- 1Programa de Pós-Graduação em Farmácia, CCS, Universidade Federal de Santa Catarina, Florianópolis, Brazil
- 2Department of Global Health and Infection, Brighton and Sussex Medical School, University of Sussex, Brighton, United Kingdom
- 3Programa de Pós-Graduação em Química, CFM, Departamento de Química, Universidade Federal de Santa Catarina, Florianópolis, Brazil
Drug-resistant tuberculosis threatens to undermine global control programs by limiting treatment options. New antimicrobial drugs are required, derived from new chemical classes. Natural products offer extensive chemical diversity and inspiration for synthetic chemistry. Here, we isolate, synthesize and test a library of 52 natural and synthetic compounds for activity against Mycobacterium tuberculosis. We identify seven compounds as antimycobacterial, including the natural products isobavachalcone and isoneorautenol, and a synthetic chromene. The plant-derived secondary metabolite damnacanthal was the most active compound with the lowest minimum inhibitory concentration of 13.07 μg/mL and a favorable selectivity index value. Three synthetic polyacetylene compounds demonstrated antimycobacterial activity, with the lowest MIC of 17.88 μg/mL. These results suggest new avenues for drug discovery, expanding antimicrobial compound chemistries to novel anthraquinone and polyacetylene scaffolds in the search for new drugs to treat drug-resistant bacterial diseases.
Introduction
Tuberculosis (TB) is an infectious disease that is among the top 10 causes of death worldwide, and the leading bacterial cause of death. In 2019, an estimated 10 million people developed TB and 1.4 million people died as result of the disease (World Health Organization, 2020). TB treatment requires the use of multiple drugs for at least 6 months. This lengthy therapy together with adverse drug reactions contribute to patient non-adherence, resulting in treatment failure and the development of drug-resistant Mycobacterium tuberculosis. The emergence of multidrug-resistant (MDR) TB and extensively drug resistant (XDR) TB is undermining global control efforts. In 2019, 3.3% of new TB cases and 17.7% of previously treated cases were rifampicin-resistant (RR)/MDR-TB. There were an estimated 465,000 incident cases of RR-TB in the same year, of which 78% were MDR-TB (World Health Organization, 2020). Therefore, there is an urgent need, recognized by the World Health Organization (World Health Organization, 2014), for new drugs to treat drug-resistant TB, and to shorten therapy for drug-sensitive TB. However, despite sustained international efforts, only pretomanid, delamanid and bedaquiline have been marketed as new drugs for TB treatment in the last 40 years (The Working Group for New TB Drugs, 2020). Intensified research and innovation are needed to meet the End TB Strategy targets set for 2030, a key priority of which is to discover new drugs based on new chemical entities (World Health Organization, 2014).
Over the past 60,000 years, plant-derived medicines have been used as decoctions, infusions and tinctures to improve human health (Solecki and Shanidar, 1975). Numerous studies have attempted to correlate ethnological knowledge with the scientific evidence base (De Smet, 1997). Natural products are an essential source of biologically active components (Thomford et al., 2018; Wright, 2019), and naturally occurring secondary metabolites have inspired the development of therapeutic drugs for infectious, cardiovascular, and degenerative diseases (Thomford et al., 2018; Lautié et al., 2020). Natural products are composed of numerous structural diversities, often containing complex hydrocarbon skeletons that have been explored to produce libraries of biologically relevant derivatives (Salomon and Schmidt, 2012; Pascolutti and Quinn, 2014). Dorstenia plant species are used in sub-Saharan African and South American countries as herbal medicines to treat cough, pneumonia and other infectious diseases such as malaria, syphilis, and hepatitis (Togola et al., 2008; Teklehaymanot, 2009; Bieski et al., 2012; Adem et al., 2018). Prenylated flavonoids obtained from Dorstenia species showed antibacterial activity against a broad-spectrum of bacteria, including M. tuberculosis (Mbaveng et al., 2012). Secondary metabolites from Erythrina senegalensis, the Senegal coral tree, have been demonstrated to exhibit strong inhibition of methicillin resistant Staphylococcus aureus, Enterococcus faecalis, and Bacillus subtilis (Koné et al., 2004). Damnacanthal, an anthraquinone obtained from Pentas schimperi, displayed moderate activity against the Trypanosoma cruzi amastigote (Sandjo et al., 2016b). As numerous biologically active molecules have been inspired by the organic constituents of plants, our group prepared a series of pyridine and chromene derivatives (Pollo et al., 2017; Martin et al., 2018). These compounds were evaluated for their antiparasitic activities against Leishmania amazonensis and T. cruzi amastigotes. Three pyridines and three chromenes inhibited T. cruzi with IC50 values less than 7 μM. Similarly, a coumarin scaffold was used to generate anti-TB agents, while polyacetylenes from plants and pyridine derivatives have been shown to express antimycobacterial activities (Rogoza et al., 2010; Somagond et al., 2019; Kumar et al., 2020).
Here, we report the extraction, synthesis, and anti-M. tuberculosis activity of 52 compounds: six plant secondary metabolites from Dorstenia kameruniana, Dorstenia mannii, P. schimperi, and E. senegalensis, and 46 synthetic compounds including 20 dihydropyridines, 12 pyranocoumarins, seven chromenes, one oxazinone, one conjugated ester and five polyacetylenes. This study identifies anthraquinone and polyacetylene compounds as the basis for novel drug discovery toward new therapeutic options for drug-resistant TB.
Materials and Methods
Origin of the Natural Products
Isobavachalcone (C1) was isolated from D. kameruniana, while 4-hydroxylonchocarpine (C21) and 6,8-diprenyleriodictyol (C24) were obtained from D. mannii (Abegaz et al., 1998; Ngadjui et al., 1998). Damnacanthal (C22) and its reduced derivative (C25) were isolated from P. schimperi (Kuete et al., 2015a). Isoneorautenol (C23) was extracted from E. senegalensis (Kuete et al., 2014). The structures of these natural secondary metabolites are displayed in Figures 1, 2.
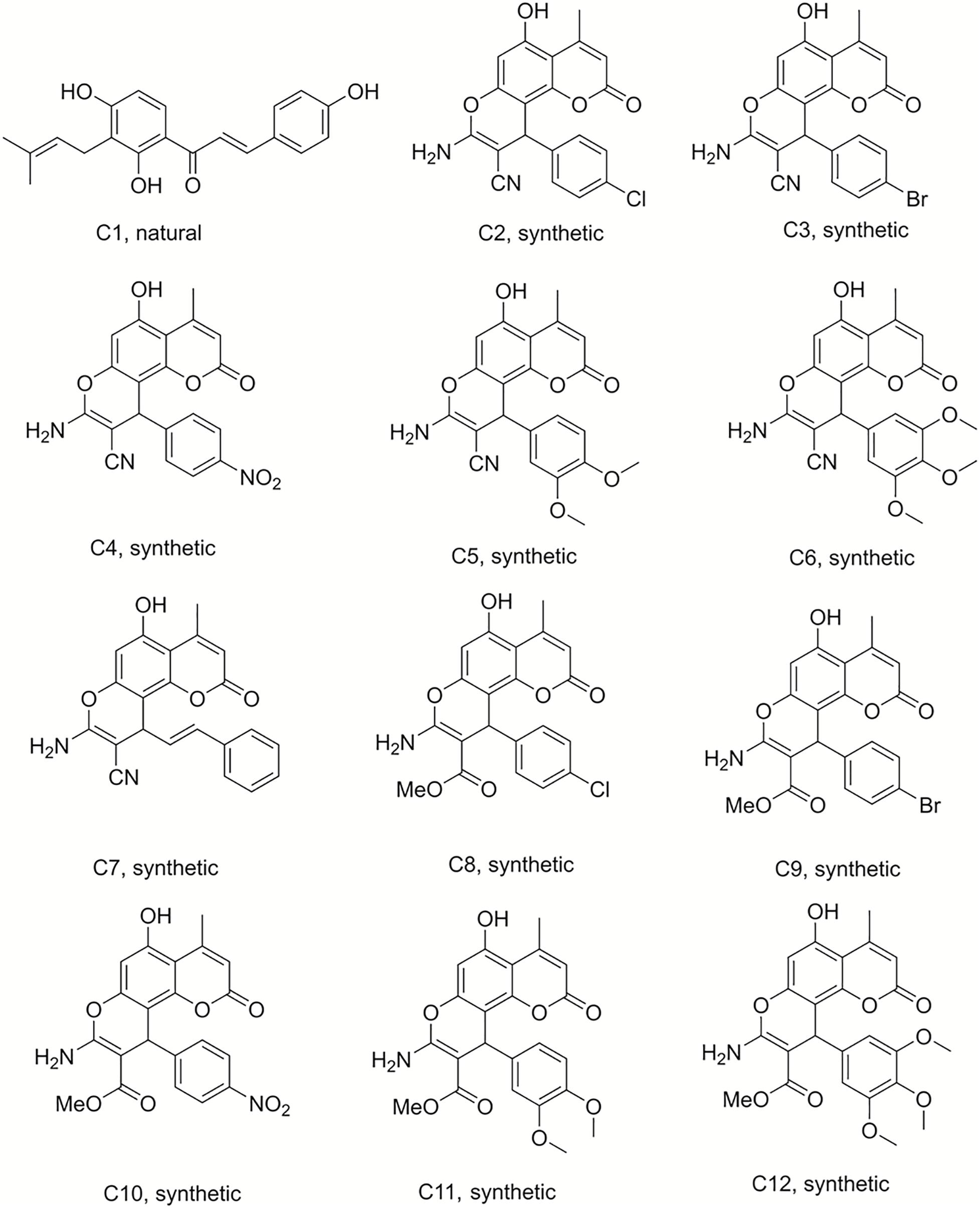
Figure 1. Structures of the compounds tested for anti-M. tuberculosis activity: Chalcone C1, and the pyranocoumarins C2–C12 (Abegaz et al., 1998; Martin et al., 2018).
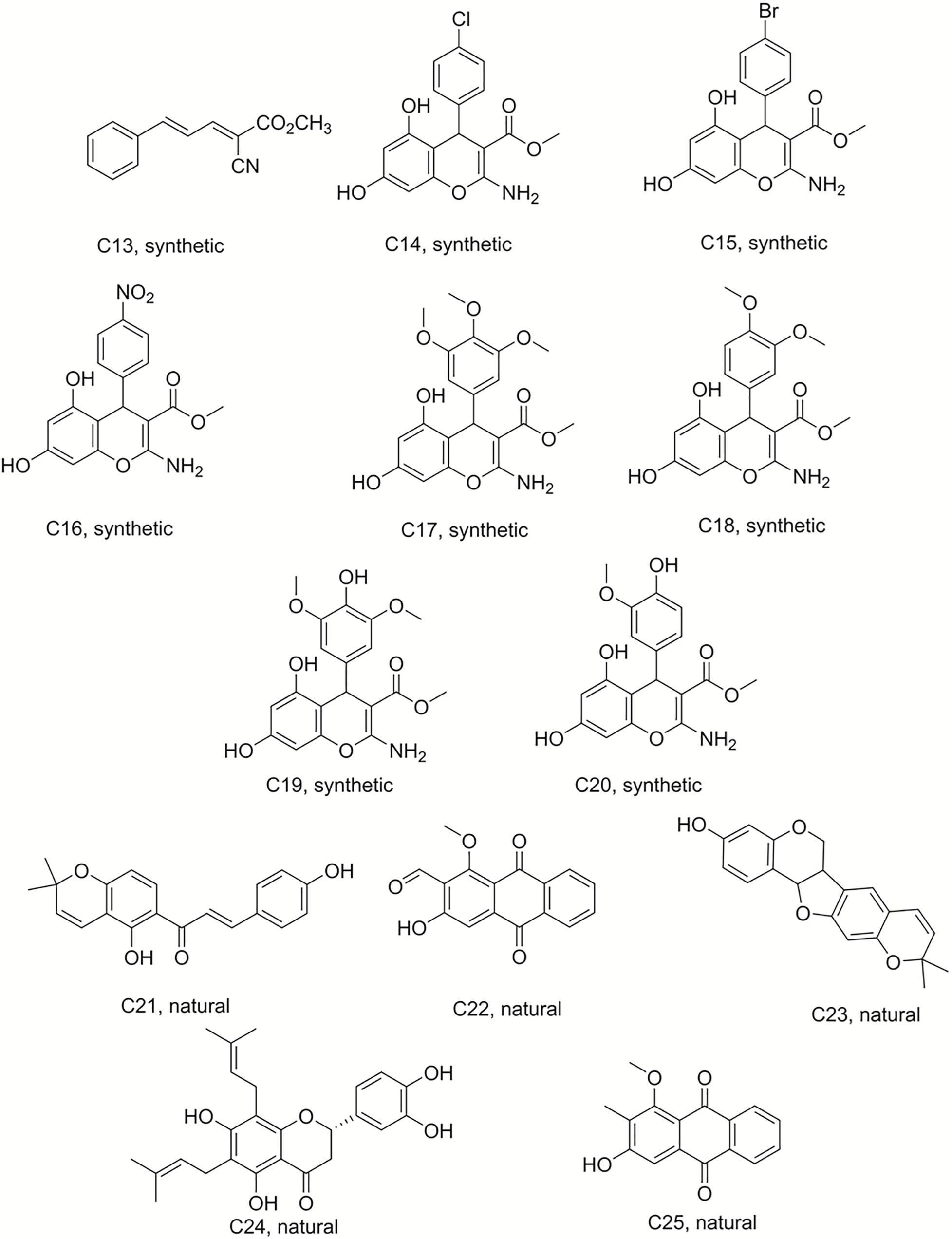
Figure 2. Structures of the compounds tested for anti-M. tuberculosis activity: Ester C13, chromenes C14–C20, and natural products C21–C25 (Ngadjui et al., 1998; Kuete et al., 2014, 2015a; Martin et al., 2018).
Preparation of the Pyranocoumarin Derivatives
Compound 50 (C50) was synthesized by treating phloroglucinol at 60°C for 6 h with one equivalent of ethyl acetoacetate and a catalytic amount of polyphosphoric acid. This coumarin was then used as the starting material to prepare a series of pyranocoumarins. C50, arylaldehydes, malononitrile, and K2CO3 were submitted to reflux conditions to generate compounds C2–C7. Compounds C8–C12 were obtained using the same one-pot conditions except that malononitrile was replaced by methyl α-cyanoacetate. C13 was a by-product formed from the Knoevenagel condensation reaction of cinnamaldehyde and α-cyanoacetate in the same reaction conditions. The structures are detailed in Figures 1, 2, 4 (Martin et al., 2018).
Preparation of Chromenes
Chromene derivatives C14–C20 were obtained by a direct reaction of phloroglucinol, arylaldehydes and methyl α-cyanoacetate in alkaline reflux conditions. We have previously described the synthesis and the identification of C2–C20 (Martin et al., 2018), shown in Figure 2.
Preparation of Dihydropyridine Derivatives and Analogs
Bismuth chloride was used to promote the reaction, which was carried out in tetrahydrofuran under reflux conditions and was stirred for 6 h to synthesize compounds C29–C33 (Sandjo et al., 2016a). C34–C41 were obtained from the same reaction conditions replacing ethyl benzoylacetate with ethyl acetylacetate in reflux and free catalyst conditions to prepare these dihydropyridine analogs. C42–C49 were also synthesized in reflux conditions without any catalyst, replacing ammonium acetate with aniline. The preparation of these dihydropyridine analogs has been described (Pollo et al., 2017), and their structures are displayed in Figures 3, 4.
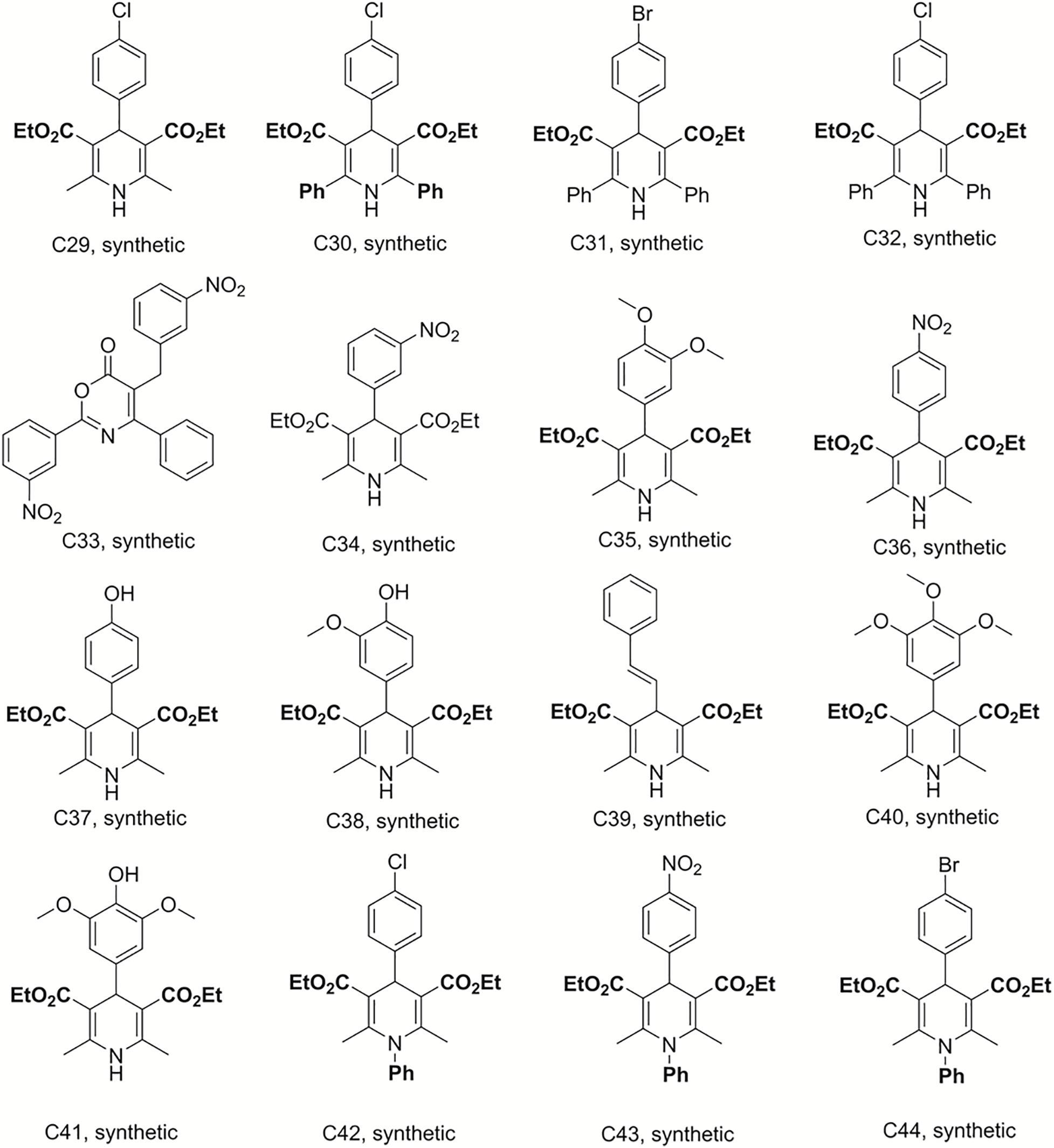
Figure 3. Structures of the compounds tested for anti-M. tuberculosis activity: Dihydropyridines C29–C44 (Sandjo et al., 2016a; Pollo et al., 2017).
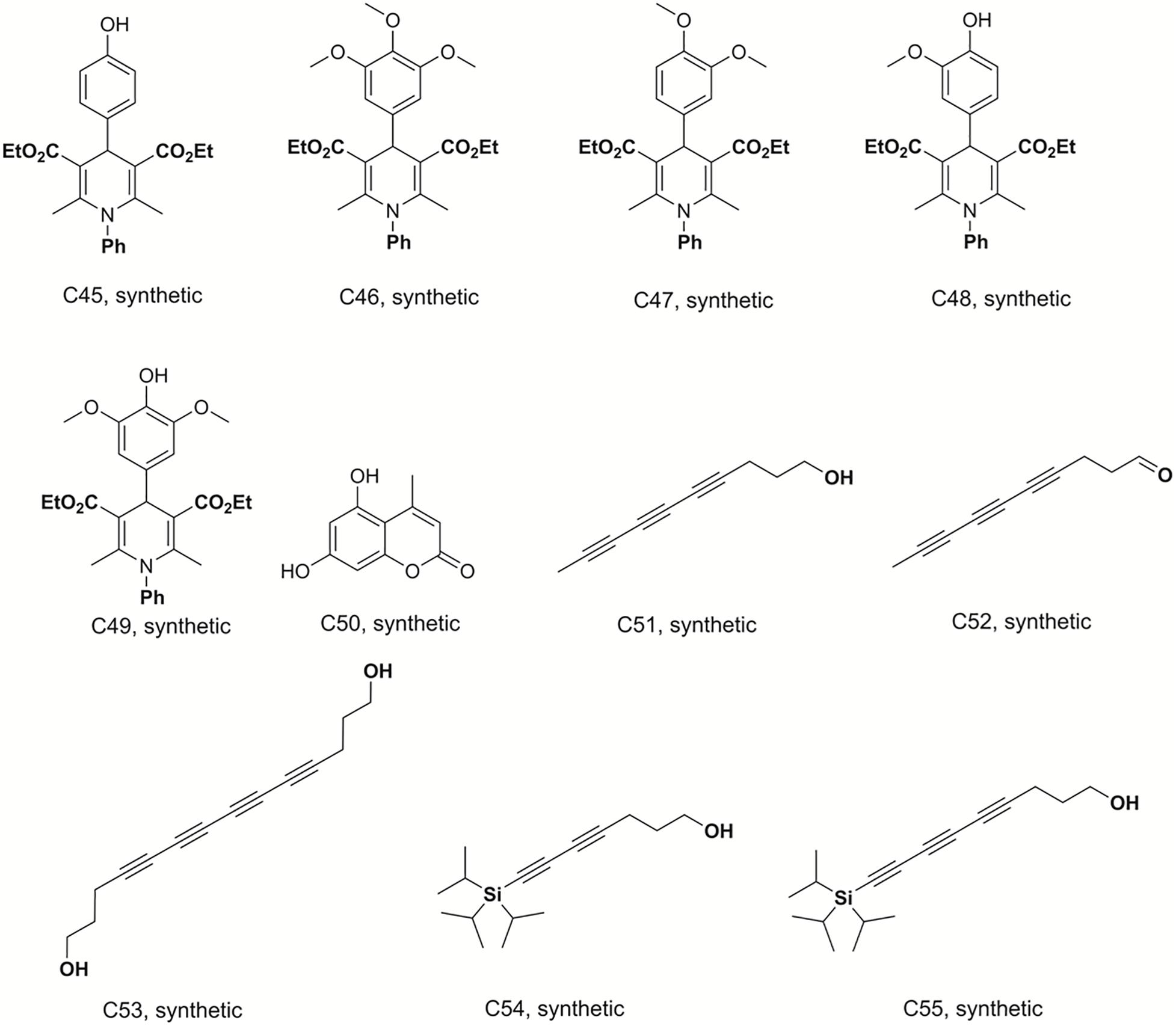
Figure 4. Structures of the compounds tested for anti-M. tuberculosis activity: Dihydropyridines C45–C49, coumarin C50, and polyacetylenes C51–C55 (Sandjo et al., 2016a; Pollo et al., 2017; Machado et al., 2018; Martin et al., 2018).
Preparation of the Polyacetylene Derivatives
Deca-4,6,8-triyn-1-ol (C51), deca-4,6,8-triynal (C52), 7-(triisopropylsilyl)hepta-4,6-diyn-1-ol (C54) and 9-(triisopropylsilyl)nona-4, 6-,8-triyn-1-ol (C55) were previously synthesized, identified and reported by Machado and co-workers (Machado et al., 2018). Tetradeca-4,6,8,10-tetrayne-1,14-diol (C53) was prepared by homodimerization reaction in a 25 mL, two neck, round-bottom flask equipped with rubber septum and a magnetic stir bar, filled with a solution of hepta-4,6-diyn-1-ol (0.1 g; 0.925 mmol; 1 equiv.) in CH3CN (10 mL). To this solution was added Cu(OAc)2 (0.353 g; 1.94 mmol; 2.1 equiv) and K2CO3 (0.153 g; 1.11 mmol; 1.2 equiv. The resulting mixture was stirred vigorously at room temperature overnight (∼18 h). The organic extract was washed with brine (20 mL), dried over MgSO4, filtered, and concentrated under reduced pressure. Purification by column chromatography on silica gel (elution with 40% EtOAc-hexanes) afforded 0.063 g (32 %) of tetradeca-4,6,8,10-tetrayne-1,14-diol as a pale yellow oil. mp 116.5 - 117°C; R.f: 0.75 (40% hexane-ethyl acetate; 1H NMR (300 MHz, CD3OD) δ in ppm: 3.61(t, 4H), 2.44 (t, J = 7.15 Hz, 4H), 1.74 (m, 4H). 13C-NMR (75 MHz, CD3OD) δ in ppm: 81.4; 66.1; 62.1; 61.2; 60.8; 31.9; 16.5. The structures of these compounds are shown in Figure 4.
Bacteria and Culture Conditions
Mycobacterium tuberculosis H37Rv reference strain was cultured in Middlebrook 7H9 broth (Sigma-Aldrich) supplemented with albumin dextrose catalase (ADC, 10% v/v) and Tween 80 (0.05% v/v) at 37°C. Optical density was measured using a spectrophotometer (Promega) at absorbance 600 nm. Colony forming units (CFU) were determined by serially diluting cultures onto Middlebrook 7H10 agar (Sigma-Aldrich) supplemented with 0.5% glycerol and oleic acid albumin dextrose catalase (OADC, 10% v/v) and incubated at 37°C for 4 weeks. All M. tuberculosis work was conducted in containment level three laboratories, following institutional biosafety and biosecurity standards for working with hazard group three pathogens.
Antimycobacterial Activity
All compounds were prepared as 10 mg/mL stock solutions in sterile dimethyl sulfoxide (DMSO), except for rifampicin which was prepared with 90% w/v methanol. Single use aliquots of compounds were prepared and stored at −20°C. Log phase M. tuberculosis cultures were adjusted from 2 × 105 to 5 × 105 CFU/mL, added to 96 well microtiter plates containing test compounds at a final concentration of 100 μg/mL (1% DMSO final concentration), and incubated for 7 days at 37°C. To determine cell viability, CellTiter-Blue (Promega) was added to the plates at a final concentration of 10% v/v and incubated overnight (Franzblau et al., 1998). Fluorescence was measured at excitation 580–640 nm and emission 520 nm using a Glomax Discover plate reader (Promega). Hits were classified as any compound that inhibited growth by ≥40% compared to drug-free M. tuberculosis controls. This cut-off was selected to capture the range of antimicrobial activity of related chemical compounds, rather than highlight individual compounds with superior activity. Minimum inhibitory concentrations (MIC) of hit compounds were determined using a resazurin microtiter plate assay (REMA) with CellTiter-Blue (Promega) as described above, with two-fold serial dilutions from 100 μg/mL to 1.56 μg/mL. The MIC experiments were repeated in triplicate. MICs were calculated by non-linear regression, fitting these data to a modified Gompertz equation for MIC determination, using GraphPad Prism 8. Validation of this assay for established TB drugs (isoniazid and linezolid) is detailed in Supplementary Figure 1.
Cytotoxicity Assay
The human monocytic THP-1 cell line was maintained at 37°C, 5% CO2 in supplemented RPMI 1640 medium (Gibco, Life Technologies) containing 2 mM L-glutamine (Gibco, Life Technologies) and 10% heat inactivated FBS (Pan Biotech). The cells were passaged every 4 days. To measure compound cytotoxicity, 5 × 104 monocytes per well were added to 96 well plates and treated for 24 h with the compounds at concentrations ranging from 200 μg/mL to 1.56 μg/mL. Cell viability was determined by fluorescence quantification after 2 h incubation with CellTiter-Blue (Promega), according to the manufacturer’s instructions. Fluorescence was measured at excitation 580–640 nm and emission 520 nm using a Glomax Discover plate reader (Promega). Fluorescence values were adjusted for media fluorescence and the inhibitory concentration 50% (IC50) was calculated using Graphpad Prism 8. The selectivity index (SI) of each compound was calculated by dividing the IC50 by the corresponding M. tuberculosis MIC value.
Results and Discussion
The discovery of biologically active new chemical entities is crucial to developing novel chemotherapeutic agents against drug resistant bacterial infections, including TB. Chemistry uses synthetic approaches and analytic techniques to identify and isolate natural products and to produce small molecules bearing diverse hydrocarbon skeletons for preclinical studies (Lombardino and Lowe, 2004; Campbell et al., 2018). To contribute to the search for new anti-tubercular agents, a library of 52 natural and synthetic compounds (Figures 1–4) were tested against log phase M. tuberculosis. The library contained compounds with diverse chemistries including 11 pyranocoumarins (C2–C12), seven chromenes (C14–C20), one conjugated arylester (C13), two chalcones (C1 and C21), two anthraquinones (C22 and C25), one pterocarpan (C23), one flavanone (C24), 20 dihydropyridines (C29–C32 and C34–C49), one oxazinone (C33), one coumarin (C50), and five polyacetylenes (C51–C55).
Antimycobacterial Activity
The initial screen of the compound library at 100 μg/mL, using the colorimetric CellTiter-Blue assay to measure mycobacterial viability, identified 17 of the 52 compounds that inhibited M. tuberculosis survival by at least 40% in comparison to drug-free bacilli (Figure 5). Five compounds inhibited M. tuberculosis at a level similar to the first line anti-TB drug rifampicin. To verify the results of the initial screen and to establish compound activity, minimum inhibitory concentrations (MICs) were determined for all 17 hits using the microbroth dilution method. Compound C22 (the natural product damnacanthal) displayed the greatest activity, with a MIC of 13.07 μg/mL, followed by the polyacetylene C53 with a MIC of 17.88 μg/mL (Figure 6 and Table 1). Compounds C1, C10, C23, C51, and C52 were demonstrated to have MICs against M. tuberculosis between 25 and 71 μg/mL (Figure 6 and Table 1). Compounds C13, C14, C15, C24, C36, C38, C40, C41, C45, and C49 resulted in MICs ≥100 μg/mL. Thus, we identified seven chemically diverse compounds that inhibited M. tuberculosis.
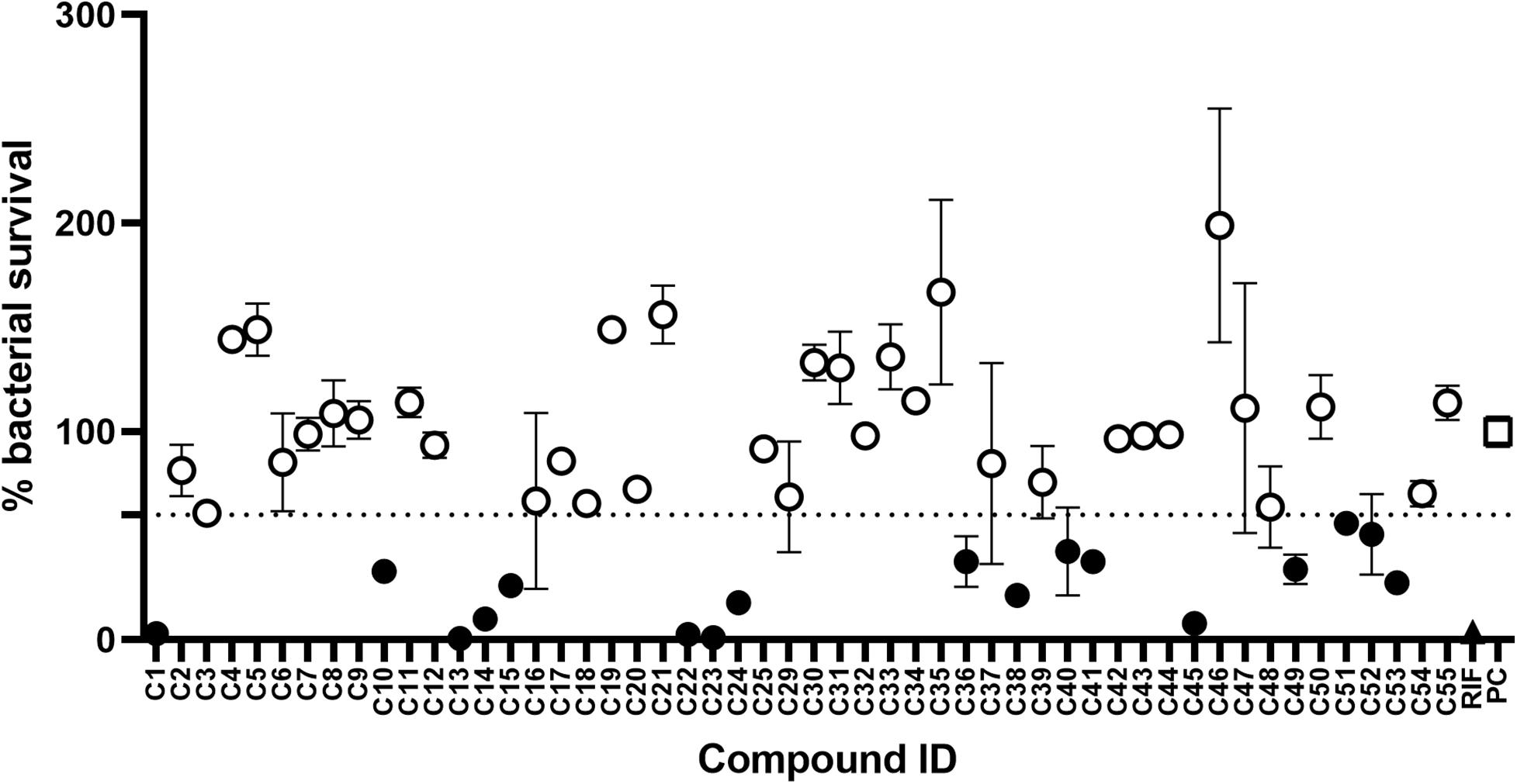
Figure 5. Compound library screen against Mycobacterium tuberculosis. Log phase M. tuberculosis was treated with compounds at 100 μg/mL for 7 days, bacterial survival was measured after overnight incubation with CellTiter-Blue. Data points are expressed as mean % survival compared to drug-free controls. Error bars represent the standard deviation. Hits were classified as any compound that inhibited growth by ≥40%. Filled circles identify hit compounds. Empty circles indicate compounds not classified as hits. The mean bacterial survival ≤60% shown as a dotted line. Filled triangle (RIF) marks rifampicin-treated bacilli. Empty square (PC) indicates drug-free positive controls.
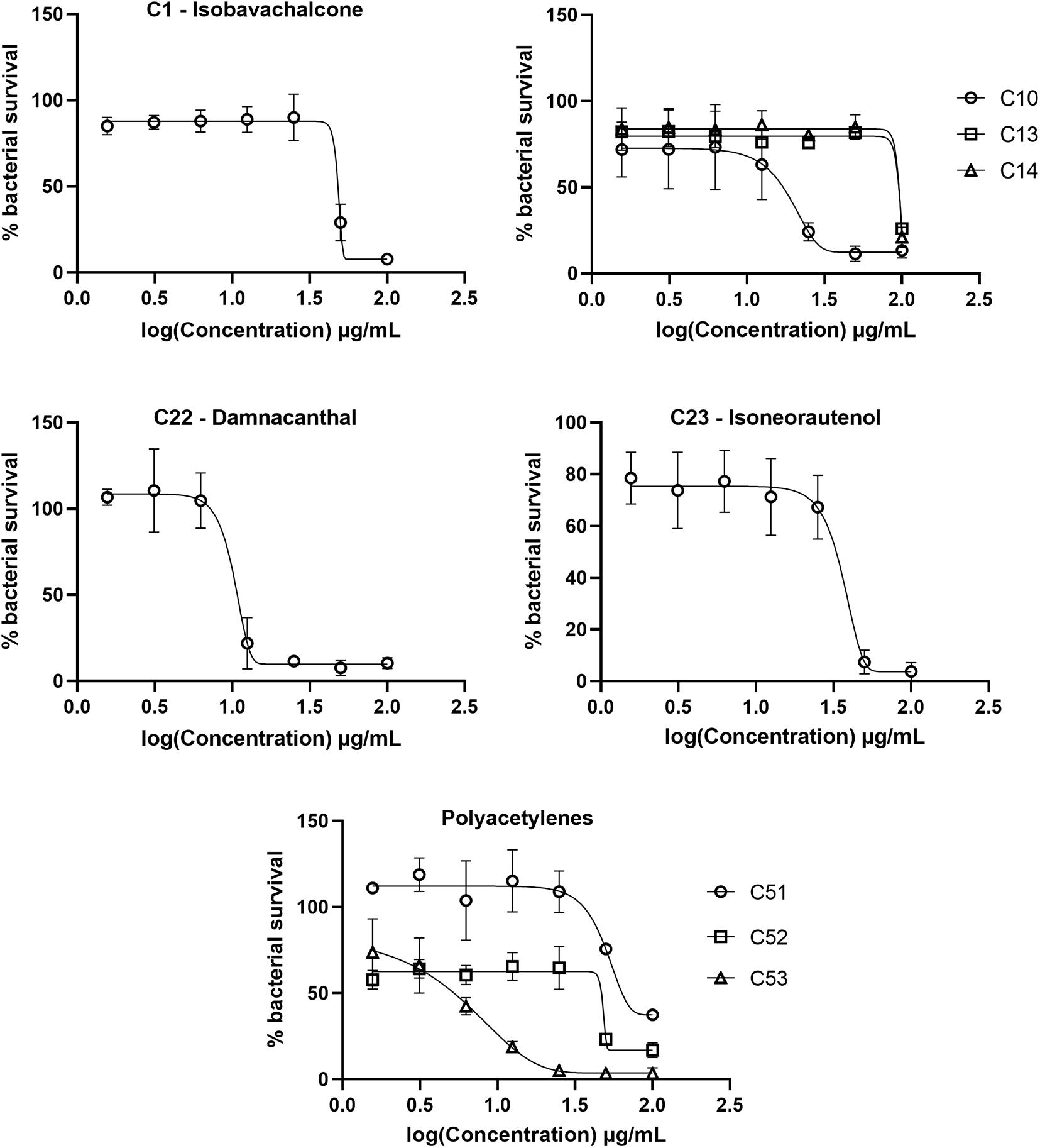
Figure 6. Dose response curves of Mycobacterium tuberculosis treated with hit compounds. M. tuberculosis was treated for 7 days with compounds ranging in concentration from 100 μg/mL to 1.56 μg/mL. Bacterial survival was measured after overnight incubation with CellTiter-Blue to establish minimum inhibitory concentrations (MICs). Data points are expressed as mean % survival relative to drug-free controls from duplicate biological replicates. Error bars represent the standard deviation.
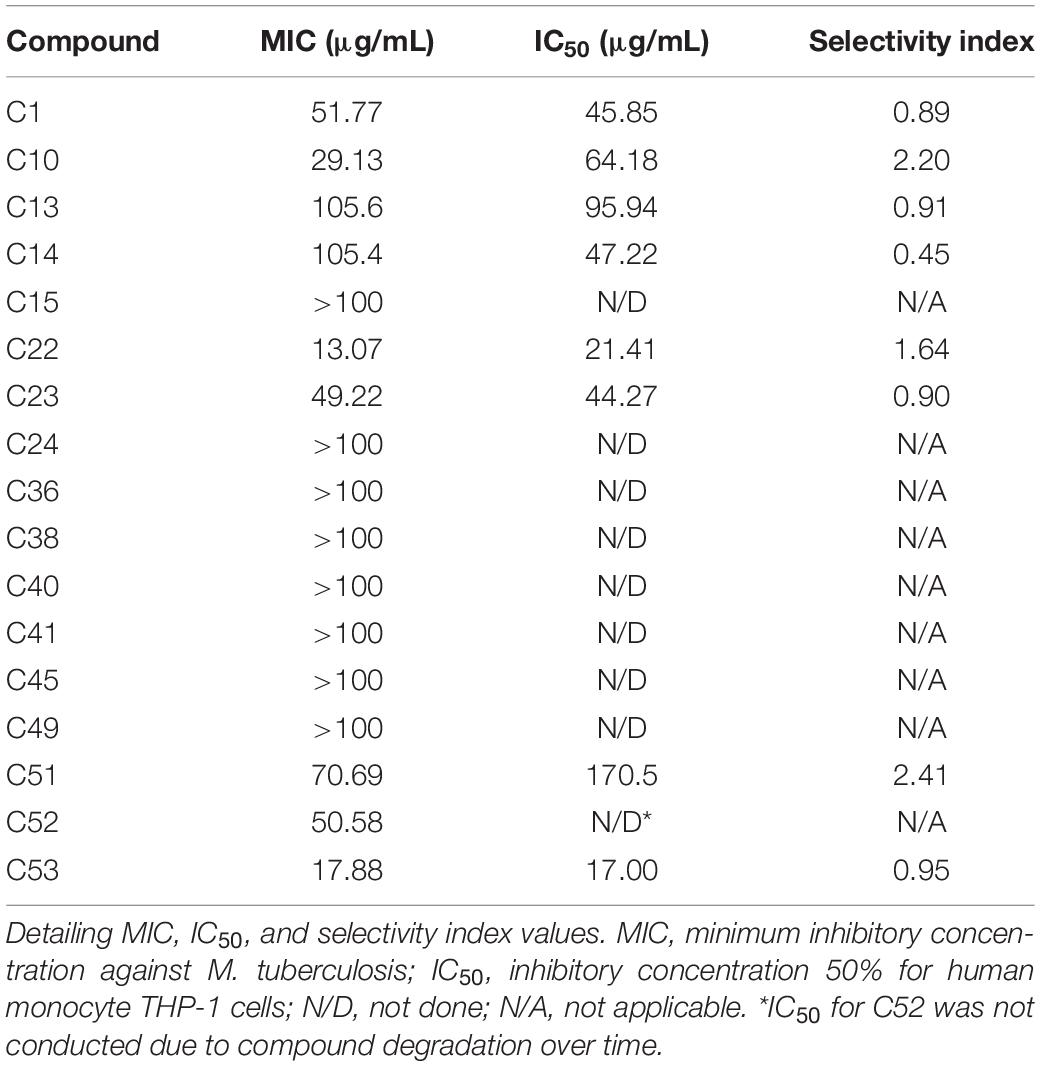
Table 1. Antimycobacterial and cytotoxic activities of hit compounds against Mycobacterium tuberculosis.
The top hit against M. tuberculosis was damnacanthal (C22 – MIC of 13.07 μg/mL), a naturally occurring secondary metabolite isolated from the tropical plant P. schimperi (Figure 6), which has previously been demonstrated to have antibacterial activity against S. aureus and Pseudomonas aeruginosa. Further investigation revealed that its inhibitory effect on S. aureus might be related to an increase in toxic reactive oxygen species (Comini et al., 2011). This natural metabolite has also been demonstrated to have prominent antifungal activity against Aspergillus ochraceus, Aspergillus niger and Candida lipolytica (Ali et al., 2000). It also displayed anti-parasitic properties against T. cruzi amastigotes (Sandjo et al., 2016b). The antimycobacterial activity of damnacanthal (C22) is likely linked to its aldehyde functional group as compound C25 (rubiadin 1-methyl ether), its reduced form, showed no anti-M. tuberculosis activity.
The dimeric polyacetylene C53 (tetradeca-4,6,8,10-tetrayne-1,14-diol) was the second most active compound against M. tuberculosis, with a MIC of 17.88 μg/mL. Several naturally occurring polyacetylene alcohols with acyclic hydrocarbon backbones have been reported with moderate to significant antimycobacterial activity against Mycobacterium fortuitum, Mycobacterium avium, Mycobacterium aurum, and M. tuberculosis H37Ra (Kobaisy et al., 1997; Schinkovitz et al., 2008; Haoxin et al., 2012). These metabolites also showed a wide spectrum of antibacterial action against Gram positive bacteria (S. aureus and B. subtilis), Gram negative bacteria (Escherichia coli and P. aeruginosa), Candida albicans, M. tuberculosis, and isoniazid-resistant M. avium (Kobaisy et al., 1997; Christensen, 2011), supporting the antimicrobial activity presented here using a synthetic polyacetylene. Other synthetic polyacetylenes of the series tested (C51 and C52) were active against M. tuberculosis (Figure 6), while C54 and C55 were not. C52 was more active than C51, with MICs of 50.58 μg/mL and 70.69 μg/mL, respectively, and both compounds differ from each other by the hybridization of atoms in the C-O bond. C53, obtained from the homodimerization of C51, showed the highest anti-M. tuberculosis activity among the polyacetylenes, suggesting that the observed bioactivity might be promoted by the high conjugated π-electron system.
The natural product isobavachalcone (C1), isolated from D. kameruniana, was moderately inhibitory to M. tuberculosis with a MIC of 51.77 μg/mL (Figure 6). This compound has been previously determined to be active against M. tuberculosis (Chiang et al., 2010), verifying our library screen. Antibacterial activity against both Gram positive and Gram negative bacteria (Mbaveng et al., 2008) has also been observed, alongside antifungal activity, inhibiting C. albicans (ElSohly et al., 2001). Prenylated chalcones structurally close to isobavachalcone have also been identified as broad-spectrum antibacterial agents (Sugamoto et al., 2011). Isoneorautenol (C23), a natural secondary metabolite isolated from E. senegalensis, had a MIC of 49.22 μg/mL against M. tuberculosis (Figure 6), and has also been shown to inhibit growth of the fast-growing non-pathogenic Mycobacterium smegmatis and Gram negative bacteria (Mitscher et al., 1988; Mbaveng et al., 2015). Of note, the natural product 4-hydroxylonchocarpine (C21), a flavonoid class chalcone isolated from D. mannii, was not mycobactericidal despite previous reports of antibacterial activity (Kuete et al., 2013).
The synthetic chromene C10 showed good antimycobacterial activity with a MIC of 29.13 μg/mL against M. tuberculosis (Figure 6). This compound, prepared for a previous study, demonstrated anti-parasitic action against both T. cruzi and L. amazonensis (Martin et al., 2018). Chromene derivatives bearing trisubstituted amines were previously reported as antimycobacterial against M. tuberculosis H37Rv (Raj and Lee, 2020). C10, the most active compound among the pyranocoumarin derivatives, differs from the others with the substituent group on the aryl moiety and the ester function on the pyran ring. C16, different from C10 by the coumarin ring, was not active against M. tuberculosis. However, when the NO2 group in C16 was replaced by the halogen atom (compound C14), a weak antimycobacterial activity was observed (Figure 6). C13 also contains a high conjugated π-electron system, although not as linear as in the C53 structure, which might support the weak activity of this compound against M. tuberculosis (Figure 6).
Compound Toxicity
Compounds that exhibited significant antimycobacterial activity were assessed for toxicity toward human monocytic (THP-1) cells in vitro, and the IC50 and selectivity index (SI) values were calculated for each compound. Compounds C1, C13, C14, C23, and C53 exhibited SI values lower than 1, while compounds C10, C22, and C51 exhibited SI values greater than 1, but limited to a maximum of 2.41 (Table 1). Therefore, efforts will be required to improve the selectivity of these compounds for mycobacteria.
Several of these compounds have been previously described to have moderate to low cytotoxicity to healthy cells. During evaluation of isobavachalcone (C1) on intracellular parasites, C1 showed some toxicity to THP-1 cells with a cytotoxic concentration 50% (CC50) of 11.65 μg/mL. Compound C22 in the same study inhibited the growth of THP-1 cells with a CC50 of 8.87 μg/mL (Sandjo et al., 2016b). Compounds C13 and C14 were also identified to be moderately toxic to human monocyte cells alongside their intracellular antiprotozoal activity (Martin et al., 2018), correlating with our findings here. Isoneorautenol (C23) was reported as antiproliferative without showing cytotoxicity to AML12 hepatocytes at concentrations up to 123.46 μM (Kuete et al., 2014; Kuete et al., 2015b). No cytotoxicity studies have been performed on the polyacetylenes (C51–C53). The intermediate MIC values for these compounds against M. tuberculosis (as shown in this study) are close to the IC50 values, resulting in greater potential for cytotoxicity. The reduction of this toxicity should be a priority for further compound development.
Conclusion
New drugs are required to treat drug-resistant TB and the rising threat of antimicrobial drug resistance (AMR). Natural products, and synthetic compounds derived and inspired by natural products, offer an extensive diversity of bioactive chemical structures for drug discovery. Natural product screens offer the opportunity to identify new chemical entities, with likely novel modes of action to overcome existing bacterial drug resistance mechanisms. Here, we report the anti-TB activity of 52 natural and synthetic compounds selected to have different hydrocarbon scaffolds and reported antimicrobial or antiparasitic activities. We identify two compounds (C22 damnacanthal, and the dimeric polyacetylene C53) with MIC values <20 μg/mL against M. tuberculosis, and five with MIC values between 25 and 71 μg/mL. The synthetic chromene C10 and polyacetylene C51 with moderate antimycobacterial activity displayed low toxicity compared to the other active compounds. This study suggests that the anthraquinone damnacanthal (C22) and synthetic polyacetylenes (C53, C52, and C51) deserve further chemical investigation as novel antimycobacterial scaffolds, and biological experimentation to elucidate mechanism of action in the search for new antimicrobial drugs.
Data Availability Statement
The original contributions presented in the study are included in the article/Supplementary Material, further inquiries can be directed to the corresponding author/s.
Author Contributions
LP, EM, VM, MLB, MWB, and LS conducted the compound isolation, synthesis, and characterization. DC, LW, and SW conducted the antimicrobial activity and cytotoxicity work. DC, LW, SW, and LS wrote the manuscript. All authors contributed to the design of the experiments and reviewed the manuscript.
Funding
Brazilian authors would like to thank CNPq and CAPES for their financial support through the post-graduation program. SW, LW, and DC acknowledge funding from the Wellcome Trust Institutional Strategic Support Fund (204833/Z/16/A) and (204538/Z/16/Z), and the University of Brighton Santander Travel Award.
Conflict of Interest
The authors declare that the research was conducted in the absence of any commercial or financial relationships that could be construed as a potential conflict of interest.
Supplementary Material
The Supplementary Material for this article can be found online at: https://www.frontiersin.org/articles/10.3389/fmicb.2020.622629/full#supplementary-material
Supplementary Figure 1 | Dose response curves of M. tuberculosis treated with isoniazid or linezolid. M. tuberculosis was treated for 7 days with drug concentrations ranging from 200 μg/mL to 0.09 μg/mL, in three-fold serial dilutions. Bacterial survival was measured after overnight incubation with CellTiter-Blue to establish minimum inhibitory concentrations (MICs). Data points are expressed as mean % survival relative to drug-free controls from duplicate biological replicates. Error bars represent the standard deviation.
References
Abegaz, B. M., Ngadjui, B. T., Dongo, E., and Tamboue, H. (1998). Prenylated chalcones and flavones from the leaves of Dorstenia kameruniana. Phytochemistry 49, 1147–1150. doi: 10.1016/S0031-9422(98)00061-2
Adem, F. A., Kuete, V., Mbaveng, A. T., Heydenreich, M., Ndakala, A., Inguru, B., et al. (2018). Cytotoxic benzylbenzofuran derivatives from Dorstenia kameruniana. Fitoterapia 128, 26–30. doi: 10.1016/j.fitote.2018.04.019
Ali, A. M., Ismail, N. H., Mackeen, M. M., Yazan, L. S., Mohamed, S. M., Ho, A. S. H., et al. (2000). Antiviral, cytotoxic and antimicrobial activities of anthraquinones isolated from the roots of Morinda elliptica. Pharm Biol. 38, 298–301. doi: 10.1076/1388-0209(200009)3841-AFT298
Bieski, I. G. C., Santos, F. R., Oliveira, R. M., Espinosa, M. M., Macedo, M., Albuquerque, U. P., et al. (2012). Ethnopharmacology of medicinal plants of the pantanal region (Mato Grosso, Brazil). Evid. Based Comp. Altern. Med. 2012, 1–36. doi: 10.1155/2012/272749
Campbell, I. B., Macdonald, S. J. F., and Procopiou, P. A. (2018). Medicinal chemistry in drug discovery in big pharma: past, present and future. Drug Discov. Today 23, 219–234. doi: 10.1016/j.drudis.2017.10.007
Chiang, C. C., Cheng, M. J., Peng, C. F., Huang, H. Y., and Chen, I. S. (2010). A novel dimeric coumarin analog and antimycobacterial constituents from Fatoua pilosa. Chem. Biodiversity. 7, 1728–1736. doi: 10.1002/cbdv.200900326
Christensen, L. P. (2011). Aliphatic C17-polyacetylenes of the falcarinol type as potential health promoting compounds in food plants of the Apiaceae family. Recent Pat. Food Nutr. Agric. 3, 64–77. doi: 10.2174/2212798411103010064
Comini, L. R., Montoya, S. C. N., Páez, P. L., Argüello, G. A., Albesa, I., and Cabrera, J. L. (2011). Antibacterial activity of anthraquinone derivatives from Heterophyllaea pustulata (Rubiaceae). J. PhotochemPhotobiol. B 102, 108–114. doi: 10.1016/j.jphotobiol.2010.09.009
De Smet, P. A. (1997). Role of plant-derived drugs and herbal medicines in healthcare. Drugs 54, 801–840. doi: 10.2165/00003495-199754060-00003
ElSohly, H. N., Joshi, A. S., Nimrod, A. C., Walker, L. A., and Clark, A. M. (2001). Antifungal chalcones from Maclura tinctoria. Planta Med. 67, 87–89. doi: 10.1055/s-2001-10621
Franzblau, S. G., Witzig, R. S., McLaughlin, J. C., Torres, P., Madico, G., Hernandez, A., et al. (1998). Rapid, low-technology MIC determination with clinical Mycobacterium tuberculosis isolates by using the microplate Alamar Blue assay. J. Clin. Microbiol. 36, 362–366. doi: 10.1128/JCM.36.2.362-366.1998
Haoxin, L., O’Neill, T., Webster, D., Johnson, J. A., and Gray, C. A. (2012). Anti-mycobacterial diynes from the Canadian medicinal plant Aralia nudicaulis. J. Ethnopharmacol. 140, 141–144. doi: 10.1016/j.jep.2011.12.048
Kobaisy, M., Abramowski, Z., Lermer, L., Saxena, G., Hancock, R. E. W., and Towers, G. H. N. (1997). Antimycobacterial polyynes of Devil’s Club (Oplopanax horridus), a North American native medicinal plant. J. Nat. Prod. 60, 1210–1213. doi: 10.1021/np970182j
Koné, W. M., Antindehou, K. K., Terreaux, C., Hostettmann, K., Traoré, D., and Dosso, M. (2004). Traditional medicine in North Cote-d’Ivoire: screening of 50 medicinal plants for antibacterial activity. J. Ethnopharmacol. 93, 43–49. doi: 10.1016/j.jep.2004.03.006
Kuete, V., Donfack, A. R. N., Mbaveng, A. T., Zeino, M., Tane, P., and Efferth, T. (2015a). Cytotoxicity of anthraquinones from the roots of Pentas schimperi towards multi-factorial drug-resistant cancer cells. Invest. New Drugs 33, 861–869. doi: 10.1007/s10637-015-0268-9
Kuete, V., Mbaveng, A. T., Zeino, M., Fozing, C. D., Ngameni, B., Kapche, G. D., et al. (2015b). Cytotoxicity of three naturally occurring flavonoid derived compounds (artocarpesin, cycloartocarpesin and isobavachalcone) towards multi-factorial drug-resistant cancer cells. Phytomedicine 22, 1096–1102. doi: 10.1016/j.phymed.2015.07.006
Kuete, V., Noumedem, J. A., and Nana, F. (2013). Chemistry and pharmacology of 4-hydroxylonchocarpin: a review. Chin. J. Integr. Med. 19, 475–480. doi: 10.1007/s11655-013-1195-7
Kuete, V., Sandjo, L. P., Kwamou, G. M., Wiench, B., Nkengfack, A. E., and Efferth, T. (2014). Activity of three cytotoxic isoflavonoids from Erythrina excelsa and Erythrina senegalensis (neobavaisoflavone, sigmoidin H and isoneorautenol) toward multi-factorial drug resistant cancer cells. Phytomedicine 21, 682–688. doi: 10.1016/j.phymed.2013.10.017
Kumar, G., Krishna, V. S., Sriram, D., and Jachak, S. M. (2020). Pyrazole–coumarin and pyrazole–quinoline chalcones as potential antitubercular agents. Arch. Pharm. 353, 1–14. doi: 10.1002/ardp.202000077
Lautié, E., Russo, O., Ducrot, P., and Boutin, J. A. (2020). Unraveling plant natural chemical diversity for drug discovery purposes. Front. Pharmacol. 11:397. doi: 10.3389/fphar.2020.00397
Lombardino, J. G., and Lowe, J. A. III (2004). The role of the medicinal chemist in drug discovery – then and now. Nat. Rev. Drug Discov. 3, 853–862. doi: 10.1038/nrd1523
Machado, V. R., Biavatti, M. W., and Danheiser, R. L. (2018). A short and efficient synthesis of the polyacetylene natural product deca-4,6,8-triyn-1-ol. Tetrahedron Lett. 59, 3405–3408. doi: 10.1016/j.tetlet.2018.07.059
Martin, E. F., Mbaveng, A. T., Moraes, M. H., Kuete, V., Biavatti, M. W., Steindel, M., et al. (2018). Prospecting for cytotoxic and antiprotozoal 4−aryl−4H−chromenes and 10−aryldihydropyrano[2,3−f] chromenes. Arch. Pharm. (Weinheim) 351, 1–11. doi: 10.1002/ardp.201800100
Mbaveng, A. T., Kuete, V., Ngameni, B., Beng, V. P., Ngadjui, B. T., Meyer, J. J. M., et al. (2012). Antimicrobial activities of the methanol extract and compounds from the twigs of Dorstenia mannii (Moraceae). BMC Comp. Altern. Med. 12:83. doi: 10.1186/1472-6882-12-83
Mbaveng, A. T., Ngameni, B., Kuete, V., Simo, I. K., Ambassa, P., Roy, R., et al. (2008). Antimicrobial activity of the crude extracts and five flavonoids from the twigs of Dorstenia barteri (Moraceae). J. Ethnopharmacol. 116, 483–489. doi: 10.1016/j.jep.2007.12.017
Mbaveng, A. T., Sandjo, L. P., Tankeo, S. B., Ndifor, A. R., Pantaleon, A., Nagdjui, B. T., et al. (2015). Antibacterial activity of nineteen selected natural products against multi-drug resistant Gram-negative phenotypes. SpringerPlus. 4, 823–831. doi: 10.1186/s40064-015-1645-8
Mitscher, L. A., Okwute, S. K., Gollapudi, S. R., Drake, S., and Avona, E. (1988). Antimicrobial pterocarpans of Nigerian Erythrina mildbraedii. Phytochemistry 27, 3449–3452. doi: 10.1016/0031-9422(88)80746-5
The Working Group for New TB Drugs (2020). New Drugs for TB Clinical Pipeline. Available online at: https://www.newtbdrugs.org/pipeline/clinical (accessed October 19, 2020).
Ngadjui, B. T., Abegaz, B. M., Dongo, E., Tamboue, H., and Fogue, S. K. (1998). Geranylated and prenylated flavonoids from the twigs of Dorstenia mannii. Phytochemistry 48, 349–354. doi: 10.1016/S0031-9422(97)01120-5
Pascolutti, M., and Quinn, R. J. (2014). Natural products as lead structures: chemical transformations to create lead-like libraries. Drug Discov. Today 19, 215–221. doi: 10.1016/j.drudis.2013.10.013
Pollo, L. A. E., Moraes, M. H., Cisilotto, J., Creczynski-Pasa, T. B., Biavatti, M. W., Steindel, M., et al. (2017). Synthesis and in vitro evaluation of Ca2+ channel blockers 1,4-dihydropyridines analogues against Trypanosoma cruzi and Leishmania amazonensis: SAR analysis. Parasitol. Int. 66, 789–797. doi: 10.1016/j.parint.2017.08.005
Raj, L., and Lee, J. (2020). 2H/4H-Chromenes – A versatile biological attractive scaffold. Front. Chem. 8:623. doi: 10.3389/fchem.2020.00623
Rogoza, L. N., Salakhutdinov, N. F., and Tolstikov, G. A. (2010). Antituberculosis activity of natural and synthetic compounds. Chem. Sustain. Dev. 10, 343–375.
Salomon, C. E., and Schmidt, L. E. (2012). Natural products as leads for tuberculosis drug development. Curr. Top. Med. Chem. 12, 735–765. doi: 10.2174/156802612799984526
Sandjo, L. P., Kuete, V., Nana, F., Kirsch, G., and Efferth, T. (2016a). Synthesis and cytotoxicity of 1,4-Dihydropyridines and an unexpected 1,3-Oxazin-6-one. HelvChim. Acta 99, 310–314. doi: 10.1002/hlca.201500265
Sandjo, L. P., Moraes, M. H., Kuete, V., Kamdoum, B. C., Ngadjui, B. T., and Steindel, M. (2016b). Individual and combined antiparasitic effect of six plant metabolites against Leishmania amazonensis and Trypanosoma cruzi. Bioorg. Med. Chem. Lett. 26, 1772–1775. doi: 10.1016/j.bmcl.2016.02.044
Schinkovitz, A., Stavri, M., Gibbons, S., and Bucar, F. (2008). Antimycobacterial polyacetylenes from Levisticum officinale. Phytother. Res. 22, 681–684. doi: 10.1002/ptr.2408
Solecki, E., and Shanidar, I. V. (1975). A Neanderthal flower burial in northern Iraq. Science 190, 880–881. doi: 10.1126/science.190.4217.880
Somagond, S. M., Kamble, R. R., Bayannavar, P. K., Shaikh, S. K. J., Joshi, S. D., Kumbar, V. M., et al. (2019). Click chemistry based regioselective one-pot synthesis of coumarin-3-yl-methyl-1,2,3-triazolyl-1,2,4-triazol-3(4H)-ones as newer potent antitubercular agents. Arch. Pharm. (Weinheim) 352, 1–13. doi: 10.1002/ardp.201900013
Sugamoto, K., Matsusita, Y. I., Matsui, K., Kurogi, C., and Matsui, T. (2011). Synthesis and antibacterial activity of chalcones bearing prenyl or geranyl groups from Angelica keiskei. Tetrahedron. 67, 5346–5359. doi: 10.1016/j.tet.2011.04.104
Teklehaymanot, T. (2009). Ethnobotanical study of knowledge and medicinal plants use by the people in Dek Island in Ethiopia. J. Ethnopharmacol. 124, 69–78. doi: 10.1016/j.jep.2009.04.005
Thomford, N. E., Senthebane, D. A., Rowe, A., Munro, D., Seele, P., Maroyi, A., et al. (2018). Natural products for drug discovery in the 21st century: innovations for novel drug discovery. Int. J. Mol. Sci. 19, 1578–1606. doi: 10.3390/ijms19061578
Togola, A., Austarheim, I., Theis, A., Diallo, D., and Paulse, B. S. (2008). Ethnopharmacological uses of Erythrina senegalensis: a comparison of three areas in Mali, and a link between traditional knowledge and modern biological science. J. Ethobiol. Ethnomed. 4, 6–14. doi: 10.1186/1746-4269-4-6
Keywords: Mycobacterium tuberculosis, drug discovery, natural product, synthetic polyacetylenes, antimicrobial drug resistance
Citation: Pollo LAE, Martin EF, Machado VR, Cantillon D, Wildner LM, Bazzo ML, Waddell SJ, Biavatti MW and Sandjo LP (2021) Search for Antimicrobial Activity Among Fifty-Two Natural and Synthetic Compounds Identifies Anthraquinone and Polyacetylene Classes That Inhibit Mycobacterium tuberculosis. Front. Microbiol. 11:622629. doi: 10.3389/fmicb.2020.622629
Received: 28 October 2020; Accepted: 29 December 2020;
Published: 18 January 2021.
Edited by:
Maria Rosalia Pasca, University of Pavia, ItalyReviewed by:
Divakar Sharma, Indian Institute of Technology Delhi, IndiaDiana Machado, New University of Lisbon, Portugal
Copyright © 2021 Pollo, Martin, Machado, Cantillon, Wildner, Bazzo, Waddell, Biavatti and Sandjo. This is an open-access article distributed under the terms of the Creative Commons Attribution License (CC BY). The use, distribution or reproduction in other forums is permitted, provided the original author(s) and the copyright owner(s) are credited and that the original publication in this journal is cited, in accordance with accepted academic practice. No use, distribution or reproduction is permitted which does not comply with these terms.
*Correspondence: Simon J. Waddell, s.waddell@bsms.ac.uk; Louis P. Sandjo, p.l.sandjo@ufsc.br