- 1Department of Liver Surgery and Liver Transplantation, Renji Hospital, School of Medicine, Shanghai Jiao Tong University, Shanghai, China
- 2Shanghai Engineering Research Center of Transplantation and Immunology, Shanghai, China
Interleukin-21 (IL-21) is a cytokine that plays a crucial role in regulating immune responses, affecting various immune cell types, including T cells, B cells, natural killer (NK) cells, and dendritic cells. IL-21 is primarily produced by CD4+ T cells, particularly follicular helper T (Tfh) cells and Th17 cells, and has been shown to be extensively involved in regulating both innate and adaptive immunity. IL-21 is particularly significant in the differentiation, proliferation, and effector functions of T cells and B cells. In the context of organ transplantation, IL-21 contributes to the promotion of acute transplant rejection and the development of chronic rejection, which is primarily antibody-mediated. This review summarizes relevant studies on IL-21 and discusses its multifaceted roles in transplant immune rejection, providing insights into therapeutic strategies for either inhibiting graft rejection or promoting tolerance. It also explores the feasibility of blocking the IL-21 signaling pathway within current immunosuppressive regimens, aiming to provide further clinical references.
1 Introduction
Organ transplantation is the only viable therapeutic option for patients with end-stage conditions such as cirrhosis and renal failure. In allogeneic organ transplantation, polymorphisms in the MHC genes between individuals facilitate immune cell recognition of “foreign” components, initiating immune signaling between the donor and recipient. This process triggers an immune response against the donor graft, which carries “foreign” antigens, ultimately leading to transplant rejection. In innate immunity, NK cell infiltration into the graft followed by the release of granzyme B and interferon-γ is considered one of the hallmarks of acute rejection (1, 2). Macrophages migrate to the local environment of the graft and exert phagocytic activity (3), and they can also function as antigen-presenting cells (APCs) to activate T cells (4), releasing various inflammatory cytokines (e.g., IL-1, IL-6, TNF-α) to amplify the immune response (5). In adaptive immunity, T cell-mediated rejection (TCMR), which involves cytotoxic CD8+ T cell responses against mismatched MHC class I allogeneic antigens, is considered the core mechanism of acute transplant rejection (6, 7). This process induces target cell apoptosis via the release of perforin and granzyme B or through the Fas/FasL pathway. Antibody-mediated rejection (AMR) is considered the primary pathway for chronic graft injury and dysfunction, where donor-specific antibodies (DSA) secreted by plasma cells lead to chronic vascular changes in the graft. For instance, in liver transplantation, DSA can cause occlusive arterial lesions (8, 9), and in lung transplantation, it leads to intimal thickening of pulmonary arteriovenous vessels (10–12). In summary, transplant rejection is a dynamic and complex process mediated by both innate and adaptive immunity. Various immune cells utilize different mechanisms to recognize and attack the donor graft, leading to both acute rejection and chronic graft injury and dysfunction.
Cytokines profoundly influence the fate of immune cells and regulate immune responses. They are often regarded as the third signal for T cell activation and differentiation (13). IL-21, as an important pleiotropic cytokine, has become one of the focal points of research in immunology and organ transplantation in recent years (14–19). As a member of the type I cytokine family, IL-21 profoundly affects immune cell fate and is involved in immune regulation in diseases such as autoimmune disorders, allergies, transplant rejection, and cancer. As shown in Figure 1, IL-21 enhances the cytotoxic activity of NK cells and the expression of IFN-γ (20). The IL-21 signaling pathway non-redundantly promotes the differentiation of naïve T cells into Tfh cells, which assist B cells in secreting antibodies, as evidenced by a significant reduction in both the number and proportion of Tfh cells following IL-21 signaling loss (21). IL-21 particularly influences B cell fate, playing a critical and indispensable role in numerous biological processes, such as promoting germinal center responses, class switching of antibodies, differentiation of B cells into plasma cells, and ultimately leading to the formation of donor-specific antibodies (22, 23). Exogenous IL-21 significantly promotes inflammatory infiltration in the allograft, induces the proliferation of CD4+ T cells and B cells, and accelerates rejection in allogeneic kidney-transplanted mice (15). Moreover, some previous studies have reported elevated mRNA or protein levels of IL-21 and IL-21R in allografts, and this suggests that IL-21 is deeply involved in transplant rejection. Endomyocardial biopsies from patients with allogeneic heart transplant rejection revealed high levels of IL-21 and IL-21R mRNA (24). During acute rejection in a rat allogeneic kidney transplant model, the mRNA and protein levels of IL-21 expressed by mononuclear leukocytes were significantly increased (25). Besides, Chronic lung allograft dysfunction (CLAD) after lung transplantation is one of the major challenges in the field. A study on donor-specific antibodies (DSA) and non-HLA antibodies after lung transplantation showed that CLAD lung lymphoid aggregates with local antibodies contained larger numbers of IgG plasma cells and exhibited significantly greater IL-21 expression (26). The effects of IL-21 on immune cells will be discussed in detail in subsequent sections. In summary, IL-21 affects the effector functions of various immune cells, which play a significant role in the pathophysiological processes of transplant rejection. Therefore, IL-21 is a key mediator in transplant rejection.
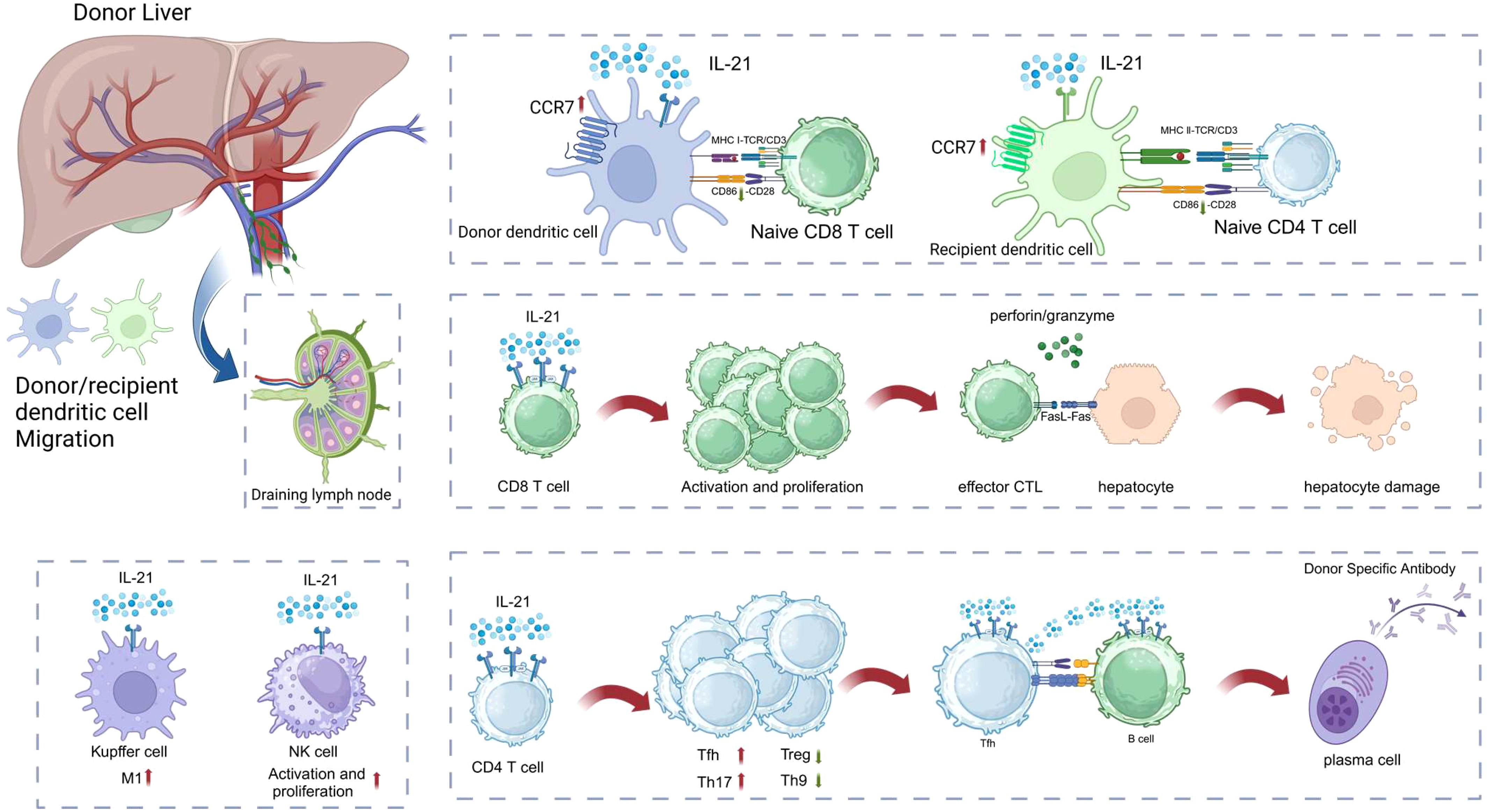
Figure 1. IL-21 affects a variety of immune cell effector functions, deeply involved in organ transplantation rejection. The Figure was created with www.biorender.com.
This review focuses on the functions of IL-21, exploring its effects on immune cells and its role in the pathophysiological mechanisms of transplant rejection, aiming to provide potential diagnostic and therapeutic insights for clinical practice.
2 Structure, receptor, secretion regulation and downstream signaling of IL-21
IL-21 was discovered in 2000 (27, 28)and is classified as a type I four-α-helix bundle cytokine, sharing a common γ-chain with IL-2, IL-4, IL-7, IL-9, and IL-15. Initially, IL-21 was thought to be primarily produced by Tfh and Th17 cells (21). However, recent studies have identified peripheral helper T cells (Tph), a subset of CD4+ T cells, as another source of IL-21 production (29). The secretion of IL-21 is mediated by ROCK2 via the RhoA-ROCK signaling pathway (30). Because the IL-21 gene is adjacent to the IL-2 gene, its transcriptional levels are significantly upregulated following T cell activation, similar to IL-2. A calcium signal alone is sufficient to induce IL-21 gene expression, and this calcium signal can be blocked by calcineurin inhibitors, such as tacrolimus. NFAT or NFATc2 directly activates the transcription of IL-21, while T-bet inhibits IL-21 expression by suppressing the binding of NFATc2 to the promoter (31). Furthermore, studies have shown that the production of IL-21 is dependent on the B7/CD28 co-stimulatory pathway (32). ICOS is another critical co-stimulatory molecule that influences the binding of c-Maf to the IL-21 locus during Tfh and Th17 cell development (33, 34). c-Maf activates the promoter and enhancer regions of the IL-21 gene. Other cytokine signals also play a crucial role in regulating IL-21 expression. IL-6, IL-12, and IL-23 have been shown to effectively induce IL-21 production in CD4+ T cells (35, 36), while TGF-β, which has immune-suppressive effects, significantly inhibits c-Maf-mediated IL-21 secretion in CD4+ T cells (37, 38). These findings highlight that IL-21 expression is regulated by multiple factors and is an important cytokine in immune responses.
The specific receptor for IL-21, IL-21R, is primarily expressed in lymphoid tissues but has also been identified in other cell types, such as synovial cells and epithelial cells (39). It shares a common gamma (γ) chain with the receptors for IL-2, IL-4, IL-7, IL-9, and IL-15. Similar to other type I cytokines, such as IL-2 and IL-15, IL-21 exerts its biological functions through the JAK/STAT signaling pathway (40, 41). Upon binding to IL-21R, Janus tyrosine kinase family members JAK1 and JAK3 are activated, leading to the phosphorylation of STAT1 and STAT3, with weaker phosphorylation of STAT5. STAT proteins are present as dimeric complexes in the cytoplasm of quiescent cells and are activated by growth factors and cytokines upon receptor activation. After activation, these proteins translocate to the nucleus, where they interact with regulatory regions of target genes (40, 42, 43). The activation of STAT1 induces the transcription of a wide array of genes, including T-bet, a key regulator in Th1 lineage differentiation and immunoglobulin class switch recombination. In addition, STAT1 also plays a critical role in Tfh and Th17 responses. A study on IL-6 and STAT1 demonstrated that IL-6 signaling specifically activates STAT1 in CD4+ T cells, and the absence of IL-6 severely impairs Tfh differentiation (44). In another study, inhibition of STAT1-mediated transcription was shown to effectively alleviate Th1- and Th17-mediated inflammatory diseases (45). Furthermore, STAT1 is critical for the generation of T-bet+ memory B cells, which facilitate tissue-resident humoral memory by producing IgG responses upon subsequent infection (46–48). STAT3 has highly complex functions, interacting with NF-κB family members to regulate the expression of numerous cytokines and mediators such as IL-6, IL-1β, prostacyclin, and cyclooxygenase-2. These interactions play critical roles in autoimmune diseases, as well as in the induction and maintenance of pro-tumor inflammatory environments (49, 50). STAT5, on the other hand, is associated with promoting cellular transformation and preventing apoptosis (51, 52). IL-21 functions through both autocrine and paracrine mechanisms, promoting and maintaining the phenotypes of Tfh and Th17 cells (21). Additionally, the phosphoinositide 3-kinase (PI3K/Akt) pathway and the mitogen-activated protein kinase (MAPK) pathway are also critical downstream signaling pathways of IL-21. Through these pathways and their downstream genes, IL-21 participates in immune responses (43). For instance, IL-21 enhances human NK cell proliferation and effector functions, such as increased granzyme and perforin expression, via the JAK/STAT and PI3K/MAPK pathways (53). It also downregulates the expression of inhibitory receptors Ly49 and NKG2D on human NK cells by inducing STAT3 tyrosine phosphorylation (54, 55).
3 Effects of IL-21 on immune cells
3.1 Macrophage
IL-21 enhances the phagocytic capacity of primary human monocytes and GM-CSF-derived macrophages through the MAPK, PI3K-Akt, and JAK-STAT signaling pathways (56, 57), while also supporting protease activity and the expression of matrix metalloproteinase 12 (58). Macrophage polarization remains a crucial area of immunological investigation, and notably, IL-21 modulates macrophage polarization in context-dependent manners. Locally administered IL-21 in tumors has been shown to shift tumor-associated macrophages (TAMs) from the M2 phenotype towards the M1 phenotype, exerting anti-tumor effects (59). In contrast, in the presence of LPS, IL-21 decreases the expression of CD86, iNOS, and TLR4, promoting the polarization of macrophages from the M1 to the M2 phenotype (60). IL-21 also fosters M2 polarization in primary alveolar macrophages, and following lung transplantation, elevated levels of IL-21 and M2 phenotype markers have been detected in patients with idiopathic pulmonary arterial hypertension (61). In rheumatoid arthritis (RA) patients, IL-21 has been found to impair the pro-inflammatory activity of M1-like macrophages in synovial fluid and to inhibit LPS-induced secretion of inflammatory mediators by synovial fluid macrophages, thereby exerting anti-inflammatory effects (62).
During organ transplantation, the restoration of blood flow to an isolated organ triggers ischemia-reperfusion injury (IRI). A study indicated an upregulation of IL-21 expression in the graft, suggesting its potential involvement in IRI (63). It has been demonstrated that in the context of IRI, Kupffer cells shift towards the M1 phenotype, exacerbating inflammatory damage (64, 65). Besides, macrophage infiltration is closely associated with the severity and outcome of allogeneic kidney transplantation. Significant infiltration of CD68+ cells has been detected in graft biopsies from cases of acute rejection (66). Strong expression of IL-21 and IL-21R mRNA was observed in leukocytes isolated from the vascular perfusion of rat kidney allografts, with the majority of IL-21R-positive cells being monocytes. This suggests that IL-21R is induced in intravascular monocytes within the graft in response to allogeneic transplantation (25).
3.2 Dendritic cells
Dendritic cells (DCs), as professional antigen-presenting cells (APCs), are renowned for their powerful ability to activate naïve T cells and are considered a bridge between innate and adaptive immunity. Early in this century, studies demonstrated that IL-21 inhibits the activation and maturation of DCs, as evidenced by lower expression of MHC class II molecules, increased antigen uptake, and reduced T cell activation in vitro (67, 68), with similar findings observed in chickens (69). Additionally, IL-21 stimulates the secretion of granzyme B by human plasmacytoid dendritic cells (pDCs), which downregulates TLR-induced CD4+ T cell proliferation through pre-activated pDCs (70). In a type 1 diabetes model, IL-21 regulates antigen transport in DCs, facilitating the acquisition of C-C chemokine receptor 7 (CCR7), thereby promoting their migration to draining lymph nodes (71). The authors found that in a virus-induced diabetes model, DCs in the pancreatic draining lymph nodes of wild-type mice had high CCR7 expression, whereas IL-21R-/- mice showed significantly reduced CCR7 levels. Flow cytometry revealed that CCR7+MHCII+ DCs in IL-21R-/- mice were only about 30% of those in wild-type mice, indicating IL-21R directly regulates CCR7 expression. Although IL-21R-/- DCs could uptake antigen normally, they failed to upregulate CCR7, reducing antigen-bearing DC migration to lymph nodes. This demonstrates that IL-21/IL-21R signaling is essential for DC migration in virus-induced type 1 diabetes, but whether this applies to transplantation requires further validation. Although similar studies are limited, this finding is important because this will cause a cascade of reactions: DCs take up antigen in presence of CCR7. Increased CCR7 expression mediates increased migration to the SLO along a CCL19/CCL21 gradient. Enhanced homing of DCs to SLO increases local T cell responses. Furthermore, IL-21 induces the apoptosis of conventional dendritic cells (cDCs) through STAT3 and Bim, a process that can be inhibited by granulocyte-macrophage colony-stimulating factor (GM-CSF) (72). ATR-107, a human anti-IL-21R monoclonal antibody, has been shown to induce DC activation and maturation, characterized by upregulation of co-stimulatory molecules such as CD86 and CD40 (73).
The migration of DCs to secondary lymphoid organs (SLOs) following organ transplantation is essential for the presentation and recognition of allogeneic antigens. As shown in Figure 2, there are three well-established pathways for allogeneic antigen recognition: the direct pathway, the indirect pathway, and the semi-direct pathway (74). The direct pathway occurs when the graft vasculature connects with the recipient’s circulation, enabling donor-derived DCs to migrate directly to the recipient’s SLO, where they are recognized by recipient CD8+ T cells for their mismatched MHC class I molecules, triggering a strong acute rejection response, a phenomenon known as the “passenger leukocyte” (75). In a fully mismatched rat spontaneous allogeneic liver transplant model (PVG→DA), donor-derived DCs were found in the recipient’s celiac lymph nodes (76). The indirect pathway involves the recipient’s DCs capturing foreign antigens and migrating to the recipient’s SLO, where they present antigens via MHC class II molecules to activate CD4+ T cells that are specific for the allogeneic antigens, which is more closely associated with chronic rejection (77). The semi-direct pathway involves the transfer of donor MHC molecules to the recipient’s DCs, allowing them to present donor antigens directly to the recipient’s T cells by “cross-modifying” the surface of recipient DCs (78). Interestingly, the transfer of MHC II molecules is a bidirectional process. A previous study (79) on mouse kidney and heart transplantation found that donor and recipient MHC II molecules could be bidirectionally transferred, forming double-positive cells and facilitating crosstalk between direct and indirect antigen presentation. Another study (80) in heart transplantation showed that the semidirect pathway is crucial for CD8+ T cell-mediated rejection, even without donor APCs. Moreover, in hematopoietic stem cell transplantation, “cross-dressed” DCs, while unable to independently induce T cell proliferation, enhance immunological synapse formation and strengthen indirect antigen presentation (81). Thus, the migration of DCs to SLOs is a critical process, and as mentioned above, IL-21 aids DCs in acquiring CCR7. Therefore, IL-21 seems to have multifaceted effects on DCs in the context of transplant rejection.
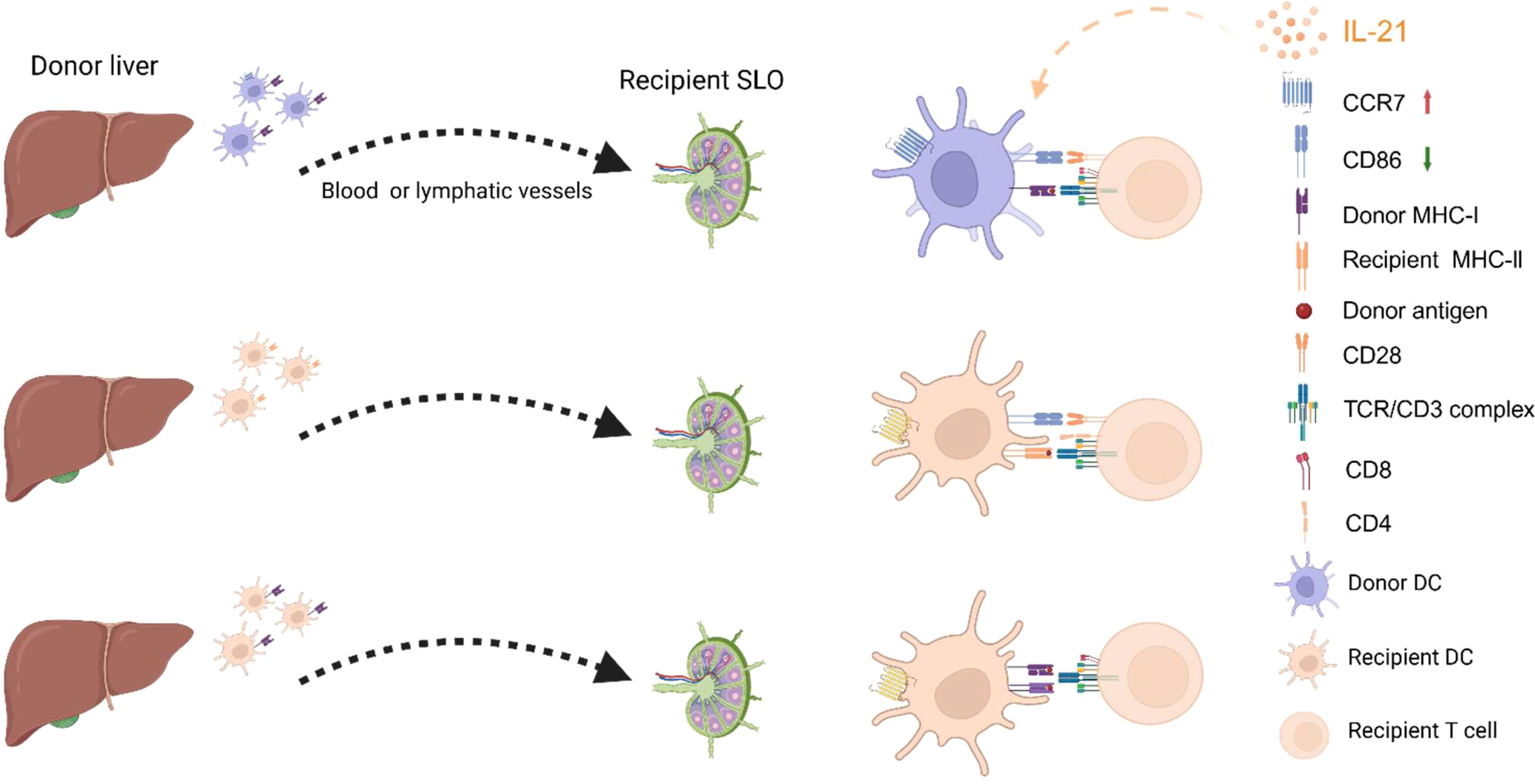
Figure 2. Pathways of Allogeneic Antigen Recognition and the Impact of IL-21 on DCs. From top to bottom, the figure shows the pathways of direct recognition, indirect recognition, and semi-direct recognition. On the one hand, IL-21 inhibits DC maturation by reducing the expression of surface co-stimulatory molecules such as CD86 and CD40 (not shown in the figure). On the other hand, IL-21 maintains the expression of CCR7 on DCs, enabling them to migrate to secondary lymphoid organs (SLOs). The figure was created with www.biorender.com.
3.3 NK cells
Due to the negative regulation of NK cells by MHC class I-specific inhibitory receptors, mismatched transplantation may trigger NK cell alloreactivity, resulting in graft injury and dysfunction (82). As previously mentioned, IL-21 enhances NK cell cytotoxicity and effector functions, which is generally considered a risk factor in allogeneic transplantation (20). Additionally, IL-21 promotes NK cell expansion (83), reverses NK cell exhaustion (84), and restores NK cell function in chronic HBV infection (85). Subsequent studies have shown that IL-21 has a biphasic effect on immature NK cells at different doses: low doses enhance their proliferation, while high doses reduce it (86). Interestingly, IL-21R knockout mice did not show defects in NK cell numbers or activation, and did not respond to IL-21, indicating that IL-21 is not necessary for NK cell production and development (20, 87).
In liver transplantation, NK cells display an interesting biphasic effect, with donor-derived NK cells responsible for inducing tolerance, while recipient-derived NK cells contribute to rejection (88). The latter is easy to understand, as NK cell infiltration and increased IFN-γ expression are commonly observed after transplantation (89), while NK cell exhaustion or reduced IFN-γ expression leads to improved graft survival (90). Regarding the former, a study by Marc Martínez-Llordella et al. on peripheral blood gene expression in liver transplant recipients who had discontinued immunosuppressive therapy suggested that the molecular pathways involved in the activation and effector functions of innate immune cell types (NK and γδTCR T cells) are central to maintaining operational tolerance after liver transplantation (91). In a study aimed at identifying blood biomarkers capable of predicting and diagnosing “operational tolerance” to safely reduce or even discontinue immunosuppressive therapy in liver transplant patients, the authors identified 367 differentially expressed genes in tolerant transplant recipients and further refined the gene set to 13 genes. These 13 genes exhibited the highest expression levels in patients with operational tolerance and were highly enriched in NK cells, suggesting that NK cells may play a crucial role in maintaining tolerance (92). Therefore, more research is needed to explore how IL-21 affects NK cell function and consequently influences liver transplant rejection.
3.4 T cells
T cells and B cells are the primary target cells of IL-21, and the expression of IL-21R on their cell membranes is upregulated following TCR and BCR signaling. As shown in Figure 3, we primarily discuss how IL-21 regulates the fate of CD4 T cell major subsets, CD8 T cells, and B cells in the context of organ transplantation.
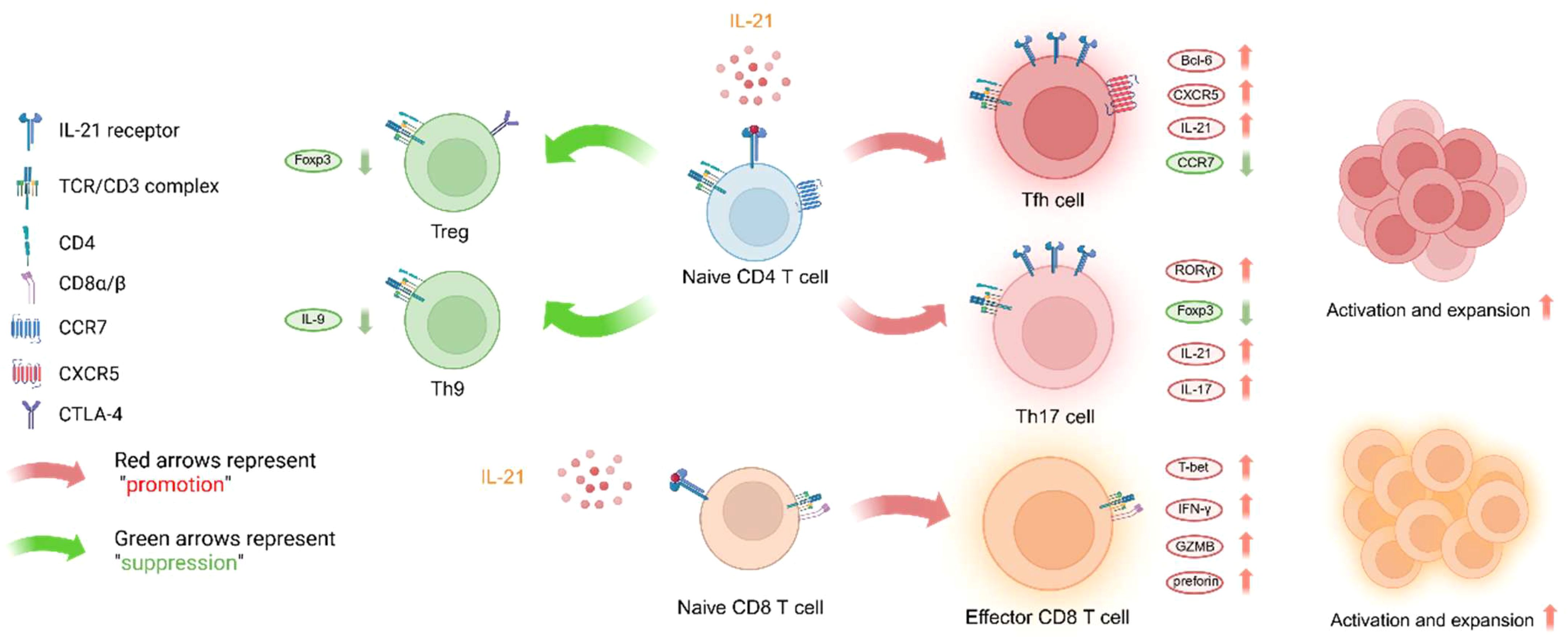
Figure 3. IL-21 influences T cell lineage differentiation and effector functions. Since there is limited research on the effect of IL-21 on Th22 effector function, this subset will not be discussed here. The figure was created with www.biorender.com.
3.4.1 Tfh cells
Naive CD4+ T cells expressing chemokine receptor 7 (CCR7) are attracted to the T cell zones of lymphoid organs by the concentration gradients of CCL19 and CCL21. Upon activation by dendritic cells, these T cells upregulate CXCR5 expression and downregulate CCR7, enabling them to migrate to the T-B cell boundary of lymphoid follicles. There, they interact with B cells that recognize antigenic proteins. These activated T cells are referred to as T follicular helper (Tfh) cells (93). Tfh cells play a critical role in aiding B cells to differentiate into plasma cells, making them essential for antibody production (94). IL-21 is an extremely important cytokine for the polarization of naive T cells into Tfh cells (21). Initially, IL-21 was considered indispensable for Tfh cell development (95, 96), but subsequent studies in animals with IL-21 signaling deficiencies also revealed the formation of Tfh cells (97, 98). Nevertheless, the absence of IL-21 signaling leads to reduced levels of Bcl-6 protein and a diminished proportion of Tfh cells, as Bcl-6 is a crucial transcription factor for the differentiation and function of Tfh cells (99, 100). Bcl-6 antagonizes the transcription factors that drive the differentiation of naive CD4+ T cells into Th1, Th2, and Th17 cells (101). IL-21 produced by Tfh cells can act in an autocrine manner to maintain Tfh differentiation and in a paracrine manner to act on germinal center (GC) B cells, promoting their growth, survival, and class-switch recombination (102). Tfh cells are key players in maintaining the GC response and selecting GC B cells; without Tfh cells, the GC will collapse (103). During homologous T: B cell interactions, Tfh cells provide essential co-stimulatory signals such as CD40L-CD40 and ICOS-ICOSL to B cells, which enable B cells to undergo class-switch recombination and affinity maturation. This leads to the rapid proliferation of B cells into antibody-secreting plasma cells and memory B cells (104), generating an effective humoral immune response, but also triggering AMR in organ transplantation.
Tfh cells, which produce IL-21, are core participants in driving antibody-mediated rejection AMR by promoting B cell differentiation, proliferation, and the production of DSA (105). AMR is currently the leading cause of kidney transplant failure (106). Significant increases in circulating Tfh cells and CD86+CD38+ B cells numbers and serum IL-21 levels were observed in kidney transplant patients with AMR, and these markers were positively correlated with serum creatinine levels (107). Compared to patients without DSA, those who developed DSA after kidney transplantation had higher pre-transplant plasma IL-21 levels and increased cTfh cells (108). Another study reported that kidney transplant patients with signs of chronic rejection had a significantly higher percentage of cTfh cells and lower PD-1 expression compared to stable patients (109). Tfh cells can co-localize with B cells to promote B cell effector functions in lymph nodes and kidney grafts (19, 107, 110), resulting in chronic graft injury. In chronic AMR patients, an increase in circulating Tfh cells (cTfh, particularly cTfh2 and cTfh17) producing IL-21 is observed in blood and kidney biopsies, while follicular regulatory T cells (Tfr) are reduced (111). In the presence of IL-6 and IL-21, studies using IL-21R antagonists in co-cultures with naive T cells show a significant reduction in the proportion of Tfh cells and an increase in Tfr cells (18). In fully mismatched mouse skin graft models, lower levels of circulating DSA and reduced inflammatory cytokine levels were observed. DSA can contribute to acute rejection by forming the membrane attack complex (MAC) and can also participate in chronic rejection by inducing endothelial cell activation (112, 113). Allograft vasculopathy can indicate the occurrence of AMR. In a murine heart transplantation study, the administration of IL-21 receptor fusion protein blocked the development of chronic allograft vasculopathy in wild-type recipients of B6-bm12 heart allografts, significantly prolonging graft survival (114). Studies using IL-21R antagonists in humanized mouse skin graft models found a significant reduction in the proportion of pSTAT3-positive CD4+ T cells, fewer infiltrating CD4+, CD8+, and CD20+ cells in grafts, and significantly lower levels of skin inflammation markers such as Ker17 and Ki-67 (115). In a study of liver transplant recipients, it was found that cTfh cells helped B cells differentiate into plasmablasts in an IL-21-dependent manner in vitro, suggesting that cTfh cells may participate in alloreactivity after liver transplantation by aiding B cell differentiation into plasmablasts and plasma cells (116). Another study indicated that cTfh2 is associated with acute graft rejection after liver transplantation, with higher proportions of B cells associated with cTfh2 and elevated serum IL-21 levels in patients with rejection compared to those without rejection (117). In lung transplantation, AMR is also thought to be related to graft dysfunction and chronic rejection, with DSA participating in the occurrence and development of obliterative bronchiolitis syndrome (10, 118, 119).
The first-line immunosuppressant used in clinical solid organ transplantation is tacrolimus. Previous reports have shown that adding tacrolimus to in vitro cell cultures does not inhibit the production of Tfh cells. Specifically, after tacrolimus treatment, the number of CD4+CXCR5+ Tfh cells was reduced by only 7% compared to the control group. Tacrolimus could only partially prevent Tfh activation (CD4+CXCR5+PD-1+ Tfh cells were reduced by 48%) and plasmablast differentiation (120). Another study reported that pre-treatment with tacrolimus one week before transplantation significantly reduced the levels of cTfh and lymph node Tfh cells, but had no significant effect on B cells, Tfr, or Treg cells (121). Following transplantation, under tacrolimus-based immunosuppressive therapy, the cTfh levels in patients remained relatively stable (116, 122). Despite partial functional impairment, these cells still retained the ability to assist B cell differentiation into plasma cells and antibody production. These findings suggest that current immunosuppressive regimens appear to have limited efficacy in combating AMR. Considering the critical role of IL-21 signaling in Tfh cells, targeting IL-21 as a new immunosuppressive approach may represent a promising strategy.
3.4.2 Th17 cells
Th17 cells are known for their high expression of IL-17, and the increased expression of IL-17 in local tissues is closely associated with allograft rejection in vivo (123, 124). In kidney transplantation, exposure to IL-17 leads to the production of inflammatory mediators by renal epithelial cells (125), which induces neutrophil recruitment and contributes to acute rejection. An imbalance between Th17/Treg cells results in severe renal interstitial and tubular damage (126). In an allogeneic rat liver transplantation model, strong expression of IL-17A, IL-6, TGF-β, IL-8, and myeloperoxidase (MPO) was observed in the grafts, and the proportion of Th17 cells in circulation significantly increased, indicating that Th17 cells contribute to liver allograft rejection in rats (127). Peripheral blood analysis of patients six months after liver transplantation showed that the Th17/Treg ratio in stable recipients was similar to pre-transplant values, while it was significantly elevated in patients with acute allograft rejection, suggesting that the Th17/Treg ratio may be a predictive factor for acute rejection (128).
Th17 cells are also a major source of IL-21, with their IL-21 mRNA and protein levels being five times higher than those of Th1 and Th2 cells (129). A study on kidney allografts from patients experiencing rejection found that Th17 cells accelerate graft destruction by promoting lymphocyte neogenesis through IL-21 production. Complement activation and high IL-21 expression were detected in grafts with high levels of Th17 infiltration (130). IL-21 plays a crucial role in Th17 effector functions, promoting CD4+ T cell proliferation and maintaining Th17 cell identity by upregulating RORγt and downregulating Foxp3, thereby inhibiting Treg differentiation (131–133). Th17 cells also assist B cell activation, facilitating the generation of antigen-specific B cells and promoting antibody class switching to IgG1, IgG2a, IgG2b, and IgG3 (134). In IL-21R knockout mice, Th17 frequencies decrease and the response is defective (129, 135).
The role of IL-21 in Th17 differentiation remains controversial. While both IL-21 and TGF-β are considered essential for human Th17 cell differentiation (136), studies in mice suggest otherwise. In murine models, IL-6, IL-1β, and TGF-β are the primary cytokines driving naïve CD4+ T cells toward the Th17 lineage, with IL-21 being dispensable. In IL-21 and IL-21R knockout mice, IL-6-induced Th17 differentiation occurs independently of IL-21 and is even stronger than IL-21-induced differentiation in vitro (137), These findings indicate that while IL-21 is not required for Th17 differentiation in mice, it remains a critical factor for Th17 cell function.
3.4.3 Treg cells
As mentioned earlier, IL-21 can downregulate the expression of Foxp3 in CD4 T cells, and Foxp3 is one of the important transcription factors and markers for Treg differentiation. Therefore, IL-21 can directly impair Treg homeostasis. In addition, IL-21 inhibits the production of IL-2 in T cells. Although conventional T cell responses are not impaired because IL-21 can substitute for IL-2 as a T cell growth factor, IL-21 cannot replace IL-2 in supporting the Treg compartment. Thus, IL-21 signaling in conventional T cells can indirectly affect Treg homeostasis by reducing the availability of IL-2 (138).
In organ transplantation, Treg cells are generally considered beneficial for promoting transplant tolerance. Treg cells secrete CD25, which depletes IL-2 availability in other lymphocytes, thereby inhibiting the survival of surrounding T cells (139). Additionally, they can suppress effector T cell activation by competing with CD28 for binding to CD80/CD86 through their surface immune checkpoint molecule CTLA-4. Alessandra et al. reported that IL-21 signaling enhanced T cell signaling and mixed lymphocyte responses in T cells treated with IL-21 pOrf plasmid and stimulated with anti-CD3/anti-CD28, suppressed Treg generation and function, and observed strong immune tolerance in a mouse islet transplant model after IL-21R blocking agent and CTLA-4 globulin treatment (140). The study by Yeqi Nian and colleagues (18) showed that treatment with an IL-21R antagonist in mice after allogeneic skin transplantation significantly reduced the Tfh/Tfr ratio in their splenocytes, thereby lowering the levels of donor-specific IgG antibodies and significantly inhibiting AMR. Christoph Bucher et al. (141)reported that IL-21 inhibited the differentiation of inducible Tregs and further suggested that IL-21 blockade is a promising target to reduce graft-versus-host disease (GVHD) damage. The maturation of dendritic cells in the liver is much lower than in peripheral lymphoid organs, manifested by low expression of MHC-II, CD80/CD86, and high expression of TGF-β, which favors the expansion and functional maintenance of Tregs (142–144). In patients without rejection, the Treg population gradually returns to normal levels over time, whereas in patients with acute rejection, it remains at lower levels (145), suggesting that Tregs are associated with the fate of allogeneic liver grafts. Therefore, from the perspective of Tregs, IL-21 is an anti-tolerance cytokine antagonizes Treg-induced immune tolerance.
3.4.4 Th1 and Th2 cells
Since Th1 and Th2 cells are the earliest classified CD4 T cell subpopulations and antagonize each other in driving naive CD4 T cell differentiation toward the opposing lineage (146), this section discusses both subpopulations together. Early studies reported that IL-21 can upregulate the transcription of Th1-related genes and promote Th1 responses (147), which is understandable because IL-21 is a strong activator of STAT1, which in turn upregulates the Th1-related transcription factor T-bet. However, later studies indicated that IL-21 is a Th2 cytokine, which specifically inhibits the production of IFN-γ by Th1 cells and amplifies the Th2 response (148). Additionally, contradictory reports regarding IL-21’s role in Th2 responses have emerged. One study stated that in an OVA-induced airway inflammation model, IL-21 receptor signaling is an indispensable part of the development of Th2 effector responses, mediating Th2 cell survival or migration to peripheral tissues. However, IL-21R signaling is not required to control the development of severe Leishmania infection, suggesting it may be redundant in Th1 responses (149). Another study in the same OVA-induced airway inflammation model showed that IL-21 reduced cytokine production polarizing toward Th2 and induced Th2 cell apoptosis by downregulating Bcl-2 (150). It is well known that Th2 cells primarily contribute to the development of parasitic infections and allergic diseases, in which IL-21 plays a dual role. IL-21 can promote allergic airway inflammation by driving apoptosis of FoxP3(+) regulatory T cells (151), but it can also suppress IL-4-mediated IgE secretion (152), further exemplifying IL-21’s multifunctionality.
It is widely acknowledged that Th1 responses dominate in solid organ transplants. Various inflammatory cytokines produced by Th1 cells, such as TNF-α and IFN-γ, are hallmark markers of transplant rejection and can mediate target tissue damage (153). Considering that IL-21 primarily participates in regulating the fate of Tfh and Th17/Treg cells, but not as a definitive cytokine for Th1/Th2 lineage differentiation, this section does not further discuss it.
3.4.5 Th9 cells
Th9 cells are defined by the secretion of IL-9 (154) and primarily exert effects in parasitic infections, allergic reactions, and certain autoimmune diseases. It has been shown that IL-2-JAK3-STAT5 signaling is essential for Th9 differentiation, while IL-21 inhibits Th9 differentiation. This difference is mediated by Bcl-6, whose increased expression suppresses IL-9 production (155). An early study (156) had shown that IL-21 could support the polarization of human Th9 cells in the presence of IL-4 and TGF-β, which indicated the effect of IL-21 on Th9 differentiation may exhibit species-specific differences.
To some extent, Th9 cells are believed to induce immune tolerance, which is beneficial in transplantation. IL-9 secreted by Th9 cells can recruit mast cells and promote their proliferation. Mast cells can negatively regulate immune responses through surface co-inhibitory molecules and secretion of immunosuppressive factors, such as IL-4 and IL-10, to maintain immune homeostasis (157). Additionally, mast cells are essential in Treg-dependent peripheral immune tolerance, and neutralization of IL-9 significantly accelerates allograft rejection in mice (158). Current research findings do not support the involvement of Th9 and IL-9 in kidney transplant rejection. No detectable IL-9 gene expression has been observed in allogeneic kidney transplant tissues. Additionally, a study on kidney transplantation in rats reported that IL-9 is expressed in normal kidneys but is nearly undetectable during rejection, while IL-9Rα is barely expressed in normal kidney tissue (159). A similar conclusion was drawn in a mouse heart transplantation model. L.F. Poulin et al. observed that allogeneic heart grafts were rejected within nine days in both wild-type and IL-9 knockout mice (160). In a study by E. Fábrega and colleagues on 30 liver transplant recipients who had not experienced rejection for 8 years, their serum IL-9 levels were significantly higher than those of healthy controls (n=30) (161). They then conducted another study dividing 50 liver transplant recipients into two groups: Group I, consisting of 15 patients with acute rejection, and Group II, consisting of 35 patients without acute rejection. On postoperative days 1 and 7, there were no significant differences in serum IL-9 levels between Group I and Group II. However, the serum IL-9 levels of all 50 liver transplant recipients remained higher than those of healthy controls (n=34), suggesting that IL-9 may be only minimally involved in acute liver transplant rejection (162).
3.4.6 CD8 T cells
It is well-known that CD8 T cells play a crucial role in anti-tumor immunity, and IL-21 can effectively enhance this effect (163). However, CD8 T cell-mediated rejection (TCMR) is a key feature of acute rejection after allogeneic transplantation. Naïve CD8 T cells recognize alloantigens presented on MHC class I molecules by APCs (antigen-presenting cells) in secondary lymphoid organs. Upon co-stimulation with APCs, they become activated, exit the secondary lymphoid organs, enter the circulation, and migrate to local tissues to eliminate antigen-expressing target cells, resulting in graft injury. The degree of CD8 T cell infiltration into the graft is often considered a measure of the severity of acute rejection (164–166). Previous studies have reported that using spleen cells from IL-21R knockout mice significantly reduced the incidence and mortality of GVHD (graft-versus-host disease) after bone marrow transplantation (167). In addition, in a study on xenogeneic graft-versus-host disease (X-GVHD) (168), the authors found that in mice treated with IL-21 monoclonal antibody after receiving human PBMCs, the number of CD8 T cells in peripheral blood and spleen decreased, while the number and proportion of Treg cells in the spleen increased. This suggests that IL-21 blockade may be an attractive strategy in X-GVHD. Vinh Nguyen et al. (169) later used IL-21R-deficient donor T cells to induce acute GVHD and found a reduction in peak donor CD8 T cell numbers and a decrease in CTL effector functions. This further suggested that effector CTL maturation depends on IL-21R signaling in antigen-specific donor CD8 T cells. Memory T cells, due to their lower activation threshold, can respond rapidly upon reencounter with the antigen, mediating acute rejection. Overexpression of IL-21 has been shown to promote the accumulation of a large number of CD8^+ memory T cells, reducing the proportion of naïve T cells (170, 171).
IL-21 promotes CD8 CTL (cytotoxic T lymphocyte) activity through the transcription factor T-bet (172), making it an important signal for CD8 T cell expansion and effector functions (173, 174). The expression of IL-21R on the surface of CD8 T cells enhances their activation (175). In an allogeneic mixed lymphocyte reaction, IL-21 was observed to effectively expand both memory (CD44^high) and naïve (CD44^low) CD8 T cells, increasing IFN-γ production in vitro. However, in IL-21R knockout mice, CD8 T cell expansion and cytotoxicity were impaired (176). During chronic viral infections, IL-21 derived from Tfh cells maintains the effector CD8 T cell response (177, 178).
3.5 B cells
IL-21 has a significant impact on the fate of germinal centers (GCs) and B cells. Germinal centers (GCs) are specialized anatomical regions within lymphoid organs where activated B cells undergo high-frequency somatic mutations, antibody affinity maturation, rapid proliferation, and produce memory B cells and long-lived antibody-secreting plasma cells. IL-21 signaling promotes the formation and maintenance of GCs. IL-21 enhances the synergistic induction of c-MYC and phosphorylated ribosomal protein S6 by B cell receptor (BCR) and CD40 (179–181). Both c-MYC and S6 are part of the mTOR pathway, a core pathway for cell growth and metabolism that is essential for GC B cells to respond effectively and support their rapid and extensive clonal expansion (182). As shown in Figure 4, IL-21 signaling significantly increases and maintains the expression of the key transcriptional regulator of GC B cells, Bcl-6 (97, 98, 184). Bcl-6 is indispensable for GC formation, and GC B cells lacking Bcl-6 cannot migrate to the lymphoid follicle center to differentiate into long-lived plasma cells (185). Although GCs can form without IL-21 signaling, mice deficient in IL-21 or IL-21R expression have defective GC structures, reduced numbers of GC B cells (98), and decreased B cell proliferation (18, 186), while plasma cell differentiation requires extensive B cell clonal expansion (187). GC B cells undergo AID-mediated somatic hypermutation, and only those B cells with high affinity are positively selected, then differentiate into plasma cells with the help of Tfh cells. IL-21 has been identified as a key differentiation factor for plasma cells derived from GCs and promotes the expression of the plasma cell master transcription factor B lymphocyte-induced maturation protein 1 (BLIMP1) (97, 186, 188). BLIMP1 further suppresses the expression of genes such as AID, Bcl-6, PAX5, and c-Myc, ultimately terminating the GC program and positively regulating XBP1 expression to prepare B cells for antibody production and secretion (189). IL-21 induction of Bcl-6 and Blimp-1 expression appears to be contradictory, in fact, IL-21 exerts a spatiotemporal effect on B cells, making its regulation of these factors dynamic. Initially, IL-21 activates STAT3, enhancing the expression of Bcl-6, which supports the proliferation of GC B cells. At this stage, Bcl-6 dominates, allowing GC B cells to continue proliferating and undergo somatic hypermutation in the germinal center. As the immune response progresses, IL-21 drives B cell differentiation into plasma cells through STAT3-mediated upregulation of Blimp-1. In the later stages of the immune response, IL-21 induces Blimp-1, promoting the differentiation of high-affinity B cells into long-lived plasma cells or memory B cells, ensuring long-term immune protection. Additionally, IL-21 signaling upregulates the expression of CD86 on the surface of B cells (190), further enhancing T:B co-stimulatory signals. Interestingly, in the absence of BCR signaling and T cell co-stimulation, IL-21 stimulation can induce B cell apoptosis, which is thought to be related to the elimination of self-reactive B cells (191). Moreover, Breg cells, a subset of B cells with immunoregulatory functions, have recently been found to develop and expand in response to IL-21 and CD40 signaling, specifically driving the development of the B10 subset (IL-10 secreting Breg cells) (192), a function that has been subsequently confirmed in research (193, 194).
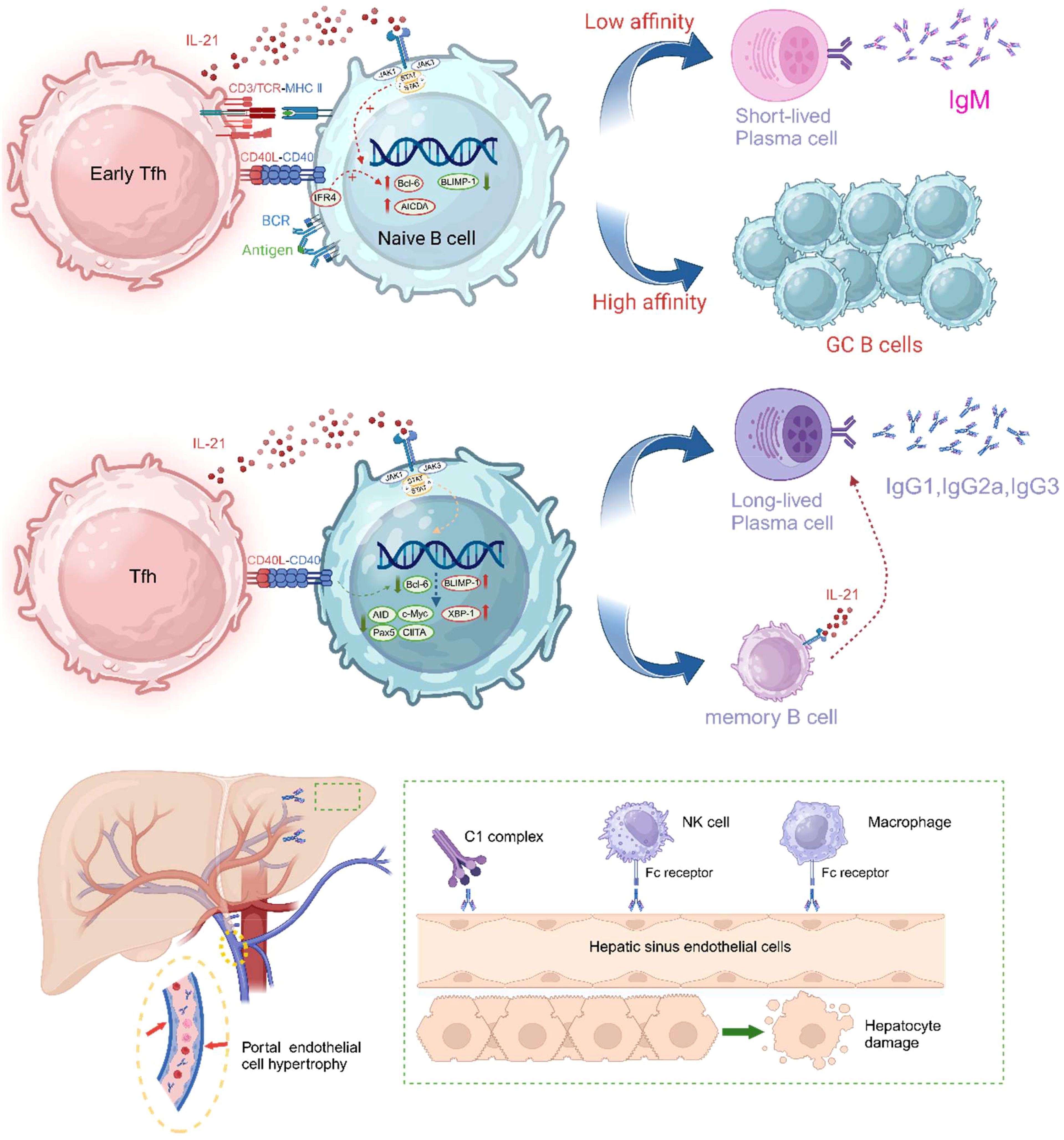
Figure 4. IL-21 promotes the formation and maintenance of germinal centers and plays a decisive role in the differentiation of GC-derived B cells into plasma cells. The CD40L-CD40 signaling pathway has been shown to work synergistically with IL-21 to promote Blimp-1 activation and plasma cell differentiation. CD40 signaling enhances STAT3-driven Blimp-1 upregulation by reducing Bcl-6 expression. Other co-stimulatory signals, such as ICOS-ICOSL and CD28-CD80/86, also promote plasma cell differentiation. Subsequently, DSA can injure endothelial cells by activating the complement system or through ADCC (antibody-dependent cellular cytotoxicity). In liver transplant patients experiencing AMR, hypertrophy of portal vein endothelial cells, obstructive portal vein disease, and microvasculitis are commonly observed. The drawing was inspired in part by Aldo J. Montano-Loza et al. (183). The figure was created with www.biorender.com.
In solid organ transplantation, alloreactive B cells are the direct source of DSA. Combined with the previously discussed role of Tfh cells in AMR, the importance of IL-21 in the pathophysiology of AMR is self-evident. Kitty de Leur et al. reported that IL-21R blockade inhibited plasma cell differentiation, significantly reducing levels of IgM and IgG2 (195). Nian, Y et al. verified in a fully mismatched mouse skin transplant model that IL-21R blockade induced defects in germinal center structures and reduced DSA production (18). Van Besouw NM et al. suggested that the number of IL-21 producing donor-specific cells before and after transplantation could predict renal transplant rejection (196). In a study of serum cytokines in liver transplant patients, it was found that in the group with acute rejection, IL-21 mRNA expression patterns and serum IL-21 levels showed a steady increase, suggesting that IL-21 exhibits pro-inflammatory characteristics in liver transplant rejection patients (197).
Tacrolimus has limited effects on B cell proliferation and antibody production, whereas mycophenolic acid and rapamycin are more potent in this regard (198). Therefore, targeting the IL-21 signaling pathway may compensate for tacrolimus’ insufficiency in suppressing AMR. Some research teams have explored strategies to enhance tacrolimus-based immunosuppression by targeting downstream signaling pathways involved in T cell and B cell activation. Park JS et al. (199)reported that combining a STAT3 inhibitor with tacrolimus increased Treg numbers in lupus-susceptible mouse spleen cells while decreasing the proportions of GC B cells and plasma cells. Tobias Deuse et al. (200) demonstrated that the JAK1/JAK3 inhibitor R507, when combined with tacrolimus, had a synergistic effect, significantly prolonging the survival of allogeneic heart transplants compared to tacrolimus alone and reducing donor-specific IgM levels. While these studies do not specifically target IL-21 signaling, they highlight the potential of modulating JAK-STAT pathways to improve immunosuppressive efficacy. Given that IL-21 signals predominantly through JAK1 and STAT3, direct blockade of the IL-21 pathway may represent an additional avenue for combination therapy to enhance tacrolimus’ efficacy in preventing AMR.
4 Clinical trials related to IL-21
Clinical studies involving the IL-21 signaling pathway as an intervention in the field of organ transplantation are relatively limited, but significant work has been done in the fields of autoimmune diseases and cancer immunotherapy. The safety and tolerability of NNC0114-0006 in SLE patients were evaluated in a Phase I trial (NCT01689025), with researchers aiming to assess the incidence of adverse events (AEs) and pharmacokinetic data (201). Unfortunately, this clinical study was forced to terminate due to the enrollment of only 10 participants. ATR-107 is a fully humanized monoclonal antibody targeting IL-21R (202). Its pharmacodynamic studies have shown positive results. Intravenous administration of 60mg or 120mg can maximize the occupancy of IL-21 receptors, and this occupancy effect can persist for up to 42 days after a single dose. Unfortunately, 76% (35 out of 46) of the subjects receiving ATR-107 developed anti-ATR-107 antibodies (ADA). These ADAs were detected between 42- and 105-days post-administration. The high immunogenicity of ATR-107 is considered a major obstacle in its drug development. NNC01140006 is a monoclonal antibody targeting IL-21. Previous safety trials indicated that it was well tolerated in healthy subjects and RA patients, with initial signs of reduced RA activity observed in the 25 mg/kg dose group (203). Subsequently, a multicenter, randomized, parallel-group, placebo-controlled, double-blind, phase 2 clinical trial aimed at evaluating the protective effect of NNC01140006 combined with liraglutide on β-cell function in adults with recent-onset type 1 diabetes showed that at week 54, the MMTT-stimulated C-peptide concentration in the combination treatment group decreased by 10% (ratio to baseline 0.90), significantly better than the 39% decrease in the placebo group (ratio 0.61). The decrease in all active treatment groups at week 54 (approximately 0.50 percentage points) was greater than in the placebo group (0.10 percentage points), but the difference was not statistically significant. No adverse events related to the trial treatment were observed throughout the trial. This suggests that the combination of anti-IL-21 antibody and liraglutide may help protect β-cell function in adults with recent-onset type 1 diabetes, with good safety, and warrant further evaluation (204). BOS161721 is a humanized IgG1 monoclonal antibody with three mutations (M252Y/S254T/T256E) that inhibits IL-21 bioactivity. It has been shown to be well-tolerated at various doses, dose-dependently inhibiting IL-21-induced pSTAT3, and reversing the downregulation of genes crucial for IL-21-induced tolerance and T-cell exhaustion (205). The subsequent clinical trial (206) investigating the efficacy of BOS161721 in SLE patients has been completed. However, based on the data currently provided by the researchers, the trial results do not appear to be particularly promising. There was no significant difference in the SRI-4 response, which is associated with improved outcomes, between the placebo group and the BOS161721 120 mg treatment group (hereinafter referred to as the treatment group) (51.4% vs. 53.3%, p=0.8434). However, the difference between the placebo group and the treatment group was significant in terms of the absence of new severe disease activity (73.0% vs. 90.7%, p=0.0141). Therefore, further trials may be necessary to validate the efficacy of BOS161721. In patients with metastatic melanoma and renal cell carcinoma, intravenous infusion of recombinant human IL-21 resulted in upregulation of perforin and granzyme B mRNA levels in CD8 T cells and NK cells (207). A Phase II study in metastatic melanoma patients confirmed the biological activity of IL-21, with 9 of 40 patients showing partial remission and 16 showing stable disease (208). Another study on metastatic melanoma patients showed that IL-21-induced polyclonal CTL, in combination with CTLA4 blockade, controlled refractory metastatic melanoma. This combination therapy achieved durable complete remission in patients where both monoclonal CTL and anti-CTLA4 therapy had previously failed (209). When recombinant human IL-21 was used in combination with the monoclonal antibody cetuximab, which targets the epidermal growth factor receptor, enhanced anti-tumor activity was observed, with serum sCD25 levels increasing in a dose-dependent manner, and 60% of patients achieving stable disease (210). While recombinant IL-21 has been investigated in cancer patients to enhance anti-tumor immunity, in transplantation, IL-21 is more likely to contribute to allograft rejection. Therefore, in contrast to oncology settings where IL-21 administration is beneficial, transplantation research is supposed to focus on IL-21 blockade as a potential immunosuppressive strategy. These tumor-related clinical studies have demonstrated that IL-21 can activate CD8+ T cells and NK cells (upregulating perforin and granzyme B) and induce polyclonal CTL responses, a mechanism similar to IL-21-driven alloreactive T cell activation in transplant rejection. Therefore, researchers focusing on transplant immunology may find inspiration from the interventions used in these clinical studies. For instance, this work (209) showed promising results with exogenous IL-21 combined with CTLA-4 blockade therapy. This naturally raises the question of whether combining IL-21 blockade with a CTLA-4-related drug already used in transplantation, such as Belatacept (Previous work (120) had shown that Belatacept has a limited effect in the generation of both Tfh cells and donor antigen-driven plasmablast), could yield more favorable results in suppressing transplant rejection. Although this idea has been validated by researchers in a murine allogeneic islet transplantation model with positive results (140), more data are still needed for support. We believe it could serve as similar inspiration for readers.
5 Conclusion
IL-21 plays a crucial role in transplant rejection as a key immunomodulatory factor. This review comprehensively discusses the IL-21 signaling pathway, its role in immune cell function regulation, and how these immune cells contribute to the pathophysiology of transplant rejection. IL-21 is not only a critical factor for the development and functional maintenance of Tfh and Th17 cells but also plays a central role in the regulation of immune effector cells such as B cells and NK cells. Its role in transplant immune responses is complex and multifaceted.
The biological function of IL-21 is mediated by its receptor, IL-21R, and it affects target cells through the JAK/STAT signaling pathway. IL-21 activates STAT1, STAT3, and other signaling pathways to induce the activation and expansion of CD8 T cells, enhancing their cytotoxicity. Similar effects are observed in NK cells, enhancing their immune surveillance of transplant tissues, thereby participating in acute transplant rejection. Additionally, IL-21 is essential for the differentiation and maintenance of CD4 T cells, particularly Tfh and Th17 cells, which play significant roles in AMR. IL-21 influences B cells by promoting the clonal expansion of germinal center B cells and is indispensable for the terminal differentiation of B cells into plasma cells. Therefore, IL-21 not only exacerbates T cell-mediated rejection (TCMR) through its effects on cellular immune responses but also drives AMR by enhancing humoral immune responses.
The role of IL-21 in transplant immunity suggests that it may become a potential therapeutic target. Interventions targeting the IL-21 signaling pathway, such as using IL-21R antagonists or IL-21 antibodies, may provide new strategies for treating transplant rejection. Such interventions could inhibit excessive immune responses, reduce the incidence of rejection, and promote transplant organ tolerance. Furthermore, existing immunosuppressive protocols, such as those based on tacrolimus, seem limited in their effect on AMR. Therefore, combining tacrolimus with IL-21 signaling blockade may be a promising therapeutic approach.
In summary, IL-21 plays a multifaceted role in transplant rejection. The complexity of the IL-21 signaling pathway offers an opportunity for in-depth research on immune regulation. Further studies will help elucidate its specific role in transplant immunity and drive the development of IL-21-targeted immunotherapy strategies. In the future, therapies targeting IL-21 signaling may offer new directions for improving long-term transplant organ tolerance and enhancing the clinical outcomes of transplantation.
Author contributions
XZ: Writing – original draft. YP: Writing – original draft. QX: Supervision, Writing – review & editing. KH: Supervision, Writing – review & editing.
Funding
The author(s) declare that no financial support was received for the research and/or publication of this article.
Conflict of interest
The authors declare that the research was conducted in the absence of any commercial or financial relationships that could be construed as a potential conflict of interest.
Generative AI statement
The author(s) declare that no Generative AI was used in the creation of this manuscript.
Publisher’s note
All claims expressed in this article are solely those of the authors and do not necessarily represent those of their affiliated organizations, or those of the publisher, the editors and the reviewers. Any product that may be evaluated in this article, or claim that may be made by its manufacturer, is not guaranteed or endorsed by the publisher.
Abbreviations
IL-21, Interleukin-21; Tfh cells, Follicular helper T; Th17, T helper 17; MHC, Major Histocompatibility Complex; NK cells, Natural Killer cells; AMR, Antibody Mediated Rejection; ROCK2, Rho Associated Coiled Coil Containing Protein Kinase 2; NFAT, Nuclear Factor of Activated T-cells; ICOS, Inducible Costimulator; IFN-γ, Interferon-gamma; c-Maf, Cellular Maf; JAK, Janus Kinase; STAT, Signal Transducers and Activators of Transcription; NF-κB, Nuclear Factor Kappa-light-chain-enhancer of activated B cells; LPS, Lipopolysaccharide; TLR4, Toll-like receptor 4; TAM, Tumor-Associated Macrophage; IRI, Ischemia-Reperfusion Injury; APC, Antigen-Presenting Cell; DC, Dendritic Cells; CCR, C-C chemokine Receptor 7; SLO, Secondary Lymphoid Organs; TCR, T Cell Receptor; DSA, Donor-Specific Antibody; GC, Germinal Centre; Bcl-6, B-cell lymphoma 6; BLIMP-1, B Lymphocyte-Induced Maturation Protein 1; RORγt, Retinoic Acid Receptor-Related Orphan Receptor Gamma t; Treg, Regulatory T Cell; TGF-β, Transforming Growth Factor Beta; CTLA-4, Cytotoxic T-Lymphocyte Antigen 4; GVHD, Graft-Versus-Host Disease; Foxp3, Forkhead Box P3; c-Myc, Cellular Myelocytomatosis Oncogene; XBP-1, X-box Binding Protein 1; PAX5, Paired Box 5; ADCC, Antibody-Dependent Cellular Cytotoxicity).
References
1. Ji W, Chen J, Mi Y, Wang G, Xu X, Wang W. Role of natural killer cells in liver transplantation treatment of liver cancer. Exp Ther Med. (2017) 14:2380–4. doi: 10.3892/etm.2017.4748
2. Kummer JA, Wever PC, Kamp AM, ten Berge IJ, Hack CE, Weening JJ. Expression of granzyme A and B proteins by cytotoxic lymphocytes involved in acute renal allograft rejection. Kidney Int. (1995) 47:70–7. doi: 10.1038/ki.1995.8
3. Pennathur S, Pasichnyk K, Bahrami NM, Zeng L, Febbraio M, Yamaguchi I, et al. The macrophage phagocytic receptor CD36 promotes fibrogenic pathways on removal of apoptotic cells during chronic kidney injury. Am J Pathol. (2015) 185:2232–45. doi: 10.1016/j.ajpath.2015.04.016
4. Wu H, Xu X, Li J, Gong J, Li M. TIM−4 blockade of KCs combined with exogenous TGF−β injection helps to reverse acute rejection and prolong the survival rate of mice receiving liver allografts. Int J Mol Med. (2018) 42:346–58. doi: 10.3892/ijmm.2018.3606
5. Kloc M, Uosef A, Kubiak JZ, Ghobrial RM. Macrophage proinflammatory responses to microorganisms and transplanted organs. Int J Mol Sci. (2020) 21:9669. doi: 10.3390/ijms21249669
6. Le Moine A, Goldman M, Abramowicz D. Multiple pathways to allograft rejection. Transplantation. (2002) 73:1373–81. doi: 10.1097/00007890-200205150-00001
7. Rocha PN, Plumb TJ, Crowley SD, Coffman TM. Effector mechanisms in transplant rejection. Immunol Rev. (2003) 196:51–64. doi: 10.1046/j.1600-065X.2003.00090.x
8. Demetris AJ, Bellamy C, Hübscher S, O’leary J, Randhawa P, Feng S, et al. 2016 comprehensive update of the Banff working group on liver allograft pathology: introduction of antibody-mediated rejection. Am J Transplant. (2016) 16:2816–35. doi: 10.1111/ajt.13909
9. O'Leary JG, Michelle Shiller S, Bellamy C, Nalesnik MA, Kaneku H, Jennings LW, et al. Acute liver allograft antibody-mediated rejection: an inter-institutional study of significant histopathological features. Liver Transpl. (2014) 20:1244–55. doi: 10.1002/lt.23948
10. Levine DJ, Glanville AR, Aboyoun C, Belperio J, Benden C, Berry GJ, et al. Antibody-mediated rejection of the lung: a consensus report of the International Society for Heart and Lung Transplantation. J Heart Lung Transplant. (2016) 35:397–406. doi: 10.1016/j.healun.2016.01.1223
11. von der Thüsen JH, Vandermeulen E, Vos R, Weynand B, Verbeken EK, Verleden SE. The histomorphological spectrum of restrictive chronic lung allograft dysfunction and implications for prognosis. Mod Pathol. (2018) 31:780–90. doi: 10.1038/modpathol.2017.180
12. Wallace WD, Li N, Andersen CB, Arrossi AV, Askar M, Berry GJ, et al. Banff study of pathologic changes in lung allograft biopsy specimens with donor-specific antibodies. J Heart Lung Transplant. (2016) 35:40–8. doi: 10.1016/j.healun.2015.08.021
13. Corthay A. A three-cell model for activation of naive T helper cells. Scand J Immunol. (2006) 64:93–6. doi: 10.1111/j.1365-3083.2006.01782.x
14. Bailly E, Macedo C, Ossart J, Louis K, Gu X, Ramaswami B, et al. Interleukin-21 promotes Type-1 activation and cytotoxicity of CD56dimCD16bright natural killer cells during kidney allograft antibody–mediated rejection showing a new link between adaptive and innate humoral allo-immunity. Kidney Int. (2023) 104:707–23. doi: 10.1016/j.kint.2023.04.024
15. Guo L, Lv J, Zhang J, Deng H, Feng S, Liu S, et al. Elevated serum IL-21 levels are associated with stable immune status in kidney transplant recipients and a mouse model of kidney transplantation. Aging (Albany N Y.). (2020) 12:18396. doi: 10.18632/aging.103713
16. Issa F, Cross AR. IL–21-producing alloreactive T cells expand in parallel to donor-specific antibodies. Transplantation. (2023) 107:1019–20. doi: 10.1097/TP.0000000000004492
17. Mendoza Rojas A, van Gelder T, de Kuiper R, Reijerkerk D, Clahsen-van Groningen MC, Hesselink DA, et al. Pre-transplant donor-reactive IL-21 producing T cells as a tool to identify an increased risk for acute rejection. Sci Rep. (2021) 11:12445. doi: 10.1038/s41598-021-91967-w
18. Nian Y, Xiong Z, Zhan P, Wang Z, Xu Y, Wei J, et al. IL-21 receptor blockade shifts the follicular T cell balance and reduces de novo donor-specific antibody generation. Front Immunol. (2021) 12:661580. doi: 10.3389/fimmu.2021.661580
19. Zhang H, Cavazzoni CB, Podestà MA, Bechu ED, Ralli G, Chandrakar P, et al. IL-21–producing effector Tfh cells promote B cell alloimmunity in lymph nodes and kidney allografts. JCI insight 8. (2023) 8(20):e169793. doi: 10.1172/jci.insight.169793
20. Kasaian MT, Whitters MJ, Carter LL, Lowe LD, Jussif JM, Deng B, et al. IL-21 limits NK cell responses and promotes antigen-specific T cell activation: a mediator of the transition from innate to adaptive immunity. Immunity. (2002) 16:559–69. doi: 10.1016/S1074-7613(02)00295-9
21. Eto D, Lao C, DiToro D, Barnett B, Escobar TC, Kageyama R, et al. IL-21 and IL-6 are critical for different aspects of B cell immunity and redundantly induce optimal follicular helper CD4 T cell (Tfh) differentiation. PloS One. (2011) 6:e17739. doi: 10.1371/journal.pone.0017739
22. Mesin L, Ersching J, Victora GD. Germinal center B cell dynamics. Immunity. (2016) 45:471–82. doi: 10.1016/j.immuni.2016.09.001
23. Nutt SL, Hodgkin PD, Tarlinton DM, Corcoran LM. The generation of antibody-secreting plasma cells. Nat Rev Immunol. (2015) 15:160–71. doi: 10.1038/nri3795
24. Baan CC, Balk AH, Dijke IE, Korevaar SS, Peeters AM, de Kuiper RP, et al. Interleukin-21: an interleukin-2 dependent player in rejection processes. Transplantation. (2007) 83:1485–92. doi: 10.1097/01.tp.0000264998.23349.54
25. Hecker A, Kaufmann A, Hecker M, Padberg W, Grau V. Expression of interleukin-21, interleukin-21 receptor alpha and related type I cytokines by intravascular graft leukocytes during acute renal allograft rejection. Immunobiology. (2009) 214:41–9. doi: 10.1016/j.imbio.2008.04.004
26. Miyamoto E, Vosoughi D, Wang J, Al-Refaee J, Berra G, Daigneault T, et al. Local intragraft humoral immune responses in chronic lung allograft dysfunction. J Heart Lung Transplant. (2025) 44:105–17. doi: 10.1016/j.healun.2024.07.019
27. Ozaki K, Kikly K, Michalovich D, Young PR, Leonard WJ. Cloning of a type I cytokine receptor most related to the IL-2 receptor β chain. Proc Natl Acad Sci. (2000) 97:11439–44. doi: 10.1073/pnas.200360997
28. Parrish-Novak J, Dillon SR, Nelson A, Hammond A, Sprecher C, Gross JA, et al. Interleukin 21 and its receptor are involved in NK cell expansion and regulation of lymphocyte function. Nature. (2000) 408:57–63. doi: 10.1038/35040504
29. Rao DA, Gurish MF, Marshall JL, Slowikowski K, Fonseka CY, Liu Y, et al. Pathologically expanded peripheral T helper cell subset drives B cells in rheumatoid arthritis. Nature. (2017) 542:110–4. doi: 10.1038/nature20810
30. Huber M, Brüstle A, Reinhard K, Guralnik A, Walter G, Mahiny A, et al. IRF4 is essential for IL-21-mediated induction, amplification, and stabilization of the Th17 phenotype. Proc Natl Acad Sci. (2008) 105:20846–51. doi: 10.1073/pnas.0809077106
31. Kim H-P, Korn LL, Gamero AM, Leonard WJ. Calcium-dependent activation of interleukin-21 gene expression in T cells. J Biol Chem. (2005) 280:25291–7. doi: 10.1074/jbc.M501459200
32. Penel-Sotirakis K, Simonazzi E, Peguet-Navarro J, Rozieres A. Differential capacity of human skin dendritic cells to polarize CD4+ T cells into IL-17, IL-21 and IL-22 producing cells. PloS One. (2012) 7:e45680. doi: 10.1371/journal.pone.0045680
33. Morita R, Schmitt N, Bentebibel S-E, Ranganathan R, Bourdery L, Zurawski G, et al. Human blood CXCR5+ CD4+ T cells are counterparts of T follicular cells and contain specific subsets that differentially support antibody secretion. Immunity. (2011) 34:108–21. doi: 10.1016/j.immuni.2010.12.012
34. Dardalhon V, Awasthi A, Kwon H, Galileos G, Gao W, Sobel RA, et al. IL-4 inhibits TGF-β-induced Foxp3+ T cells and, together with TGF-β, generates IL-9+ IL-10+ Foxp3– effector T cells. Nat Immunol. (2008) 9:1347–55. doi: 10.1038/ni.1677
35. Crotty S. T follicular helper cell biology: a decade of discovery and diseases. Immunity. (2019) 50:1132–48. doi: 10.1016/j.immuni.2019.04.011
36. Ma CS, Suryani S, Avery DT, Chan A, Nanan R, Santner-Nanan B, et al. Early commitment of naïve human CD4+ T cells to the T follicular helper (TFH) cell lineage is induced by IL-12. Immunol Cell Biol. (2009) 87:590–600. doi: 10.1038/icb.2009.64
37. Chaurio RA, Anadon CM, Costich TL, Payne KK, Biswas S, Harro CM, et al. TGF-β-mediated silencing of genomic organizer SATB1 promotes Tfh cell differentiation and formation of intra-tumoral tertiary lymphoid structures. Immunity. (2022) 55:115–28. doi: 10.1016/j.immuni.2021.12.007
38. Hiramatsu Y, Suto A, Kashiwakuma D, Kanari H, Kagami S-I, Ikeda K, et al. c-Maf activates the promoter and enhancer of the IL-21 gene, and TGF-β inhibits c-Maf-induced IL-21 production in CD4+ T cells. J leukocyte Biol. (2010) 87:703–12. doi: 10.1189/jlb.0909639
39. Davis ID, Brady B, Kefford RF, Millward M, Cebon J, Skrumsager BK, et al. Clinical and biological efficacy of recombinant human interleukin-21 in patients with stage IV Malignant melanoma without prior treatment: a phase IIa trial. Clin Cancer Res. (2009) 15:2123–9. doi: 10.1158/1078-0432.CCR-08-2663
40. Spolski R, Leonard WJ. Interleukin-21: basic biology and implications for cancer and autoimmunity. Annu Rev Immunol. (2008) 26:57–79. doi: 10.1146/annurev.immunol.26.021607.090316
41. Asao H, Okuyama C, Kumaki S, Ishii N, Tsuchiya S, Foster D, et al. Cutting edge: the common γ-chain is an indispensable subunit of the IL-21 receptor complex. J Immunol. (2001) 167:1–5. doi: 10.4049/jimmunol.167.1.1
42. Søndergaard H, Skak K. IL-21: roles in immunopathology and cancer therapy. Tissue Antigens. (2009) 74:467–79. doi: 10.1111/j.1399-0039.2009.01382.x
43. Zeng R, Spolski R, Casas E, Zhu W, Levy DE, Leonard WJ. The molecular basis of IL-21–mediated proliferation. Blood. (2007) 109:4135–42. doi: 10.1182/blood-2006-10-054973
44. Choi YS, Eto D, Yang JA, Lao C, Crotty S. Cutting edge: STAT1 is required for IL-6-mediated Bcl6 induction for early follicular helper cell differentiation. J Immunol. (2013) 190:3049–53. doi: 10.4049/jimmunol.1203032
45. Park J, Son MJ, Ho CC, Lee SH, Kim Y, An J, et al. Transcriptional inhibition of STAT1 functions in the nucleus alleviates Th1 and Th17 cell-mediated inflammatory diseases. Front Immunol. (2022) 13:1054472. doi: 10.3389/fimmu.2022.1054472
46. Wang X, Lupardus P, LaPorte SL, Garcia KC. Structural biology of shared cytokine receptors. Annu Rev Immunol. (2009) 27:29–60. doi: 10.1146/annurev.immunol.24.021605.090616
47. Seidel HM, Milocco LH, Lamb P, Darnell JE Jr., Stein RB, Rosen J. Spacing of palindromic half sites as a determinant of selective STAT (signal transducers and activators of transcription) DNA binding and transcriptional activity. Proc Natl Acad Sci. (1995) 92:3041–5. doi: 10.1073/pnas.92.7.3041
48. Tolomeo M, Cavalli A, Cascio A. STAT1 and its crucial role in the control of viral infections. Int J Mol Sci. (2022) 23:4095. doi: 10.3390/ijms23084095
49. Yu H, Pardoll D, Jove R. STATs in cancer inflammation and immunity: a leading role for STAT3. Nat Rev Cancer. (2009) 9:798–809. doi: 10.1038/nrc2734
50. Wang HQ, Man QW, Huo FY, Gao X, Lin H, Li SR, et al. STAT3 pathway in cancers: Past, present, and future. MedComm. (2022) 3:e124. doi: 10.1002/mco2.v3.2
51. Bromberg J, Darnell JE. The role of STATs in transcriptional control and their impact on cellular function. Oncogene. (2000) 19:2468–73. doi: 10.1038/sj.onc.1203476
52. Li Y-J, Zhang C, Martincuks A, Herrmann A, Yu H. STAT proteins in cancer: orchestration of metabolism. Nat Rev Cancer. (2023) 23:115–34. doi: 10.1038/s41568-022-00537-3
53. Skak K, Frederiksen KS, Lundsgaard D. Interleukin-21 activates human natural killer cells and modulates their surface receptor expression. Immunology. (2008) 123:575–83. doi: 10.1111/j.1365-2567.2007.02730.x
54. Zhu S, Phatarpekar PV, Denman CJ, Senyukov VV, Somanchi SS, Nguyen-Jackson HT, et al. Transcription of the activating receptor NKG2D in natural killer cells is regulated by STAT3 tyrosine phosphorylation. Blood J Am Soc Hematol. (2014) 124:403–11. doi: 10.1182/blood-2013-05-499707
55. Burgess SJ, Marusina AI, Pathmanathan I, Borrego F, Coligan JE. IL-21 down-regulates NKG2D/DAP10 expression on human NK and CD8+ T cells. J Immunol. (2006) 176:1490–7. doi: 10.4049/jimmunol.176.3.1490
56. Vallières F, Girard D. Mechanism involved in interleukin-21-induced phagocytosis in human monocytes and macrophages. Clin Exp Immunol. (2017) 187:294–303. doi: 10.1111/cei.12886
57. Vallières F, Girard D. IL-21 enhances phagocytosis in mononuclear phagocyte cells: identification of spleen tyrosine kinase as a novel molecular target of IL-21. J Immunol. (2013) 190:2904–12. doi: 10.4049/jimmunol.1201941
58. Rückert R, Bulfone-Paus S, Brandt K. Interleukin-21 stimulates antigen uptake, protease activity, survival and induction of CD4+ T cell proliferation by murine macrophages. Clin Exp Immunol. (2008) 151:487–95. doi: 10.1111/j.1365-2249.2007.03581.x
59. Xu M, Liu M, Du X, Li S, Li H, Li X, et al. Intratumoral delivery of IL-21 overcomes anti-Her2/Neu resistance through shifting tumor-associated macrophages from M2 to M1 phenotype. J Immunol. (2015) 194:4997–5006. doi: 10.4049/jimmunol.1402603
60. Li S-N, Wang W, Fu S-P, Wang J-F, Liu H-M, Xie S-S, et al. IL-21 modulates release of proinflammatory cytokines in LPS-stimulated macrophages through distinct signaling pathways. Mediators Inflammation. (2013) 2013:548073. doi: 10.1155/2013/548073
61. Hashimoto-Kataoka T, Hosen N, Sonobe T, Arita Y, Yasui T, Masaki T, et al. Interleukin-6/interleukin-21 signaling axis is critical in the pathogenesis of pulmonary arterial hypertension. Proc Natl Acad Sci. (2015) 112:E2677–86. doi: 10.1073/pnas.1424774112
62. Jian L, Li C, Wang X, Sun L, Ma Z, Zhao J. IL-21 impairs pro-inflammatory activity of M1-like macrophages exerting anti-inflammatory effects on rheumatoid arthritis. Autoimmunity. (2022) 55:75–85. doi: 10.1080/08916934.2021.2007374
63. Cicora F, Roberti J, Lausada N, González P, Guerrieri D, Stringa P, et al. Donor preconditioning with rabbit anti-rat thymocyte immunoglobulin ameliorates ischemia reperfusion injury in rat kidney transplantation. Transplant Immunol. (2012) 27:1–7. doi: 10.1016/j.trim.2012.03.004
64. Heymann F, Peusquens J, Ludwig-Portugall I, Kohlhepp M, Ergen C, Niemietz P, et al. Liver inflammation abrogates immunological tolerance induced by Kupffer cells. Hepatology. (2015) 62:279–91. doi: 10.1002/hep.27793
65. Yang F, Wang S, Liu Y, Zhou Y, Shang L, Feng M, et al. IRE1α aggravates ischemia reperfusion injury of fatty liver by regulating phenotypic transformation of kupffer cells. Free Radical Biol Med. (2018) 124:395–407. doi: 10.1016/j.freeradbiomed.2018.06.043
66. Bergler T, Jung B, Bourier F, Kühne L, Banas MC, Rümmele P, et al. Infiltration of macrophages correlates with severity of allograft rejection and outcome in human kidney transplantation. PloS One. (2016) 11:e0156900. doi: 10.1371/journal.pone.0156900
67. Brandt K, Bulfone-Paus S, Foster DC, Rückert R. Interleukin-21 inhibits dendritic cell activation and maturation. Blood. (2003) 102:4090–8. doi: 10.1182/blood-2003-03-0669
68. Strengell M, Lehtonen A, Matikainen S, Julkunen I. IL-21 enhances SOCS gene expression and inhibits LPS-induced cytokine production in human monocyte-derived dendritic cells. J leukocyte Biol. (2006) 79:1279–85. doi: 10.1189/jlb.0905503
69. Rothwell L, Hu T, Wu Z, Kaiser P. Chicken interleukin-21 is costimulatory for T cells and blocks maturation of dendritic cells. Dev Comp Immunol. (2012) 36:475–82. doi: 10.1016/j.dci.2011.08.013
70. Karrich JJ, Jachimowski LC, Nagasawa M, Kamp A, Balzarolo M, Wolkers MC, et al. IL-21–stimulated human plasmacytoid dendritic cells secrete granzyme B, which impairs their capacity to induce T-cell proliferation. Blood J Am Soc Hematol. (2013) 121:3103–11. doi: 10.1182/blood-2012-08-452995
71. Van Belle TL, Nierkens S, Arens R, von Herrath MG. Interleukin-21 receptor-mediated signals control autoreactive T cell infiltration in pancreatic islets. Immunity. (2012) 36:1060–72. doi: 10.1016/j.immuni.2012.04.005
72. Wan C-K, Oh J, Li P, West EE, Wong EA, Andraski AB, et al. The cytokines IL-21 and GM-CSF have opposing regulatory roles in the apoptosis of conventional dendritic cells. Immunity. (2013) 38:514–27. doi: 10.1016/j.immuni.2013.02.011
73. Xue L, Hickling T, Song R, Nowak J, Rup B. Contribution of enhanced engagement of antigen presentation machinery to the clinical immunogenicity of a human interleukin (IL)-21 receptor-blocking therapeutic antibody. Clin Exp Immunol. (2016) 183:102–13. doi: 10.1111/cei.12711
74. Duneton C, Winterberg PD, Ford ML. Activation and regulation of alloreactive T cell immunity in solid organ transplantation. Nat Rev Nephrol. (2022) 18:663–76. doi: 10.1038/s41581-022-00600-0
75. Talmage DW, Dart G, Radovich J, Lafferty KJ. Activation of transplant immunity: effect of donor leukocytes on thyroid allograft rejection. Science. (1976) 191:385–8. doi: 10.1126/science.1082167
76. Rokahr, Sharland, Sun, Wang, Sheil, Yan, et al. Paradoxical early immune activation during acceptance of liver allografts compared with rejection of skin grafts in a rat model of transplantation. Immunology. (1998) 95:257–63. doi: 10.1046/j.1365-2567.1998.00840.x
77. Benichou G, Fedoseyeva E, Lehmann PV, Olson CA, Geysen HM, McMillan M, et al. Limited T cell response to donor MHC peptides during allograft rejection. Implications for selective immune therapy in transplantation. J Immunol (Baltimore Md: 1950). (1994) 153:938–45. doi: 10.4049/jimmunol.153.3.938
78. Herrera OB, Golshayan D, Tibbott R, Ochoa FS, James MJ, Marelli-Berg FM, et al. A novel pathway of alloantigen presentation by dendritic cells. J Immunol. (2004) 173:4828–37. doi: 10.4049/jimmunol.173.8.4828
79. Brown K, Sacks SH, Wong W. Extensive and bidirectional transfer of major histocompatibility complex class II molecules between donor and recipient cells in vivo following solid organ transplantation. FASEB J. (2008) 22:3776–84. doi: 10.1096/fj.08-107441
80. Harper SJ, Ali JM, Wlodek E, Negus MC, Harper IG, Chhabra M, et al. CD8 T-cell recognition of acquired alloantigen promotes acute allograft rejection. Proc Natl Acad Sci U S A. (2015) 112:12788–93. doi: 10.1073/pnas.1513533112
81. Markey KA, Koyama M, Gartlan KH, Leveque L, Kuns RD, Lineburg KE, et al. Cross-dressing by donor dendritic cells after allogeneic bone marrow transplantation contributes to formation of the immunological synapse and maximizes responses to indirectly presented antigen. J Immunol. (2014) 192:5426–33. doi: 10.4049/jimmunol.1302490
82. Ruggeri L, Capanni M, Martelli MF, Velardi A. Cellular therapy: exploiting NK cell alloreactivity in transplantation. Curr Opin Hematol. (2001) 8:355–9. doi: 10.1097/00062752-200111000-00007
83. Denman CJ, Senyukov VV, Somanchi SS, Phatarpekar PV, Kopp LM, Johnson JL, et al. Membrane-bound IL-21 promotes sustained ex vivo proliferation of human natural killer cells. PloS One. (2012) 7:e30264. doi: 10.1371/journal.pone.0030264
84. Seo H, Jeon I, Kim B-S, Park M, Bae E-A, Song B, et al. IL-21-mediated reversal of NK cell exhaustion facilitates anti-tumour immunity in MHC class I-deficient tumours. Nat Commun. (2017) 8:15776. doi: 10.1038/ncomms15776
85. Tang L, Li Q, Chen L, Li X, Gu S, He W, et al. IL-21 collaborates with anti-TIGIT to restore NK cell function in chronic HBV infection. J Med Virol. (2023) 95:e29142. doi: 10.1002/jmv.v95.10
86. Toomey JA, Gays F, Foster D, Brooks CG. Cytokine requirements for the growth and development of mouse NK cells in vitro. J Leucocyte Biol. (2003) 74:233–42. doi: 10.1189/jlb.0303097
87. Perez SA, Mahaira LG, Sotiropoulou PA, Gritzapis AD, Iliopoulou EG, Niarchos DK, et al. Effect of IL-21 on NK cells derived from different umbilical cord blood populations. Int Immunol. (2006) 18:49–58. doi: 10.1093/intimm/dxh348
88. Harmon C, Sanchez-Fueyo A, O’farrelly C, Houlihan D. Natural killer cells and liver transplantation: orchestrators of rejection or tolerance? Am J Transplant. (2016) 16:751–7. doi: 10.1111/ajt.13565
89. Maier S, Tertilt C, Chambron N, Gerauer K, Hüser N, Heidecke C-D, et al. Inhibition of natural killer cells results in acceptance of cardiac allografts in CD28–/– mice. Nat Med. (2001) 7:557–62. doi: 10.1038/87880
90. Obara H, Nagasaki K, Hsieh CL, Ogura Y, Esquivel CO, Martinez OM, et al. IFN-γ, produced by NK cells that infiltrate liver allografts early after transplantation, links the innate and adaptive immune responses. Am J Transplant. (2005) 5:2094–103. doi: 10.1111/j.1600-6143.2005.00995.x
91. Martínez-Llordella M, Lozano JJ, Puig-Pey I, Orlando G, Tisone G, Lerut J, et al. Using transcriptional profiling to develop a diagnostic test of operational tolerance in liver transplant recipients. J Clin Invest. (2008) 118:2845–57. doi: 10.1172/JCI35342
92. Li L, Wozniak L, Rodder S, Heish S, Talisetti A, Wang Q, et al. A common peripheral blood gene set for diagnosis of operational tolerance in pediatric and adult liver transplantation. Am J Transplant. (2012) 12:1218–28. doi: 10.1111/j.1600-6143.2011.03928.x
93. Walters GD, Vinuesa CG. T follicular helper cells in transplantation. Transplantation. (2016) 100:1650–5. doi: 10.1097/TP.0000000000001217
94. Ma CS, Deenick EK. Human T follicular helper (Tfh) cells and disease. Immunol Cell Biol. (2014) 92:64–71. doi: 10.1038/icb.2013.55
95. Vogelzang A, McGuire HM, Yu D, Sprent J, Mackay CR, King C. A fundamental role for interleukin-21 in the generation of T follicular helper cells. Immunity. (2008) 29:127–37. doi: 10.1016/j.immuni.2008.06.001
96. Nurieva RI, Chung Y, Hwang D, Yang XO, Kang HS, Ma L, et al. Generation of T follicular helper cells is mediated by interleukin-21 but independent of T helper 1, 2, or 17 cell lineages. Immunity. (2008) 29:138–49. doi: 10.1016/j.immuni.2008.05.009
97. Linterman MA, Beaton L, Yu D, Ramiscal RR, Srivastava M, Hogan JJ, et al. IL-21 acts directly on B cells to regulate Bcl-6 expression and germinal center responses. J Exp Med. (2010) 207:353–63. doi: 10.1084/jem.20091738
98. Zotos D, Coquet JM, Zhang Y, Light A, D'Costa K, Kallies A, et al. IL-21 regulates germinal center B cell differentiation and proliferation through a B cell–intrinsic mechanism. J Exp Med. (2010) 207:365–78. doi: 10.1084/jem.20091777
99. Vinuesa CG, Linterman MA, Yu D, MacLennan IC. Follicular helper T cells. Annu Rev Immunol. (2016) 34:335–68. doi: 10.1146/annurev-immunol-041015-055605
100. Crotty S. Follicular helper CD4 T cells (Tfh). Annu Rev Immunol. (2011) 29:621–63. doi: 10.1146/annurev-immunol-031210-101400
101. Liu D, Yan J, Sun J, Liu B, Ma W, Li Y, et al. BCL6 controls contact-dependent help delivery during follicular TB cell interactions. Immunity. (2021) 54:2245–2255. e4. doi: 10.1016/j.immuni.2021.08.003
102. Bryant VL, Ma CS, Avery DT, Li Y, Good KL, Corcoran LM, et al. Cytokine-mediated regulation of human B cell differentiation into Ig-secreting cells: predominant role of IL-21 produced by CXCR5+ T follicular helper cells. J Immunol. (2007) 179:8180–90. doi: 10.4049/jimmunol.179.12.8180
103. de Vinuesa CG, Cook MC, Ball J, Drew M, Sunners Y, Cascalho M, et al. Germinal centers without T cells. J Exp Med. (2000) 191:485–94. doi: 10.1084/jem.191.3.485
104. Shulman Z, Gitlin AD, Weinstein JS, Lainez B, Esplugues E, Flavell RA, et al. Dynamic signaling by T follicular helper cells during germinal center B cell selection. Science. (2014) 345:1058–62. doi: 10.1126/science.1257861
105. Baan CC, de Graav GN, Boer K. T follicular helper cells in transplantation: the target to attenuate antibody-mediated allogeneic responses? Curr Transplant Rep. (2014) 1:166–72. doi: 10.1007/s40472-014-0019-4
106. Sellarés J, De Freitas D, Mengel M, Reeve J, Einecke G, Sis B, et al. Understanding the causes of kidney transplant failure: the dominant role of antibody-mediated rejection and nonadherence. Am J Transplant. (2012) 12:388–99. doi: 10.1111/j.1600-6143.2011.03840.x
107. Liu J, Tang T, Qu Z, Wang L, Si R, Wang H, et al. Elevated number of IL-21+ TFH and CD86+CD38+ B cells in blood of renal transplant recipients with AMR under conventional immuno-suppression. Int J Immunopathol Pharmacol. (2022) 36:20587384211048027. doi: 10.1177/20587384211048027
108. Macedo C, Hadi K, Walters J, Elinoff B, Marrari M, Zeevi A, et al. Impact of induction therapy on circulating T follicular helper cells and subsequent donor-specific antibody formation after kidney transplant. Kidney Int Rep. (2019) 4:455–69. doi: 10.1016/j.ekir.2018.11.020
109. Shi J, Luo F, Shi Q, Xu X, He X, Xia Y. Increased circulating follicular helper T cells with decreased programmed death-1 in chronic renal allograft rejection. BMC Nephrol. (2015) 16:182. doi: 10.1186/s12882-015-0172-8
110. de Graav G, Dieterich M, Hesselink D, Boer K, Clahsen-van Groningen M, Kraaijeveld R, et al. Follicular T helper cells and humoral reactivity in kidney transplant patients. Clin Exp Immunol. (2015) 180:329–40. doi: 10.1111/cei.12576
111. Chen W, Bai J, Huang H, Bi L, Kong X, Gao Y, et al. Low proportion of follicular regulatory T cell in renal transplant patients with chronic antibody-mediated rejection. Sci Rep. (2017) 7:1322. doi: 10.1038/s41598-017-01625-3
112. Colvin RB, Smith RN. Antibody-mediated organ-allograft rejection. Nat Rev Immunol. (2005) 5:807–17. doi: 10.1038/nri1702
113. Jindra PT, Hsueh A, Hong L, Gjertson D, Shen X-D, Gao F, et al. Anti-MHC class I antibody activation of proliferation and survival signaling in murine cardiac allografts. J Immunol. (2008) 180:2214–24. doi: 10.4049/jimmunol.180.4.2214
114. Khattar M, Baum CE, Schroder P, Breidenbach JD, Haller ST, Chen W, et al. Interleukin 21 (IL-21) regulates chronic allograft vasculopathy (CAV) in murine heart allograft rejection. PloS One. (2019) 14:e0225624. doi: 10.1371/journal.pone.0225624
115. de Leur K, Luk F, van den Bosch TP, Dieterich M, van der Laan LJ, Hendriks RW, et al. The effects of an IL-21 receptor antagonist on the alloimmune response in a humanized mouse skin transplant model. Transplantation. (2019) 103:2065–74. doi: 10.1097/TP.0000000000002773
116. Zhang K, Sun Y-L, Yang F, Shi Y-C, Jin L, Liu Z-W, et al. A pilot study on the characteristics of circulating T follicular helper cells in liver transplant recipients. Transplant Immunol. (2018) 47:32–6. doi: 10.1016/j.trim.2018.01.003
117. Zhang K, Sun Y-L, Zhou S-N, Xu R-N, Liu Z-W, Wang F-S, et al. Circulating CXCR3-CCR6-CXCR5+ CD4+ T cells are associated with acute allograft rejection in liver transplantation. Immunol Lett. (2019) 213:55–61. doi: 10.1016/j.imlet.2019.03.003
118. Safavi S, Robinson DR, Soresi S, Carby M, Smith JD. De novo donor HLA-specific antibodies predict development of bronchiolitis obliterans syndrome after lung transplantation. J Heart Lung Transplant. (2014) 33:1273–81. doi: 10.1016/j.healun.2014.07.012
119. Morrell MR, Pilewski JM, Gries CJ, Pipeling MR, Crespo MM, Ensor CR, et al. De novo donor-specific HLA antibodies are associated with early and high-grade bronchiolitis obliterans syndrome and death after lung transplantation. J Heart Lung Transplant. (2014) 33:1288–94. doi: 10.1016/j.healun.2014.07.018
120. de Graav GN, Hesselink DA, Dieterich M, Kraaijeveld R, Verschoor W, Roelen DL, et al. Belatacept does not inhibit follicular T cell-dependent B-cell differentiation in kidney transplantation. Front Immunol. (2017) 8:641. doi: 10.3389/fimmu.2017.00641
121. Wallin EF, Hill DL, Linterman MA, Wood KJ. The calcineurin inhibitor tacrolimus specifically suppresses human T follicular helper cells. Front Immunol. (2018) 9:1184. doi: 10.3389/fimmu.2018.01184
122. Cano-Romero FL, Goya RL, Utrero-Rico A, Gómez-Massa E, Arroyo-Sánchez D, Suárez-Fernández P, et al. Longitudinal profile of circulating T follicular helper lymphocytes parallels anti-HLA sensitization in renal transplant recipients. Am J Transplant. (2019) 19:89–97. doi: 10.1111/ajt.14987
123. Van Kooten C, Boonstra JG, Paape ME, Fossiez F, Banchereau J, Lebecque S, et al. Interleukin-17 activates human renal epithelial cells in vitro and is expressed during renal allograft rejection. J Am Soc Nephrol. (1998) 9:1526–34. doi: 10.1681/ASN.V981526
124. Vanaudenaerde B, Dupont L, Wuyts W, Verbeken E, Meyts I, Bullens D, et al. The role of interleukin-17 during acute rejection after lung transplantation. Eur Respir J. (2006) 27:779–87. doi: 10.1183/09031936.06.00019405
125. Loong CC, Hsieh HG, Lui WY, Chen A, Lin CY. Retracted: Evidence for the early involvement of interleukin 17 in human and experimental renal allograft rejection. J Pathol. (2002) 197:322–32. doi: 10.1002/path.v197:3
126. Chung BH, Oh HJ, Piao SG, Sun IO, Kang SH, Choi SR, et al. Higher infiltration by Th17 cells compared with regulatory T cells is associated with severe acute T-cell-mediated graft rejection. Exp Mol Med. (2011) 43:630–7. doi: 10.3858/emm.2011.43.11.071
127. Xie X-J, Ye Y-F, Zhou L, Xie H-Y, Jiang G-P, Feng X-W, et al. Th17 promotes acute rejection following liver transplantation in rats. J Zhejiang Univ Sci B. (2010) 11:819–27. doi: 10.1631/jzus.B1000030
128. Assadiasl S, Toosi MN, Mohebbi B, Ansaripour B, Soleimanifar N, Sadr M, et al. Th17/Treg cell balance in stable liver transplant recipients. Transplant Immunol. (2022) 71:101540. doi: 10.1016/j.trim.2022.101540
129. Korn T, Bettelli E, Gao W, Awasthi A, Jäger A, Strom TB, et al. IL-21 initiates an alternative pathway to induce proinflammatory TH17 cells. Nature. (2007) 448:484–7. doi: 10.1038/nature05970
130. Deteix C, Attuil-Audenis V, Duthey A, Patey N, McGregor B, Dubois V, et al. Intragraft Th17 infiltrate promotes lymphoid neogenesis and hastens clinical chronic rejection. J Immunol. (2010) 184:5344–51. doi: 10.4049/jimmunol.0902999
131. Shi Y, Chen Z, Zhao Z, Yu Y, Fan H, Xu X, et al. IL-21 induces an imbalance of Th17/Treg cells in moderate-to-severe plaque psoriasis patients. Front Immunol. (2019) 10:1865. doi: 10.3389/fimmu.2019.01865
132. Niu X, He D, Zhang X, Yue T, Li N, Zhang JZ, et al. IL-21 regulates Th17 cells in rheumatoid arthritis. Hum Immunol. (2010) 71:334–41. doi: 10.1016/j.humimm.2010.01.010
133. Tan Y, Chen W, Liu C, Zheng X, Guo A, Long J. Effect of IL-21 on the balance of Th17 cells/Treg cells in the pathogenesis of Graves’ disease. Endocr Res. (2019) 44:138–47. doi: 10.1080/07435800.2019.1600535
134. Mitsdoerffer M, Lee Y, Jäger A, Kim H-J, Korn T, Kolls JK, et al. Proinflammatory T helper type 17 cells are effective B-cell helpers. Proc Natl Acad Sci. (2010) 107:14292–7. doi: 10.1073/pnas.1009234107
135. Huang Y, Matsumura Y, Hatano S, Noguchi N, Murakami T, Iwakura Y, et al. IL-21 inhibits IL-17A-producing γδ T-cell response after infection with Bacillus Calmette-Guérin via induction of apoptosis. Innate Immun. (2016) 22:588–97. doi: 10.1177/1753425916664125
136. Yang L, Anderson DE, Baecher-Allan C, Hastings WD, Bettelli E, Oukka M, et al. IL-21 and TGF-β are required for differentiation of human TH17 cells. Nature. (2008) 454:350–2. doi: 10.1038/nature07021
137. Sonderegger I, Kisielow J, Meier R, King C, Kopf M. IL-21 and IL-21R are not required for development of Th17 cells and autoimmunity in vivo. Eur J Immunol. (2008) 38:1833–8. doi: 10.1002/eji.200838511
138. Attridge K, Wang CJ, Wardzinski L, Kenefeck R, Chamberlain JL, Manzotti C, et al. IL-21 inhibits T cell IL-2 production and impairs Treg homeostasis. Blood J Am Soc Hematol. (2012) 119:4656–64. doi: 10.1182/blood-2011-10-388546
139. Lindqvist CA, Christiansson LH, Simonsson B, Enblad G, Olsson-Strömberg U, Loskog AS. T regulatory cells control T-cell proliferation partly by the release of soluble CD25 in patients with B-cell Malignancies. Immunology. (2010) 131:371–6. doi: 10.1111/j.1365-2567.2010.03308.x
140. Petrelli A, Carvello M, Vergani A, Lee KM, Tezza S, Du M, et al. IL-21 is an antitolerogenic cytokine of the late-phase alloimmune response. Diabetes. (2011) 60:3223–34. doi: 10.2337/db11-0880
141. Bucher C, Koch L, Vogtenhuber C, Goren E, Munger M, Panoskaltsis-Mortari A, et al. IL-21 blockade reduces graft-versus-host disease mortality by supporting inducible T regulatory cell generation. Blood J Am Soc Hematol. (2009) 114:5375–84. doi: 10.1182/blood-2009-05-221135
142. Pillarisetty VG, Shah AB, Miller G, Bleier JI, DeMatteo RP. Liver dendritic cells are less immunogenic than spleen dendritic cells because of differences in subtype composition. J Immunol. (2004) 172:1009–17. doi: 10.4049/jimmunol.172.2.1009
143. Matta BM, Raimondi G, Rosborough BR, Sumpter TL, Thomson AW. IL-27 production and STAT3-dependent upregulation of B7-H1 mediate immune regulatory functions of liver plasmacytoid dendritic cells. J Immunol. (2012) 188:5227–37. doi: 10.4049/jimmunol.1103382
144. Rastellini C, Lu L, Ricordi C, Starzl TE, Rao AS, Thomson AW. Granulocyte/macrophage colony-stimulating factor-stimulated hepatic dendritic cell progenitors prolong pancreatic islet allograft survival. Transplantation. (1995) 60:1366.
145. Demirkiran A, Kok A, Kwekkeboom J, Kusters JG, Metselaar HJ, Tilanus HW, et al. Low circulating regulatory T-cell levels after acute rejection in liver transplantation. Liver Transpl. (2006) 12:277–84. doi: 10.1002/(ISSN)1527-6473
146. Mosmann TR, Cherwinski H, Bond MW, Giedlin MA, Coffman RL. Two types of murine helper T cell clone. I. Definition according to profiles of lymphokine activities and secreted proteins. J Immunol (Baltimore MD: 1950). (1986) 136:2348–57. doi: 10.4049/jimmunol.136.7.2348
147. Strengell M, Sareneva T, Foster D, Julkunen I, Matikainen S. IL-21 up-regulates the expression of genes associated with innate immunity and Th1 response. J Immunol. (2002) 169:3600–5. doi: 10.4049/jimmunol.169.7.3600
148. Wurster AL, Rodgers VL, Satoskar AR, Whitters MJ, Young DA, Collins M, et al. Interleukin 21 is a T helper (Th) cell 2 cytokine that specifically inhibits the differentiation of naive Th cells into interferon γ–producing Th1 cells. J Exp Med. (2002) 196:969–77. doi: 10.1084/jem.20020620
149. Fröhlich A, Marsland BJ, Sonderegger I, Kurrer M, Hodge MR, Harris NL, et al. IL-21 receptor signaling is integral to the development of Th2 effector responses in vivo. Blood. (2007) 109:2023–31. doi: 10.1182/blood-2006-05-021600
150. Lin PY, Jen HY, Chiang BL, Sheu F, Chuang YH. Interleukin-21 suppresses the differentiation and functions of T helper 2 cells. Immunology. (2015) 144:668–76. doi: 10.1111/imm.2015.144.issue-4
151. Tortola L, Pawelski H, Sonar SS, Ampenberger F, Kurrer M, Kopf M. IL-21 promotes allergic airway inflammation by driving apoptosis of FoxP3+ regulatory T cells. J Allergy Clin Immunol. (2019) 143:2178–2189. e5. doi: 10.1016/j.jaci.2018.11.047
152. Suto A, Nakajima H, Hirose K, Suzuki K, Kagami S-I, Seto Y, et al. Interleukin 21 prevents antigen-induced IgE production by inhibiting germ line Cϵ transcription of IL-4–stimulated B cells. Blood J Am Soc Hematol. (2002) 100:4565–73. doi: 10.1182/blood-2002-04-1115
153. Dwyer GK, Mathews LR, Villegas JA, Lucas A, de Peredo AG, Blazar BR, et al. IL-33 acts as a costimulatory signal to generate alloreactive Th1 cells in graft-versus-host disease. J Clin Invest. (2022) 132(12):e150927. doi: 10.1172/JCI150927
154. Chen J, Guan L, Tang L, Liu S, Zhou Y, Chen C, et al. T helper 9 cells: a new player in immune-related diseases. DNA Cell Biol. (2019) 38:1040–7. doi: 10.1089/dna.2019.4729
155. Liao W, Spolski R, Li P, Du N, West EE, Ren M, et al. Opposing actions of IL-2 and IL-21 on Th9 differentiation correlate with their differential regulation of BCL6 expression. Proc Natl Acad Sci. (2014) 111:3508–13. doi: 10.1073/pnas.1301138111
156. Wong MT, Ye JJ, Alonso MN, Landrigan A, Cheung RK, Engleman E, et al. Regulation of human Th9 differentiation by type I interferons and IL-21. Immunol Cell Biol. (2010) 88:624–31. doi: 10.1038/icb.2010.53
157. Galli SJ, Kalesnikoff J, Grimbaldeston MA, Piliponsky AM, Williams CM, Tsai M. Mast cells as “tunable” effector and immunoregulatory cells: recent advances. Annu Rev Immunol. (2005) 23:749–86. doi: 10.1146/annurev.immunol.21.120601.141025
158. Lu L-F, Lind EF, Gondek DC, Bennett KA, Gleeson MW, Pino-Lagos K, et al. Mast cells are essential intermediaries in regulatory T-cell tolerance. Nature. (2006) 442:997–1002. doi: 10.1038/nature05010
159. Ganbold A, Andersen S, Tay SS, Cunningham E, Ilie V, Krishnan S, et al. Expression of common gamma chain signalling cytokines and their receptors distinguishes rejection from tolerance in a rat organ transplant model. Transpl Immunol. (2012) 27:89–94. doi: 10.1016/j.trim.2012.08.001
160. Poulin LF, Richard M, Le Moine A, Kiss R, McKenzie AN, Goldman M, et al. Interleukin-9 promotes eosinophilic rejection of mouse heart allografts. Transplantation. (2003) 76:572–7. doi: 10.1097/01.TP.0000071201.32424.D2
161. Fábrega E, López-Hoyos M, San Segundo D, Casafont F, Moraleja I, Sampedro B, et al. Interleukin-9 in stable liver transplant recipients, Transplant. Proc. (2012) 44(6):1536–8. doi: 10.1016/j.transproceed.2012.05.014
162. Fábrega E, López-Hoyos M, San Segundo D, Casafont F, Mieses MA, Sampedro B, et al. Serum levels of interleukin-9 during acute rejection in liver transplantation, Transplant. Proc. (2012) 44(6):1533–5. doi: 10.1016/j.transproceed.2012.05.013
163. Kumano M, Hara I, Furukawa J, Oniki S, Nagai H, Miyake H, et al. Interleukin-21 activates cytotoxic T lymphocytes and natural killer cells to generate antitumor response in mouse renal cell carcinoma. J Urol. (2007) 178:1504–9. doi: 10.1016/j.juro.2007.05.115
164. Moreau A, Varey E, Anegon I, Cuturi M-C. Effector mechanisms of rejection. Cold Spring Harb Perspect Med. (2013) 3:a015461. doi: 10.1101/cshperspect.a015461
165. Choudhary NS, Saigal S, Bansal RK, Saraf N, Gautam D, Soin AS. Acute and chronic rejection after liver transplantation: what a clinician needs to know. J Clin Exp Hepatol. (2017) 7:358–66. doi: 10.1016/j.jceh.2017.10.003
166. Zhou L-X, Jiang Y-Z, Li X-Q, Zhang J-M, Li S-P, Wei L, et al. Myeloid-derived suppressor cells-induced exhaustion of CD8+ T-cell participates in rejection after liver transplantation. Cell Death Dis. (2024) 15:507. doi: 10.1038/s41419-024-06834-z
167. Meguro A, Ozaki K, Oh I, Hatanaka K, Matsu H, Tatara R, et al. IL-21 is critical for GVHD in a mouse model. Bone Marrow Transplant. (2010) 45:723–9. doi: 10.1038/bmt.2009.223
168. Hippen KL, Bucher C, Schirm DK, Bearl AM, Brender T, Mink KA, et al. Blocking IL-21 signaling ameliorates xenogeneic GVHD induced by human lymphocytes. Blood. (2012) 119:619–28. doi: 10.1182/blood-2011-07-368027
169. Nguyen V, Rus H, Chen C, Rus V. CTL-promoting effects of IL-21 counteract murine lupus in the parent→ F1 graft-versus-host disease model. J Immunol. (2016) 196:1529–40. doi: 10.4049/jimmunol.1501824
170. Allard EL, Hardy MP, Leignadier J, Marquis M, Rooney J, Lehoux D, et al. Overexpression of IL-21 promotes massive CD8+ memory T cell accumulation. Eur J Immunol. (2007) 37:3069–77. doi: 10.1002/eji.200637017
171. Cui W, Liu Y, Weinstein JS, Craft J, Kaech SM. An interleukin-21-interleukin-10-STAT3 pathway is critical for functional maturation of memory CD8+ T cells. Immunity. (2011) 35:792–805. doi: 10.1016/j.immuni.2011.09.017
172. Sutherland AP, Joller N, Michaud M, Liu SM, Kuchroo VK, Grusby MJ. IL-21 promotes CD8+ CTL activity via the transcription factor T-bet. J Immunol. (2013) 190:3977–84. doi: 10.4049/jimmunol.1201730
173. Casey KA, Mescher MF. IL-21 promotes differentiation of naive CD8 T cells to a unique effector phenotype. J Immunol. (2007) 178:7640–8. doi: 10.4049/jimmunol.178.12.7640
174. Zoon CK, Wan W, Graham L, Bear HD. Addition of interleukin-21 for expansion of T-cells for adoptive immunotherapy of murine melanoma. Int J Mol Sci. (2015) 16:8744–60. doi: 10.3390/ijms16048744
175. Liu W, Dienz O, Roberts B, Moussawi M, Rincon M, Huber SA. IL-21R expression on CD8+ T cells promotes CD8+ T cell activation in coxsackievirus B3 induced myocarditis. Exp Mol Pathol. (2012) 92:327–33. doi: 10.1016/j.yexmp.2012.03.009
176. Zeng R, Spolski R, Finkelstein SE, Oh S, Kovanen PE, Hinrichs CS, et al. Synergy of IL-21 and IL-15 in regulating CD8+ T cell expansion and function. J Exp Med. (2005) 201:139–48. doi: 10.1084/jem.20041057
177. Zander R, Kasmani MY, Chen Y, Topchyan P, Shen J, Zheng S, et al. Tfh-cell-derived interleukin 21 sustains effector CD8+ T cell responses during chronic viral infection. Immunity. (2022) 55:475–493. e5. doi: 10.1016/j.immuni.2022.01.018
178. Xin G, Schauder DM, Lainez B, Weinstein JS, Dai Z, Chen Y, et al. A critical role of IL-21-induced BATF in sustaining CD8-T-cell-mediated chronic viral control. Cell Rep. (2015) 13:1118–24. doi: 10.1016/j.celrep.2015.09.069
179. Dvorscek AR, McKenzie CI, Robinson MJ, Ding Z, Pitt C, O'Donnell K, et al. IL-21 has a critical role in establishing germinal centers by amplifying early B cell proliferation. EMBO Rep. (2022) 23:e54677. doi: 10.15252/embr.202254677
180. Zotos D, Quast I, Li-Wai-Suen CS, McKenzie CI, Robinson MJ, Kan A, et al. The concerted change in the distribution of cell cycle phases and zone composition in germinal centers is regulated by IL-21. Nat Commun. (2021) 12:7160. doi: 10.1038/s41467-021-27477-0
181. Luo W, Conter L, Elsner RA, Smita S, Weisel F, Callahan D, et al. IL-21R signal reprogramming cooperates with CD40 and BCR signals to select and differentiate germinal center B cells. Sci Immunol. (2023) 8:eadd1823. doi: 10.1126/sciimmunol.add1823
182. Ersching J, Efeyan A, Mesin L, Jacobsen JT, Pasqual G, Grabiner BC, et al. Germinal center selection and affinity maturation require dynamic regulation of mTORC1 kinase. Immunity. (2017) 46:1045–1058. e6. doi: 10.1016/j.immuni.2017.06.005
183. Montano-Loza AJ, Rodríguez-Perálvarez ML, Pageaux G-P, Sanchez-Fueyo A, Feng S. Liver transplantation immunology: Immunosuppression, rejection, and immunomodulation. J Hepatol. (2023) 78:1199–215. doi: 10.1016/j.jhep.2023.01.030
184. Ozaki K, Spolski R, Ettinger R, Kim H-P, Wang G, Qi C-F, et al. Regulation of B cell differentiation and plasma cell generation by IL-21, a novel inducer of Blimp-1 and Bcl-6. J Immunol. (2004) 173:5361–71. doi: 10.4049/jimmunol.173.9.5361
185. Kitano M, Moriyama S, Ando Y, Hikida M, Mori Y, Kurosaki T, et al. Bcl6 protein expression shapes pre-germinal center B cell dynamics and follicular helper T cell heterogeneity. Immunity. (2011) 34:961–72. doi: 10.1016/j.immuni.2011.03.025
186. Zhang Y, Tech L, George LA, Acs A, Durrett RE, Hess H, et al. Plasma cell output from germinal centers is regulated by signals from Tfh and stromal cells. J Exp Med. (2018) 215:1227–43. doi: 10.1084/jem.20160832
187. Scharer CD, Barwick BG, Guo M, Bally AP, Boss JM. Plasma cell differentiation is controlled by multiple cell division-coupled epigenetic programs. Nat Commun. (2018) 9:1698. doi: 10.1038/s41467-018-04125-8
188. Xu Y, Huang X, Li F, Liu T, Yang T, Chen F, et al. IL-21 enhances STAT3/Blimp-1 signaling pathway in B cells and contributes to plasma cell differentiation in newly diagnosed patients with myasthenia gravis. Immunol Res. (2021) 69:59–70. doi: 10.1007/s12026-020-09164-2
189. Yi SG, Gaber AO, Chen W. B-cell response in solid organ transplantation. Front Immunol. (2022) 13:895157. doi: 10.3389/fimmu.2022.895157
190. Attridge K, Kenefeck R, Wardzinski L, Qureshi OS, Wang CJ, Manzotti C, et al. IL-21 promotes CD4 T cell responses by phosphatidylinositol 3-kinase–dependent upregulation of CD86 on B cells. J Immunol. (2014) 192:2195–201. doi: 10.4049/jimmunol.1302082
191. Jin H, Carrio R, Yu A, Malek TR. Distinct activation signals determine whether IL-21 induces B cell costimulation, growth arrest, or Bim-dependent apoptosis. J Immunol. (2004) 173:657–65. doi: 10.4049/jimmunol.173.1.657
192. Yoshizaki A, Miyagaki T, DiLillo DJ, Matsushita T, Horikawa M, Kountikov EI, et al. Regulatory B cells control T-cell autoimmunity through IL-21-dependent cognate interactions. Nature. (2012) 491:264–8. doi: 10.1038/nature11501
193. Hu Y, Yu P, Yu X, Hu X, Kawai T, Han X. IL-21/anti-Tim1/CD40 ligand promotes B10 activity in vitro and alleviates bone loss in experimental periodontitis in vivo. Biochim Biophys Acta (BBA)-Molecular Basis Dis. (2017) 1863:2149–57. doi: 10.1016/j.bbadis.2017.06.001
194. Bankó Z, Pozsgay J, Szili D, Tóth M, Gáti T, Nagy G, et al. Induction and differentiation of IL-10–producing regulatory B cells from healthy blood donors and rheumatoid arthritis patients. J Immunol. (2017) 198:1512–20. doi: 10.4049/jimmunol.1600218
195. De Leur K, Dor FJ, Dieterich M, van der Laan LJ, Hendriks RW, Baan CC. IL-21 receptor antagonist inhibits differentiation of B cells toward plasmablasts upon alloantigen stimulation. Front Immunol. (2017) 8:306. doi: 10.3389/fimmu.2017.00306
196. van Besouw NM, Yan L, de Kuiper R, Klepper M, Reijerkerk D, Dieterich M, et al. The number of donor-specific IL-21 producing cells before and after transplantation predicts kidney graft rejection. Front Immunol. (2019) 10:748. doi: 10.3389/fimmu.2019.00748
197. Afshari A, Yaghobi R, Azarpira N, Zadeh BG, Hosseini SAM, Darbouy M, et al. Evaluation of Interleukin-21, 23 and 27 mRNA expression and protein level in liver transplant patients. Iranian J Allergy Asthma Immunol. (2018) 17(4):298–307. doi: 10.18502/ijaai.v17i4.89
198. Heidt S, Roelen DL, Eijsink C, van Kooten C, Claas FH, Mulder A. Effects of immunosuppressive drugs on purified human B cells: evidence supporting the use of MMF and rapamycin. Transplantation. (2008) 86:1292–300. doi: 10.1097/TP.0b013e3181874a36
199. Park J-S, Kim S-M, Hwang S-H, Choi S-Y, Kwon JY, Kwok S-K, et al. Combinatory treatment using tacrolimus and a STAT3 inhibitor regulate Treg cells and plasma cells. Int J immunopathology Pharmacol. (2018) 32:2058738418778724. doi: 10.1177/2058738418778724
200. Deuse T, Hua X, Taylor V, Stubbendorff M, Baluom M, Chen Y, et al. Significant reduction of acute cardiac allograft rejection by selective janus kinase-1/3 inhibition using R507 and R545. Transplantation. (2012) 94:695–702. doi: 10.1097/TP.0b013e3182660496
201. An Investigation of Safety and Tolerability of NNC0114-0006 in Subjects With Systemic Lupus Erythematosus (SLE) . ClinicalTrials.gov. Available online at: https://clinicaltrials.gov/ct2/show/NCT01689025?term=NCT01689025&rank=1 (Accessed October 8, 2015).
202. Hua F, Comer GM, Stockert L, Jin B, Nowak J, Pleasic-Williams S, et al. Anti-IL21 receptor monoclonal antibody (ATR-107): Safety, pharmacokinetics, and pharmacodynamic evaluation in healthy volunteers: a phase I, first-in-human study. J Clin Pharmacol. (2014) 54:14–22. doi: 10.1002/jcph.v54.1
203. Ignatenko S, Skrumsager BK, Mouritzen U, Safety PK. and PD of recombinant anti-interleukin-21 monoclonal antibody in a first-in-human trial. Int J Clin Pharmacol Ther. (2016) 54:243–52. doi: 10.5414/CP202474
204. von Herrath M, Bain SC, Bode B, Clausen JO, Coppieters K, Gaysina L, et al. Anti-interleukin-21 antibody and liraglutide for the preservation of β-cell function in adults with recent-onset type 1 diabetes: a randomised, double-blind, placebo-controlled, phase 2 trial. Lancet Diabetes Endocrinol. (2021) 9:212–24. doi: 10.1016/S2213-8587(21)00019-X
205. Hussaini A, Mukherjee R, Berdieva DM, Glogowski C, Mountfield R, Ho PT, et al. Single ascending dose study to assess the safety, pharmacokinetics, and pharmacodynamics of BOS161721 in healthy subjects. Clin Transl Sci. (2020) 13:337–44. doi: 10.1111/cts.12715
206. Study to Assess the Safety, Pharmacokinetics and Pharmacodynamics of BOS161721 in Healthy Subjects. Available online at: https://clinicaltrials.gov/study/NCT03036865?cond=NCT03036865&rank=1&tab=history&a=6version-content-panel (Accessed March 17, 2025).
207. Frederiksen KS, Lundsgaard D, Freeman JA, Hughes SD, Holm TL, Skrumsager BK, et al. IL-21 induces in vivo immune activation of NK cells and CD8+ T cells in patients with metastatic melanoma and renal cell carcinoma. Cancer Immunology Immunotherapy. (2008) 57:1439–49. doi: 10.1007/s00262-008-0479-4
208. Petrella TM, Tozer R, Belanger K, Savage KJ, Wong R, Smylie M, et al. Interleukin-21 has activity in patients with metastatic melanoma: a phase II study. J Clin Oncol. (2012) 30:3396–401. doi: 10.1200/JCO.2011.40.0655
209. Chapuis AG, Lee SM, Thompson JA, Roberts IM, Margolin KA, Bhatia S, et al. Combined IL-21–primed polyclonal CTL plus CTLA4 blockade controls refractory metastatic melanoma in a patient. J Exp Med. (2016) 213:1133–9. doi: 10.1084/jem.20152021
Keywords: interleukin-21 (IL-21), T lymphocyte, B lymphocyte, transplant rejection, antibody-mediated rejection (AMR)
Citation: Zeng X, Pan Y, Xia Q and He K (2025) The effects of interleukin-21 in the biology of transplant rejection. Front. Immunol. 16:1571828. doi: 10.3389/fimmu.2025.1571828
Received: 06 February 2025; Accepted: 09 April 2025;
Published: 01 May 2025.
Edited by:
Athanasia Mouzaki, University of Patras, GreeceReviewed by:
Idoia Gimferrer, Bloodworks Northwest Research Institute, United StatesCraig Alan Byersdorfer, University of Minnesota Medical Center, United States
Copyright © 2025 Zeng, Pan, Xia and He. This is an open-access article distributed under the terms of the Creative Commons Attribution License (CC BY). The use, distribution or reproduction in other forums is permitted, provided the original author(s) and the copyright owner(s) are credited and that the original publication in this journal is cited, in accordance with accepted academic practice. No use, distribution or reproduction is permitted which does not comply with these terms.
*Correspondence: Kang He, aGVrYW5nOTI5QDE2My5jb20=; Qiang Xia, eGlhcWlhbmdAc2hzbXUuZWR1LmNu