- Science for Life Laboratory, Department of Medical Biochemistry and Microbiology, Uppsala University, Uppsala, Sweden
Paraneoplastic syndromes represent a clinically heterogeneous group of disorders that arise in cancer patients. Although their underlying mechanisms are only partly understood, immune or endocrine mechanisms are believed to play key roles. Autoimmune-mediated paraneoplastic syndromes (AMPS) are typically characterized by the presence of autoantibodies, making their identification important for both AMPS diagnosis and early cancer detection. This review synthesizes emerging insights into the pathogenesis of AMPS, with a particular focus on how genomic instability in cancer cells promotes immune recognition of altered self-proteins. Mechanisms such as ectopic expression, protein modifications (such as isoaspartylation), and gene amplifications can disrupt immune tolerance, leading to autoimmunity. Additionally, chronic inflammation and the formation of tertiary lymphoid structures within the tumor microenvironment contribute to both antitumor immunity and autoimmunity. Immune checkpoint inhibitors (ICIs), have revolutionized cancer treatment by enhancing antitumor immunity, but they can also induce immune-related adverse events (irAEs), some of which mimic AMPS. These irAEs highlight the critical roles of both humoral and cellular immunity in AMPS development. By exploring the relationships between ICI treatment, immune tolerance, and tumor-specific antigens, this review aims to clarify the mechanisms driving AMPS and their dual role in cancer control and immune-mediated disease. Bridging these knowledge gaps may inform the development of novel therapeutic strategies for managing AMPS and in optimizing the use of ICIs in cancer care.
1 Introduction
Paraneoplastic syndromes (PS) comprise a diverse group of disorders that affect different organ systems in cancer patients, arising independently of the primary tumor or its metastases. Their clinical manifestations are often distant from the tumor site and are thought to result from humeral factors produced by cancer cells or by immune system reactions. Due to their rarity and clinical heterogeneity, the reported incidence of PS varies widely, ranging from 7% to 33% of cancer patients (1, 2). These syndromes typically manifest within ±3 years of cancer diagnosis and are more frequently identified after cancer is detected (3).
The mechanisms underlying PS are incompletely understood and diverse. Non-autoimmune-mediated PS are often driven by endocrine imbalances (4), such as hyponatremia, hypercalcemia, or Cushing’s syndrome, as well as cytokine dysregulation (5), including neutrophilia, Trousseau’s syndrome, and thrombocytosis. These cases are associated with poor patient outcomes (5). In contrast, autoimmune-mediated paraneoplastic syndromes are linked to better cancer prognoses, suggesting that autoimmune flares are a sign of enhanced antitumor responses.
Autoimmune-mediated paraneoplastic syndromes (AMPS) most commonly affect the central nervous system (CNS) but can also manifest in the peripheral nervous system (PNS), skin, or blood (6). A hallmark of AMPS is the presence of autoantibodies, which have gained attention for their role as disease biomarkers (7). These autoantibodies are important in the diagnosis of paraneoplastic syndromes and may support early cancer detection in this group of patients. For instance, individual autoantibodies, such as p53 (8), HER2 (9), and NY-ESO-1 (10), as well as autoantibody panels, have shown predictive potential for cancer diagnosis (11, 12). However, these biomarkers still fail to meet clinical standards (13). Important observations have been made linking cancer alterations with commonly associated autoantibodies and the development of AMPS (Table 1).
Despite advances in autoantibody research, the cellular and molecular mechanisms underlying AMPS pathogenesis remain poorly understood. This review aims to summarize the current state of research on AMPS exploring the mechanisms driving autoimmune disorders in cancer. Specifically, it will address how ectopic expression, protein structural alterations, and overexpression of self-proteins may trigger autoimmune responses. Overlapping clinical presentations between AMPS and immune-related adverse events (irAEs) of immune checkpoint inhibitor (ICI) treatment will be discussed, highlighting the need to understand autoimmunity in cancer. Further, the roles of chronic inflammation within the tumor microenvironment (TME) and localized immune tolerance in sustaining these responses will be examined. With this discussion, we aim to bridge existing knowledge gaps and provide a framework to better understand AMPS pathogenesis.
2 Protein overexpression, ectopic expression and structure changes drive autoimmunity in cancer
Ectopic expression refers to the production of a self-protein in atypical tissues. Malignant cells frequently exhibit ectopic expression of otherwise tissue-restricted proteins as a consequence of genomic instability. Genomic instability may also trigger missense mutations that may lead to changes in protein structure. Similarly, an antigen may be overexpressed due to chromosome amplifications. In cancer, any of these alterations can induce immune recognition and targeting of malignant cells. In the following AMPS cases, evidence has been provided of how genetic or proteomic alterations can lead to immune recognition and the development of autoimmunity.
2.1 Anti-Hu: encephalomyelitis
The Hu protein family comprises RNA-binding proteins primarily expressed in neuronal cells, where they play a role in mRNA stabilization and translation (40). HuD, a member of this family, is known to be expressed in several cancer types (41). Small cell lung cancer (SCLC) patients with paraneoplastic sensory neuropathy or encephalomyelitis present high anti-Hu antibody titers (42). These antibodies target tumor-expressed Hu proteins, which would otherwise be restricted to neuronal cells. Interestingly, while all SCLC patients with neurological AMPS are anti-Hu positive, 17% of all SCLC patients, irrespective of paraneoplastic presentation, harbor detectable anti-Hu titers (43).
Changes in protein structure can occur after protein folding, contributing to the development of autoimmunity. HuD (also known as ELAVL4) commonly undergoes isoaspartylation, a modification that can impair its physiological activity. In the healthy CNS, PIMT (peptidyl-isomerase methyltransferase) repairs this damage, restoring HuD to its native, functional form (44). However, because PIMT expression is restricted to certain tissues (45), HuD-expressing tumors accumulate isoaspartylated HuD. This ectopically expressed protein variant can be recognized as a non-self-antigen, triggering an immune response in patients with HuD-expressing cancers. In rare cases, the development of anti-HuD autoantibodies may lead to cross-reactivity and recognition of the wild-type HuD protein in the CNS (46).
Pulido M. and colleagues tested serum from seven anti-HuD positive SCLC patients, four of whom had paraneoplastic syndromes against both native and isoaspartylated HuD. All seven samples reacted with both protein isoforms, suggesting not only immune stimulation of isoaspartylated HuD but also cross-reactivity toward native HuD (46). This cross-reactivity explains the paraneoplastic presentation in some of these patients. Isoaspartyl post-translational modifications has been shown to cause immunogenicity and break self-tolerance before (47).
2.2 Anti-recoverin: cancer-associated retinopathy
Cancer-associated retinopathy (CAR) has been observed across various cancer types, predominantly in SCLC and breast cancer. CAR is characterized by progressive sight loss due to autoimmune targeting of retinal proteins. The rarity of this syndrome has made it difficult to reliably identify the underlying autoantibody, although markers against specific retinal proteins like transducin and recoverin have been reported (48, 49).
Sera from 143 cancer patients (99 SCLC and 44 non-small cell lung cancer [NSCLC]) was tested for anti-recoverin antibodies by immunoblotting. Anti-recoverin positivity was observed in 15% and 20% of SCLC and NSCLC cases, respectively. No healthy controls showed anti-recoverin positivity (50). Tumor samples were available from 44 SCLC and 40 NSCLC patients. Recoverin expression was detected by immunohistochemistry in 68% of SCLC and 85% of NSCLC patients (50). All symptomatic CAR-SCLC patients test positive for anti-recoverin autoantibodies, likely due to ectopic expression of retinal proteins (51). A clinical report of a uterine carcinosarcoma patient also revealed serum positivity for anti-recoverin antibodies. Postmortem immunofluorescence analysis detected recoverin expression in the tumor cells (52).
2.3 Anti-VGCC: paraneoplastic LEMS
SCLC cells can aberrantly express voltage-gated calcium channels (VGCCs), typically found in presynaptic nerve terminals of the CNS and at neuromuscular junctions (53). The autoimmune disorder Lambert–Eaton myasthenic syndrome (LEMS) arises from the production of anti-P/Q-type VGCC autoantibodies, leading to muscle weakness, dry mouth, and neurodegenerative features (54). LEMS is a paraneoplastic syndrome in approximately 62% of cases (55), with all SCLC-LEMS patients exhibiting anti-P/Q-type VGCC autoantibodies (56), and 25% of them presenting neurological symptoms in addition to the predominant muscle weakness (57).
Not all anti-VGCC positive patients develop LEMS. One study reported that half of the patients (5 out of 10) with anti-VGCC autoantibodies did not exhibit any symptoms of LEMS or neurological damage (58).
2.4 Anti-NMDAR: encephalitis
Another autoimmune disorder with neurological symptoms is anti-N-methyl-D-aspartate receptor (NMDAR) encephalitis, which involves the disruption of a key receptor for excitatory neurotransmission specific to the CNS (59). NMDAR is often expressed in ovarian teratomas (OT), and approximately 37% of anti-NMDAR encephalitis cases are associated with ovarian teratomas, where the encephalitis resolves after tumor resection (60). Further, reports have shown that in these cases, the antibodies targeting NMDAR are generated within the tumor tissue (61). Moreover, patients with ovarian teratomas but without neurological symptoms do not exhibit anti-NMDAR antibodies, supporting their association with neurological complications (62–64).
2.5 Anti-RPC1: systemic sclerosis
Anti-RNA polymerase III subunit C (RPC1) autoantibodies are commonly found in patients with systemic sclerosis (SSc) (65). A study of eight RPC+ paraneoplastic SSc patients with different forms of cancer revealed that the tumors in five of these patients exhibited genetic alterations in the POLR3A locus. Three of them had a missense mutation, and all five presented with loss of heterozygosity. Every patient harbored at least one genetic alteration, strongly suggesting that these genetic changes triggered an immune response against both the cancer-expressed and the wild-type RNA polymerase protein (66).
2.6 Anti-Yo: cerebellar degeneration
Cerebellar degeneration-related protein 2 (CDR2) and CDR2-like (CDR2L), are neuronal cell proteins typically expressed in Purkinje cells (referred to as Yo antigens) (67) and have been implicated in paraneoplastic cerebellar degeneration. Anti-Yo autoantibody titers were found in a cohort of patients with paraneoplastic cerebellar damage due to ovarian carcinoma. In this group, 17 of 17 tumor samples tested had at least one genetic alteration. Specifically, 59% of cases exhibited a chromosome 17q gain, where CDR2L and CDR2 are located, and 65% of cases had mutations in either or both CDR2L/CDR2 genes (68).
Similarly, a study of 29 anti-Yo positive breast carcinoma patients revealed that all had at least one genetic alteration such as mutations (62.5%), amplifications (61.5%), or 17q gains (38.4%) in the CDR2L/CDR2 genes, which also contributed to paraneoplastic cerebellar degeneration (69). An interesting observation discussed by the authors is that the tumors were highly infiltrated by B and T cells and tended to metastasize early. Not only showing strong anti-tumor responses but also a high tumor invasiveness in this AMPS cohort (69).
2.7 Anti-AChR: myasthenia gravis
Myasthenia Gravis (MG) is an autoimmune disorder that is tightly linked to neoplasia, particularly thymoma. It is estimated that 30% to 40% of thymoma patients develop MG (70, 71). Unlike many cancer-associated autoimmune syndromes, MG is not associated with a favorable prognosis in thymoma patients (72). MG is characterized by progressive muscle weakness that worsens with physical movement, driven by autoantibody activity against neuromuscular-associated proteins. The most common MG autoantibody is anti-acetylcholine receptor (AChR) (85% of MG cases (73)), a protein responsible for signal transmission at the neuromuscular junction (74).
MG-associated thymoma and non-paraneoplastic thymoma tumors were compared by bulk RNA sequencing on two independent studies (75, 76). Both results showed AChR overexpression in MG-associated thymoma samples - 3 and 1.07 fold increase respectively - initially suggesting that protein overexpression may drive autoantibody production. Interestingly, the NEFM (Neurofilament Medium Chain) gene coding for a neuronal protein NEFM was found to be highly expressed (30-fold (75) and >100-fold (76)) in MG-associated thymoma cases. Schultz et al. (1999) showed that NEFM contains a linear epitope that closely resembles an AChR antigen, and that NEFM-specific antibodies could recognize the AChR -subunit, suggesting that anti-AChR activity is promoted by immunity against NEFM (77). Similar epitopes in thymoma MG samples that may trigger cross-reacting antibodies against titin and RYR have also been proposed (75).
3 Immune checkpoint inhibitors and immune-related adverse events
In the previous section, we discussed cases of AMPS that arise independently of cancer treatment. Immune-modulating treatments such as ICIs can trigger irAEs, some of which share similarities with AMPS. This has become increasingly relevant given the quick adaptation of ICIs into the clinic, driven by their ability to improve overall survival while causing fewer general adverse effects than traditional therapies (78–80).
Immune checkpoints prevent overactivation of cytotoxic T cells, ensuring that self-tolerance is maintained. PD-L1 for instance, dampens T cell cytotoxicity through interaction with PD-1 expressed by T cells. This regulatory function is controlled by immune cells but can also be mediated in immune-privileged tissues such as the lung, liver, and placenta (81). Cancer cells exploit immune checkpoints by upregulating inhibitory signals such as PD-L1 (interacting with PD-1 (82) and CD80 (interacting with CTLA-4 (83)) to evade immune destruction and create an immunosuppressive tumor microenvironment. By inhibiting these pathways, ICIs enhance T cell recognition of cancer cells, but also increase the risk of autoimmunity, particularly in individuals with a history of autoimmune disorders (84, 85).
Several irAEs observed in ICI-treated patients overlap with AMPS; disorders like myositis, encephalitis, SSc, and MG are among the most prevalent overlapping conditions. While clinical similarities suggest that ICI treatment could increase the risk of developing AMPS, there are many contrasting features between them. For example, a systematic review by Buckley et al. (2025) on ICI-related encephalitis, found that only 46.9% of cases were autoantibody-positive (86), different from AMPS cases, where autoantibodies are significantly more frequent (100% in SCLC paraneoplastic encephalitis (42), 75% in general paraneoplastic encephalitides (87)). Similarly, in Hamada et al. (2021), only 67% of ICI-related MG cases were positive for any MG-related autoantibody (88) compared to over 95% in thymoma MG (89). A similar trend of seronegative cases has been noted in ICI-related SSc (90).
While seropositive irAEs seem to be a case where ICIs facilitate development of AMPS, a significant proportion of irAE cases are seronegative and appear to have mechanistic differences, where T cell cytotoxicity is the main driver of autoimmunity. Whether these autoreactive T cells are expanded through antitumor immunity, as observed in AMPS, remains to be explored.
Given the high prevalence of severe irAEs of ICI-treated patients (20% (91)), predictive screening tools could help physicians tailor treatment strategies to individual patients. Biomarkers such as blood counts, autoantibodies, HLA genotype, and microRNA expression profiles have been identified as prognostic and diagnostic tools in autoimmune diseases (92). Several studies suggest that pre-existing autoantibodies may indicate an increased risk of developing irAEs following ICI therapy (93, 94). Screening for autoantibodies before initiating ICI therapy could help classify patients based on their likelihood of developing severe irAEs, enabling safer and personalized treatment approaches.
4 Chronic inflammation and localized tolerance breaks
While the presence of autoantibodies is a hallmark of AMPS, not all patients with detectable autoantibodies develop autoimmune symptoms. This discrepancy suggests that additional factors, such as chronic inflammation and localized immune responses within the TME, may determine whether autoimmunity manifests systemically. Understanding these factors is critical for understanding the connection between antitumor immunity and autoimmune pathology.
The disorders summarized earlier demonstrate that AMPS patients harbor autoantibodies capable of inducing immune responses with both cancer-resolving and autoimmune consequences. However, it remains unclear why some patients with autoantibodies benefit from a stronger antitumor response without developing systemic autoimmunity. This observation points to the importance of additional immune triggers beyond humoral activity in the development of AMPS.
Strong antitumor responses, a common feature of AMPS patients, are often accompanied by the presence of tertiary lymphoid structures (TLS) within the chronically inflamed TME (95). TLS are organized immune cell aggregates comprising T cells, B cells, dendritic cells, and macrophages. Functionally resembling secondary lymphoid organs (SLO), TLS promote antigen presentation, B cell priming, and T cell activation (96). In some AMPS cases, the autoimmune response is mediated entirely within the tumor. For example, Al-Diwani et al. (2022) observed that ex vivo cultures of B cells dissociated from ovarian teratomas generated anti-NMDAR antibodies, while B cells from cervical lymph nodes did not produce autoantibodies after tumor resection. This finding supports the idea that autoimmunity is initiated within the tumor-associated TLS (61). Similarly, Mazor et al. (2022) demonstrated that tumor-specific B cells are primed and clonally expanded within the tumor, often originating from naturally occurring self-reactive B cells (97). T cells can also expand and generate antitumor responses within the TLS (98). These findings suggest that TLS facilitate localized antitumor immunity, allowing B and T cells to target self-antigens without eliciting systemic autoimmunity.
This localized immune activity aligns with the observed improved patient prognosis in the presence of TLS (99, 100). Many AMPS cases reviewed here, such as anti-NMDAR encephalitis and anti-Yo cerebellar degeneration, involve highly infiltrated tumors (60, 68, 69). TLS-mediated immunity represents a controlled break in immune tolerance, enabling effective clearance of malignant cells. While circulating autoantibodies are detectable in patient serum, T cells are typically confined to the tumor. A breach in this localization (where T cells migrate from the tumor into circulation) could be the defining trigger for systemic AMPS.
Activated T cells tend to accumulate in inflamed tissues due to retention signals such as the chemokine CXCL10 (101). In the absence of systemic inflammation or additional chronic inflammatory events, these T cells are unlikely to enter the bloodstream. However, in cases of invasive cancers with high levels of circulating tumor cells or early metastatic spread, inflammation at the tumor periphery may promote signals that induce T cell migration. This process could extend the anti-self immune response to healthy tissues, contributing to the onset of paraneoplastic syndromes (Figure 1).
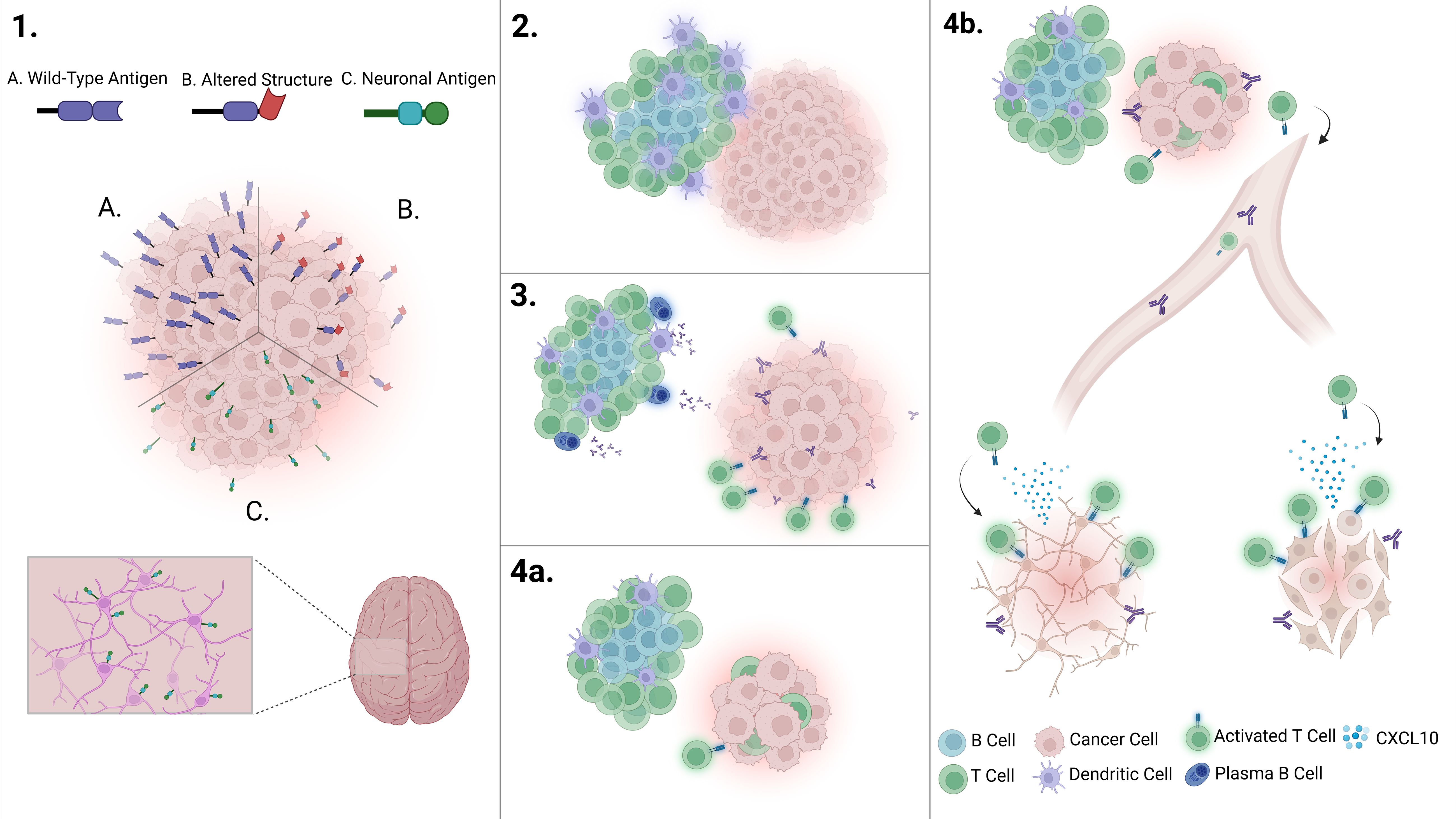
Figure 1. Overview of the pathogenesis of autoimmune-mediated paraneoplastic syndromes. 1. Immune recognition of a tumor occurs through (A) antigen overexpression, (B) altered protein structure, or (C) ectopic antigen expression, initiating an immune response and promoting inflammation. 2. Chronic inflammation within the tumor microenvironment supports the formation of tertiary lymphoid structures (TLS). 3. As the TLS matures, it facilitates the activation of autoreactive tumor-targeting T cells and autoantibody-producing plasma cells, leading to a highly infiltrated tumor. 4a. A robust immune response successfully eliminates the tumor without triggering autoimmune symptoms. While autoantibodies remain detectable in circulation, autoreactive B and T cells remain localized within the tumor microenvironment. 4b. In contrast, remote inflammatory events can recruit T cells, promoting the extravasation of autoreactive T cells. Once in circulation, these T cells can recognize and attack healthy tissues, leading to autoimmunity. Created in BioRender. Perez Bucio, (C) (2025) https://BioRender.com/x3cnql9.
Recent studies suggest that a humoral immune response alone is insufficient to trigger autoimmune symptoms but rather serves as a marker of broken self-antigen tolerance. For instance, in paraneoplastic SSc (66) and anti-Yo-related paraneoplastic cerebellar degeneration (68, 69), the majority of patients exhibited highly invasive or early metastatic malignancies. This suggests that the expansion of an immune response beyond the tumor, driven by T cell migration, could trigger autoimmune disorders. In contrast, patients with autoantibodies but no paraneoplastic symptoms often have a better prognosis, indicating that localized breaches in self-tolerance, mediated by TLS in the TME, serve as a controlled mechanism for combating malignancy.
A break in localized self-tolerance may be the defining trigger for paraneoplastic syndromes. This could explain the high specificity but low sensitivity of autoantibody markers; only a subset of autoantibody-positive patients, presumably those with T cell extravasation into the bloodstream due to extended inflammation, develop paraneoplastic features. Tumor-associated TLS are linked with better prognosis and higher immune infiltration, underscoring their role in cancer immunity. Treatment with ICIs can also facilitate the development of autoimmunity through mechanisms that are often independent of autoantibodies.
5 Conclusion
The roles of autoantibodies and T cell autoreactivity in cancer-related autoimmunity remain incompletely understood, as preclinical and clinical data are still limited. A deeper understanding of AMPS could help us clarify the intersection of TLS biology, immune tolerance, and cancer immunity. Resolving how localized immune responses within the tumor microenvironment contribute to either paraneoplastic syndromes or effective tumor resolution may uncover novel therapeutic targets and biomarkers. The similarities between AMPS and ICI-induced irAEs evidence the complex balance between antitumor activity and autoimmunity, highlighting the need for personalized approaches to cancer immunotherapy. Future research focused on unraveling the mechanisms underlying TLS formation, T cell migration, and systemic immune tolerance breaks, will enable a deeper understanding of these processes and will improve both cancer treatment and the management of autoimmune complications.
Author contributions
CP: Software, Writing – original draft, Visualization, Conceptualization. AB: Data curation, Conceptualization, Writing – review & editing, Validation, Supervision. NL: Funding acquisition, Validation, Conceptualization, Supervision, Project administration, Writing – review & editing.
Funding
The author(s) declare that financial support was received for the research and/or publication of this article. This work was supported by the Swedish Cancer Society.
Conflict of interest
The authors declare that the research was conducted in the absence of any commercial or financial relationships that could be construed as a potential conflict of interest.
Generative AI statement
During the preparation of this work the author(s) used ChatGPT-4 by Open AI in order to edit contents of this manuscript and refine the language of this manuscript. After using this tool/service, the author(s) reviewed and edited the content as needed and take(s) full responsibility for the content of the published article. The author(s) declare that Generative AI was used in the creation of this manuscript.
Publisher’s note
All claims expressed in this article are solely those of the authors and do not necessarily represent those of their affiliated organizations, or those of the publisher, the editors and the reviewers. Any product that may be evaluated in this article, or claim that may be made by its manufacturer, is not guaranteed or endorsed by the publisher.
References
1. Zuffa M, Kubancok J, Rusnák I, Mensatoris K, Horváth A. Early paraneoplastic syndrome in medical oncology: clinicopathological analysis of 1694 patients treated over 20 years. Neoplasma. (1984) 31:231–6.
2. Moldovan T, Boynton D, Kuperus J, Parker J, Noyes SL, Brede CM, et al. Incidence and clinical relevance of paraneoplastic syndromes in patients with renal cell carcinoma. Urol Oncol Semin Orig Investig. (2023) 41:392. doi: 10.1016/j.urolonc.2023.06.015
3. Pelosof LC, Gerber DE. Paraneoplastic syndromes: an approach to diagnosis and treatment. Mayo Clin Proc. (2010) 85:838–54. doi: 10.4065/mcp.2010.0099
4. Dimitriadis GK, Angelousi A, Weickert MO, Randeva HS, Kaltsas G, Grossman A. Paraneoplastic endocrine syndromes. Endocr Relat Cancer. (2017) 24:R173–90. doi: 10.1530/erc-17-0036
5. Kanaji N. Prognosis of patients with paraneoplastic syndromes associated with lung cancer. Austin J Pulm Respir Med. (2014) 1:1006.
6. Darnell RB, Posner JB. Paraneoplastic syndromes. 198 Madison Avenue, New York, New York 10016: Oxford University Press (2010).
7. Chatterjee M, Hurley LC, Tainsky MA. Paraneoplastic antigens as biomarkers for early diagnosis of ovarian cancer. Gynecol Oncol Rep. (2017) 21:37–44. doi: 10.1016/j.gore.2017.06.006
8. Meng R, Wang Y, He L, He Y, Du Z. Potential diagnostic value of serum p53 antibody for detecting colorectal cancer: a meta-analysis. Oncol Lett. (2018) 16:3729–36. doi: 10.3892/ol.2018.8070
9. Tabuchi Y, Shimoda M, Kagara N, Naoi Y, Tanei T, Shimomura A, et al. Protective effect of naturally occurring anti-HER2 autoantibodies on breast cancer. Breast Cancer Res Treat. (2016) 157:55–63. doi: 10.1007/s10549-016-3801-4
10. Saito T, Kurokawa Y, Fujitani K, Kawabata R, Takeno A, Mikami J, et al. Serum NY-ESO-1 antibody as a predictive biomarker for postoperative recurrence of gastric cancer: a multicenter prospective observational study. Br J Cancer. (2024) 130:1157–65. doi: 10.1038/s41416-023-02540-3
11. Patel AJ, Tan T, Richter AG, Naidu B, Blackburn JM, Middleton GW. A highly predictive autoantibody-based biomarker panel for prognosis in early-stage NSCLC with potential therapeutic implications. Br J Cancer. (2021) 126:238–46. doi: 10.1038/s41416-021-01572-x
12. Yang B, Li X, Ren T, Yin Y. Autoantibodies as diagnostic biomarkers for lung cancer: a systematic review. Cell Death Discov. (2019) 5:126. doi: 10.1038/s41420-019-0207-1
13. Zaenker P, Ziman MR. Serologic autoantibodies as diagnostic cancer biomarkers—a review. Cancer Epidemiol Biomarkers Prev. (2013) 22:2161–81. doi: 10.1158/1055-9965.epi-13-0621
14. Titulaer MJ, Lang B, Verschuuren JJ. Lambert–Eaton myasthenic syndrome: from clinical characteristics to therapeutic strategies. Lancet Neurol. (2011) 10:1098–107. doi: 10.1016/s1474-4422(11)70245-9
15. Graus F, Saiz A, Dalmau J. Antibodies and neuronal autoimmune disorders of the CNS. J Neurol. (2009) 257:509–17. doi: 10.1007/s00415-009-5431-9
16. Moritz CP, Tholance Y, Boutahar N, Borowczyk C, Berger AE, Paul S, et al. The antibody repertoire of autoimmune sensory neuronopathies targets pathways of the innate and adaptative immune system. An autoantigenomic approach. J Transl Autoimmun. (2025) 8:100277. doi: 10.1016/j.jtauto.2025.100277
17. Dalmau J, Graus F, Rosenblum MK, Posner JB. Anti-HU-associated paraneoplastic encephalomyelitis/sensory neuronopathy: a clinical study of 71 patients. Medicine. (1992) 71:59–72. doi: 10.1097/00005792-199203000-00001
18. Lancaster E, Dalmau J. Neuronal autoantigens—pathogenesis, associated disorders and antibody testing. Nat Rev Neurol. (2012) 8:380–90. doi: 10.1038/nrneurol.2012.99
19. Mandel-Brehm C, Dubey D, Kryzer TJ, O’Donovan BD, Tran B, Vazquez SE, et al. Kelch-like protein 11 antibodies in Seminoma-Associated paraneoplastic encephalitis. N Engl J Med. (2019) 381:47–54. doi: 10.1056/nejmoa1816721
20. Pranzatelli MR. The immunopharmacology of the opsoclonus-myoclonus syndrome. Clin Neuropharmacol. (1996) 19:1–47. doi: 10.1097/00002826-199619010-00001
21. Skeie GO, Romi F. Paraneoplastic myasthenia gravis: immunological and clinical aspects. Eur J Neurol. (2008) 15:1029–33. doi: 10.1111/j.1468-1331.2008.02242.x
22. Dalmau J, Gleichman AJ, Hughes EG, Rossi JE, Peng X, Lai M, et al. Anti-NMDA-receptor encephalitis: case series and analysis of the effects of antibodies. Lancet Neurol. (2008) 7:1091–8. doi: 10.1016/s1474-4422(08)70224-2
23. Yang H, Peng Q, Yin L, Li S, Shi J, Zhang Y, et al. Identification of multiple cancer-associated myositis-specific autoantibodies in idiopathic inflammatory myopathies: a large longitudinal cohort study. Arthritis Res Ther. (2017) 19:259. doi: 10.1186/s13075-017-1469-8
24. Schwartz RA. Acanthosis nigricans. J Am Acad Dermatol. (1994) 31:1–19. doi: 10.1016/s0190-9622(94)70128-8
25. Schwartz RA. Sign of leser-trélat. J Am Acad Dermatol. (1996) 35:88–95. doi: 10.1016/s0190-9622(96)90502-2
26. McGavran MH, Unger RH, Recant L, Polk HC, Kilo C, Levin ME. A glucagon-secreting alpha-cell carcinoma of the pancreas. N Engl J Med. (1966) 274:1408–13. doi: 10.1056/nejm196606232742503
27. Cohen PR. Sweet’s syndrome—a comprehensive review of an acute febrile neutrophilic dermatosis. Orphanet J Rare Dis. (2007) 2:34. doi: 10.1186/1750-1172-2-34
28. García-Porrúa C, González-Gay MA. Cutaneous vasculitis as a paraneoplastic syndrome in adults. Arthritis Rheumatol. (1998) 41:1133–5. doi: 10.1002/1529-0131(199806)41:6<1133::aid-art23>3.0.co;2-s
29. Anderson HJ, Huang S, Lee JB. Paraneoplastic pemphigus/paraneoplastic autoimmune multiorgan syndrome: Part I. clinical overview and pathophysiology. J Am Acad Dermatol. (2023) 91:1–10. doi: 10.1016/j.jaad.2023.08.020
30. Huang S, Anderson HJ, Lee JB. Paraneoplastic pemphigus/paraneoplastic autoimmune multiorgan syndrome: Part II. Diagnosis and management. J Am Acad Dermatol. (2023) 91:13–22. doi: 10.1016/j.jaad.2023.08.084
31. Nadal R, McMahan ZH, Antonarakis ES. Paraneoplastic palmar fasciitis and polyarthritis syndrome in a patient with advanced prostate cancer. Clin Genitourin Cancer. (2013) 11:e15–23. doi: 10.1016/j.clgc.2013.05.008
32. Kassan SS, Moutsopoulos HM. Clinical manifestations and early diagnosis of Sjögren syndrome. Arch Intern Med. (2004) 164:1275. doi: 10.1001/archinte.164.12.1275
33. Anton E. More on polymyalgia rheumatica (PMR) as a paraneoplastic rheumatic syndrome in the elderly (Bicytopenia and PMR preceding acute myeloid leukemia). J Clin Rheumatol. (2007) 13:114. doi: 10.1097/01.rhu.0000260650.43402.b6
34. Romanowska-Próchnicka K, Dziewit M, Lesiak A, Reich A, Olesińska M. Scleroderma and scleroderma-like syndromes. Front Immunol. (2024) 15:1351675. doi: 10.3389/fimmu.2024.1351675
35. Martínez-Lavín M. Hypertrophic osteoarthropathy. Best Pract Res Clin Rheumatol. (2020) 34:101507. doi: 10.1016/j.berh.2020.101507
36. Beck LH, Bonegio RG, Lambeau G, Beck DM, Powell DW, Cummins TD, et al. M-type phospholipase A2 receptor as target antigen in idiopathic membranous nephropathy. N Engl J Med. (2009) 361:11–21. doi: 10.1056/nejmoa0810457
37. Chong WH, Molinolo AA, Chen CC, Collins MT. Tumor-induced osteomalacia. Endocr Relat Cancer. (2011) 18:R53–77. doi: 10.1530/erc-11-0006
38. Deliège M, Bastens B, Matus G, Blétard N, Houbiers G, Courtois A, et al. Stauffer syndrome: a rare paraneoplastic complication of renal cell carcinoma to be kept in mind. Case Rep literature survey Acta Gastroenterol Belg. (2024) 87:40–3. doi: 10.51821/87.1.11402
39. Potter MJ, Adamus G, Szabo SM, Lee R, Mohaseb K, Behn D. Autoantibodies to transducin in a patient with melanoma-associated retinopathy. Am J Ophthalmol. (2002) 134:128–30. doi: 10.1016/s0002-9394(02)01431-9
40. Zhu H, Hasman RA, Barron VA, Luo G, Lou H. A nuclear function of HU proteins as neuron-specific alternative RNA processing regulators. Mol Biol Cell. (2006) 17:5105–14. doi: 10.1091/mbc.e06-02-0099
41. Lazarova DL, Spengler BA, Biedler JL, Ross RA. HuD, a neuronal-specific RNA-binding protein, is a putative regulator of N-myc pre-mRNA processing/stability in Malignant human neuroblasts. Oncogene. (1999) 18:2703–10. doi: 10.1038/sj.onc.1202621
42. Dalmau J, Furneaux HM, Gralla RJ, Kris MG, Posner JB. Detection of the anti-Hu antibody in the serum of patients with small cell lung cancer—a quantitative western blot analysis. Ann Neurol. (1990) 27:544–52. doi: 10.1002/ana.410270515
43. Verschuuren JJ, Perquin M, Velde GT, De Baets M, Vriesman PVB, Twijnstra A. Anti-Hu antibody titre and brain metastases before and after treatment for small cell lung cancer. J Neurol Neurosurg Psychiatry. (1999) 67:353–7. doi: 10.1136/jnnp.67.3.353
44. Vigneswara V, Lowenson JD, Powell CD, Thakur M, Bailey K, Clarke S, et al. Proteomic identification of novel substrates of a protein isoaspartyl methyltransferase repair enzyme. J Biol Chem. (2006) 281:32619–29. doi: 10.1074/jbc.m605421200
45. Shirasawa T, Endoh R, Zeng Y, Sakamoto K, Mori H. Protein l-isoaspartyl methyltransferase: developmentally regulated gene expression and protein localization in the central nervous system of aged rat. Neurosci Lett. (1995) 188:37–40. doi: 10.1016/0304-3940(95)11389-e
46. Pulido MA, DerHartunian MK, Qin Z, Chung EM, Kang DS, Woodham AW, et al. Isoaspartylation appears to trigger small cell lung cancer-associated autoimmunity against neuronal protein ELAVL4. J Neuroimmunol. (2016) 299:70–8. doi: 10.1016/j.jneuroim.2016.09.002
47. Mamula MJ, Gee RJ, Elliott JI, Sette A, Southwood S, Jones P, et al. Isoaspartyl post-translational modification triggers autoimmune responses to self-proteins. J Biol Chem. (1999) 274:22321–7. doi: 10.1074/jbc.274.32.22321
48. Adamus G, Bonnah R, Brown L, David L. Detection of autoantibodies against heat shock proteins and collapsin response mediator proteins in autoimmune retinopathy. BMC Ophthalmol. (2013) 13:48. doi: 10.1186/1471-2415-13-48
49. Milam AH, Saari JC, Jacobson SG, Lubinski WP, Feun LG, Alexander KR. Autoantibodies against retinal bipolar cells in cutaneous melanoma-associated retinopathy. Invest Ophthalmol Vis Sci. (1993) 34:91–100.
50. Bazhin AV, Savchenko MS, Shifrina ON, Demoura SA, Chikina SY, Jaques G, et al. Recoverin as a paraneoplastic antigen in lung cancer: the occurrence of anti-recoverin autoantibodies in sera and recoverin in tumors. Lung Cancer. (2004) 44:193–8. doi: 10.1016/j.lungcan.2003.10.006
51. Ohguro H, Yokoi Y, Ohguro I, Mamiya K, Ishikawa F, Yamazaki H, et al. Clinical and immunologic aspects of cancer-associated retinopathy. Am J Ophthalmol. (2004) 137:1117–9. doi: 10.1016/j.ajo.2004.01.010
52. Goldstein SM. Cancer-associated retinopathy. Arch Ophthalmol. (1999) 117:1641. doi: 10.1001/archopht.117.12.1641
53. Catterall WA. Voltage-gated calcium channels. Cold Spring Harb Perspect Biol. (2011) 3:a003947. doi: 10.1101/cshperspect.a003947
54. Kesner VG, Oh SJ, Dimachkie MM, Barohn RJ. Lambert-Eaton myasthenic syndrome. Neurol Clin. (2018) 36:379–94. doi: 10.1016/j.ncl.2018.01.008
55. O’Neill JH, Murray NMF, Newsom-Davis J. The Lambert-Eaton myasthenic syndrome. Brain. (1988) 111:577–96. doi: 10.1093/brain/111.3.577
56. Lennon VA, Kryzer TJ, Griesmann GE, O’Suilleabhain PE, Windebank AJ, Woppmann A, et al. Calcium-channel antibodies in the Lambert-Eaton syndrome and other paraneoplastic syndromes. N Engl J Med. (1995) 332:1467–75. doi: 10.1056/nejm199506013322203
57. Payne M, Bradbury P, Lang B, Vincent A, Han C, Newsom-Davis J, et al. Prospective study into the incidence of Lambert Eaton myasthenic syndrome in small cell lung cancer. J Thorac Oncol. (2010) 5:34–8. doi: 10.1097/jto.0b013e3181c3f4f1
58. Maddison P, Lang B. Paraneoplastic neurological autoimmunity and survival in small-cell lung cancer. J Neuroimmunol. (2008) 201-202:159–62. doi: 10.1016/j.jneuroim.2008.05.024
59. Hansen KB, Yi F, Perszyk RE, Menniti FS, Traynelis SF. NMDA receptors in the central nervous system. Methods Mol Biol. (2017) 1677:1–80. doi: 10.1007/978-1-4939-7321-7_1
60. Acién P, Acién M, Ruiz-Maciá E, Martín-Estefanía C. Ovarian teratoma-associated anti-NMDAR encephalitis: a systematic review of reported cases. Orphanet J Rare Dis. (2014) 9:157. doi: 10.1186/s13023-014-0157-x
61. Al-Diwani A, Theorell J, Damato V, Bull J, McGlashan N, Green E, et al. Cervical lymph nodes and ovarian teratomas as germinal centres in NMDA receptor-antibody encephalitis. Brain. (2022) 145:2742–54. doi: 10.1093/brain/awac088
62. Mangler M, De Perez IT, Teegen B, Stöcker W, Prüss H, Meisel A, et al. Seroprevalence of anti-N-methyl-d-aspartate receptor antibodies in women with ovarian teratoma. J Neurol. (2013) 260:2831–5. doi: 10.1007/s00415-013-7074-0
63. Gong S, Zhou M, Shi G, Guo J, Chen N, Yang R, et al. Absence of NMDA receptor antibodies in patients with ovarian teratoma without encephalitis. Neurol Neuroimmunol Neuroinflamm. (2017) 4:e340. doi: 10.1212/nxi.0000000000000344
64. Trillsch F, Eichhorn P, Oliveira-Ferrer L, Kuempfel T, Burges A, Mahner S, et al. No need for NMDA receptor antibody screening in neurologically asymptomatic patients with ovarian teratomas. J Neurol. (2017) 265:431–2. doi: 10.1007/s00415-017-8717-3
65. Kotani H, Matsuda KM, Yamaguchi K, Ono C, Kogo E, Ogawa K, et al. Diversity and epitope spreading of anti-RNA polymerase III antibodies in systemic sclerosis: a potential biomarker for skin and lung involvement. Arthritis Rheumatol. (2024) 77:67–79. doi: 10.1002/art.42975
66. Joseph CG, Darrah E, Shah AA, Skora AD, Casciola-Rosen LA, Wigley FM, et al. Association of the autoimmune disease scleroderma with an immunologic response to cancer. Science. (2013) 343:152–7. doi: 10.1126/science.1246886
67. Raspotnig M, Kråkenes T, Herdlevær I, Haugen M, Vedeler C. Expression of cerebellar degeneration-related proteins CDR2 and CDR2L in human and rat brain tissue. J Neuroimmunol. (2021) 362:577766. doi: 10.1016/j.jneuroim.2021.577766
68. Small M, Treilleux I, Couillault C, Pissaloux D, Picard G, Paindavoine S, et al. Genetic alterations and tumor immune attack in Yo paraneoplastic cerebellar degeneration. Acta Neuropathol. (2018) 135:569–79. doi: 10.1007/s00401-017-1802-y
69. Peter E, Treilleux I, Wucher V, Jougla E, Vogrig A, Pissaloux D, et al. Immune and genetic signatures of breast carcinomas triggering Anti-YO-associated paraneoplastic cerebellar degeneration. Neurol Neuroimmunol Neuroinflamm. (2022) 9:e200015. doi: 10.1212/nxi.0000000000200015
70. Venuta F, Rendina EA, De Giacomo T, Della Rocca G, Antonini G, Ciccone AM, et al. Thymectomy for myasthenia gravis: a 27-year experience. Eur J Cardiothorac Surg. (1999) 15:621–5. doi: 10.1016/s1010-7940(99)00052-4
71. Zhang Z, Cui Y, Jia R, Xue L, Liang H. Myasthenia gravis in patients with thymoma affects survival rate following extended thymectomy. Oncol Lett. (2016) 11:4177–82. doi: 10.3892/ol.2016.4528
72. Zhao K, Liu Y, Jing M, Cai W, Jin J, Zhu Z, et al. Long-term prognosis in patients with thymoma combined with myasthenia gravis: a propensity score-matching analysis. Front Med. (2024) 11:1407830. doi: 10.3389/fmed.2024.1407830
73. Romi F, Skeie GO, Aarli JA, Gilhus NE. The severity of myasthenia gravis correlates with the serum concentration of titin and ryanodine receptor antibodies. Arch Neurol. (2000) 57:1596. doi: 10.1001/archneur.57.11.1596
74. Cetin H, Beeson D, Vincent A, Webster R. The structure, function, and physiology of the fetal and adult acetylcholine receptor in muscle. Front Mol Neurosci. (2020) 13:581097. doi: 10.3389/fnmol.2020.581097
75. Radovich M, Pickering CR, Felau I, Ha G, Zhang H, Jo H, et al. The integrated genomic landscape of thymic epithelial tumors. Cancer Cell. (2018) 33:244–258.e10. doi: 10.1016/j.ccell.2018.01.003
76. Yasumizu Y, Ohkura N, Murata H, Kinoshita M, Funaki S, Nojima S, et al. Myasthenia gravis-specific aberrant neuromuscular gene expression by medullary thymic epithelial cells in thymoma. Nat Commun. (2022) 13:5090. doi: 10.1038/s41467-022-31951-8
77. Schultz A, Hoffacker V, Wilisch A, Nix W, Gold R, Schalke B, et al. Neurofilament is an autoantigenic determinant in myasthenia gravis. Ann Neurol. (1999) 46:167–75. doi: 10.1002/1531-8249(199908)46:2<167::aid-ana5>3.0.co;2-7
78. Hektoen HH, Tsuruda KM, Fjellbirkeland L, Nilssen Y, Brustugun OT, Andreassen BK. Real-world evidence for pembrolizumab in non-small cell lung cancer: a nationwide cohort study. Br J Cancer. (2024) 132:93–102. doi: 10.1038/s41416-024-02895-1
79. Kato K, Cho BC, Takahashi M, Okada M, Lin C, Chin K, et al. Nivolumab versus chemotherapy in patients with advanced oesophageal squamous cell carcinoma refractory or intolerant to previous chemotherapy (ATTRACTION-3): a multicentre, randomised, open-label, phase 3 trial. Lancet Oncol. (2019) 20:1506–17. doi: 10.1016/s1470-2045(19)30626-6
80. Janjigian YY, Shitara K, Moehler M, Garrido M, Salman P, Shen L, et al. First-line nivolumab plus chemotherapy versus chemotherapy alone for advanced gastric, gastro-oesophageal junction, and oesophageal adenocarcinoma (CheckMate 649): a randomised, open-label, phase 3 trial. Lancet. (2021) 398:27–40. doi: 10.1016/s0140-6736(21)00797-2
81. Chen R, Zhu Y, Shen Y, Xu Q, Tang H, Cui N, et al. The role of PD-1 signaling in health and immune-related diseases. Front Immunol. (2023) 14:1163633. doi: 10.3389/fimmu.2023.1163633
82. Muenst S, Schaerli AR, Gao F, Däster S, Trella E, Droeser RA, et al. Expression of programmed death ligand 1 (PD-L1) is associated with poor prognosis in human breast cancer. Breast Cancer Res Treat. (2014) 146:15–24. doi: 10.1007/s10549-014-2988-5
83. Vackova J, Polakova I, Johari SD, Smahel M. CD80 expression on tumor cells alters tumor microenvironment and efficacy of cancer immunotherapy by CTLA-4 blockade. Cancers. (2021) 13:1935. doi: 10.3390/cancers13081935
84. Puzanov I, Diab A, Abdallah K, Bingham CO, Brogdon C, Dadu R, et al. Managing toxicities associated with immune checkpoint inhibitors: consensus recommendations from the Society for Immunotherapy of Cancer (SITC) Toxicity Management Working Group. J Immunother Cancer. (2017) 5:95. doi: 10.1186/s40425-017-0300-z
85. Brown LJ, Weppler A, Bhave P, Allayous C, Patrinely JR, Ott P, et al. Combination anti-PD1 and ipilimumab therapy in patients with advanced melanoma and pre-existing autoimmune disorders. J Immunother Cancer. (2021) 9:e002121. doi: 10.1136/jitc-2020-002121
86. Buckley MW, Warner AB, Brahmer J, Cappelli LC, Sharfman WH, Fuchs E, et al. Immune-related encephalitis after immune checkpoint inhibitor therapy. Oncologist. (2024) 30:e1–9. doi: 10.1093/oncolo/oyae186
87. Hébert J, Riche B, Vogrig A, Muñiz-Castrillo S, Joubert B, Picard G, et al. Epidemiology of paraneoplastic neurologic syndromes and autoimmune encephalitides in France. Neurol Neuroimmunol Neuroinflamm. (2020) 7:e883. doi: 10.1212/nxi.0000000000000883
88. Hamada N, Maeda A, Takase-Minegishi K, Kirino Y, Sugiyama Y, Namkoong H, et al. Incidence and distinct features of immune checkpoint inhibitor-related myositis from idiopathic inflammatory myositis: a single-center experience with systematic literature review and meta-analysis. Front Immunol. (2021) 12:803410. doi: 10.3389/fimmu.2021.803410
89. Vinciguerra C, Bevilacqua L, Lupica A, Ginanneschi F, Piscosquito G, Rini N, et al. Diagnosis and management of seronegative myasthenia gravis: lights and shadows. Brain Sci. (2023) 13:1286. doi: 10.3390/brainsci13091286
90. Macklin M, Yadav S, Jan R, Reid P. Checkpoint inhibitor-associated scleroderma and scleroderma mimics. Pharmaceuticals. (2023) 16:259. doi: 10.3390/ph16020259
91. Jayathilaka B, Mian F, Franchini F, Au-Yeung G, IJzerman M. Cancer and treatment specific incidence rates of immune-related adverse events induced by immune checkpoint inhibitors: a systematic review. Br J Cancer. (2024) 132:51–57. doi: 10.1038/s41416-024-02887-1
92. Chennamadhavuni A, Abushahin L, Jin N, Presley CJ, Manne A. Risk factors and biomarkers for immune-related adverse events: a practical guide to identifying high-risk patients and rechallenging immune checkpoint inhibitors. Front Immunol. (2022) 13:779691. doi: 10.3389/fimmu.2022.779691
93. Daban A, Gonnin C, Phan L, Saldmann A, Granier C, Lillo-Lelouet A, et al. Preexisting autoantibodies as predictor of immune related adverse events (irAEs) for advanced solid tumors treated with immune checkpoint inhibitors (ICIs). Oncoimmunology. (2023) 12:2204754. doi: 10.1080/2162402x.2023.2204754
94. Borgers JSW, Van Wesemael TJ, Gelderman KA, Rispens T, Verdegaal EME, Moes DJAR, et al. Autoantibody-positivity before and seroconversion during treatment with anti-PD-1 is associated with immune-related adverse events in patients with melanoma. J Immunother Cancer. (2024) 12:e009215. doi: 10.1136/jitc-2024-009215
95. Teillaud J, Houel A, Panouillot M, Riffard C, Dieu-Nosjean M. Tertiary lymphoid structures in anticancer immunity. Nat Rev Cancer. (2024) 24:629–46. doi: 10.1038/s41568-024-00728-0
96. Xia J, Xie Z, Niu G, Lu Z, Wang Z, Xing Y, et al. Single-cell landscape and clinical outcomes of infiltrating B cells in colorectal cancer. Immunology. (2022) 168:135–51. doi: 10.1111/imm.13568
97. Mazor RD, Nathan N, Gilboa A, Stoler-Barak L, Moss L, Solomonov I, et al. Tumor-reactive antibodies evolve from non-binding and autoreactive precursors. Cell. (2022) 185:1208–1222.e21. doi: 10.1016/j.cell.2022.02.012
98. Kinker GS, Vitiello GAF, Diniz AB, Cabral-Piccin MP, Pereira PHB, Carvalho MLR, et al. Mature tertiary lymphoid structures are key niches of tumour-specific immune responses in pancreatic ductal adenocarcinomas. Gut. (2023) 72:1927–41. doi: 10.1136/gutjnl-2022-328697
99. Germain C, Gnjatic S, Tamzalit F, Knockaert S, Remark R, Goc J, et al. Presence of B cells in tertiary lymphoid structures is associated with a protective immunity in patients with lung cancer. Am J Respir Crit Care Med. (2014) 189:832–44. doi: 10.1164/rccm.201309-1611oc
100. Dieu-Nosjean M, Antoine M, Danel C, Heudes D, Wislez M, Poulot V, et al. Long-term survival for patients with non-small-cell lung cancer with intratumoral lymphoid structures. J Clin Oncol. (2008) 26:4410–7. doi: 10.1200/jco.2007.15.0284
Keywords: autoimmune-mediated paraneoplastic syndromes, autoantibodies, self-tolerance, tumor microenvironment, immune related adverse events (irAEs)
Citation: Pérez-Bucio C, Behere A and Landegren N (2025) Mechanisms of autoimmune-mediated paraneoplastic syndromes: immune tolerance and disease pathogenesis. Front. Immunol. 16:1608934. doi: 10.3389/fimmu.2025.1608934
Received: 09 April 2025; Accepted: 21 April 2025;
Published: 09 May 2025.
Edited and Reviewed by:
Cuiling Zheng, Chinese Academy of Medical Sciences and Peking Union Medical College, ChinaCopyright © 2025 Pérez-Bucio, Behere and Landegren. This is an open-access article distributed under the terms of the Creative Commons Attribution License (CC BY). The use, distribution or reproduction in other forums is permitted, provided the original author(s) and the copyright owner(s) are credited and that the original publication in this journal is cited, in accordance with accepted academic practice. No use, distribution or reproduction is permitted which does not comply with these terms.
*Correspondence: Nils Landegren, bmlscy5sYW5kZWdyZW5AaW1iaW0udXUuc2U=