- 1Departamento de Biología Funcional, Área de Microbiología, Universidad de Oviedo, Oviedo, Spain
- 2Instituto Universitario de Oncología del Principado de Asturias, Universidad de Oviedo, Oviedo, Spain
- 3Instituto de Investigación Sanitaria del Principado de Asturias (ISPA), Universidad de Oviedo, Oviedo, Spain
- 4Fundación MEDINA, Centro de Excelencia en Investigación de Medicamentos Innovadores en Andalucía, Granada, Spain
- 5Departamento de Ingeniería Química y Tecnología del Medio Ambiente, Área de Ingeniería Química, Universidad de Oviedo, Oviedo, Spain
Actinobacteria are the main producers of bioactive natural products essential for human health. Although their diversity in the atmosphere remains largely unexplored, using a multidisciplinary approach, we studied here 27 antibiotic producing Actinobacteria strains, isolated from 13 different precipitation events at three locations in Northern and Southern Spain. Rain samples were collected throughout 2013–2016, from events with prevailing Western winds. NOAA HYSPLIT meteorological analyses were used to estimate the sources and trajectories of the air-mass that caused the rainfall events. Five-day backward air masses trajectories of the diverse events reveals a main oceanic source from the North Atlantic Ocean, and in some events long range transport from the Pacific and the Arctic Oceans; terrestrial sources from continental North America and Western Europe were also estimated. Different strains were isolated depending on the precipitation event and the latitude of the sampling site. Taxonomic identification by 16S rRNA sequencing and phylogenetic analysis revealed these strains to belong to two Actinobacteria genera. Most of the isolates belong to the genus Streptomyces, thus increasing the number of species of this genus isolated from the atmosphere. Furthermore, five strains belonging to the rare Actinobacterial genus Nocardiopsis were isolated in some events. These results reinforce our previous Streptomyces atmospheric dispersion model, which we extend herein to the genus Nocardiopsis. Production of bioactive secondary metabolites was analyzed by LC-UV-MS. Comparative analyses of Streptomyces and Nocardiopsis metabolites with natural product databases led to the identification of multiple, chemically diverse, compounds. Among bioactive natural products identified 55% are antibiotics, both antibacterial and antifungal, and 23% have antitumor or cytotoxic properties; also compounds with antiparasitic, anti-inflammatory, immunosuppressive, antiviral, insecticidal, neuroprotective, anti-arthritic activities were found. Our findings suggest that over time, through samples collected from different precipitation events, and space, in different sampling places, we can have access to a great diversity of Actinobacteria producing an extraordinary reservoir of bioactive natural products, from remote and very distant origins, thus highlighting the atmosphere as a contrasted source for the discovery of novel compounds of relevance in medicine and biotechnology.
Introduction
In nature, members of the Phylum Actinobacteria continue to be the main producers of structurally diverse bioactive natural products, essential for human health. Among Actinobacteria, species of the Streptomyces genus are the most prolific source of novel compounds of medical and industrial relevance as antibiotic and anticancer drugs urgently needed to overcome clinical resistance and toxicity problems. Although traditionally considered soil bacteria, there is increasing evidence that Streptomyces species are ubiquitous, being present not only on terrestrial ecosystems, but also in some of the most extreme and less explored environments on our planet such as the oceans and the atmosphere.
New trends in drug discovery include the search for novel bioactive Actinobacteria in unexplored or underexplored environments. Previous reports in oceanic and atmospheric environments of the Cantabrian Sea region (North Spain, Northeast Atlantic) revealed that phylogenetically diverse Actinobacteria, with a great pharmacological potential, are widespread among intertidal and subtidal seaweeds (Braña et al., 2015; Sarmiento-Vizcaíno et al., 2016) and also among deep-sea coral reefs ecosystems (Sarmiento-Vizcaíno et al., 2017b), where a novel barotolerant actinobacterium, Myceligenerans cantabricum, was isolated (Sarmiento-Vizcaíno et al., 2015). Some of these marine strains were the source of nine new biologically active natural products with antibiotic properties against clinically relevant antibiotic resistant pathogens and cytotoxic activities toward diverse human cancer cell lines (Braña et al., 2017a, b; Sarmiento-Vizcaíno et al., 2017b; Ortiz-López et al., 2018; Rodríguez et al., 2018).
Strains belonging to three Streptomyces species widespread among these coastal and deep-sea habitats (Streptomyces cyaneofuscatus, Streptomyces carnosus, and Streptomyces albidoflavus) were also isolated from different cloud precipitation events happened in the Cantabrian Sea Coast (Braña et al., 2015; Sarmiento-Vizcaíno et al., 2016). Since then, atmospheric precipitations (hailstone, rainwater and snow) were used as natural sampling tools for the study of actinobacterial diversity in the atmosphere. Bioactive strains corresponding to about 3–4% of known Streptomyces species were isolated after precipitations and found to produce a great number of natural products with different biological activities, mainly as antimicrobial and anticancer agents (Sarmiento-Vizcaíno et al., 2018). These atmospheric-derived strains also produced 38 molecules not found in Natural products databases, thus revealing the atmosphere as a novel and promising source for natural product discovery.
Based on previous observations of cultivable Streptomyces species isolated in recent years from different precipitation events on the Cantabrian coast, an atmospheric dispersal model was proposed to explain the cosmopolitan distribution of highly halotolerant Streptomyces species (Sarmiento-Vizcaíno et al., 2016). According to this model, coupled to the Earth’s hydrological cycle, marine bioaerosols forming clouds contribute to the transfer of Streptomyces from oceans into the atmosphere, were they travel dispersed by winds, falling down to the earth by precipitation. Further support for this model came from a culture-independent approach, which reported Actinobacteria among the most dominant phyla in atmospheric precipitations in Japan, also showing seasonal variations in correlation with estimated air mass trajectories (Hiraoka et al., 2017). Connections between oceans, clouds and atmosphere, and their relevance in atmospheric chemistry and climate were addressed through the study of sea spray aerosols (Cochran et al., 2017). Actinobacterial transfer into sea spray aerosols in an experimental ocean-atmosphere mesocosm was also reported (Michaud et al., 2018).
In a culture dependent approach, we provide here further insights into the phylogenetic and secondary metabolic diversity of bioactive atmospheric Actinobacteria isolated from rainwater in precipitations events from Westerly winds in Spain over 4 years’ time. This approach involved rainwater sampling from different locations in Spain, meteorological analyses, taxonomical and phylogenetic analyses with identification at species level. Antimicrobial assays, metabolic profiling and LC-UV-MS analyses of compounds produced were used to assess the Actinobacteria biosynthetic diversity.
Materials and Methods
Sampling of Atmospheric Precipitations
Atmospheric precipitations samples, including rainwater, hailstone and snow were collected within years 2013–2016 at the North of Spain, at the Cantabrian Sea coastal region of Asturias (Figure 1). This is a remarkably wet and rainy region, whose climate is under the influence of the Atlantic Ocean. Samples of 2–3 mL were taken in sterile recipients at the localities of Gijón (43° 32′ N, 5° 39′ W), and Oviedo (43° 21′ N, 5° 52′ W) and plated on selective agar media as previously described (Braña et al., 2015; Sarmiento-Vizcaíno et al., 2016). An additional rain sample (50 mL) was collected in 2016, in Seville (37° 23′ N, 5° 59′ W), Andalusia, South of Spain. Seville has a Mediterranean climate and is considered one of the warmest cities in continental Europe. During all precipitation events sampled here the prevailing wind direction has been Western.
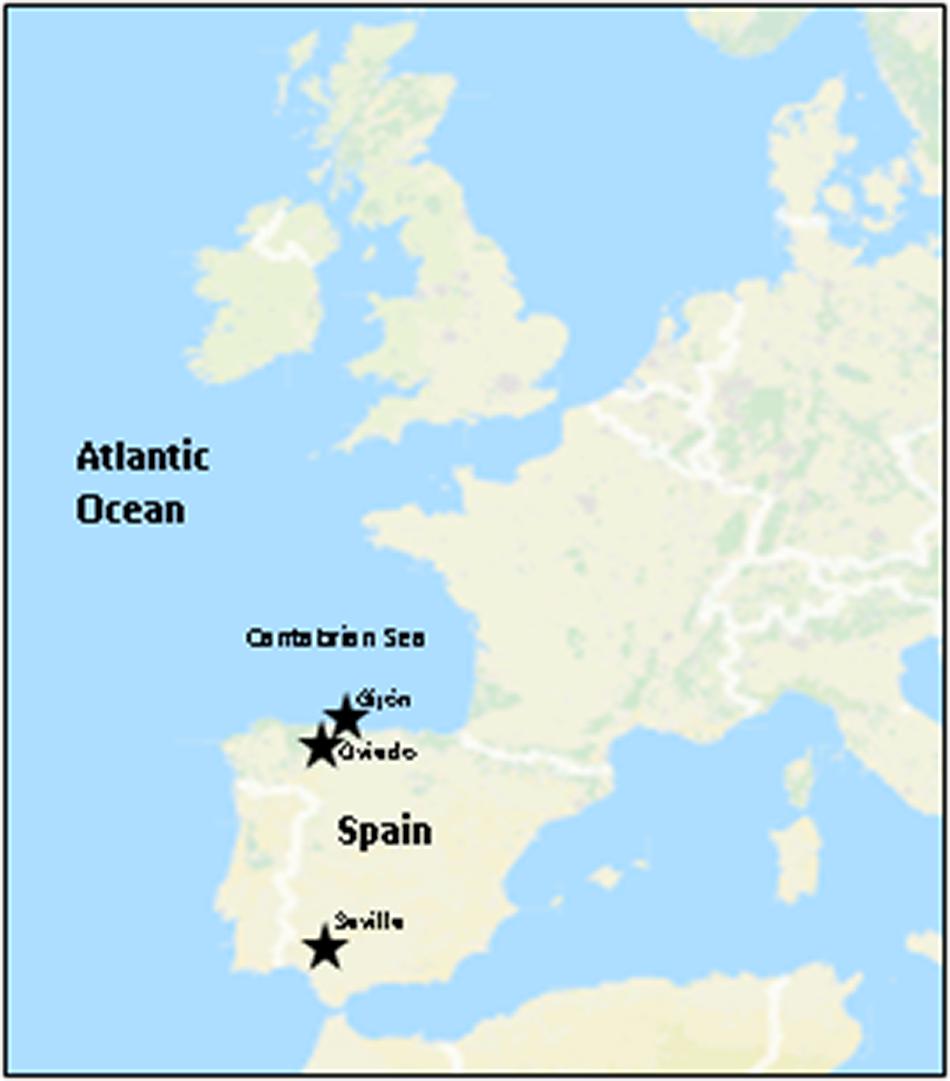
Figure 1. Sampling locations in Spain. Overview of the European Seas (Atlantic Ocean). Stars indicate the sampling locations in Northern and Southern Spain.
Isolation of Actinobacteria Strains and Culture Media
A collection of cultivable Actinobacteria strains were obtained after plating of precipitation samples on selective agar media, prepared with cycloheximide (80 μg mL–1) as antifungal and nalidixic acid (20 μg mL–1) as anti-Gram negative bacteria, using MOPS BLEB 1/6 (Oxoid) basal medium as previously reported (Sarmiento-Vizcaíno et al., 2016). Two different media either prepared with distilled water or with a supplement of 3.5% NaCl were used in selection plates. After 2–3 weeks of incubation at 28°C, colonies were selected based on different morphological features and pigment production on R5A agar plates. Isolated pure cultures were conserved in 20% glycerol at both −20° and −70°C. For halotolerance studies, MOPS BLEB 1/6 (Oxoid) was used as the basal medium, adding NaCl at 0, 3.5, 7.0, and 10.5% (w/v) final concentrations. R5A medium was used for secondary metabolite production as previously described (Sarmiento-Vizcaíno et al., 2018).
Bioactive Strains Selection
The antimicrobial activities of isolates were determined by agar diffusion methods using the following indicator microorganisms: the Gram-positive bacteria Micrococcus luteus ATCC 14452 and Streptomyces 85E ATCC 55824, the Gram-negative Escherichia coli ESS, and the yeast Saccharomyces cerevisiae var. carlsbergensis as previously reported (Sarmiento-Vizcaíno et al., 2018). Analyses were performed in TSA1/2 (Merck) against bacteria and in Sabouraud 1/2 (Pronadisa) against yeast. For antibiotic production Actinobacteria cultures were routinely cultured. Figure 2 shows an example of bioassays performed against Micrococcus luteus as indicator bacteria. Agar plugs of 7 mm diameter from Actinobacteria cultures on solid R5A medium (Figure 2A) were assay for initial selection of bioactive isolates. Also Kirby-Bauer based test using with 6-mm-diameter AA Discs (Whatman), loaded with ethyl acetate extracts of bioactive isolates, were performed (Figure 2B). Agar plugs assays detect all diffusible compounds produced by actinobacterial strains, both polar and apolar, whereas the AA discs bioassays only detect diffusible apolar molecules which were extracted with ethyl acetate.
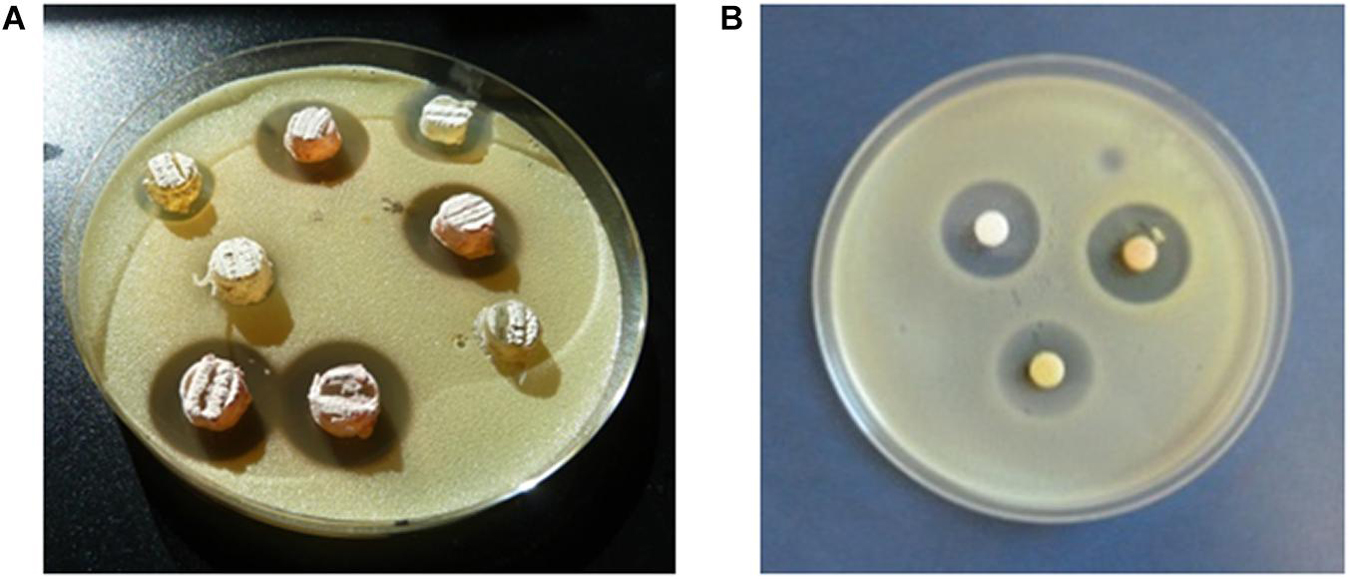
Figure 2. Bioassay diffusion assays. Micrococcus luteus was used as indicator microorganism. The zones of complete inhibition are measured as the diameters in mm. (A) Agar plugs. (B) AA discs loaded with ethyl acetate extracts of the isolates.
Air Mass Backward Trajectories Analyses
To estimate the long-range transport journey of air masses that originated the precipitation events herein studied, backward trajectories were generated using the HYSPLIT model (Hybrid Single Particle Lagrangian Integrated Trajectory) from the Global Data Assimilation System of National Oceanic and Atmospheric Administration, United States (Stein et al., 2015). To track the transport pathways of air masses and determine the origin of diverse air parcels, 5-day backward trajectories (used generally in bioaerosol studies) were obtained using the NOAA model.1 To find out the trajectories of atmospheric air masses, the sampling locations were used as the backward trajectory start point with altitudes over the sea level of 30, 1,000 and 3,000 m (Gijón), as previously reported (Sarmiento-Vizcaíno et al., 2018); 300, 1,000, and 3,000 m (Oviedo) and 7, 1,000, and 3,000 m (Seville).
16S RNA Analysis Identification and Phylogenetic Analysis
For taxonomic identification of the strains, DNA was extracted with a microbial isolation kit (Ultra Clean, MoBio Laboratories, Inc.) and standard methods were used for checking the purity (Russell and Sambrook, 2001). Partial 16S rRNA gene sequences of the bacterial strains were obtained by using the 616V (forward) and 699R (reverse) primers (Arahal et al., 2008) in PCR amplification as previously described (Braña et al., 2015). The nucleotide sequences were compared to sequences in databases using the BLAST program (Basic Local Alignment Search Tool) against the NCBI (National Centre for Biotechnology Information), submitted and deposited in the EMBL sequence database with accession numbers LR702033-LR702059. Phylogenetic analysis of the strains based on 16S rRNA sequences was performed as previously reported (Sarmiento-Vizcaíno et al., 2018).
Chromatographic Analysis
Plugs of R5A plates (about 7 mL) were extracted using ethyl acetate in neutral and acidic (1% v/v formic acid) conditions. After evaporation, the organic fraction residue was redissolved in 100 μL of a mixture of DMSO and methanol (50:50). The analyses of the samples were performed by reversed phase liquid chromatography as previously described (Braña et al., 2015; Sarmiento-Vizcaíno et al., 2016).
Identification of Compounds by LC-UV-Vis and LC-UV-HRMS Analyses
Samples were first analyzed and evaluated using an in-house HPLC-UV-Vis database. LC-UV-HRMS analyses were carried out as previously reported (Pérez-Victoria et al., 2016; Sarmiento-Vizcaíno et al., 2018) and major peaks in each chromatogram were searched against the MEDINA’s internal database and also against the Dictionary of Natural Products (DNP) (Chapman & Hall/CRC, 2015).
Results
Isolation and Characterization of Bioactive Atmospheric Actinobacteria by Sampling Multiple Precipitation Events in Spain
The strains herein studied were obtained from a unique Actinobacteria collection generated, during 4 years’ time frame (2013–2016) from diverse atmospheric precipitation events in Spain, as previously reported (Sarmiento-Vizcaíno et al., 2018). After a dereplication process involving phenotypical features, antibiotic activity and also meteorological analyses (see next section), 27 morphologically different bioactive strains isolated from rainwater from storm clouds transported by Western winds were selected for this study. Table 1 shows the results of initial antibiotic analyses of selected strains against a panel of indicator microorganisms (bacteria and fungi) by using agar diffusion assays (Figure 2A). The strains were isolated from samples collected in 12 rainfall events, and one hailstone event (A-241) at three different locations in Spain. The three different sampling places are shown in Figure 1. Among the 27 bioactive isolates, 18 were obtained from samples collected in the North Spain (43° N), 12 in the Cantabrian Sea coast (Gijón) and six strains at 28 km inland (Oviedo); finally 9 strains were isolated from a single rainfall event in South Spain (Seville, 37° N).
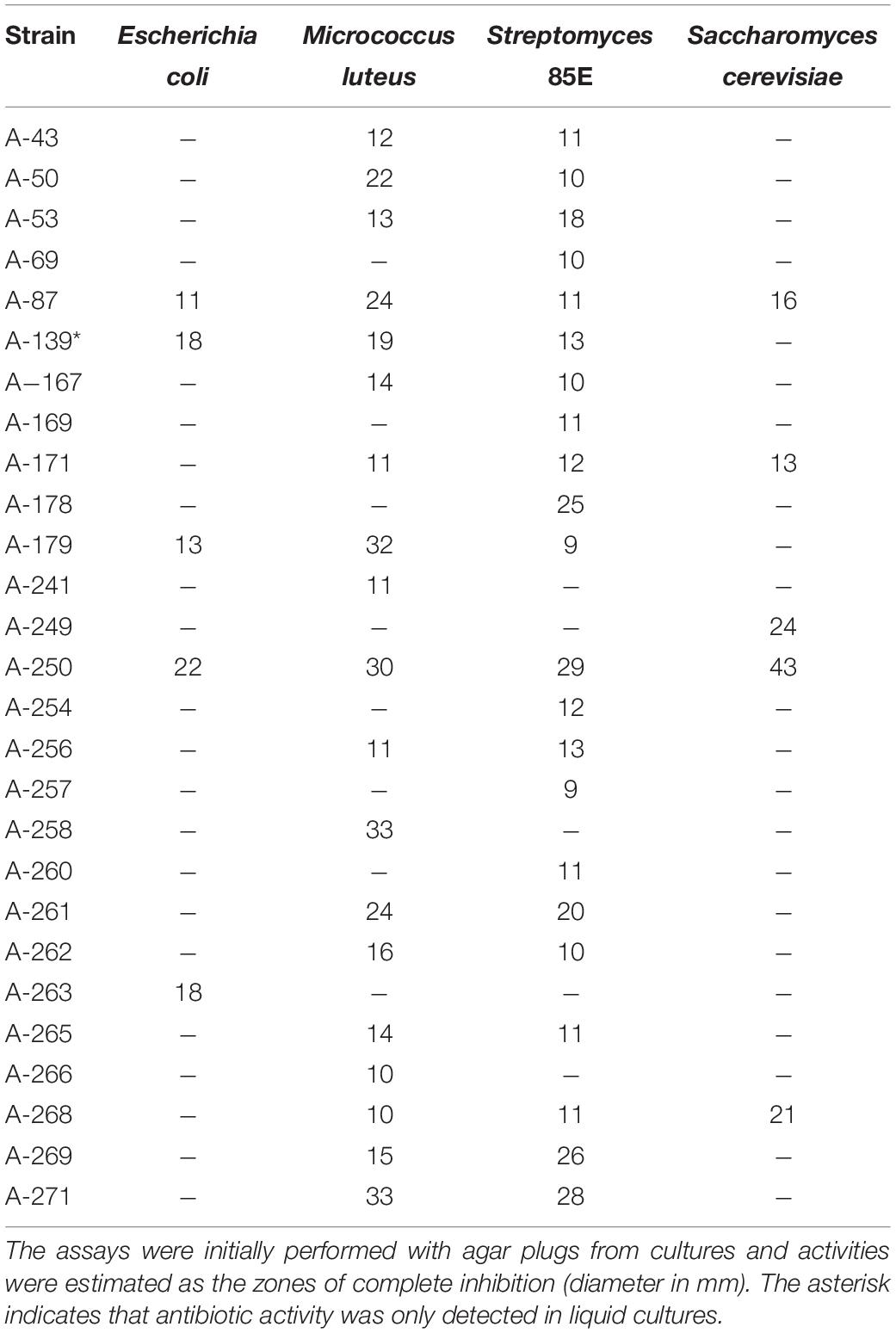
Table 1. Antibiotic activities of atmospheric Actinobacteria cultures against a panel of Gram-negative, Gram-positive bacteria and fungi.
Backward Transport Trajectories Analyses
Meteorological analyses were performed to estimate the sources and trajectories of the different air masses that originated the precipitation events in which the selected strains were isolated. These sources were estimated using 5 days HYSPLIT backward trajectories. As shown in Figure 3, most backward trajectories showed air masses traveled eastward off the Atlantic Ocean toward continental Europe. As estimated, the air masses reaching the three sampling sites in Spain were predominantly of marine origin. In the atmospheric precipitation events herein studied, different air masses were transported by westerly winds (traveling at different altitudes) mainly from the Atlantic Ocean. In some events, that will be further stated, the trajectory also involves long-range transport from continental America, the Arctic Ocean and even the Northern Pacific Ocean, to downwind areas, such as the sampling place in continental Europe.
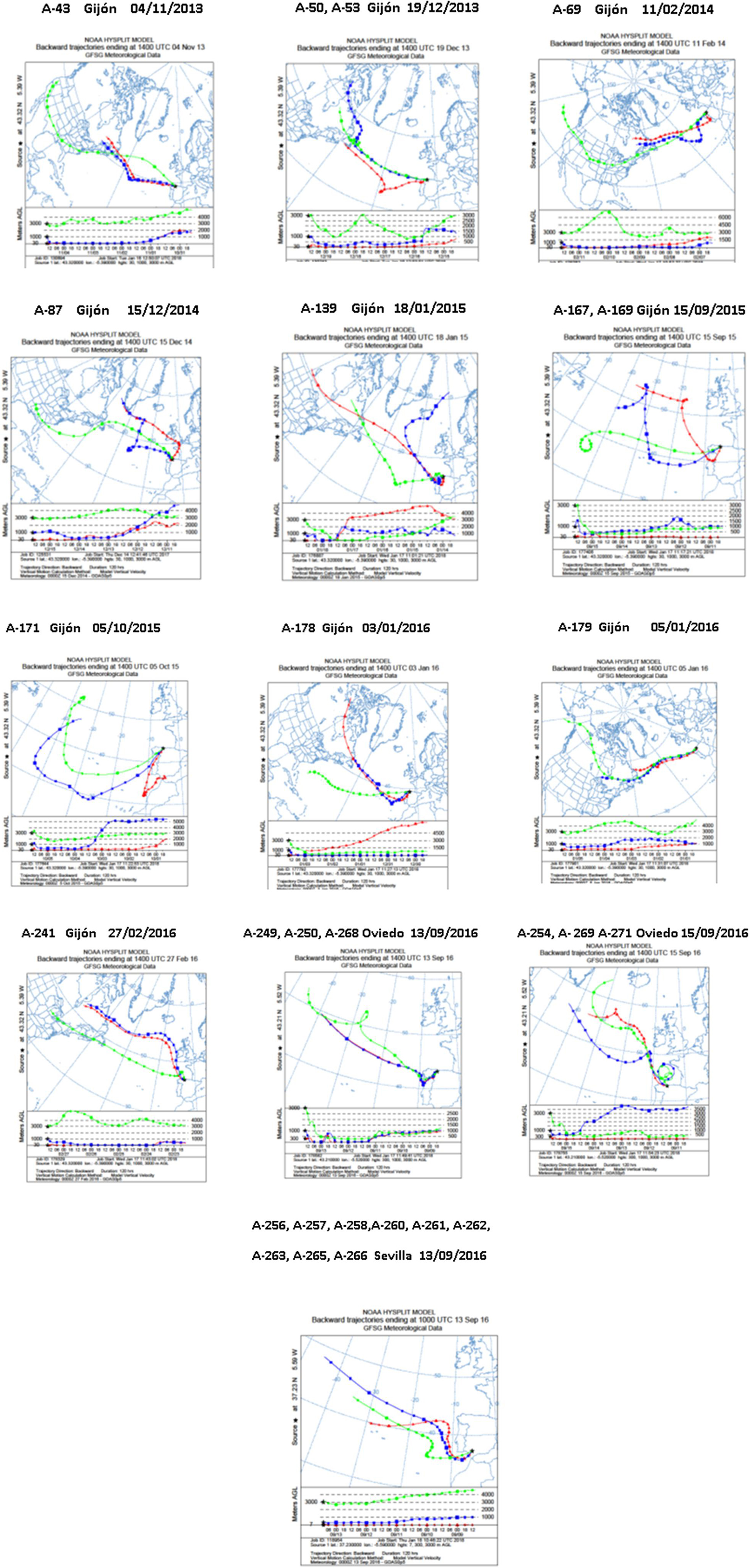
Figure 3. Five-day backward trajectories of air masses generating the storms that arrived at Spain and caused the diverse precipitation events. They were calculated with the NOAA HYSPLIT Model with three different transects with different arriving height as previously reported (Sarmiento-Vizcaíno et al., 2018). The sampling locations were used as the backward trajectory start point with altitudes over sea level of 30, 1,000, and 3,000 m (Gijón), 300, 1,000, and 3,000 m (Oviedo), and 7, 1,000, and 3,000 m (Seville). Sampling places are indicated by the black asterisks.
Taxonomic Identification and Phylogenetic Analyses of Bioactive Isolates
Identification of airborne-derived bioactive strains was determined by sequencing fragments of their 16S rRNA gene. Nucleotide sequences were then deposited in the EMBL database, and corresponding accession numbers are shown on Table 2. Phylogenetic analyses of isolates (Figure 4), based on 16S rRNA gene alignments, demonstrate that all isolates belong to two different genera among the Phylum Actinobacteria, since they share 99–100% identity with known actinobacterial species. As shown in Table 2, the identified strains have their closest homologs in previous species isolated from very diverse oceanic and terrestrial habitats. Among 27 studied isolates, 23 belonged to the Streptomyces genus, as previous reports in this environment. Interestingly, all these species are different from the ones isolated in a hailstone precipitation event from clouds transported by prevalent Northwestern winds (Sarmiento-Vizcaíno et al., 2018), thus suggesting that depending on the wind direction different strains can be isolated.
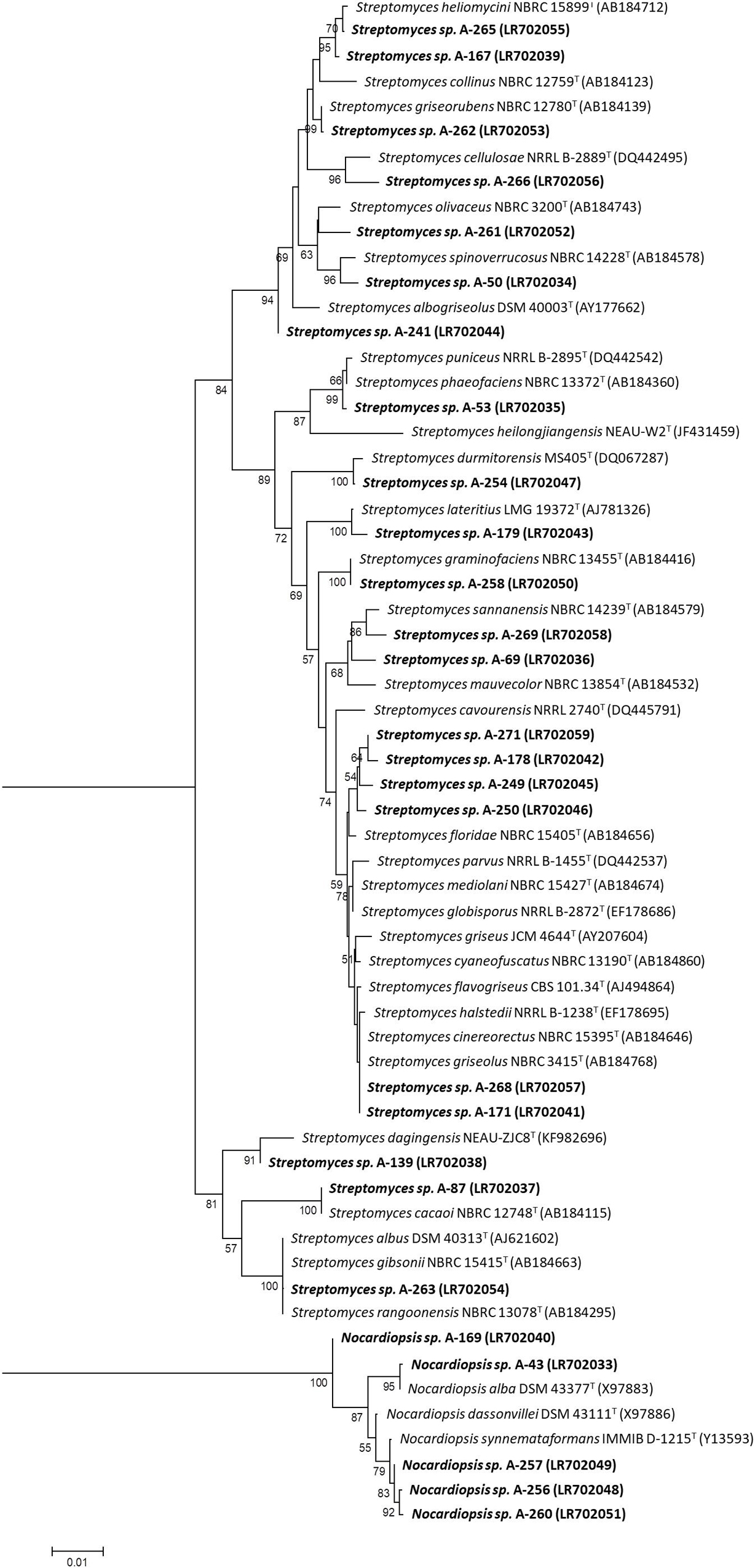
Figure 4. Neighbor-joining phylogenetic tree generated by distance matrix analysis of 16S rRNA gene sequences from atmospheric Actinobacteria (Streptomyces and Nocardiopsis) strains (highlighted) and nearest phylogenetic relatives. The numbers on branch nodes indicate bootstrap values (1,000 resamplings; only values > 50% are shown). Bar represents1% sequence divergence.
In addition, isolates belonging to the actinobacterial genus Nocardiopsis were herein identified in two precipitation events. A Nocardiopsis alba homolog, isolated in one of the North sampling places (Gijón), and several Nocardiopsis synnemataformans homologs in the South sampling place (Seville), which differ approximately in 6 latitudinal degrees. Nocardiopsis species were previously reported both in terrestrial and aquatic ecosystems (Bennur et al., 2015; Table 2) and are considered of pharmaceutical and biotechnological relevance due to its ability to produce diverse bioactive secondary metabolites (Bennur et al., 2016; Ibrahim et al., 2018).
A generalized feature of all Actinobacteria here studied is their ability to tolerate high NaCl concentrations, in the range 3.5–10.5% (Table 2). This high halotolerance is in agreement with previous reports within Streptomyces (Sarmiento-Vizcaíno et al., 2018) and in Nocardiopsis species, which are considered as the most abundant halophilic actinobacteria (Hamedi et al., 2013).
Metabolite Profiling Analysis and Identification of Bioactive Secondary Metabolites Produced
Chemical diversity of atmospheric Actinobacteria was assessed by metabolic profiling analyses of ethyl acetate extracts of bioactive strains, obtained in neutral and acidic conditions, screened for antibiotic production using agar diffusion with AA discs (Figure 2B), against a panel of indicator microorganisms (Table 3). Strong antibiotic activities were observed in all extracts, which were particularly active against M. luteus. The extracts were then analyzed for production of secondary metabolites by LC-UV and LC/HRMS analyses in combination with searches in UV and MS databases or the DNP after generation of a molecular formula of each peak based on HRMS results. Most of the strains show complex metabolic profiles producing multiple secondary metabolites in R5A medium (Supplementary Material 1). Figure 5 displays UV210 nm chromatograms corresponding to Nocardiopsis sp. A-256 and Streptomyces sp. A-254 samples.
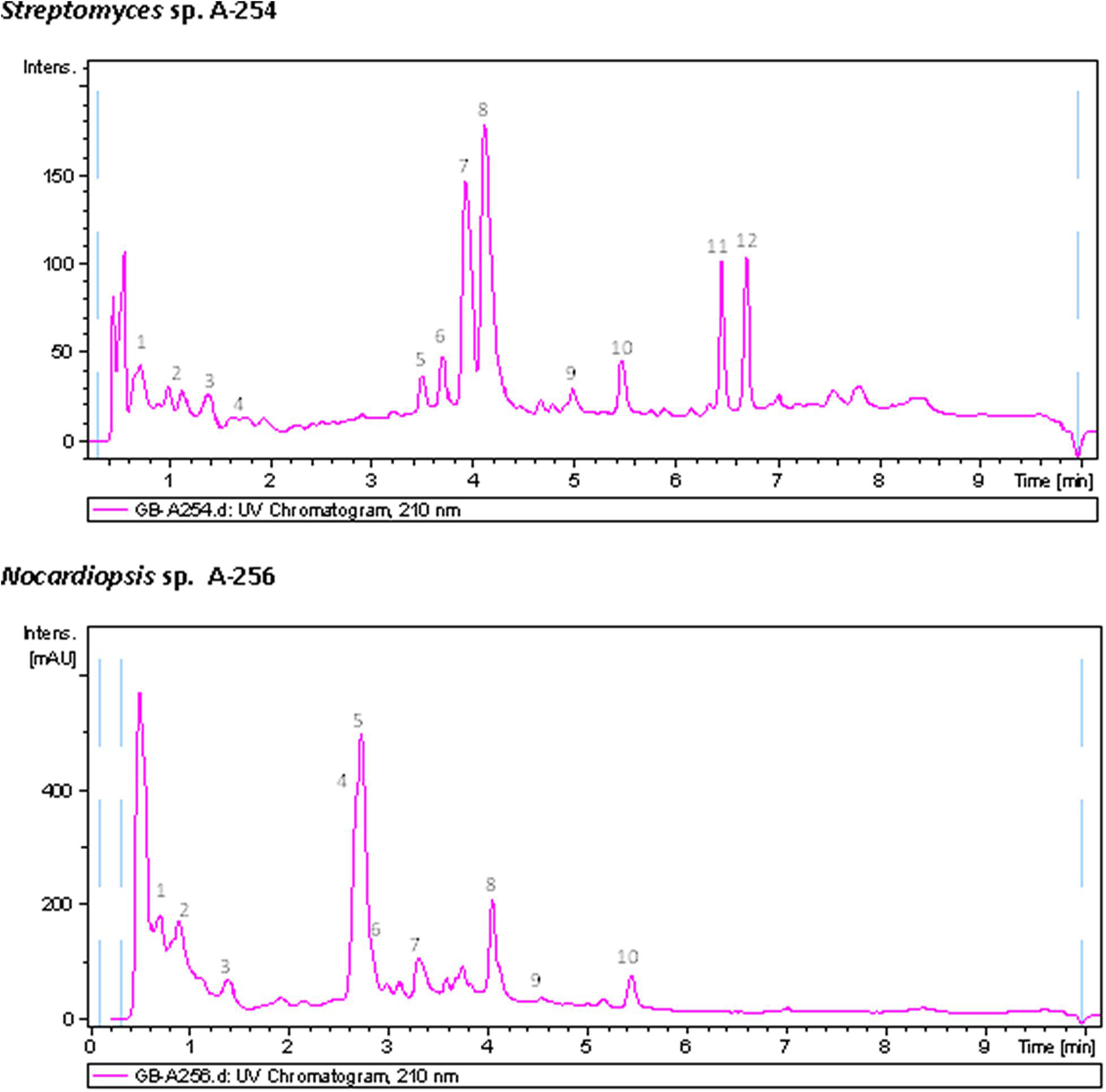
Figure 5. UV210 nm chromatogram of samples A-254 and A-256 with peaks annotated showing dereplicated components. Dereplicated components in sample A-254: (1) Cyclo(prolylvalyl), (2) Cyclo(leucylprolyl), (3) Cyclo(phenylalanylprolyl), (4) Cyclo(prolytryptophyl), (5) C21H38NO9(related to ravidomycin but with a molecular formula not found in the Dictionary of Natural Products), (6) Deacetylravidomycin, (7) C30H33NO9 (related to ravidomycin but with a molecular formula not found in the Dictionary of Natural Products), (8) Ravidomycin, (9) C40H57NO10 (molecular formula not found in the Dictionary of Natural Products), (10) Tetronomycin, (11) Salaceyin A and (12) Salaceyin B. Dereplicated components in sample A-256: (1) Bisucaberin B, (2) Cyclo(leucylprolyl), (3) Kahakamide A, (4) Endophenazine D, (5) Dihydroxyphenazine, (6) 1-Hydroxy-6-methoxyphenazine, (7) 1-Phenazinecarboxylic acid, (8) Piperafizine B, (9) 3-Benzylidene-6-(4-methoxybenzylidene)-2,5- piperazinedione, (10) 4′-Methoxyneihumicin or XR 330.
Comparative analysis of Streptomyces and Nocardiopsis metabolites detected with natural product databases led to the identification of a total of 169 compounds detected after LC/MS dereplication in the ethyl acetate extracts of all strains metabolites, 139 were identified in the Dictionary of Natural Products, as shown in Table 4. Concerning the biological activity of identified natural products, the most frequent are antibiotics, with a total of 77 antibacterial and antifungal compounds, and also 32 antitumor or cytotoxic agents, 9 antiparasitic, 5 anti-inflammatory, 5 immunosuppressive, 3 antiviral, 2 insecticidal, 1 neuroprotective, 1 antiarthritic,1 plant hormone, 1 siderophore, 1 photoprotective and other products of diverse pharmacological and biotechnological relevance. Some compounds were only found to be produced by strains belonging to the Nocardiopsis genus, such as the antibacterial and anti-Trypanosoma brucei dihydroxyphenazine (A-256, A-257, A-260); the plant hormone Indol Acetic Acid (strain A-260), the antimicrobial kahakamide A, and the immunosuppressant N-(2-hydroxyphenyl)acetamide (A-257), among others.

Table 4. Identified compounds produced by atmospheric derived Actinobacteria strains and their biological activities.
Of great interest, 30 compounds had molecular formulae determined by HRMS not reported for any molecule included inNatural Products Databases(Supplementary Material 2). These molecules, 28 produced by Streptomyces species and two by Nocardiopsis sp. A-169, deserve further research since they might be new natural products and thus candidates for the discovery of new biologically active substances. Table 5 shows the number of identified compounds, the number of novel molecules produced by each strain, and the results of meteorological analyses to estimate the sources and trajectories of the different air masses that caused the precipitation events, estimated with a 5-day NOAA Hysplit Model (Figure 3). Concerning novel molecules, 20 were produced by strains isolated in the Northern Spain sampling places and 10 by strains isolated in Southern Spain. The air masses of the Southern precipitation event (strains A-258, A-261, A-262, A-266) originate in the Atlantic Ocean. The air masses corresponding to the Northern Spain precipitation events were also sourced in the Atlantic Ocean (strains A-167, A-169, A-249, and A-171), but in some cases (strains A-53, A-254, A-269, A-271) they originate in the Arctic Ocean, and continental America, strain A-87 in United States and strain A-139 in Canada.
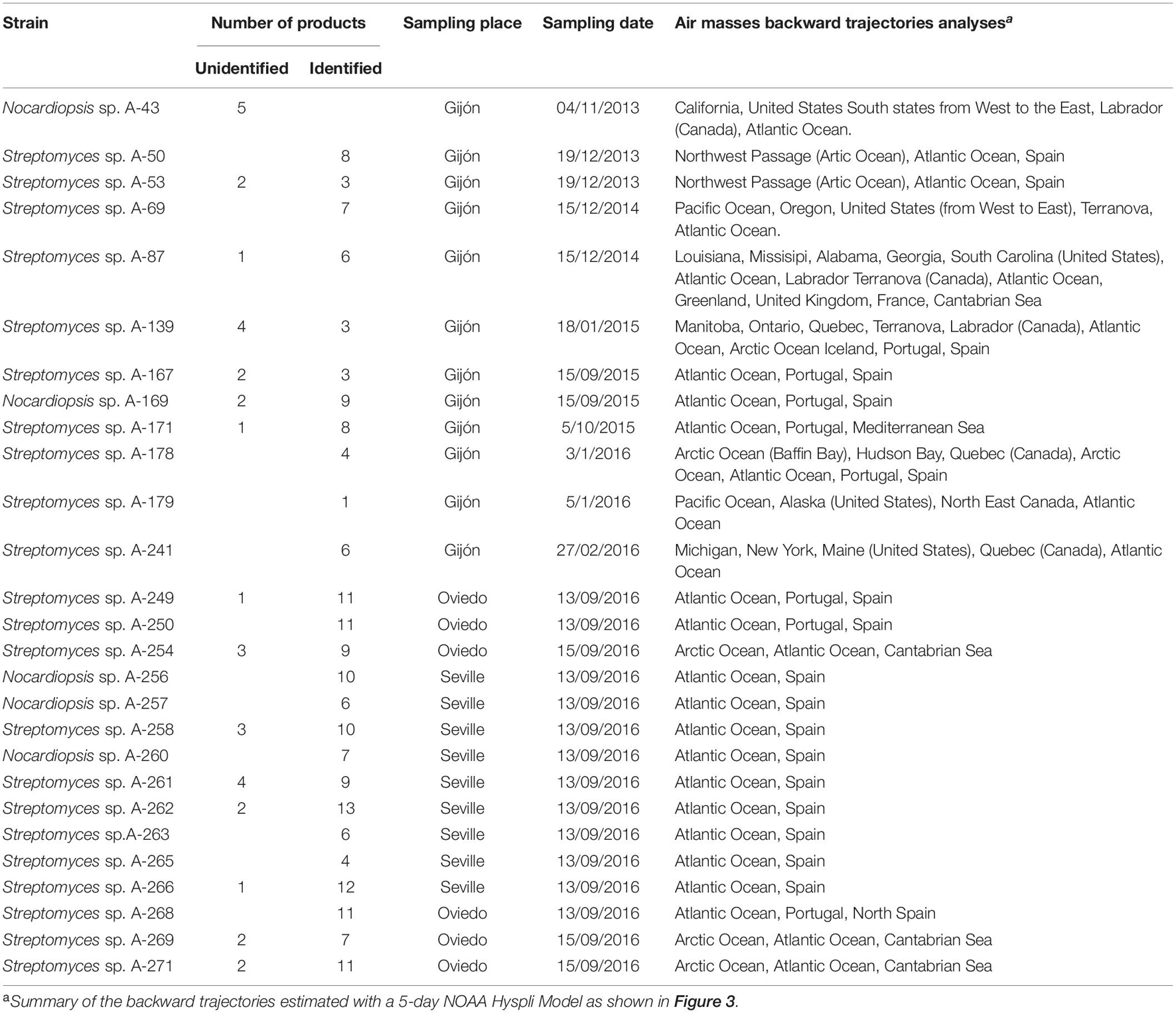
Table 5. Number of compounds and sources of the producing Actinobacteria strains isolated from rainwater precipitations.
Discussion
Exploration of the diversity of Actinobacteria producing biologically active natural products in the atmosphere was herein addressed by sampling multiple precipitation events with prevalent Westerly winds over 4 years in different sampling sites in Spain. Most of the isolates obtained from rainwater samples tolerate high salt concentrations and are homologs of known species isolated from very diverse terrestrial and marine ecosystems throughout the planet, in places as deep as the Mariana Trench sediments (10,898 m depth) in the Pacific Ocean, and as high as the Himalaya Mountains (8,849 m) (Table 2). Taxonomic identification and phylogenetic analyses of the atmospheric-derived Actinobacteria reported here, revealed Streptomyces as the most dominant genus, thus increasing the number of cultivable Streptomyces species able to survive and disperse via the atmosphere. Bioactive members of the rare actinobacterial genus Nocardiopsis were also isolated homologous to two species, Nocardiopsis alba and Nocardiopsis synnemataformans. The global number of Nocardiopsis species described so far on Earth is estimated in 50–53.2
The most relevant feature of the atmospheric Actinobacteria strains studied is that they are producers of multiple chemically diverse secondary metabolites, as analyzed by LC-UV-MS. Ten of the strains produced more than ten compounds each, up to a maximum of 15 (Table 5). From a total of 169 compounds detected after LC/MS dereplication, 82.25% were identified in the Dictionary of Natural Products, whereas, remarkably, the remaining 17.75%, not found in DPN, might be new molecules and deserve further research. After a literature search, 55% of the identified compounds were found to be biologically active as antibiotics (both against Gram-positive and Gram- negative bacteria and against fungi) and 23% have antitumor or cytotoxic activities; compounds with antiparasitic, anti-inflammatory, immunosuppressive, antiviral, insecticidal, neuroprotective, antiarthritic and other diverse biological activities were also detected in the extracts. The number of the compounds produced by these strains is estimated to be much higher than the one presented here, since only diffusible apolar molecules produced in a single culture conditions were analyzed, and possible diffusible polar or volatile molecules were not studied.
Meteorological analyses of the air masses involving 5 days HYSPLIT backward trajectories indicate a main oceanic source from the North Atlantic Ocean and also terrestrial sources from continental North America and Western Europe. In some events even long-range transport from the Pacific and the Arctic Oceans were also estimated. These bacteria remain viable after their atmospheric transport by winds across oceans and continents at planetary level. They could travel downwind and be dispersed via the atmosphere during long periods of time before they fall down to earth by precipitation. These findings provide further support for the Streptomyces atmospheric dispersal cycle (Sarmiento-Vizcaíno et al., 2016), which is herein extended to other members of the phylum Actinobacteria, such as Nocardiopsis genus.
The Streptomyces species herein identified are different from the ones previously isolated in a North-western wind precipitation event, sampled in North Spain and sourced in West Greenland and North Iceland and Canada (Sarmiento-Vizcaíno et al., 2018), thus indicating the relevance of winds in Streptomyces biogeographical distribution. Also, different Nocardiopsis species were isolated in different sampling places, which approximately differ in 6 latitudinal degrees, 37° N in South Spain to 43° N in North Spain sampling place. Latitude has been shown to delineate Streptomyces biogeography patterns in North America terrestrial environments (Choudoir et al., 2016).
Our findings make evident that across time, during different precipitation events, and space, by changing the latitude of the sampling place, we can have access to a striking diversity of Actinobacteria producing an extraordinary reservoir of bioactive natural products from remote and very distant origins, thus highlighting the relevance of the atmosphere as a here and now stablished source for the discovery of novel compounds of relevance in medicine and biotechnology.
Conclusion
Results here obtained on Actinobacteria isolated in rainwater from storm clouds transported by Western winds in Spain highlights the relevance of the atmosphere as a main source of diverse Streptomyces and Nocardiopsis species, and increases our knowledge of the biogeography of these Actinobacteria genera on Earth. Our findings included also an amazing reservoir of bioactive molecules produced by these Actinobacteria, and take another step forward on the potential of atmospheric precipitations for the discovery of natural products active as antibiotic and antitumor agents, among others.
Data Availability Statement
The datasets presented in this study can be found in online repositories. The names of the repository/repositories and accession number(s) can be found in the article/Supplementary Material.
Author Contributions
AS-V and GB isolated the strains. AS-V performed the bioactivity assays, taxonomic identification, and phylogenetic analyses of the strains, and extraction of compounds produced, and analyzed the compounds by LC-UV. GB analyzed the air masses backward trajectories. JM and FR performed the metabolite profiling analysis and identified the compounds produced by LC-MS. LG and GB conceived and coordinated the project. GB wrote the manuscript which has been revised and approved by all authors.
Funding
This study was financially supported by the Universidad de Oviedo (Ayuda PAPI-18-PUENTE-6).
Conflict of Interest
The authors declare that the research was conducted in the absence of any commercial or financial relationships that could be construed as a potential conflict of interest.
Publisher’s Note
All claims expressed in this article are solely those of the authors and do not necessarily represent those of their affiliated organizations, or those of the publisher, the editors and the reviewers. Any product that may be evaluated in this article, or claim that may be made by its manufacturer, is not guaranteed or endorsed by the publisher.
Acknowledgments
We are grateful to José L. Martínez and Daniel Serna (Servicios científico-técnicos, edificio Severo Ochoa, Universidad de Oviedo) for their help in strains identification, and Julia Espadas for providing the Seville sample. This is a contribution of the Asturias Marine Observatory.
Supplementary Material
The Supplementary Material for this article can be found online at: https://www.frontiersin.org/articles/10.3389/fmicb.2021.773095/full#supplementary-material
Supplementary Material 1 | UV210 nm chromatograms corresponding to all samples.
Supplementary Material 2 | Compounds whose molecular formulae was not found in the Dictionary of natural products.
Footnotes
- ^ http://ready.arl.noaa.gov/hypub-bin/trajtype.pl?runtype=archive
- ^ https://www.ncbi.nlm.nih.gov/Taxonomy/Browser/wwwtax.cgi
References
Aizawa, T., Kim, S. Y., Takahashi, S., Koshita, M., Tani, M., Futamura, Y., et al. (2014). Alkyldihydropyrones, new polyketides synthesized by a type III polyketide synthase from Streptomyces reveromyceticus. J. Antibiot. 67, 819–823. doi: 10.1038/ja.2014.80
Akeda, Y., Shibata, K., Ping, X., Tanaka, T., and Taniguchi, M. (1995). AKD-2A, B, C and D, new antibiotics from Streptomyces sp. OCU-42815. Taxonomy, fermentation, isolation, structure elucidation and biological activity. J. Antibiot. 48, 363–368. doi: 10.7164/antibiotics.48.363
Alshaibani, M., Zin, N. M., Jalil, J., Sidik, N., Ahmad, S. J., Kamal, N., et al. (2017). Isolation, Purification, and Characterization of Five Active Diketopiperazine Derivatives from Endophytic Streptomyces SUK 25 with Antimicrobial and Cytotoxic Activities. J. Microbiol. Biotechnol. 27, 1249–1256. doi: 10.4014/jmb.1608.08032
Aoki, Y., Matsumoto, D., Kawaide, H., and Natsume, M. (2011). Physiological role of germicidins in spore germination and hyphal elongation in Streptomyces coelicolor A3(2). J. Antibiot. 64, 607–611.
Arahal, D. R., Sánchez, E., Macián, M. C., and Garay, E. (2008). Value of recN sequences for species identification and as a phylogenetic marker within the family “Leuconostocaceae”. Int. Microbiol. 11, 33–39.
Arteca, R. (1996). Plant Growth Substances: Principles and Applications. New York, NY: Chapman&Hall.
Asahi, K., Nagatsu, J., and Suzuki, S. (1966). Xanthocidin, a new antibiotic. J. Antibiot. 19, 195–199.
Bao, X., Liu, Z., Ni, M., Xia, C., Xu, S., Yang, S., et al. (2020). Synthesis and Assessment of 3-Substituted Phenazines as Novel Antichlamydial Agents. Med. Chem. 16, 413–421. doi: 10.2174/1573406415666190708145639
Bennur, T., Kumar, A. R., Zinjarde, S., and Javdekar, V. (2015). Nocardiopsis species: Incidence, ecological roles and adaptations. Microbiol. Res. 174, 33–47. doi: 10.1016/j.micres.2015.03.010
Bennur, T., Ravi Kumar, A., and Zinjarde, S. S. (2016). JavdekarV. Nocardiopsis species: a potential source of bioactive compounds. J. Appl. Microbiol. 120, 1–16. doi: 10.1111/jam.12950
Berner, I., Konetschny-Rapp, S., Jung, G., and Winkelmann, G. (1988). Characterization of ferrioxamine E as the principal siderophore of Erwinia herbicola (Enterobacter agglomerans). Biol. Met. 1, 51–56. doi: 10.1007/BF01128017
Berrue, F., Ibrahim, A., Boland, P., and Kerr, R. G. (2009). Newly isolated marine Bacillus pumilus (SP21): A source of novel lipoamides and other antimicrobial agents. Pure Appl. Chem. 81, 1027–1031.
Bertasso, M., Holzenkämpfer, M., Zeeck, A., Stackebrandt, E., Beil, W., and Fiedler, H.-P. (2003). Ripromycin and other polycyclic macrolactams from Streptomyces sp. Tü 6239: taxonomy, fermentation, isolation and biological properties. J. Antibiot. 56, 364–371. doi: 10.7164/antibiotics.56.364
Bianchi, G., Dallavalle, S., Merlini, L., Nasini, G., and Quaroni, S. (2003). A new azoxyalkene from a strain of an actinomadura-like fungus. Planta Med. 69, 574–576. doi: 10.1055/s-2003-40633
Blunt, J., and Munro, M. H. G. (2008). Dictionary of marine naturalproducts, with CD-ROM. Boca Raton, FL: Chapman & Hall/CRC Press.
Braña, A. F., Fiedler, H. P., Nava, H., González, V., Sarmiento-Vizcaíno, A., Molina, A., et al. (2015). Two Streptomyces species producing antibiotic, antitumor, and anti-inflammatory compounds are widespread among intertidal macroalgae and deep-sea coral reef invertebrates from the central Cantabrian Sea. Microb. Ecol. 69, 512–524.
Braña, A. F., Sarmiento-Vizcaíno, A., Osset, M., Pérez-Victoria, I., Martín, J., de Pedro, N., et al. (2017a). a New Natural Product with Cytotoxic Activity Produced by Streptomyces sp. M-207 Associated with the Deep-Sea Coral Lophelia pertusa. Mar. Drugs 15:144. doi: 10.3390/md15050144
Braña, A. F., Sarmiento-Vizcaíno, A., Pérez-Victoria, I., Martín, J., Otero, L., Palacios-Gutiérrez, J. J., et al. (2019). Desertomycin G, a New Antibiotic with Activity against Mycobacterium tuberculosis and Human Breast Tumor Cell Lines Produced by Streptomyces althioticus MSM3, Isolated from the Cantabrian Sea Intertidal Macroalgae Ulva sp. Mar. Drugs 17:md17020114. doi: 10.3390/md17020114
Braña, A. F., Sarmiento-Vizcaíno, A., Pérez-Victoria, I., Otero, L., Fernández, J., Palacios, J. J., et al. (2017b). Branimycins B and C, Antibiotics Produced by the Abyssal Actinobacterium Pseudonocardia carboxydivorans M-227. J. Nat. Prod. 80, 569–573. doi: 10.1021/acs.jnatprod.6b01107
Bryans, J., Charlton, P., Chicarelli-Robinson, I., Collins, M., Faint, R., Latham, C., et al. (1996). Inhibition of plasminogen activator inhibitor-1 activity by two diketopiperazines, XR330 and XR334 produced by Streptomyces sp. J. Antibiot. 49, 1014–1021.
Bycroft, B. W., and Payne, D. J. (2013). Dictionary of Antibiotics and Related Substances, 2nd Edn. Boca Raton, FL: CRC Press.
Chan, K. G., Puthucheary, S. D., Chan, X. Y., Yin, W. F., Wong, C. S., Too, W. S., et al. (2011). Quorum sensing in Aeromonas species isolated from patients in Malaysia. Curr. Microbiol. 62, 167–172. doi: 10.1007/s00284-010-9689-z
Chapman & Hall/CRC (2015). Dictionary of Marine Natural Products (DMNP 2014). Boca Raton, FL: Chapman & Hall/CRC.
Che, X. F., Zheng, C. L., Akiyama, S., and Tomoda, A. (2011). 2-Aminophenoxazine-3-one and 2-amino-4,4α-dihydro-4α,7-dimethyl-3H-phenoxazine-3-one cause cellular apoptosis by reducing higher intracellular pH in cancer cells. Proc. Jpn. Acad. Ser. B Phys. Biol. Sci. 87, 199–213.
Chen, Q., Mulzer, M., Shi, P., Beuning, P. J., Coates, G. W., and O’Doherty, G. A. (2011). De novo asymmetric synthesis of fridamycin E. Org. Lett. 13, 6592–6595. doi: 10.1021/ol203041b
Choudoir, M. J., Doroghazi, J. R., and Buckley, D. H. (2016). Latitude delineates patterns of biogeography in terrestrial Streptomyces. Environ. Microbiol. 2016:13420. doi: 10.1111/1462-2920.13420
Cochran, R. E., Ryder, O. S., Grassian, V. H., and Prather, K. A. (2017). Sea Spray Aerosol: The Chemical Link between the Oceans, Atmosphere, and Climate. AccChem. Res. 50, 599–604. doi: 10.1021/acs.accounts.6b00603
Cocito, C. (1979). Antibiotics of the virginiamycin family, inhibitors which contain synergistic components. Microbiol. Rev. 43, 145–192.
Cook, F. D., Edwards, O. E., Gillespie, D. C., and Peterson, E. R. (1971). 1 Hydroxy 6 Methoxy Phenazines. United States Patent 3609153. Alexandria, VA: United States Patent and Trademark Office.
Cui, C. B., Liu, H. B., Gu, J. Y., Gu, Q. Q., Cai, B., Zhang, D. Y., et al. (2007). Echinosporins as new cell cycle inhibitors and apoptosis inducers from marine-derived Streptomyces albogriseolus. Fitoterapia 78, 238–240.
Darshan, N., and Manonmani, H. K. (2015). Prodigiosin and its potentialapplications. J. Food Sci. Technol. 52, 5393–5407.
Dashti, Y., Grkovic, T., Abdelmohsen, U. R., Hentschel, U., and Quinn, R. J. (2014). Production of induced secondary metabolites by a co-culture of sponge-associated actinomycetes, Actinokineospora sp. EG49 and Nocardiopsis sp. RV163. Mar. Drugs 12, 3046–3059. doi: 10.3390/md12053046
de Nys, R., Givskov, M., Kumar, N., Kjelleberg, S., and Steinberg, P. D. (2006). Furanones. Prog. Mol. Subcell Biol. 42, 55–86. doi: 10.1007/3-540-30016-3_2
Ding, Y., Li, Y., Li, Z., Zhang, J., Lu, C., Wang, H., et al. (2016). Alteramide B is a microtubule antagonist of inhibiting Candida albicans. Biochim. Biophys. Acta 1860, 2097–2106. doi: 10.1016/j.bbagen.2016.06.025
Elson, A. L., Box, S. J., and Gilpin, M. L. (1988). New quinone antibiotics of the granaticin type, isolated from Streptomyces lateritius. I. Production, isolation and properties. J. Antibiot. 41, 570–572.
Espinosa, A., Socha, A. M., Ryke, E., and Rowley, D. C. (2012). Antiamoebic properties of the actinomycete metabolites echinomycin A and tirandamycin A. Parasitol. Res. 111, 2473–2477. doi: 10.1007/s00436-012-3019-2
Frändberg, E., Petersson, C., Lundgren, L. N., and Schnürer, J. (2000). Streptomyces halstedii K122 produces the antifungal compounds bafilomycin B1 and C1. Can. J. Microbiol. 46, 753–758.
Fu, P., Wang, S., Hong, K., Li, X., Liu, P., Wang, Y., et al. (2011). Cytotoxic bipyridines from the marine-derived actinomycete Actinoalloteichus cyanogriseus WH1-2216-6. J. Nat. Prod. 74, 1751–1756.
Fukuchi, N., Furihata, K., Nakayama, J., Goudo, T., Takayama, S., Isogai, A., et al. (1995). Rotihibins, novel plant growth regulators from Streptomyces graminofaciens. J. Antibiot. 48, 1004–1010.
Fukushima, K., Yazawa, K., and Arai, T. (1973). Biological activities of albonoursin. J. Antibiot. 26, 175–176. doi: 10.7164/antibiotics.26.175
Garcez, W. S., Martins, D., Garcez, F. R., Marques, M. R., Pereira, A. A., Oliveira, L. A., et al. (2000). Effect of spores of saprophytic fungi on phytoalexin accumulation in seeds of frog-eye leaf spot and stem canker-resistant and -susceptible soybean (Glycine m ax L.) cultivars. J. Agric Food Chem. 48, 3662–3665.
Gebhardt, K., Schimana, J., Krastel, P., Dettner, K., Rheinheimer, J., Zeeck, A., et al. (2002). Endophenazines A-D, new phenazine antibiotics from the arthropod associated endosymbiont Streptomyces anulatus. I. Taxonomy, fermentation, isolation and biological activities. J. Antibiot. 55, 794–800. doi: 10.7164/antibiotics.55.794
Gomes, N. G. M., Pereira, R. B., Andrade, P. B., and Valentão, P. (2019). Double the Chemistry, Double the Fun: Structural Diversity and Biological Activity of Marine-Derived Diketopiperazine Dimers. Mar. Drugs 17:551.
Gómez, C., Olano, C., Palomino-Schätzlein, M., Pineda-Lucena, A., Carbajo, R. J., Braña, A. F., et al. (2012). Novel compounds produced by Streptomyces lydicus NRRL 2433 engineered mutants altered in the biosynthesis of streptolydigin. J. Antibiot. 65, 341–348. doi: 10.1038/ja.2012.37
Goodfellow, M., and Williams, S. T. (1983). Ecology of Actinomycetes. Annu. Rev. Microbiol. 37, 189–216. doi: 10.1146/annurev.mi.37.100183.001201
Grammatikova, N. E., Bibikova, M. V., Spiridonova, I. A., Kabanov, A. E., and Katlinskĭ, A. V. (2003). Streptomyces griseolus # 182–a novel organism producing oligomycin antibiotics. Taxonomy, fermentation, and isolation. Antibiot. Khimioter. 48, 11–15.
Greenstein, M., Monji, T., Yeung, R., Maiese, W. M., and White, R. J. (1986). Light-dependent activity of the antitumor antibiotics ravidomycin and desacetylravidomycin. Antimicrob. Agents Chemother. 29, 861–866. doi: 10.1128/aac.29.5.861
Guo, S., Wang, Y., Wang, W., Hu, H., and Zhang, X. (2020). Identification of new arylamine N-acetyltransferases and enhancing 2-acetamidophenol production in Pseudomonas chlororaphis HT66. Microb. Cell Fact. 19:105. doi: 10.1186/s12934-020-01364-7
Hamedi, J., Mohammadipanah, F., and Ventosa, A. (2013). Systematic and biotechnological aspects of halophilic and halotolerant actinomycetes. Extremophiles 17, 1–13.
Hanka, L. J., Martin, D. G., and Reineke, L. M. (1972). Two new antimetabolites of biotin: alpha-methyldethiobiotin and alpha-methylbiotin. Antimicrob. Agents Chemother. 1, 135–138. doi: 10.1128/aac.1.2.135
Hans, R., Höfle, G., Gerth, K., and Washausen, P. (1997). Heterocyclic compounds obtainable from Sorangium cellulosum bacteria, their preparation process, and agents containing these compounds. Patent WO1997031912A1. Geneva: WIPO.
Harder, P. A., O’Keefe, D. P., Romesser, J. A., Leto, K. J., and Omer, C. A. (1991). Isolation and characterization of Streptomyces griseolus deletion mutants affected in cytochrome P-450-mediated herbicide metabolism. MGG Mol. General Genet. 227:BF00259676. 10.1007/BF00259676
Hashimoto, M., Hayashi, K., Murai, M., Fujii, T., Nishikawa, M., Kiyoto, S., et al. (1992). WS9326A, a novel tachykinin antagonist isolated from Streptomyces violaceusniger no. 9326. II. Biological and pharmacological properties of WS9326A and tetrahydro-WS9326A (FK224). 45, 1064–1070. doi: 10.7164/antibiotics.45.1064
He, Y. W., Wu, J., Cha, J. S., and Zhang, L. H. (2010). Rice bacterial blight pathogen Xanthomonas oryzae pv. oryzae produces multiple DSF-family signals in regulation of virulence factor production. BMC Microbiol. 10:187. doi: 10.1186/1471-2180-10-187
Heidari, B., and Mohammadipanah, F. (2018). Isolation and identification of two alkaloid structures with radical scavenging activity from Actinokineospora sp. UTMC 968, a new promising source of alkaloid compounds. Mol. Biol. Rep. 45, 2325–2332. doi: 10.1007/s11033-018-4395-1
Henkel, T., Breiding-Mack, S., Zeeck, A., Grabley, S., Hammann, P. E., Hutter, K., et al. (1991). Liebigs. Ann. Chem. 1991:575.
Himaja, M., Tesmine Jose, M. V., Ramana, R. A., and Munirajasekhar, D. (2010). Synthesis and biological evaluation of indole-3-carboxylic acid derivatives of amino acids and peptides. Int. Res. J. Pharmacy 1, 436–440.
Hiraoka, S., Miyahara, M., Fujii, K., Machiyama, A., and Iwasaki, W. (2017). Seasonal Analysis of Microbial Communities in Precipitation in the Greater Tokyo Area, Japan. Front. Microbiol. 8:1506. doi: 10.3389/fmicb.2017.01506
Hu, Y., Martinez, E. D., and MacMillan, J. B. (2012). Anthraquinones from a marine-derived Streptomyces spinoverrucosus. J. Nat. Prod. 75, 1759–1764. doi: 10.1021/np3004326
Hussain, A., Rather, M. A., Dar, M. S., Dangroo, N. A., Aga, M. A., Qayum, A., et al. (2018). Streptomyces puniceus strain AS13., Production, characterization and evaluation of bioactive metabolites: A new face of dinactin as an antitumor antibiotic. Microbiol. Res. 207, 196–202. doi: 10.1016/j.micres.2017.12.004
Hwang, S., Le, L. T. H. L., Jo, S. I., Shin, J., Lee, M. J., and Oh, D. C. (2020). Pentaminomycins C-E: Cyclic Pentapeptides as Autophagy Inducers from a Mealworm Beetle Gut Bacterium. Microorganisms 8:1390. doi: 10.3390/microorganisms8091390
Ibrahim, A. H., Desoukey, S. Y., Fouad, M. A., Kamel, M. S., Gulder, T. A. M., and Abdelmohsen, U. R. (2018). Natural Product Potential of the Genus Nocardiopsis. Mar. Drugs 16:E147. doi: 10.3390/md16050147
Igarashi, M., Sasao, C., Yoshida, A., Naganawa, H., Hamada, M., and Takeuchi, T. (1995). Ochracenomicins A, B and C, new benz[a]anthraquinone antibiotics from Amicolatopsis sp. J. Antibiot. 48, 335–337. doi: 10.7164/antibiotics.48.335
Islam, M. T., Laatsch, H., and von Tiedemann, A. (2016). Inhibitory Effects of Macrotetrolides from Streptomyces spp. On Zoosporogenesis and Motility of Peronosporomycete Zoospores Are Likely Linked with Enhanced ATPase Activity in Mitochondria. Front. Microbiol. 7:1824. doi: 10.3389/fmicb.2016.01824
Jawed, H., Shah, S. U., Jamall, S., and Simjee, S. U. (2010). N-(2-hydroxy phenyl) acetamide inhibits inflammation-related cytokines and ROS in adjuvant-induced arthritic (AIA) rats. Int. Immunopharmacol. 10, 900–905. doi: 10.1016/j.intimp.2010.04.028
Jeong, S. Y., Shin, H. J., Kim, T. S., Lee, H. S., Park, S. K., and Kim, H. M. (2006). Streptokordin, a new cytotoxic compound of the methylpyridine class from a marine-derived Streptomyces sp. KORDI-3238. J. Antibiot. 59, 234–240. doi: 10.1038/ja.2006.33
Jizba, J., Přikrylová, V., Ujhelyiova, L., and Varkonda, Š (2008). “Insecticidal properties of nonactic acid and homononactic acid, the precursors of macrotetrolide antibiotics.”. Folia Microbiol. 37, 299–303.
Jizba, J., Sedmera, P., Zima, J., Beran, M., Blumauerová, M., Kandybin, N. V., et al. (1991). Macrotetrolide antibiotics produced by Streptomyces globisporus. Folia Microbiol. 36, 437–443. doi: 10.1007/BF02884062
Jung, D., Yum, S. J., and Jeong, H. G. (2017). Characterization and evaluation of antimicrobial activity of actinonin against foodborne pathogens. Food Sci. Biotechnol. 26, 1649–1657.
Kakeya, H., Morishita, M., Kobinata, K., Osono, M., Ishizuka, M., and Osada, H. (1998). Isolation and biological activity of a novel cytokine modulator, cytoxazone. J. Antibiot. 51, 1126–1128. doi: 10.7164/antibiotics.51.1126
Kamei, H., Oka, M., Hamagishi, Y., Tomita, K., Konishi, M., and Oki, T. (1990). Piperafizines A and B, potentiators of cytotoxicity of vincristine. J. Antibiot. 43, 1018–1020. doi: 10.7164/antibiotics.43.1018
Kanou, M., Ohta, Y., Tanaka, S., Yokoyama, Y., Tanba, M., and Seawa, T. (1998). New compound, its production and anticancer agent, Japanese Kokai Tokkyo Koho (Japanese Patent), JP. 10259174. Tokyo: Japan Patent Office.
Kawasaki, T., Yamada, Y., Maruta, T., Maeda, A., and Hayakawa, Y. (2010). Hatomarubigin E, a biosynthetic intermediate of hatomarubigins C and a substrate of HrbU O-methyltransferase. J. Antibiot. 63, 725–727. doi: 10.1038/ja.2010.118
Keller-Juslén, C., King, H. D., Kuhn, M., Loosli, H. R., Pache, W., Petcher, T. J., et al. (1982). Tetronomycin, a novel polyether of unusual structure. J. Antibiot. 35, 142–150. doi: 10.7164/antibiotics.35.142
Kelly, P., Hadi-Nezhad, F., Liu, D. Y., Lawrence, T. J., Linington, R. G., Ibba, M., et al. (2020). Targeting tRNA-synthetase interactions towards novel therapeutic discovery against eukaryotic pathogens. PLoS Negl. Trop. Dis. 14:e0007983. doi: 10.1371/journal.pntd.0007983
Kharel, M. K., Pahari, P., Shepherd, M. D., Tibrewal, N., Nybo, S. E., Shaaban, K. A., et al. (2012). Angucyclines: Biosynthesis, mode-of-action, new natural products, and synthesis. Nat. Prod. Rep. 29, 264–325. doi: 10.1039/c1np00068c
Kim, N., Shin, J. C., Kim, W., Hwang, B. Y., Hong, Y. S., and Lee, D. (2006). Cytotoxic 6-alkylsalicylic acidsfrom the endophytic Streptomyces laceyi. J. Antibiot. 59, 797–800.
Kim, S.-K. (ed.) (2013). Marine microbiology: bioactive compounds and biotechnological applications. Hoboken, NJ: Wiley.
Kim, Y. B., Kim, Y. H., Park, J. Y., and Kim, S. K. (2004). Synthesis and biological activity of new quinoxaline antibiotics of echinomycin analogues. Bioorg. Med. Chem. Lett. 14, 541–544. doi: 10.1016/j.bmcl.2003.09.086
Kohno, J., Nishio, M., Kawano, K., Nakanishi, N., Suzuki, S., Uchida, T., et al. (1996). TMC-1 A, B, C and D, new antibiotics of the manumycin group produced by Streptomyces sp. Taxonomy, production, isolation, physico-chemical properties, structure elucidation and biological properties. J. Antibiot. 49, 1212–1220. doi: 10.7164/antibiotics.49.1212
Kumar, S. N., Nambisan, B., Sundaresan, A., Mohandas, C., and Anto, R. J. (2014). Isolation and identification of antimicrobial secondary metabolites from Bacillus cereus associated with a rhabditid entomopathogenic nematode. Ann. Microbiol. 64, 209–218. doi: 10.1007/s13213-013-0653-6
Labeda, D. P., Doroghazi, J. R., Ju, K. S., and Metcalf, W. W. (2014). Taxonomic evaluation of Streptomyces albus and related species using multilocus sequence analysis and proposals to emend the description of Streptomyces albus and describe Streptomyces pathocidini sp. nov. Int. J. Syst. Evol. Microbiol. 64(Pt 3), 894–900. doi: 10.1099/ijs.0.058107-0
Lam, Y. K., Bogen, D., Chang, R. S., Faust, K. A., Hensens, O. D., Zink, D. L., et al. (1991). Novel and potent gastrin and brain cholecystokinin antagonists from Streptomyces olivaceus. Taxonomy, fermentation, isolation, chemical conversions, and physico-chemical and biochemical properties. J. Antibiot. 44, 613–625.
Laursen, J. B., and Nielsen, J. (2004). Phenazine natural products: biosynthesis, synthetic analogues, and biological activity. Chem. Rev. 104, 1663–1686. doi: 10.1021/cr020473j
Liu, C., Wang, X., Yan, Y., Wang, J., Zhang, B., Zhang, J., et al. (2013). Streptomyces heilongjiangensis sp. nov., a novel actinomycete that produces borrelidin isolated from the root surface of soybean [Glycine max (L.) Merr]. Int. J. Syst. Evol. Microbiol. 63(Pt 3), 1030–1036. doi: 10.1099/ijs.0.041483-0
Lu, C. H., Li, Y. Y., Wang, H. X., Wang, B. M., and Shen, Y. M. (2013). A new phenoxazine derivative isolated from marine sediment actinomycetes, Nocardiopsis sp. 236. Drug Discov. Ther. 7, 101–104.
Lu, H., Zhou, X., Wang, L., and Jin, L. (2020). Synthesis and Antibacterial Evaluation of N-phenylacetamide Derivatives Containing 4-arylthiazole Moieties. Molecules 25:1772. doi: 10.3390/molecules25081772
Luzhetskyy, A., Hoffmann, J., Pelzer, S., Wohlert, S. E., Vente, A., and Bechthold, A. (2008). Aranciamycin analogs generated by combinatorial biosynthesis show improved antitumor activity. Appl. Microbiol. Biotechnol. 80, 15–19. doi: 10.1007/s00253-008-1515-1
Ma, J., Cao, B., Liu, C., Guan, P., Mu, Y., Jiang, Y., et al. (2018). Actinofuranones D-I from a Lichen-Associated Actinomycetes, Streptomyces gramineus, and Their Anti-Inflammatory Effects. Molecules 23:2393. doi: 10.3390/molecules23092393
Ma, J., Huang, H., Xie, Y., Liu, Z., Zhao, J., Zhang, C., et al. (2017). Biosynthesis of ilamycins featuring unusual building blocks and engineered production of enhanced anti-tuberculosis agents. Nat. Commun. 8:391. doi: 10.1038/s41467-017-00419-5
Managamuri, U., Vijayalakshmi, M., Ganduri, V. S. R. K., Rajulapati, S. B., Bonigala, B., Kalyani, B. S., et al. (2017). Isolation, identification, optimization, and metabolite profiling of Streptomyces sparsus VSM-30. 3 Biotech. 7:217. doi: 10.1007/s13205-017-0835-1
Maruyama, H. B., Suhara, Y., Suzuki-Watanabe, J., Maeshima, Y., and Shimizu, N. A. (1975). new antibiotic, fumaramidmycin I. Production, biological properties and characterization of producer strain. J. Antibiot. 28, 636–647.
Meyer, C. E. (1971). Tirandamycin, a new antibiotic isolation and characterization. J. Antibiot. 24, 558–560. doi: 10.7164/antibiotics.24.558
Meyers, E., Pansy, F. E., Perlman, D., Smith, D. A., and Weisenborn, F. L. (1965). The in vitro activity of Nonactin and its homologs: Monactin, Dinactin and Trinactin. J. Antibiot. 18, 128–129.
Michaud, J. M., Thompson, L. R., Kaul, D., Espinoza, J. L., Richter, R. A., Xu, Z. Z., et al. (2018). Taxon-specific aerosolization of bacteria and viruses in an experimental ocean-atmosphere mesocosm. Nat. Commun. 9:2017. doi: 10.1038/s41467-018-04409
Miyata, S., Hashimoto, M., Masui, Y., Ezaki, M., Takase, S., Nishikawa, M., et al. (1992). WS-7338, new endothelin receptor antagonists isolated from Streptomyces sp. No. 7338. I. Taxonomy, fermentation, isolation, physico-chemical properties and biological activities. J. Antibiot. 45, 74–82. doi: 10.7164/antibiotics.45.74
Moree, W. J., McConnell, O. J., Nguyen, D. D., Sanchez, L. M., Yang, Y. L., Zhao, X., et al. (2014). Microbiota of healthy corals are active against fungi in a light-dependent manner. ACS Chem. Biol. 9, 2300–2308. doi: 10.1021/cb500432j
Nagahama, N., Suzuki, M., Awataguchi, S., and Okuda, T. (1967). Studies on a new antibiotic, albocycline. I. Isolation, purification and properties. J. Antibiot. 20, 261–266.
Nakayama, M., Takahashi, Y., Itoh, H., Kamiya, K., Shiratsuchi, M., and Otani, G. (1989). Novel antifungal antibiotics maniwamycins A and B. I. Taxonomy of the producing organism, fermentation, isolation, physico-chemical properties and biological properties. J. Antibiot. 42, 1535–1540. doi: 10.7164/antibiotics.42.1535
Okamoto, M., Yoshida, K., Nishikawa, M., Ando, T., Iwami, M., Kohsaka, M., et al. (1986). FR-900452, a specific antagonist of platelet activating factor (PAF) produced by Streptomyces phaeofaciens. I. Taxonomy, fermentation, isolation, and physico-chemical and biological characteristics. J. Antibiot. 39, 198–204.
Omura, S., Nakagawa, A., Fukamachi, N., Otoguro, K., and Kobayashi, B. (1986). Aggreceride, a new platelet aggregation inhibitor from Streptomyces. J. Antibiot. 39, 1180–1181. doi: 10.7164/antibiotics.39.1180
Ortiz-López, F. J., Alcalde, E., Sarmiento-Vizcaíno, A., Díaz, C., Cautain, B., García, L. A., et al. (2018). New 3-Hydroxyquinaldic Acid Derivatives from Cultures of the Marine Derived Actinomycete Streptomyces cyaneofuscatus M-157. Mar. Drugs 16:371. doi: 10.3390/md16100371
Pan, T., He, H., Li, C., Zhao, J., Zhang, Y., Li, J., et al. (2016). Streptomyces daqingensis sp. nov., isolated from saline-alkaline soil. Int. J. Syst. Evol. Microbiol. 66, 1358–1363. doi: 10.1099/ijsem.0.000887
Park, C. N., Lee, D., Kim, W., Hong, Y., Ahn, J. S., and Kim, B. S. (2007). Antifungal activity of salaceyin A against Colletotrichum orbiculare and Phytophthora capsici. J. Basic Microbiol. 47, 332–339.
Park, H. B., Lee, J. K., Lee, K. R., and Kwon, H. C. (2014). Angumycinones A and B, two new angucyclic quinones from Streptomyces sp. KMC004 isolated from acidic mine drainage. Tetrahed. Lett. 55, 63–66.
Paściak, M., Pawlik, K., Gamian, A., Szponar, B., Skóra, J., and Gutarowska, B. (2014). An airborne actinobacteriaNocardiopsisalba isolated from bioaerosol of a mushroom compost facility. Aerobiologia 30, 413–422.
Patel, N. M., Moore, J. D., Blackwell, H. E., and Amador-Noguez, D. (2016). Identification of Unanticipated and Novel N-Acyl L-Homoserine Lactones (AHLs) Using a Sensitive Non-Targeted LC-MS/MS Method. PLoS One 11:e0163469. doi: 10.1371/journal.pone.0163469
Pathom-Aree, W., Stach, J. E., Ward, A. C., Horikoshi, K., Bull, A. T., and Goodfellow, M. (2006). Diversity of actinomycetes isolated from Challenger Deep sediment (10,898 m) from the Mariana Trench. Extremophiles 10, 181–189. doi: 10.1007/s00792-005-0482-z
Paul, V. J., Frautschy, S., Fenical, W., and Nealson, K. H. (1981). Antibiotics in microbial ecology : Isolation and structure assignment of several new antibacterial compounds from the insect-symbiotic bacteriaXenorhabdus spp. J. Chem. Ecol. 7, 589–597. doi: 10.1007/BF00987707
Pérez-Victoria, I., Martín, J., and Reyes, F. (2016). Combined LC/UV/MS and NMR Strategies for the Dereplication of Marine Natural Products. Planta Med. 82, 857–871.
Petrović, S., Vasić, V., Mitrović, T., Lazović, S., and Leskovac, A. (2017). The impact of concentration and administration time on the radiomodulating properties of undecylprodigiosin in vitro. Arh. Hig. Rada Toksikol. 68, 1–8. doi: 10.1515/aiht-2017-68-289
Proschak, A., Zhou, Q., Schöner, T., Thanwisai, A., Kresovic, D., Dowling, A., et al. (2014). Biosynthesis of the insecticidal xenocyloins in Xenorhabdus bovienii. Chembiochem 15, 369–372. doi: 10.1002/cbic.201300694
Qiao, J., Chen, L., Li, Y., Wang, J., Zhang, W., and Chen, S. (2012). Whole-genome sequence of Nocardiopsis alba strain ATCC BAA-2165, associated with honeybees. J. Bacteriol. 194, 6358–6359. doi: 10.1128/JB.01522-12
Rather, S. A., Kumar, S., Rah, B., Arif, M., Ali, A., and Qazi, P. (2013). A potent cytotoxic metabolite from terrestrial actinomycete, Streptomyces collinus. Med. Chem. Res. 23, 382–387. doi: 10.1007/s00044-013-0640-2
Rodríguez, V., Martín, J., Sarmiento-Vizcaíno, A., de la Cruz, M., García, L. A., Blanco, G., et al. (2018). Anthracimycin B, a Potent Antibiotic against Gram-Positive Bacteria Isolated from Cultures of the Deep-Sea Actinomycete Streptomyces cyaneofuscatus M-169. Mar. Drugs 16:406. doi: 10.3390/md16110406
Russell, D. W., and Sambrook, J. F. (2001). Molecular Cloning: A Laboratory Manual, 3rd Edn. New York, NY: Cold Spring Harbor Laboratory Press.
Sánchez-Suárez, J., Coy-Barrera, E., Villamil, L., and Díaz, L. (2020). Streptomyces-Derived Metabolites with Potential Photoprotective Properties-A Systematic Literature Review and Meta-Analysis on the Reported Chemodiversity. Molecules 25:3221. doi: 10.3390/molecules25143221
Santos, O. C. S., Soares, A. R., Machado, F. L. S., Romanos, M. T. V., Muricy, G., Giambiagi-deMarval, M., et al. (2015). Investigation of biotechnological potential of sponge-associated bacteria collected in Brazilian coast. Lett. Appl. Microbiol. 60, 140–147. doi: 10.1111/lam.12347
Santos, J. D., Vitorino, I., de la Cruz, M., Díaz, C., Cautain, B., Annang, F., et al. (2020). Diketopiperazines and other bioactive compounds from bacterial symbionts of marine sponges. Antonie Van Leeuwenhoek 113, 875–887. doi: 10.1007/s10482-020-01398-2
Sarmiento-Vizcaíno, A., Braña, A. F., González, V., Nava, H., Molina, A., Llera, E., et al. (2016). Atmospheric Dispersal of Bioactive Streptomyces albidoflavus Strains Among Terrestrial and Marine Environments. Microb. Ecol. 71, 375–386. doi: 10.1007/s00248-015-0654-z
Sarmiento-Vizcaíno, A., González, V., Braña, A. F., Palacios, J. J., Otero, L., Fernández, J., et al. (2017b). Pharmacological Potential of Phylogenetically Diverse Actinobacteria Isolated from Deep-Sea Coral Ecosystems of the Submarine Avilés Canyon in the Cantabrian Sea. Microb. Ecol. 73, 338–352. doi: 10.1007/s00248-016-0845-2
Sarmiento-Vizcaíno, A., Braña, A. F., Pérez-Victoria, I., Martín, J., de Pedro, N., Cruz, M., et al. (2017a). Paulomycin G, a New Natural Product with Cytotoxic Activity against Tumor Cell Lines Produced by Deep-Sea Sediment Derived Micromonospora matsumotoense M-412 from the Avilés Canyon in the Cantabrian Sea. Mar. Drugs 15:271. doi: 10.3390/md15090271
Sarmiento-Vizcaíno, A., Espadas, J., Martín, J., Braña, A. F., Reyes, F., García, L. A., et al. (2018). Atmospheric Precipitations, Hailstone and Rainwater, as a Novel Source of Streptomyces Producing Bioactive Natural Products. Front. Microbiol. 9:773. doi: 10.3389/fmicb.2018.00773
Sarmiento-Vizcaíno, A., González, V., Braña, A. F., Molina, A., Acuña, J. L., García, L. A., et al. (2015). Myceligenerans cantabricum sp. nov., a barotolerant actinobacterium isolated from a deep cold-water coral. Int. J. Syst. Evol. Microbiol. 65(Pt 4), 1328–1334. doi: 10.1099/ijs.0.000107
Savic, M., Bratic, I., and Vasiljevic, B. (2007). Streptomyces durmitorensis sp. nov., a producer of an FK506-like immunosuppressant. Int. J. Syst. Evol. Microbiol. 57(Pt 9), 2119–2124.
Schrey, S. D., Erkenbrack, E., Früh, E., Fengler, S., Hommel, K., Horlacher, N., et al. (2012). Production of fungal and bacterial growth modulating secondary metabolites is widespread among mycorrhiza-associated streptomycetes. BMC Microbiol. 12:164. doi: 10.1186/1471-2180-12-164
Schleissner, C., Pérez, M., Losada, A., Rodríguez, P., Crespo, C., Zúñiga, P., et al. (2011). Antitumor actinopyranones produced by Streptomyces albus POR04-15-053 isolated from a marine sediment. J. Nat. Prod. 74, 1590–1596. doi: 10.1021/np200196j
Schumacher, R. W., Harrigan, B. L., and Davidson, B. S. (2001). Kahakamides A and B, new neosidomycin metabolites from a marine-derived actinomycete. Tetrahedron Lett. 42, 5133–5135.
Secor, P. R., Jennings, L. K., James, G. A., Kirker, K. R., Pulcini, E. D., McInnerney, K., et al. (2012). Phevalin (aureusimine B) production by Staphylococcus aureus biofilm and impacts on human keratinocyte gene expression. PLoS One 7:e40973. doi: 10.1371/journal.pone.0040973
Sehgal, S. N., Czerkawski, H., Kudelski, A., Pandev, K., Saucier, R., and Vézina, C. (1983). Ravidomycin (AY-25,545), a new antitumor antibiotic. J. Antibiot. 36, 355–361. doi: 10.7164/antibiotics.36.355
Shigemori, H., Myung, A. B., Kazunaga, Y., Takuma, S., and Junichi, K. (1992). Alteramide A, a new tetracyclic alkaloid from a bacterium Alteromonas sp. associated with the marine sponge Halichondria okadai. J. Organic Chem. 57, 4317–4320. doi: 10.1021/jo00041a053
Shimizu, S., Suzuki, M., Tomoda, A., Arai, S., Taguchi, H., Hanawa, T., et al. (2004). Phenoxazine compounds produced by the reactions with bovine hemoglobin show antimicrobial activity against non-tuberculosis mycobacteria. Tohoku J. Exp. Med. 203, 47–52.
Shirling, E. B., and Gottlieb, D. (1968). Cooperative description of type cultures of Streptomyces. II. Species descriptions from first study. Int. J. Syst. Bacteriol. 18, 69–189.
Siegel, M. R., Sisler, H. D., and Johnson, F. (1966). Relationship of structure to fungitoxicity of cycloheximide and related glutarimide derivatives. Biochem. Pharmacol. 15, 1213–1223. doi: 10.1016/0006-2952(66)90286-3
Silva, L. J., Crevelin, E. J., Souza, W. R., Moraes, L. A., Melo, I. S., and Zucchi, T. D. (2014). Streptomyces araujoniae Produces a Multiantibiotic Complex with Ionophoric Properties to Control Botrytis cinerea. Phytopathology 104, 1298–1305. doi: 10.1094/PHYTO-11-13-0327-R
Singh, L. S., Sharma, H., and Talukdar, N. C. (2014). Production of potent antimicrobial agent by actinomycete, Streptomyces sannanensis strain SU118 isolated from phoomdi in Loktak Lake of Manipur, India. BMC Microbiol. 14:278. doi: 10.1186/s12866-014-0278-3
Songia, S., Mortellaro, A., Taverna, S., Fornasiero, C., Scheiber, E. A., Erba, E., et al. (1997). Characterization of the new immunosuppressive drug undecylprodigiosin in human lymphocytes: retinoblastoma protein, cyclin-dependent kinase-2, and cyclin-dependent kinase-4 as molecular targets. J. Immunol. Baltim. 158, 3987–3995.
Stadler, M., Bauch, F., Henkel, T., Mühlbauer, A., Müller, H., Spaltmann, F., et al. (2001). Antifungal actinomycete metabolites discovered in a differential cell-based screening using a recombinant TOPO1 deletion mutant strain. Arch. Pharm. 334, 143–147.
Stankovic, N., Senerovic, L., Ilic-Tomic, T., Vasiljevic, B., and Nikodinovic-Runic, J. (2014). Properties and applications of undecylprodigiosin and other bacterial prodigiosins. Appl. Microbiol. Biotechnol. 98, 3841–3858.
Stein, A. F., Draxler, R. R., Rolph, G. D., Stunder, B. J. B., Cohen, M. D., and Ngan, F. (2015). NOAA’s HYSPLIT Atmospheric Transport and Dispersion Modeling System. Bull. Am. Meteorol. Soc. 96, 2059–2077. doi: 10.1175/BAMS-D-14-00110.1
Sullia, S. B., and Griffin, D. H. (1977). Inhibition of DNA synthesis by cycloheximide and blasticidin-S is independent of their effect on protein synthesis. Biochim. Biophys. Acta 475, 14–22. doi: 10.1016/0005-2787(77)90334-3
Tadtong, S., Meksuriyen, D., Tanasupawat, S., Isobe, M., and Suwanborirux, K. (2007). Geldanamycin derivatives and neuroprotective effect on cultured P19-derived neurons. Bioorg. Med. Chem. Lett. 17, 2939–2943.
Taechowisan, T., Wanbanjob, A., Tuntiwachwuttikul, P., and Liu, J. (2010). Anti-inflammatory effects of lansai C and D cause inhibition of STAT-1 and NF-κB activations in LPS-induced RAW 264. 7 Cells Food Agricult. Immunol. 21, 57–64.
Tan, X. Y., Wang, X., Liu, Q. S., Xie, X. Q., Li, Y., Li, B. Q., et al. (2018). Inhibition of silkworm vacuolar-type ATPase activity by its inhibitor Bafilomycin A1 induces caspase-dependent apoptosis in an embryonic cell line of silkworm. Arch. Insect Biochem. Physiol. 99:e21507. doi: 10.1002/arch.21507
Tang, Y. Q., Sattler, I., Thiericke, R., Grabley, S., and Feng, X. Z. (2000). Feigrisolides A, B, C and D, new lactones with antibacterial activities from Streptomyces griseus. J. Antibiot. 53, 934–943. doi: 10.7164/antibiotics.53.934
Tanouchi, Y., and Shichi, H. (1987). Immunosuppressive effects of polynactins (tetranactin, trinactin and dinactin) on experimental autoimmune uveoretinitis in rats. Jpn. J. Ophthalmol. 31, 218–229.
Tanouchi, Y., and Shichi, H. (1988). Immunosuppressive and anti-proliferative effects of a macrotetrolide antibiotic, tetranactin. Immunology 63, 471–475.
Thongchai, T., Asawin, W., Pittaya, T., and Jikai, L. (2010). Anti-inflammatory effects of lansai C and D cause inhibition of STAT-1 and NF-κB activations in LPS-induced RAW 264. 7 Cells Food Agricult. Immunol. 21, 57–64. doi: 10.1080/09540100903419592
Ubukata, M., Osada, H., Kudo, T., and Isono, K. (1993). Respinomycins A1, A2 B, C and D, a novel group of anthracycline antibiotics. I. Taxonomy, fermentation, isolation and biological activities. J. Antibiot. 46, 936–941. doi: 10.7164/antibiotics.46.936
Umland, S. P., Shah, H., Jakway, J. P., Shortall, J., Razac, S., Garlisi, C. G., et al. (1999). Effects of cyclosporin A and dinactin on T-cell proliferation, interleukin-5 production, and murine pulmonary inflammation. Am. J. Respir. Cell Mol. Biol. 20, 481–492. doi: 10.1165/ajrcmb.20.3.3266
Vaden, R. M., Oswald, N. W., Potts, M. B., MacMillan, J. B., and White, M. A. (2017). FUSION-Guided Hypothesis Development Leads to the Identification of N6,N6-Dimethyladenosine, a Marine-Derived AKT Pathway Inhibitor. Mar. Drugs 15:75. doi: 10.3390/md15030075
Vijaya Kumar, E. K., Kenia, J., Mukhopadhyay, T., and Nadkarni, S. R. (1999). Methylsulfomycin I, a new cyclic peptide antibiotic from a Streptomyces sp. HIL Y-9420704. J. Nat. Prod. 62, 1562–1564. doi: 10.1021/np990088y
Vu, H. T., Nguyen, D. T., Nguyen, H. Q., Chu, H. H., Chu, S. K., Chau, M. V., et al. (2018). Antimicrobial and Cytotoxic Properties of Bioactive Metabolites Produced by Streptomyces cavourensis YBQ59 Isolated from Cinnamomum cassia Prels in Yen Bai Province of Vietnam. Curr. Microbiol. 75, 1247–1255. doi: 10.1007/s00284-018-1517-x
Wang, D., Wang, C., Gui, P., Liu, H., Khalaf, S. M. H., Elsayed, E. A., et al. (2017). Identification, Bioactivity, and Productivity of Actinomycins from the Marine-Derived Streptomyces heliomycini. Front. Microbiol. 8:1147. doi: 10.3389/fmicb.2017.01147
Wang, X. M., Wang, X., Dong, M., Li, Z. M., Liu, Z. X., et al. (2020). Synthesis and biological activities of 1H-indole-1-carboxylic acid aryl esters as a marine antifouling coating. J. Coat Technol. Res. 17, 553–561
Welander Paula, V., Ryan, C. H., Lichun, Z., Alex, L. S., Roger, E. S., and Dianne, K. N. (2009). Hopanoids Play a Role in Membrane Integrity and pH Homeostasis in Rhodopseudomonas palustris TIE-1. J. Bacteriol. 191, 6145–6156. doi: 10.1128/JB.00460-09
Williamson, N. R., Fineran, P. C., Leeper, F. J., and Salmond, G. P. C. (2006). The biosynthesis and regulation of bacterial prodiginines. Nat. Rev. Microbiol. 4, 887–899. doi: 10.1038/nrmicro1531
Wu, C. Z., Jang, J. H., Ahn, J. S., and Hong, Y. S. (2012). New geldanamycin analogs from Streptomyces hygroscopicus. J. Microbiol. Biotechnol. 22, 1478–1481. doi: 10.4014/jmb.1206.06026
Xu, J., and Yang, Q. (2010). Isolation and characterization of rice straw degrading Streptomyces griseorubens C-5. Biodegradation 21, 107–116. doi: 10.1007/s10532-009-9285-8
Ye, L., Zhang, H., Xu, H., Zou, Q., Cheng, C., Dong, D., et al. (2010). Phenazine-1-carboxylic acid derivatives: design, synthesis and biological evaluation against Rhizoctonia solani Kuhn. Bioorg. Med. Chem. Lett. 20, 7369–7371. doi: 10.1016/j.bmcl.2010.10.050
Keywords: Streptomyces, Nocardiopsis, actinomycetes, antibiotic, antimicrobial, antitumor
Citation: Sarmiento-Vizcaíno A, Martín J, Reyes F, García LA and Blanco G (2021) Bioactive Natural Products in Actinobacteria Isolated in Rainwater From Storm Clouds Transported by Western Winds in Spain. Front. Microbiol. 12:773095. doi: 10.3389/fmicb.2021.773095
Received: 09 September 2021; Accepted: 14 October 2021;
Published: 10 November 2021.
Edited by:
Pierre Amato, UMR 6296 Institut de Chimie de Clermont-Ferrand (ICCF), FranceReviewed by:
Loh Teng Hern Tan, Monash University Malaysia, MalaysiaMaria King, Texas A&M University, United States
Copyright © 2021 Sarmiento-Vizcaíno, Martín, Reyes, García and Blanco. This is an open-access article distributed under the terms of the Creative Commons Attribution License (CC BY). The use, distribution or reproduction in other forums is permitted, provided the original author(s) and the copyright owner(s) are credited and that the original publication in this journal is cited, in accordance with accepted academic practice. No use, distribution or reproduction is permitted which does not comply with these terms.
*Correspondence: Gloria Blanco, gbb@uniovi.es