- 1Affiliated Hospital of Shandong Second Medical University, Weifang, China
- 2Department of Hematology and Oncology, Shenzhen Children’s Hospital, Shenzhen, China
Chimeric antigen receptor natural Killer (CAR-NK) cells therapy represents a next-generation immunotherapeutic approach following CAR-T cells therapy, offering inherent “off-the-shelf” compatibility and mitigated off-tumor toxicity. Despite these advantages, clinical translation remains constrained by poor in vivo persistence and functional exhaustion in immunosuppressive tumor microenvironments (TME). This review examines recent advancements in synthetic biology aimed at enhancing the physiological characteristics of CAR-NK cells. By delineating the synergy between NK cells and synthetic biology toolkits, this work provides a roadmap for developing next-generation CAR-NK therapies capable of addressing solid tumor challenges while maintaining favorable safety profiles.
Introduction
Natural killer (NK) cells constitute critical effectors of innate immunity, deploying multifaceted cytotoxic mechanisms through dynamic integration of activating (e.g., NKG2D) and inhibitory (e.g., KIR, NKG2A) receptors. NK cells execute tumoricidal activity through three mechanistically distinct pathways: perforin/granzyme-mediated lysis; death receptor (FasL/TRAIL)-induced apoptosis; and antibody-dependent cellular cytotoxicity (ADCC) via FcγRIIIa (CD16) engagement (1). CAR-NK cells leverage the innate cytotoxic capacity of NK cells and gain specificity against particular tumor-associated antigens, thus improving their effectiveness in targeting malignant cells. Distinct from CAR-T cells, CAR-NK cells maintain dual targeting mechanisms both through their engineered receptors and their native activating receptors. Notably, CAR-NK cells do not require human leukocyte antigen (HLA) matching (2), maximumly eliminate graft-versus-host disease and cytokine release syndrome risks (3), and showed superior TME infiltration capabilities (4). Moreover, there are broader sources of NK cells (5), making them suitable for “off-the-shelf” therapeutic applications. This feature significantly enhances their accessibility and reduces the time required for treatment preparation, which is crucial for patients with aggressive cancers. Despite these merits, CAR-NK cells face cardinal translational barriers in terms of transient persistence and TME-imposed suppression (6). To address these challenges, researchers are exploring various approaches that can enhance the durability and effectiveness of CAR-NK cells. Synthetic biology approaches showed promising effects in CAR-NK cells therapy by focusing on the design, construction, and assembly of modular components. Validating these innovations in clinical trials will bridge current limitations to therapeutic application.
NK cells biology and sources
NK cells constitute critical effectors of the innate immune system, characterized by their unique capacity to detect and eliminate malignant or infected cells through a sophisticated balance of activating and inhibitory receptors (7). Notably, NK cells also mediate ADCC via CD16 engagement with antibody-opsonized targets, triggering cytotoxic granule polarization and release (8) (Figure 1A). Activating receptor signaling converges on Syk/ZAP70 axis, orchestrating cytotoxic particle synthesis, trafficking, and exocytosis (9–11). Sustained activation further induces FasL/TRAIL expression, activating extrinsic apoptosis pathways in target cells (12). The immunomodulatory capacity of NK cells extends to cytokine production, with IFN-γ secretion enhancing macrophage activation and antigen presentation, while TNF-α release promotes both direct tumor apoptosis and inflammatory amplification (13). Cellular ontogeny studies reveal NK cell proliferation and differentiation depend on cytokine-regulated signaling cascades: The PI3K-Akt-mTOR axis governs developmental progression (14), while JAK/STAT activation sustains precursor cell expansion and survival (15) (Figure 1B). Intervention in the biological features of NK cells will be a great innovative help for treatment, which is crucial for patients with aggressive cancers.
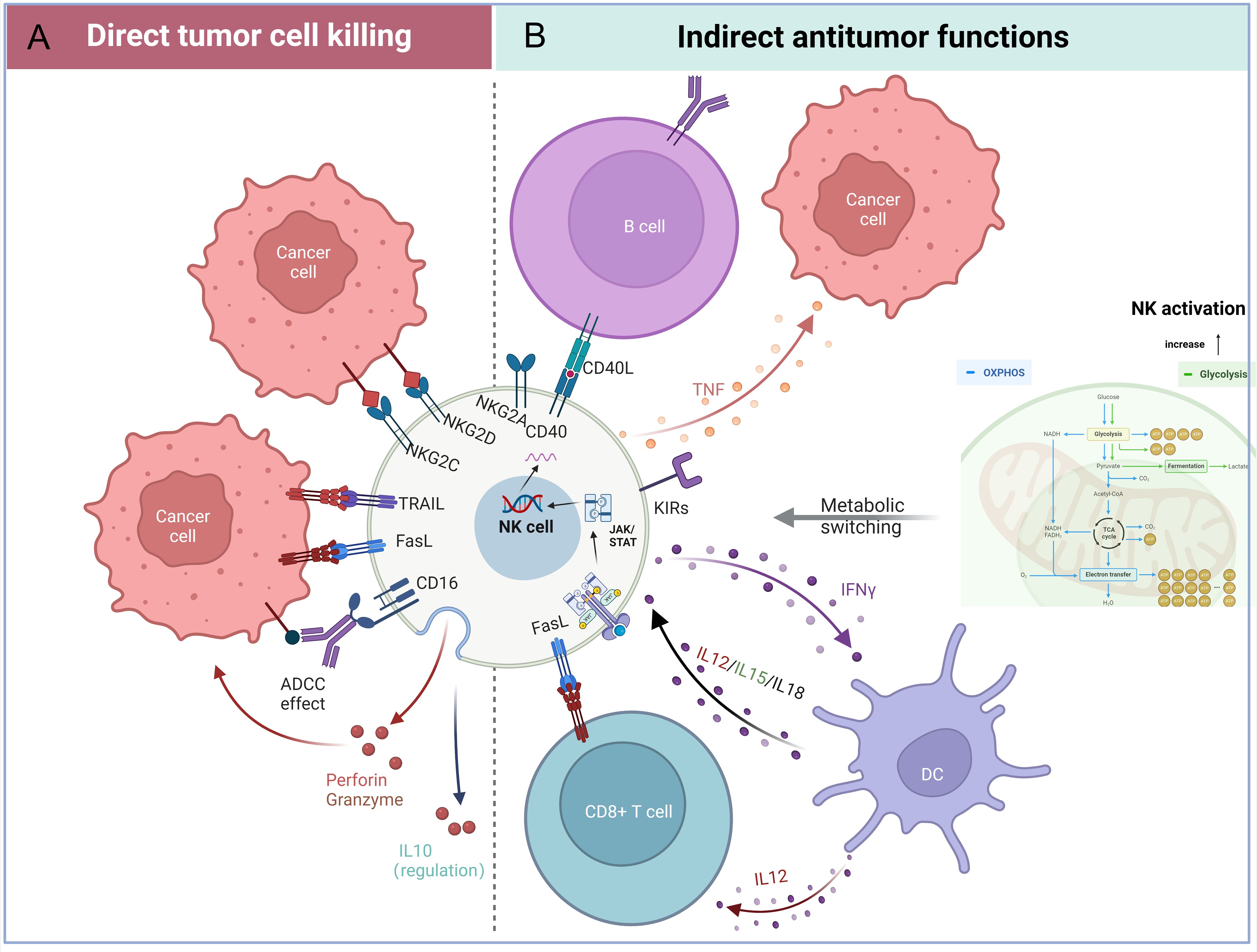
Figure 1. Functional attributes of natural killer (NK) cells in tumor immunity. (A) Direct tumor-killing mechanisms: NK cells eliminate cancer cells through activating receptors (e.g., NKG2D), ADCC via CD16, and the FasL/TRAIL-mediated death pathways. (B) Indirect antitumor effects: NK cells secrete immunomodulatory factors, including interferon-gamma (IFN-γ), cytokines, and chemokines, to recruit and activate adaptive immune cells. Additionally, metabolic reprogramming in the tumor microenvironment enhances NK cell effector functions. (Figure created with BioRender).
Current NK cell sources exhibit distinct therapeutic tradeoffs (Figure 2A). NK cells originate from the bone marrow and can be derived from various sources, including peripheral blood, umbilical cord blood, NK cells lines, and induced pluripotent stem cells (iPSCs). Peripheral blood-derived NK cells demonstrate immediate cytotoxicity but limited persistence (16). Umbilical cord blood variants show enhanced expansion capacity through reduced cytotoxic potential (17). Immortalized cell lines offer superior scalability and effector function but suffer from poor in vivo survival and culture challenges (18). While iPSC-derived NK cell products provide theoretically unlimited expansion potential, they carry inherent tumorigenic risks and demonstrate genetic instability during prolonged in vitro culture processes (5). These critical safety concerns mandate comprehensive genomic stability monitoring, inducible safety genes, and rigorous tumorigenicity assessments through standardized assays (including teratoma formation tests and karyotype analysis) prior to clinical implementation (19). The inherent dichotomy between functional potency and practical manufacturability presents fundamental challenges in engineering optimal CAR-NK therapeutics. Emerging synthetic biology strategies aim to overcome these limitations through precision engineering of NK cell durability, functionality, and tumor-resistant phenotypes.
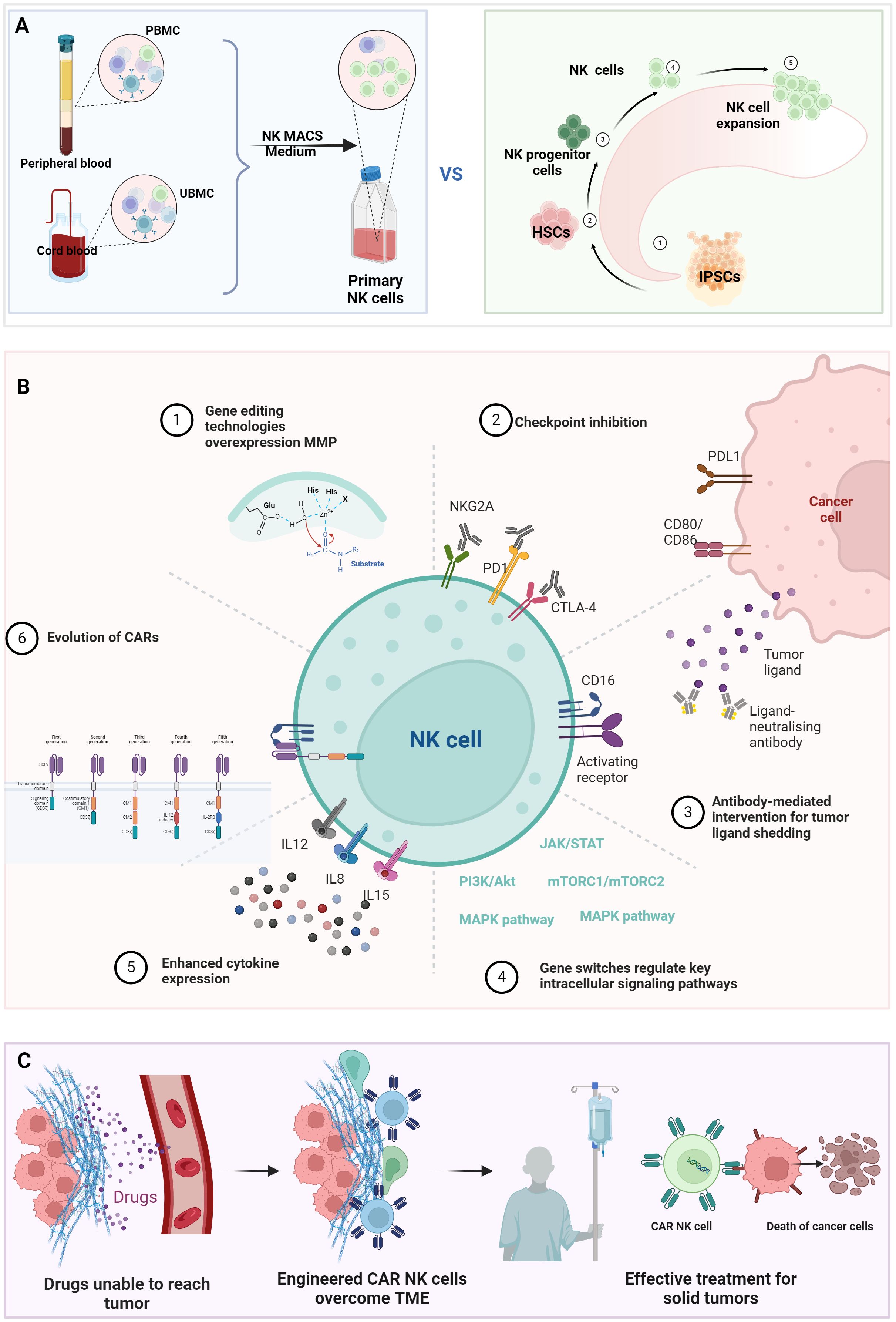
Figure 2. Bioengineering strategies to enhance NK cell antitumor efficacy. (A) Comparative profiles of NK cell sources: Functional and phenotypic differences among NK cell subsets derived from peripheral blood, umbilical cord blood, iPSC, or engineered cell lines. (B) Genetic engineering for functional optimization: (1). TME penetration: CRISPR-edited homing receptors (e.g., CXCR4/CXCR3) or matrix-degrading enzymes (MMPs); (2). Immune checkpoint modulation. (3). Knockout of inhibitory receptors (e.g., NKG2A) or overexpression of activating receptors (e.g., NKG2D, DNAM-1); (4). Intrinsic pathway activation: Constitutive signaling via STAT3, PI3K-AKT, or mTOR pathways; (5). Cytokine engineering: Armored CAR-NK cells secreting IL-15/IL-21 for autocrine survival; (6). CAR evolution: Multigenerational CAR designs (1st–5th gen). (C) CAR-NK-mediated TME reprogramming. (Figure created with BioRender).
Evolution of CAR architectures for NK cells
Chimeric Antigen Receptors (CARs) are synthetic constructs combining antibody-mediated specificity with cellular activation capacity, first successfully implemented in T cells. A canonical CAR architecture comprises three functional modules: an extracellular antigen-binding domain (scFv) for antigen recognition, a transmembrane domain, and an intracellular signaling domain [Figure 2B (6)]. First-generation CARs featured a solitary CD3ζ signaling domain that enabled antigen-specific activation but showed restricted clinical efficacy due to transient persistence (20). Second-generation designs incorporated a single co-stimulatory domain (CD28 or 4-1BB) alongside CD3ζ, markedly enhancing lymphocyte proliferation, metabolic fitness, and in vivo persistence – breakthroughs that revolutionized hematologic malignancy treatment (21, 22). However, these constructs remain vulnerable to immunosuppressive checkpoints and exhibit poor solid tumor penetration. Third-generation CARs addressed these limitations through dual co-stimulation (e.g., CD28 + 4-1BB), though excessive signaling occasionally precipitated T cell exhaustion via chronic activation (23, 24). Fourth-generation ‘armored’ CARs introduced inducible cytokine expression systems (e.g., IL-12) to remodel TME post-target engagement, though off-target cytokine release risks necessitated stringent safety controls (25). Fifth-generation platforms integrate tunable activation switches and fail-safe mechanisms, enabling real-time modulation of CAR activity to balance potency and toxicity (26, 27). This iterative engineering progression informs current CAR-NK cell development, which synergizes NK cells’ intrinsic advantages with synthetic biology precision editing.
Synthetic biology toolkit witches regulate key physiological characteristics
The synthetic biology toolkits enable the systematic integration of genetic components that fine-tune NK cell behavior, amplify therapeutic efficacy, and impose spatiotemporal control over in vivo activity. A cornerstone of this approach involves rewiring intracellular signaling pathways governing antigen recognition, activation dynamics, and cytotoxic execution.
Critical to CAR-NK cell utility is target identification, where synthetic biology enhances ADCC through CD16 engineering. Genetic modifications enhancing IgG Fc binding affinity have demonstrated improved ADCC durability and potency (28). Currently, a CAR-NK cell product containing optimized CD16a is undergoing clinical evaluation. The preliminary results of the trial were encouraging: objective response rates of >50% in 17 patients, including 7 complete responses (29). Complementarily, synthetic receptor systems mimicking natural activating ligands like NKG2D counteract tumor immune evasion strategies driven by ligand shedding (30). Counter-regulation of inhibitory checkpoints further amplifies tumor targeting: CRISPR-mediated NKG2A ablation prevents HLA-E-mediated suppression, markedly enhancing cytotoxicity against resistant malignancies (31) [Figure 2B (2, 3)]. For precision targeting, synthetic biology is fundamentally transforming CAR-NK cell engineering by implementing logic-gated (OR, AND, and NOT) receptor architectures that enhance tumor specificity and mitigate off-target toxicity (32–34). OR-gated dual CAR systems employ independently functioning activation domains that synergistically activate NK cells upon recognition of either target antigen, effectively addressing tumor antigen heterogeneity (35). AND-gated CAR systems require sequential engagement of two distinct tumor antigens - primary CAR activation induces expression of a secondary CAR, creating a failsafe mechanism that spares healthy cells expressing single antigens. NOT-gated inhibitory CAR architectures incorporate immune checkpoint-derived signaling domains that dominantly suppress activation when encountering healthy tissue biomarkers (36).
Upon target engagement, synthetic circuits amplify cytotoxic payload delivery. Tet-On systems enable controlled perforin/granzyme release, synergizing with engineered soluble factor-related apoptosis-inducing ligand (TRAIL) expression to activate both intrinsic and extrinsic apoptosis pathways (37, 38). Cytokine engineering further augments antitumor immunity: chimeric receptors incorporating CD28/IL-12 signaling domains drive IFN-γ hypersecretion (39), while IL-21 co-expression potentiates cytotoxicity against epithelial tumors (40). In a clinical trial in which CD19 and IL-15 co-express CAR structures to improve survival rate, this therapy was effective and well tolerated in patients with lymphocyte clearance from a large number of relapsed or refractory CD19-positive malignancies, with a complete response rate of 64%. Besides, CAR-NK cells are detectable in the patient’s peripheral blood for more than 12 month (41).
Overcoming transient in vivo persistence remains paramount. PI3K/AKT pathway modulation via IL-15/CCL21 co-expression synergizes to sustain CAR-NK proliferative capacity and metabolic fitness (42, 43). In addition to increasing persistence, long term survival can also be achieved by introducing anti-apoptotic genes (such as BCL-2) to reduce activation-induced apoptosis (44). In terms of NK cells sources, iPSC-derived CAR-NK cells exhibit superior longevity compared to peripheral blood counterparts (45).
Safety engineering remains integral to clinical translation. Inducible caspase 9 (iCasp9) suicide switches enable rapid ablation during cytokine release syndromes (3). TME-responsive circuits, such as TGF-β-inducible CAR activation, prevent off-tumor toxicity by restricting effector function to malignant niches (46). Collectively, these synthetic biology innovations establish a robust framework for developing next-generation CAR-NK therapeutics with enhanced specificity, persistence, and safety profiles.
Synthetic biology techniques reprogramming the TME
The TME suppresses immune cell cytotoxicity through three interconnected mechanisms (47–50). First, cancer-related fibroblasts secrete immunosuppressive factors including TGF-β, VEGF, IL-6, CXCL12, and PD-L1, while depositing extracellular matrix components like collagen and fibronectin that physically impede immune infiltration. Second, TME metabolites such as adenosine and lactate directly impair CD8+ T cell and NK cell effector functions. Third, aberrant tumor vasculature creates hypoxic niches that drive lactate accumulation via Warburg effect reprogramming, simultaneously fueling tumor proliferation and polarizing macrophages toward pro-tumoral M2 phenotypes through HIF-1α signaling. These immunosuppressive networks necessitate innovative strategies to enhance CAR-NK cell functionality within hostile TME (Figure 2C).
To enhance tumor infiltration, overexpression matrix metalloproteinase (MMP) engineering enables penetration through stromal barriers (51) [Figure 2B (1)]. Synthetic biology approaches counteract TME-driven immune evasion through multiple mechanisms. CRISPR-mediated knockout of inhibitory checkpoints like PD-1 on CAR-NK cells disrupts tumor immune resistance pathways (52) [Figure 2B (2,3)]. This modification can help overcome the immune evasion strategies employed by tumors, leading to improved therapeutic outcomes. TGF-β resistance has been achieved via CRISPR/Cas9-mediated TGFBR2 knockout, effectively neutralizing this potent immunosuppressive cytokine (46). Moreover, fused DNAX-activation protein 12 (DAP12) NK cells demonstrated improved efficacy and persistence in TGF-β–secreting TME (53).
Metabolic reprogramming strategies address TME-induced dysfunction: GLUT1 overexpression enhances glycolytic flux in hypoxic/lactate-rich conditions, sustaining CAR-NK cell metabolic fitness and survival (54). Advanced control systems incorporate hypoxia-responsive elements (HREs) to dynamically regulate CAR expression based on TME oxygen tension (55) [Figure 2B (4)]. Furthermore, engineered secretion of CCL5 enables CAR-NK cells to orchestrate antitumor immunity by recruiting T cells and dendritic cells [Figure 2B (5)], converting “cold” tumors into immunologically “hot” microenvironments, boots the cytotoxic of immune cells (34, 42). While these advancements demonstrate significant progress in overcoming TME constraints, clinical translation requires continued optimization of CAR-NK cell durability, specificity, and safety profiles through iterative synthetic biology innovations.
Strategies combining other immunotherapies
The therapeutic potential of CAR-NK cells is amplified through strategic combination with complementary modalities that address tumor resistance mechanisms. Synergy with immune checkpoint inhibitors counteracts TME-driven immunosuppression, as evidenced by enhanced antitumor responses when combining PD-1/CTLA-4 blockade with CAR-NK cells through dual activation of innate and adaptive immunity (56). Combine cellular therapy approaches leverage distinct cytotoxic mechanisms: CAR-NK/CAR-T cell co-administration demonstrates improved solid tumor control via spatial cooperativity, where NK cells eliminate CAR-T-resistant antigen-low variants while T cells target bulk tumor populations (57). Traditional cytotoxic therapies potentiate CAR-NK efficacy through immunogenic modulation – radiotherapy-induced DNA damage upregulates NKG2D ligands while chemotherapy depletes immunosuppressive myeloid populations, collectively enhancing tumor visibility and NK cell infiltration (58). This multidimensional strategy surmounts tumor heterogeneity by simultaneously targeting malignant clones, stromal barriers, and immune evasion pathways.
Future research
The clinical application prospects for CAR-NK cells are robust, with ongoing research expected to yield innovative approaches that enhance their therapeutic potential in cancer treatment. Innovation in synthetic biology enables researchers to design and engineer NK cells with unprecedented specificity and functionality. The field of synthetic biology is poised to well benefit the development and application of CAR-NK cells in cancer therapy.
Synthetic biology can facilitate the development of modular CAR constructs that can be easily adapted to target various antigens, thus streamlining the process of CAR design and production (30). Traditional CAR therapies typically focus on a single target antigen, which can lead to tumor escape mechanisms, especially in heterogeneous cancers where antigen loss is common. Dual-target CAR-NK cells can simultaneously engage multiple tumor-associated antigens, thereby reducing the likelihood of tumor evasion and enhancing overall therapeutic efficacy (32). The ability to design CARs that can recognize multiple antigens simultaneously is particularly valuable in treating complex malignancies, such as acute myeloid leukemia, where antigenic heterogeneity poses a significant challenge. In addition, adding a protective CAR to prevent normal cells from being accidentally injured is also a suitable way. Additionally, the integration of artificial intelligence (AI) with synthetic biology is fundamentally transforming CAR-NK cell development through many key mechanisms (59, 60): optimizing structures with algorithms; customizing personalized treatment regimens; and imitating treatment effects. Through the integration of AI technologies, CAR-NK design will shift from a “trial-and-error mode” to a “prediction-verification-optimization” closed loop, significantly accelerating the development of next-generation therapies. However, there is no ‘off of shelf’ NK cell in the market. Critical challenges persist in manufacturing scale-up and safety assurance. Good manufacturing practice-compliant scale-up requires standardized protocols for closed-system bioreactor platforms and automated cell processing systems. Safety assurance demands the implementation of multiplexed pathogen and residual pluripotent cells testing (61, 62). While iPSC-derived CAR-NK platforms progress toward off-the-shelf availability, standardized potency metrics, and reduced ex vivo manipulation costs remain prerequisites for global accessibility. Long-term monitoring must address theoretical risks of insertional oncogenesis from viral vectors and HLA-independent alloimmunity. The field requires harmonized clinical-grade production protocols validated through multicenter trials, coupled with real-world evidence frameworks tracking durable remission patterns.
Beyond CAR-NK cell optimization, synthetic biology has revolutionized microbial-based cancer therapeutics through two groundbreaking approaches: tumor-targeted bacterial vectors (63) and xenogeneic antigen engineering (64), these have greatly broadened the path of tumor therapies.
Through convergent innovation across synthetic biology, and computational immunology, optimized NK sources, CAR-NK cells are poised to transcend niche applications from robust hematologic malignancy control to engineered tissue-homing systems addressing solid TME. Realizing these potential demands sustained interdisciplinary collaboration to navigate the intricate interplay between cellular physiological characteristics, tumor adaptability, and host immunity.
Author contributions
SJ: Writing – original draft. CJ: Visualization, Writing – review & editing. XC: Writing – review & editing.
Funding
The author(s) declare that no financial support was received for the research and/or publication of this article.
Conflict of interest
The authors declare that the research was conducted in the absence of any commercial or financial relationships that could be construed as a potential conflict of interest.
Generative AI statement
The author(s) declare that no Generative AI was used in the creation of this manuscript.
Publisher’s note
All claims expressed in this article are solely those of the authors and do not necessarily represent those of their affiliated organizations, or those of the publisher, the editors and the reviewers. Any product that may be evaluated in this article, or claim that may be made by its manufacturer, is not guaranteed or endorsed by the publisher.
References
1. Shimasaki N, Jain A, Campana D. NK cells for cancer immunotherapy. Nat Rev Drug Discovery. (2020) 19:200–18. doi: 10.1038/s41573-019-0052-1
2. Moretta A, Bottino C, Vitale M, Pende D, Biassoni R, Mingari MC, et al. Receptors for hla class-i molecules in human natural killer cells. Annu Rev Immunol. (1996) 14:619–48. doi: 10.1146/annurev.immunol.14.1.619
3. Gong Y, Klein Wolterink RGJ, Wang J, Bos GMJ, Germeraad WTV. Chimeric antigen receptor natural killer (CAR-NK) cell design and engineering for cancer therapy. J Hematol OncolJ Hematol Oncol. (2021) 14:73. doi: 10.1186/s13045-021-01083-5
4. Wang W, Liu Y, He Z, Li L, Liu SB, Jiang MQ, et al. Breakthrough of solid tumor treatment: CAR-NK immunotherapy. Cell Death Discovery. (2024) 10:40. doi: 10.1038/s41420-024-01815-9
5. Cichocki F, van der Stegen SJC, Miller JS. Engineered and banked iPSCs for advanced NK- and T-cell immunotherapies. Blood. (2023) 141:846–55. doi: 10.1182/blood.2022016205
6. Dagher OK, Posey AD. Forks in the road for CAR T and CAR NK cell cancer therapies. Nat Immunol. (2023) 24:1994–2007. doi: 10.1038/s41590-023-01659-y
7. Moretta L, Moretta A. Unravelling natural killer cell function: triggering and inhibitory human NK receptors. EMBO J. (2004) 23:255–9. doi: 10.1038/sj.emboj.7600019
8. Bhatnagar N, Ahmad F, Hong HS, Eberhard J, Lu IN, Ballmaier M, et al. FcγRIII (CD16)-mediated ADCC by NK cells is regulated by monocytes and FcγRII (CD32). Eur J Immunol. (2014) 44:3368–79. doi: 10.1002/eji.201444515
9. Van den Broek MF, Kägi D, Hengartner H. Effector pathways of natural killer cells. Curr Top Microbiol Immunol. (1998) 230:123–31. doi: 10.1007/978-3-642-46859-9_9
10. Trapani JA, Smyth MJ. Functional significance of the perforin/granzyme cell death pathway. Nat Rev Immunol. (2002) 2:735–47. doi: 10.1038/nri911
11. Djeu JY, Jiang K, Wei S. A view to a kill: signals triggering cytotoxicity. Clin Cancer Res Off J Am Assoc Cancer Res. (2002) 8:636–40.
12. Chua HL, Serov Y, Brahmi Z. Regulation of FasL expression in natural killer cells. Hum Immunol. (2004) 65:317–27. doi: 10.1016/j.humimm.2004.01.004
13. Gómez-Lomelí P, Bravo-Cuellar A, Hernández-Flores G, Suárez LFJ, Lemarroy AA, Díaz JML, et al. Increase of IFN-γ and TNF-γ production in CD107a + NK-92 cells co-cultured with cervical cancer cell lines pre-treated with the HO-1 inhibitor. Cancer Cell Int. (2014) 14:100. doi: 10.1186/s12935-014-0100-1
14. Mace EM. Phosphoinositide-3-kinase signaling in human natural killer cells: new insights from primary immunodeficiency. Front Immunol. (2018) 9:445. doi: 10.3389/fimmu.2018.00445
15. Gotthardt D, Trifinopoulos J, Sexl V, Putz EM. JAK/STAT cytokine signaling at the crossroad of NK cell development and maturation. Front Immunol. (2019) 10:2590. doi: 10.3389/fimmu.2019.02590
16. Caruso S, De Angelis B, Del Bufalo F, Ciccone R, Donsante S, Volpe G, et al. Safe and effective off-the-shelf immunotherapy based on CAR.CD123-NK cells for the treatment of acute myeloid leukaemia. J Hematol OncolJ Hematol Oncol. (2022) 15:163. doi: 10.1186/s13045-022-01376-3
17. Liu E, Tong Y, Dotti G, Shaim H, Savoldo B, Mukherjee M, et al. Cord blood NK cells engineered to express IL-15 and a CD19-targeted CAR show long-term persistence and potent antitumor activity. Leukemia. (2018) 32:520–31. doi: 10.1038/leu.2017.226
18. Wrona E, Borowiec M, Potemski P. CAR-NK cells in the treatment of solid tumors. Int J Mol Sci. (2021) 22:5899. doi: 10.3390/ijms22115899
19. Knoepfler PS. Key anticipated regulatory issues for clinical use of human induced pluripotent stem cells. Regener Med. (2012) 7:713–20. doi: 10.2217/rme.12.51
20. Eshhar Z, Waks T, Gross G, Schindler DG. Specific activation and targeting of cytotoxic lymphocytes through chimeric single chains consisting of antibody-binding domains and the gamma or zeta subunits of the immunoglobulin and T-cell receptors. Proc Natl Acad Sci. (1993) 90:720–4. doi: 10.1073/pnas.90.2.720
21. Long AH, Haso WM, Shern JF, Wanhainen KM, Murgai M, Ingaramo M, et al. 4-1BB costimulation ameliorates T cell exhaustion induced by tonic signaling of chimeric antigen receptors. Nat Med. (2015) 21:581–90. doi: 10.1038/nm.3838
22. Finney HM, Akbar AN, Lawson ADG. Activation of resting human primary T cells with chimeric receptors: costimulation from CD28, inducible costimulator, CD134, and CD137 in series with signals from the TCRζ Chain. J Immunol. (2004) 172:104–13. doi: 10.4049/jimmunol.172.1.104
23. Schubert ML, Schmitt A, Neuber B, Krauss AH, Kunz A, Wang L, et al. Third-generation CAR T cells targeting CD19 are associated with an excellent safety profile and might improve persistence of CAR T cells in treated patients. Blood. (2019) 134:51. doi: 10.1182/blood-2019-125423
24. Abate-Daga D, Davila ML. CAR models: next-generation CAR modifications for enhanced T-cell function. Mol Ther - Oncolytics. (2016) 3:16014. doi: 10.1038/mto.2016.14
25. Chmielewski M, Abken H. TRUCKs: the fourth generation of CARs. Expert Opin Biol Ther. (2015) 15:1145–54. doi: 10.1517/14712598.2015.1046430
26. Huang R, Li X, He Y, Zhu W, Gao L, Liu Y, et al. Recent advances in CAR-T cell engineering. J Hematol OncolJ Hematol Oncol. (2020) 13:86. doi: 10.1186/s13045-020-00910-5
27. Tokarew N, Ogonek J, Endres S, Von Bergwelt-Baildon M, Kobold S. Teaching an old dog new tricks: next-generation CAR T cells. Br J Cancer. (2019) 120:26–37. doi: 10.1038/s41416-018-0325-1
28. Li P, Nagarajan S, Zhu C, Selvaraj P. Recombinant CD16A-Ig forms a homodimer and cross-blocks the ligand binding functions of neutrophil and monocyte Fcγ receptors. Mol Immunol. (2002) 38:527–38. doi: 10.1016/S0161-5890(01)00088-8
29. Bachanova V, Ghobadi A, Patel K, Park JH, Flinn IW, Shah P, et al. Safety and efficacy of FT596, a first-in-class, multi-antigen targeted, off-the-shelf, iPSC-derived CD19 CAR NK cell therapy in relapsed/refractory B-cell lymphoma. Blood. (2021) 138:823. doi: 10.1182/blood-2021-151185
30. Leivas A, Valeri A, Córdoba L, Ortiz AG, Ortiz A, Vega LS, et al. NKG2D-CAR-transduced natural killer cells efficiently target multiple myeloma. Blood Cancer J. (2021) 11:146. doi: 10.1038/s41408-021-00537-w
31. Bexte T, Albinger N, Al Ajami A, Wendel P, Buchinger L, Gessner A, et al. CRISPR/Cas9 editing of NKG2A improves the efficacy of primary CD33-directed chimeric antigen receptor natural killer cells. Nat Commun. (2024) 15:8439. doi: 10.1038/s41467-024-52388-1
32. Yang N, Zhang C, Zhang Y, Fan YT, Zhang J, Lin XJ, et al. CD19/CD20 dual-targeted chimeric antigen receptor-engineered natural killer cells exhibit improved cytotoxicity against acute lymphoblastic leukemia. J Transl Med. (2024) 22:274. doi: 10.1186/s12967-024-04990-6
33. Fedorov VD, Themeli M, Sadelain M. PD-1– and CTLA-4–based inhibitory chimeric antigen receptors (iCARs) divert off-target immunotherapy responses. Sci Transl Med. (2013) 5:215ra172. doi: 10.1126/scitranslmed.3006597
34. Sandberg ML, Wang X, Martin AD, Nampe DP, Gabrelow GB, Li CZ, et al. A carcinoembryonic antigen-specific cell therapy selectively targets tumor cells with HLA loss of heterozygosity in vitro and in vivo. Sci Transl Med. (2022) 14:eabm0306. doi: 10.1126/scitranslmed.abm0306
35. Han X, Wang Y, Wei J, Han W. Multi-antigen-targeted chimeric antigen receptor T cells for cancer therapy. J Hematol OncolJ Hematol Oncol. (2019) 12:128. doi: 10.1186/s13045-019-0813-7
36. Bassan D, Weinberger L, Yi J, Kim T, Weist MR, Adams GB, et al. HER2 and HLA-A*02 dual CAR-T cells utilize LOH in a NOT logic gate to address on-target off-tumor toxicity. J Immunother Cancer. (2023) 11:e007426. doi: 10.1136/jitc-2023-007426
37. Gu X, He D, Li C, Wang H, Yang G. Development of inducible CD19-CAR T cells with a tet-on system for controlled activity and enhanced clinical safety. Int J Mol Sci. (2018) 19:3455. doi: 10.3390/ijms19113455
38. Motais B, Charvátová S, Hrdinka M, Hájek R, Bago JR. Anti-BCMA-CAR NK cells expressing soluble TRAIL: promising therapeutic approach for multiple myeloma in combination with bortezomib and γ-secretase inhibitors. Blood. (2022) 140:12683–4. doi: 10.1182/blood-2022-166167
39. Jang J, Stanojevic M, Piccinelli S, Sheffer M, Birch GC, Shapiro RM, et al. Mesothelin targeting IL12-engineered CAR memory-like NK cells demonstrate promising efficacy in acute myeloid leukemia. Blood. (2024) 144:917. doi: 10.1182/blood-2024-204369
40. Zhang Y, Zhang C, He M, Xing W, Hou R, Zhang H. Co-expression of IL-21-Enhanced NKG2D CAR-NK cell therapy for lung cancer. BMC Cancer. (2024) 24:119. doi: 10.1186/s12885-023-11806-1
41. Liu E, Marin D, Banerjee P, Macapinlac HA, Thompson P, Basar R, et al. Use of CAR-transduced natural killer cells in CD19-positive lymphoid tumors. N Engl J Med. (2020) 382:545–53. doi: 10.1056/NEJMoa1910607
42. Kagoya Y, Tanaka S, Guo T, Anczurowski M, Wang CH, Saso K, et al. A novel chimeric antigen receptor containing a JAK–STAT signaling domain mediates superior antitumor effects. Nat Med. (2018) 24:352–9. doi: 10.1038/nm.4478
43. Wang X, Luo W, Chen Z, Hu Y, Mei H. Co-expression of IL-15 and CCL21 strengthens CAR-NK cells to eliminate tumors in concert with T cells and equips them with higher PI3K/AKT/mTOR signal signature. Blood. (2024) 144:4816. doi: 10.1182/blood-2024-205045
44. Wang H, Han P, Qi X, Li F, Li M, Fan LL, et al. Bcl-2 enhances chimeric antigen receptor T cell persistence by reducing activation-induced apoptosis. Cancers. (2021) 13:197. doi: 10.3390/cancers13020197
45. Li Y, Hermanson DL, Moriarity BS, Kaufman DS. Human iPSC-derived natural killer cells engineered with chimeric antigen receptors enhance anti-tumor activity. Cell Stem Cell. (2018) 23:181–192.e5. doi: 10.1016/j.stem.2018.06.002
46. Ren Y, Xue M, Hui X, Liu XY, Farooq MA, Chen YR, et al. Chimeric cytokine receptor TGF-β RII/IL-21R improves CAR-NK cell function by reversing the immunosuppressive tumor microenvironment of gastric cancer. Pharmacol Res. (2025) 212:107637. doi: 10.1016/j.phrs.2025.107637
47. Elhanani O, Ben-Uri R, Keren L. Spatial profiling technologies illuminate the tumor microenvironment. Cancer Cell. (2023) 41:404–20. doi: 10.1016/j.ccell.2023.01.010
48. Wei X, Chen Y, Jiang X, Liu YD, Mo YZ, Ren DX, et al. Mechanisms of vasculogenic mimicry in hypoxic tumor microenvironments. Mol Cancer. (2021) 20:7. doi: 10.1186/s12943-020-01288-1
49. Vitale I, Manic G, Coussens LM, Kroemer G, Galluzzi L. Macrophages and metabolism in the tumor microenvironment. Cell Metab. (2019) 30:36–50. doi: 10.1016/j.cmet.2019.06.001
50. Anderson NM, Simon MC. The tumor microenvironment. Curr Biol CB. (2020) 30:R921–5. doi: 10.1016/j.cub.2020.06.081
51. Caruana I, Savoldo B, Hoyos V, Weber G, Liu H, Kim ES, et al. Heparanase promotes tumor infiltration and antitumor activity of CAR-redirected T lymphocytes. Nat Med. (2015) 21:524–9. doi: 10.1038/nm.3833
52. Liu WN, So WY, Harden SL, Fong SY, Wong MXY, Tan WWS, et al. Successful targeting of PD-1/PD-L1 with chimeric antigen receptor-natural killer cells and nivolumab in a humanized mouse cancer model. Sci Adv. (2022) 8:eadd1187. doi: 10.1126/sciadv.add1187
53. Burga RA, Yvon E, Chorvinsky E, Fernandes R, Cruz CRY, Bollard CM. Engineering the TGFβ Receptor to enhance the therapeutic potential of natural killer cells as an immunotherapy for neuroblastoma. Clin Cancer Res. (2019) 25:4400–12. doi: 10.1158/1078-0432.CCR-18-3183
54. Guerrero JA, Klysz DD, Chen Y, Malipatlolla M, Lone J, Fowler C, et al. GLUT1 overexpression in CAR-T cells induces metabolic reprogramming and enhances potency. Nat Commun. (2024) 15:8658. doi: 10.1038/s41467-024-52666-y
55. Liu Y, Chen J, Tian J, Hao Y, Ma XX, Zhou YH, et al. Engineered CAR-NK cells with tolerance to H2O2 and hypoxia can suppress postoperative relapse of triple-negative breast cancers. Cancer Immunol Res. (2024) 12:1574–88. doi: 10.1158/2326-6066.CIR-23-1017
56. Hosseinalizadeh H, Wang LS, Mirzaei H, Amoozgar Z, Tian L, Yu J. Emerging combined CAR-NK cell therapies in cancer treatment: Finding a dancing partner. Mol Ther. (2025), S1525001624008955. doi: 10.1016/j.ymthe.2024.12.057
57. Li G, Wu X, Chan IH, Trager JB. Abstract 4235: A combination of CAR-NK and CAR-T cells results in rapid and persistent anti-tumor efficacy while reducing CAR-T cell mediated cytokine release and T-cell proliferation. Cancer Res. (2020) 80:4235. doi: 10.1158/1538-7445.AM2020-4235
58. He J, Yan Y, Zhang J, Wei Z, Li H, Xing L. Synergistic treatment strategy: combining CAR-NK cell therapy and radiotherapy to combat solid tumors. Front Immunol. (2023) 14:1298683. doi: 10.3389/fimmu.2023.1298683
59. Qiu S, Chen J, Wu T, Li L, Wang G, Wu HT, et al. CAR-Toner: an AI-driven approach for CAR tonic signaling prediction and optimization. Cell Res. (2024) 34:386–8. doi: 10.1038/s41422-024-00936-1
60. Lafeuille P, Shafquat A, Sang C, Beigi M, Maura F, Aptekar J. Using synthetic data produced by artificial intelligence (AI) to generate insights for chimeric antigen receptor T-cell (CAR T-cell) clinical trials. Blood. (2024) 144:7484. doi: 10.1182/blood-2024-203356
61. Knorr DA, Ni Z, Hermanson D, Hexum MK, Bendzick L, Cooper LJN, et al. Clinical-scale derivation of natural killer cells from human pluripotent stem cells for cancer therapy. Stem Cells Transl Med. (2013) 2:274–83. doi: 10.5966/sctm.2012-0084
62. Baghbaderani BA, Tian X, Neo BH, Burkall A, Dimezzo T, Sierra G, et al. cGMP-manufactured human induced pluripotent stem cells are available for pre-clinical and clinical applications. Stem Cell Rep. (2015) 5:647–59. doi: 10.1016/j.stemcr.2015.08.015
63. Chang Z, Guo X, Li X, Wang Y, Zang ZS, Pei SY, et al. Bacterial immunotherapy leveraging IL-10R hysteresis for both phagocytosis evasion and tumor immunity revitalization. Cell. (2025) 188:1842–1857.e20. doi: 10.1016/j.cell.2025.02.002
Keywords: NK cells, chimeric antigen receptor, synthetic biology, immunotherapy, TME
Citation: Ji S, Jin C and Cui X (2025) Enhancing the physiological characteristics of chimeric antigen receptor natural killer cells by synthetic biology. Front. Immunol. 16:1592121. doi: 10.3389/fimmu.2025.1592121
Received: 12 March 2025; Accepted: 02 April 2025;
Published: 17 April 2025.
Edited by:
Wenyi Jin, City University of Hong Kong, Hong Kong SAR, ChinaReviewed by:
Liang Yang, Chinese Academy of Sciences (CAS), ChinaYui-Leung Lau, Chinese Academy of Sciences (CAS), China
Copyright © 2025 Ji, Jin and Cui. This is an open-access article distributed under the terms of the Creative Commons Attribution License (CC BY). The use, distribution or reproduction in other forums is permitted, provided the original author(s) and the copyright owner(s) are credited and that the original publication in this journal is cited, in accordance with accepted academic practice. No use, distribution or reproduction is permitted which does not comply with these terms.
*Correspondence: Xinjiang Cui, eGluamlhbmcxMDIyQDE2My5jb20=; Cheng Jin, MjEwNjQwNDhnQGNvbm5lY3QucG9seXUuaGs=