- 1State Key Laboratory of Oral & Maxillofacial Reconstruction and Regeneration, Key Laboratory of Oral Biomedicine Ministry of Education, Hubei Key Laboratory of Stomatology, School & Hospital of Stomatology, Wuhan University, Wuhan, China
- 2Department of Oral Medicine, School and Hospital of Stomatology, Wuhan University, Wuhan, China
Objective: As a distinctive subset of T cells, intraepithelial lymphocytes (IELs) are found in the epithelium of mucosal barrier and serve as the primary defenders of the intestinal mucosal immune system. IELs exhibit phenotypic and functional diversity with high expression of activated marker molecules, tissue-homing integrins, NK cell receptors, cytotoxic T cell-related molecules, and cytokines. Meanwhile, IELs demonstrate differentiation plasticity, antigen recognition diversity, self-reactivity, and rapid “memory” effect, which enable them to play a crucial role in regulating responses, maintaining mucosal barriers, promoting immune tolerance, and providing resistance to infections. In addition, IELs have been explored in autoimmune diseases, inflammatory diseases, and cancers. However, the specific involvement and underlying mechanisms of IELs in oral diseases have not been systematically discussed.
Methods: A systematic literature review was conducted using the PubMed/MEDLINE databases to identify and analyze relevant literatures on the roles of IELs in oral diseases.
Results: The literature review revealed the characteristics of IELs and emphasized the potential roles of IELs in the pathogenesis of oral lichen planus, oral cancers, periodontal diseases, graft-versus-host disease, and primary Sjogren’s syndrome.
Conclusion: This review mainly focuses on the involvement of IELs in oral diseases, with a particular emphasis on the main functions and underlying mechanisms by which IELs influence the pathogenesis and progression of these conditions.
Introduction
Being a distinctive subgroup of T cells located in the mucosal epithelium, intraepithelial lymphocytes (IELs) differ from other T cells in terms of tissue distribution, phenotype, subgroup differentiation, and function (1). As a class of killing lymphocyte population, IELs exhibit potent, rapidly activated cytolytic and immunomodulatory effectors (2). Meanwhile, IELs resist pathogenic infections by supporting epithelial cells and regulating the barrier function of acquired and innate immunity (3).
By far, emerging studies have explored the role of IELs in chronic inflammation, autoimmune diseases, and tumors as a potential regulator of pathogenesis and a future therapeutic target. Moreover, IELs’ involvement in oral diseases has gradually garnered public attention. In oral lichen planus (OLP), significant infiltration of CD8+ IELs were observed (4). IELs infiltration was found in oral squamous cell carcinoma and was strongly associated with prognosis (5–7). γδ IELs were the first line of defense against luminal microorganisms and could induce inflammatory factors, which mediated the development and progression of periodontitis (8). IELs were increased in the gallbladder of patients with hematopoietic stem cell transplantation (HCT) (9). Currently, a scoring system for oral graft-versus-host disease (GVHD) pathology grading based on IELs infiltration and other pathological characteristics have been established. Intraepithelial B-lymphocytes (B-IELs) exist in salivary gland of primary Sjogren’s syndrome (pSS) patients, which act as a clear indicator of pSS and could be used as an objective alternative to scoring of striated ducts with hyperplasia (10). Furthermore, it is acknowledged that the complex microbial environment presents in the oral cavity, including various carrier molecules and cytokine networks associated with different oral diseases. These factors may play a role in the activation and function of IELs in oral diseases.
In this review, the characteristics of IELs was outlined and novel insights into the potential role of IELs in human oral diseases, including OLP, OSCC, PD, GVHD, and pSS were provided.
Subsets and development of IELs
Approximately 90% of all IELs are TCR+. These cells can be further divided into induced and natural TCR+ IELs (also called conventional or type a and unconventional or type b IELs, respectively (11)). Induced TCR+ IELs are derived from conventional Ag-specific T cells that were activated in the periphery and subsequently entered the epithelium. This group of IELs includes CD4+ and CD8αβ+ subsets. Natural TCR+ IELs include TCRαβ+ and TCRγδ+ subsets, which immediately enter the IELs compartment following their generation. TCR- IELs include subsets resembling innate lymphoid cells (ILCs) that are found outside the intestinal epithelium. Humans contain ILC1-like IELs expressing NKp44 (NCR2/CD336) (12–14). A subset of IELs that express NKp44 and resemble peripheral ILC3 cells have also been identified in humans (15). Another subset of TCR- IELs, present in mice and humans, express intracellular CD3 (iCD3) chains and are called iCD3+ TCR- IELs (16). One subset of TCR- IELs, identified in mice and humans, express iCD3 chains together with surface CD8αα and are referred to as innate CD8αα+ (iCD8α) cell (16–18) (Figure 1).
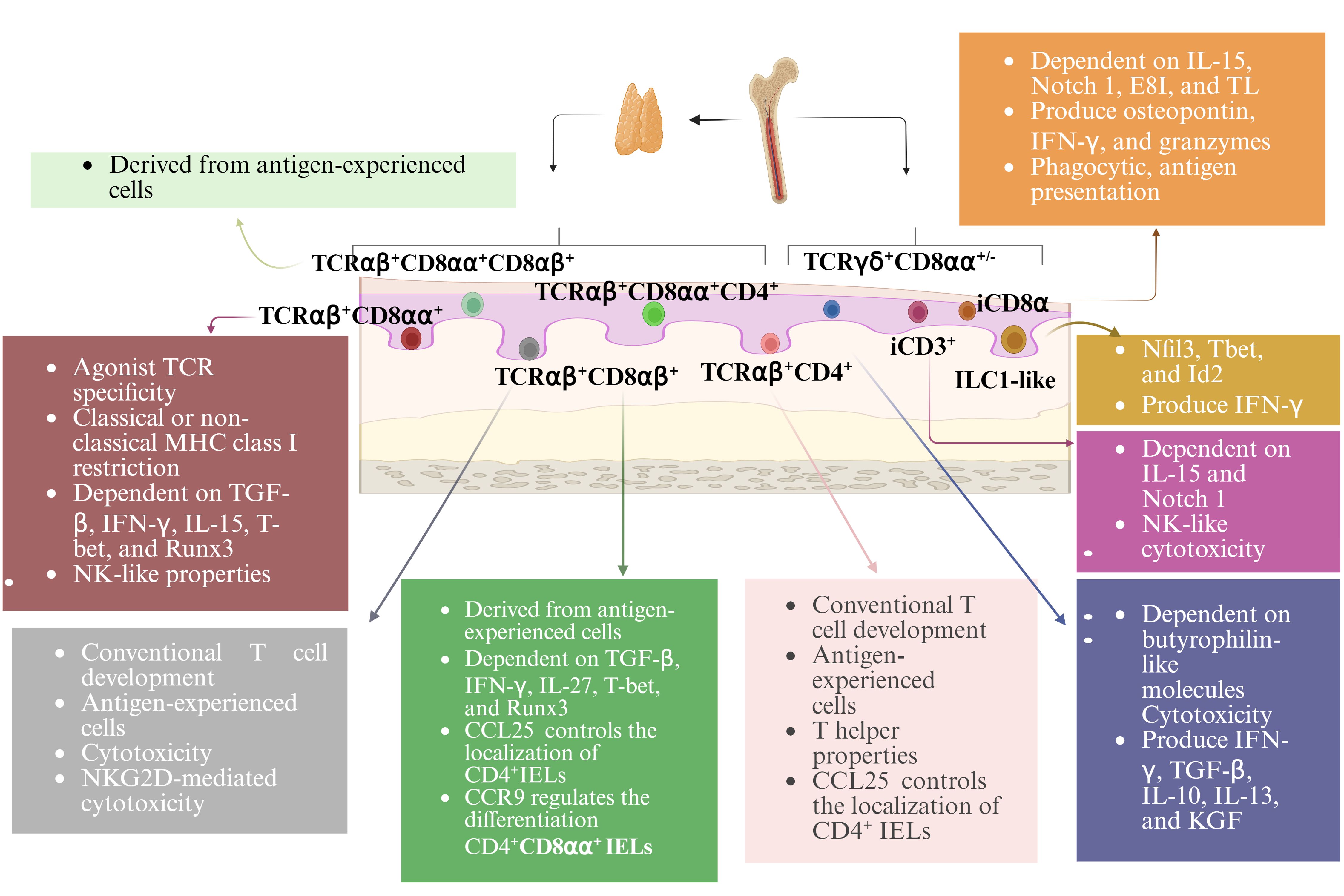
Figure 1. Subsets and development of IELs. With the exception of TCRγδ+ IELs, all TCR+ IELs develop in the thymus. All TCR- IELs develop extrathymically. Induced TCR+ IELs follow a conventional thymic development and the selection pathway, whereas natural CD8αα+TCRαβ+ IELs undergo agonist selection. IELs require a variety of transcription factors for their development and function. Many TCR+ IELs initiate CD8αα expression upon entry into the epithelium. The development, maintenance, and homeostasis of IELs require a variety of factors.
Induced TCR+ IELs are derived from conventional age experienced T cells that enter the intestinal epithelium. Their ontogeny follows the conventional intrathymic development pathway. Natural CD8αα+TCRαβ+ IELs undergo agonist selection. Being consistent with their lack of Ag-specific receptors, TCR- IELs develop extrathymically (19). The development of murine NKp46+ ILC1-like IELs is dependent on the transcription factors Nfil3 and Id2 (12), which requires for the development of all peripheral ILC subsets. The development of these cells also requires T-bet expression (12) (Figure 1).
Activation and maintenance of IELs
In vivo, the activation IELs can be achieved, at least in part, by TCR ligation. Positive selection of the agonist-driven IELs in the thymus suggests that the mature IELs at the epithelial barrier can subsequently be activated by specific TCR ligands. TCR activation of IELs can be achieved by cell surface receptors, such as non-classical MHC molecules (20, 21). After TCR signaling complexes are stimulated by anti-CD3 antibodies, the balanced IELs show higher expression of CD44, Ly-6C, OX40, FasL, and CD25, and reduced CD45RB protein expression, which indicates the expression of both cytotoxic mediators and cytokine transcripts (22, 23)(Figure 2).
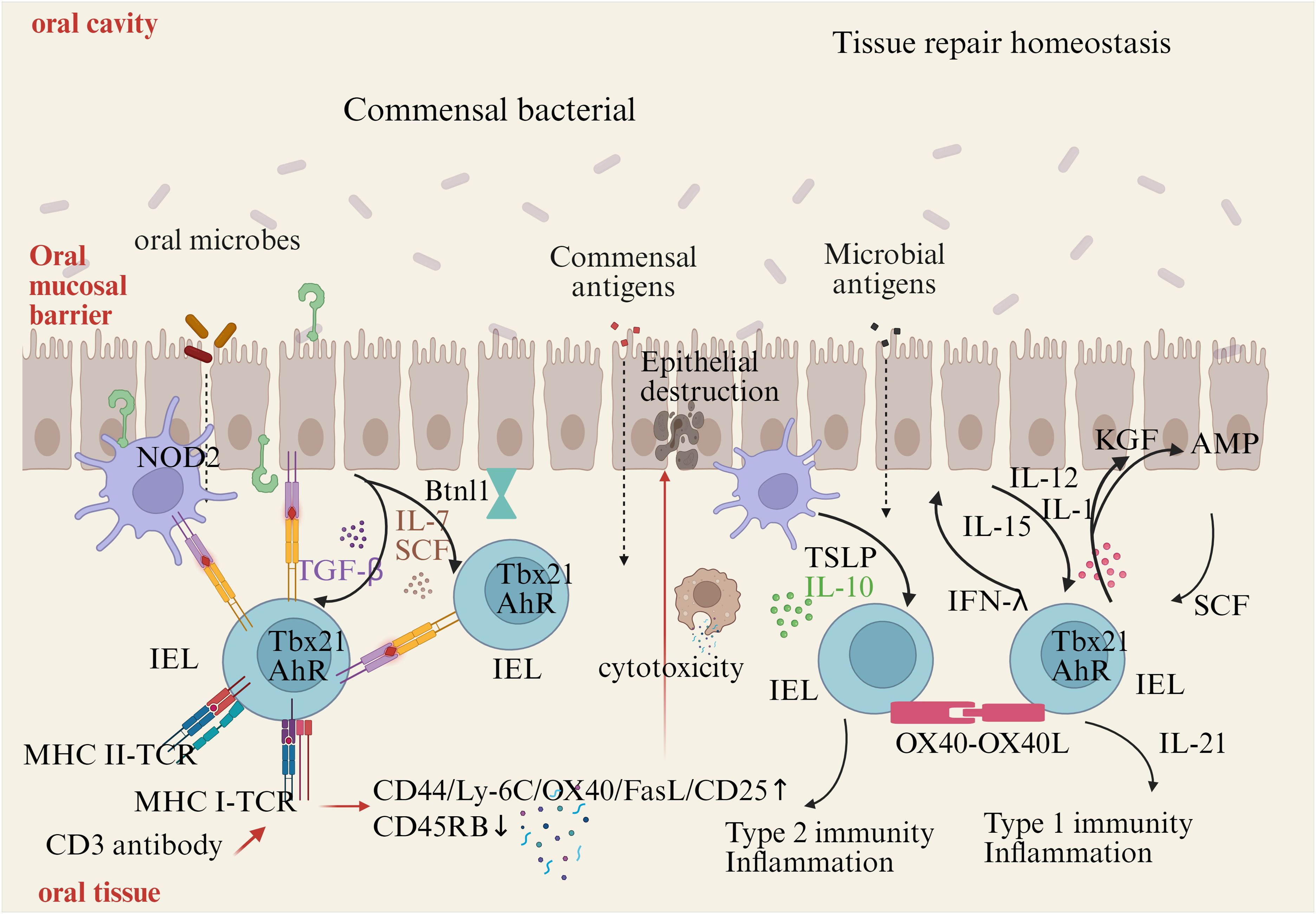
Figure 2. Activation and maintenance of IELs. Commensal bacteria can contribute to IELs maintenance. Signaling via TLR2 and myeloid differentiation primary response gene 88 (MyD88) increases IL-15 production, an important survival factor for IELs. Antigen presenting cells, such as dendritic cells (DCs) or macrophages, also produce IL-15 in a NOD2 dependent manner. IL-15 is bound to the IL-15Rα on the producing cells, and is presented in trans to the IELs, which carries the IL-15Rβ/Cγ chain receptor complex, and signals via the transcription factor Tbx21. IL-7 and stem cell factor (SCF) are additional examples for IEC derived cytokines important for IELs survival, while arylhydrocarbon receptor expression (AhR) and tissue-specific factors, such as butyrophilin-like 1 (Btnl1), play an additional role in maintaining IELs. Infections cause disruption or damage to the epithelial barrier. Dependent on the type of insult, IEC and DCs produce cytokines like thymic stromal lymphopoietin (TSLP), IL-10, IL-12, or SCF, thereby directing the type of immune response. Additional stimulation may be derived from IEL-IEL cross-talk, such as via OX40-XO40L interactions. IELs produce pro-inflammatory cytokines such as interferons (IFNs) and tumor necrosis factor (TNF), and cytotoxic factors such as Fas ligand (FasL) and granzymes, as well as antimicrobial peptides (AMPs) to contain the infection and contribute to wound healing and restoration of homeostasis by secreting growth factors such as KGF. Aberrant IELs activation and potentiation by cytokines might be involved in the development of chronic inflammation.
Cytokines are essential for IELs activation. IELs rapidly produce effector cytokines and cytotoxic molecules, such as keratinocyte growth factor (KGF), insulin-like growth factor 1 (IGF1), and transforming growth factor (TGF-β) in the stage early activation (24). What’s more, the expression of KGF is limited to γδ-τCR+ IELs. IELs can express the receptors of TNF, leukemia inhibitory factor, thymic stromal lymphopoietin (TSLP), stem cell factor (SCF; c-Kit ligand), TGF-β, IL-12, IL-15, and IL-1. TGF-β is required for the maintenance of native CD8αα IELs and the expression of CD103. High levels of IL-15 can trigger CD8+ T cells to become cytotoxic independently of the TCR (25, 26). The stimulation of IL-15 on IELs results in increased production of IFN-γ and TNF, granzyme-dependent cytotoxicity, and enhanced expression of NK receptors (27).
IELs constitutively express transcripts of genes, which are associated with activated cytotoxic T cells, such as granzyme A, granzyme B, serine, Fas ligand (FasL), and chemokine C-C motif ligand (CCL). IELs express cytotoxic T lymphocyte-associated protein 4, Ly49E-G, NK cell inhibitory receptor Ig superfamily-related gp49B, and programmed cell death 1 (PD-1) (28). Unlike conventional CD8+ T cells, IELs express high levels of Tnfsf6 transcripts during steady state (29), but do not express the protein encoding FasL on their surface until additional activation occurs (29, 30).
Maintaining IELs requires the involvement of multiple cytokines and signaling pathways. The ligand-activated transcription factor and the aryl hydrocarbon receptor (AHR) are essential for the maintaining of IELs (31, 32). Maintenance and activation of IELs also critically depend on the interaction between epithelial cells and microbes. The myeloid differentiation primary response gene 88 (MyD88) can activate NF-κB, which is essential for IELs maintenance (33). IL-15 produces signals by expressing the type 1 transcription factor T-box in T cells (T-bet) to sustain IEL precursors (34). Protein containing a nucleotide-binding oligomerization domain (NOD) 2 is also crucial for maintaining IELs (35) (Figure 2).
IELs and human oral diseases
The oral mucosa consists of stratified squamous epithelium, lamina propria (LP) and MALT, mucosal-associated lymphoid tissue (MALTs). Dendritic cell (DCs), Langerhans cells (LCs) and, intraepithelial lymphocyte (IELs) reside in the epithelial layer. Numerous microorganisms and antigens and stimuli from exogenous substances (36) such as food undergo sophisticated regulation by immune responses in the oral mucosa. T cells especially IELs are crucial for this defense (37). CD8αα+ IELs in the oral-pharyngeal mucosa have not been identified and characterized until recently year. And TGF-β regulates the development/generation of oral CD8αα+ IELs (38).
IELs in oral lichen planus
OLP is a chronic inflammatory disease that occurs in the oral mucosa and is labelled as oral potentially malignant disorder (OPMD) by the World Health Organization (WHO). Although the etiology is unknown, T-cell-mediated immune response is pivotal in the pathogenesis of OLP. T cells infiltrate the lamina propria, secrete cytokines to construct an inflammatory environment and mediate keratinocyte apoptosis, participating in the development of OLP.
OLP is characterized by subepithelial lymphocyte infiltration and elevated IELs (4). Most subepithelial and intraepithelial lymphocytes are CD8+ T cells (4). The infiltrates in the lamina propria of OLP lesions are mostly CD4+ Th cells, while T cells near the basement membrane region are dominated by cytotoxic CD8+ T cells (4). There is approximately a 1:2 ratio of CD4+ IELs/CD8+ IELs in OLP (39). Our previous study identified a significant infiltration of elongated CD8α+ IELs in the basal cell layer of OLP (40). Enomoto A et al. proposed that CD8+ IELs could serve as a predictive biomarker for OLP remission. They found that CD8+ IELs were associated with the remission rate in a subgroup that exhibited higher T-bet/FOXP3 subset balance (indicating inducible cytotoxic immunity) and determined a predicted cut-off value for CD8+ IELs (16 cells/high-power fields) (41).
Gene expression analysis of OLP oral mucosa samples disclose increased transcript expression of killer cell lectin-like receptor subfamily G member 1 (KLRG1), CD8A, and granzyme K (GZMK) (42). CD8 T cells and KLRG1+ T cells localized within the intraepithelial regions, both at the basal layers and more superficially and often adjacent to keratinocytes (42). Cytotoxic CD8+ IELs cluster in areas of basement membrane disruption (43) and adjacent to degenerating keratinocytes (44), which indicates that CD8+ IELs may be engaged in epithelial cell destruction by typical cytotoxic mechanisms of CD8 T cells in OLP, such as release of granzymes and direct killing of target cells.
CD8A encodes the CD8α chain that is a cell surface glycoprotein expressed on cytotoxic T cells, and it plays a pivotal role in antigen recognition and the immune response against infected or abnormal cells (45). Research indicates that CD8αα is a crucial modulator of the IEL’s dynamic migration between the inner epithelium and the lamina propria. While CD8ααCD4 IELs were in the lamina propria, adoptively transferred CD8αCD4 IELs were found within the recipient mice’s epithelium (46).
CD8 IELs are often tissue-resident memory T (Trm) cells in OLP lesions. Compared to nonerosive oral lichen planus (NEOLP), CD8+ Trm cells was increased in erosive oral lichen planus (EOLP), which were adjacent to the epithelium and its products may induce epithelial erosion. CD8+ Trm cells in particular exhibited higher expression levels of GZMA, GZMK, TNF, PRF1, and other genes associated with inflammatory factors, when compared to other subgroups. It may have contributed to the worsening of the clinical manifestations of OLP. CD8+ Trm marker gene CD69, GNLY which can play a cytotoxic role, and multiple pro-inflammatory factor-related genes, such as GZMB, IFNG, TNF, and PRF1, were significantly increased in the CD8+ Trm subgroup in EOLP (47).
The expression of TNF, IL17A/IL17RA, IFNGR1, etc. was higher in EOLP than in normal oral mucosa. And the signals of IFNGR1 and IL17RA were significantly enhanced in EOLP compared with NEOLP. CD8+ Trm cells in EOLP produced significantly higher levels of TNF-α, IFN-γ, and IL-17 than those in NEOLP, with the increase in IFN-γ being statistically significant. Therefore, CD8+ Trm cells may affect the clinical manifestations of OLP through the secretion of IFN-γ (47).
Biologic therapies targeting cytokines such as anti-TNF-α, anti-IL17, and anti-IL12/23 have been employed with variable outcomes. TNF-α inhibitors (etanercept, infliximab, and adalimumab) have shown promise. Additionally, therapies such as Alefacept and agents targeting IL-17 and related pathways (e.g., ustekinumab, guselkumab, secukinumab, and tildrakizumab) have demonstrated efficacy, particularly in reducing the Th1 and Th17/Tc17 cellular mucosal infiltrate, suggesting a key role for IL-17-producing T cells in OLP pathogenesis (48).
The integrin αE (CD103) β7 (αEβ7) is expressed by IELs, dendritic cells and regulatory T cells, and mediates cell migration and homing (49). The percentage of CD103 γδT cells was upregulated in OLP γδ IELs are the main group of IELs with highly motility (50). The interaction between the IELs and the pathogen is critical for γδ IELs surveillance and direct host defense (51). TCRγδ+ IELs recognize autoantigen molecules expressed by epithelial cells to activate the NKG2D receptor pathway and play a dynamic defense role in the epithelium (52). IL-15 secreted by epithelial cells can induce NKG2D activation and upregulation on the surface of IELs, thus preventing the activation of the inhibitory receptor NKG2A and then activating the ability of IELs to destroy epithelial cells (17, 53). Research has found the co-localization of IELs with IL-7 secreted by epithelial cells (11), which suggests that IL-7 that is secreted by epithelial cells may mediate the close interaction between IELs and epithelial cells in OLP.
MAIT cells express transcription factors retinoic acid receptor-related orphan receptor gamma-t (RORγt) and T-bet, regulating the secretion of IL-17, IFN-γ. etc (54–56). Additionally, MAIT cells can secrete Th2-type cytokines like IL-13 under chronic inflammation stimulation (57). This cytokine secretion potentially enables MAIT cells to modulate Th1, Th2, and Th17 cells, suggesting a potential immunomodulatory capacity exerted by MAIT cells. In OLP patients, TNF and IFN-γ upregulate endothelial adhesion molecules like CD31, CD106, CD54, and CD62E in blood vessels and stimulate the production of the chemokine CCL5 by keratinocytes (58–60). Circulating T cells are recruited to OLP lesions through these adhesion molecules and chemokines, and the release of TNF and IFN-γ by activated MAIT cells may participate in these processes, thereby promoting T cell recruitment.
Current studies on the interaction between MAIT and CD8+ T cells primarily focus on vaccine research. Provine et al. highlighted the ability of MAIT cells to sense immune activation signals triggered by viral vectors and integrate them to augment CD8+ T-cell responses, with locally produced chemokine CXCL20 likely playing a significant role in this process (61). Additionally, IFN-γ promotes CD8+ T cell activation and maintains MHC II expression, with MAIT cells potentially involved in this process, thereby modulating the OLP inflammatory response (62).
IELs in oral squamous cell carcinoma
Oral squamous cell carcinoma is the most prevalent type of oral cancer, with a 5-year survival rate of approximately 50%. The significantly high rates of local recurrence and cervical lymph node metastasis complicate surgical removal of OSCC, leading to poor prognoses and posing significant threats to human health and well-being. The pathogenesis of OSCC has not been fully elucidated, which is a consequence of complicated multiple-factors synergetic effects and associated with the changes of oncogenes and tumor suppressor genes and a series of tumor immunological responses (63–65).
IELs infiltration and expression of tumor related factors were observed in OSCC. In lip carcinogenesis, there was an increase in peritumoral and intratumoral CD3+, CD8+, CD20+ and CD68+ cells. In the intraepithelial region, CD8+ cells are correlated with CD20+ and CD68+ cells (66). In tongue cancers, tumor nest-infiltrating CD8+ IELs frequently expressed PD-1, an inhibitory receptor, in sharp contrast to those in the stroma or in the lichen planus. Conversely, CD8+ IELs only infrequently expressed NKG2D, an activating receptor, in contrast to those in the stroma or in the lichen planus. No CD8+ IELs expressed Ki-67, a proliferation associated marker, whereas those in the stroma frequently expressed it (67). CD8+ IELs in tongue cancer tumor nests was phenotypically inactivated, which indicated the first immune escape in OSCC tumor nests (67).
FOXP3+ IELs were significantly increased in OSCC patients (68). Tumor-infiltrating FOXP31+ IELs were significantly more frequent in oropharynx cancer and OSCC and patients without lymph node metastasis (68). Additionally, high infiltration of regulatory FOXP31+ IELs and relatively high levels of BDCA21 and FOXP31 cells in stromal (peripheral) regions of the tumors were found in head and neck squamous cell carcinoma (68).
A higher number of CD8+ T cells was significantly associated with poorer outcome. In the tumor-bearing part of involved lymph node tissue, more CD8+ T cells were observed than in primary cancer. CD8+CD103+ Trm cell infiltration in T2 tumors was higher than in T1 or T4 tumors (69). However, patients with a higher density of CD8+ T cells in their cancer survive longer than patients with lower numbers. Patients with Trm cell-high cancers had better overall survival than patients with Trm cell-low tumors. In addition, Trm cell infiltration was absent in metastatic disease or at recurrence, serves as a marker of better survival. The checkpoint molecule TIM3, was expressed significantly higher on Trm and non-Trm cells in the lymph node compared with primary tumors, which was also seen in recurrences. The role of TIM3, as a therapeutic target remains to be defined (69).
The early progression of oral precancerous lesions to cancer was enhanced in IL-23 receptor-deficient mice, which suggested the importance of IL-23. Exogenous IL-23 can promote the activation of CD8+ IELs with high expression of IL-23R (70). IL-23 is known to modulate the homeostasis of neutrophil infiltration into tissues by inducing expression of IL-17 and G-CSF18, which are linked to tumor growth (71). IL-23 can induce IL-17 production by tumor-resident immune cells, including CD4+ Th17 cells, natural killer T (NKT) cells, γδ T cells and CD8+ cytotoxic T lymphocytes (CTLs). IL-23 also opposes the action of IFN-γ and the subsequent production of cytotoxic mediators such as perforin, granzymes, and Fas ligand (FasL), and can also inhibit IFNγ-mediated MHC-I upregulation (72).
The duction of IFN-γ by T-cell induction by IL-23 antagonized the local inflammatory response as well as IELs infiltration in the tumor immune microenvironment (TME) (73). Low-density CD4FOXP3 IELs infiltration was observed within the OSCC invasion front and tumor center, which suggested a poor prognosis. The rate of local failure in older cancer patients improved with increasing levels of CD3+ IELs and CD8+ IELs (74). In low-risk oropharyngeal and hypopharyngeal SCC, high infiltration of CD8+ IELs may improve disease-free survival (75). The cytotoxic activity and tumor infiltrating ability of CD8+ T cells might be largely inhibited owing to a local protective tumor microenvironment induced or fostered by IL-23.
IELs in periodontal disease
Periodontal disease is a chronic inflammatory disease characterized by an inflammatory environment, mainly affecting the gingiva, bone, and ligament (76). Periodontal disease includes gingivitis and periodontitis.
Junctional epithelium (JE), the first line of periodontal defense against bacterial infection, constitutively expresses ICAM-1, cytokines and chemokines, together to maintain the physiological homeostasis of JE (77). IELs are localized to the middle layer of JE, in which the number of TCR+ lymphocytes is higher than that in systemic immune organs, such as spleen and lymph nodes (78). IELs in JE express TCR and CD3 in conventional and germ-free mouse (79). TCR-positive T cells constitute the main population of IELs (80, 81).
The gingiva contains a significant population of Vγ6+ γδ T cells (82, 83). γδ IELs are the first line of defense against luminal microorganisms and they are adjacent to dental biofilm, which implies their possible role in the host-microbiota interactions in the gingiva (82). The microbiome is both necessary and sufficient for the observed increase in γδ IELs (82, 84, 85). Furthermore, adult mice treated with antibiotics had a substantial decrease in the frequency of γδ IELs in their gingiva (82), which indicated that the microbiota may have impacts on the development and maintenance of γδ IELs (85).
γδ IELs are involved in regulating the oral microbiota (85) and can induce a large amount of IL-17, which mediates the development and progression of periodontitis (8). IL-17 plays a predominantly protective role in periodontal diseases (86). Vγ6+γδ T cell can produce large amounts of IL-17A to accelerate bone formation at the fracture site by stimulating the proliferation of mesenchymal progenitors and the differentiation of osteoblasts (87). However, some studies have shown that the presence of γδ T cells and IL-17 in periodontal tissue is positively associated with the severity of periodontitis, which may be related to the ability of IL-17 to recruit inflammatory cells (88, 89). γδ T cells can inhibit periodontal bone loss and promote gingival repair by producing restorative cytokines, such as amphiregulin (a member of the epidermal growth factor family) (90, 91).
IELs in graft-versus-host disease
GVHD is a common and significant complication of allogeneic hematopoietic cell transplantation (allo-HCT). Both apoptotic bodies and IELs were increased in the gallbladder of patients with HCT (9). Oral chronic GVHD (cGVHD) occurs after approximately 70% of HCT, including lichenoid mucosal responses, restricted mouth opening, and salivary gland dysfunction. According to literature reported, patients with refractory Hodgkin’s lymphoma developed tongue GVHD after receiving allo-HCT. Tongue biopsy showed changes in moss and keratinized tissue, accompanied by epithelial T cell infiltration, which was consistent with cGVHD (92).
A large cohort analysis on histopathological grading of oral mucosal cGVHD indicated that a points-based grading tool (0 to 19, grade 0 to IV, 0→IV: mild→severe) was established. The evaluation indicators of this tool include IELs and band-like inflammatory infiltrate, atrophic epithelium with basal cell liquefaction degeneration, including apoptosis, as well as separation of epithelium and pseudo-rete ridges. From grade 0 to IV, IELs infiltration ranged from no/occasional to widespread (93). The grade II-IV biopsy specimens demonstrated a histopathological diagnosis of active mucosal lichenoid-like cGVHD, which highlighted the importance of correlating clinical presentation with the dynamic histopathological processes for improved patient stratification. Most importantly, this tool could be used for assessing treatments, pathological processes, and immune cellular content to provide further insights into this debilitating disease (93). Recently, the histological NIH cGVHD grading for defining features of salivary gland cGVHD (sg-cGVHD) with awarded points was designed (Table 1) (94). Peri-ductal and acinar lymphocytic infiltration is an important consideration in the NIH cGVHD grading form.
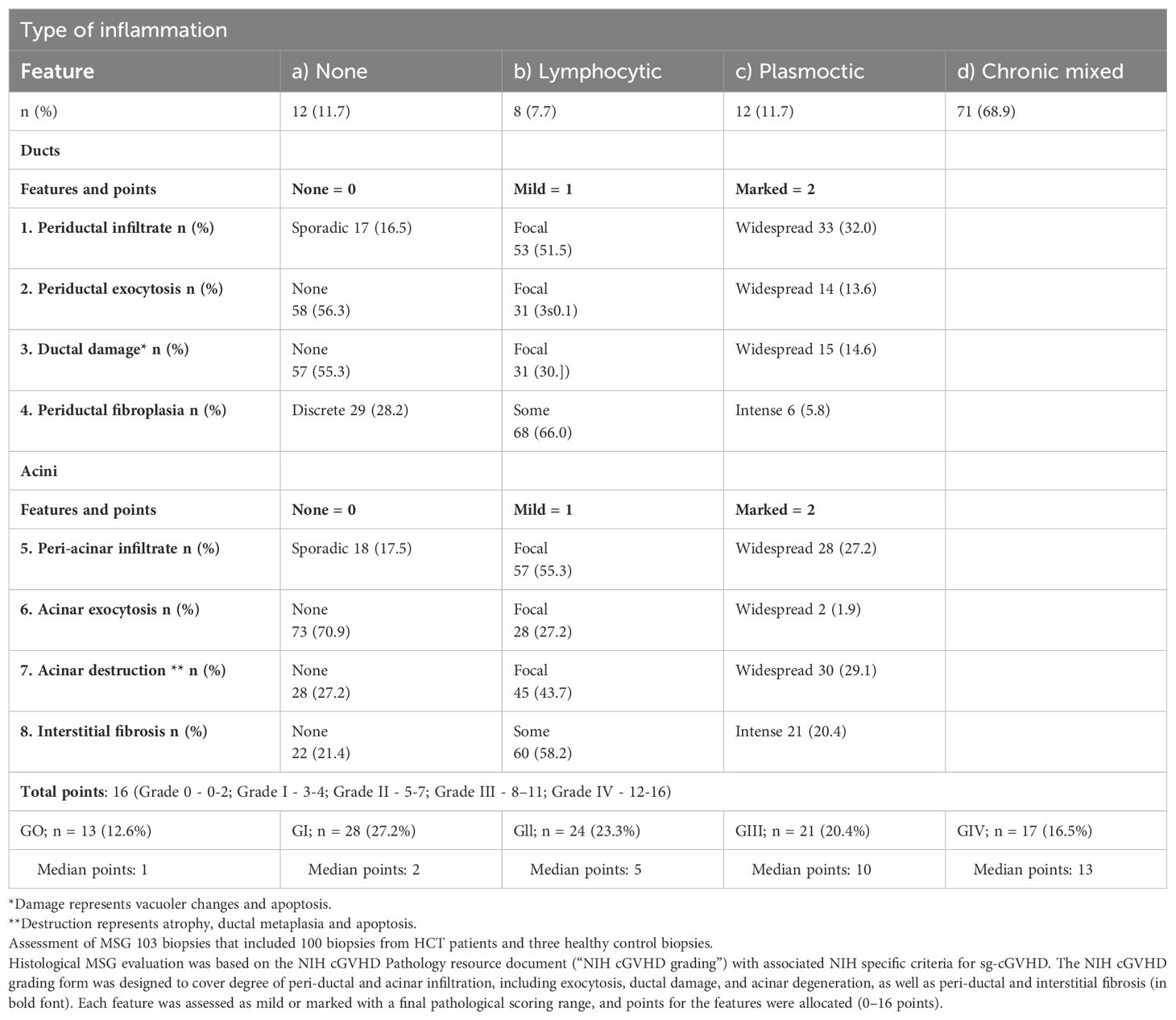
Table 1. (94) Grading of minor salivary gland immuno-histopathology post-allogenic hematopoietic cell transplantation.
High expression of CD4, CD8, and FOXP3 in GVHD confirmed that oral cGVHD was primarily driven by T cells and involved by macrophages (95). The presence of a CD4/CD8 double-positive T cell population in adult allo-HCT recipients was predictive of grade II GVHD (96). Research proved that Notch signaling promoted T cell pathogenesis and GVHD after allo-HCT, in which δ-like Notch ligand DLL4 played a dominant role. IELs expressing CD3 in human and mouse can differentiate along the transcription factor Id2-independent pathway under NOTCH1 signaling (16), which is involved in the occurrence and development of GVHD.
IELs in primary Sjogren’s syndrome
pSS is a chronic inflammatory autoimmune disease that primarily affects the function of exocrine glands such as salivary and lacrimal glands. In tissues of pSS, lymphocytes infiltrate into salivary and lacrimal glands to produce autoantibodies (97).
Intraepithelial T-lymphocytes (T-IELs) scattered throughout the striated ductal epithelium of salivary glands of pSS. Intraepithelial B-lymphocytes (B-IELs) were found in almost all striated ducts with hyperplasia in lymphoepithelial lesions (LELs). B-IELs and B-IELs/T-IELs ratios increased significantly with higher severity of LELs, which was even more pronounced in the parotid than in the labial gland (98). The presence of B-IELs in salivary gland biopsies patients is a clear indicator of pSS and can be used as an objective alternative to LEL scoring (10).
Fc receptor-like protein 4 (FcRL4) is normally expressed on a small subset of mucosa-associated B-cells, as well as on MALT lymphoma B-cells. pSS patients have an increased risk of developing MALT lymphomas, preferentially in the parotid glands. FcRL4 mRNA expression level in parotid MALT lymphoma is increased compared to parotid gland tissue of pSS patients without lymphoma. However, numbers of FcRL4+ B-cells in labial gland biopsies taken at the time of pSS diagnosis, are not predictive for later development of MALT lymphoma (99). Pathway analysis showed upregulation of B cell activation, cell cycle and metabolic pathways. FcRL4+ B cells are expected to be an important treatment target in pSS (100).
In pSS, ductal cells produce a wide variety of cytokines (101), which can contribute to the activation of the FcRL4+ B-IELs, and, particularly, FcRL4+ B-IELs express the highest levels of FcRL4.
FcRL4+ B-IELs have strong expression of CD20 that makes them highly susceptible targets for rituximab therapy (102, 103). Treatment with rituximab did not only reduce the total number of B cells, but also FcRL4+ B-IELs, which resulted in a significant decrease in the number of LELs and normalization of the epithelial lining (99, 104). Apparently, when FcRL4+ B-IELs are depleted from the epithelium, stimulation of ductal cells by FcRL4+ B-IELs is no longer present, which enables the restoration of epithelium. Of note, blocking the CD28 mediated co-stimulation with abatacept did not affect the presence of FcRL4+ B-IELs and the numbers and severity of LELs concomitantly (105).
Therefore, it has been proposed that identification of B-lymphocyte–containing ducts should be added to the diagnostic histopathological work-up of patients suspected of pSS (10).
Conclusion and the future development direction
The increasing evidence provides new insights into the role of IELs in the pathogenesis of OLP, OSCC, PD, GVHD, and pSS (Figure 3). Notably, IELs are increased in OLP lesions, thus killing epithelial cells directly or indirectly through cytotoxicity and destroying the basement membrane. IELs are a double-edged sword in OSCC. The infiltration of IELs around and within the tumor of OSCC has the potential to promote the tumor growth, and immune molecules related to regulating its cytotoxicity may regulate the prognosis of OSCC. However, in low-risk OSCC, survival could be improved due to IELs. IELs in junctional epithelium are involved in regulating the host-microbiota interactions to mediate the development of periodontal disease. Additionally, IELs in glands are increased in patients with pSS and are involved in the destruction of the gland and ductal tissue. However, the exact role played by IELs in the occurrence and development of oral diseases is still largely unknown. Future studies should not only investigate the biological functions and precise molecular mechanisms of IELs in oral diseases, but also address the clinical applications of IELs, aiming to facilitate the clinical translation.
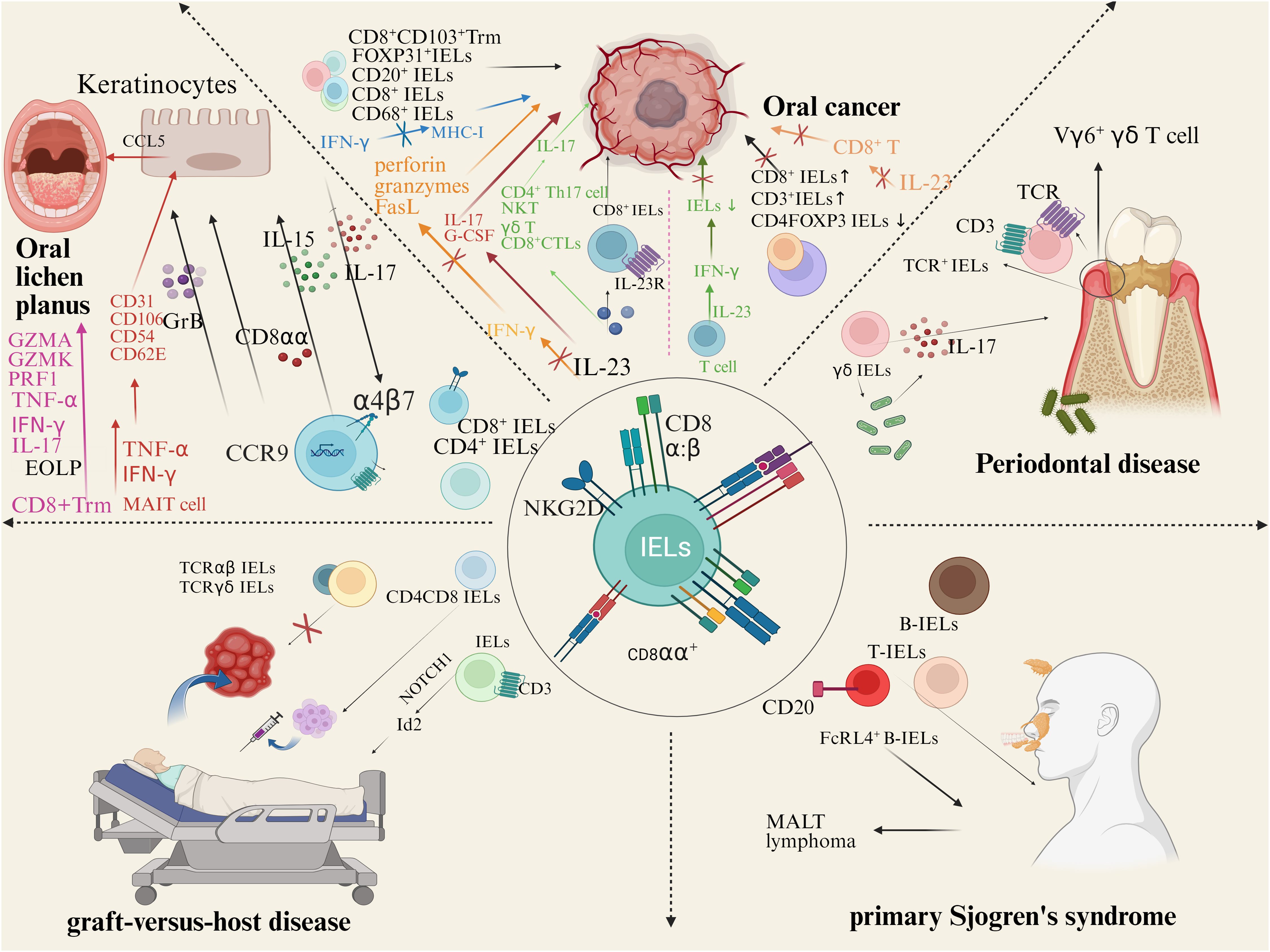
Figure 3. IELs and human oral diseases. There is approximately a 1:2 ratio of CD4+IELs/CD8+IELs in OLP. CD8αα and Integrin αE (CD103) β7 (αEβ7) mediates cell migration and homing. TCRγδ+ IELs recognize autoantigen molecules expressed by damaged keratinocytes, activate the NKG2D receptor pathway. IL-15 secreted by epithelial cells can induce NKG2D activation and upregulation on the surface of IELs, preventing the activation of the inhibitory receptor NKG2A. Co-localization of IELs with IL-7 secreted by epithelial cells. In lip carcinogenesis, peritumoral and intratumoral CD3+, CD8+, CD20+ and CD68+ cells increase. In tongue cancers, CD8+ IELs frequently expressed PD-1. IL-23 promote the activation of CD8+ IELs. IELs in junctional epithelium express TCR and CD3. Most gingival γδ IELs are adjacent to dental biofilm. γδ IELs induce a large amount of IL-17, which mediates the development and progression of PD. IELs were increased in the gallbladder of patients with HCT. High expression of CD4, CD8, and FOXP3 in GVHD confirmed that oral cGVHD is primarily driven by T cells. IELs expressing CD3 in human and mouse can differentiate along the transcription factor Id 2-independent pathway under NOTCH1 signaling. TCRαβ/CD19 cell depletion is often used in HCT. γδ T cells may undergo CXCR4 signaling to recruit alloreactive CD4 T cells to target tissues. B-IELs were found in almost all striated ducts with hyperplasia in lymphoepithelial lesions (LELs). FcRL4+ B-IELs are present in the salivary glands of pSS patients. B-IELs/T-IELs ratios increased significantly with higher severity of LELs.
Author contributions
D-YZ: Writing – original draft. C-FB: Writing – review & editing. GZ: Writing – review & editing.
Funding
The author(s) declare that financial support was received for the research and/or publication of this article. This work was supported by grants from the National Natural Science Foundation of China and the Scientific Research Project of Traditional Chinese Medicine from Hubei Provincial Administration of Traditional Chinese Medicine (Nos. 82270983 and ZY2023M024).
Conflict of interest
The authors declare that the research was conducted in the absence of any commercial or financial relationships that could be construed as a potential conflict of interest.
Generative AI statement
The author(s) declare that no Generative AI was used in the creation of this manuscript.
Publisher’s note
All claims expressed in this article are solely those of the authors and do not necessarily represent those of their affiliated organizations, or those of the publisher, the editors and the reviewers. Any product that may be evaluated in this article, or claim that may be made by its manufacturer, is not guaranteed or endorsed by the publisher.
References
1. Van Kaer L, Olivares-Villagomez D. Development, homeostasis, and functions of intestinal intraepithelial lymphocytes. J Immunol. (2018) 200:2235–44. doi: 10.4049/jimmunol.1701704
2. Kawaguchi M, Nanno M, Umesaki Y, Matsumoto S, Okada Y, Cai Z, et al. Cytolytic activity of intestinal intraepithelial lymphocytes in germ-free mice is strain dependent and determined by T cells expressing gamma delta T-cell antigen receptors. Proc Natl Acad Sci USA. (1993) 90:8591–4. doi: 10.1073/pnas.90.18.8591
3. Baghdadi MB, Kim TH. The multiple roles of enteric glial cells in intestinal homeostasis and regeneration. Semin Cell Dev Biol. (2023) 150-151:43–9. doi: 10.1016/j.semcdb.2023.01.005
4. Afzali S, Mohammadisoleimani E, Mansoori Y, Mohaghegh P, Bahmanyar M, Mansoori B, et al. The potential roles of Th17 cells in the pathogenesis of oral lichen planus. Inflammation Res. (2023) 72:1513–24. doi: 10.1007/s00011-023-01763-7
5. Eichberger J, Spoerl S, Spanier G, Erber R, Taxis J, Schuderer J, et al. TIGIT expression on intratumoral lymphocytes correlates with improved prognosis in oral squamous cell carcinoma. Biomedicines. (2022) 10:3236. doi: 10.3390/biomedicines10123236
6. Gaafar NM, Osman TA, Ahmed IA, Elsheikh M, Dongre H, Jacobsen MR, et al. Characterization of immune cell infiltrate in tumor stroma and epithelial compartments in oral squamous cell carcinomas of Sudanese patients. Clin Exp Dent Res. (2022) 8:130–40. doi: 10.1002/cre2.v8.1
7. Boxberg M, Leising L, Steiger K, Jesinghaus M, Alkhamas A, Mielke M, et al. Composition and clinical impact of the immunologic tumor microenvironment in oral squamous cell carcinoma. J Immunol. (2019) 202:278–91. doi: 10.4049/jimmunol.1800242
8. Feng Y, Chen Z, Tu SQ, Wei JM, Hou YL, Kuang ZL, et al. Role of interleukin-17A in the pathomechanisms of periodontitis and related systemic chronic inflammatory diseases. Front Immunol. (2022) 13:862415. doi: 10.3389/fimmu.2022.862415
9. Gonzalez IA, Linn R. Clinicopathologic characterization of gallbladder graft-versus-host disease in the pediatric population. Hum Pathol. (2023) 139:9–16. doi: 10.1016/j.humpath.2023.06.006
10. van Ginkel MS, van der Sluis T, Bulthuis MLC, Buikema HJ, Haacke EA, Arends S, et al. Digital image analysis of intraepithelial B-lymphocytes to assess lymphoepithelial lesions in salivary glands of Sjogren’s syndrome patients. Rheumatol (Oxford). (2022) 62:428–38. doi: 10.1093/rheumatology/keac212
11. Qiu Y, Wang W, Xiao W, Yang H. Role of the intestinal cytokine microenvironment in shaping the intraepithelial lymphocyte repertoire. J Leukoc Biol. (2015) 97:849–57. doi: 10.1189/jlb.3RU1014-465R
12. Fuchs A, Vermi W, Lee JS, Lonardi S, Gilfillan S, Newberry RD, et al. Intraepithelial type 1 innate lymphoid cells are a unique subset of IL-12- and IL-15-responsive IFN-gamma-producing cells. Immunity. (2013) 38:769–81. doi: 10.1016/j.immuni.2013.02.010
13. Simoni Y, Fehlings M, Kløverpris HN, McGovern N, Koo SL, Loh CY, et al. Human innate lymphoid cell subsets possess tissue-type based heterogeneity in phenotype and frequency. Immunity. (2017) 46:148–61. doi: 10.1016/j.immuni.2016.11.005
14. Talayero P, Mancebo E, Calvo-Pulido J, Rodríguez-Muñoz S, Bernardo I, Laguna-Goya R, et al. Innate lymphoid cells groups 1 and 3 in the epithelial compartment of functional human intestinal allografts. Am J Transplant. (2016) 16:72–82. doi: 10.1111/ajt.13435
15. Van Acker A, Gronke K, Biswas A, Martens L, Saeys Y, Filtjens J, et al. A murine intestinal intraepithelial NKp46-negative innate lymphoid cell population characterized by group 1 properties. Cell Rep. (2017) 19:1431–43. doi: 10.1016/j.celrep.2017.04.068
16. Ettersperger J, Montcuquet N, Malamut G, Guegan N, Lopez-Lastra S, Gayraud S, et al. Interleukin-15-dependent T-cell-like innate intraepithelial lymphocytes develop in the intestine and transform into lymphomas in celiac disease. Immunity. (2016) 45:610–25. doi: 10.1016/j.immuni.2016.07.018
17. Olivares-Villagomez D, Van Kaer L. Intestinal intraepithelial lymphocytes: sentinels of the mucosal barrier. Trends Immunol. (2018) 39:264–75. doi: 10.1016/j.it.2017.11.003
18. Van Kaer L, Algood HMS, Singh K, Parekh VV, Greer MJ, Piazuelo MB, et al. CD8alphaalpha(+) innate-type lymphocytes in the intestinal epithelium mediate mucosal immunity. Immunity. (2014) 41:451–64. doi: 10.1016/j.immuni.2014.08.010
19. Kumar AA, Delgado AG, Piazuelo MB, Van Kaer L, Olivares-Villagómez D. Innate CD8alphaalpha(+) lymphocytes enhance anti-CD40 antibody-mediated colitis in mice. Immun Inflammation Dis. (2017) 5:109–23. doi: 10.1002/iid3.2017.5.issue-2
20. Adams EJ, Chien YH, Garcia KC. Structure of a gammadelta T cell receptor in complex with the nonclassical MHC T22. Science. (2005) 308:227–31. doi: 10.1126/science.1106885
21. Adams EJ, Strop P, Shin S, Chien YH, Garcia KC. An autonomous CDR3delta is sufficient for recognition of the nonclassical MHC class I molecules T10 and T22 by gammadelta T cells. Nat Immunol. (2008) 9:777–84. doi: 10.1038/ni.1620
22. Wang HC, Klein JR. Multiple levels of activation of murine CD8(+) intraepithelial lymphocytes defined by OX40 (CD134) expression: effects on cell-mediated cytotoxicity, IFN-gamma, and IL-10 regulation. J Immunol. (2001) 167:6717–23. doi: 10.4049/jimmunol.167.12.6717
23. Swamy M, Abeler-Dörner L, Chettle J, Mahlakõiv T, Goubau D, Chakravarty P, et al. Intestinal intraepithelial lymphocyte activation promotes innate antiviral resistance. Nat Commun. (2015) 6:7090. doi: 10.1038/ncomms8090
24. Fan X, Rudensky AY. Hallmarks of tissue-resident lymphocytes. Cell. (2016) 164:1198–211. doi: 10.1016/j.cell.2016.02.048
25. Meresse B, Chen Z, Ciszewski C, Tretiakova M, Bhagat G, Krausz TN, et al. Coordinated induction by IL15 of a TCR-independent NKG2D signaling pathway converts CTL into lymphokine-activated killer cells in celiac disease. Immunity. (2004) 21:357–66. doi: 10.1016/j.immuni.2004.06.020
26. Itsumi M, Yoshikai Y, Yamada H. IL-15 is critical for the maintenance and innate functions of self-specific CD8(+) T cells. Eur J Immunol. (2009) 39:1784–93. doi: 10.1002/eji.200839106
27. Ismail AS, Behrendt CL, Hooper LV. Reciprocal interactions between commensal bacteria and gamma delta intraepithelial lymphocytes during mucosal injury. J Immunol. (2009) 182:3047–54. doi: 10.4049/jimmunol.0802705
28. Fahrer AM, Konigshofer Y, Kerr EM, Ghandour G, Mack DH, Davis MM, et al. Attributes of gammadelta intraepithelial lymphocytes as suggested by their transcriptional profile. Proc Natl Acad Sci USA. (2001) 98:10261–6. doi: 10.1073/pnas.171320798
29. Shires J, Theodoridis E, Hayday AC. Biological insights into TCRgammadelta+ and TCRalphabeta+ intraepithelial lymphocytes provided by serial analysis of gene expression (SAGE). Immunity. (2001) 15:419–34. doi: 10.1016/S1074-7613(01)00192-3
30. Wang H, Zhang D, Han Q, Zhao X, Zeng X, Xu Y, et al. Role of distinct CD4(+) T helper subset in pathogenesis of oral lichen planus. J Oral Pathol Med. (2016) 45:385–93. doi: 10.1111/jop.2016.45.issue-6
31. Li Y, Innocentin S, Withers DR, Roberts NA, Gallagher AR, Grigorieva EF, et al. Exogenous stimuli maintain intraepithelial lymphocytes via aryl hydrocarbon receptor activation. Cell. (2011) 147:629–40. doi: 10.1016/j.cell.2011.09.025
32. Stange J, Veldhoen M. The aryl hydrocarbon receptor in innate T cell immunity. Semin Immunopathol. (2013) 35:645–55. doi: 10.1007/s00281-013-0389-1
33. Konjar S, Ferreira C, Blankenhaus B, Veldhoen M. Intestinal barrier interactions with specialized CD8 T cells. Front Immunol. (2017) 8:1281. doi: 10.3389/fimmu.2017.01281
34. Klose CS, Blatz K, d'Hargues Y, Hernandez PP, Kofoed-Nielsen M, Ripka JF, et al. The transcription factor T-bet is induced by IL-15 and thymic agonist selection and controls CD8alphaalpha(+) intraepithelial lymphocyte development. Immunity. (2014) 41:230–43. doi: 10.1016/j.immuni.2014.06.018
35. Jiang W, Wang X, Zeng B, Liu L, Tardivel A, Wei H, et al. Recognition of gut microbiota by NOD2 is essential for the homeostasis of intestinal intraepithelial lymphocytes. J Exp Med. (2013) 210:2465–76. doi: 10.1084/jem.20122490
36. Dobbins WR. Human intestinal intraepithelial lymphocytes. Gut. (1986) 27:972–85. doi: 10.1136/gut.27.8.972
37. Wu RQ, Zhang DF, Tu E, Chen QM, Chen W. The mucosal immune system in the oral cavity-an orchestra of T cell diversity. Int J Oral Sci. (2014) 6:125–32. doi: 10.1038/ijos.2014.48
38. Wu R, Zhang D, Zanvit P, Jin W, Wang H, Chen W. Identification and regulation of TCRalphabeta(+)CD8alphaalpha(+) intraepithelial lymphocytes in murine oral mucosa. Front Immunol. (2020) 11:1702. doi: 10.3389/fimmu.2020.01702
39. Flores-Hidalgo A, Phero J, Steward-Tharp S, Williamson M, Paquette D, Krishnan D, et al. Immunophenotypic and gene expression analyses of the inflammatory microenvironment in high-grade oral epithelial dysplasia and oral lichen planus. Head Neck Pathol. (2024) 18:17. doi: 10.1007/s12105-024-01624-7
40. Yang JY, Wang F, Zhou G. Characterization and function of circulating mucosal-associated invariant T cells and gammadeltaT cells in oral lichen planus. J Oral Pathol Med. (2022) 51:74–85. doi: 10.1111/jop.13250
41. Enomoto A, Sato E, Yasuda T, Isomura T, Nagao T, Chikazu D. Intraepithelial CD8+ lymphocytes as a predictive diagnostic biomarker for the remission of oral lichen planus. Hum Pathol. (2018) 74:43–53. doi: 10.1016/j.humpath.2017.12.008
42. Soler-Ferran D, Louis F, Woo SB, Greenberg SA. Infiltration of mature KLRG1 expressing cytotoxic T cells in oral lichen planus. Head Neck Pathol. (2022) 16:1124–9. doi: 10.1007/s12105-022-01472-3
43. Zhou XJ, Sugerman PB, Savage NW, Walsh LJ, Seymour GJ. Intra-epithelial CD8+ T cells and basement membrane disruption in oral lichen planus. J Oral Pathol Med. (2002) 31:23–7. doi: 10.1046/j.0904-2512.2001.10063.x
44. Khan A, Farah CS, Savage NW, Walsh LJ, Harbrow DJ, Sugerman PB. Th1 cytokines in oral lichen planus. J Oral Pathol Med. (2003) 32:77–83. doi: 10.1034/j.1600-0714.2003.00077.x
45. Bismuth G, Boumsell L. Controlling the immune system through semaphorins. Sci STKE. (2002) 2002:re4. doi: 10.1126/stke.1282002re4
46. Takei Y, Nemoto Y, Morikawa R, Tanaka S, Oshima S, Nagaishi T, et al. CD8alphaalpha(+) T cells show amoeboid shape and frequent morphological change in vitro, and localize to small intestinal intraepithelial region in vivo. Biochem Biophys Res Commun. (2020) 523:328–35. doi: 10.1016/j.bbrc.2019.12.021
47. Qing M, Yang D, Shang Q, Peng J, Deng J, Lu J, et al. CD8(+) tissue-resident memory T cells induce oral lichen planus erosion via cytokine network. Elife. (2023) 12:e83981. doi: 10.7554/eLife.83981.sa2
48. Nukaly HY, Halawani IR, Alghamdi SMS, Alruwaili AG, Binhezaim A, Algahamdi RAA, et al. Oral lichen planus: A narrative review navigating etiologies, clinical manifestations, diagnostics, and therapeutic approaches. J Clin Med. (2024) 13:5280. doi: 10.3390/jcm13175280
49. Jenkinson SE, Whawell SA, Swales BM, Corps EM, Kilshaw PJ, Farthing PM. The alphaE(CD103)β7 integrin interacts with oral and skin keratinocytes in an E-cadherin-independent manner*. Immunology. (2011) 132:188–96. doi: 10.1111/j.1365-2567.2010.03352.x
50. Edelblum KL, Shen L, Weber CR, Marchiando AM, Clay BS, Wang Y, et al. Dynamic migration of gammadelta intraepithelial lymphocytes requires occludin. Proc Natl Acad Sci U.S.A. (2012) 109:7097–102. doi: 10.1073/pnas.1112519109
51. Edelblum KL, Singh G, Odenwald MA, Lingaraju A, El Bissati K, McLeod R, et al. gammadelta intraepithelial lymphocyte migration limits transepithelial pathogen invasion and systemic disease in mice. Gastroenterology. (2015) 148:1417–26. doi: 10.1053/j.gastro.2015.02.053
52. Bhagat G, Naiyer AJ, Shah JG, Harper J, Jabri B, Wang TC, et al. Small intestinal CD8+TCRgammadelta+NKG2A+ intraepithelial lymphocytes have attributes of regulatory cells in patients with celiac disease. J Clin Invest. (2008) 118:281–93. doi: 10.1172/JCI30989
53. Hoytema VKD, Mucida D. Intraepithelial lymphocytes. Curr Biol. (2017) 27:R737–9. doi: 10.1016/j.cub.2017.05.073
54. Fan Q, Nan H, Li Z, Li B, Zhang F, Bi L, et al. New insights into MAIT cells in autoimmune diseases. BioMed Pharmacother. (2023) 159:114250. doi: 10.1016/j.biopha.2023.114250
55. Leeansyah E, Svärd J, Dias J, Buggert M, Nyström J, Quigley MF, et al. Arming of MAIT cell cytolytic antimicrobial activity is induced by IL-7 and defective in HIV-1 infection. PloS Pathog. (2015) 11:e1005072. doi: 10.1371/journal.ppat.1005072
56. Lepore M, Kalinichenko A, Colone A, Paleja B, Singhal A, Tschumi A, et al. Parallel T-cell cloning and deep sequencing of human MAIT cells reveal stable oligoclonal TCRbeta repertoire. Nat Commun. (2014) 5:3866. doi: 10.1038/ncomms4866
57. Kelly J, Minoda Y, Meredith T, Cameron G, Philipp MS, Pellicci DG, et al. Chronically stimulated human MAIT cells are unexpectedly potent IL-13 producers. Immunol Cell Biol. (2019) 97:689–99. doi: 10.1111/imcb.12281
58. Li J, Ireland GW, Farthing PM, Thornhill MH. Epidermal and oral keratinocytes are induced to produce RANTES and IL-8 by cytokine stimulation. J Invest Dermatol. (1996) 106:661–6. doi: 10.1111/1523-1747.ep12345482
59. Little MC, Watson RE, Pemberton MN, Griffiths CE, Thornhill MH. Activation of oral keratinocytes by mercuric chloride: relevance to dental amalgam-induced oral lichenoid reactions. Br J Dermatol. (2001) 144:1024–32. doi: 10.1046/j.1365-2133.2001.04193.x
60. Meager A. Cytokine regulation of cellular adhesion molecule expression in inflammation. Cytokine Growth Factor Rev. (1999) 10:27–39. doi: 10.1016/S1359-6101(98)00024-0
61. Provine NM, Amini A, Garner LC, Spencer AJ, Dold C, Hutchings C, et al. MAIT cell activation augments adenovirus vector vaccine immunogenicity. Science. (2021) 371:521–6. doi: 10.1126/science.aax8819
62. Wang Y, Zhou J, Fu S, Wang C, Zhou B. A study of association between oral lichen planus and immune balance of th1/th2 cells. Inflammation. (2015) 38:1874–9. doi: 10.1007/s10753-015-0167-4
63. Saloura V, Izumchenko E, Zuo Z, Bao R, Korzinkin M, Ozerov I, et al. Immune profiles in primary squamous cell carcinoma of the head and neck. Oral Oncol. (2019) 96:77–88. doi: 10.1016/j.oraloncology.2019.06.032
64. Markopoulos AK. Current aspects on oral squamous cell carcinoma. Open Dent J. (2012) 6:126–30. doi: 10.2174/1874210601206010126
65. Choi S, Myers JN. Molecular pathogenesis of oral squamous cell carcinoma: implications for therapy. J Dent Res. (2008) 87:14–32. doi: 10.1177/154405910808700104
66. Magalhães IA, Costa GAJ, Borges MMF, Carlos ACAM, Mesquita KC, Arruda LMA, et al. Deficiency in infiltration of CD8/CD3 positive lymphocytes and macrophages plays a role in lip carcinogenesis: an immunohistochemical study. Braz J Otorhinolaryngol. (2024) 90:101379. doi: 10.1016/j.bjorl.2023.101379
67. Katou F, Ohtani H, Watanabe Y, Nakayama T, Yoshie O, Hashimoto K. Differing phenotypes between intraepithelial and stromal lymphocytes in early-stage tongue cancer. Cancer Res. (2007) 67:11195–201. doi: 10.1158/0008-5472.CAN-07-2637
68. Bron L, Jandus C, Andrejevic-Blant S, Speiser DE, Monnier P, Romero P, et al. Prognostic value of arginase-II expression and regulatory T-cell infiltration in head and neck squamous cell carcinoma. Int J Cancer. (2013) 132:E85–93. doi: 10.1002/ijc.v132.3
69. Liu S, Wang P, Wang P, Zhao Z, Zhang X, Pan Y, et al. Tissue-resident memory CD103+CD8+ T cells in colorectal cancer: its implication as a prognostic and predictive liver metastasis biomarker. Cancer Immunol Immunother. (2024) 73:176. doi: 10.1007/s00262-024-03709-2
70. Hu MD, Edelblum KL. Sentinels at the frontline: the role of intraepithelial lymphocytes in inflammatory bowel disease. Curr Pharmacol Rep. (2017) 3:321–34. doi: 10.1007/s40495-017-0105-2
71. Langowski JL, Zhang X, Wu L, Mattson JD, Chen T, Smith K, et al. IL-23 promotes tumor incidence and growth. Nature. (2006) 442:461–5. doi: 10.1038/nature04808
72. Langowski JL, Kastelein RA, Oft M. Swords into plowshares: IL-23 repurposes tumor immune surveillance. Trends Immunol. (2007) 28:207–12. doi: 10.1016/j.it.2007.03.006
73. Fukuda M, Ehara M, Suzuki S, Ohmori Y, Sakashita H. IL-23 promotes growth and proliferation in human squamous cell carcinoma of the oral cavity. Int J Oncol. (2010) 36:1355–65. doi: 10.3892/ijo_00000620
74. Ruhle A, Todorovic J, Spohn SSK, Gkika E, Becker C, Knopf A, et al. Prognostic value of tumor-infiltrating immune cells and immune checkpoints in elderly head-and-neck squamous cell carcinoma patients undergoing definitive (chemo)radiotherapy. Radiat Oncol. (2022) 17:181. doi: 10.1186/s13014-022-02153-9
75. Distel LV, Fickenscher R, Dietel K, Hung A, Iro H, Zenk J, et al. Tumor infiltrating lymphocytes in squamous cell carcinoma of the oro- and hypopharynx: prognostic impact may depend on type of treatment and stage of disease. Oral Oncol. (2009) 45:e167–74. doi: 10.1016/j.oraloncology.2009.05.640
76. Hajishengallis G. Periodontitis: from microbial immune subversion to systemic inflammation. Nat Rev Immunol. (2015) 15:30–44. doi: 10.1038/nri3785
77. Nakamura M. Histological and immunological characteristics of the junctional epithelium. Jpn Dent Sci Rev. (2018) 54:59–65. doi: 10.1016/j.jdsr.2017.11.004
78. Sheridan BS, Lefrancois L. Intraepithelial lymphocytes: to serve and protect. Curr Gastroenterol Rep. (2010) 12:513–21. doi: 10.1007/s11894-010-0148-6
79. Tsukamoto Y, Usui M, Yamamoto G, Takagi Y, Tachikawa T, Yamamoto M, et al. Role of the junctional epithelium in periodontal innate defense and homeostasis. J Periodontal Res. (2012) 47:750–7. doi: 10.1111/j.1600-0765.2012.01490.x
80. Hayday A, Theodoridis E, Ramsburg E, Shires J. Intraepithelial lymphocytes: exploring the Third Way in immunology. Nat Immunol. (2001) 2:997–1003. doi: 10.1038/ni1101-997
81. Tonetti MS, Imboden M, Gerber L, Lang NP. Compartmentalization of inflammatory cell phenotypes in normal gingiva and peri-implant keratinized mucosa. J Clin Periodontol. (1995) 22:735–42. doi: 10.1111/j.1600-051X.1995.tb00255.x
82. Wilharm A, Tabib Y, Nassar M, Reinhardt A, Mizraji G, Sandrock I, et al. Mutual interplay between IL-17-producing gammadelta T cells and microbiota orchestrates oral mucosal homeostasis. Proc Natl Acad Sci USA. (2019) 116:2652–61. doi: 10.1073/pnas.1818812116
83. Wald S, Leibowitz A, Aizenbud Y, Saba Y, Zubeidat K, Barel O, et al. gammadelta T cells are essential for orthodontic tooth movement. J Dent Res. (2021) 100:731–8. doi: 10.1177/0022034520984774
84. Jia L, Wu G, Alonso S, Zhao C, Lemenze A, Lam YY, et al. A transmissible gammadelta intraepithelial lymphocyte hyperproliferative phenotype is associated with the intestinal microbiota and confers protection against acute infection. Mucosal Immunol. (2022) 15:772–82. doi: 10.1038/s41385-022-00522-x
85. Chen Y, Du J, Liu Y, Luo Z, Guo L, Xu J, et al. gammadeltaT cells in oral tissue immune surveillance and pathology. Front Immunol. (2022) 13:1050030. doi: 10.1111/prd.12544
86. Yu JJ, Ruddy MJ, Wong GC, Sfintescu C, Baker PJ, Smith JB, et al. An essential role for IL-17 in preventing pathogen-initiated bone destruction: recruitment of neutrophils to inflamed bone requires IL-17 receptor-dependent signals. Blood. (2007) 109:3794–802. doi: 10.1182/blood-2005-09-010116
87. Ono T, Okamoto K, Nakashima T, Nitta T, Hori S, Iwakura Y, et al. IL-17-producing gammadelta T cells enhance bone regeneration. Nat Commun. (2016) 7:10928. doi: 10.1038/ncomms10928
88. Awang RA, Lappin DF, MacPherson A, Riggio M, Robertson D, Hodge P, et al. Clinical associations between IL-17 family cytokines and periodontitis and potential differential roles for IL-17A and IL-17E in periodontal immunity. Inflammation Res. (2014) 63:1001–12. doi: 10.1007/s00011-014-0776-7
89. Bunte K, Beikler T. Th17 cells and the IL-23/IL-17 axis in the pathogenesis of periodontitis and immune-mediated inflammatory diseases. Int J Mol Sci. (2019) 20:3394. doi: 10.3390/ijms20143394
90. Krishnan S, Prise IE, Wemyss K, Schenck LP, Bridgeman HM, McClure FA, et al. Amphiregulin-producing gammadelta T cells are vital for safeguarding oral barrier immune homeostasis. Proc Natl Acad Sci USA. (2018) 115:10738–43. doi: 10.1073/pnas.1802320115
91. Hovav AH, Wilharm A, Barel O, Prinz I. Development and function of gammadeltaT cells in the oral mucosa. J Dent Res. (2020) 99:498–505. doi: 10.1177/0022034520908839
92. Grafanaki K, Lygeros S, Spyridonidis A, Liga M. Tongue graft-versus-host disease: remission with ruxolitinib. BMJ Case Rep. (2022) 15:e247888. doi: 10.1136/bcr-2021-247888
93. Tollemar V, Tudzarovski N, Warfvinge G, Yarom N, Remberger M, Heymann R, et al. Histopathological grading of oral mucosal chronic graft-versus-host disease: large cohort analysis. Biol Blood Marrow Transplant. (2020) 26:1971–9. doi: 10.1016/j.bbmt.2020.06.031
94. Tollemar V, Arvidsson H, Häbel H, Tudzarovski N, Legert KG, Le Blanc K, et al. Grading of minor salivary gland immuno-histopathology post-allogenic hematopoietic cell transplantation. Heliyon. (2023) 9:e15517. doi: 10.1016/j.heliyon.2023.e15517
95. Motta A, Zhan Q, Larson A, Lerman M, Woo SB, Soiffer RJ, et al. Immunohistopathological characterization and the impact of topical immunomodulatory therapy in oral chronic graft-versus-host disease: A pilot study. Oral Dis. (2018) 24:580–90. doi: 10.1111/odi.2018.24.issue-4
96. Hess NJ, Turicek DP, Riendeau J, McIlwain SJ, Contreras Guzman E, Nadiminti K, et al. Inflammatory CD4/CD8 double-positive human T cells arise from reactive CD8 T cells and are sufficient to mediate GVHD pathology. Sci Adv. (2023) 9:eadf0567. doi: 10.1126/sciadv.adf0567
98. Manoussakis MN, Kapsogeorgou EK. The role of intrinsic epithelial activation in the pathogenesis of Sjogren’s syndrome. J Autoimmun. (2010) 35:219–24. doi: 10.1016/j.jaut.2010.06.011
99. Haacke EA, Bootsma H, Spijkervet FKL, Visser A, Vissink A, Kluin PM, et al. FcRL4(+) B-cells in salivary glands of primary Sjogren’s syndrome patients. J Autoimmun. (2017) 81:90–8. doi: 10.1016/j.jaut.2017.03.012
100. Verstappen GM, Ice JA, Bootsma H, Pringle S, Haacke EA, de Lange K, et al. Gene expression profiling of epithelium-associated FcRL4(+) B cells in primary Sjogren’s syndrome reveals a pathogenic signature. J Autoimmun. (2020) 109:102439. doi: 10.1016/j.jaut.2020.102439
101. Yeo L, Lom H, Juarez M, Snow M, Buckley CD, Filer A, et al. Expression of FcRL4 defines a pro-inflammatory, RANKL-producing B cell subset in rheumatoid arthritis. Ann Rheum Dis. (2015) 74:928–35. doi: 10.1136/annrheumdis-2013-204116
102. Falini B, Tiacci E, Pucciarini A, Bigerna B, Kurth J, Hatzivassiliou G, et al. Expression of the IRTA1 receptor identifies intraepithelial and subepithelial marginal zone B cells of the mucosa-associated lymphoid tissue (MALT). Blood. (2003) 102:3684–92. doi: 10.1182/blood-2003-03-0750
103. Delli K, Haacke EA, Kroese FG, Pollard RP, Ihrler S, van der Vegt B, et al. Towards personalized treatment in primary Sjogren’s syndrome: baseline parotid histopathology predicts responsiveness to rituximab treatment. Ann Rheum Dis. (2016) 75:1933–8. doi: 10.1136/annrheumdis-2015-208304
104. Pijpe J, Meijer JM, Bootsma H, van der Wal JE, Spijkervet FK, Kallenberg CG, et al. Clinical and histologic evidence of salivary gland restoration supports the efficacy of rituximab treatment in Sjogren’s syndrome. Arthritis Rheum. (2009) 60:3251–6. doi: 10.1002/art.v60:11
Keywords: intraepithelial lymphocytes, oral lichen planus, oral squamous carcinoma, periodontal disease, graft-versus-host disease, primary Sjogren’s syndrome
Citation: Zhou D-Y, Bao C-F and Zhou G (2025) Intraepithelial lymphocytes in human oral diseases. Front. Immunol. 16:1597088. doi: 10.3389/fimmu.2025.1597088
Received: 20 March 2025; Accepted: 14 April 2025;
Published: 08 May 2025.
Edited by:
Karol Sestak, PreCliniTria, LLC., United StatesReviewed by:
Roberto Rosales-Reyes, National Autonomous University of Mexico, MexicoHouriah Y. Nukaly, Batterjee Medical College, Saudi Arabia
Copyright © 2025 Zhou, Bao and Zhou. This is an open-access article distributed under the terms of the Creative Commons Attribution License (CC BY). The use, distribution or reproduction in other forums is permitted, provided the original author(s) and the copyright owner(s) are credited and that the original publication in this journal is cited, in accordance with accepted academic practice. No use, distribution or reproduction is permitted which does not comply with these terms.
*Correspondence: Gang Zhou, emhvdWdhbmdAd2h1LmVkdS5jbg==