- 1Shandong Province University Clinical Immunology Translational Medicine Laboratory, The First Affiliated Hospital of Shandong First Medical University & Shandong Provincial Qianfoshan Hospital, Jinan, Shandong, China
- 2School of Clinical and Basic Medicine, Shandong First Medical University & Shandong Academy of Medical Sciences, Jinan, Shandong, China
- 3Institute of Infection and Immunity, Medical Science and Technology Innovation Center, Shandong First Medical University & Shandong Academy of Medical Sciences, Jinan, Shandong, China
Memory T (TM) cells play critical roles in protective immunity and immunopathology, and their generation and maintenance have attracted a lot of interests. In recent decades, informative investigations into CD8+ TM cell precursors have greatly enhanced our understanding of fate decision during CD8+ TM cell differentiation. Yet, much less is known about the generation of CD4+ TM cells and their precursors. In this review, we present advances in identifying precursors of CD4+ TM cells under Th1, Th2 and Th17 conditions, as well as current understanding of how intrinsic factors, extrinsic factors and positioning profiles contribute to determining fate choices of CD4+ T cells between effector and memory. However, the path toward a general theory of CD4+ TM cell generation has been hindered by technological limitations and diversity and plasticity of CD4+ T subsets at effector and memory phases. We thoroughly discuss the differences and similarities in differentiation of CD4+ TM cells under Th1, Th2, and Th17 conditions, and explore the prospects for identifying common precursors of specific CD4+ TM cells under various types of infections and exposures.
1 Introduction
In response to pathogen infection or antigen exposure, naive T cells primed by antigen-presenting cells proliferate and differentiate into functional effector T cells. Following elimination of immunologic threat, major effector cells (about 90-95%) die during contraction phase, and only a small proportion survives and develops into long-lived memory T (TM) cells which are capable of self-renewal and surviving in the absence of further antigen stimulation (Figure 1) (1–3). CD8+ and CD4+ TM cells can be typically subdivided into CD62L+CCR7+central memory T (TCM) cells, CD62L-CCR7- effector-like memory T (TEM) cells and CD69+CD103+or- tissue-resident memory T (TRM) cells based on their functions and migration patterns (4, 5). As TM cells play critical roles in protective immunity and immunopathology, elucidating mechanisms underlying their generation and maintenance is essential for the design of future vaccines capable of eliciting T cell-based immunity (6).
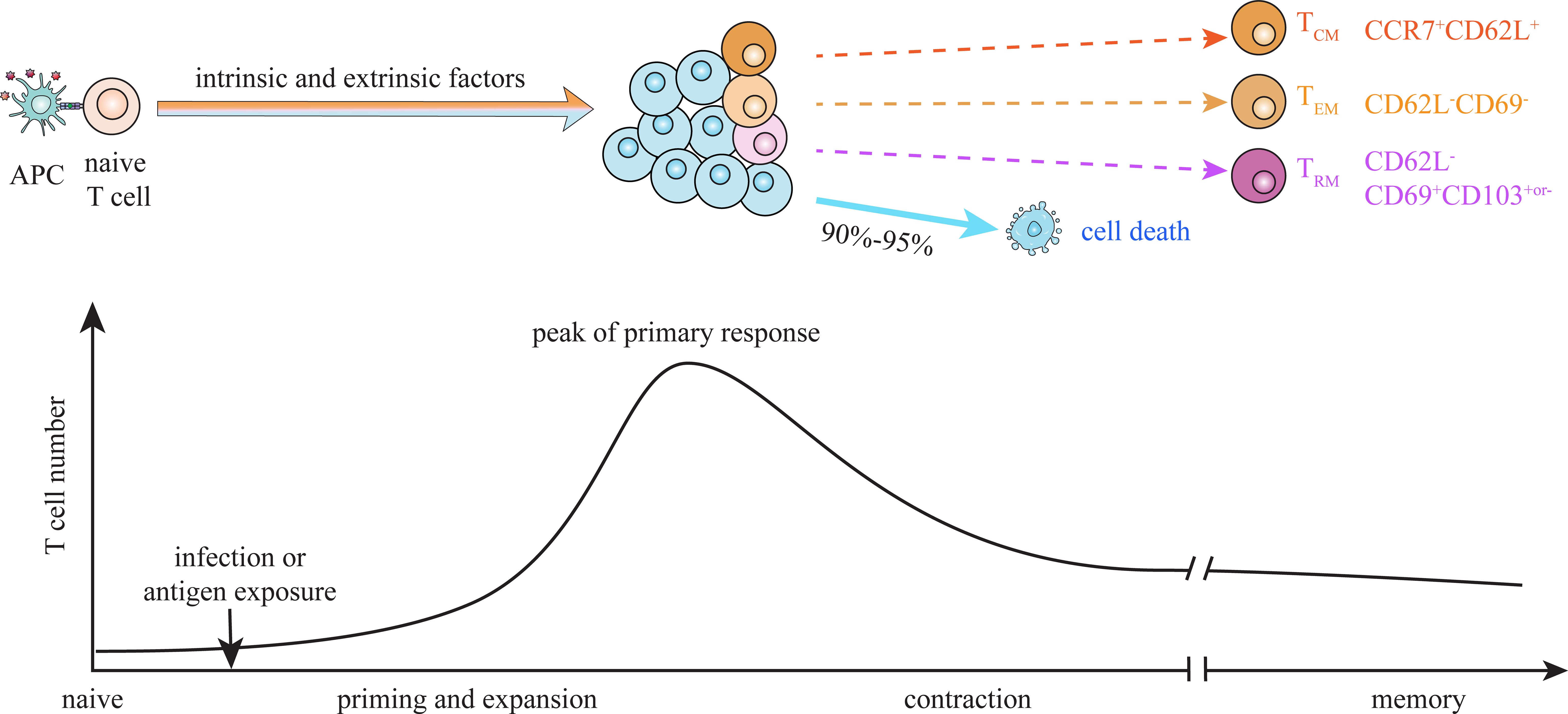
Figure 1. Dynamics of T cell memory formation. Upon infection or antigen exposure, naïve T cells are primed and activated by antigen-presenting cells (APCs) through pMHC-TCR interaction. Activated T cells vigorously expand and differentiate into functional effector cells during priming, and contract after antigen clearance. A small proportion of antigen-activated T cells survive contraction and become long-lived memory T (TM) cells. The differentiation fate of activated T cells between effector and memory is suggested to be dictated by dynamic interaction of multiple intrinsic and extrinsic factors during priming stage. Heterogeneous populations at the peak of primary response exhibit different potential to give rise to terminal effector cells and TM cells. Central memory (TCM, CD62L+CCR7+), effector-like memory (TEM, CD62L-CD69-) and tissue resident memory (TRM, CD62L-CD69+CD103+or-) cells are suggested to derive from distinct progenitors, which emerge at priming and expansion stage.
The core theories of memory generation are primarily derived from studies on differentiation of CD8+ TM cells (1, 7). The identification of CD127hiKLRG1lo memory precursor effector cells (MPECs) and CD127loKLRG1hi short-lived effector cells (SLECs) at the peak of primary response (day 7–8 post-infection) provides a guiding framework for a deeper understanding of the fate decision between effector and memory CD8+ T cells (Figure 2) (8–11). TCF1hi cells within CD127hi MPEC pool, which exhibit stem-like properties and undergo less cytotoxic differentiation, substantially give rise to TCM cells, while TCF1loCD127hi population contracts and becomes TEM cells following infection elimination (3, 12–14). Preferential localization of TCF1hi TCM precursors in paracortex (T cell zone) of secondary lymphoid organs (SLOs), through CCR7-mediated chemotaxis toward CCL19/21, facilitates their encounter with IL-7, thus enhancing their transition into CD8+ TCM cells (Figure 2) (15–20). Meanwhile, T-bet-induced CXCR3 drives the migration of activated CD8+ T cells to peripheral region of SLOs and inflamed non-lymphoid tissues (NLTs), facilitating their interaction with inflammatory signals and thereby promoting their differentiation into CD127loKLRG1hi terminal effector cells (9, 19, 21). CD69+CD103+ CD8+ TRM cells are suggested to derive from a MPEC-like (CD127hi) population residing in NLTs, and Hobit and Blimp-1 potentially identify TRM precursors in different NLTs (22–25). Moreover, early-activated TCF1hi and TCF1lo cells (day 2–4 post-infection), which already possess distinct memory potential, exhibit reversible plasticity between effector and memory fates in response to inflammatory stimulation or the withdrawal of such stimulation (12, 14, 26, 27). The differentiation fate of antigen-activated CD8+ T cells between effector and memory is collaboratively determined by intrinsic factors, extrinsic factors and positioning profiles that facilitate intrinsic-extrinsic interactions during the whole priming phase under acute infection (1, 3, 28–31).
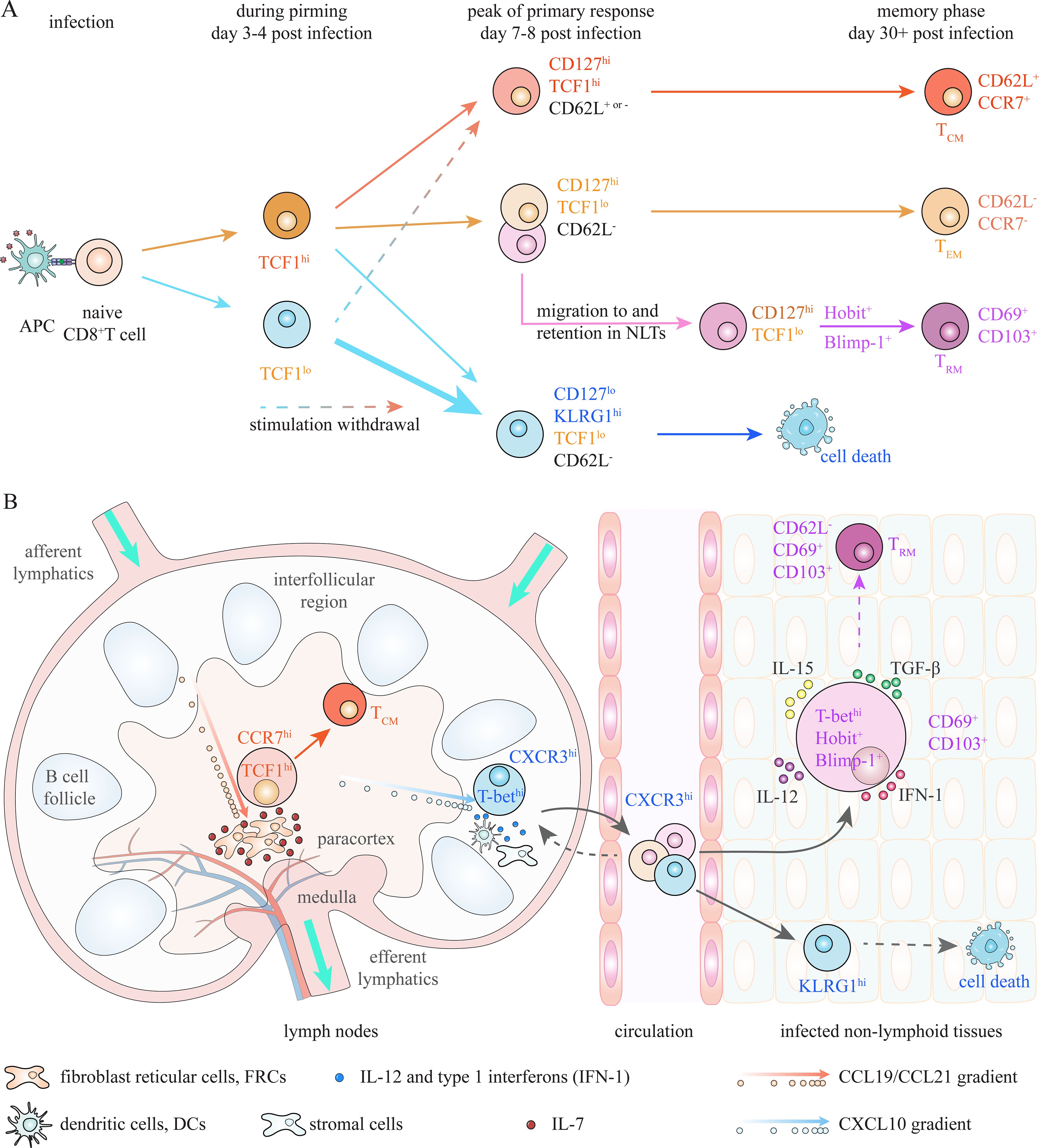
Figure 2. Advancing insights into precursors of CD8+ TM cells. (A) At the peak of primary response, activated CD8+ T cells can be subdivided into CD127hiKLRG1lo memory precursor effector cells (MPECs), which possess greater potential to generate TM cells, and KLRG1hiCD127lo short-lived effector cells (SLECs), which mostly dismiss during contraction. TCF1hi cells within CD127hi MPEC pool, which substantially survive contraction and generate TCM cells, are identified as TCM precursors. Meanwhile, circulating effector-like memory T (TEM) cells and tissue-resident memory T (TRM) cells are suggested to derive from TCF1loCD127hi progenitors. Moreover, the diversification of T cell fate is suggested to occur at even earlier stage. Activated TCF1hi and TCF1lo T cells at day 3–4 post-infection already possess distinct memory potential; however, they exhibit plasticity when microenvironment changes. Fate commitment of CD8+ T cells between effector and memory depends on dynamic interactions of intrinsic and extrinsic factors during whole priming stage. (B) Localization in proper niches to encounter appropriate extrinsic factors is also critical for effector and memory differentiation. Localization of TCF1hiCD127hi TCM precursors in the paracortex (T cell zone) of lymphoid nodes (LNs), where there is high amount of IL-7 produced by fibroblast reticular cells (FRCs), through CCR7 mediated chemoattraction towards FRC-derived CCL19/CCL21, is essential for generation of CD8+ TCM cells. Meanwhile, CXCR3-mediated migration of CD8+ T cells to interfollicular region of LNs and non-lymphoid tissues (NLTs), through chemotaxis towards CXCL10, facilitates their interaction with high amount of antigen and inflammatory signals, which is essential for effector differentiation. Moreover, optimal TRM generation depends on interaction with local extrinsic factors in NLTs. Expression of CD69 and/or CD103, which is regulated by several intrinsic (T-bet, Hobit and Blimp-1) and extrinsic (TGF-β) factors, is suggested to be required for retention of activated CD8+ T cells in NLTs.
Though there is no unifying framework, the current understanding of CD8+ TM cell generation provides a valuable guidance for investigations into differentiation of CD4+ TM cells. However, much less is known about generation of CD4+ TM cells and their precursors to date (5, 32). Unlike CD8+ T cells, antigen-activated CD4+ T cells can differentiate into Th1, Th2, and Th17 effector cells, which are typically identified by secretion of signature cytokines, under different types of infections and exposures (33). Th1 cells, characterized by IFNγ secretion, are induced by the master transcription factor T-bet in response to intracellular viral and bacterial infections. IL-4-secreting Th2 cells are promoted by transcription factor Gata3 upon exposure to extracellular parasites/helminths and allergens. The differentiation of IL-17-secreting Th17 cells depends on expression of transcription factor RORγt in response to fungal and extracellular bacterial infections. Additionally, Th1/2/17 responses are all associated with Bcl6-dependent parallel differentiation of CXCR5+PD-1+ follicular helper T (Tfh) cells, which play pivotal roles in promoting B cell responses within germinal center (GC) (34). Th1/2/17 and Tfh cells both could survive contraction and become TM cells after immunological threat is eliminated (5, 35–39). The heterogeneity of activated CD4+ T cells adds complexity to studying the generation of CD4+ TM cells.
Drawing on insights from the theory of CD8+ TM cell generation, this paper presents current advances in identifying precursors of Th1, Th2, Th17, and Tfh memory cells, as well as elucidating the mechanisms underlying fate decision of CD4+ TM cells. We also discuss the challenges and prospects for identifying common precursor of specific CD4+ TM cells under various types of infections and exposures.
2 Advancing insights into CD4+ TM cell precursors during Th1 responses
2.1 Diverse effector and memory CD4+ T cell subsets
Consistent with CD8+ TM subsets, CD4+ TM cells can be traditionally categorized into CD62L+CCR7+ TCM cells, CD62L-CCR7- TEM cells, and CD69+ TRM cells under bacterial and viral infections (Figure 3) (36, 40, 41). TCM cells, which circulate through lymph nodes, can efficiently generate secondary Th1 effectors upon rechallenge, while TEM cells, which migrate between NLTs, are able to rapidly produce IFNγ following rechallenge (36, 37, 41, 42). TRM cells, which mainly reside in NLTs, mediate rapid local recall responses (43). Additionally, CCR7-CXCR5+ Tfh memory cells, which are essential for secondary B cell responses, have been identified as a distinct memory population, although they exhibit phenotypic similarities with CCR7+CXCR5+ TCM cells, including the expression of TCF1, CD127 and CXCR5 (37, 41, 44–46). The diverse TM subsets are suggested to derive from relevant effector cell populations at peak of primary responses (35, 39). Currently, two commonly used approaches, based on expression of CXCR6/CXCR5/CCR7 and Ly6C/PSGL1/FR4, are employed to identify effector and memory populations by flow cytometry.
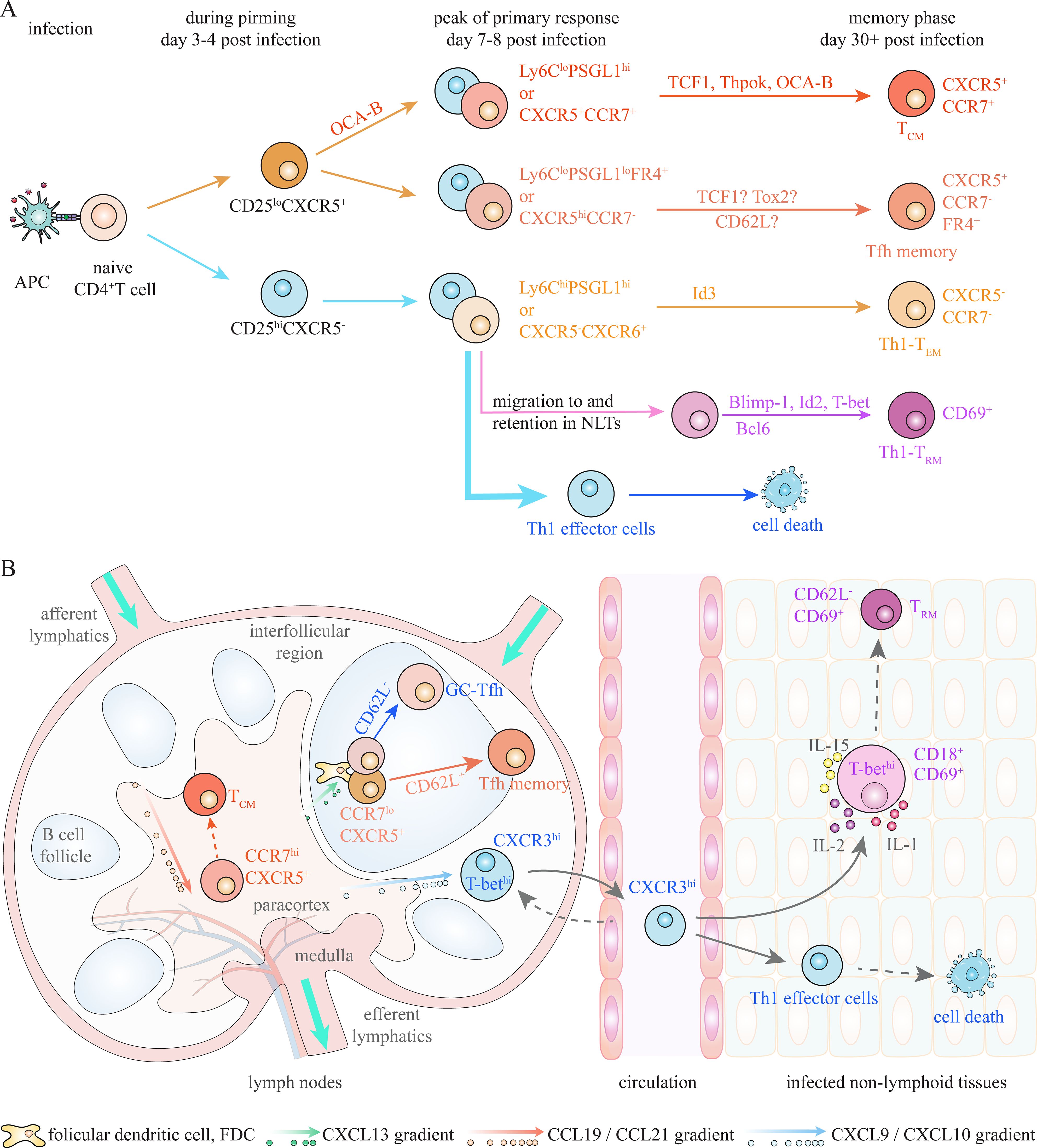
Figure 3. Potential memory precursors during Th1/Tfh responses under infection. (A) Activated CD4+ T cells at the peak of primary response can be subdivided into several populations, which enrich precursors of TCM, Th1-TEM, Th1-TRM and Tfh memory cells respectively, based on expression of Ly6C/PSGL1/FR4 and/or CXCR5/CCR7/CXCR6. Multiple factors have been revealed to regulate generation of CD4+ TM cells during Th1/Tfh responses under bacterial and viral infections, and thus can act as putative marker for memory precursors. Moreover, IL-2/CD25 signaling plays critical role in determining fate choices between Th1 effector and Tfh/TCM cells at early stage of priming. (B) Spatial localization of CD4+ T cells also contributes to effector and memory differentiation under infections. CCR7 retains activated CD4+ T cells in paracortex (T cell zone), despite co-expression of CXCR5, which is essential for generation of TCM cells. Migration to interfollicular region and entry into peripheral non-lymphoid tissues (NLTs), which is mediated by CXCR3-CXCL9/10 chemotaxis, promotes optimal Th1 effector differentiation. NLTs residency of activated T cells, which is partly regulated by CD69 and T-bet-induced CD18, is required for Th1-TRM generation. CXCR5-mediated positioning in germinal center (GC) is required for GC-Tfh and Tfh memory cell differentiation. A CD62L+PD-1lo subpopulation of Tfh-like (CXCR5+BCL6+) cells, which exhibit less preference to interact with B cells, is suggested to efficiently generate Tfh memory cells.
The first approach subdivides activated CD4+ T cells into CXCR6+CXCR5- Th1-like cells and CXCR6-CXCR5+ Tfh-like cells at the peak of immune response during viral infection (37, 39, 41). CXCR5-CXCR6+T-bet+ IFNγ-secreting Th1 effector cells can partially survive contraction and give rise to CXCR5-CCR7- Th1-TEM cells (36, 38, 39, 41). CCR7+ cells within CXCR5+ Tfh-like population at effector phase, which exhibit a less differentiated state, primarily develop into TCM cells identified by the CCR7+CXCR5+ phenotype (37, 41, 42). CXCR5+CCR7- Tfh cells during the priming phase can give rise to CXCR5hiPD-1hi GC-Tfh cells and CXCR5+CCR7- Tfh memory cells (39, 46, 47). However, CXCR5 expression on Tfh cells has been shown to decrease or even disappear during memory phase; therefore, CXCR5-/lo TM cells might actually contain Tfh memory cells (37, 42, 45). In addition, CXCR5-/lo TM cells also contain a CCR7+ TCM-like population (36, 41, 42). This suggests a certain degree of unreliability of this approach in identifying above CD4+ T cell subsets during effector and memory phases.
Another approach partitions activated CD4+ T cells into Ly6ChiPSGL1hi, Ly6CloPSGL1hi, and Ly6CloPSGL1lo populations at effector phase, and these populations are also observed at memory stage (44, 45, 48, 49). Ly6ChiPSGL1hi population primarily consist of differentiated Th1 effector cells, which can give rise to Ly6Chi Th1-TEM cells (44, 45, 48, 49). Ly6CloPSGL1lo population, with exclusively high expression of FR4, contains Tfh cells at both effector and memory phases (37, 45, 48). Single-cell transcriptomic experiments suggest that FR4 is a reliable marker for distinguishing effector and memory Tfh cells from CCR7+ TCM cells and their precursors, while CXCR5 is expressed on both Tfh and non-Tfh cells (37, 45). Ly6CloPSGL1hi effector population, which exhibits greater memory potential, is a heterogenous population comprising CXCR5- Th1-like cells and CXCR5+ Tfh-like cells (44, 45). This population may contain TCM precursors, as CD62L+CCR7+ TCM cells are preferentially enriched in the Ly6CloPSGL1hi TM cells at memory phase (37, 44, 45, 48).
Based on the above information, activated CD4+ T cells at the peak of priming consist of at least three populations: CXCR5-CXCR6hiLy6Chi Th1 effector cells, CCR7+CXCR5+ cells enriched for TCM precursors, and CCR7-CXCR5+FR4+ Tfh cells (37, 39, 41, 45). It is suggested that a proportion of cells in each population survive contraction and become CD4+ TM cells following infection elimination (35, 39, 41, 42). Additional heterogeneity clearly exists within these populations; however, mechanisms driving their transition into CD4+ TM cells remain under investigation.
2.2 Intrinsic factors that determine fate decision between effector and TM cells
Fate mapping studies have demonstrated that single naïve CD4+ T cells can give rise to effector and memory Th1 and Tfh cell populations during infection (38, 50). Similar to CD8+ T cells, differentiation fate of CD4+ TM cells is suggested to be determined during the priming phase of infection (32, 39, 41). A microarray study indicates that the generation of CD4+ TM cells relies on a combination of programs that inhibit proliferation and apoptosis while promoting DNA damage repair and lipid metabolism during Th1 response in malaria infection (51). Multiple factors have been revealed to be essential for CD4+ TM cell differentiation.
It is well-established that CD127 and TCF1 play decisive roles in CD8+ TM cell differentiation (3, 8, 9, 12–14). However, CD127 expression is unable to distinguish CD4+ TM cell precursors from terminal Th1 effector cells, although it is required for maintenance and homeostasis of CD4+ TM cells (20, 37, 41, 45, 48, 52). TCF1 has been shown to be involved in maintaining stemness and promoting formation of TCM cells; however, it also promotes Tfh polarization and inhibits Th1 effector differentiation under viral infections (39, 52–57). Another T cell intrinsic factor, Thpok, which is essential for the differentiation of CCR7+ TCM precursors, also plays critical role in Tfh cell differentiation under lymphocytic choriomeningitis virus (LCMV) infection (41, 58). Therefore, neither TCF-1 nor Thpok can faithfully identify CD4+ TCM precursors. In addition, Bcl6, which is indispensable for Tfh differentiation, contributes to the generation of CCR7+ TCM cells primarily through repressing Blimp-1 expression (42, 59, 60). Id3 also contributes to generation of CXCR5+CCR7+ TCM cells and CXCR5+CCR7- Tfh-like memory cells under LCMV infection (36). It is challenging to identify reliable precursors of TCM cells due to the shared programs underlying differentiation of TCM and Tfh cells. Recently, OCA-B (Pou2af1), which increases Th1-like cells and reduces Tfh-like cells during priming, has been shown to be necessary and sufficient to drive the generation of CD44+CD62L+ TCM cells under LCMV infection, and thus can prospectively identify TCM precursors (61).
Meanwhile, Th1-TEM cells have been shown to derive from in vitro activated IFNγ-secreting Th1-like effector cells (62, 63). Expression of CD30 (Tnfrsf8) and inhibition of ACC1 are demonstrated to enhance generation of Th1 memory cells, although these studies did not investigate Tfh cells and diverse TM lineages (64, 65). A recent study reveals that expression of Id3 identifies a memory precursor-like population within CXCR5- Th1-like cells, which can survive contraction phase and become CXCR5-CCR7- Th1-like TEM cells (36).
Furthermore, TCR-dependent CD25 (IL-2Rα) expression is suggested to predict the differentiation fate of activated CD4+ T cells as early as day 3 post-infection (Figure 3A) (42, 57, 66). Indeed, IL-2/IL-2R signaling have been demonstrated to play a crucial role in determining fate choices between effector and memory CD8+ T cells (67). Following the initial induction of CD25 after TCR activation, its rapid downregulation on activated CD8+ T cells during priming and diminished IL-2 signaling is essential for generation of CD8+ TM cells, whereas prolonged IL-2/CD25 signaling promotes terminal effector differentiation (67). Similarly, early-activated CD25hi CD4+ T cells (day 3 post-infection), which lack CXCR5 expression, almost exclusively develop into terminal Th1 effector cells, whereas CD25lo cells, which generally express CXCR5, give rise to Tfh and TCM cells (42, 57, 66, 68). Moreover, early-activated CD25lo cells are predominantly enriched for OCA-Bhi cells, although a proportion of CD25hi cells also express lower level of OCA-B (61). It is indicated that high expression of OCA-B at the early stage of priming contributes to the commitment of CD25lo cells to a TCM cell fate. However, whether early-activated CD25hi and CD25lo CD4+ T cells exhibit plasticity in response to changes in the microenvironment remains to be further investigated. Notably, it has been demonstrated that IL2/CD25 signaling promotes survival of activated T cells and thus formation of TCM and TEM cells, through upregulating re-expression of CD127 during contraction phase (69–71). Nevertheless, IL-2/CD25 signaling at early stage of priming contributes to determining the differentiation fates between Th1 effector cells and Tfh/TCM cells during Th1 response (57).
2.3 Positioning profiles that dictate differentiation fate between effector and TCM cells
As well as intrinsic factors, spatially distributed antigens and cytokines within microenvironment also play essential roles in CD4+ T effector and memory differentiation (Figure 3B) (32, 33). Inflammatory cytokines within inflamed NLTs, such as IL-12 and IFNγ, are critical for the differentiation of IFNγ-secreting Th1 effector cells, partly through promoting T-bet expression (72–76). Meanwhile, high level of IL-7 in SLOs produced by fibroblast reticular cells (FRCs) promotes generation of CD4+ TCM cells, although its role in this process is not as prominent as in the formation of CD8+ TCM cells (20). T-bet-induced CXCR3 expression on activated CD4+ T cells mediates their migration rapidly out of lymph organs and into inflamed peripheral NLTs, thereby promoting optimal Th1 effector differentiation during influenza virus (IAV) infection (77, 78). Meanwhile, upregulation of CD62L in TCF1hi cells leads to their enrichment in SLOs rather than accumulation in lungs, and thus contributes to TCM cell formation during Th1 response in response to IAV infection (52).
Moreover, precise localization of activated CD4+ T cells within SLOs also contributes to determining their fate choices between effector and memory (Figure 3B). Peripheral region within secondary lymphoid organs (SLOs) provides abundant antigen and inflammatory cytokines (IL-12, and IFNγ), while IL-7-producing fibroblast reticular cells (FRCs) are restricted in the center (T cell zone) of SLOs (7, 79, 80). CXCR3 directs the migration of activated CD4+ T cells to interfollicular region of LNs, which is strongly correlated with increased Th1 effector differentiation under viral infection (81, 82). Retention of activated T cells in the T cell zone, regulated by CCR7-mediated chemotaxis towards FRC-produced CCL19/CCL21, facilitates their encounter with high amount of IL-7 and protects them from excessive inflammatory stimulation, thus promoting CD4+ TCM generation during Th1 responses (15, 20, 83, 84). In line with this, CCR7+CXCR5+ T cells, which enrich precursors of CD4+ TCM cells, predominantly localize in T cell area of SLOs (37, 41, 42). Additionally, Ly6CloPSGL1hi cells with greater memory potential also preferentially localize in T cell zone, while Ly6Chi cells, which mainly give rise to terminal Th1 effectors, migrate to peripheral sites of spleen under LCMV infection (48).
Similar to CD8+ T cells, retention in T cell area of SLOs is required for CD4+ TCM formation, whereas migration to periphery of SLOs and entry into NLTs promote Th1 effector differentiation. The balance between CCR7 and CXCR3 expression contributes to dictating differentiation fate between CD4+ TCM and Th1 effector cells. Yet, spatial requirement of Th1-TEM cell generation remains unclear.
2.4 Identifying precursors of Tfh memory cells during Th1 responses
There are also several factors that have recently been shown to be involved in generation of Tfh memory cells during Th1 responses. In addition to its role in Tfh lineage polarization, TCF1 has been shown to be essential for the generation and maintenance of Tfh memory cells (52–56). Tox2 is also required for GC-Tfh differentiation and Tfh memory cell generation under IAV infection (85). Moreover, PD-1+CXCR5+ Tfh-like cells with sustained expression of Tigit preferentially differentiate into GC-Tfh cells, although Tigit is not functionally critical for differentiation and function of GC-Tfh cells (84). Meanwhile, Tigit-negative Tfh-like cells upregulate CD127 expression by day 14 post-infection (after GC formation) and give rise to CXCR5+ TM cells with or without CCR7 expression (84). Recently, a CD62L+PD-1lo subpopulation within CXCR5+BCL6+ Tfh cells, which highly expresses KLF2 and CD127, exhibits memory precursor-like transcriptional profiles and readily generates PD-1hi Tfh effector cells upon recall (86). In addition, the CD62L+PD-1lo population exhibits a reduced preference for B cell interaction (86). The observation is consistent with the suggestion that avoiding excessive stimulation from B cells is essential for Tfh memory cell generation (46, 84, 85). Although functional requirements of Tigit and CD62L in generation of Tfh memory cells is unclear, they can putatively act as phenotypic markers for distinguishing between progenitors of GC-Tfh and Tfh memory cells.
Spatial requirement for development of Tfh memory cells appears to be different from TCM and Th1 effector cells. Expression of CXCR5, which facilitates positioning of pre-Tfh cells at T:B border and entry into follicle to appropriately interact with B cells, is essential for further GC-Tfh and Tfh memory cell differentiation (84, 87–91). Upregulation of PD-1 also promotes accumulation of Tfh-like cells in the GC territory, partly through inhibiting expression of CXCR3 which can otherwise distract Tfh-like cells from GC localization (91, 92). Meanwhile, CCR7 expression retains activated CD4+ T cells in T cell zone and thus inhibits their differentiation towards Tfh fate, despite co-expression of CXCR5 (84, 89, 90). Expression of CXCR5, as well as downregulation of CCR7 and CXCR3, drives migration of activated CD4+ T cells toward B cell follicles and GCs, thus facilitating differentiation of GC-Tfh and Tfh memory cells.
2.5 Identifying precursors of Th1-TRM cells in NLTs
Th1-TRM cells, which exhibit Th1 effector and memory profiles, are suggested to derive from Th1 effector cells residing in NLTs under bacterial and viral infections (Figure 3A) (93, 94). IL-2/CD25 signaling has also been demonstrated to enhance Th1-TRM generation in the lung through promoting optimal effector differentiation during IAV and LCMV infection (95, 96). Th1-associated T-bet, Blimp-1, and Id2 are demonstrated to be required for Th1-TRM generation in liver and small intestine during Salmonella and LCMV infections (93, 94). Meanwhile, Hobit and Blimp-1, which plays crucial role in CD8+ TRM cell generation, have been shown to be dispensable for CD4+ TRM cell formation in the colon during experimental colitis, although their deficiency impairs the expression of pro-inflammatory cytokines in CD4+ TRM cells (24, 25, 97). Differential requirements for Blimp-1 indicate that the mechanisms underlying Th1-TRM formation may vary across different tissues and types of infections. On the other hand, IL-15 receptor-mediated direct IL-15 signaling within the first week promotes the generation of viral-specific Th1-TRM cells through enhancing their survival and persistence in the lung during IAV infection (96). Tfh-associated Bcl6 contributes to Th1-TRM generation in the small intestine under LCMV infection, probably through enhancing memory attributes (94). It is suggested that the factors contributing to effector differentiation and survival are both required for Th1-TRM cell generation. However, none of these factors exhibit the ability to reliably distinguish TRM precursors from terminal Th1 effector cells within NLTs.
Similar to CD8+ TRM cells, the transition of activated CD4+ T cells into Th1-TRM cells also depends on their interaction with local inflammatory (IL-2 and IL-1) and survival (IL-15) cytokines in microenvironment of livers and lungs during bacterial and viral infections (95, 96, 98). The factors that promote entry and retention of activated CD4+ T cells in NLTs are essential for the generation of TRM cells. IL-2/CD25 signaling contributes to Th1-TRM generation through promoting residency of activated CD4+ T cells in the lung during IAV and LCMV infection (95, 96). Blimp-1 has also been shown to promote the accumulation of potential early TRM precursors in small intestine, thus enhancing Th1-TRM generation during LCMV infection (94). T-bet-induced CXCR3, which is highly expressed on Th1 effector and TRM cells, promotes entry of activated CD4+ T cells into liver and lung during Salmonella and IAV infections; however, its role in TRM cell generation is unclear (77, 98). Unlike CD8+ TRM cells, CD103 appears not to be uniformly required for the retention of activated CD4+ T cells in various NLTs, as it is frequently absent from Th1-TRM cells (40, 43, 94, 98). CD69, which is highly expressed on Th1-TRM cells in multiple NLTs, are currently regarded as a phenotypic marker for TRM cells and their precursors (Figure 3B), although its role in NLTs residency of Th1 effector and TRM cells is ambiguous (40, 95, 96, 98). Additionally, CD18, induced by highly expressed T-bet, is suggested to be essential for positioning of activated CD4+ T cells into liver niches and thus TRM generation under Salmonella infection (Figure 3B) (93). Yet, whether CD69 and CD18 are capable of identifying precursors of Th1-TRM cells within different NLTs under various types of infections remains to be further clarified. Variations in the programs governing the generation of Th1-TRM cells across different types of infections and tissues increase the complexity of identifying their reliable precursors.
3 Potential precursors of Th2 memory cells
In comparison to Th1 cells, memory generation of Th2 cells has been much less studied. Th2 memory cells, including TCM cells in lymph nodes, TEM cells, TRM cells in lungs, and Tfh-like memory cells all can be generated after antigen clearance under helminth/parasite infection (63, 87, 99, 100). However, Th2 and Tfh-like cells in LNs exhibit great plasticity under parasite infection, and a proportion of activated CD4+ T cells appear to co-express Tfh marker Bcl6/CXCR5 and Th2 marker Gata3/IL-4 (101, 102). Meanwhile, entry of activated CD4+T cells into lungs, which promotes terminal IL-4+IL-13+ Th2 effector cell differentiation, depends on downregulation of the hallmark Tfh transcription factor Bcl6, indicating a distinction between them (99, 101). The lack of clear delineation between Th2 and Tfh cells complicates the investigation into their memory generation under Th2 conditions.
Without considering the existence of Tfh cells, antigen-activated CD44hiCD62L+ CD4+T cells, which preferentially accumulate in LNs rather than lungs, are suggested to enrich TCM precursors (103, 104). CD44hiCD62L+ cells, with high co-expression of CCR7, maintain a less differentiated state as indicated by lower expression of Gata3 and IL-4 (103–105). It is consistent with the observation that CCR7-mediated retention of activated CD4+ T cells in T cell zone inhibits Th2 effector differentiation (87, 106). Meanwhile, CXCR5-mediated migration towards peripheral region of LNs, in a CXCL13-dependent manner, is required for differentiation of both IL-4-producing Th2-like cells and Tfh cells (87, 106). It is indicated that positioning into periphery and center of SLOs also contributes to differentiation of Th2 effector cells and TCM cells, respectively, during Th2 responses. On the other hand, retention of Th2 effector cells in NLTs facilitates their encounter with local extrinsic signals and thus promotes Th2-TRM cell generation. Local IL-7 signaling is essential for maintenance of Th2-TRM cells in lungs (105). IL-2/CD25 signaling has been shown to promote Th2-TRM generation through enhancing optimal effector differentiation and residency of Th2 cells in lungs (99). CD69, which is highly expressed on Th2-TRM cells, might be required for their generation through promoting lung residency of Th2 effector cells (99). Although the underlying mechanisms are not well-defined, these investigations indicate similarities in spatial requirements for memory generation between Th2 cells and Th1/CD8+ T cells. In addition, ablation of ACC1, which is required for Th1 memory cell formation, also enhances generation of Th2 memory cells via regulating fatty acid oxidation under helminth infection (65). It suggests that Th2 memory cell generation depends on some mechanisms shared with Th1 cells.
Despite the similarities mentioned above, it should not be simply presumed that factors involved in formation of CD8+ TM and Th1 memory cells play the same role in Th2 memory cell generation. CD127, the key marker for identifying CD8+ TM cell precursors, is barely expressed on Th2-like effector cells in LNs during priming and thus cannot mark Th2 memory precursors, although it has been shown to be essential for homeostasis of Th2 memory cells (104, 105). Ly6C and PSGL1, which are used to identify memory precursors from Th1 effector cells, are barely expressed on ovalbumin-specific Th2-like effector cells at the peak of Th2 responses (104). CXCR5, a key marker to distinguish between Th1 effector cells and Tfh/TCM cells, is expressed on both Tfh and Th2 cells in lymph nodes during priming (87, 106). RNA-seq data also demonstrate that TCF1, Thpok and Id3, which are essential for TCM and Th1-TEM generation under viral infection, are comparably expressed on CD62L- Th2 cells and TCM precursor-enriched CD62L+ Th2 cells (104). Overall, independent investigations into generation of Th2 memory cells are definitely required.
4 Potential precursors of Th17 memory cells
Few investigations have been performed into memory generation during Th17 responses, while the heterogeneity of TM cells and the existence of Tfh memory cells have remained largely unexamined. Th17 memory cells are shown to derive from IL-17+ or RORγT+ Th17-like effectors; however, how effector cells contribute to the ultimate Th17 memory cell pool remains ill-defined (107, 108). IL-7 and IL-15 have been shown to promote the maintenance of Th17 memory cells at inflammatory site and draining lymphoid tissues (109). IL-23/IL-23R signaling directly drives effector to memory conversion of Th17 cells via upregulation of CD127 and IL-15 receptor during contraction phase, while IL-2 prominently impairs IL-23-induced Th17 memory cell generation (110). It suggests that surviving signals are also required for Th17 memory generation. CD30, which promotes Th1 memory generation, plays critical role in generation of Th17 memory cells, and thus can serve as a prospective marker for Th17 memory precursors (64). Moreover, retention of Th17 cells in the lung and skin to interact with IL-1α and local IL-23 is required for CD69+ Th17-TRM generation, although factors that mediate their residency remain unclear (111, 112). Though the information are fragmented, the differentiation of Th17 memory cells exhibits both similarities and differences with CD8+ TM cells and Th1/2 memory cells.
5 Challenges to develop a general model of CD4+ TM cell generation
Despite the above advances in characterizing precursors and elucidating fate decision mechanisms of CD4+ TM cells, current knowledge remains insufficient. A deeper understanding of generation of CD4+ TM cells has been hindered by several technological and biological challenges. The first challenge is to trace antigen-specific CD4+ T cells in vivo. TCR-transgenic T cell adoptive transfer system and peptide:MHC tetramer technology, which are vital tools for studying antigen-specific CD8+ T cells, both exhibit limitations in studying CD4+ T cells (5, 32). Moreover, the number and expansion capability of antigen-specific naïve CD4+ T cells is much lower than CD8+ T cells, and CD4+ TM cells are suggested to be less stable over time (32, 42, 113, 114). Low number of antigen-specific CD4+ TM cells makes them harder to be detected in vivo.
In addition to technical limitations, the functional and phenotypic heterogeneity of CD4+ T cells at effector and memory phase poses significant challenges to establishing a unifying framework for CD4+ TM cell generation. As mentioned above, Th1/2/17 and Tfh populations both can give rise to TM cells after immunological threat is eliminated (5, 35–39). Plenty of T cell intrinsic and extrinsic factors, which contribute to generation and maintenance of CD4+ TM cells, are also involved in polarization of naïve T cells towards Th1/2/17 and Tfh lineages (5, 33, 39, 58, 115). For instance, TCF1 and Thpok, which are shown to be required for generation of TCM cells, also promotes Tfh cell differentiation during Th1 responses (41, 52, 55, 58). Bcl6, which plays pivotal role in Tfh differentiation, is also required for TCM and Th1-TRM generation (58, 59, 94). Moreover, lack of a unifying approach to faithfully identify Th1/Th2/Th17 and Tfh populations complicates the study of CD4+ TM cell differentiation. Th1, Th2 and Th17 cells are generally identified by secretion of IFN-γ, IL-4 and IL-17 respectively; however, Bcl6+ follicular helper T (Tfh) cells are also able to produce these hallmark cytokines (34). Besides, the two commonly used approaches, based on expression of Ly6C/PSGL1/FR4 and CXCR6/CXCR5/CCR7, both cannot unambiguously identify the diverse CD4+ T cell subsets during effector and memory phases of Th1 responses (32, 37, 39, 45). Expression of CXCR5 and production of IL-4 are also not able to discriminate between Th2 and Tfh cells under parasite infection (101, 102). The developmental relationships between Th/Tfh polarization and memory generation remain incompletely elucidated, largely due to shared mechanistic pathways and phenotypic overlaps between diverse CD4+ T cell subsets.
Furthermore, the plasticity of CD4+ T cell subsets at population level also increases complexity in studying CD4+ T memory generation (116). During Th1 responses, CXCR5+ TM cells give rise to secondary Th1 and Tfh effector cells upon rechallenge, indicating heterogeneity or plasticity of them (44). Recent data demonstrate that CXCR5+ memory cells contain a TCM-like (CCR7+ or Ly6CloPSGL1+) population, which primarily gives rise to secondary Th1 effectors and generates few Tfh cells (41, 45, 117). However, remaining Tfh memory cells (Ly6CloPSGL1loFR4+ or CXCR5+CCR7lo), which efficiently generate Tfh effector cells, equally give rise to secondary Th1 effectors upon challenge (41, 45). It might be because Tfh-like memory cells indeed possess plasticity, or they still represent a heterogeneous population. In addition, Gata3+ Th2 and Bcl6+ Tfh cells exhibit great plasticity, as each can give rise to both cell types following helminth challenge (101, 102). On the other hand, a specific CD62L+CXCR5+Bcl6+ Tfh cell population has recently been shown to efficiently generate Tfh effectors during secondary response, indicating lineage commitment of it (86). Besides, Th1-TEM cells (Ly6Chi and/or CXCR5lo) almost exclusively generate secondary Th1 effector cells upon rechallenge (38, 42, 44, 45, 49). Some other fate mapping and single-cell studies also indicate that CD4+ TM cells exhibit minimal plasticity and might be lineage-committed upon recall (38, 39). A possible explanation is that the plasticity of CD4+ TM cells at population level is associated with additional heterogeneity (116). Nonetheless, the plasticity at the population level currently hinders a deeper understanding of CD4+ TM cell generation.
6 Prospects for identifying potential common precursors of specific CD4+ TM cells
As discussed above, CD4+ TM cells are suggested to derive from antigen-activated T cell progenitors, and the memory fate is primarily dictated during priming phase of primary response (39, 62). However, the lack of a guiding model significantly hinders further investigations into the mechanisms underlying generation of CD4+ TM cells. Identification and characterization of potential common precursors of specific CD4+ TM cells under Th1, Th2 and Th17 conditions would be beneficial for further investigating the programs of fate decision between effector and memory CD4+ T cells.
Unfortunately, the current theory of CD8+ TM cell generation provides only limited guidance for identifying precursors of CD4+ TM cells. As a reliable marker for CD8+ TM precursors (8, 9), role of CD127 in generation of CD4+ TM cells has attracted considerable attentions. Multiple studies demonstrate that CD127 mediated IL-7 signaling is required for maintenance and homeostasis of CD4+ TM cells; however, CD127 seems to be downregulated at effector phase and thus cannot identify memory precursors at the peak of Th1, Th2 and Th17 responses (48, 52, 69, 84, 86, 104, 108, 110, 118). Nonetheless, revealing programs that regulate re-expression of CD127 in activated CD4+ T cells during contraction phase might be a breakthrough for identifying common memory precursors, as IL-7/CD127signaling has been shown to be essential for survival of activated CD4+ T cells during this phase (52, 84, 110, 119). On the other hand, the dual roles of TCF1 in CD4+ TM cell differentiation and Tfh polarization make it an unreliable marker for memory precursors during Th1/Tfh response under viral infections (39, 52–57). Besides, RNA-seq data indicates that TCF1 is not involved in formation of TCM precursor-like CD62L+ T cells during Th2 response (104). The exact role of TCF1 in memory generation and Th/Tfh lineage polarization still needs to be defined. Collectively, CD127 and TCF1 are not reliable markers for discriminating CD4+ T memory precursors from terminally differentiated effectors.
Programs of CD4+ TM cell differentiation also exhibit great differences under Th1, Th2 or Th17 conditions (5, 43, 94). Commonly used markers during Th1 responses, such as Ly6C, PSGL1 and CXCR5, seem unable to distinguish between TCM precursors, Th2 effector cells, and Tfh cells under Th2 conditions (101, 102, 104). Besides, whether TCM-associated factors during Th1 response, such as TCF1, Thpok and OCA-B, also participate in regulating memory formation under Th2 and Th17 conditions remains ill-defined (32, 58, 104). The mechanisms of TCM and Tfh memory cell differentiation under various types of infections and exposures are far from clear. Moreover, the generation of CD4+ TEM and TRM cells are closely related to Th1, Th2, Th17 and even Tfh effector cell differentiation (5, 43, 94). For instance, the Th1 hallmark transcription factor T-bet is indispensable for terminal effector differentiation and the generation of TEM and TRM cells through multiple mechanisms during Th1 responses, whereas it is clearly not involved in effector and memory differentiation under Th2 and Th17 conditions (81, 93, 120). Whether Gata3 and RORγt contributes to TEM and TRM generation during Th2 and Th17 responses remains unclear. It seems that the more we delve into the differences between subsets of CD4+ T cells, the harder it becomes to develop a general model of their memory generation.
Nevertheless, we cannot exclude the possibility that there exists a common mechanism for generation of multiple CD4+ TM populations under different conditions. TCR-dependent IL-2/CD25 signaling at early priming stage contributes to Th1/2/17 effector cell differentiation, whereas its absence favors the formation of Tfh and TCM cells during both Th1 and Th2 responses (57, 66, 95, 99, 121). IL-2/CD25 signaling during priming stage is also required for Th1-TRM and Th2-TRM generation through promoting effector differentiation and NLT residency (95, 96, 99). Although it has been argued that IL2/CD25 signaling promotes survival and persistence of CD4+ T cells at later stage (69, 70), it indeed contributes to determining the fate choice between Th1/2/17 effector cells and Tfh/TCM cells at the early stage during priming (Figure 4). Some other factors, including ACC1 and CD30, have also been shown to participate in regulating the transition of Th1/2/17 effector cells into lineages-committed Th1/2/17 memory cells under multiple conditions (64, 65). It is indicated that activated CD4+ T cells undergo some common processes that regulate their transition into memory cells, although decisive factors that dictate the fate decision between effector and memory under diverse conditions have not been revealed.
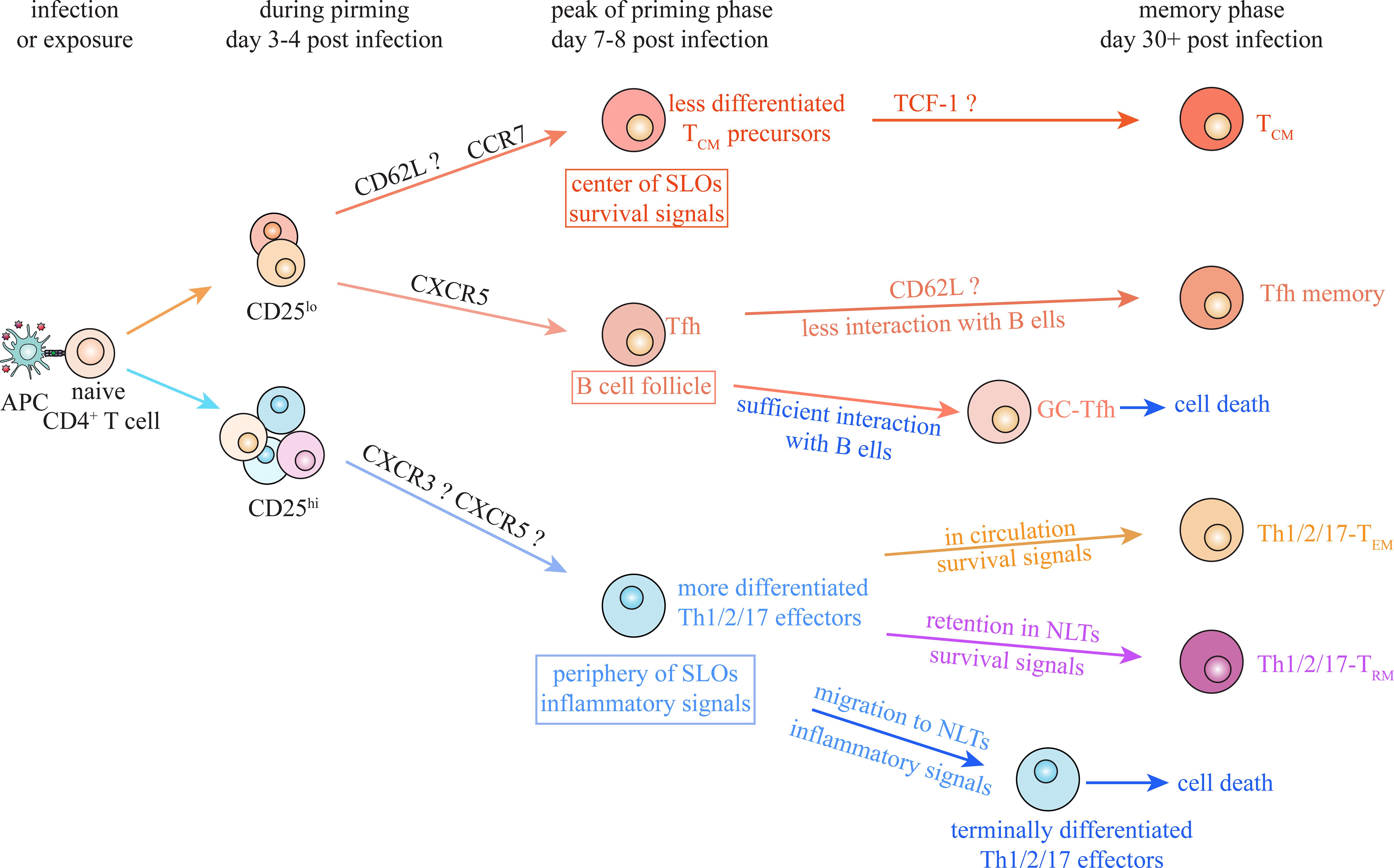
Figure 4. Putative common model of CD4+ TM cell generation. Despite the differences between CD4+ T cell subsets, there are some common mechanisms underlying memory generation during Th1, Th2 and Th17 responses. IL-2/CD25 signaling contributes to determining the fate choice between Th1/2/17 effector and Tfh/TCM at early stage of priming under multiple conditions. Moreover, migration of activated CD4+ T cells toward periphery of secondary lymphoid organs (SLOs) and inflamed non-lymphoid tissues (NLTs), facilitates their encounter with inflammatory signals and thus promotes their effector differentiation. Meanwhile, positioning of activated CD4+ T cells into appropriate niches, avoiding excessive stimulation and receiving survival signals, is suggested to be essential for generation of specific CD4+ TM subsets under various types of infections and exposures.
On the other hand, positioning of activated CD4+ T cells, which facilitates their interaction with specific extrinsic signals, plays a critical role in effector and memory differentiation of Th1, Th2, Th17 and Tfh cells (Figure 4). Localization in periphery and center of SLOs to interact with inflammatory and survival signals controls effector and TCM differentiation, respectively, during both Th1 and Th2 responses (20, 37, 41, 42, 52, 65, 84, 103). Retention of activated T cells in NLTs to interact with local inflammatory and survival signals is suggested to be indispensable for TRM generation during Th1, Th2 and Th17 responses, though factors regulating their residency in various NLTs remain unclear (93–96, 98, 99, 105, 111, 112, 122). Localization in B cell follicle (and GC area) and insufficient interaction with B cells both are suggested to be required for Tfh memory generation under viral infections and antigen exposures (84, 86). Consistent spatial requirements for differentiation of specific CD4+ TM subsets also provide valuable perspectives for identifying common memory precursors under various types of infections and exposures.
7 Concluding remarks
CD4+ TM cells play critical roles in protective immunity and immunopathology. Revealing underlying mechanisms of their generation and maintenance is crucial for developing therapeutic approaches targeting CD4+ TM cells in human diseases. The intriguing MPEC/SLEC model has led to remarkable advances in the understanding of generation of CD8+ TCM, TEM and TRM cells. However, there is a lack of guiding model for further investigations into generation of CD4+ TM cells, due to the diversity and plasticity of multiple CD4+ T subsets. Differentiation of specific CD4+ TM subsets under Th1, Th2 and Th17 conditions depends on both common and distinct underlying mechanisms. The shared features discussed above might provide a path to a putative common model on generation of CD4+TM subsets under all types of infections and exposures. Clearer delineations of Tfh memory, TCM, TEM and TRM cell populations at memory stage, as well as Tfh and Th1/2/17 populations at priming stage, are definitely required. Future works are also needed to reveal undergo programs governing memory generation under specific conditions and to clarify the consistency of these programs across different types of infections and exposures. Considering that transcriptional networks and positioning in proper niches to receive extrinsic stimulation both contributes to effector and memory differentiation, integrating spatial transcriptomics and single-cell RNA-seq technology will be an informative approach for further investigations (123). In addition, the advancing MHC-II multimer technology will be a valuable tool for studying endogenous antigen-specific CD4+ T cells (124). Identifying potential common and distinct precursors for each CD4+ TM cell subset would be highly beneficial for elucidating underlying mechanisms for their generation.
On the other hand, recent findings have suggested that CD8+ cytotoxic T (Tc) cells also differentiate into multiple subsets, in a manner similar to CD4+ T cells (125). Conventional IFNγ-producing Tc1 cells, IL-4-producing Tc2 cells, and IL-17-producing Tc17 cells, which are polarized by specific cytokine microenvironments, are all capable of differentiating into long-lived TM cells (125–127). However, whether each CD8+ TM cell subset derives from distinct or common precursor populations and whether they exhibit plasticity remains largely unclear. Elucidating the mechanisms underlying the generation of multiple CD4+ TM cell subsets may provide valuable insights into the differentiation of memory CD8+ Tc1, Tc2, and Tc17 cells.
Author contributions
JL: Writing – original draft. WS: Writing – review & editing. HT: Writing – review & editing.
Funding
The author(s) declare that financial support was received for the research and/or publication of this article. This work was supported by National Natural Science Foundation of China (82371740, 82371830, 82271803) and Scientific Research Cultivation Project in Emerging Strategic Fields (202403).
Conflict of interest
The authors declare that the research was conducted in the absence of any commercial or financial relationships that could be construed as a potential conflict of interest.
Generative AI statement
The author(s) declare that no Generative AI was used in the creation of this manuscript.
Publisher’s note
All claims expressed in this article are solely those of the authors and do not necessarily represent those of their affiliated organizations, or those of the publisher, the editors and the reviewers. Any product that may be evaluated in this article, or claim that may be made by its manufacturer, is not guaranteed or endorsed by the publisher.
References
1. Tsuda S, Pipkin ME. Transcriptional control of cell fate determination in antigen-experienced cd8 T cells. Cold Spring Harb Perspect Biol. (2022) 14:a037945. doi: 10.1101/cshperspect.a037945
2. Kretschmer L, Busch DH, Buchholz VR. A single-cell perspective on memory T-cell differentiation. Cold Spring Harb Perspect Biol. (2021) 13. doi: 10.1101/cshperspect.a038067
3. Gräbnitz F, Stark D, Shlesinger D, Petkidis A, Borsa M, Yermanos A, et al. Asymmetric cell division safeguards memory cd8 T-cell development. Cell Rep. (2023) 42. doi: 10.1016/j.celrep.2023.112468
4. Jameson SC, Masopust D. Understanding subset diversity in T cell memory. Immunity. (2018) 48:214–26. doi: 10.1016/j.immuni.2018.02.010
5. Künzli M, Masopust D. Cd4+ T cell memory. Nat Immunol. (2023) 24:903–14. doi: 10.1038/s41590-023-01510-4
6. Sun L, Su Y, Jiao A, Wang X, Zhang B. T cells in health and disease. Signal Transduction Targeted Ther. (2023) 8:235. doi: 10.1038/s41392-023-01471-y
7. Duckworth BC, Qin RZ, Groom JR. Spatial determinates of effector and memory cd8+ T cell fates. Immunol Rev. (2022) 306:76–92. doi: 10.1111/imr.13044
8. Kaech SM, Tan JT, Wherry EJ, Konieczny BT, Surh CD, Ahmed R. Selective expression of the interleukin 7 receptor identifies effector cd8 T cells that give rise to long-lived memory cells. Nat Immunol. (2003) 4:1191–8. doi: 10.1038/ni1009
9. Joshi NS, Cui W, Chandele A, Lee HK, Urso DR, Hagman J, et al. Inflammation directs memory precursor and short-lived effector cd8+ T cell fates via the graded expression of T-bet transcription factor. Immunity. (2007) 27:281–95. doi: 10.1016/j.immuni.2007.07.010
10. Lacombe MH, Hardy MP, Rooney J, Labrecque N. Il-7 receptor expression levels do not identify cd8+ Memory T lymphocyte precursors following peptide immunization. J Immunol. (2005) 175:4400–7. doi: 10.4049/jimmunol.175.7.4400
11. Hand TW, Morre M, Kaech SM. Expression of il-7 receptor alpha is necessary but not sufficient for the formation of memory cd8 T cells during viral infection. Proc Natl Acad Sci U S A. (2007) 104:11730–5. doi: 10.1073/pnas.0705007104
12. Pais Ferreira D, Silva JG, Wyss T, Fuertes Marraco SA, Scarpellino L, Charmoy M, et al. Central memory cd8+ T cells derive from stem-like tcf7hi effector cells in the absence of cytotoxic differentiation. Immunity. (2020) 53:985–1000.e11. doi: 10.1016/j.immuni.2020.09.005
13. Johnnidis JB, Muroyama Y, Ngiow SF, Chen Z, Manne S, Cai Z, et al. Inhibitory signaling sustains a distinct early memory cd8+ T cell precursor that is resistant to DNA damage. Sci Immunol. (2021) 6:eabe3702. doi: 10.1126/sciimmunol.abe3702
14. Silva JG, Pais Ferreira D, Dumez A, Wyss T, Veber R, Danilo M, et al. Emergence and fate of stem cell-like tcf7+ Cd8+ T cells during a primary immune response to viral infection. Sci Immunol. (2023) 8:eadh3113. doi: 10.1126/sciimmunol.adh3113
15. Bajénoff M, Glaichenhaus N, Germain RN. Fibroblastic Reticular Cells Guide T Lymphocyte Entry into and Migration within the Splenic T Cell Zone1. J Immunol. (2008) 181:3947–54. doi: 10.4049/jimmunol.181.6.3947
16. Sharma N, Benechet AP, Lefrançois L, Khanna KM. Cd8 T Cells Enter the Splenic T Cell Zones Independently of Ccr7, but the Subsequent Expansion and Trafficking Patterns of Effector T Cells after Infection Are Dysregulated in the Absence of Ccr7 Migratory Cues. J Immunol (Baltimore Md: 1950). (2015) 195:5227–36. doi: 10.4049/jimmunol.1500993
17. Jung YW, Rutishauser RL, Joshi NS, Haberman AM, Kaech SM. Differential localization of effector and memory cd8 T cell subsets in lymphoid organs during acute viral infection. J Immunol. (2010) 185:5315–25. doi: 10.4049/jimmunol.1001948
18. Jung YW, Kim HG, Perry CJ, Kaech SM. Ccr7 expression alters memory cd8 T-cell homeostasis by regulating occupancy in il-7– and il-15–dependent niches. Proc Natl Acad Sci. (2016) 113:8278–83. doi: 10.1073/pnas.1602899113
19. Duckworth BC, Lafouresse F, Wimmer VC, Broomfield BJ, Dalit L, Alexandre YO, et al. Effector and stem-like memory cell fates are imprinted in distinct lymph node niches directed by cxcr3 ligands. Nat Immunol. (2021) 22:434–48. doi: 10.1038/s41590-021-00878-5
20. Knop L, Deiser K, Bank U, Witte A, Mohr J, Philipsen L, et al. Il-7 Derived from Lymph Node Fibroblastic Reticular Cells Is Dispensable for Naive T cell Homeostasis but Crucial for Central Memory T cell Survival. Eur J Immunol. (2020) 50:846–57. doi: 10.1002/eji.201948368
21. Hickman HD, Reynoso GV, Ngudiankama BF, Cush SS, Gibbs J, Bennink JR, et al. Cxcr3 chemokine receptor enables local cd8(+) T cell migration for the destruction of virus-infected cells. Immunity. (2015) 42:524–37. doi: 10.1016/j.immuni.2015.02.009
22. Mackay LK, Minnich M, Kragten NAM, Liao Y, Nota B, Seillet C, et al. Hobit and blimp1 instruct a universal transcriptional program of tissue residency in lymphocytes. Science. (2016) 352:459–63. doi: 10.1126/science.aad2035
23. Parga-Vidal L, Taggenbrock RLRE, Beumer-Chuwonpad A, Aglmous H, Kragten NAM, Behr FM, et al. Hobit and blimp-1 regulate trm abundance after lcmv infection by suppressing tissue exit pathways of trmprecursors. Eur J Immunol. (2022) 52:1095–111. doi: 10.1002/eji.202149665
24. Parga-Vidal L, Behr FM, Kragten NAM, Nota B, Wesselink TH, Kavazović I, et al. Hobit identifies tissue-resident memory T cell precursors that are regulated by eomes. Sci Immunol. (2021) 6:eabg3533. doi: 10.1126/sciimmunol.abg3533
25. Behr FM, Kragten NAM, Wesselink TH, Nota B, van Lier RAW, Amsen D, et al. Blimp-1 rather than hobit drives the formation of tissue-resident memory cd8+ T cells in the lungs. Front Immunol. (2019) 10:400. doi: 10.3389/fimmu.2019.00400
26. Lin W-Hsuan W, Nish Simone A, Yen B, Chen Y-H, Adams William C, Kratchmarov R, et al. Cd8+ T lymphocyte self-renewal during effector cell determination. Cell Rep. (2016) 17:1773–82. doi: 10.1016/j.celrep.2016.10.032
27. Abadie K, Clark EC, Valanparambil RM, Ukogu O, Yang W, Daza RM, et al. Reversible, tunable epigenetic silencing of tcf1 generates flexibility in the T cell memory decision. Immunity. (2024) 57:271–86.e13. doi: 10.1016/j.immuni.2023.12.006
28. Solouki S, Huang W, Elmore J, Limper C, Huang F, August A. Tcr signal strength and antigen affinity regulate cd8+ Memory T cells. J Immunol. (2020) 205:1217–27. doi: 10.4049/jimmunol.1901167
29. Montacchiesi G, Pace L. Epigenetics and cd8+ T cell memory. Immunol Rev. (2022) 305:77–89. doi: 10.1111/imr.13057
30. Raynor JL, Chapman NM, Chi H. Metabolic control of memory T-cell generation and stemness. Cold Spring Harb Perspect Biol. (2021) 13:a037770. doi: 10.1101/cshperspect.a037770
31. Huang H, Long L, Zhou P, Chapman NM, Chi H. Mtor signaling at the crossroads of environmental signals and T-cell fate decisions. Immunol Rev. (2020) 295:15–38. doi: 10.1111/imr.12845
32. Osum KC, Jenkins MK. Toward a general model of cd4+ T cell subset specification and memory cell formation. Immunity. (2023) 56:475–84. doi: 10.1016/j.immuni.2023.02.010
33. Saravia J, Chapman NM, Chi H. Helper T cell differentiation. Cell Mol Immunol. (2019) 16:634–43. doi: 10.1038/s41423-019-0220-6
34. Olatunde AC, Hale JS, Lamb TJ. Cytokine-skewed tfh cells: functional consequences for B cell help. Trends Immunol. (2021) 42:536–50. doi: 10.1016/j.it.2021.04.006
35. Krueger PD, Osum KC, Jenkins MK. Cd4+ Memory T-cell formation during type 1 immune responses. Cold Spring Harb Perspect Biol. (2021) 13:a038141. doi: 10.1101/cshperspect.a038141
36. Shaw LA, Deng TZ, Omilusik KD, Takehara KK, Nguyen QP, Goldrath AW. Id3 expression identifies cd4+ Memory th1 cells. Proc Natl Acad Sci U S A. (2022) 119:e2204254119. doi: 10.1073/pnas.2204254119
37. Andreatta M, Tjitropranoto A, Sherman Z, Kelly MC, Ciucci T, Carmona SJ. A cd4+ T cell reference map delineates subtype-specific adaptation during acute and chronic viral infections. Elife. (2022) 11. doi: 10.7554/eLife.76339
38. Tubo NJ, Fife BT, Pagan AJ, Kotov DI, Goldberg MF, Jenkins MK. Most microbe-specific naive cd4+ T cells produce memory cells during infection. Science. (2016) 351:511–4. doi: 10.1126/science.aad0483
39. Soon MSF, Lee HJ, Engel JA, Straube J, Thomas BS, Pernold CPS, et al. Transcriptome dynamics of cd4+ T cells during malaria maps gradual transit from effector to memory. Nat Immunol. (2020) 21:1597–610. doi: 10.1038/s41590-020-0800-8
40. Nguyen QP, Deng TZ, Witherden DA, Goldrath AW. Origins of cd4+ Circulating and tissue-resident memory T-cells. Immunology. (2019) 157:3–12. doi: 10.1111/imm.13059
41. Ciucci T, Vacchio MS, Gao Y, Tomassoni Ardori F, Candia J, Mehta M, et al. The emergence and functional fitness of memory cd4+ T cells require the transcription factor thpok. Immunity. (2019) 50:91–105.e4. doi: 10.1016/j.immuni.2018.12.019
42. Pepper M, Pagan AJ, Igyarto BZ, Taylor JJ, Jenkins MK. Opposing signals from the bcl6 transcription factor and the interleukin-2 receptor generate T helper 1 central and effector memory cells. Immunity. (2011) 35:583–95. doi: 10.1016/j.immuni.2011.09.009
43. Beura LK, Fares-Frederickson NJ, Steinert EM, Scott MC, Thompson EA, Fraser KA, et al. Cd4(+) resident memory T cells dominate immunosurveillance and orchestrate local recall responses. J Exp Med. (2019) 216:1214–29. doi: 10.1084/jem.20181365
44. Hale JS, Youngblood B, Latner DR, Mohammed A-U-R, Ye L, Akondy RS, et al. Distinct memory cd4(+) T cells with commitment to T follicular helper- and T helper 1-cell lineages are generated after acute viral infection. Immunity. (2013) 38:805–17. doi: 10.1016/j.immuni.2013.02.020
45. Künzli M, Schreiner D, Pereboom TC, Swarnalekha N, Litzler LC, Lötscher J, et al. Long-lived T follicular helper cells retain plasticity and help sustain humoral immunity. Sci Immunol. (2020) 5:eaay5552. doi: 10.1126/sciimmunol.aay5552
46. Robinson AM, Higgins BW, Shuparski AG, Miller KB, McHeyzer-Williams LJ, McHeyzer-Williams MG. Evolution of antigen-specific follicular helper T cell transcription from effector function to memory. Sci Immunol. (2022) 7:eabm2084. doi: 10.1126/sciimmunol.abm2084
47. Choi YS, Yang JA, Yusuf I, Johnston RJ, Greenbaum J, Peters B, et al. Bcl6 expressing follicular helper cd4 T cells are fate committed early and have the capacity to form memory. J Immunol. (2013) 190:4014–26. doi: 10.4049/jimmunol.1202963
48. Marshall HD, Chandele A, Jung YW, Meng H, Poholek AC, Parish IA, et al. Differential expression of ly6c and T-bet distinguish effector and memory th1 cd4+ Cell properties during viral infection. Immunity. (2011) 35:633–46. doi: 10.1016/j.immuni.2011.08.016
49. Hu Z, Blackman MA, Kaye KM, Usherwood EJ. Functional heterogeneity in the cd4+ T cell response to murine Γ-herpesvirus 68. J Immunol (Baltimore Md: 1950). (2015) 194:2746–56. doi: 10.4049/jimmunol.1401928
50. Khatun A, Kasmani MY, Zander R, Schauder DM, Snook JP, Shen J, et al. Single-cell lineage mapping of a diverse virus-specific naive cd4 T cell repertoire. J Exp Med. (2021) 218. doi: 10.1084/jem.20200650
51. Song N, Sengupta S, Khoruzhenko S, Welsh RA, Kim A, Kumar MR, et al. Multiple genetic programs contribute to cd4 T cell memory differentiation and longevity by maintaining T cell quiescence. Cell Immunol. (2020) 357:104210. doi: 10.1016/j.cellimm.2020.104210
52. Nish SA, Zens KD, Kratchmarov R, Lin W-HW, Adams WC, Chen Y-H, et al. Cd4+ T cell effector commitment coupled to self-renewal by asymmetric cell divisions. J Exp Med. (2017) 214:39–47. doi: 10.1084/jem.20161046
53. Xia Y, Sandor K, Pai JA, Daniel B, Raju S, Wu R, et al. Bcl6-dependent tcf-1+ Progenitor cells maintain effector and helper cd4+ T cell responses to persistent antigen. Immunity. (2022) 55:1200–15.e6. doi: 10.1016/j.immuni.2022.05.003
54. Gullicksrud JA, Li F, Xing S, Zeng Z, Peng W, Badovinac VP, et al. Differential requirements for tcf1 long isoforms in cd8+ and cd4+ T cell responses to acute viral infection. J Immunol. (2017) 199:911–9. doi: 10.4049/jimmunol.1700595
55. Xu L, Cao Y, Xie Z, Huang Q, Bai Q, Yang X, et al. The transcription factor tcf-1 initiates the differentiation of tfh cells during acute viral infection. Nat Immunol. (2015) 16:991–9. doi: 10.1038/ni.3229
56. Wu T, Shin Hyun M, Moseman EA, Ji Y, Huang B, Harly C, et al. Tcf1 is required for the T follicular helper cell response to viral infection. Cell Rep. (2015) 12:2099–110. doi: 10.1016/j.celrep.2015.08.049
57. Charley KR, Ramstead AG, Matous JG, Kumaki Y, Sircy LM, Hale JS, et al. Effector-phase il-2 signals drive th1 effector and memory responses dependently and independently of tcf-1. J Immunol. (2023) 212:586–95. doi: 10.4049/jimmunol.2300570
58. Vacchio MS, Ciucci T, Gao Y, Watanabe M, Balmaceno-Criss M, McGinty MT, et al. A thpok-directed transcriptional circuitry promotes bcl6 and maf expression to orchestrate T follicular helper differentiation. Immunity. (2019) 51:465–78.e6. doi: 10.1016/j.immuni.2019.06.023
59. Ciucci T, Vacchio MS, Chen T, Nie J, Chopp LB, McGavern DB, et al. Dependence on bcl6 and blimp1 drive distinct differentiation of murine memory and follicular helper cd4+ T cells. J Exp Med. (2022) 219. doi: 10.1084/jem.20202343
60. Yu D, Rao S, Tsai LM, Lee SK, He Y, Sutcliffe EL, et al. The transcriptional repressor bcl-6 directs T follicular helper cell lineage commitment. Immunity. (2009) 31:457–68. doi: 10.1016/j.immuni.2009.07.002
61. Sun W, Hughes EP, Kim H, Perovanovic J, Charley KR, Perkins B, et al. Oca-B/pou2af1 is sufficient to promote cd4+ T cell memory and prospectively identifies memory precursors. Proc Natl Acad Sci. (2024) 121:e2309153121. doi: 10.1073/pnas.2309153121
62. Harrington LE, Janowski KM, Oliver JR, Zajac AJ, Weaver CT. Memory cd4 T cells emerge from effector T-cell progenitors. Nature. (2008) 452:356–60. doi: 10.1038/nature06672
63. Löhning M, Hegazy AN, Pinschewer DD, Busse D, Lang KS, Höfer T, et al. Long-lived virus-reactive memory T cells generated from purified cytokine-secreting T helper type 1 and type 2 effectors. J Exp Med. (2008) 205:53–61. doi: 10.1084/jem.20071855
64. Suzuki AS, Yagi R, Kimura MY, Iwamura C, Shinoda K, Onodera A, et al. Essential role for cd30-transglutaminase 2 axis in memory th1 and th17 cell generation. Front Immunol. (2020) 11:1536. doi: 10.3389/fimmu.2020.01536
65. Endo Y, Onodera A, Obata-Ninomiya K, Koyama-Nasu R, Asou HK, Ito T, et al. Acc1 determines memory potential of individual cd4+ T cells by regulating de novo fatty acid biosynthesis. Nat Metab. (2019) 1:261–75. doi: 10.1038/s42255-018-0025-4
66. Snook JP, Kim C, Williams MA. Tcr signal strength controls the differentiation of cd4+ Effector and memory T cells. Sci Immunol. (2018) 3:eaas9103. doi: 10.1126/sciimmunol.aas9103
67. Kalia V, Sarkar S. Regulation of effector and memory cd8 T cell differentiation by il-2—a balancing act. Front Immunol. (2018) 9:2987. doi: 10.3389/fimmu.2018.02987
68. DiToro D, Winstead CJ, Pham D, Witte S, Andargachew R, Singer JR, et al. Differential il-2 expression defines developmental fates of follicular versus nonfollicular helper T cells. Science. (2018) 361. doi: 10.1126/science.aao2933
69. Dooms H, Wolslegel K, Lin P, Abbas AK. Interleukin-2 enhances cd4+ T cell memory by promoting the generation of il-7r alpha-expressing cells. J Exp Med. (2007) 204:547–57. doi: 10.1084/jem.20062381
70. McKinstry KK, Strutt TM, Bautista B, Zhang W, Kuang Y, Cooper AM, et al. Effector cd4 T-cell transition to memory requires late cognate interactions that induce autocrine il-2. Nat Commun. (2014) 5:5377. doi: 10.1038/ncomms6377
71. Bautista BL, Devarajan P, McKinstry KK, Strutt TM, Vong AM, Jones MC, et al. Short-Lived Antigen Recognition but Not Viral Infection at a Defined Checkpoint Programs Effector Cd4 T Cells to Become Protective Memory. J Immunol. (2016) 197:3936–49. doi: 10.4049/jimmunol.1600838
72. Stobie L, Gurunathan S, Prussin C, Sacks DL, Glaichenhaus N, Wu C-Y, et al. The role of antigen and il-12 in sustaining th1 memory cells in vivo: il-12 is required to maintain memory/effector th1 cells sufficient to mediate protection to an infectious parasite challenge. Proc Natl Acad Sci. (2000) 97:8427–32. doi: 10.1073/pnas.160197797
73. Athie-Morales V, Smits HH, Cantrell DA, Hilkens CMU. Sustained il-12 signaling is required for th1 development 1. J Immunol. (2004) 172:61–9. doi: 10.4049/jimmunol.172.1.61
74. Martínez-López M, Iborra S, Conde-Garrosa R, Sancho D. Batf3-dependent cd103(+) dendritic cells are major producers of il-12 that drive local th1 immunity against leishmania major infection in mice. Eur J Immunol. (2015) 45:119–29. doi: 10.1002/eji.201444651
75. Afkarian M, Sedy JR, Yang J, Jacobson NG, Cereb N, Yang SY, et al. T-bet is a stat1-induced regulator of il-12r expression in naïve cd4+ T cells. Nat Immunol. (2002) 3:549–57. doi: 10.1038/ni794
76. Bradley LM, Dalton DK, Croft M. A direct role for ifn-gamma in regulation of th1 cell development. J Immunol. (1996) 157:1350–8. doi: 10.4049/jimmunol.157.4.1350
77. Dhume K, Finn CM, Strutt TM, Sell S, McKinstry KK. T-Bet Optimizes Cd4 T-Cell Responses against Influenza through Cxcr3-Dependent Lung Trafficking but Not Functional Programming. Mucosal Immunol. (2019) 12:1220–30. doi: 10.1038/s41385-019-0183-z
78. Prizant H, Patil N, Negatu S, Bala N, McGurk A, Leddon SA, et al. Cxcl10+ Peripheral activation niches couple preferred sites of th1 entry with optimal apc encounter. Cell Rep. (2021) 36:109523. doi: 10.1016/j.celrep.2021.109523
79. Leal JM, Huang JY, Kohli K, Stoltzfus C, Lyons-Cohen MR, Olin BE, et al. Innate cell microenvironments in lymph nodes shape the generation of T cell responses during type I inflammation. Sci Immunol. (2021) 6. doi: 10.1126/sciimmunol.abb9435
80. Groom JR. Regulators of T-cell fate: integration of cell migration, differentiation and function. Immunol Rev. (2019) 289:101–14. doi: 10.1111/imr.12742
81. Groom JR, Richmond J, Murooka TT, Sorensen EW, Sung JH, Bankert K, et al. Cxcr3 chemokine receptor-ligand interactions in the lymph node optimize cd4+ T helper 1 cell differentiation. Immunity. (2012) 37:1091–103. doi: 10.1016/j.immuni.2012.08.016
82. Woodruff MC, Heesters BA, Herndon CN, Groom JR, Thomas PG, Luster AD, et al. Trans-nodal migration of resident dendritic cells into medullary interfollicular regions initiates immunity to influenza vaccine. J Exp Med. (2014) 211:1611–21. doi: 10.1084/jem.20132327
83. Li J, Huston G, Swain SL. Il-7 promotes the transition of cd4 effectors to persistent memory cells. J Exp Med. (2003) 198:1807–15. doi: 10.1084/jem.20030725
84. Zhu F, McMonigle RJ, Schroeder AR, Xia X, Figge D, Greer BD, et al. Spatiotemporal resolution of germinal center tfh cell differentiation and divergence from central memory cd4+ T cell fate. Nat Commun. (2023) 14:3611. doi: 10.1038/s41467-023-39299-3
85. Horiuchi S, Wu H, Liu W-C, Schmitt N, Provot J, Liu Y, et al. Tox2 is required for the maintenance of gc tfh cells and the generation of memory tfh cells. Sci Adv. (2021) 7:eabj1249. doi: 10.1126/sciadv.abj1249
86. Feng H, Zhao Z, Zhao X, Bai X, Fu W, Zheng L, et al. A novel memory-like tfh cell subset is precursor to effector tfh cells in recall immune responses. J Exp Med. (2024) 221. doi: 10.1084/jem.20221927
87. Leon B, Ballesteros-Tato A, Browning JL, Dunn R, Randall TD, Lund FE. Regulation of T(H)2 development by cxcr5+ Dendritic cells and lymphotoxin-expressing B cells. Nat Immunol. (2012) 13:681–90. doi: 10.1038/ni.2309
88. Kerfoot SM, Yaari G, Patel JR, Johnson KL, Gonzalez DG, Kleinstein SH, et al. Germinal center B cell and T follicular helper cell development initiates in the interfollicular zone. Immunity. (2011) 34:947–60. doi: 10.1016/j.immuni.2011.03.024
89. Hardtke S, Ohl L, Förster R. Balanced expression of cxcr5 and ccr7 on follicular T helper cells determines their transient positioning to lymph node follicles and is essential for efficient B-cell help. Blood. (2005) 106:1924–31. doi: 10.1182/blood-2004-11-4494
90. Haynes NM, Allen CDC, Lesley R, Ansel KM, Killeen N, Cyster JG. Role of cxcr5 and ccr7 in follicular th cell positioning and appearance of a programmed cell death gene-1high germinal center-associated subpopulation1. J Immunol. (2007) 179:5099–108. doi: 10.4049/jimmunol.179.8.5099
91. Groom JR. Moving to the suburbs: T-cell positioning within lymph nodes during activation and memory. Immunol Cell Biol. (2015) 93:330–6. doi: 10.1038/icb.2015.29
92. Shi J, Hou S, Fang Q, Liu X, Liu X, Qi H. Pd-1 controls follicular T helper cell positioning and function. Immunity. (2018) 49:264–74.e4. doi: 10.1016/j.immuni.2018.06.012
93. Depew CE, Nguyen AT, Franke MC, Calderon J, Sciammas R, McSorley SJ. Cutting edge: optimal formation of hepatic tissue-resident memory cd4 T cells requires T-bet regulation of cd18. J Immunol. (2023) 211:180–5. doi: 10.4049/jimmunol.2300017
94. Nguyen QP, Takehara KK, Deng TZ, O’Shea S, Heeg M, Omilusik KD, et al. Transcriptional programming of cd4+Trm differentiation in viral infection balances effector- and memory-associated gene expression. Sci Immunol. (2023) 8:eabq7486. doi: 10.1126/sciimmunol.abq7486
95. Hondowicz BD, Kim KS, Ruterbusch MJ, Keitany GJ, Pepper M. Il-2 is required for the generation of viral-specific cd4(+) th1 tissue-resident memory cells and B cells are essential for maintenance in the lung. Eur J Immunol. (2018) 48:80–6. doi: 10.1002/eji.201746928
96. Strutt TM, Dhume K, Finn CM, Hwang JH, Castonguay C, Swain SL, et al. Il-15 supports the generation of protective lung-resident memory cd4 T cells. Mucosal Immunol. (2018) 11:668–80. doi: 10.1038/mi.2017.101
97. Zundler S, Becker E, Spocinska M, Slawik M, Parga-Vidal L, Stark R, et al. Hobit- and blimp-1-driven cd4+ Tissue-resident memory T cells control chronic intestinal inflammation. Nat Immunol. (2019) 20:288–300. doi: 10.1038/s41590-018-0298-5
98. Depew CE, Rixon JA, McSorley SJ. Optimal generation of hepatic tissue-resident memory cd4 T cells requires il-1 and il-2. Proc Natl Acad Sci. (2023) 120:e2214699120. doi: 10.1073/pnas.2214699120
99. Hondowicz BD, An D, Schenkel JM, Kim KS, Steach HR, Krishnamurty AT, et al. Interleukin-2-dependent allergen-specific tissue-resident memory cells drive asthma. Immunity. (2016) 44:155–66. doi: 10.1016/j.immuni.2015.11.004
100. Rahimi RA, Nepal K, Cetinbas M, Sadreyev RI, Luster AD. Distinct functions of tissue-resident and circulating memory th2 cells in allergic airway disease. J Exp Med. (2020) 217:e20190865. doi: 10.1084/jem.20190865
101. Ballesteros-Tato A, Randall TD, Lund FE, Spolski R, Leonard WJ, León B. T follicular helper cell plasticity shapes pathogenic T helper 2 cell-mediated immunity to inhaled house dust mite. Immunity. (2016) 44:259–73. doi: 10.1016/j.immuni.2015.11.017
102. Glatman Zaretsky A, Taylor JJ, King IL, Marshall FA, Mohrs M, Pearce EJ. T follicular helper cells differentiate from th2 cells in response to helminth antigens. J Exp Med. (2009) 206:991–9. doi: 10.1084/jem.20090303
103. Polonsky M, Rimer J, Kern-Perets A, Zaretsky I, Miller S, Bornstein C, et al. Induction of cd4 T cell memory by local cellular collectivity. Science. (2018) 360:eaaj1853. doi: 10.1126/science.aaj1853
104. Liu J, Du L, Yi S, Li L, Lin Y, Bi Y, et al. Murine lung cdc1s inhibit memory th2 cell generation by reducing cd62l+ Memory precursors via il-12. Allergy. (2022) 77:3467–70. doi: 10.1111/all.15470
105. Yeon S-M, Halim L, Chandele A, Perry CJ, Kim SH, Kim S-U, et al. Il-7 plays a critical role for the homeostasis of allergen-specific memory cd4 T cells in the lung and airways. Sci Rep. (2017) 7:11155–. doi: 10.1038/s41598-017-11492-7
106. Moschovakis GL, Bubke A, Dittrich-Breiholz O, Braun A, Prinz I, Kremmer E, et al. Deficient ccr7 signaling promotes th2 polarization and B-cell activation in vivo. Eur J Immunol. (2012) 42:48–57. doi: 10.1002/eji.201141753
107. Fan N-W, Wang S, Ortiz G, Chauhan SK, Chen Y, Dana R. Autoreactive memory th17 cells are principally derived from T-bet+Rorγt+ Th17/1 effectors. J Autoimmun. (2022) 129:102816. doi: 10.1016/j.jaut.2022.102816
108. Van VQ, Raymond M, Baba N, Rubio M, Wakahara K, Susin SA, et al. Cd47high expression on cd4 effectors identifies functional long-lived memory T cell progenitors. J Immunol. (2012) 188:4249–55. doi: 10.4049/jimmunol.1102702
109. Chen Y, Chauhan SK, Tan X, Dana R. Interleukin-7 and -15 maintain pathogenic memory th17 cells in autoimmunity. J Autoimmun. (2017) 77:96–103. doi: 10.1016/j.jaut.2016.11.003
110. Chen Y, Shao C, Fan N-W, Nakao T, Amouzegar A, Chauhan SK, et al. The functions of il-23 and il-2 on driving autoimmune effector T-helper 17 cells into the memory pool in dry eye disease. Mucosal Immunol. (2021) 14:177–86. doi: 10.1038/s41385-020-0289-3
111. Hoffmann JP, Srivastava A, Yang H, Iwanaga N, Remcho TP, Hewes JL, et al. Vaccine-elicited il-1r signaling results in th17 trm-mediated immunity. Commun Biol. (2024) 7:433. doi: 10.1038/s42003-024-06138-0
112. Whitley SK, Li M, Kashem SW, Hirai T, Igyártó BZ, Knizner K, et al. Local il-23 is required for proliferation and retention of skin-resident memory th17 cells. Sci Immunol. (2022) 7:eabq3254. doi: 10.1126/sciimmunol.abq3254
113. Homann D, Teyton L, Oldstone MBA. Differential regulation of antiviral T-cell immunity results in stable cd8+ but declining cd4+ T-cell memory. Nat Med. (2001) 7:913–9. doi: 10.1038/90950
114. Pepper M, Linehan JL, Pagán AJ, Zell T, Dileepan T, Cleary PP, et al. Different routes of bacterial infection induce long-lived th1 memory cells and short-lived th17 cells. Nat Immunol. (2010) 11:83–9. doi: 10.1038/ni.1826
115. Geng J, Wei H, Shi B, Wang Y-H, Greer BD, Pittman M, et al. Bach2 negatively regulates T follicular helper cell differentiation and is critical for cd4+ T cell memory. J Immunol. (2019) 202:2991–8. doi: 10.4049/jimmunol.1801626
116. Crotty S. Do memory cd4 T cells keep their cell-type programming: plasticity versus fate commitment?: complexities of interpretation due to the heterogeneity of memory cd4 T cells, including T follicular helper cells. Cold Spring Harb Perspect Biol. (2018) 10. doi: 10.1101/cshperspect.a032102
117. Gao X, Luo K, Wang D, Wei Y, Yao Y, Deng J, et al. T follicular helper 17 (Tfh17) cells are superior for immunological memory maintenance. Elife. (2023) 12. doi: 10.7554/eLife.82217
118. Barata JT, Durum SK, Seddon B. Flip the coin: il-7 and il-7r in health and disease. Nat Immunol. (2019) 20:1584–93. doi: 10.1038/s41590-019-0479-x
119. Shanmuganad S, Ferguson A, Paranjpe A, Cianciolo EE, Katz JD, Herold MJ, et al. Subset-specific and temporal control of effector and memory cd4+ T cell survival. bioRxiv. (2023). doi: 10.1101/2023.03.01.530323
120. Mackay Laura K, Wynne-Jones E, Freestone D, Pellicci Daniel G, Mielke Lisa A, Newman Dane M, et al. T-box transcription factors combine with the cytokines tgf-B and il-15 to control tissue-resident memory T cell fate. Immunity. (2015) 43:1101–11. doi: 10.1016/j.immuni.2015.11.008
121. Ballesteros-Tato A, León B, Graf Beth A, Moquin A, Adams Pamela S, Lund Frances E, et al. Interleukin-2 inhibits germinal center formation by limiting T follicular helper cell differentiation. Immunity. (2012) 36:847–56. doi: 10.1016/j.immuni.2012.02.012
122. Tieu R, Zeng Q, Zhao D, Zhang G, Feizi N, Manandhar P, et al. Tissue-resident memory T cell maintenance during antigen persistence requires both cognate antigen and interleukin-15. Sci Immunol. (2023) 8:eadd8454. doi: 10.1126/sciimmunol.add8454
123. Gulati GS, D’Silva JP, Liu Y, Wang L, Newman AM. Profiling cell identity and tissue architecture with single-cell and spatial transcriptomics. Nat Rev Mol Cell Biol. (2024) 26:11–31. doi: 10.1038/s41580-024-00768-2
124. Chang J. Mhc multimer: A molecular toolbox for immunologists. Mol Cells. (2021) 44:328–34. doi: 10.14348/molcells.2021.0052
125. St. Paul M, Ohashi PS. The roles of cd8+ T cell subsets in antitumor immunity. Trends Cell Biol. (2020) 30:695–704. doi: 10.1016/j.tcb.2020.06.003
126. Hinks TSC, Hoyle RD, Gelfand EW. Cd8+ Tc2 cells: underappreciated contributors to severe asthma. Eur Respir Rev. (2019) 28:190092. doi: 10.1183/16000617.0092-2019
Keywords: CD4 + T memory cells, memory precursor, fate decision, T memory subsets, Th1/2/17 and Tfh subsets
Citation: Liu J, Song W and Tang H (2025) Advances and challenges in identifying precursors of memory CD4+ T cells. Front. Immunol. 16:1540045. doi: 10.3389/fimmu.2025.1540045
Received: 05 December 2024; Accepted: 14 April 2025;
Published: 05 May 2025.
Edited by:
Koji Tokoyoda, Tottori University, JapanReviewed by:
Benedict Seddon, University College London, United KingdomMakoto Kurachi, Kanazawa University, Japan
Copyright © 2025 Liu, Song and Tang. This is an open-access article distributed under the terms of the Creative Commons Attribution License (CC BY). The use, distribution or reproduction in other forums is permitted, provided the original author(s) and the copyright owner(s) are credited and that the original publication in this journal is cited, in accordance with accepted academic practice. No use, distribution or reproduction is permitted which does not comply with these terms.
*Correspondence: Hua Tang, dGFuZ2h1YXpoYW5nMjE4QDE2My5jb20=