186/s40425-019-0779-6
54. Wang DY, Salem JE, Cohen JV, Chandra S, Menzer C, Ye F, et al. Fatal toxic effects associated with immune checkpoint inhibitors: a systematic review and metaanalysis. JAMA Oncol. (2018) 4:1721–8. doi: 10.1001/jamaoncol.2018.3923
55. Kennedy LB and Salama AKS. A review of cancer immunotherapy toxicity. CA Cancer J Clin. (2020) 70:86–104. doi: 10.3322/caac.21596
56. Boutros C, Tarhini A, Routier E, Lambotte O, Ladurie FL, Carbonnel F, et al. Safety profiles of anti-CTLA-4 and anti-PD-1 antibodies alone and in combination. Nat Rev Clin Oncol. (2016) 13:473–86. doi: 10.1038/nrclinonc.2016.58
57. Lee C, Drobni ZD, Zafar A, Gongora CA, Zlotoff DA, Alvi RM, et al. Pre-existing autoimmune disease increases the risk of cardiovascular and noncardiovascular events after immunotherapy. JACC Cardio Oncol. (2022) 4:660–9. doi: 10.1016/j.jaccao.2022.11.008
58. Miller FW. The increasing prevalence of autoimmunity and autoimmune diseases: an urgent call to action for improved understanding, diagnosis, treatment, and prevention. Curr Opin Immunol. (2023) 80:102266. doi: 10.1016/j.coi.2022.102266
59. Donia M, Kimper-Karl ML, Hoyer KL, Bastholt L, Schmidt H, and Svane IM. The majority of patients with metastatic melanoma are not represented in pivotal phase III immunotherapy trials. Eur J Cancer. (2017) 74:89–95. doi: 10.1016/j.ejca.2016.12.017
60. Reid P and Cappelli LC. Treatment of rheumatic adverse events of cancer immunotherapy. Best Pract Res Clin Rheumatol. (2022) 36:101805. doi: 10.1016/j.berh.2022.101805
61. Lopez-Olivo MA, Kachira JJ, Abdel-Wahab N, Pundole X, Aldrich JD, Carey P, et al. A systematic review and meta-analysis of observational studies and uncontrolled trials reporting on the use of checkpoint blockers in patients with cancer and pre-existing autoimmune disease. Eur J Cancer. (2024) 207:114148. doi: 10.1016/j.ejca.2024.114148
62. Pizuorno MaChado A, Shatila M, Liu C, Wang J, Altan M, Zhang HC, et al. Immune-related adverse events after immune checkpoint inhibitor exposure in adult cancer patients with pre-existing autoimmune diseases. J Cancer Res Clin Oncol. (2023) 149:6341–50. doi: 10.1007/s00432-023-04582-9
63. Plaçais L, Dalle S, Dereure O, Trabelsi S, Dalac S, Legoupil D, et al. Risk of irAEs in patients with autoimmune diseases treated by immune checkpoint inhibitors for stage III or IV melanoma: results from a matched case-control study. Ann Rheum Dis. (2022) 81:1445–52. doi: 10.1136/ard-2022-222186
64. Rong Y, Bentley JP, Bhattacharya K, Yang Y, Chang Y, Earl S, et al. Incidence and risk factors of immune-related adverse events induced by immune checkpoint inhibitors among older adults with non-small cell lung cancer. Cancer Med. (2024) 13:e6879. doi: 10.1002/cam4.6879
65. Yeung C, Kartolo A, Holstead R, Moffat GT, Hanna L, Hopman W, et al. Safety and clinical outcomes of immune checkpoint inhibitors in patients with cancer and preexisting autoimmune diseases. J Immunother. (2021) 44:362–70. doi: 10.1097/CJI.0000000000000377
66. Cortellini A, Buti S, Santini D, Perrone F, Giusti R, Tiseo M, et al. Clinical outcomes of patients with advanced cancer and pre-existing autoimmune diseases treated with anti-programmed death-1 immunotherapy: a real-world transverse study. Oncologist. (2019) 24:e327–37. doi: 10.1634/theoncologist.2018-0618
67. Aung WY, Lee CS, Morales J, Rahman H, and Seetharamu N. Safety and efficacy of immune checkpoint inhibitors in cancer patients and preexisting autoimmune disease: A systematic review and meta-analysis in non-small-cell lung cancer. Clin Lung Cancer. (2023) 24:598–612. doi: 10.1016/j.cllc.2023.05.006
68. Leonardi GC, Gainor JF, Altan M, Kravets S, Dahlberg SE, Gedmintas L, et al. Safety of programmed death-1 pathway inhibitors among patients with non-Small-Cell lung cancer and preexisting autoimmune disorders. J Clin Oncol. (2018) 36:1905–12. doi: 10.1200/JCO.2017.77.0305
69. Pease D, Shyne M, Joshi A, Lee A, and Patel M. P635 Effectiveness and tolerance of immune checkpoint blockade in a real-world lung cancer patient population. J ImmunoTher Cancer. (2018) 6:115. doi: 10.1186/s40425-018-0423-x
70. Cytryn S, Efuni E, Velcheti V, and Sandigursky S. P2.04–48 use of immune checkpoint inhibitors in patients with advanced lung cancer and pre-existing autoimmune diseases. J Thorac Oncol. (2019) 14:S726–7. doi: 10.1016/j.jtho.2019.08.1553
71. Tison A, Quere G, Misery L, Funck-Brentano E, Danlos F-X, Bonniaud B, et al. OP0196 Safety and efficacy of immune checkpoint inhibitors in patients with cancer and preexisting autoimmune diseases: A nationwide multicenter retrospective study. Ann Rheum Dis. (2018) 77:147–7. doi: 10.1136/annrheumdis-2018-eular.5840
72. Khozin S, Walker MS, Jun M, Chen L, Stepanski E, Rubinstein WS, et al. Real-world outcomes of patients with advanced non-small cell lung cancer (aNSCLC) and autoimmune disease (AD) receiving immune checkpoint inhibitors (ICIs). J Clin Oncol. (2019) 37:110–0. doi: 10.1200/JCO.2019.37.15_suppl.110
73. Tison A, Quéré G, Misery L, Funck-Brentano E, Danlos FX, Routier E, et al. Safety and efficacy of immune checkpoint inhibitors in patients with cancer and preexisting autoimmune disease: a nationwide, multicenter cohort study. Arthritis Rheumatol. (2019) 71:2100–11. doi: 10.1002/art.41068
74. Alexander S, Swami U, Kaur A, Gao Y, Fatima M, Ginn MM, et al. Safety of immune checkpoint inhibitors in patients with cancer and pre-existing autoimmune disease. Ann Transl Med. (2021) 9:1033. doi: 10.21037/atm-20-8124
75. Ardizzoni A, Azevedo S, Rubio-Viqueira B, Rodríguez-Abreu D, Alatorre-Alexander J, Smit HJM, et al. Primary results from TAIL: a global single-arm safety study of atezolizumab monotherapy in a diverse population of patients with previously treated advanced non-small cell lung cancer. J Immunother Cancer. (2021) 9:e001865. doi: 10.1136/jitc-2020-001865
76. Calvo V, Fernández MA, Collazo-Lorduy A, Franco F, Núñez B, and Provencio M. Use of immune checkpoint inhibitors in patients with solid tumors and pre-existing autoimmune or inflammatory disease: real-world data. Lung Cancer Manag. (2021) 10:LMT51. doi: 10.2217/lmt-2021-0003
77. Debieuvre D, Juergens RA, Asselain B, Audigier-Valette C, Auliac JB, Barlesi F, et al. Two-year survival with nivolumab in previously treated advanced non-small-cell lung cancer: A real-world pooled analysis of patients from France, Germany, and Canada. Lung Cancer. (2021) 157:40–7. doi: 10.1016/j.lungcan.2021.04.022
78. Fountzilas E, Lampaki S, Koliou GA, Koumarianou A, Levva S, Vagionas A, et al. Real-world safety and efficacy data of immunotherapy in patients with cancer and autoimmune disease: the experience of the Hellenic Cooperative Oncology Group. Cancer Immunol Immunother. (2022) 71:327–37. doi: 10.1007/s00262-021-02985-6
79. Sawhney P, Patel G, Ohana D, Luong MK, Wong YNS, Lee AJX, et al. 208P Safety and efficacy of immune checkpoint inhibitors in NSCLC patients with autoimmune disease: A UK tertiary cancer centre experience. J Thorac Oncol. (2021) 16:S810. doi: 10.1016/S1556-0864(21)02050-5
80. Zhang S, Pease DF, Kulkarni AA, Kumar M, Shanley RM, Xu B, et al. Real-world outcomes and clinical predictors of immune checkpoint inhibitor monotherapy in advanced lung cancer. Clin Med Insights Oncol. (2021) 15:11795549211004489. doi: 10.1177/11795549211004489
81. Ardizzoni A, Azevedo S, Rubio-Viqueira B, Rodriguez-Abreu D, Alatorre-Alexander J, Smit HJM, et al. Final results from TAIL: updated long-term efficacy of atezolizumab in a diverse population of patients with previously treated advanced non-small cell lung cancer. J Immunother Cancer. (2022) 10:e005581. doi: 10.1136/jitc-2022-005581
82. Higgins JP, Trinh AV, Watson ML, Beardslee T, Goyal S, KudChadkar R, et al. A safety analysis of programmed death 1 pathway inhibitors in patients with solid tumor Malignancies and preexisting autoimmune disease. J Clin Rheumatol. (2022) 28:338–45. doi: 10.1097/RHU.0000000000001863
83. Ohe Y, Yamazaki N, Yamamoto N, Murakami H, Yoh K, Kitano S, et al. The real-world safety of atezolizumab as second-line or later treatment in Japanese patients with non-small-cell lung cancer: a post-marketing surveillance study. Jpn J Clin Oncol. (2022) 52:623–32. doi: 10.1093/jjco/hyac024
84. Ansel S, Rulach R, Trotter N, and Steele N. Pembrolizumab for advanced non-small cell lung cancer (NSCLC): Impact of autoimmune comorbidity and outcomes following treatment completion. J Oncol Pharm Pract. (2023) 29:785–93. doi: 10.1177/10781552221079356
85. Reid P, Sandigursky S, Song J, Lopez-Olivo MA, Safa H, Cytryn S, et al. Safety and effectiveness of combination versus monotherapy with immune checkpoint inhibitors in patients with preexisting autoimmune diseases. Oncoimmunology. (2023) 12:2261264. doi: 10.1080/2162402X.2023.2261264
86. Androdias G, Noroy L, Psimaras D, Birzu C, Pelletier J, Beigneux Y, et al. Impact of immune checkpoint inhibitors on the course of multiple sclerosis. Neurol Neuroimmunol Neuroinflamm. (2024) 11:e200202. doi: 10.1212/NXI.0000000000200202
87. Asao T, Shukuya T, Uemura K, Kitadai R, Yamamoto G, Mouri A, et al. Risk and survival of patients with non-small cell lung cancer and pre-existing autoimmune disorders receiving immune checkpoint blockade therapy: Survival analysis with inverse probability weighting from a nationwide, multi-institutional, retrospective study (NEJ047). Lung Cancer. (2024) 194:107894. doi: 10.1016/j.lungcan.2024.107894
88. Ihara Y, Sawa K, Imai T, Nonomiya Y, Shimomura Y, Ishihara A, et al. Immune checkpoint inhibitor is associated with improved survival in advanced non-small cell lung cancer occurring in patients with autoimmune disease. Biol Pharm Bull. (2024) 47:454–61. doi: 10.1248/bpb.b23-00713
89. Fukihara J, Sakamoto K, Koyama J, Ito T, Iwano S, Morise M, et al. Prognostic impact and risk factors of immune-related pneumonitis in patients with non-Small Cell lung cancer who received programmed death 1 inhibitors. Clin Lung Cancer. (2019) 20:442–450.e4. doi: 10.1016/j.cllc.2019.07.006128
90. Hu W, Wang G, Wang Y, Riese MJ, and You M. Uncoupling therapeutic efficacy from immune-related adverse events in immune checkpoint blockade. iScience. (2020) 23:101580. doi: 10.1016/j.isci.2020.101580
91. Available online at: https://clinicaltrials.gov/study/NCT03816345 (Accessed April, 2025).
92. Abdel-Wahab N, Shah M, Lopez-Olivo MA, and Suarez-Almazor ME. Use of immune checkpoint inhibitors in the treatment of patients with cancer and preexisting autoimmune disease: a systematic review. Ann Intern Med. (2018) 168:121. doi: 10.7326/M17-2073
93. Barroso-Sousa R, Barry WT, Garrido-Castro AC, Hodi FS, Min L, Krop IE, et al. Incidence of endocrine dysfunction following the use of different immune checkpoint inhibitor regimens: A systematic review and meta-analysis. JAMA Oncol. (2018) 4:173–82. doi: 10.1001/jamaoncol.2017.3064
94. Schett G, Emery P, Tanaka Y, Burmester G, Pisetsky DS, Naredo E, et al. Tapering biologic and conventional DMARD therapy in rheumatoid arthritis: current evidence and future directions. Ann Rheum Dis. (2016) 75:1428–37. doi: 10.1136/annrheumdis-2016-209201
95. Wu C, Zhong L, Wu Q, Lin S, and Xie X. The safety and efficacy of immune-checkpoint inhibitors in patients with cancer and pre-existing autoimmune diseases. Immunotherapy. (2021) 13:527–39. doi: 10.2217/imt-2020-0230
96. Verheijden RJ, de Groot JS, Fabriek BO, Hew MN, May AM, and Suijkerbuijk KPM. Corticosteroids for immune-related adverse events and checkpoint inhibitor efficacy: analysis of six clinical trials. J Clin Oncol. (2024) 42:3713–24. doi: 10.1200/JCO.24.00191
97. Thompson JA, Schneider BJ, Brahmer J, Zaid MA, Achufusi A, Armand P, et al. NCCN guidelines® Insights: management of immunotherapy-related toxicities, version 2.2024. J Natl Compr Canc Netw. (2024) 22:582–92. doi: 10.6004/jnccn.2024.0057
98. Gutzmer R, Koop A, Meier F, Hassel JC, Terheyden P, Zimmer L, et al. Programmed cell death protein-1 (PD-1) inhibitor therapy in patients with advanced melanoma and preexisting autoimmunity or ipilimumab-triggered autoimmunity. Eur J Cancer. (2017) 75:24–32. doi: 10.1016/j.ejca.2016.12.038
99. Richter MD, Pinkston O, KottsChade LA, Finnes HD, Markovic SN, and Thanarajasingam U. Brief report: cancer immunotherapy in patients with preexisting rheumatic disease: the mayo clinic experience. Arthritis Rheumatol. (2018) 70:356–60. doi: 10.1002/art.40397
100. Hansen M, Zakria D, and Johnson D. Outcomes and Disease Characteristics in Patients with Rheumatoid Arthritis Treated with Immune Checkpoint Inhibitors for Malignancy at a Single Academic Institution. Arthritis Rheumatol. (2021) 73. Available online at: https://acrabstracts.org/abstract/outcomes-and-disease-characteristics-in-patients-with-rheumatoid-arthritis-treated-with-immune-checkpoint-inhibitors-for-malignancy-at-a-single-academic-institution/ (Accessed April, 2025).
101. Roberts J, Ennis D, Hudson M, Ye C, Saltman A, Himmel M, et al. Rheumatic immune-related adverse events associated with cancer immunotherapy: A nationwide multi-center cohort. Autoimmun Rev. (2020) 19:102595. doi: 10.1016/j.autrev.2020.102595
102. Efuni E, Cytryn S, Boland P, Niewold TB, Pavlick A, Weber J, et al. Risk of toxicity after initiating immune checkpoint inhibitor treatment in patients with rheumatoid arthritis. J Clin Rheumatol. (2021) 27:267–71. doi: 10.1097/RHU.0000000000001314
103. Halle BR, Betof Warner A, Zaman FY, Haydon A, Bhave P, Dewan AK, et al. Immune checkpoint inhibitors in patients with pre-existing psoriasis: safety and efficacy. J Immunother Cancer. (2021) 9:e003066. doi: 10.1136/jitc-2021-003066
104. Fane LS, Efird JT, Jindal C, and Biswas T. Dermatological autoimmune considerations of immune checkpoint therapy. Biomedicines. (2022) 10:2339. doi: 10.3390/biomedicines10102339
105. Barroso-Sousa R, Ott PA, Hodi FS, Kaiser UB, Tolaney SM, and Min L. Endocrine dysfunction induced by immune checkpoint inhibitors: Practical recommendations for diagnosis and clinical management. Cancer. (2018) 124:1111–21. doi: 10.1002/cncr.31200
106. Sinicrope FA and Turk MJ. Immune checkpoint blockade: timing is everything. J Immunother Cancer. (2024) 12:e009722. doi: 10.1136/jitc-2024-009722
107. Tahir SA, Gao J, Miura Y, Blando J, Tidwell RSS, Zhao H, et al. Autoimmune antibodies correlate with immune checkpoint therapy-induced toxicities. Proc Natl Acad Sci. (2019) 116:22246–51. doi: 10.1073/pnas.1908079116
108. Sakakida T, Ishikawa T, Chihara Y, Harita S, Uchino J, Tabuchi Y, et al. Safety and efficacy of PD-1/PD-L1 blockade in patients with preexisting antinuclear antibodies. Clin Trans Oncol. (2020) 22:919–27. doi: 10.1007/s12094-019-02214-8
109. Osorio JC, Ni A, Chaft JE, Pollina R, Kasler MK, Stephens D, et al. Antibody-mediated thyroid dysfunction during T-cell checkpoint blockade in patients with non-small-cell lung cancer. Ann Oncol. (2017) 28:583–9. doi: 10.1093/annonc/mdw640
110. Toi Y, Sugawara S, Sugisaka J, Ono H, Kawashima Y, Aiba T, et al. Profiling preexisting antibodies in patients treated with anti-PD-1 therapy for advanced non-small cell lung cancer. JAMA Oncol. (2019) 5:376–83. doi: 10.1001/jamaoncol.2018.5860
111. Ghosh N, Postow M, Zhu C, Jannat-Khah D, Li QZ, Vitone G, et al. Lower baseline autoantibody levels are associated with immune-related adverse events from immune checkpoint inhibition. J ImmunoTher Cancer. (2022) 10:e004008. doi: 10.1136/jitc-2021-004008
112. Boland P, Pavlick AC, and Weber J and Sandigursky S. Immunotherapy to treat Malignancy in patients with pre-existing autoimmunity. J Immunother Cancer. (2020) 8(1):e000356. doi: 10.1136/jitc-2019-000356
113. Khan S, Khan SA, Luo X, Fattah FJ, Saltarski J, Gloria-Mccutchen Y, et al. Immune dysregulation in cancer patients developing immune-related adverse events. Br J Cancer. (2019) 120:63–8. doi: 10.1038/s41416-018-0155-1
114. Tarhini AA, Zahoor H, Lin Y, Malhotra U, Sander C, Butterfield LH, et al. Baseline circulating IL-17 predicts toxicity while TGF-beta1 and IL-10 are prognostic of relapse in ipilimumab neoadjuvant therapy of melanoma. J Immunother Cancer. (2015) 3:39. doi: 10.1186/s40425-015-0081-1
115. Valpione S, Pasquali S, Campana LG, Piccin L, Mocellin S, Pigozzo J, et al. Sex and interleukin-6 are prognostic factors for autoimmune toxicity following treatment with anti-CTLA4 blockade. J Trans Med. (2018) 16:94. doi: 10.1186/s12967-018-1467-x
116. Tyan K, Baginska J, Brainard M, Giobbie-Hurder A, Severgnini M, Manos M, et al. Cytokine changes during immune-related adverse events and corticosteroid treatment in melanoma patients receiving immune checkpoint inhibitors. Cancer Immunol Immunother. (2021) 70:2209–21. doi: 10.1007/s00262-021-02855-1
117. Kang DH, Park C-K, Chung C, Oh I-J, Kim Y-C, Park D, et al. Baseline serum interleukin-6 levels predict the response of patients with advanced non-small cell lung cancer to PD-1/PD-L1 inhibitors. Immune Netw. (2020) 20:e27–7. doi: 10.4110/in.2020.20.e27
118. Hirashima T, Kanai T, Suzuki H, Yoshida H, Matsushita A, Kawasumi H, et al. The levels of interferon-gamma release as a biomarker for non-small-cell lung cancer patients receiving immune checkpoint inhibitors. Anticancer Res. (2019) 39:6231–40. doi: 10.21873/anticanres.13832
119. Oyanagi J, Koh Y, Sato K, Mori K, Teraoka S, Akamatsu H, et al. Predictive value of serum protein levels in patients with advanced non-small cell lung cancer treated with nivolumab. Lung Cancer. (2019) 132:107–13. doi: 10.1016/j.lungcan.2019.03.020
120. Callahan MK, Yang A, Tandon S, Xu Y, Subudhi SK, Roman RA, et al. Evaluation of serum IL-17 levels during ipilimumab therapy: correlation with colitis. J Clin Oncol. (2011) 29:2505–5. doi: 10.1200/jco.2011.29.15_suppl.2505
121. Marschner D, Falk M, Javorniczky NR, Hanke-Müller K, Rawluk J, Schmitt-Graeff A, et al. MicroRNA-146a regulates immune-related adverse events caused by immune checkpoint inhibitors. JCI Insight. (2020) 5:e132334. doi: 10.1172/jci.insight.132334
122. Abdel-Wahab N, Diab A, Yu RK, Futreal A, Criswell LA, Tayar JH, et al. Genetic determinants of immune-related adverse events in patients with melanoma receiving immune checkpoint inhibitors. Cancer Immunol Immunother. (2021) 70:1939–49. doi: 10.1007/s00262-020-02797-0
123. Friedlander P, Wood K, Wassmann K, Christenfeld AM, Bhardwaj N, and Oh WK. A whole-blood RNA transcript-based gene signature is associated with the development of CTLA-4 blockade-related diarrhoea in patients with advanced melanoma treated with the checkpoint inhibitor tremelimumab. J Immunother Cancer. (2018) 6:90. doi: 10.1186/s40425-018-0408-9
124. Shahabi V, Berman D, Chasalow SD, Wang L, Tsuchihashi Z, Hu B, et al. Gene expression profiling of whole blood in ipilimumab-treated patients for identification of potential biomarkers of immune-related gastrointestinal adverse events. J Trans Med. (2013) 11:75. doi: 10.1186/1479-5876-11-75
125. Jin Y, Chen DL, Wang F, Yang CP, Chen XX, You JQ, et al. The predicting role of circulating tumor DNA landscape in gastric cancer patients treated with immune checkpoint inhibitors. Mol Cancer. (2020) 19:154. doi: 10.1186/s12943-020-01274-7
126. Oh DY, Cham J, Zhang L, Fong G, Kwek SS, Klinger M, et al. Immune toxicities elicted by CTLA-4 blockade in cancer patients are associated with early diversification of the T-cell repertoire. Cancer Res. (2017) 77:1322–30. doi: 10.1158/0008-5472.CAN-16-2324
127. Subudhi SK, Aparicio A, Gao J, Zurita AJ, Araujo JC, Logothetis CJ, et al. Clonal expansion of CD8 T cells in the systemic circulation precedes development of ipilimumab-induced toxicities. Proc Natl Acad Sci U S A. (2016) 113:11919–24. doi: 10.1073/pnas.1611421113
128. Sung C, An J, Lee S, Park J, Lee KS, Kim IH, et al. Integrative analysis of risk factors for immune-related adverse events of checkpoint blockade therapy in cancer. Nat Cancer. (2023) 4:844–59. doi: 10.1038/s43018-023-00572-5
129. Pavan A, Calvetti L, Dal Maso A, Attili I, Del Bianco P, Pasello G, et al. Peripheral blood markers identify risk of immune-related toxicity in advanced non-small cell lung cancer treated with immune-checkpoint inhibitors. Oncologist. (2019) 24:1128–36. doi: 10.1634/theoncologist.2018-0563
130. Chu X, Zhao J, Zhou J, Zhou F, Jiang T, Jiang S, et al. Association of baseline peripheral-blood eosinophil count with immune checkpoint inhibitor-related pneumonitis and clinical outcomes in patients with non-small cell lung cancer receiving immune checkpoint inhibitors. Lung Cancer. (2020) 150:76–82. doi: 10.1016/j.lungcan.2020.08.015
131. Fujisawa Y, Yoshino K, Otsuka A, Funakoshi T, Fujimura T, Yamamoto Y, et al. Fluctuations in routine blood count might signal severe immune-related adverse events in melanoma patients treated with nivolumab. J Dermatol Sci. (2017) 88:225–31. doi: 10.1016/j.jdermsci.2017.07.007
132. Abolhassani A-R, Schuler G, Kirchberger MC, and Heinzerling L. C-reactive protein as an early marker of immune-related adverse events. J Cancer Res Clin Oncol. (2019) 145:2625–31. doi: 10.1007/s00432-019-03002-1
133. Champiat S, Lambotte O, Barreau E, Belkhir R, Berdelou A, Carbonnel F, et al. Management of immune checkpoint blockade dysimmune toxicities: a collaborative position paper. Ann Oncol. (2016) 27:559–74. doi: 10.1093/annonc/mdv623
134. Brahmer JR, Abu-Sbeih H, Ascierto PA, Brufsky J, Cappelli LC, Cortazar FB, et al. Society for immunotherapy of cancer (SITC) clinical practice guideline on immune checkpoint inhibitor-related adverse events. J Immunother Cancer. (2021) 9:e002435. doi: 10.1136/jitc-2021-002435
135. Naidoo J, Murphy C, Atkins MB, Brahmer JR, Champiat S, Feltquate D, et al. Society for Immunotherapy of Cancer (SITC) consensus definitions for immune checkpoint inhibitor-associated immune-related adverse events (irAEs) terminology. J Immunother Cancer. (2023) 11:e006398. doi: 10.1136/jitc-2022-006398
136. Brahmer JR, Lacchetti C, Schneider BJ, Atkins MB, Brassil KJ, Caterino JM, et al. Management of immune-related adverse events in patients treated with immune checkpoint inhibitor therapy: American society of clinical oncology clinical practice guideline. J Clin Oncol. (2018) 36:1714–68. doi: 10.1200/JCO.2017.77.6385
137. Schneider BJ, Naidoo J, Santomasso BD, Adkins LC, Anadkat SM, Atkins MB, et al. Management of immune-related adverse events in patients treated with immune checkpoint inhibitor therapy: ASCO guideline update. J Clin Oncol. (2021) 39:4073126. doi: 10.1200/JCO.21
138. Haanen J, Obeid M, Spain L, Carbonnel F, Wang Y, Robert C, et al. Management of toxicities from immunotherapy: ESMO Clinical Practice Guideline for diagnosis, treatment and follow-up. Ann Oncol. (2022) 33:1217–38. doi: 10.1016/j.annonc.2022.10.001
139. Allouchery M, Lombard T, Martin M, Rouby F, Sassier M, Bertin C, et al. Safety of immune checkpoint inhibitor rechallenge after discontinuation for grade ≥2 immune-related adverse events in patients with cancer. J Immunother Cancer. (2020) 8:e001622. doi: 10.1136/jitc-2020-001622
140. Dolladille C, Ederhy S, Sassier M, Cautela J, Thuny F, Cohen AA, et al. Immune checkpoint inhibitor rechallenge after immune-related adverse events in patients with cancer. JAMA Oncol. (2020) 6:865–71. doi: 10.1001/jamaoncol.2020.0726
141. Pollack MH, Betof A, Dearden H, Rapazzo K, Valentine I, Brohl AS, et al. Safety of resuming anti-PD-1 in patients with immune-related adverse events (irAEs) during combined anti-CTLA-4 and anti-PD1 in metastatic melanoma. Ann Oncol. (2018) 29:250–5. doi: 10.1093/annonc/mdx642
142. Abu-Sbeih H, Ali FS, Naqash AR, Owen DH, Patel S, Otterson GA, et al. Resumption of immune checkpoint inhibitor therapy after immune-mediated colitis. J Clin Oncol. (2019) 37:2738–45. doi: 10.1200/JCO.19.00320
143. Simonaggio A, Michot JM, Voisin AL, Le Pavec J, Collins M, Lallart A, et al. Evaluation of readministration of immune checkpoint inhibitors after immune-related adverse events in patients with cancer. JAMA Oncol. (2019) 5:1310–7. doi: 10.1001/jamaoncol.2019.1022
144. Santini FC, Rizvi H, Plodkowski AJ, Ni A, Lacouture ME, Gambarin-Gelwan M, et al. Safety and efficacy of re-treating with immunotherapy after immune-related adverse events in patients with NSCLC. Cancer Immunol Res. (2018) 6:1093–9. doi: 10.1158/2326-6066.CIR-17-0755
145. SChadendorf D, Wolchok JD, Hodi FS, Chiarion-Sileni V, Gonzalez R, Rutkowski P, et al. Efficacy and safety outcomes in patients with advanced melanoma who discontinued treatment with nivolumab and ipilimumab because of adverse events: A pooled analysis of randomized phase II and III trials. J Clin Oncol. (2017) 35:3807–14. doi: 10.1200/JCO.2017.73.2289
146. Yasunaga M. Antibody therapeutics and immunoregulation in cancer and autoimmune disease. Semin Cancer Biol. (2020) 64:1–12. doi: 10.1016/j.semcancer.2019.06.001
147. Dimitriou F, Hogan S, Menzies AM, Dummer R, and Long GV. Interleukin-6 blockade for prophylaxis and management of immune-related adverse events in cancer immunotherapy. Eur J Cancer. (2021) 157:214–24. doi: 10.1016/j.ejca.2021.08.031
148. Balkwill F. Tumour necrosis factor and cancer. Nat Rev Cancer. (2009) 9:361–71. doi: 10.1038/nrc2628
149. McLornan DP, Pope JE, Gotlib J, and Harrison CN. Current and future status of JAK inhibitors. Lancet. (2021) 398:803–16. doi: 10.1016/S0140-6736(21)00438-4
150. Xue C, Yao Q, Gu X, Shi Q, Yuan X, Chu Q, et al. Evolving cognition of the JAK-STAT signaling pathway: autoimmune disorders and cancer. Signal Transduct Target Ther. (2023) 8:204. doi: 10.1038/s41392-023-01468-7
151. Mathew D, Marmarelis ME, Foley C, Bauml JM, Ye D, Ghinnagow R, et al. Combined JAK inhibition and PD-1 immunotherapy for non-small cell lung cancer patients. Science. (2024) 384:eadf1329. doi: 10.1126/science.adf1329
152. Jiang H, Chen W, Wang J, and Zhang R. Selective N-terminal modification of peptides and proteins: Recent progresses and applications. Chin Chem Lett. (2022) 33:80–8. doi: 10.1016/j.cclet.2021.06.011
153. Yu J, Song Y, and Tian W. How to select IgG subclasses in developing anti-tumor therapeutic antibodies. J Hematol Oncol. (2020) 13:45. doi: 10.1186/s13045-020-00876-4
154. Zhang W, Chen X, Chen X, Li J, Wang H, Yan X, et al. Fc-Fc interactions and immune inhibitory effects of IgG4: implications for anti-PD-1 immunotherapies. J Immunother Cancer. (2024) 12:e009034. doi: 10.1136/jitc-2024-009034
155. Lin W, Zhang Y, Yang Y, Lin B, Zhu M, Xu J, et al. Anti-PD-1/Her2 bispecific antibody IBI315 enhances the treatment effect of Her2-positive gastric cancer through gasdermin B-cleavage induced pyroptosis. Adv Sci (Weinh). (2023) 10:e2303908. doi: 10.1002/advs.202303908
156. Kist de Ruijter L, Hooiveld-Noeken JS, Giesen D, Lub-de Hooge MN, Kok IC, Brouwers AH, et al. First-in-human study of the biodistribution and pharmacokinetics of 89Zr-CX-072, a novel immunopet tracer based on an anti-PD-L1 probody. Clin Cancer Res. (2021) 27:5325–33. doi: 10.1158/1078-0432.CCR-21-0453
157. Sun Y, Hu L, Yang P, Zhang M, Wang X, Xiao H, et al. pH low insertion peptide-modified programmed cell death-ligand 1 potently suppresses T-cell activation under acidic condition. Front Immunol. (2021) 12:794226. doi: 10.3389/fimmu.2021.794226
158. Kiaie SH, Salehi-Shadkami H, Sanaei MJ, Azizi M, Shokrollahi Barough M, Nasr MS, et al. Nano-immunotherapy: overcoming delivery challenge of immune checkpoint therapy. J Nanobiotechnol. (2023) 21:339. doi: 10.1186/s12951-023-02083-y
159. Hu D, Zhang W, Tang J, Zhou Z, Liu X, and Shen Y. Improving safety of cancer immunotherapy via delivery technology. Biomaterials. (2021) 265:120407. doi: 10.1016/j.biomaterials.2020.120407
Keywords: lung cancer, immune checkpoint inhibitors (ICBs), immune-related adverse events (IRAE), autoimmune disease (AD), flare, predictive biomarker
Citation: Yang H, Yu S and Ge H (2025) Leveraging immune checkpoint inhibitors in lung cancer patients with pre-existing autoimmune disease: clinical insights, optimal timing, and predictive biomarkers for optimal treatment outcomes. Front. Immunol. 16:1539260. doi: 10.3389/fimmu.2025.1539260
Received: 04 December 2024; Accepted: 01 May 2025;
Published: 21 May 2025.
Edited by:
Cleber Machado-Souza, Pelé Pequeno Príncipe Research Institute, BrazilReviewed by:
Charlée Nardin, Centre Hospitalier Universitaire de Besançon, FranceHui Tang, Peking Union Medical College Hospital (CAMS), China
Copyright © 2025 Yang, Yu and Ge. This is an open-access article distributed under the terms of the Creative Commons Attribution License (CC BY). The use, distribution or reproduction in other forums is permitted, provided the original author(s) and the copyright owner(s) are credited and that the original publication in this journal is cited, in accordance with accepted academic practice. No use, distribution or reproduction is permitted which does not comply with these terms.
*Correspondence: Hong Ge, Z2Vob25nNjE2QDEyNi5jb20=
†These authors have contributed equally to this work