- 1Department of Urology, The First Affiliated Hospital of Anhui Medical University, Anhui Medical University, Hefei, Anhui, China
- 2Institute of Urology, Anhui Medical University, Hefei, Anhui, China
- 3Anhui Province Key Laboratory of Urological and Andrological Diseases Research and Medical Transformation, Anhui Medical University, Hefei, Anhui, China
Exposure of renal tubular epithelial cells (RTCs) to kidney stones or calcium oxide crystals triggers the production of reactive oxygen species (ROS), leading to oxidative stress. This oxidative milieu incites cellular injury and elicits an inflammatory cascade within the RTCs. Notably, the cellular membranes of the compromised cells facilitate the adherence and subsequent retention of crystals, which is instrumental in the pathogenesis of kidney stones. The pathways of ROS production are diverse, involving numerous signaling cascades. Recent researchers’ endeavors have elucidated that selective antioxidants can attenuate intracellular ROS concentrations by modulating these intricate signaling cascades. This reduction in ROS levels has been empirically demonstrated to significantly curtail the accumulation of calcium oxalate crystals within renal tissues in animal models, heralding a novel therapeutic paradigm for the amelioration of nephrolithiasis. In this review, we endeavor to elucidate the contributory role of ROS in kidney stone and explore the protective mechanisms by which certain antioxidants safeguard renal function.
1 Introduction
Urolithiasis, a prevalent disorder within the urinary system, emerges as the third most common urological affliction, trailing only behind urinary tract infections and prostate-related disorders. This pathological state of biomineralization, primarily manifesting within the renal system, is characterized by the crystallization and solidification of urinary constituents. The primary manifestation of this condition, kidney stones, impinges upon approximately 10-15% of the global populace (1). Existing literature delineates a differential propensity for nephrolithiasis influenced by factors such as gender, ethnicity, and geographic localization. Contemporary studies underscore a notable ascendancy in prevalence amongst females, a trend potentially ascribed to shifts in dietary and lifestyle paradigms (2). Specifically, in Eastern nations such as China and Japan, the embracement of Western dietary practices, marked by elevated protein consumption, correlates with an amplified incidence of nephrolithiasis (3). Calcium oxalate (CaOx) stones, constituting the quintessential component of urinary calculi, account for upwards of 80% of all diagnosed cases (4). It is generally believed that the formation process mainly includes urine supersaturation, heterogeneous nucleation, crystal growth and aggregation, and crystal adhesion and deposition in renal tubular cell, though the precise mechanisms remain enigmatic. Despite the advent of minimally invasive surgical interventions, the recurrence of urinary stones persists at a formidable rate, with a 50% recurrence within a five-year span and a potential surge to 75% over a decade, thereby levying substantial burdens upon individuals and healthcare infrastructures alike (5). This backdrop accentuates the imperative for the delineation and deployment of efficacious therapeutic strategies for nephrolithiasis, with an emphasis on minimizing adverse effects.
Recent research has elucidated a significant correlation between the injury of RTCs, the insurgence of calcium oxalate (CaOx) crystals presence, and the concomitant inflammatory responses and stone formation, thereby illuminating their contributory role in the pathophysiology of nephrolithiasis. A pivotal factor in this process is the induction of oxidative stress (OS) through ROS, which significantly contributes to stone formation. The injury of RTCs membrane integrity engenders a conducive environment for calcium crystal adhesion, thereby precipitating further stone development. Oxidative stress typically results from an imbalance between ROS production and antioxidant defenses. Under physiological conditions, the levels of ROS production are low and can be cleared by the endogenous antioxidant system. In contrast, an overproduction of ROS, outstripping the scavenging capacity of antioxidants, leads to oxidative stress, contributing to stone development (6–8).
The origins of ROS within the human body are multifarious, entailing the involvement of an array of signaling pathways. Recent investigations suggest that modulating some of these pathways, such as the MEK-ERK pathway and redox-sensitive pathways implicated in viral infections, can reduce ROS levels in vivo, correlating with diminished stone formation in animal models (9). This review aims to dissect the role of ROS in the etiology of nephrolithiasis and assess the potential of antioxidants as therapeutic agents in the management of calcium oxalate kidney stones, underpinning the prospective therapeutic ramifications of oxidative stress mitigation in the prevention of stone recurrence.
2 ROS
2.1 Definition and production pathway of reactive oxygen species
ROS, including free radicals, atoms, or molecules with unpaired electrons and their metabolites, comprise oxygen radicals like superoxide anion radicals (O2•−) and hydroxyl radicals (•OH), as well as non-radical oxidants such as hydrogen peroxide (H2O2) and singlet oxygen (¹O2). ROS’s reactive nature facilitates its role in various regulatory processes and signal transduction pathways. These include regulating proliferation, activating or deactivating biomolecules, and controlling transcriptional activity. However, excessive ROS may cause harmful effects through oxidative modifications of cellular components, particularly damaging proteins, lipids, carbohydrates, and nucleotides (10).
The primary cellular sources of ROS include mitochondria and NADPH oxidase (NOX) (7). The electron transport chain (ETC), situated on the inner mitochondrial membrane (IMM), plays a crucial role within mitochondria. NADH and FADH2 introduce electrons into the ETC through complex I (CI) and complex II (CII). Electrons then move from complex I (CI) and complex II (CII) to complex III (CIII). Normally, electrons pass from CIII to complex IV (CIV) and combine with oxygen to form water. However, under specific conditions such as reduced oxygen levels, diminished mitochondrial membrane potential(MMP), or impaired mitochondrial function, electron transfer can become abnormal. Abnormal electron transport may result in the return of electrons from CIII to CI, which is known as reverse electron transfer (RET). Reverse electron transfer results in a cyclic flow of electrons moving back and forth between CI and CIII. During this cycle, interaction of the electrons with oxygen generates superoxide anion (O2•−). Subsequently, superoxide can undergo conversion into other forms of ROS, like hydrogen peroxide (H2O2) (11) (Figure 1).
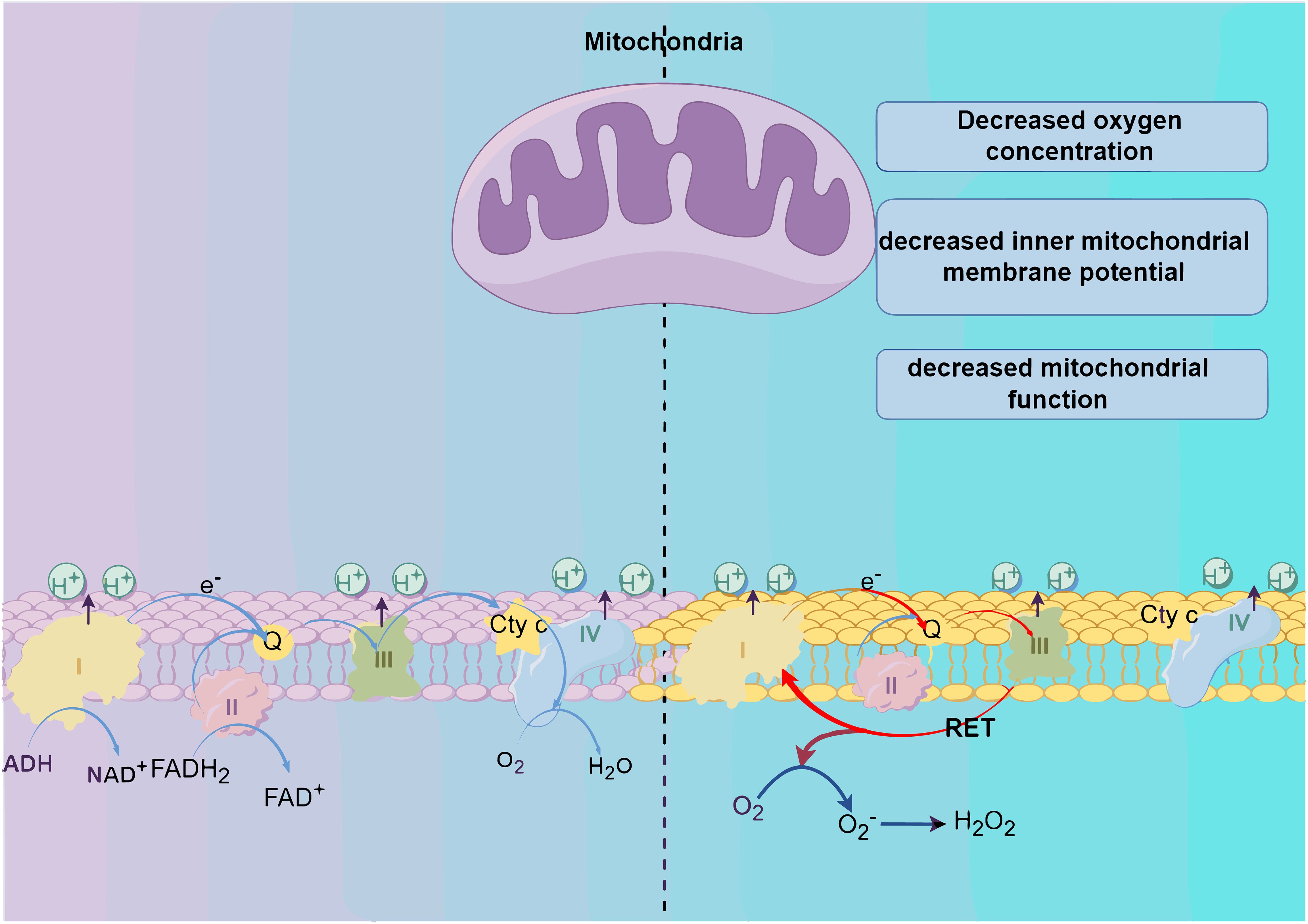
Figure 1. Production of reactive oxygen species (ROS). The generation of reactive oxygen species (ROS) primarily originates from the oxidative respiratory chains within mitochondria. Alterations in the internal environment, such as a decrease in the level of oxygen, mitochondrial membrane potential, or mitochondrial function, trigger reverse electron transfer (RET), facilitating electron circulation between C I and C II. Ultimately, this process culminates in the reaction of electrons with oxygen and subsequent ROS production.
In the cytoplasm, NADPH oxidases (NOXs) are crucial in maintaining cellular redox balance (12). The process begins with glucose metabolism through glycolysis, yielding pyruvate as the end product. This pyruvate is then transported to the mitochondria to enter the tricarboxylic acid (TCA) cycle. This process generates reducing equivalents, specifically NADH and FADH2, which subsequently enter the mitochondrial electron transport chain for oxidative phosphorylation. NOXs operate by facilitating the electron transfer from NADPH to oxygen, resulting in the generation of superoxide. This regulation of NOXs activity is controlled by transforming growth factor β (TGF-β) (13). Notably, in vivo exposure of renal tubular cells to oxalic acid triggers reactive oxygen species (ROS) production via the TGF-β1-NADPH-ROS pathway (14). Moreover, ROS generated by NOX can prompt mitochondrial ROS production, serving as a crucial pathway for ROS amplification or propagation (15). Given the high energy demands of the kidney, with its mitochondrial content and oxygen consumption being second only to the heart, RTCs are particularly vulnerable to damage from ROS imbalance, eventually leading to crystal accumulation and aggregation.
2.2 Physiological and pathological effects of reactive oxygen species
The role of ROS as crucial signaling molecules in cell proliferation and survival has been well established (16). At physiological levels, ROS facilitate cell proliferation and differentiation, making the maintenance of basal ROS levels essential for normal cell growth and development (17). Moreover, ROS act as signaling molecules that activate transcription factors and upregulate the expression of protective genes such as antioxidant enzymes, thus bolstering cellular resistance to oxidative stress (18). Additionally, ROS also interact with antioxidant molecules such as glutathione and peroxidase, playing a vital role in regulating intracellular redox homeostasis and thereby influencing cellular function. However, deviations from optimal ROS levels, either excessively high or low, can lead to detrimental effects.
Organisms have developed numerous antioxidant defense systems to mitigate damage from ROS. These defenses include non-enzymatic systems, such as vitamins C and E, carotenoids, reduced glutathione, and polyphenols, and enzymatic systems like superoxide dismutase (SOD), catalase (CAT), and glutathione peroxidase (GPX). Together, these systems synergistically safeguard cells against oxidative damage (19).
3 The role of ROS in the formation of calcium oxalate renal stones
It is now well-recognized that the injury to RTCs and subsequent formation of stones attributed to oxalate is due to the interaction of oxalate with the kidney generating ROS (20). Oxidative stress (OS) is defined as a state of excessive oxidation within an organism, primarily caused by either an overproduction or inadequate clearance of ROS in response to harmful stimuli in both the internal and external surroundings. This results in the accumulation of ROS within the body or cells, leading to oxidative damage and disruption of the body’s oxidative/antioxidant balance system. It manifests as harm to cellular membranes, proteins, DNA, and lipids (21).
Studies conducted in vitro on cells, in vivo on animals, and clinical case studies have all shown that RTCs exposed to oxalate and/or calcium oxalate crystals generate excessive ROS, triggering oxidative stress and inflammatory reactions. Prolonged oxidative stress exacerbates damage to these cells. As oxidative stress continues to exacerbate damage to RTCs, the ability for phosphatidylserine (PS), situated on the cell’s inner side, to move outward increases and its ability to move inward decreases, resulting in PS flipping to the cell surface. Phosphatidylserine (PS), as a phospholipid of the renal tubular epithelial cell membrane that can adsorb crystals, flips to the cell surface, enhancing the adhesiveness of stone crystals at the flipped sites (22).
Joshi et al. discovered that a diet high in oxalate causes increased OS in the kidneys. They noted that under conditions of elevated ROS levels, intracellular TXNIP (thioredoxin-interacting protein) detaches from the antioxidant enzyme Trx1 (thioredoxin 1). This detachment leads to the activation of NLRP3, mediated by TXNIP (23). Activating the NLRP3 inflammasome releases inflammatory factors such as IL-1β and IL-18, triggering an inflammatory response. This response causes damage and necrosis in RTECs, setting the stage for CaOx stone formation (24). And increased ROS production can lower MMP, potentially causing mitochondrial and lysosomal dysfunction. This dysfunction can impair macrophages’ phagocytosis, reducing their capacity to eliminate calcium oxalate crystals during stone formation. Consequently, this can further encourage stone formation (25) (Figure 2A).
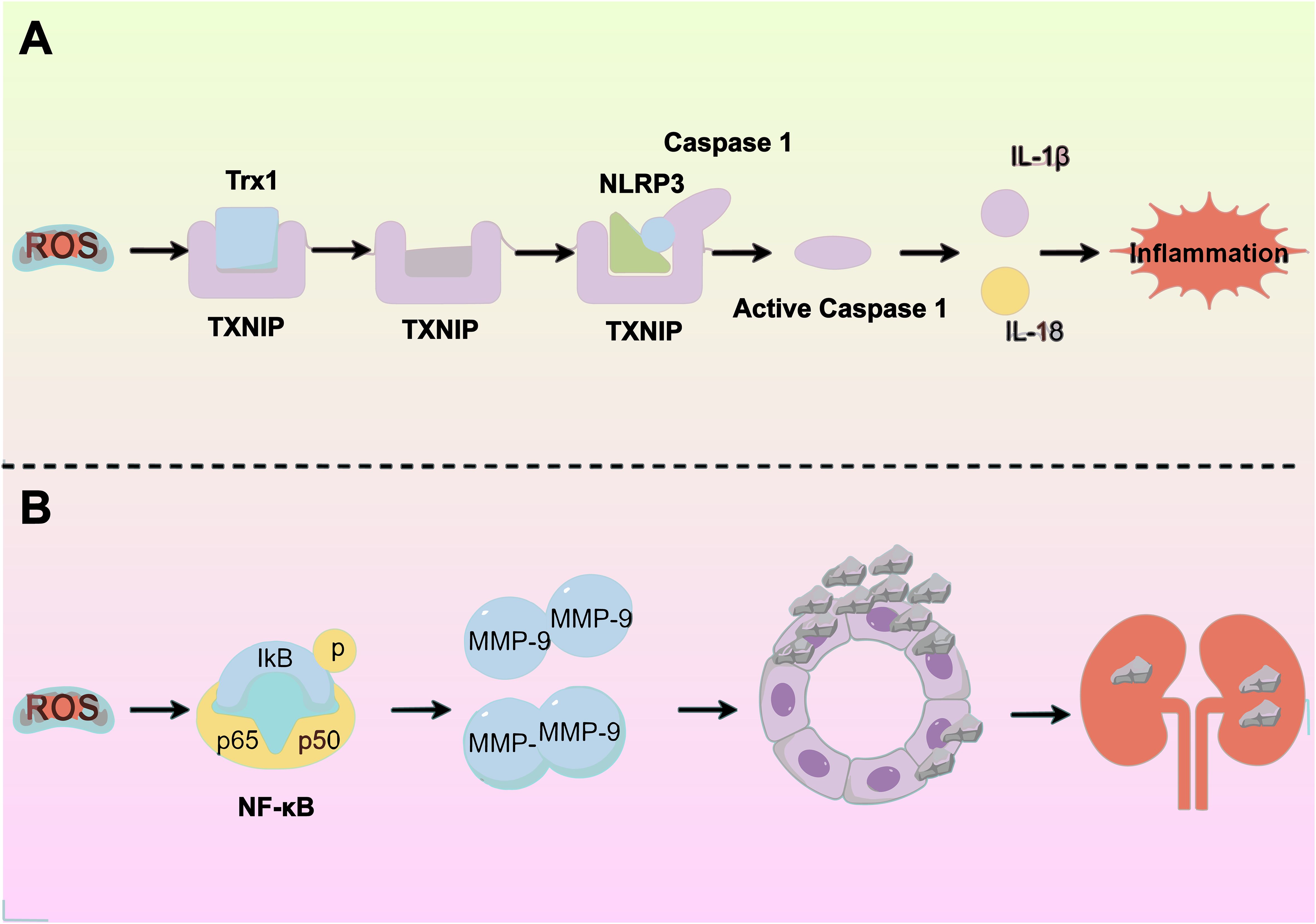
Figure 2. Exposure to oxalate and/or calcium oxalate crystals triggers renal tubular epithelial cells to produce excess reactive oxygen species (ROS), leading to oxidative stress (OS) and inflammatory responses. This process is driven by several molecular mechanisms: (A) Activation of the NLRP3 inflammasome releases inflammatory factors, worsening tubular epithelial damage. (B) Elevated ROS levels upregulate MMP-9 expression, increasing crystal adherence.
Additionally, Wiger et al. discovered that high ROS levels increase AP-1 (activator protein-1) and NF-κB (nuclear factor κB) levels, subsequently increasing the expression of matrix metalloproteinases (MMPs). MMPs, crucial components of the extracellular matrix (ECM), serve as effective crystal-binding molecules that play a significant role in the adhesion, internalization, and eventual formation of renal stones. Notably, MMP-9 facilitates dendritic cell migration, triggers the cleavage of the E-calmodulin receptor CD103 on dendritic cells, and encourages epithelial-mesenchymal transition, leading to sediment adhesion and aggregation. Additionally, MMP-9’s activity in degrading collagen and osteoblast cleavage products enhances inflammatory cell recruitment, a process linked to kidney stone development (3) (Figure 2B).
Furthermore, Under physiological conditions, urinary proteins including osteopontin and uromodulin (Tamm-Horsfall protein) inhibit kidney stone formation and support renal function (26, 27). However, elevated reactive oxygen species (ROS) under oxidative stress induce oxidative modifications of these protective proteins. Such redox alterations enhance calcium oxalate crystallization processes by promoting crystal nucleation, growth, and aggregation, thereby increasing lithogenic risk (28).
Tight junctions serve as crucial barriers in renal tubular epithelial cells, preventing the entry of water, ions, macromolecules, and pathogens into the renal tubules through the spaces between cells. Impairment of these tight junctions can obstruct intercellular channels, leading to damage of tubular epithelial cells and inciting inflammatory responses. This damage facilitates the accumulation of kidney stones within the tubules. Additionally, excessive production of ROS can degrade and redistribute tight junction proteins, such as ZO-1 and occludin, via the Akt/ASK1/p38 MAPK signaling pathway. This disruption of tight junction integrity contributes to stone formation (29).
Furthermore, the membranes of RTCs predominantly consist of phospholipids with unsaturated fatty acids. ROS have a high affinity for the unsaturated bonds in these fatty acids, leading to lipid peroxidation. This oxidative damage impairs the RTCs and enhance the adhesiveness of calcium oxalate stones to these cells. Consequently, this enhances the aggregation, nucleation, and growth of stone crystals in the kidneys.
4 Antioxidant
4.1 Sources and classification of antioxidants
Antioxidants are compounds that inhibit the oxidation of other compounds. The process of oxidation involves the transfer of electrons from a substance to an oxidizing agent, resulting in the generation of free radicals that initiate a cascade reaction. When this chain reaction occurs within a cell, it results in cellular damage or apoptosis. Antioxidants’ role is to eliminate free radicals, terminate chain reactions, and inhibit further oxidative reactions, while they themselves are oxidized (30, 31).
Antioxidants are abundant in daily life, with major sources including endogenously synthesized antioxidants, such as water-soluble and lipid-soluble antioxidants and antioxidative enzymes;natural antioxidants from food, such as various types of flavonoids, flavanols, anthocyanins, etc.; and common synthetic antioxidants such as butyl hydroxybenzoate (BHA), butylated hydroxytoluene (BHT), EDTA, etc. (32). Antioxidants are categorized into enzymatic antioxidants (including superoxide dismutase (SOD), catalase (CAT), glutathione peroxidase (GPx), and thioredoxin (Trx) systems) and non-enzymatic antioxidants (such as flavanes, phenols, and carotenoids) (33). Additionally, certain minerals like selenium and zinc function as antioxidants. These minerals are crucial components of antioxidant enzymes and are essential for maintaining their activity.
4.2 Mechanisms of antioxidant action
Antioxidants play a crucial role in the body by inhibiting oxidative reactions and reducing cellular damage. They can protect cell membranes; for example, vitamin E acts as a peroxide scavenger, capturing peroxyl free radicals to halt free radical chain reactions, which helps prevent lipid peroxidation (34). Rachel et al. demonstrated that antioxidants like superoxide dismutase (SOD) can convert superoxide into oxygen and hydrogen peroxide, thereby protecting cells against harm caused by free radicals (35).
Moreover, antioxidants affect various biological processes, including apoptosis, inflammation, and immune responses. For instance, vitamin C can inhibit endothelial cell oxidative stress induced by tumor necrosis factor-alpha (TNF-α), thus protecting vascular endothelial cells from damage (36). Additionally, phenolic compounds, another type of antioxidant, can halt the propagation of free radicals by exchanging protons with them (37). Furthermore, antioxidants can modulate the activity of inflammation-related transcription factors like NF-κB, thereby diminishing inflammatory responses (38). They also regulate the activity of other transcription factors such as AP-1, Sp-1, affecting gene expression (39).
While antioxidants offer numerous benefits to the body, it’s important to be mindful of their dual effects. At low concentrations, antioxidants protect against oxidative cell damage; however, at high concentrations, they may become toxic and disrupt cellular redox balance. Additionally, antioxidants can chelating metal ions, reducing their oxidizing capacity. Yet, this interaction might also result in increased levels of reactive hydroperoxides from these ions, eventually generating harmful free radicals (40). Consequently, the exploration and application of antioxidants in therapeutic contexts present significant challenges that need to be addressed.
5 The role of antioxidants in the treatment of kidney stones
5.1 The role of SOD in the treatment of kidney stones
Superoxide Dismutase (SOD) is a crucial antioxidant enzyme in cells, playing a pivotal role in shielding them from oxidative stress damage (41). There are three types of SOD: Cu/Zn-SOD (SOD1) found primarily in the cytoplasm, Mn-SOD (SOD2) located in mitochondria, and EC-SOD (SOD3) present in the extracellular matrix of mammalian tissues (42, 43). SOD1 catalyzes the reaction between superoxide (O2•−) and hydroperoxide (H2O2), aiding in the reduction of intracellular superoxide levels (44). SOD2 helps maintain intracellular redox balance by countering oxidative stress in mitochondria (45). They differ in their genetic structure, evolution, and expression patterns. These enzymes play a crucial role in organisms by helping to maintain oxidative balance and protect cells from the effects of oxidative stress.
Kang et al. discovered that increasing SOD activity significantly lowers the incidence of kidney stone formation and kidney injury, highlighting SOD’s ability to inhibit autophagy and endoplasmic stress response (46).The process of kidney stone formation is highly intricate, such as mitochondrial dysfunction, which disrupts energy metabolism, intracellular calcium ion balance, and the antioxidative defense system, and can lead to tubular cell damage, apoptosis, and the formation of stones through the combination of cellular inclusions and mitochondrial debris with calcium oxalate crystals in the tubular lumen (47). Antioxidant supplementation, by restoring the enzymatic activities of SOD, catalase (CAT), glutathione peroxidase, and glutathione (GSH), can prevent or mitigate the severity of stone deposits (48). Therefore, SOD, particularly Mn-SOD in mitochondria, plays a critical protective role against kidney stone formation.
5.2 The role of vitamin E in the treatment of kidney stones
Vitamin E, a fat-soluble antioxidant, plays a critical role in protecting polyunsaturated fatty acids within cell membranes from oxidative damage, thereby preserving cell membrane integrity (49). Known for its potent antioxidative properties, vitamin E counters free radical damage and has shown potential benefits in a range of diseases, including cancer, cataracts, Alzheimer’s disease, asthma, allergies, and diabetes. Specifically, Thamilselvan et al. demonstrated that vitamin E therapy can prevent calcium oxalate crystal deposition in the kidneys induced by hyperoxaluria, enhancing the antioxidant status of renal tissues (50). By trapping free radicals, vitamin E reduces their cytotoxic effects, which in turn helps protect cells from oxidative harm and may inhibit the attachment of calcium oxide apatite crystals, thereby preventing kidney stone formation (51). Furthermore, vitamin E is suggested to have a protective role in progressive renal failure, especially as oxidative stress exacerbates the progression of chronic inflammatory nephropathy (52).
5.3 The role of tea polyphenols in the treatment of kidney stones
Tea contains a collection of polycyclic phenolic compounds known as tea polyphenols, primarily consist of flavanols like catechins, along with flavan derivatives such as theaflavins (TF) and thearubigins (TR) (53). These compounds function as antioxidants by donating hydrogen atoms to inhibit free radical production, thus protecting cells from oxidative damage (54).
Research has found that NF-κB can regulate the expression of genes encoding adhesion molecules, COX-2, and inflammatory cytokines (such as TNF-α, IL-6, and CRP). These factors contribute to kidney stone formation by activating NADPH oxidase, which stimulates ROS production and impairs endothelial function through a vicious cycle mechanism [2]. Tea polyphenols counteract this by enhancing cellular antioxidant defenses, stimulating the Nrf2-Keap1-ARE signaling pathway, while suppressing the NF-κB signaling pathway (55). Catechins, the main substances in tea polyphenols, trap free radicals, thereby preventing oxidative stress-induced cellular damage. Their protective effect extends to inhibiting apoptosis caused by oxidative stress and chelating metal ions like copper and iron to reduce free radical production (56). Furthermore, Ye et al. found that theaflavins can up-regulate the expression of SIRT1, attenuating oxidative stress injury in the kidney induced by calcium oxalate. This phenomenon is partly attributed to the suppression of miR-128-3p, which normally suppresses SIRT1, suggesting new potential therapeutic targets for calcium oxalate kidney stones (57).
5.4 The role of sulforaphane in the treatment of kidney stones
SFN (sulforaphane), is an isothiocyanate produced through glucosidase hydrolysis in cruciferous plants, known for its antioxidant properties (58). Nrf2 is a key transcription factor in the oxidative stress response, binding to the antioxidant response element (ARE) in the promoter regions of cytoprotective genes. Normally, nuclear factor erythroid 2-related factor 2 (Nrf2) is regulated by the Keap1 complex and degraded in the cytoplasm. However, under oxidative stress, Nrf2 dissociates from Keap1, avoiding degradation. This stabilized Nrf2 then enters the nucleus, forms heterodimers with Maf protein, and activates genes associated with antioxidant and detoxification by binding to AREs. This action reduces ROS levels and alleviates renal damage caused by oxidative stress (59) (Figure 3).
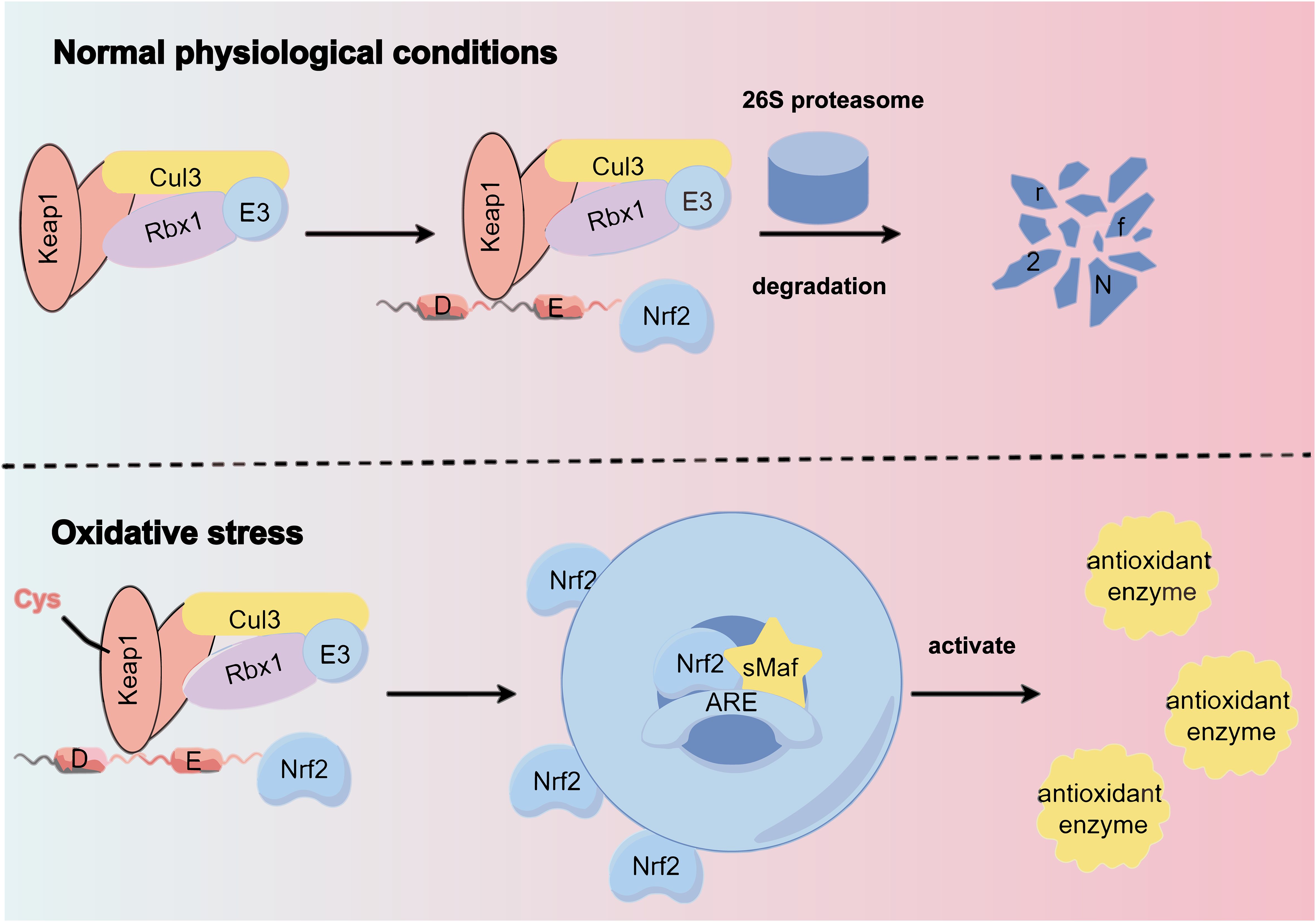
Figure 3. Under normal physiological conditions, the Keap1 complex binds to Nrf2, promoting its ubiquitination and subsequent degradation by the 26S proteasome. However, oxidative stress alters specific cysteine residues in Keap1, causing a conformational change that disrupts Nrf2 ubiquitination. This allows Nrf2 to translocate to the nucleus and bind to the antioxidant response element (ARE) of target genes via heterodimerization with sMAF protein, inducing the expression of cellular protective genes.
Liu et al. found that SFN significantly lowers Toll-like receptor 4 (TLR4) and interferon regulatory factor 1 (IRF1) levels in a CaOx kidney stone model by activating Nrf2. This activation promotes M2 macrophage activation, which can help reduce renal inflammatory injury and aid in preventing kidney stones (60). Additionally, SFN has been noted to encourage mitochondrial biogenesis through the Nrf2-PGC-1α (peroxisome proliferator-activated receptor gamma cofactor 1α) signaling pathway. This enhances mitochondrial number and function in reaction to damage caused by OS (61).
6 Conclusion
The link between ROS and the formation of CaOx has led to deep reflection on the pathogenesis of urinary stones. First, the presence of ROS may be a primary driving factor of oxidative stress in the urinary system. Their highly reactive nature allows interactions with in vivo biomolecules, leading to oxidative damage and inflammatory responses (62). These effects can intensify the crystallization of calcium oxalate in urine, thus creating conducive conditions for stone formation (63). Secondly, the relationship between ROS and the formation of urinary stones highlights the key role of redox balance in maintaining urinary stability and preventing stone formation. Oxidative stress disturbs this balance, creating an unstable cellular environment that can damage DNA, proteins, and lipids, as well as cause inflammation and harm to renal epithelial cells (64). Increased oxidative stress also results in heightened urinary solute supersaturation, a key factor in kidney stone formation (65).
Further research indicates that dietary and lifestyle factors significantly influence the generation and elimination of ROS. Habits such as smoking, excessive alcohol consumption, and having a sedentary lifestyle can lead to OS, which is an imbalance between the production of ROS and the body’s antioxidant defenses (66). Diets high in salt and fat, low in fiber, along with insufficient physical activity and other poor lifestyle habits, may exacerbate this stress, thus heightening the risk of calcium oxalate stones (67, 68). For those predisposed to stone formation, dietary and lifestyle modifications can be crucial in reducing ROS levels and the associated risk (69).
Additionally, the ROS-urinary stone connection offers insights for new therapeutic approaches. Exploring the potential of ROS scavengers or antioxidants in preventing and treating CaOx holds promise for innovative clinical treatment directions. This therapeutic strategy, by alleviating oxidative stress and blocking key stages in stone formation, could offer more effective and personalized treatment options for patients.
Antioxidants, compounds that aid in the elimination of ROS, have wide-ranging prospects for kidney health maintenance. Firstly, various antioxidants like vitamin E, flavonoids, and polyphenols have demonstrated efficacy in counteracting oxidative stress and reducing its impact on the body (70). By scavenging free radicals and other reactive oxygen molecules, antioxidants help to minimize oxidative harm to nucleic acids, proteins, and cell membranes, thus preserving the structural and functional integrity of kidney cells. Secondly, antioxidants may play a positive role in inhibiting inflammatory responses (71). Inflammation is an essential element in the progression of renal diseases, and antioxidants could potentially alleviate the extent of renal tissue damage by dampening the inflammation induced by oxidative stress (72). Furthermore, antioxidants may positively influence renal vascular health (73). Since oxidative stress is associated with vascular dysfunction, the application of antioxidants could protect the renal microcirculation by maintaining endothelial function and reducing vascular permeability, thereby helping to prevent some vascular complications related to kidney diseases.
Antioxidants hold significant potential in promoting kidney health. By improving the effectiveness of the body’s antioxidant defense system, antioxidants are expected to mitigate cell damage and inhibit inflammatory responses, thereby slowing the formation of CaOx. Future research should aim to unravel the specific mechanisms of various antioxidants in the kidney, focusing on their influence on cell signaling pathways, gene expression, and the modulation of inflammatory mediators. This will help clarify the precise impact of antioxidants on kidney health. Additionally, detailed investigations into how ROS contribute to the formation of calcium oxalate stones are essential. These studies will offer a more comprehensive view of the impact of oxidative stress on the urinary tract system and aid in the development of precise and targeted interventions.
Author contributions
HL: Conceptualization, Methodology, Visualization, Writing – original draft, Writing – review & editing, Funding acquisition. XY: Visualization, Writing – original draft, Writing – review & editing. YC: Conceptualization, Methodology, Visualization, Writing – review & editing. ZH: Conceptualization, Validation, Visualization, Writing – review & editing.
Funding
The author(s) declare that financial support was received for the research and/or publication of this article. This study was supported by National Natural Science Foundation of China (82070724, 82100808), Young Elite Scientists Sponsorship Program (2023QNRC001).
Acknowledgments
We thank Figdraw (www.figdraw.com) for expert assistance in the pattern drawing.
Conflict of interest
The authors declare that the research was conducted in the absence of any commercial or financial relationships that could be construed as a potential conflict of interest.
Generative AI statement
The author(s) declare that no Generative AI was used in the creation of this manuscript.
Publisher’s note
All claims expressed in this article are solely those of the authors and do not necessarily represent those of their affiliated organizations, or those of the publisher, the editors and the reviewers. Any product that may be evaluated in this article, or claim that may be made by its manufacturer, is not guaranteed or endorsed by the publisher.
References
1. Howles SA and Thakker RV. Genetics of kidney stone disease. Nat Rev Urol. (2020) 17:407–21. doi: 10.1038/s41585-020-0332-x
2. Stamatelou K and Goldfarb DS. Epidemiology of kidney stones. Healthcare. (2023) 11:424. doi: 10.3390/healthcare11030424
3. Wigner P, Grębowski R, Bijak M, Szemraj J, and Saluk-Bijak J. The molecular aspect of nephrolithiasis development. Cells. (2021) 10:1926. doi: 10.3390/cells10081926
4. Khan SR, Pearle MS, Robertson WG, Gambaro G, Canales BK, Doizi S, et al. Kidney stones. Nat Rev Dis Primer. (2016) 2:16008. doi: 10.1038/nrdp.2016.8
5. Güzel R, Yildirim Ü, and Sarica K. Contemporary minimal invasive surgical management of stones in children. Asian J Urol. (2023) 10:239–45. doi: 10.1016/j.ajur.2023.02.001
6. Liu H, Ye T, Yang X, Liu J, Jiang K, Lu H, et al. H19 promote calcium oxalate nephrocalcinosis-induced renal tubular epithelial cell injury via a ceRNA pathway. EBioMedicine. (2019) 50:366–78. doi: 10.1016/j.ebiom.2019.10.059
7. Su L, Zhang J, Gomez H, Kellum JA, and Peng Z. Mitochondria ROS and mitophagy in acute kidney injury. Autophagy. (2023) 19:401–14. doi: 10.1080/15548627.2022.2084862
8. Mulay SR, Eberhard JN, Desai J, Marschner JA, Kumar SVR, Weidenbusch M, et al. Hyperoxaluria requires TNF receptors to initiate crystal adhesion and kidney stone disease. J Am Soc Nephrol. (2017) 28:761–8. doi: 10.1681/ASN.2016040486
9. Fraternale A, Zara C, De Angelis M, Nencioni L, Palamara AT, Retini M, et al. Intracellular redox-modulated pathways as targets for effective approaches in the treatment of viral infection. Int J Mol Sci. (2021) 22:3603. doi: 10.3390/ijms22073603
10. Dröge W. Free radicals in the physiological control of cell function. Physiol Rev. (2002) 82:47–95. doi: 10.1152/physrev.00018.2001
11. Hernansanz-Agustín P and Enríquez JA. Generation of reactive oxygen species by mitochondria. Antioxidants. (2021) 10:415. doi: 10.3390/antiox10030415
12. Rudolf J, Raad H, Taieb A, and Rezvani HR. NADPH oxidases and their roles in skin homeostasis and carcinogenesis. Antioxid Redox Signal. (2018) 28:1238–61. doi: 10.1089/ars.2017.7282
13. Li A, Wang J, Zhu D, Zhang X, Pan R, and Wang R. Arctigenin suppresses transforming growth factor-β1-induced expression of monocyte chemoattractant protein-1 and the subsequent epithelial–mesenchymal transition through reactive oxygen species-dependent ERK/NF-κB signaling pathway in renal tubular epithelial cells. Free Radic Res. (2015) 49:1095–113. doi: 10.3109/10715762.2015.1038258
14. Rashed T, Menon M, and Thamilselvan S. Molecular mechanism of oxalate-induced free radical production and glutathione redox imbalance in renal epithelial cells: effect of antioxidants. Am J Nephrol. (2004) 24:557–68. doi: 10.1159/000082043
15. Zhang Y, Murugesan P, Huang K, and Cai H. NADPH oxidases and oxidase crosstalk in cardiovascular diseases: novel therapeutic targets. Nat Rev Cardiol. (2020) 17:170–94. doi: 10.1038/s41569-019-0260-8
16. Ray PD, Huang B-W, and Tsuji Y. Reactive oxygen species (ROS) homeostasis and redox regulation in cellular signaling. Cell Signal. (2012) 24:981–90. doi: 10.1016/j.cellsig.2012.01.008
18. Di Meo S, Reed TT, Venditti P, and Victor VM. Role of ROS and RNS sources in physiological and pathological conditions. Oxid Med Cell Longev. (2016) 2016:1245049. doi: 10.1155/2016/1245049
19. Bisbal C, Lambert K, and Avignon A. Antioxidants and glucose metabolism disorders. Curr Opin Clin Nutr Metab Care. (2010) 13:439–46. doi: 10.1097/MCO.0b013e32833a5559
20. Abhishek A, Benita S, Kumari M, Ganesan D, Paul E, Sasikumar P, et al. Molecular analysis of oxalate-induced endoplasmic reticulum stress mediated apoptosis in the pathogenesis of kidney stone disease. J Physiol Biochem. (2017) 73:561–73. doi: 10.1007/s13105-017-0587-8
21. Sies H. Oxidative stress: a concept in redox biology and medicine. Redox Biol. (2015) 4:180–3. doi: 10.1016/j.redox.2015.01.002
22. Tsujihata M. Mechanism of calcium oxalate renal stone formation and renal tubular cell injury. Int J Urol. (2008) 15:115–20. doi: 10.1111/j.1442-2042.2007.01953.x
23. Joshi S, Wang W, Peck AB, and Khan SR. Activation of the NLRP3 inflammasome in association with calcium oxalate crystal induced reactive oxygen species in kidneys. J Urol. (2015) 193:1684–91. doi: 10.1016/j.juro.2014.11.093
24. Liu Y, Sun Y, Kang J, He Z, Liu Q, Wu J, et al. Role of ROS-induced NLRP3 inflammasome activation in the formation of calcium oxalate nephrolithiasis. Front Immunol. (2022) 13:818625. doi: 10.3389/fimmu.2022.818625
25. Kumar P, Laurence E, Crossman DK, Assimos DG, Murphy MP, and Mitchell T. Oxalate disrupts monocyte and macrophage cellular function via Interleukin-10 and mitochondrial reactive oxygen species (ROS) signaling. Redox Biol. (2023) 67:102919. doi: 10.1016/j.redox.2023.102919
26. Huang H-S and Ma M-C. High sodium-induced oxidative stress and poor anticrystallization defense aggravate calcium oxalate crystal formation in rat hyperoxaluric kidneys. PloS One. (2015) 10:e0134764. doi: 10.1371/journal.pone.0134764
27. Khan SR, Joshi S, Wang W, and Peck AB. Regulation of macromolecular modulators of urinary stone formation by reactive oxygen species: Transcriptional study in an animal model of hyperoxaluria. Am J Physiol-Ren Physiol. (2014) 306:F1285–95. doi: 10.1152/ajprenal.00057.2014
28. Chaiyarit S and Thongboonkerd V. Oxidative modifications switch modulatory activities of urinary proteins from inhibiting to promoting calcium oxalate crystallization, growth, and aggregation. Mol Cell Proteomics. (2021) 20:100151. doi: 10.1016/j.mcpro.2021.100151
29. Yu L, Gan X, Liu X, and An R. Calcium oxalate crystals induces tight junction disruption in distal renal tubular epithelial cells by activating ROS/Akt/p38 MAPK signaling pathway. Ren Fail. (2017) 39:440–51. doi: 10.1080/0886022X.2017.1305968
30. Hęś M, Dziedzic K, Górecka D, Jędrusek-Golińska A, and Gujska E. Aloe vera (L.) webb.: natural sources of antioxidants – A review. Plant Foods Hum Nutr. (2019) 74:255–65. doi: 10.1007/s11130-019-00747-5
31. Sies H. Oxidative stress: oxidants and antioxidants. Exp Physiol. (1997) 82:291–5. doi: 10.1113/expphysiol.1997.sp004024
32. Neha K, Haider MR, Pathak A, and Yar MS. Medicinal prospects of antioxidants: A review. Eur J Med Chem. (2019) 178:687–704. doi: 10.1016/j.ejmech.2019.06.010
33. Liang J, Gao Y, Feng Z, Zhang B, Na Z, and Li D. Reactive oxygen species and ovarian diseases: Antioxidant strategies. Redox Biol. (2023) 62:102659. doi: 10.1016/j.redox.2023.102659
34. Traber MG and Atkinson J. antioxidant and nothing more. Free Radic Biol Med. (2007) 43:4–15. doi: 10.1016/j.freeradbiomed.2007.03.024
35. Miller RA and Britigan BE. Role of oxidants in microbial pathophysiology. Clin Microbiol Rev. (1997) 10(1):1–18 doi: 10.1128/CMR.10.1.1
36. Vertuani S, Angusti A, and Manfredini S. The antioxidants and pro-antioxidants network: an overview. Curr Pharm Des. (2004) 10:1677–94. doi: 10.2174/1381612043384655
37. Zhang P, Li T, Wu X, Nice EC, Huang C, and Zhang Y. Oxidative stress and diabetes: antioxidative strategies. Front Med. (2020) 14:583–600. doi: 10.1007/s11684-019-0729-1
38. Allan K, Kelly FJ, and Devereux G. Antioxidants and allergic disease: a case of too little or too much? Clin Exp Allergy. (2010) 40:370–80. doi: 10.1111/j.1365-2222.2009.03413.x
39. Birben E, Sahiner UM, Sackesen C, Erzurum S, and Kalayci O. Oxidative stress and antioxidant defense. World Allergy Organ J. (2012) 5:9–19. doi: 10.1097/WOX.0b013e3182439613
40. Bouayed J and Bohn T. Exogenous Antioxidants—Double-Edged Swords in Cellular Redox State: Health Beneficial Effects at Physiologic Doses versus Deleterious Effects at High Doses. Oxid Med Cell Longev. (2010) 3:228–37. doi: 10.4161/oxim.3.4.12858
41. Liu C-C, Wu C-F, Lee Y-C, Huang T-Y, Huang S-T, Wang H-S, et al. Genetic polymorphisms of mnSOD modify the impacts of environmental melamine on oxidative stress and early kidney injury in calcium urolithiasis patients. Antioxidants. (2022) 11:152. doi: 10.3390/antiox11010152
42. Zelko IN, Mariani TJ, and Folz RJ. Superoxide dismutase multigene family: a comparison of the CuZn-SOD (SOD1), Mn-SOD (SOD2), and EC-SOD (SOD3) gene structures, evolution, and expression. Free Radic Biol Med. (2002) 33:337–49. doi: 10.1016/S0891-5849(02)00905-X
43. Nozik-Grayck E, Suliman HB, and Piantadosi CA. Extracellular superoxide dismutase. Int J Biochem Cell Biol. (2005) 37:2466–71. doi: 10.1016/j.biocel.2005.06.012
44. Rubino V, La Rosa G, Pipicelli L, Carriero F, Damiano S, Santillo M, et al. Insights on the multifaceted roles of wild-type and mutated superoxide dismutase 1 in amyotrophic lateral sclerosis pathogenesis. Antioxidants. (2023) 12:1747. doi: 10.3390/antiox12091747
45. Fukai T and Ushio-Fukai M. Superoxide dismutases: role in redox signaling, vascular function, and diseases. Antioxid Redox Signal. (2011) 15:1583–606. doi: 10.1089/ars.2011.3999
46. Kang J, Sun Y, Deng Y, Liu Q, Li D, Liu Y, et al. Autophagy-endoplasmic reticulum stress inhibition mechanism of superoxide dismutase in the formation of calcium oxalate kidney stones. BioMed Pharmacother. (2020) 121:109649. doi: 10.1016/j.biopha.2019.109649
47. Chaiyarit S and Thongboonkerd V. Mitochondrial dysfunction and kidney stone disease. Front Physiol. (2020) 11:566506. doi: 10.3389/fphys.2020.566506
48. Albert A, Paul E, Rajakumar S, and Saso L. Oxidative stress and endoplasmic stress in calcium oxalate stone disease: the chicken or the egg? Free Radic Res. (2020) 54:244–53. doi: 10.1080/10715762.2020.1751835
49. Blaner WS, Shmarakov IO, and Traber MG. Vitamin A and vitamin E: will the real antioxidant please stand up? Annu Rev Nutr. (2021) 41:105–31. doi: 10.1146/annurev-nutr-082018-124228
50. Thamilselvan S and Menon M. Vitamin E therapy prevents hyperoxaluria-induced calcium oxalate crystal deposition in the kidney by improving renal tissue antioxidant status. BJU Int. (2005) 96:117–26. doi: 10.1111/j.1464-410X.2005.05579.x
51. Chmiel JA, Stuivenberg GA, Al KF, Akouris PP, Razvi H, Burton JP, et al. Vitamins as regulators of calcium-containing kidney stones — new perspectives on the role of the gut microbiome. Nat Rev Urol. (2023) 20:615–37. doi: 10.1038/s41585-023-00768-5
52. Fryer MJ. Vitamin E as a protective antioxidant in progressive renal failure. Nephrology. (2000) 5:1–7. doi: 10.1046/j.1440-1797.2000.00504.x
53. Gramza A, Korczak J, and Amarowicz R. Tea polyphenols – their antioxidant properties and biological activity – a review.
54. Wiseman SA, Balentine DA, and Frei B. Antioxidants in tea. Crit Rev Food Sci Nutr. (1997) 37:705–18. doi: 10.1080/10408399709527798
55. Yan Z, Zhong Y, Duan Y, Chen Q, and Li F. Antioxidant mechanism of tea polyphenols and its impact on health benefits. Anim Nutr. (2020) 6:115–23. doi: 10.1016/j.aninu.2020.01.001
56. Kumar Sen A, Sen DB, Zanwar AS, Balaraman R, Shah U, and Maheshwari RA. atechins and theaflavins: an overview on therapeutic application. J Nat Remedies. (2022), 330–46. doi: 10.18311/jnr/2022/30181
57. Ye T, Yang X, Liu H, Lv P, Lu H, Jiang K, et al. Theaflavin protects against oxalate calcium-induced kidney oxidative stress injury via upregulation of SIRT1. Int J Biol Sci. (2021) 17:1050–60. doi: 10.7150/ijbs.57160
58. Chang R. Research advances in the protective effect of sulforaphane against kidney injury and related mechanisms. Bio Web Conf. (2022) 55:1006. doi: 10.1051/bioconf/20225501006
59. Wei W, Ma N, Fan X, Yu Q, and Ci X. The role of Nrf2 in acute kidney injury: Novel molecular mechanisms and therapeutic approaches. Free Radic Biol Med. (2020) 158:1–12. doi: 10.1016/j.freeradbiomed.2020.06.025
60. Liu H, Yang X, Tang K, Ye T, Duan C, Lv P, et al. Sulforaphane elicts dual therapeutic effects on Renal Inflammatory Injury and crystal deposition in Calcium Oxalate Nephrocalcinosis. Theranostics. (2020) 10:7319–34. doi: 10.7150/thno.44054
61. Huang J, Liang Y, and Zhou L. Natural products for kidney disease treatment: Focus on targeting mitochondrial dysfunction. Front Pharmacol. (2023) 14:1142001. doi: 10.3389/fphar.2023.1142001
62. Mittal M, Siddiqui MR, Tran K, Reddy SP, and Malik AB. Reactive oxygen species in inflammation and tissue injury. Antioxid Redox Signal. (2014) 20:1126–67. doi: 10.1089/ars.2012.5149
63. Khan SR. Reactive oxygen species, inflammation and calcium oxalate nephrolithiasis. Transl Androl Urol. (2014), 3.
64. Farhood B, Najafi M, Salehi E, Hashemi Goradel N, Nashtaei MS, Khanlarkhani N, et al. Disruption of the redox balance with either oxidative or anti-oxidative overloading as a promising target for cancer therapy. J Cell Biochem. (2019) 120:71–6. doi: 10.1002/jcb.27594
65. Bird VY and Khan SR. How do stones form? Is unification of theories on stone formation possible?. Arch Esp Urol (2018) 70(1):12–27.
66. Sharifi-Rad M, Anil Kumar NV, Zucca P, Varoni EM, Dini L, Panzarini E, et al. Lifestyle, oxidative stress, and antioxidants: back and forth in the pathophysiology of chronic diseases. Front Physiol. (2020) 11:694. doi: 10.3389/fphys.2020.00694
67. Nouvenne A, Ticinesi A, Morelli I, Guida L, Borghi L, and Meschi T. Fad diets and their effect on urinary stone formation. Transl Androl Urol. (2014) 3(3):303-12. doi: 10.3978/j.issn.2223-4683.2014.06.01
68. Nawaz N, Tahir H, Tamiz H, Basharat S, Hassan M, and Aamir M. Association between dietary practices and calcium oxalate stone formation in urinary tract among the patients of urolithiasis. (2020) 3:.
69. Khan SR. Is oxidative stress, a link between nephrolithiasis and obesity, hypertension, diabetes, chronic kidney disease, metabolic syndrome? Urol Res. (2012) 40:95–112. doi: 10.1007/s00240-011-0448-9
70. Pizzino G, Irrera N, Cucinotta M, Pallio G, Mannino F, Arcoraci V, et al. Oxidative stress: harms and benefits for human health. Oxid Med Cell Longev. (2017) 2017:8416763. doi: 10.1155/2017/8416763
71. Arulselvan P, Fard MT, Tan WS, Gothai S, Fakurazi S, Norhaizan ME, et al. Role of antioxidants and natural products in inflammation. Oxid Med Cell Longev. (2016) 2016:5276130. doi: 10.1155/2016/5276130
72. Hong YA and Park CW. Catalytic antioxidants in the kidney. Antioxidants. (2021) 10:130. doi: 10.3390/antiox10010130
Keywords: kidney stones, reactive oxygen species, antioxidants, oxidative stress, renal
Citation: Ying X, Chen Y, Hao Z and Liu H (2025) The significance of reactive oxygen species in the formation of calcium oxalate stones and the protective effects of antioxidants on the kidneys. Front. Immunol. 16:1540075. doi: 10.3389/fimmu.2025.1540075
Received: 05 December 2024; Accepted: 06 May 2025;
Published: 21 May 2025.
Edited by:
Bilal ÇİĞ, Ahi Evran University, TürkiyeReviewed by:
Wei Zhu, First Affiliated Hospital of Guangzhou Medical University, ChinaRishi Bhardwaj, Yale University, United States
Copyright © 2025 Ying, Chen, Hao and Liu. This is an open-access article distributed under the terms of the Creative Commons Attribution License (CC BY). The use, distribution or reproduction in other forums is permitted, provided the original author(s) and the copyright owner(s) are credited and that the original publication in this journal is cited, in accordance with accepted academic practice. No use, distribution or reproduction is permitted which does not comply with these terms.
*Correspondence: ZongYao Hao, aGFvem9uZ3lhb0AxNjMuY29t; HaoRan Liu, aHJsaXU2NkBzdGFuZm9yZC5lZHU=
†These authors have contributed equally to this work