- 1Unidad de Microscopía Confocal, Instituto de Investigación Sanitaria Gregorio Marañón, Madrid, Spain
- 2Laboratorio de Inmuno-oncología, Instituto de Investigación Sanitaria Gregorio Marañón, Madrid, Spain
- 3Departamento de Inmunología, Universidad Complutense de Madrid, Madrid, Spain
- 4Servicio de Dermatología, Hospital General Universitario Gregorio Marañón, Madrid, Spain
- 5Servicio de Anatomía Patológica, Hospital General Universitario Gregorio Marañón, Madrid, Spain
Myeloid cells within tumor microenvironments exhibit significant heterogeneity and play a critical role in influencing clinical outcomes. In this study, we investigated the infiltration of various myeloid cell subtypes in a cohort of cutaneous melanomas, revealing no significant correlation between myeloid cell densities and the occurrence of distant metastasis. We further examined the phenotypic characteristics of primary melanoma tumor-associated macrophages (TAMs) utilizing the seven-phenotype classification recently proposed by Ma et al., derived from extensive pan-cancer single-cell RNA-sequencing studies. First, we analyzed the transcriptomic profile of TAMs isolated from stage IV metastasizing primary melanomas, alongside melanoma-conditioned monocytes cultured in vitro, both supporting the inflammatory cytokine-producing macrophage phenotype. Next, we employed multicolor fluorescence confocal microscopy, to assess the expression of TAM phenotype markers at the protein level in a cohort of primary melanoma samples. Notably, markers indicative of the inflammatory TAM phenotype, quantified at single-cell level, were significantly enriched in metastasizing tumors, demonstrating an independent correlation with shorter disease-free and overall survival (log-rank test, p< 0.0002). Additionally, our screening of phenotype markers expression revealed that PD-L1 positivity in tumor cells, rather than in TAMs, was associated with poor prognosis, highlighting a novel aspect of the immune landscape in cutaneous melanoma.
1 Introduction
Myeloid cells play a pivotal role in the progression of melanoma, a highly aggressive form of skin cancer (1, 2). These cells are involved in both immune surveillance and modulation of the tumor microenvironment (TME), contributing either to tumor control or its advancement. The main subtypes that may infiltrate the TME are tumor-associated macrophages (TAMs), myeloid-derived suppressor cells (MDSCs), dendritic cells (DCs), granulocytes such as tumor-associated neutrophils (TANs) and eosinophils, and mastocytes (3). The prognostic significance of myeloid cell subtypes in melanoma underscores their complex roles, since their presence or abundance may significantly shape clinical outcomes and therapeutic responses. Although several studies have associated the abundance of certain myeloid cells with prognosis in human melanoma, many rely on blood markers or small cohorts of primary tumors (4–6). Consequently, studies of primary tissues are yet to be conducted to determine their influence on prognosis and treatment response.
Macrophages progressively accumulate in most solid tumors, and an increased macrophage density is sometimes associated with poor prognosis in some types of cancer (7). The different roles of TAMs come from their ability to adapt to the abundant environmental signals in the tumor microenvironment, showing protumoral or antitumoral functions (8, 9). Although macrophage heterogeneity was initially described under two opposite phenotypes, M1 and M2, recent multi-omic analysis of macrophages in tumors like single-cell RNA-sequencing (scRNA-seq), metabolome and epigenome studies have shown that TAMs are highly heterogeneous, rendering potential distinct functional subsets (10, 11). Mulder et al. compiled 41 single-cell datasets from healthy and tumor tissues, defining numerous distinct clusters of monocytes and macrophages (MoMac-VERSE) (11). Studies such as that of Ma et al., which propose seven TAM phenotypes, reflect a significant effort to consolidate and standardize the nomenclature of TAM diversity (10). Although single-cell transcriptomic analyses allow the identification and classification of distinct TAM clusters based on gene expression, the protein-level expression of many of these genes remains largely unknown, as not all messenger RNA is translated into protein. Therefore, bridging the gap between transcriptomic macrophage types and protein expression in tumor tissues is crucial for defining distinct TAM phenotypes or functional states that may be associated with prognosis or treatment response. Furthermore, characterization of these subsets at a protein-level, including their prognostic value in early-stage melanoma, may benefit immunotherapy strategies (12).
Here we measured the density of myeloid cells in a cohort of paraffin-embedded primary melanomas and used protein expression data obtained through quantitative single-cell imaging to study the TAM heterogeneity and determine their prognostic potential in melanoma.
2 Materials and methods
2.1 RNA sequencing and transcriptomic analysis
Biopsied primary melanomas (n= 4) were homogenized and digested into single-cell suspensions (Tumor Dissociation Kit, Miltenyi Biotec), and TAMs were purified by magnetic cell sorting using CD14-microbeads (Miltenyi Biotec), as previously described (13, 14). On the other hand, healthy donor monocytes were obtained through a Ficoll-Paque gradient (Rafer) and isolated using CD14-microbeads. They were then cocultured in a six well plate at 5x105 cells/ml with BLM and A375 melanoma cell lines at a 1:2 ratio (melanoma:monocyte) for 24 hours. Cells were detached with Trypsin-EDTA (Sigma-Aldrich) and monocytes were isolated with CD14-microbeads. Once obtained (NucleoSpin RNA-purification kit, Macherey-Nagel Dueren, Germany), total RNA was processed and sequenced at BGI (https://www.bgitechsolutions.com) using the DNBseq-G400 platform. Datasets can be found at https://www.ncbi.nlm.nih.gov/geo/query/acc.cgi?acc=GSE171277 hosted at Gene Expression Omnibus (GSE171277) (15). DEGs were assessed by using DESeq2 clusterProfiler R package (16) according to gene signatures published in three previous works (10, 11, 17).
2.2 Cohort study and selection criteria
Patient samples were collected following the approval of the Gregorio Marañón Hospital ethics committee, and written informed consent was obtained for each patient. A formalin-fixed and paraffin-embedded (FFPE) primary cutaneous melanoma cohort of 88 samples was used, all >2 mm Breslow thickness and a median follow-up of 81 months, excised between 1998 and 2015 in our institution. This cohort included 35 samples from patients who were disease-free for at least 10 years of follow-up (non-metastasizing primary melanomas) and 53 clinically aggressive samples developing distant metastasis (metastasizing primary melanomas, with 33/53 melanoma-related deaths). Patients did not receive anti-tumoral treatment before melanoma resection, and only 15% of them were treated with interferon alpha after surgery, showing similar survival rates than untreated patients. Pathological American Joint Committee on Cancer staging II–IV assessment was obtained through sentinel lymph node biopsy and distant metastasis evaluation by computed tomography at the time of diagnosis. Metastasizing and non-metastasizing primary tumors had comparable Breslow thickness (Mann–Whitney, p = 0.37). Six patients at stage IV were excluded from disease-free survival analysis, but not from overall survival. Due to the value of this cohort, the number of patients screened for each marker was maintained at the minimum statistically necessary, ranging from 15 in non-expressed markers to up to 74 in the case of inflammatory TAMs, which deserved the staining of four independent markers for their classification. Screened samples were randomly chosen from the 88 available patients.
2.3 Multicolor fluorescence confocal microscopy
FFPE sections were deparaffinized and rehydrated, and antigens were retrieved by steaming in 10 mM sodium citrate buffer pH 9.0 (Dako, Glostrup, Denmark) for 7 minutes. Slides were blocked with 5 μg/ml human immunoglobulins solved in blocking serum-free medium (Dako) for 30 minutes and then incubated overnight at 4°C with 5–10 μg/ml primary antibodies (Supplementary Table S1), washed, and incubated with appropriate fluorescent secondary antibodies (Jackson Immunoresearch, West Grove, PA, USA) for 1 hour. Washes were performed in PBS containing 0.05% Tween-20. Single-cell quantification was performed for both density and protein expression at 3-5 20x fields. Mean fluorescence intensity (MFI) of proteins was obtained at manually depicted tumor cell nests or at automatically segmented CD68+, CD11b+ or CD66b+ cells using the ‘analyze particle’ plug-in of ImageJ2 software as previously shown (14, 18). A glycerol-immersion ACS_APO_20x/NA 0.60 objective was used for quantification, and an ACS_APO_10x/NA 0.30 objective for the panoramic view (SPE confocal microscope, Leica). To allow suitable triple-staining combinations, we used a novel anti-Activin A antibody (R&D, AF338, antibody #2). VEGFA, CCL20 and TNF raw data from a previous survival study (19) are shown here for the first time to detect the putative inflammatory-TAM phenotype of the MoMac-VERSE. Non-expressed markers in melanoma TAMs were antibody validated in other human control tissues (Supplementary Figure S1E). Ki67+ proliferating TAMs were so infrequent that could not be properly quantified by optical microscopy. Images are representative of markers co-staining, rather than density and/or clinical parameters.
2.4 Statistical analyses
Kaplan–Meier curves were used to analyze the correlation with patient disease-free and overall survivals using Youden’s index to determine where the cutoff point was equally specific and sensitive. The Cox regression method (univariate and multivariate) was used to identify independent prognostic variables and Mann–Whitney tests to evaluate the association with clinicopathological features. Spearman correlation and log-rank analyses were also used in this study (GraphPad software, San Diego, CA, USA), as indicated; p < 0.05 was considered statistically significant.
3 Results
3.1 Myeloid infiltration in primary melanomas
To investigate the infiltration of distinct myeloid cell populations in cutaneous melanoma, we quantified their density, both intratumorally and in the tumor periphery, in a cohort of paraffin-embedded primary melanomas (Figure 1A). Patients were classified as non-metastasizing or metastasizing, regarding subsequent development of metastasis during follow-up for 10 years. Quantification of markers for mastocytes (Triptase), TAMs (CD68), type 1 (CLEC9A) and type 2 (CD1c) DCs, TANs (CD66b), and eosinophils (Siglec-8) revealed no significant density differences associated with the metastatic behavior of the primary tumors (Figures 1B, C) or any other clinicopathological feature (Supplementary Table S2). TAMs were by far the most abundant myeloid cells both inside and outside the tumors (macrophages represented the 90% of CD11b+ myeloid cells), while DCs and eosinophils were absent or scarce, located mainly in the periphery of the tumor. Density of TANs was, however, more erratic at both inside and outside regions of the tumors, being sometimes enriched in the periphery of non-metastasizing lesions (p= 0.02). Further characterization of TANs revealed that they do not express CD15, though more than half expressed CD33, a marker commonly associated with less differentiated MDSCs (Figure 1B; Supplementary Figure S1A).
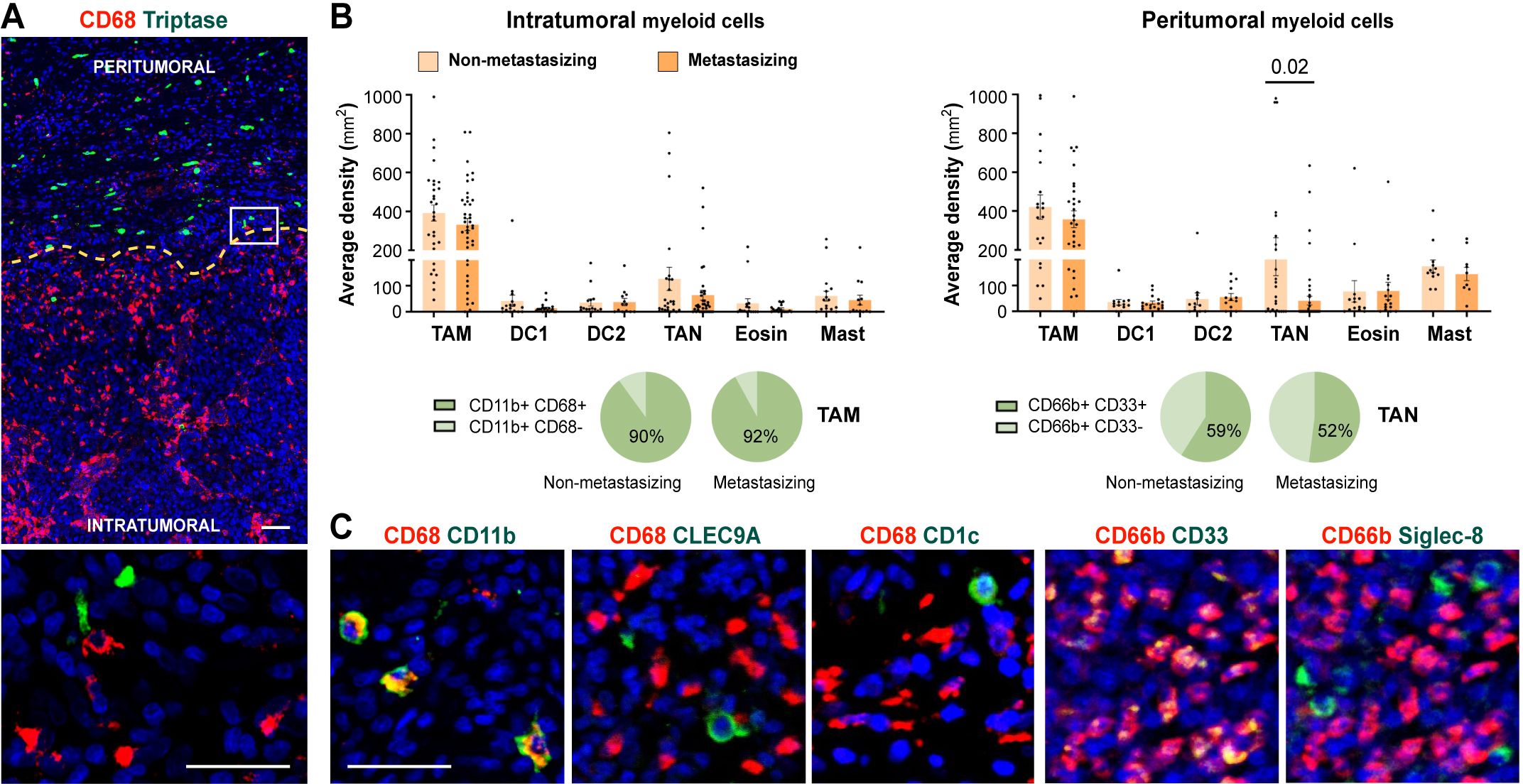
Figure 1. Myeloid cells density quantification in primary melanomas. (A) CD68+ TAMs (red) and Tryptase+ mast cells (green) enable primary melanoma delimitation of peritumoral and intratumoral areas. (B) Density quantification (mm2) of intratumoral and peritumoral myeloid cells comparing non-metastasizing and metastasizing primary melanomas. Percentages of CD11b+ CD68+/- and CD66b+ CD33+/- cells are shown, as indicated. (C) Representative images of CD68 (TAMs) and CD66b (TANs) in red, and CD11b (myeloid), CLEC9A (cDC1), CD1c (cDC2), or CD33 (MDSC) and Siglec-8 (eosinophil), in green. Dapi-stained nuclei, in blue. Scale bars, 50 μm.
3.2 Primary melanoma macrophages and melanoma-conditioned monocytes acquire inflammatory cytokine-enriched TAM signature
Although the number of infiltrating TAMs does not vary between metastasizing and non-metastasizing tumors, crosstalk between TAMs and tumor cells (TCs) may induce the acquisition of gene expression profiles that may promote tumor progression (14). TAMs isolated from primary melanomas of stage IV patients (who had metastatic disease at the time of primary tumor removal), and therefore bona fide pro-metastatic TAMs, along with monocytes co-cultured for 24 hours with two different melanoma cell lines (BLM or A375), were subjected to RNA-sequencing to analyze their transcriptional profiles. Differentially expressed genes (DEGs) were subsequently analyzed for gene set enrichment using recently compiled TAM signatures by single-cell RNA-seq (scRNA-seq) (10, 11, 17) (Supplementary Figure S1B; Supplementary Table S3). Both melanoma TAMs and melanoma-conditioned monocytes exhibited upregulated DEGs associated with cytokine-enriched inflammatory TAM phenotypes (named Inflam-TAM and Inflam-Cluster #15), alongside with a significant enrichment of the extracellular matrix-Cluster #13 of the MoMac-VERSE (Figures 2A, B). Conversely, both melanoma cell lines conditioned monocytes shared a significant downregulation of DEGs associated with the lipid-associated TAM phenotype (LA-TAM and LA-Cluster #3) (Figures 2A, B; Supplementary Figure S1C).
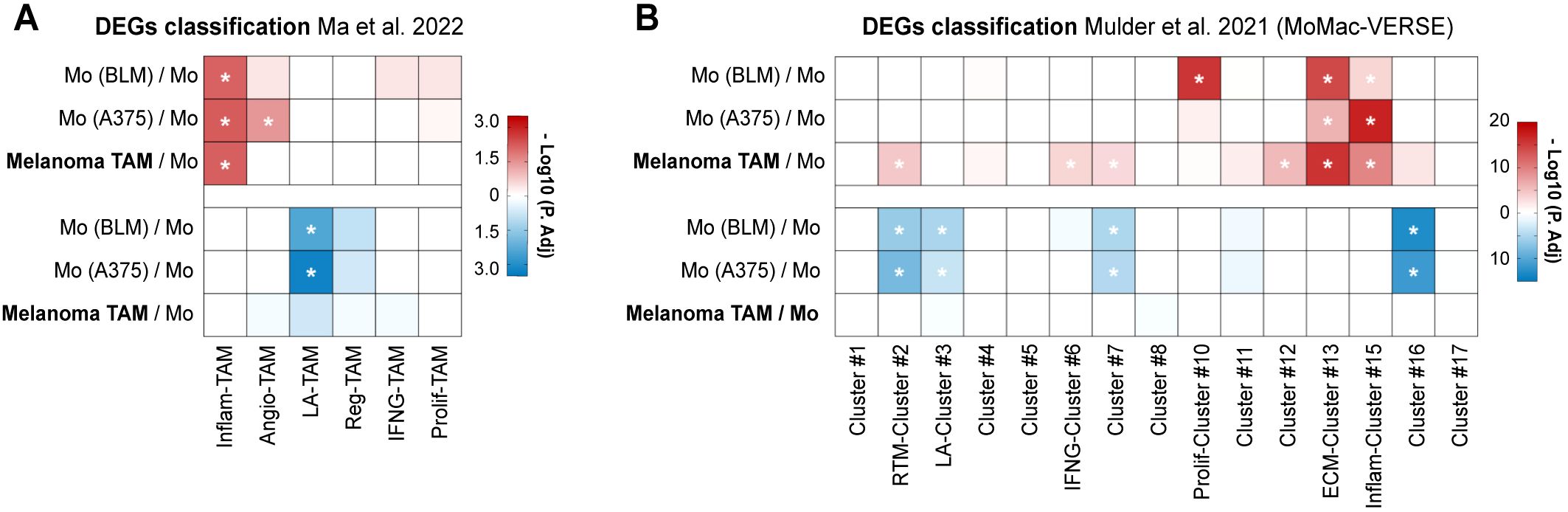
Figure 2. TAMs isolated from metastasizing primary melanomas show upregulated inflammatory gene signature. Differential expressed genes (DEGs) of TAMs isolated from stage IV primary melanomas (n= 4) and monocytes co-cultured with BLM and A375 melanoma cell lines (n= 3), compared to healthy donor monocytes, were classified according to gene sets proposed by Ma et al. (A) and Mulder et al. (B). Heatmaps summarize the adjusted-p values for both upregulated (red) and downregulated (blue) DEG classification. *, P. adj <0.01.
3.3 Single-cell protein expression of TAM markers proposed by scRNA-seq in primary melanomas and prognostic relevance of TC PD-L1
Since our transcriptomic analysis suggested that TAMs from stage IV metastasizing melanomas acquire a specific inflammatory profile among several other possible phenotypes, we designed a panel of antibodies to identify at the protein level the diverse macrophage types defined by Ma et al. at the transcriptional level. With selected antibodies, representative of each macrophage subset, we performed multiplex staining of our cohort of primary melanomas to explore their clinical significance (Figure 3A). The single-cell analysis of macrophage types was performed using multicolor fluorescence confocal microscopy, measuring the protein expression of the different markers in CD68+ TAMs, or in TCs if the studied marker was also expressed (Figures 3B–D). TAM expression of CCL8 and IDO was previously studied in the same cohort, showing no differences between non-metastasizing and metastasizing tumors (14, 18), unlike the expression of VEGFA, CCL20, TNF and Activin A, which was significantly increased in metastasizing samples (p< 0.0001, Figure 3B). As VEGFA and CCL20 are shared markers by the inflammatory and the angiogenic macrophage subsets, we analyzed the expression of specific angiogenic markers HIF1A and FLT1. None of them showed detectable expression in melanoma TAMs, which was verified by proving the validity of the antibody in other human tissue macrophages (Figures 3B; Supplementary Figures S1D, E). CXCL9, CXCL10, PD-L1, CD86, IL-10, TREM2, GPNMB, IL-4l1 and FOLR2 markers showed widespread expression by most melanoma TAMs, with no definition of a particular macrophage subset, and regardless of whether the tumor was metastasizing or not (Figures 3B, D; Supplementary Figure S1D). Interestingly, SPP1 was expressed by a small subset of macrophages, defining a unique type of TAM (Supplementary Figure S1F).
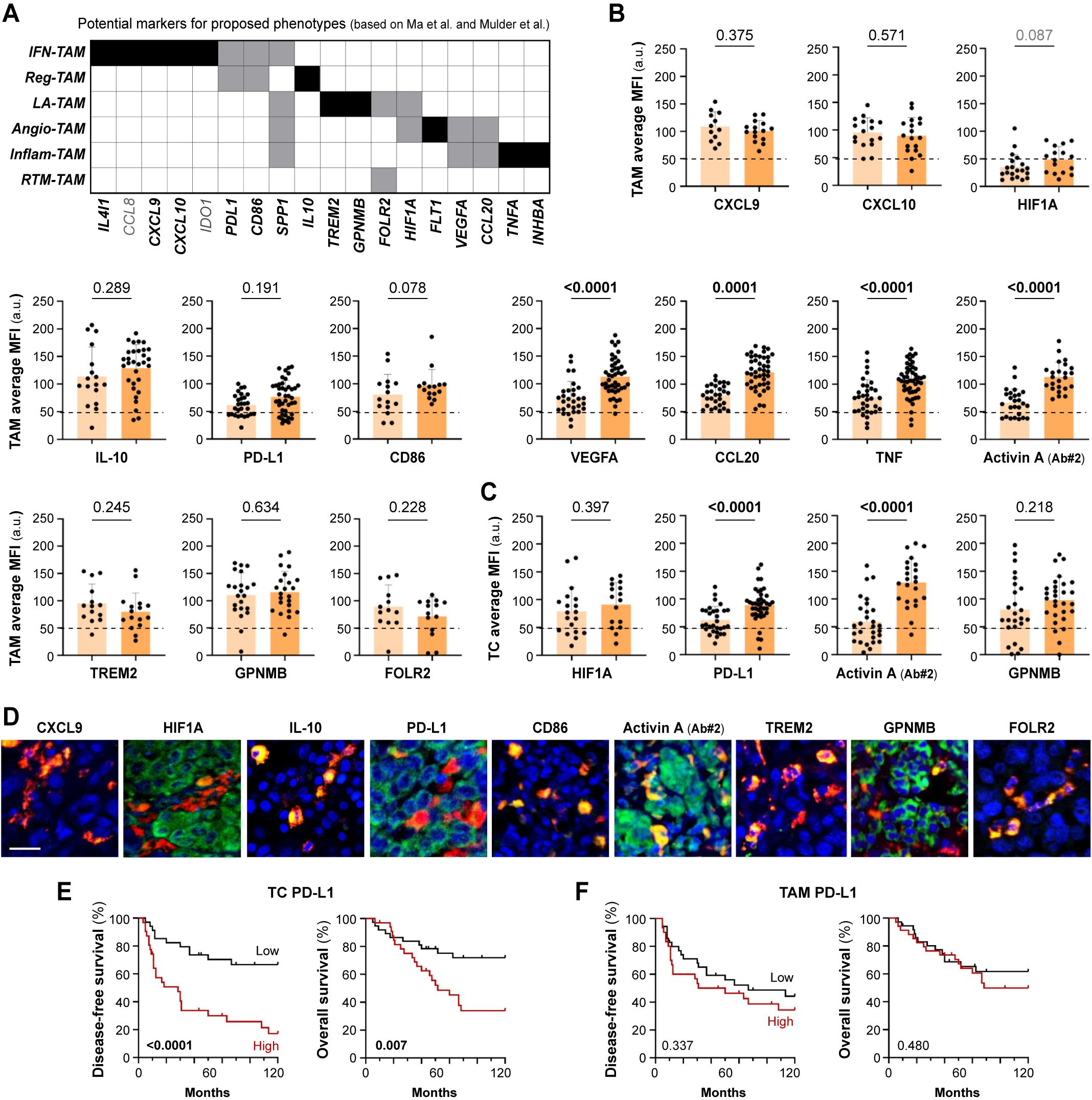
Figure 3. Protein expression of potential markers according to proposed TAM phenotypes. (A) Summary of potential markers for each proposed TAM phenotype (IFN-TAM, Reg-TAM, LA-TAM, Angio-TAM, Inflam-TAM and RTM-TAM), based on scRNA-seq pan-cancer compilations (Ma et al. and Mulder et al.) (B, C). Single-cell protein quantification of CXCL9, CXCL10, HIF1A, IL-10, PD-L1, CD86, VEGFA, CCL20, TNF, Activin A, TREM2, GPNMB and FOLR2 at TAMs (B) or TCs (C), in non-metastasizing and metastasizing primary melanomas. Average mean fluorescence intensities (MFI) by patient are shown (arbitrary units, a.u.). Mann–Whitney p values, are indicated. (D). Representative images of CD68+ TAMs (red) co-stained with indicated markers (green). Scale bar, 50 μm. (E, F). Disease-free (E) and overall (F) survival Kaplan-Meier curves. Youden’s index was used to choose a cutoff point to classify primary melanomas as ‘low’ (black) or ‘high’ (red) for PD-L1 expression (n= 65) by TAMs (90 a.u.) and TCs (85 a.u.). Log-rank p values are shown.
Several of the markers selected to study TAM heterogeneity, including HIF1A, GMNMB, Activin A, and PD-L1, were also found to be expressed by cancer cells. Unlike PD-L1 expression by TAMs, PD-L1 expression by TC was found to be enriched in metastasizing melanomas (Figures 3C, D). We used the Kaplan-Meier method to assess the clinical relevance of PD-L1 expression by TCs in primary melanomas. Samples were stratified as ‘high’ or ‘low’ expression to calculate 10-year disease-free (DFS) and overall (OS) survival curves. Tumor cell PD-L1 expression correlated with shorter DFS (Log Rank, p< 0.0001) and OS (p= 0.007) in cutaneous melanoma (Figures 3E, F). Activin A expression by TCs and TAMs was previously reported to be associated with poor prognosis of melanoma patients (14), as confirmed here with a different anti-Activin A antibody (Figures 3D–F). Macrophage expression of Activin A at single cell level correlated positively with the expression of the inflammatory marker TNF (Spearman r= 0.52, p< 0.001), but not with PD-L1, FOLR2 or HIF1A which, as shown previously, were generally expressed by most TAMs (Supplementary Figure S1G). This suggests a notable commonality in TAM differentiation towards an inflammatory activation path in metastasizing samples, rather than enrichment in particular macrophage subtypes.
3.4 Inflammatory cytokine-producing TAMs as activation biomarkers of poor prognosis in cutaneous melanoma
As both transcriptomic and single-cell protein analyses suggest the existence of an inflammatory cytokine-producing stage of pro-metastatic TAMs, we decided to perform a survival analysis using all four markers (Activin A, TNF, VEGFA, CCL20) simultaneously for sample stratification. Patients were stratified into two groups, those who highly expressed just 0–1 of the studied cytokines (non-inflammatory TAMs) vs 2–4 cytokine markers (inflammatory TAMs). Kaplan-Meier curves showed a strong correlation between inflammatory TAMs and DFS (p <0.0001) and OS (p= 0.0002) (Figure 4A). Furthermore, to determine whether the presence of inflammatory cytokine-producing TAMs and the expression of PD-L1 by TCs were independent prognostic factors, we performed a multivariate regression analysis including different clinicopathological features, such as age, gender, Breslow and staging. This analysis showed that the presence of inflammatory TAMs in tumors was an independent prognostic factor for DFS (p <0.0001) and OS (p= 0.006), as well as cancer cell PD-L1 (Table 1). Altogether, our findings suggest the potential of the inflammatory cytokine-producing path of TAM activation and the expression of Activin A or PD-L1 by TCs as clinically relevant biomarkers for patient stratification (Figures 4B, C).
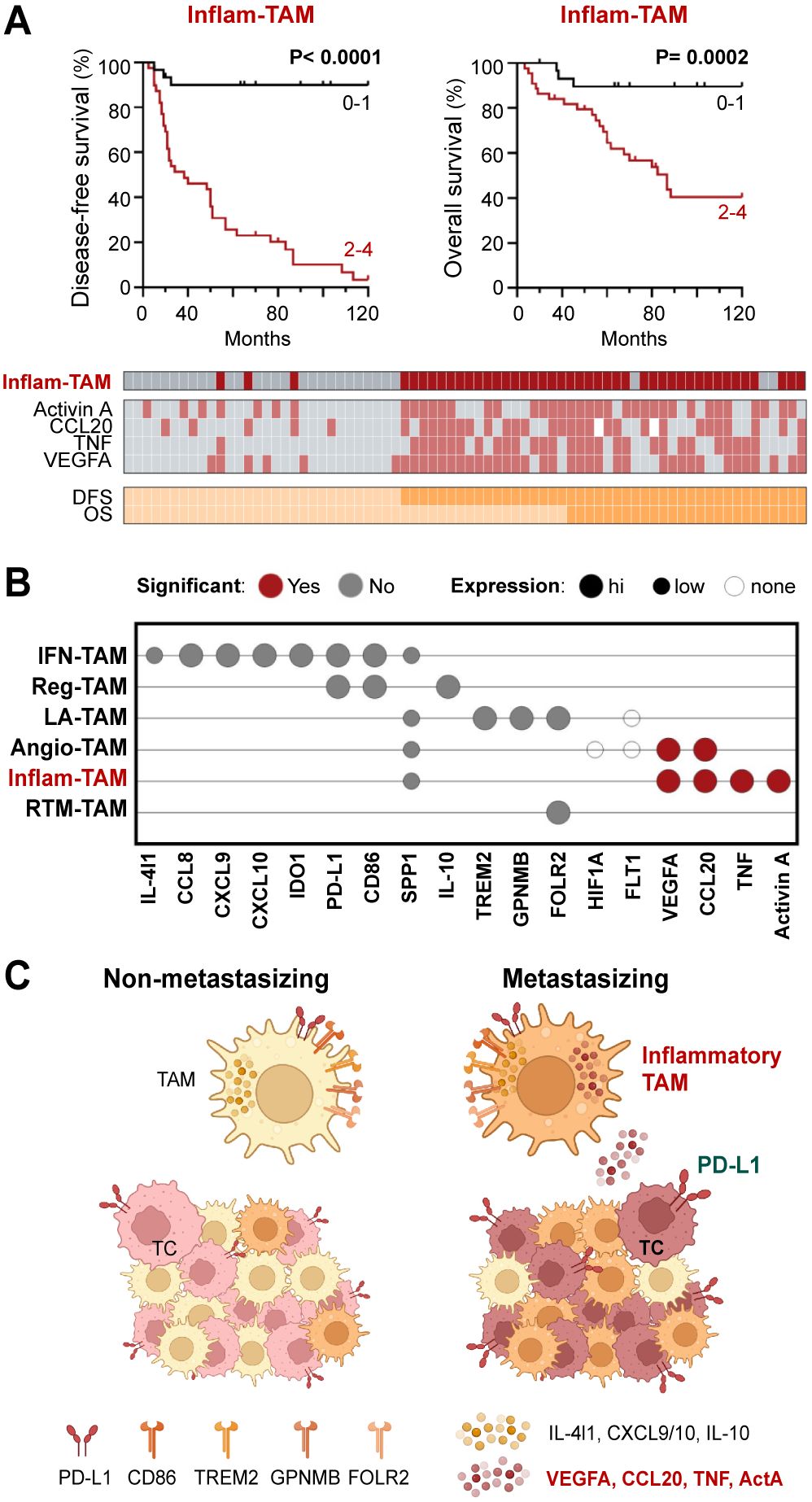
Figure 4. Inflammatory cytokine-producing TAMs (Inflam-TAMs) are associated with poor prognosis in cutaneous melanoma. (A) Disease-free and overall survival Kaplan–Meier curves. Primary melanomas were classified into two groups: expression of none-1 vs expression of 2–4 markers (Activin A, CCL20, TNF, VEGFA). Classification and expression of each marker in all analyzed primary tumors is shown. (B) Summary of the general (high, low, none) and relative (non-metastasizing vs metastasizing samples, Mann-Whitney p <0.01) expression of TAM markers at the protein level. (C) Resulting summarizing model, created with BioRender.com.
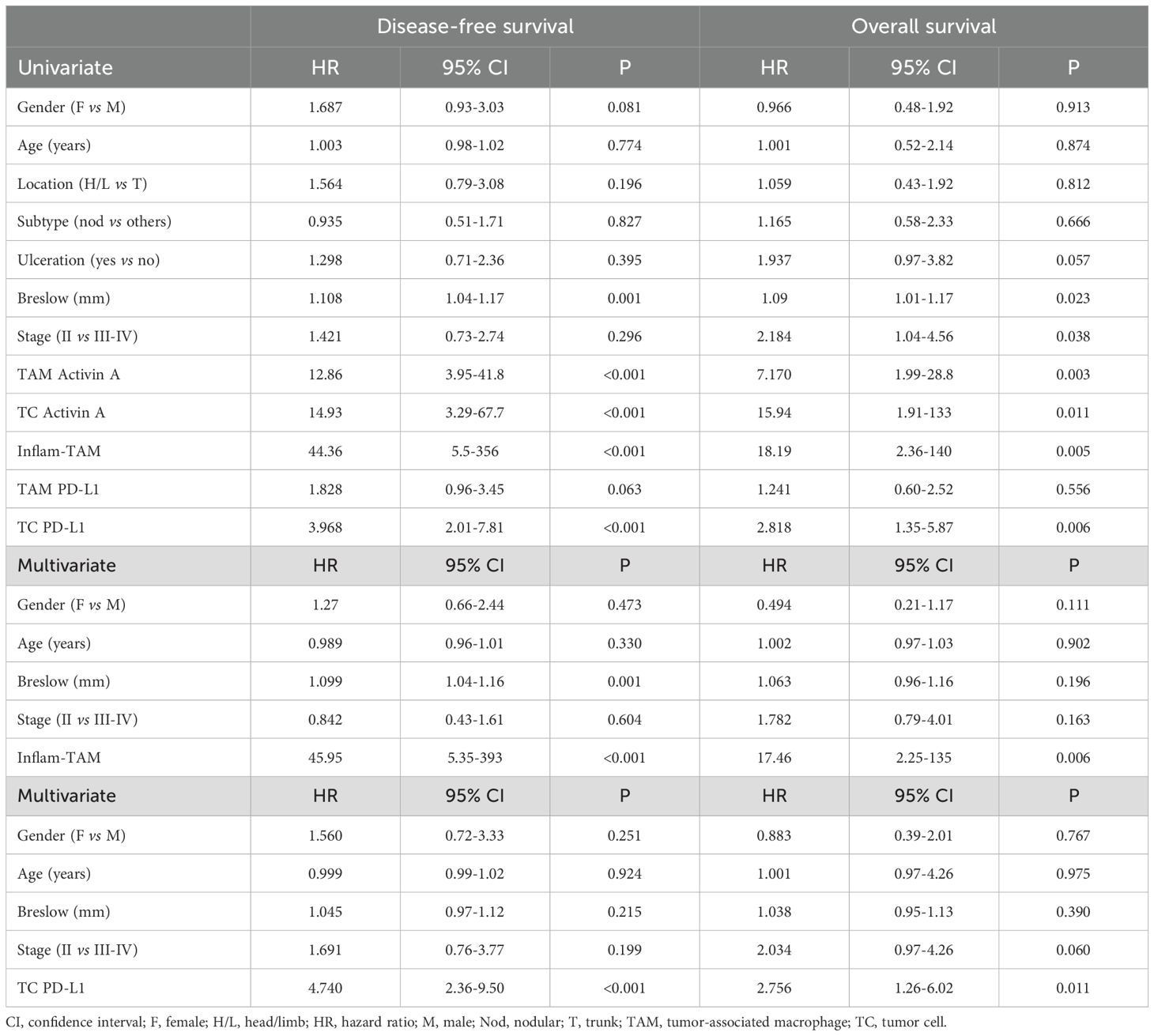
Table 1. Univariate and multivariate Cox regression analyses for 10-year disease-free and overall survival.
4 Discussion
The innate immune system, as the first line of defense, is essential for controlling early tumor progression and initiating adaptive immune responses that ensure long-term tumor-specific immunity. Myeloid cells exhibit a remarkable plasticity which allows them to adopt either pro-tumoral or anti-tumoral roles depending on the surrounding cellular environment (2). Here the presence of myeloid populations was analyzed within and around tumors through a cohort of stage II-IV primary melanomas. TAMs constituted the main subset of myeloid cells in both compartments, while the rest of the subpopulations were a minority and preferentially located in the periphery of the tumor. TANs were an exception, since when present, they tended to form dense groups concentrated in specific regions of the tumor, mainly in the periphery of non-metastasizing lesions. This contrasts with a previous report in a collection of earlier-stage I-II melanomas, where the presence of intratumoral CD66b+ neutrophils correlated with worse prognosis (20). Nevertheless, neutrophils have shown both anti- and pro-tumorigenic functions (21) and tumor staging might explain these differences.
The emergence of -omic technologies has significantly accelerated the detailed characterization of macrophages, proving that macrophage phenotypes are more complex than thought before, and that the M1-M2 classification is overly simplistic (11, 22). Numerous studies have aimed to identify key molecular signatures to classify the heterogeneity of TAMs. Notably, two recent large-scale pan-cancer scRNA-seq analyses identified multiple potential subsets of TAMs. Although different nomenclatures were used in these two and other studies, similar molecular patterns were identified and a posterior review analysis unified the results and proposed that seven TAM subsets are conserved across nearly all cancer types (10). Despite not being single-cell, our bulk RNA-seq data obtained from TAMs isolated from already metastasized primary melanomas and from monocytes conditioned by melanoma cell lines, correlated significantly with the inflammatory cytokine-enriched TAM (inflam-TAM) phenotype (10, 11). Remarkably, several markers of this specific phenotype overlapped with our previously identified prognostic secretory signature (VEGFA, CCL20, TNF, Activin A), which proved to be triggered by melanoma cells via NFkB signaling pathway and sustained by the axis Activin A>Smad2/3 (13, 14, 19). Altogether our transcriptomic and single-TAM proteomic analyses suggest that the inflam-TAM phenotype from the MoMac-VERSE holds prognostic significance in melanoma and play most probably critical pro-metastatic and immune-suppressive roles.
Protein markers IL-4I1, CCL8, CXCL9, CXCL10 and IDO, that would allow the detection of interferon-primed TAMs (IFN-TAMs, a phenotype enriched in typical M1-like genes), were generally expressed in TAMs from both non-metastasizing and metastasizing tumors. Although none of these markers were associated with prognosis in our cohort of primary melanomas, the presence of this TAM phenotype has been previously associated with the response to immunotherapy in metastatic melanoma (17, 23). Of note, in these studies interferon-primed TAMs are named inflammatory, which may lead to confusion with the inflammatory cytokine-enriched TAMs described by Ma et al. While multi-omics approaches have significantly advanced our understanding of cellular diversity, the absence of a unified nomenclature across studies, often assigning arbitrary names to cell clusters, risks creating misinterpretation and hindering cross-study comparisons.
Other markers expressed by both IFN-TAMs and immune regulatory TAMs (Reg-TAMs) are PD-L1 and CD86. Remarkably, despite no expression differences were found regarding TAM expression, PD-L1 expression by melanoma cells proved to be an independent prognostic biomarker, showing a strong negative correlation with both disease-free and overall survival. There are conflicting data from several studies regarding the prognostic value of PD-L1 expression in melanoma. A meta-analysis of these studies determined that its expression did not predict prognosis (24), however, the expression of this immune checkpoint protein was determined by classical non-quantitative IHC and distinction between TAMs and TCs was not considered. Furthermore, PD-L1 expression is also being studied as a predictive biomarker for immunotherapy in melanoma (25), so quantitative image analysis together with separate analysis of TAMs and TCs should be considered from the point of view of treatment direction. In this regard, it should be considered that enriched expression of PD-L1 on melanoma cells may directly engage PD-1+ TAM functionality and its ability to organize a coordinated anti-tumor response with CD8 T cells (26–28).
Lipid-associated TAM (LA-TAM) are characterized by the expression of lipid-related genes such as TREM2. This protein has been described as immunosuppressive in several types of cancer such as lung, colorectal and breast cancer (29–31). Another LA-TAM marker in blood-circulating monocytes, GPNMB, correlated with melanoma staging (32) and its expression by breast cancer TCs has been described as a prognostic indicator of recurrence (33). However, we did not detect expression differences for these proteins in our melanoma cohort, nor did we observe them for FOLR2, which was also widely expressed by the majority of macrophages, suggesting that this protein would not be an appropriate marker to identify the resident tumor-like TAM (RTM-TAM) subset in melanoma. In the same line, no differences were previously found for FOLR2 expression when comparing homeostatic and inflamed tissues, where the protein was broadly expressed by most of human tissue macrophages (34). Finally, despite pro-angiogenic TAMs (Angio-TAMs) have been well described in different tumors such as head and neck squamous cell carcinoma (35), their associated markers were barely detected in our melanoma cohort, suggesting that VEGFA expression might be associated with the inflammatory phenotype rather than the angiogenic subset.
Recent advances in single-cell RNA sequencing have provided a static view of TAM diversity, leading to new molecular definitions of macrophage states with potential prognostic and predictive significance in immuno-oncology. Although gene expression analysis yields valuable information, it does not consistently correlate with the presence of actual proteins within the tissue, so transcriptomic conclusions must be validated at the protein level (10, 36). Moreover, it remains critical to investigate the biological relevance and clinical impact of TAM subsets in larger patient cohorts, particularly in relation to their role in immunotherapy response.
Along with the heterogeneity of macrophage subsets, there are defined stages along four conserved paths of macrophage activation in tissues (37). These activation stages include a “phagocytic” regulatory path, an “inflammatory” cytokine-producing path, an “oxidative stress” antimicrobial path, or a “remodeling” extracellular matrix (ECM) deposition path. Our results indicate that an inflammatory “cytokine-producing” stage of most TAMs, rather than enrichment in a particular macrophage subset is associated with poor patient prognosis in cutaneous melanoma.
Data availability statement
The datasets presented in this study can be found in online repositories. The names of the repository/repositories and accession number(s) can be found in the article/Supplementary Material.
Ethics statement
The studies involving humans were approved by COMITÉ de ÉTICA DE LA INVESTIGACIÓN con MEDICAMENTOS HOSPITAL GREGORIO MARAÑÓN. The studies were conducted in accordance with the local legislation and institutional requirements. The participants provided their written informed consent to participate in this study. Ethical approval was not required for the studies on animals in accordance with the local legislation and institutional requirements because only commercially available established cell lines were used.
Author contributions
CB-A: Conceptualization, Data curation, Formal Analysis, Investigation, Methodology, Project administration, Supervision, Validation, Writing – original draft, Writing – review & editing. AN-V: Conceptualization, Data curation, Formal Analysis, Investigation, Methodology, Project administration, Validation, Writing – review & editing. LB-R: Formal Analysis, Investigation, Writing – review & editing. JA-I: Data curation, Resources, Writing – review & editing. VP-B: Data curation, Resources, Writing – review & editing. PS-M: Conceptualization, Funding acquisition, Resources, Writing – original draft, Writing – review & editing. RS: Conceptualization, Formal Analysis, Funding acquisition, Investigation, Methodology, Project administration, Resources, Supervision, Validation, Writing – original draft, Writing – review & editing.
Funding
The author(s) declare that financial support was received for the research and/or publication of this article. This work was supported by the Ministry of Science and Innovation PID2021-123507OB-100 grant (PS-M, RS) co-financed by the Cancer Research UK (C18915/A29362), FCAECC and AIRC under the Accelerator Award Programme CB-A and AN-V were Financed by the Comunidad de Madrid YEI (Youth Employment Initiative) program.
Conflict of interest
The authors declare that the research was conducted in the absence of any commercial or financial relationships that could be construed as a potential conflict of interest.
Generative AI statement
The author(s) declare that no Generative AI was used in the creation of this manuscript.
Publisher’s note
All claims expressed in this article are solely those of the authors and do not necessarily represent those of their affiliated organizations, or those of the publisher, the editors and the reviewers. Any product that may be evaluated in this article, or claim that may be made by its manufacturer, is not guaranteed or endorsed by the publisher.
Supplementary material
The Supplementary Material for this article can be found online at: https://www.frontiersin.org/articles/10.3389/fimmu.2025.1587545/full#supplementary-material
References
1. Cheng X, Wang H, Wang Z, Zhu B, Long H. Tumor-associated myeloid cells in cancer immunotherapy. J Hematol Oncol. (2023) 16:71. doi: 10.1186/s13045-023-01473-x
2. Trocchia M, Ventrici A, Modestino L, Cristinziano L, Ferrara AL, Palestra F, et al. Innate immune cells in melanoma: implications for immunotherapy. Int J Mol Sci. (2024) 25. doi: 10.3390/ijms25158523
3. Mantovani A, Marchesi F, Jaillon S, Garlanda C, Allavena P. Tumor-associated myeloid cells: diversity and therapeutic targeting. Cell Mol Immunol. (2021) 18:566–78. doi: 10.1038/s41423-020-00613-4
4. Ladanyi A. Prognostic and predictive significance of immune cells infiltrating cutaneous melanoma. Pigment Cell Melanoma Res. (2015) 28:490–500. doi: 10.1111/pcmr.2015.28.issue-5
5. Gartrell RD, Marks DK, Hart TD, Li G, Davari DR, Wu A, et al. Quantitative analysis of immune infiltrates in primary melanoma. Cancer Immunol Res. (2018) 6:481–93. doi: 10.1158/2326-6066.CIR-17-0360
6. Zilberg C, Ferguson AL, Lyons JG, Gupta R, Damian DL. The tumor immune microenvironment in primary cutaneous melanoma. Arch Dermatol Res. (2025) 317:273. doi: 10.1007/s00403-024-03758-8
7. Cassetta L, Pollard JW. A timeline of tumour-associated macrophage biology. Nat Rev Cancer. (2023) 23:238–57. doi: 10.1038/s41568-022-00547-1
8. Glass CK, Natoli G. Molecular control of activation and priming in macrophages. Nat Immunol. (2016) 17:26–33. doi: 10.1038/ni.3306
9. Lopez-Janeiro A, Padilla-Ansala C, De Andrea CE, Hardisson D, Melero I. Prognostic value of macrophage polarization markers in epithelial neoplasms and melanoma. A systematic Rev meta-analysis. Mod Pathol. (2020) 33:1458–65. doi: 10.1038/s41379-020-0534-z
10. Ma RY, Black A, Qian BZ. Macrophage diversity in cancer revisited in the era of single-cell omics. Trends Immunol. (2022) 43:546–63. doi: 10.1016/j.it.2022.04.008
11. Mulder K, Patel AA, Kong WT, Piot C, Halitzki E, Dunsmore G, et al. Cross-tissue single-cell landscape of human monocytes and macrophages in health and disease. Immunity. (2021) 54:1883–1900.e5. doi: 10.1016/j.immuni.2021.07.007
12. Swetter SM, Tsao H, Bichakjian CK, Curiel-Lewandrowski C, Elder DE, Gershenwald JE, et al. Guidelines of care for the management of primary cutaneous melanoma. J Am Acad Dermatol. (2019) 80:208–50. doi: 10.1016/j.jaad.2018.08.055
13. Samaniego R, Gutierrez-Gonzalez A, Gutierrez-Seijo A, Sanchez-Gregorio S, Garcia-Gimenez J, Mercader E, et al. CCL20 expression by tumor-associated macrophages predicts progression of human primary cutaneous melanoma. Cancer Immunol Res. (2018) 6:267–75. doi: 10.1158/2326-6066.CIR-17-0198
14. Gutierrez-Seijo A, Garcia-Martinez E, Barrio-Alonso C, Parra-Blanco V, Aviles-Izquierdo JA, Sanchez-Mateos P, et al. Activin A sustains the metastatic phenotype of tumor-associated macrophages and is a prognostic marker in human cutaneous melanoma. J Invest Dermatol. (2022) 142:653–661 e2. doi: 10.1016/j.jid.2021.07.179
15. Gutiérrez Seijo A, Samaniego García R, Sánchez-Mateos P, Barrio Alonso C, García Martínez E. Characterization of tumor associated macrophages (TAM) in the tumor microenvironment of human melanoma(2021). Available online at: https://www.ncbi.nlm.nih.gov/geo/query/acc.cgi?acc=GSE171277.
16. Wu T, Hu E, Xu S, Chen M, Guo P, Dai Z, et al. clusterProfiler 4.0: A universal enrichment tool for interpreting omics data. Innovation (Camb). (2021) 2:100141. doi: 10.1016/j.xinn.2021.100141
17. Wei C, Ma Y, Wang M, Wang S, Yu W, Dong S, et al. Tumor-associated macrophage clusters linked to immunotherapy in a pan-cancer census. NPJ Precis Oncol. (2024) 8:176. doi: 10.1038/s41698-024-00660-4
18. Barrio-Alonso C, Nieto-Valle A, Garcia-Martinez E, Gutierrez-Seijo A, Parra-Blanco V, Marquez-Rodas I, et al. Chemokine profiling of melanoma-macrophage crosstalk identifies CCL8 and CCL15 as prognostic factors in cutaneous melanoma. J Pathol. (2024) 262:495–504. doi: 10.1002/path.6252
19. Gutierrez-Seijo A, Garcia-Martinez E, Barrio-Alonso C, Pareja-Malagon M, Acosta-Ocampo A, Fernandez-Santos ME, et al. CCL20/TNF/VEGFA cytokine secretory phenotype of tumor-associated macrophages is a negative prognostic factor in cutaneous melanoma. Cancers (Basel). (2021) 13. doi: 10.3390/cancers13163943
20. Jensen TO, Schmidt H, Moller HJ, Donskov F, Hoyer M, Sjoegren P, et al. Intratumoral neutrophils and plasmacytoid dendritic cells indicate poor prognosis and are associated with pSTAT3 expression in AJCC stage I/II melanoma. Cancer. (2012) 118:2476–85. doi: 10.1002/cncr.v118.9
21. Quail DF, Amulic B, Aziz M, Barnes BJ, Eruslanov E, Fridlender ZG, et al. Neutrophil phenotypes and functions in cancer: A consensus statement. J Exp Med. (2022) 219. doi: 10.1084/jem.20220011
22. Cheng S, Li Z, Gao R, Xing B, Gao Y, Yang Y, et al. A pan-cancer single-cell transcriptional atlas of tumor infiltrating myeloid cells. Cell. (2021) 184:792–809.e23. doi: 10.1016/j.cell.2021.01.010
23. Elewaut A, Estivill G, Bayerl F, Castillon L, Novatchkova M, Pottendorfer E, et al. Cancer cells impair monocyte-mediated T cell stimulation to evade immunity. Nature. (2025) 637:716–25. doi: 10.1038/s41586-024-08257-4
24. Yang J, Dong M, Shui Y, Zhang Y, Zhang Z, Mi Y, et al. A pooled analysis of the prognostic value of PD-L1 in melanoma: evidence from 1062 patients. Cancer Cell Int. (2020) 20:96. doi: 10.1186/s12935-020-01187-x
25. Fiorentino V, Pizzimenti C, FranChina M, Pepe L, Russotto F, Tralongo P, et al. Programmed cell death ligand 1 immunohistochemical expression and cutaneous melanoma: A controversial relationship. Int J Mol Sci. (2024) 25. doi: 10.3390/ijms25010676
26. Peranzoni E, Lemoine J, Vimeux L, Feuillet V, Barrin S, Kantari-Mimoun C, et al. Macrophages impede CD8 T cells from reaching tumor cells and limit the efficacy of anti-PD-1 treatment. Proc Natl Acad Sci U S A. (2018) 115:E4041–50. doi: 10.1073/pnas.1720948115
27. Strauss L, Mahmoud MAA, Weaver JD, Tijaro-Ovalle NM, Christofides A, Wang Q, et al. Targeted deletion of PD-1 in myeloid cells induces antitumor immunity. Sci Immunol. (2020) 5. doi: 10.1126/sciimmunol.aay1863
28. Klement JD, Redd PS, Lu C, Merting AD, Poschel DB, Yang D, et al. Tumor PD-L1 engages myeloid PD-1 to suppress type I interferon to impair cytotoxic T lymphocyte recruitment. Cancer Cell. (2023) 41:620–636.e9. doi: 10.1016/j.ccell.2023.02.005
29. Katzenelenbogen Y, Sheban F, Yalin A, Yofe I, Svetlichnyy D, Jaitin DA, et al. Coupled scRNA-seq and intracellular protein activity reveal an immunosuppressive role of TREM2 in cancer. Cell. (2020) 182:872–885.e19. doi: 10.1016/j.cell.2020.06.032
30. Molgora M, Esaulova E, Vermi W, Hou J, Chen Y, Luo J, et al. TREM2 modulation remodels the tumor myeloid landscape enhancing anti-PD-1 immunotherapy. Cell. (2020) 182:886–900.e17. doi: 10.1016/j.cell.2020.07.013
31. Park MD, Reyes-Torres I, Leberichel J, Hamon P, Lamarche NM, Hegde S, et al. TREM2 macrophages drive NK cell paucity and dysfunction in lung cancer. Nat Immunol. (2023) 24:792–801. doi: 10.1038/s41590-023-01475-4
32. Turrentine J, Chung JS, Nezafati K, Tamura K, Harker-Murray A, Huth J, et al. DC-HIL+ CD14+ HLA-DR no/low cells are a potential blood marker and therapeutic target for melanoma. J Invest Dermatol. (2014) 134:2839–42. doi: 10.1038/jid.2014.248
33. Rose AA, Grosset AA, Dong Z, Russo C, Macdonald PA, Bertos NR, et al. Glycoprotein nonmetastatic B is an independent prognostic indicator of recurrence and a novel therapeutic target in breast cancer. Clin Cancer Res. (2010) 16:2147–56. doi: 10.1158/1078-0432.CCR-09-1611
34. Samaniego R, Palacios BS, Domiguez-Soto A, Vidal C, Salas A, Matsuyama T, et al. Macrophage uptake and accumulation of folates are polarization-dependent in vitro and in vivo and are regulated by activin A. J Leukoc Biol. (2014) 95:797–808. doi: 10.1189/jlb.0613345
35. Wu J, Shen Y, Zeng G, Liang Y, Liao G. SPP1(+) TAM subpopulations in tumor microenvironment promote intravasation and metastasis of head and neck squamous cell carcinoma. Cancer Gene Ther. (2024) 31:311–21. doi: 10.1038/s41417-023-00704-0
36. Jiang L, Wang M, Lin S, Jian R, Li X, Chan J, et al. A quantitative proteome map of the human body. Cell. (2020) 183:269–283.e19. doi: 10.1016/j.cell.2020.08.036
Keywords: tumor-associated macrophages, inflammatory cytokine-producing TAMs, prognostic factor, PD-L1, myeloid cells, cutaneous melanoma
Citation: Barrio-Alonso C, Nieto-Valle A, Barandalla-Revilla L, Avilés-Izquierdo JA, Parra-Blanco V, Sánchez-Mateos P and Samaniego R (2025) Translating genetics into tissue: inflammatory cytokine-producing TAMs and PD-L1 tumor expression as poor prognosis factors in cutaneous melanoma. Front. Immunol. 16:1587545. doi: 10.3389/fimmu.2025.1587545
Received: 04 March 2025; Accepted: 22 April 2025;
Published: 08 May 2025.
Edited by:
Pedro Berraondo, Cima Universidad de Navarra, SpainReviewed by:
Katarina Mirjacic Martinovic, Institute of Oncology and Radiology of Serbia, SerbiaIrma Olarte, Hospital General de México Dr. Eduardo Liceaga, Mexico
Copyright © 2025 Barrio-Alonso, Nieto-Valle, Barandalla-Revilla, Avilés-Izquierdo, Parra-Blanco, Sánchez-Mateos and Samaniego. This is an open-access article distributed under the terms of the Creative Commons Attribution License (CC BY). The use, distribution or reproduction in other forums is permitted, provided the original author(s) and the copyright owner(s) are credited and that the original publication in this journal is cited, in accordance with accepted academic practice. No use, distribution or reproduction is permitted which does not comply with these terms.
*Correspondence: Celia Barrio-Alonso, Y2VsaWJhcnJAdWNtLmVz; Paloma Sánchez-Mateos, cGFsb21hLnNhbmNoZXptYXRlb3NAc2FsdWQubWFkcmlkLm9yZw==; Rafael Samaniego, Y29uZm9jYWxAaGdnbS5lcw==
†These authors have contributed equally to this work and share first authorship
‡These authors share senior authorship