- 1Department of Microbiology and Genetics, Edificio Departamental, University of Salamanca, Salamanca, Spain
- 2Ecosystems and Environment Research Programme, Faculty of Biological and Environmental Sciences, Finland and Helsinki Institute of Sustainability Science, University of Helsinki, Helsinki, Finland
- 3Joint Laboratory of Applied Ecotoxicology, Korea Institute of Science and Technology Europe, Saarbrücken, Germany
- 4University of Manitoba, Clayton H. Riddell Faculty of Environment, Earth, and Resources, Winnipeg, MB, Canada
- 5Instituto Politécnico Nacional, Departamento de Microbiología, Escuela Nacional de Ciencias Biológicas, Mexico City, Mexico
- 6Environmental Microbiology Group, Institute of Water Research, University of Granada, Granada, Spain
- 7Department of Microbiology, Faculty of Pharmacy, University of Granada, Granada, Spain
The presence of emerging contaminants in the environment, such as pharmaceuticals, is a growing global concern. The excessive use of medication globally, together with the recalcitrance of pharmaceuticals in traditional wastewater treatment systems, has caused these compounds to present a severe environmental problem. In recent years, the increase in their availability, access and use of drugs has caused concentrations in water bodies to rise substantially. Considered as emerging contaminants, pharmaceuticals represent a challenge in the field of environmental remediation; therefore, alternative add-on systems for traditional wastewater treatment plants are continuously being developed to mitigate their impact and reduce their effects on the environment and human health. In this review, we describe the current status and impact of pharmaceutical compounds as emerging contaminants, focusing on their presence in water bodies, and analyzing the development of bioremediation systems, especially mycoremediation, for the removal of these pharmaceutical compounds with a special focus on fungal technologies.
Introduction
In recent decades, the production and consumption of pharmaceutical products have rapidly increased with the development of medicine. Approximately 3,000 compounds are used as pharmaceuticals, and the annual production quantity exceeds hundreds of tons (Carvalho and Santos, 2016; Grenni et al., 2018). Anti-inflammatory drugs, antibiotics, and analgesics are the most common drugs used around the world. Consequently, the emergence of water-soluble and pharmacologically active organic micropollutants or pharmaceutical active compounds (PhACs) has gained much attention worldwide. Humans use a variety of these pharmaceuticals for their health in everyday life, but large quantities of these drugs are also used as veterinary medicine on farms around the world, to prevent and treat animal diseases and to increase economic benefits in intensive livestock (Blanco et al., 2017; Ekpeghere et al., 2017; Gros et al., 2019; Ramírez-Morales et al., 2021).
After ingestion, pharmaceuticals are excreted in urine and feces as active substances or metabolites (Sui et al., 2015; aus der Beek et al., 2016). These pharmaceuticals are present in both influent and effluent wastewater but can also be found in surface water bodies, including freshwater ecosystems and marine environments, as well as in groundwater due to effluent leachates generated under recharge conditions (Deo, 2014; Furlong et al., 2017; Ojemaye and Petrik, 2018; Reis-Santos et al., 2018; Fekadu et al., 2019; Letsinger et al., 2019; Zainab et al., 2020). The main concern is that conventional treatment plants are ineffective in removing some of these emerging contaminants (ECs), and new techniques are being sought and studied to achieve their total elimination, particularly advances in mycoremediation (Danner et al., 2019). The importance of the study of pharmaceuticals lies in the massive increase in their consumption worldwide, as well as in the environmental repercussions that this entails, including their recalcitrance in aquatic and terrestrial ecosystems. In the contexts of wastewater and bioremediation, pharmaceutical compounds are considered as ECs due to the lack of regulation for their environmental disposal, as well as the lack of information regarding their long-term effects on the environment (Dhangar and Kumar, 2020; Valdez-Carrillo et al., 2020; Chaturvedi et al., 2021b; Rathi et al., 2021), which remains unknown (Barber et al., 2015; Ahmed et al., 2017). The fact that some drugs are marketed without medical prescription or pre-registration and, therefore, are widely consumed worldwide, meaning that they are widely distributed in the environment (Gil et al., 2017), has contributed to this growing problem.
Considering pharmaceuticals as ECs and the continual production of new PhACs, this review aims to comprehensively present the pharmaceuticals commonly detected in water, surface and groundwater and their adverse environmental effects. Advances in bioremediation technologies, which can be used as add-on treatments in wastewater treatment plants (WWTPs) to reduce unprocessed pharmaceuticals released via effluent into the environment, are presented and critically discussed with an emphasis on mycoremediation.
Common Pharmaceuticals Detected in Water (Surface and Groundwater)
Pharmaceutical compounds that reach water bodies, both surface water and groundwater, came from a number of different sources (Figure 1). The first of these is urban wastewater, which contains a high load of pharmaceuticals from human excrement, and also the inadequate disposal of expired or unused drugs due to the scarce control in their management. Another major source of pharmaceuticals is agricultural and livestock waste, especially the latter, since in large farms for intensive livestock, animals are often fed with feed supplemented containing drugs and excreta are often used in agriculture as soil amendments, reaching groundwater by leaching (Kim et al., 2008; Barrios-Estrada et al., 2018). Effluents from the pharmaceutical industry are another important source, with high concentrations of pharmaceuticals being found due to discharges from factories in Asia, Europe and America, despite strict regulation of pharmaceutical production in Europe and the United States (Lin et al., 2008; Lin and Tsai, 2009; Phillips et al., 2010; Prasse et al., 2010; Sim et al., 2011; Cardoso et al., 2014). These industries are obliged to carry out treatment before discharge into the general urban sewer network (Lindberg et al., 2004; Brown et al., 2006).
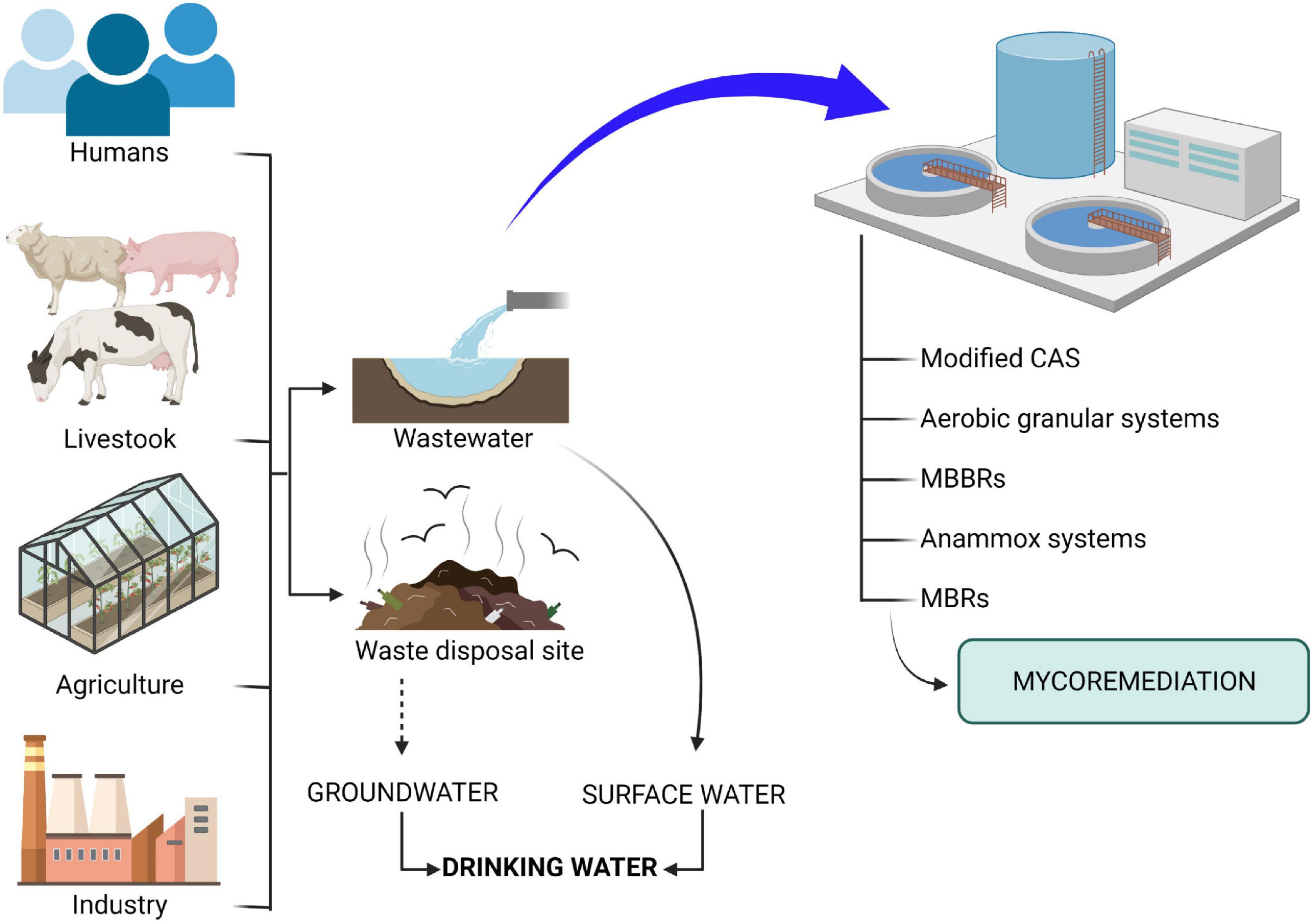
Figure 1. Pharmaceuticals route to a body of water and bioremediation technologies. (→): Direct contamination. (⇢): Contamination through different steps. The monitoring suggests that contamination accumulates in surface water and groundwater.
Pharmaceuticals found in high concentrations in wastewater include non-steroidal anti-inflammatory drugs (NSAIDs), β-blockers ad psychoactive compounds, analgesics, antibiotics, endocrine disruptors, antiretroviral drugs, and drugs to treat cancer (Roberts and Thomas, 2006; Gros et al., 2010; Lian et al., 2017). These are the PhACs most commonly detected due to the analytical methods available and their resolution, although new methods for identifying these compounds are increasingly being developed (Pivetta et al., 2020; Zhang et al., 2020). Table 1 shows the worldwide distribution of the drugs most commonly found in water (Supplementary Figure 1).
Non-steroidal anti-inflammatory drugs and analgesics are some of the most important groups of pharmaceutical products worldwide, with diverse chemical structures and similar therapeutic effects, having an estimated annual production of several hundred tons (Comber et al., 2018). Large amounts of anti-inflammatory drugs are prescribed in human care, but they are often sold in much higher amounts without a prescription (Ternes, 2001). NSAIDs and analgesics are often combined with antibiotics in veterinary medicine for problems such as pain, inflammation, fever, osteoarthritis and arthritis, and to reduce stress (Courtheyn et al., 2002; Bártíková et al., 2016). However, these two types of pharmaceuticals have numerous adverse effects in humans, including gastrointestinal disturbances, ulceration, renal failure with increased risk of post-operative bleeding, asthma, and rare allergic reactions (Ben Maamar et al., 2017; Morelli et al., 2017; Borgeat et al., 2018; Hurtado-Gonzalez et al., 2021). Approximately 35 million people use NSAIDs every day worldwide (Yu et al., 2013), and China increased its domestic production from 41,537 t in 2013 to 46,673 t in 2017 (Yan et al., 2021). They are currently monitored in effluents worldwide to check these drug concentrations and several studies show that both NSAIDs and analgesics are commonly detected in water bodies (Balakrishna et al., 2017; Świacka et al., 2021). In Cuernavaca (Mexico), high concentrations of naproxen (732–4,889 ng/L), acetaminophen (354–4,460 ng/L), and diclofenac (258–1,398 ng/L) have been detected in samples collected in different years, in the influent and effluent of a WWTP and in the surface waters of the Apatalco River (Rivera-Jaimes et al., 2018). Furthermore, the drugs diclofenac (10,221 ng/L highest concentration detected) and acetaminophen (1234-2346 ng/L), among others, have been detected in effluents from the Red Sea (Saudi Arabia) (Ali et al., 2017). On the other hand, in Brazil, acetaminophen (17.4–34.6 ng/L), diclofenac (19.4 ng/L), and ibuprofen (326.1–2,094.4 ng/L) have been detected in the surface and bottom water samples from Santos Bay (Pereira et al., 2016). These same drugs have also been detected in surface water on the northern Antarctic Peninsula region due to increased tourism in this area, with concentrations of 48.74, 15.09, and 10.05 ng/L of acetaminophen, diclofenac, and ibuprofen, reported respectively (González-Alonso et al., 2017).
Among the pharmaceutical compounds found in wastewater, antibiotics are of the greatest concern due to their persistent nature, partial metabolism, and easy movement through ecosystems (Mukhtar et al., 2020). Antibiotic production in China was approximately 92,700 tons, 48% destined for humans and the remaining for livestock; a total of 46% active metabolites were produced (Zafar et al., 2021). The antibiotics most commonly found in wastewater are sulfonamides, quinolones, tetracyclines, fluoroquinolones, and nitroimidazoles. The total concentrations of antibiotics vary depending on the body of water, in the case of wastewater, they can range between 0.0013 and 0.0125 μg/mL, in drinking water 0.0005 and 0.0214 μg/mL and river water 0.0003 and 0.0039 μg/mL (Zhang et al., 2015; Pan and Chu, 2017; Hanna et al., 2018). Antibiotic resistance of microorganisms to antimicrobials is becoming even stronger and more widespread over time and is expected to greatly increase human morbility and mortality in the near future (Bondarczuk and Piotrowska-Seget, 2019). Antibiotics have been found in rivers all over the world, including several in Spain (Ebro, Guadarrama and Manzanares Rivers), Italy (Arno River), South Korea (Han River), Taiwan (Xindian, Gaoping, Dahan and Po River), France (Seine River), United States (Ozark River), Sweden (Hoje River), and China (Pearl, Hai, Liao and Yellow Rivers) (Peng et al., 2008, 2011; Valcárcel et al., 2011; López-Serna et al., 2013; Bilal et al., 2020).
Endocrine disruptors were defined in 2002 by the International Programme on Chemical Safety (IPCS) of the United Nations Environment Programme (UNEP) and by the World Health Organization (WHO) as “an exogenous substance or mixture that alters the function(s) of the endocrine system and consequently causes adverse health effects in an intact organism or population”. Among the most common endocrine disruptors are pesticides, bisphenols and natural hormones (Gore et al., 2014; Tijani et al., 2016). These substances are not removed from water by conventional treatment processes and are found in wastewater bodies in the order of nanograms to micrograms per liter (Andrade-Eiroa et al., 2016; Gröger et al., 2020; Li et al., 2020).
Antiretroviral drugs are frequently used to treat the human immunodeficiency virus (HIV), an epidemic that has developed worldwide and has its epicenter in South Africa (Tompsett, 2020). As a result, millions of people have access to these drugs on a daily basis, with more than 40 different antiretroviral drugs being used for the treatment of HIV. These include abacavir, efavirenz, lamivudine, nevirapine, tenofovir, and zidovudine; many of which are used in combination (Russo et al., 2018; Mlunguza et al., 2020). As a consequence of the increase in the rate of HIV infection over the years, there has been a significant increase in the production and consumption of antiretroviral drugs worldwide (Nannou et al., 2020; Reddy et al., 2021). In addition, as consequence of the new pandemic coronavirus (COVID-19), antiretroviral drugs have also been used for the treatment of SARS-CoV-2. In some countries, such as China and Japan, clinical trials have been conducted to test the efficiency of using HIV drugs to treat COVID-19 (Reddy et al., 2021). At the moment, a scarcity of studies has dealt with this new issue. However, some studies have started to show a relevant problem that we will have in the very near future (Mupatsi, 2020).
In the coming decades, annual cancer cases are expected to increase to more than 20 million, which means an exponential increase in anticancer drugs and their subsequent release into wastewater (Ferlay et al., 2013). Most of these compounds are incompletely assimilated and metabolized by the human body, thus excreted in feces and urine. The most commonly administered anticancer drugs include cyclophosphamide, tamoxifen, ifosfamide and methotrexate, among others. These drugs have been detected in surface water, WWTP effluents and influents, and hospital effluents. Detected concentrations of cyclophosphamide range from 0.05 to 22,100 ng/L, ifosfamide 0.14–86,200 ng/L, methotrexate 1.6–4,756 ng/L, and tamoxifen 0.01–740 ng/L (Nassour et al., 2020). Several studies have detected these drugs in water masses, confirming that current water treatment systems fail to degrade them (Verlicchi et al., 2010; Cristóvão et al., 2019). Different international agencies have developed protocols for the handling and storing of pharmaceuticals to reduce their harmful effect on the environment (Bernabeu-Martínez et al., 2018). One of the main concerns is that these drugs may suffer biomagnification (Yadav et al., 2021).
Impact of Pharmaceuticals on the Environment and Living Organisms
Since almost all drugs are not completely metabolized by organisms (usually a small fraction of the active site of drug metabolic enzymes are occupied, the half-life of drugs are limited, and drugs are administrated in higher amounts than necessary to increase efficiency) (Coleman, 2020), the compounds that can cause the most damage once they are excreted and reached wastewater are PhACs. They are also called active pharmaceutical ingredients or APIs and metabolites, referring to the molecules resulting from these original compounds due to structural changes that take place in organisms. In addition, the resulting molecules are also subject to changes in the environment (such as oxidation, photolysis, or biotransformation). These changes can occur through both biotic and abiotic processes. Thus, many pharmaceutical products are biotransformed by microorganisms (Kümmerer, 2009; Wu et al., 2012). Ecotoxicologists are increasingly concerned about the worldwide detection of pharmaceutical residues in aquatic environments since their long-term toxic effects are being increasingly studied. However, it is challenging to know these effects because of the short time period these substances have been present in the environment (Nantaba et al., 2020; Ramírez-Morales et al., 2020; Gani et al., 2021).
Different studies analyzed the microbiome of wastewater where, in the case of hospitals, an abundance of anaerobes related to pathogenic threats such as Bifidobacteriales, Bacteroidales, and Clostridiales was found (Buelow et al., 2018; Ogwugwa et al., 2021; Palanisamy et al., 2021). They also noted that compared to other locations, hospital wastewater contains microorganisms with higher relative levels of antimicrobial and antibiotic resistance genes (Buelow et al., 2018). The mycobiome of hospital wastewater has also been analyzed, indicating the presence of different opportunistic phyla such as Mycosphaerella, Drechslera, Candida, or Cyphellophora (Olicón-Hernández et al., 2021), whose risk that they may acquire resistance to antibiotics is of great concern and may have great repercussions for global health.
Beta-Blocker and Psychoactives
β-blockers are a group of pharmaceuticals that are commonly detected in the environment. This is because many wastewater plants are not adapted to remove these micropollutants. Detected concentrations vary from 3 to 6,167 ng/L, which are already sufficient to cause neurotoxic and reproductive disorders in living organisms (Godlewska et al., 2021). Bisoprolol causes immobilization in Daphnia similis (Godoy et al., 2019) and mortality in fish and green algae (Fonseca et al., 2021). Propranolol causes growth and development problems in algae such as Synechococcus leopolensis and Cyclotella meneghiniana (Ferrari et al., 2004), mortality in crustacea (Ceriodaphnia dubia) (Huggett et al., 2002), and embryonic development problems in Danio rerio (Bittner et al., 2018).
Psychoactive substances affect thought, emotion, will and behavior (Jin et al., 2022). According to their pharmacological properties, psychoactive substances (including legal and illegal drugs) are opioids, cannabis, central nervous system depressants, central nervous system stimulants, hallucinogens, and tobacco (Schlüsener et al., 2015; Tanoue et al., 2019). These substances have different effects on humans, such as analgesia, anesthesia, inability to concentrate, excitement, anxiety, and mania. Jin et al. (2022) indicated that ecological risk assessment is a crucial part of research on psychoactive substances, as the current relevant literature is scarce. Due to the biological activity of such substances, there is a need for rapid improvement of risk assessment, including acute, cone and developmental toxicity, neurotoxicity, and endocrine-disrupting effects, among others, as well as the development of remediation technologies.
Non-steroidal Anti-inflammatory Drugs and Analgesics
Pharmaceuticals are known to have biological effects on living organisms, but there is not enough information currently available to assess the possible ecotoxicological impacts. Below are some of the toxic and ecological risks of NSAIDs and analgesics, according to various studies and summarized in Table 2: (I) population declines of Gyps vultures in Asia due to high diclofenac concentration (Cuthbert et al., 2007); (II) diclofenac impairs prostate gland synthesis and damage to the gills, liver, and kidneys of Salmo trutta f. fario (Hoeger et al., 2005); (III) histological alterations of the kidneys and gills, cytological alterations of the liver, kidneys, and gills, and deterioration of ionic regulation in Oncorhynchus mykiss (Schwaiger et al., 2004; Triebskorn et al., 2004; Gravel et al., 2009); (IV) ibuprofen, diclofenac, naproxen and ketoprofen inhibits CYP2M in Cyprinus carpio (Thibaut et al., 2006); (V) ibuprofen change breeding pattern of Oryzias latipes (Flippin et al., 2007); (VI) ibuprofen, diclofenac, and acetaminophen cause cardiovascular abnormalities, hatch and motor behavior and interruption of oocyte maturation/ovulation in D. rerio (David and Pancharatna, 2009; Lister and Van Der Kraak, 2009; Xia et al., 2017); (VII) diclofenac alters estrogenic activity, response of specific tissue biomarkers, decreased superoxide dismutase, and glutathione reductase activities in gills, and high catalase activity and levels of lipid peroxidation in the digestive gland in Mytilus galloprovincialis (Gonzalez-Rey and Bebianno, 2014). As can be inferred, high concentrations of NSAIDs and analgesics in the environment, such as acetylsalicylic acid, acetaminophen, diclofenac, ibuprofen, and naproxen, cause serious environmental problems (Parolini, 2020). In addition to fish, the main organisms affected are invertebrates, including arthropods, mollusks, cnidarians and rotifers (Parolini, 2020). NSAIDs also affect the plant growth of species such as Pisum sativum and Vigna unguiculata (Svobodníková et al., 2020; Wijaya et al., 2020; Table 2).
Antibiotics
Due to the continuous introduction of antibiotics into the environment, aquatic and soil organisms are chronically exposed to these drugs (Gothwal and Shashidhar, 2015; Bengtsson-Palme and Larsson, 2016). Moreover, because they are active at very low concentrations, they have a toxic effect on organisms, and there is a synergistic effect when they are present together with other drugs and/or xenobiotic compounds (González-Pleiter et al., 2013). Algae and aquatic plants are severely affected by antibiotics (Brain et al., 2008; Brausch et al., 2012). Many of them have been found to be photosynthesis inhibitors, as they can block the electron chain of photosystems II and increase oxidative stress (Nie et al., 2013). However, microorganisms, including bacteria and fungi, are developing resistance to antibacterial substances due to exposure to low concentrations over several generations (Kollef et al., 2017; Willyard, 2017; García et al., 2020; Wang et al., 2020). Invertebrates such as Hydra attenuata and crustaceans such as Artemia salina, Daphnia magna, and Ceriodaphnia dubia show relatively low acute toxicity in the presence of antibiotics (Wollenberger et al., 2000; Kołodziejska et al., 2013; Minguez et al., 2016). On the other hand, in fish, acute toxicity was only found at high concentrations, but there were cases in which no toxicity was observed (Santos et al., 2010; Brausch et al., 2012; Minguez et al., 2016; Table 2). The other major problem is antibiotic resistance genes (ARGs), which are genes that confer antibiotic resistance to bacteria, and can proliferate through the reproduction of antibiotic-resistant bacteria from the host or through horizontal gene transfer, are present in the environment, and thus considered as emerging environmental contaminants (Nadimpalli et al., 2020; Hu et al., 2021). Although treated wastewater contains significantly lower amounts of ARGs than untreated wastewater, several studies show that aquatic environments downstream of treatment plants can increase the amounts of ARGs because they are carried by mobile genetic elements, such as conjugative plasmids, integrative and conjugative elements, and transposons and integrons (Amos et al., 2018; Freeman et al., 2018; Jäger et al., 2018; Karkman et al., 2018; Liu et al., 2018). These effective carriers of ARGs could confer multi-resistance. One of the most detected genetic components in both effluents and aquatic environments is Class 1 integron-integrase gene (intI1) associated more frequently with ARGs and involved in horizontal gene transfer (Gillings et al., 2015; Cacace et al., 2019).
Endocrine Disruptors
Endocrine disruptors seriously affect both human and animal health, as they act directly on the endocrine system and block or mimic the natural hormones responsible for the functioning of some organs (Vieira et al., 2020). These substances have been studied extensively in humans, nevertheless, much less in the environment. It is known that they can alter the reproductive system, cause Alzheimer’s disease, thyroid problems, obesity and/or cancer (prostate, breast or endometrium cancer), among others (Heindel et al., 2015; Forte et al., 2016, 2019; Braun, 2017; Nadal et al., 2017; Marotta et al., 2019). In natural ecosystems, the reproductive system is also affected, as well as the levels of vitellogenin and hatchability and thus feminization with the consequent threat to the preservation of biodiversity (Vieira et al., 2020; Akhbarizadeh et al., 2021; Table 2).
Antiretrovirals
In contrast to other pharmaceuticals, antiretrovirals, despite being abundant in wastewater, are poorly monitored, although some studies report on them (Ngumba et al., 2016; Abafe et al., 2018; Rimayi et al., 2018; Mosekiemang et al., 2019; Mtolo et al., 2019). These drugs could pass through treated wastewater in WWTPs, reach drinking water sources, and cause serious ecotoxicological problems for human health (Hawkins, 2010; Ncube et al., 2018; Mlunguza et al., 2020). Currently, the greatest concern is that resistant strains of HIV can be created in the body through exposure to water contaminated with these drugs (Daouk et al., 2015; Ncube et al., 2018; Table 2).
Anticancer Drugs
Although anticancer drugs are designed to eliminate fast-growing cells, such as tumor cells, many of these drugs are not selective (Chari, 2008). This means that in addition to attacking healthy cells, they can cause cytotoxic, genotoxic, mutagenic, and teratogenic effects, i.e., cause adverse effects in any eukaryotic organism (Kümmerer et al., 2000; Johnson et al., 2008). For this reason, anticancer drugs are considered to be of great environmental concern, and especially the groups at greatest risk are children, pregnant women, and the elderly (Rowney et al., 2009). It has been shown that chronic exposure of two generations of D. rerio to anticancer drugs caused histopathological changes in the liver and kidney and impaired the integrity of their DNA, introducing massive changes in the entire transcriptome (Kovács et al., 2015; Gajski et al., 2016; Table 2).
Residues of pharmaceuticals in the environment typically occur as complex mixtures and even if the concentrations of an individual compound are low, the “cocktail effect” could be of significant ecotoxicological importance (Heath et al., 2016). To date, many works have focused on the study of individual organisms and analyzed a single drug or several drugs as a whole, but there are no works studying the impact of drugs on several populations simultaneously. This would provide essential information on ecotoxicity and the “domino effect” that affects individuals in a trophic chain since, in addition to bioaccumulation, the chain could be broken because a drug lethally affects a group of individuals.
Development of Bioremediation Technologies
Improving technologies for drug elimination from wastewater is an important task since pharmaceuticals have been detected in effluent from WWTPs and consequently surface water, groundwater, and drinking water globally (Bartolo et al., 2021). Although the pharmaceuticals are found in concentrations ranging from the nanogram to microgram per liter, which is too low to cause acute toxicity, they are biologically active compounds that have the potential for chronic toxicity, bioaccumulation, and biomagnification (Ruan et al., 2020). Additionally, microplastics have been shown to serve as vectors for pharmaceuticals (Santos et al., 2021), thus increasing the exposure potential. Because of incomplete elimination during conventional wastewater treatment (Reyes et al., 2021) and the potential risk posed to the environment, as discussed above, there has been pronounced interest in developing alternative treatments in recent years, specifically the biological transformation of these pollutants as a green technology (Domaradzka et al., 2015). The future inclusion of bioremediation technologies in traditional WWTP treatments is progressive as it will result in the detoxification of hazardous substances, it is less disruptive to the environment than harsh oxidative chemicals, and more cost-efficient. With perseverance, research into optimization could result in the complete eradication of target pollutants, rooting out release into the environment.
The wastewaters containing PhACs and their metabolites reaching WWTPs are commonly treated via purification systems. The potential of drug remediation via biological treatment utilizing microbes has been demonstrated (Kebede et al., 2018). Biological systems are often used in conjunction with advanced treatments and combined with conventional activated sludge (CAS) systems due to limitations associated with the process (Crini and Lichtfouse, 2019). Advanced biological treatments include modified CAS, aerobic granular systems, moving bed bioreactors (MBBRs), anammox systems, and membrane bioreactors (MBRs) (Grassi et al., 2012). However, some of these processes, such as MBRs, could result in the generation of biosolids or sewage sludge as byproducts of required maintenance. Sewage sludge, after different stabilization processes such as thermophilic anaerobic digestion, continues onto different processes, such as composting, which could facilitate the transfer of PhACs and their metabolites into various trophic levels of the food web when used as a soil amendment (Marcoux et al., 2013).
Bioremediation, utilizing native microbial monocultures or consortia or bioaugmentation, has been used for decades as a sustainable technology to manage anthropogenic pollution (Ahumada-Rudolph et al., 2021). The advantages of bioremediation include less input of hazardous chemicals, energy, and time, and it is cheap relative to other technologies (Azubuike et al., 2016). The major benefit of bioremediation is that the pollutant is chemically transformed and not only shifted from one environment to another (Mashi, 2013). However, a significant criticism of bioremediation has been that the remediation speed does not meet the requirements for the treatment capacity. Nonetheless, considering the benefits of the approach, attempts on optimizing the efficiency and decreasing retention times are being made and are reviewed below for mycoremediation. Developments in phyto- and phycoremediation of pharmaceuticals have been reported and recently reviewed (Vilvert et al., 2017; Rao et al., 2019; Kaloudas et al., 2021; Kurade et al., 2021) and thus, not included here.
Bacterial remediation has been reviewed to some extent (Shah and Shah, 2020), and, therefore, a brief overview of previously undiscussed advances are included here alongside mycoremediation. Bacterial communities have the ability to degrade and mineralize many xenobiotic compounds and have thus been used for centuries in wastewater-activated sludge (Xu et al., 2018). Bioremediation technologies have been advanced by studies elucidating the importance of facilitating biofilm growth in achieving maximum efficiency and community stability and survival (Edwards and Kjellerup, 2013). The majority of the available literature on bacterial remediation has focused on the aerobic degradation of pharmaceuticals by individual bacteria or consortia in which oxygenases are reported to be involved (Ferreira et al., 2018). Activated sludge, in which an uncharacterized bacterial consortium in suspension is responsible for the remediation, is one of the most widely used biological methods to treat pharmaceutical wastewater at a large scale (Bis et al., 2019). However, due to operational issues associated with the development of large amounts of sludge, research has been invested in developing bespoke bacterial consortia for remediation, including microalgae and bacterial-microalgae consortia (Mamta et al., 2020).
In the environment, fungi are excellent decomposers through the nonspecific nature of enzymes, both intracellular and extracellularly secreted, which exhibit significant capabilities to degrade organic material (Rouches et al., 2016). More specifically, the ligninolytic (including peroxidases and laccases) and cytochrome P450 systems have been proven to be involved in the exceptional capacity of white-rot fungi to degrade recalcitrant pollutants (Park and Choi, 2020). The nonspecific nature of these enzymes also makes them an ideal approach to deal with the diverse chemical structures of the many classes of pharmaceuticals. Many fungal species are also hyperaccumulators, capable of absorbing and bioaccumulating xenobiotics from their environment, as demonstrated by the ability of mushrooms (Braeuer et al., 2020). Furthermore, fungi are known for their capacities to adapt to severe environmental constraints (Jiao and Lu, 2020), making them more tolerant to environmental changes than other bioremediation organisms. Thus, mycoremediation, which results in the reduced toxicity of wastewater (Jelic et al., 2012; Akhtar and Mannan, 2020), offers a comparatively cost-effective, eco-friendly, and effective approach to pollution remediation.
Macromycetes, aka mushrooms or polypores, were previously proven efficient in remediating various pharmaceuticals (Migliore et al., 2012; Cruz-Morató et al., 2014), including β-blockers and psychoactive drugs, anti-inflammatory drugs, antibiotics and hormones (Table 3). Mostly, investigations into the efficiency of fungi to remediate pharmaceuticals have been performed in flask batch experiments with white-rot fungi, especially Trametes versicolor, which exhibited impressive capacities for eliminating a vast range of pharmaceuticals. In bioreactors-based studies, T. versicolor was equally efficient, able to degrade various pharmaceuticals, including codeine, diazepam, carbamazepine, and metoprolol (Asif et al., 2017). The role of redox-mediators has also been extensively studied in improving the performance of laccase-based treatments (Ashe et al., 2016; Shao et al., 2019), including the treatment of pharmaceuticals (Nguyen et al., 2013; Vasiliadou et al., 2019). Studies employing filamentous micromycetes have shown potential for pharmaceutical remediation from wastewaters as reviewed by Olicón-Hernández et al. (2017) but are limited compared to the literature on macromycetes (Table 3). The efficiency of bacteria and fungi to remediate different classes of pharmaceuticals is discussed in more detail below.
Beta-Blockers and Psychoactive Drugs
Carbamazepine, which is not adequately eliminated via standard wastewater treatments and is thus frequently detected in the environment (Ekpeghere et al., 2018), has been reported to be degraded by the macromycete T. versicolor. By employing T. versicolor, Jelic et al. (2012) achieved 94% degradation of carbamazepine (9 mg/L) after six days in flask experiments. With a reduced concentration (50 μg/L), Jelic et al. (2012) reported a lower remediation percentage of 61% achieved in seven days. The same group evaluated the fungus’s remediation efficiency of carbamazepine in an air pulsed fluidized bed bioreactor operated in batch and continuous mode. In batch mode, 96% of the drug was eliminated after 2 days, with higher efficiency achieved in the bioreactor than in flasks explained by glucose addition, pH management and air supplementation. In continuous mode, carbamazepine was reduced by 54% in the outflow compared to the inflow concentration of 200 μg/L (Jelic et al., 2012). With Pleurotus ostreatus, another white-rot fungus, 68% carbamazepine was degraded in liquid culture after seven days with no further degradation after this time (Buchicchio et al., 2016).
The filamentous fungus Trichoderma harzianum was able to degrade 72% of environmentally detected concentrations of carbamazepine (4 μg/L) (Buchicchio et al., 2016), which was superior compared to the polypore P. ostreatus. In a non-sterile bioreactor, Phanerochaete chrysosporium was able to degrade up to 80% of 5 mg/L carbamazepine when supplied with a diluted synthetic feed (Zhang and Geißen, 2012). In a fed-batch stirred bioreactor, P. chrysosporium removed yo to 60% carbamazepine (0.5 mg/L); however, it was unable to degrade diazepam (0.25–0.5 mg/L) (Rodarte-Morales et al., 2012a). In a fixed bed reactor, where the pellets of P. chrysoporium were immobilized in polyurethane, the remediation efficiency of carbamazepine and diazepam was significantly improved (Rodarte-Morales et al., 2012b).
Even though nearly complete remediation of some beta-blockers and psychoactive drugs could be achieved in flask and lab bioreactor scale experiments, large or even pilot scale studies are needed to comprehensively evaluate the effect of upscaling on the remediation efficiency and the cost-effectiveness of using fungi for these drugs as an add-on treatment in WWTPs.
Non-steroidal Anti-inflammatory Drugs and Analgesics
Bioremediation using bacterial monocultures for the treatment of NSAIDs has not to date been successful (Wojcieszyńska et al., 2014). Some studies have shown the elimination of NSAIDs by bacterial consortia in WWTPs. One study showed that eliminating acetaminophen in an MBR was mainly associated with heterotrophic bacteria. They concluded that using a microbial consortium in an MBR could be complimentary for post-treating effluents from treatment plants containing pharmaceutical products (De Gusseme et al., 2011). However, as seen with the consortia in CAS treatments, which are unidentified and often change in conjunction with the wastewater being treated, consortia in bioreactors may also change, resulting in decreased efficiency. To further explore the use of bacterial consortia in bioreactors, long-term studies need to be conducted on-site in WWTPs to evaluate the composition and stability of the bacterial assemblage, and it should be modeled how shifts could influence remediation.
In terms of mycoremediation, T. versicolor has shown very promising results in the remediation of NSAIDs (Asif et al., 2017; Tińma et al., 2021). In a continuous MBR (with a hydraulic retention time of one day), T. versicolor eliminated 55% of diclofenac added at concentrations ranging from 0.3 to 1.5 mg/L (Yang et al., 2013). Another fungus that demonstrated the potential to degrade anti-inflammatory drugs is the edible fungus Lentinula edodes (shiitake mushroom). The degradation products of piroxicam produced by L. edodes degradation has already been described (Muszyńska et al., 2019); however, the remediation percentage was not reported.
Penicillium oxalicum was capable of totally degrading diclofenac in 24 h, starting from an initial concentration of 29.6 mg/L (100 μM) (Olicón-Hernández et al., 2019). For Mucor hiemalis f. irnsingii (DSM 14200; Zygomycota), a strain isolated from a groundwater source in Germany, the diclofenac (10–50 μg/L) removal percentages ranged between 90 and 97% after 6 days (Esterhuizen-Londt et al., 2017). The same micromycete was also employed for the remediation of acetaminophen. After 24 h of exposure to environmentally relevant concentrations of acetaminophen (up to 20 μg/L), M. hiemalis was able to degrade up to 50% (Esterhuizen-Londt et al., 2016b,a). However, after 24 h, diclofenac remediation halted; nevertheless, pH maintenance could overcome this (Esterhuizen et al., 2021). The acetaminophen remediation efficiency of Phanerochaete chrysosporium (97 and 99% of 250 μg/L APAP after 3 and 7 days, respectively) was far superior to that of M. hiemalis, and co-cultivation of the two species resulted in a decreased remediation efficiency compared to P. chrysosporium in single (Esterhuizen et al., 2021).
Furthermore, Olicón-Hernández et al. (2020) studied the degradation of a mixture of acetaminophen, diclofenac, ibuprofen, ketoprofen and naproxen with P. oxalicum, starting from an initial concentration of 50 μM of each compound in both flasks and bench fluidized bioreactors. P. oxalicum showed higher degradation percentages in the bioreactor than at the flask scale. The authors reported that with glucose addition in the fluidized bed bioreactor, degradation of all drugs was complete after eight days (Olicón-Hernández et al., 2020).
In a fed-batch stirred bioreactor, P. chrysosporium oxidatively degraded up to 99% of diclofenac, ibuprofen, and naproxen each at a concentration of 0.8 mg/L (Rodarte-Morales et al., 2012a). However, in continuously stirred bioreactors, P. chrysosporium degraded diclofenac, ibuprofen, and naproxen (1 mg/L each) up to 95%.
With these preliminary flask and laboratory-scale reactor experiments, the potential of using mycoremediation to treat NSAIDs is highlighted. However, data on the performance of the fungi in WWTPs is lacking, making a consequential evaluation impossible. A potential issue that may arise in practice is the need for maintenance and controlled conditions, as highlighted by the study conducted by Esterhuizen et al. (2021), which showed the need for maintaining pH conditions.
To overcome the limitations of monocultures for the remediation of these pollutants, the use of microorganism-consortia has been explored. Consortia of microorganisms that complement each other could improve biological wastewater treatment technologies significantly. For example, Nguyen et al. (2013) found that a mixed bacterial culture in conjunction with T. versicolor in an augmented MBR better degraded PhACs than a system containing the fungus or bacteria alone (Nguyen et al., 2013). In addition, bioaugmentation technologies using adapted fungi, such as P. oxalicum, have proven an interesting technology to overcome the problem of competition with autochthonous microbiota, as demonstrated by Olicón-Hernández et al. (2021). However, more data are needed to define complementary species since the study by Esterhuizen et al. (2021) revealed that co-culture of certain species could reduce the remediation efficiency.
Antibiotics
In general, low remediation efficiencies for most antibiotics from wastewaters have been reported using CAS treatment (Chaturvedi et al., 2021a; Zou et al., 2022). Thus, CAS could be applied to treat some antibiotics; however, not all. More recently, increased antibiotic removal percentages have been reported with anoxic/anaerobic/oxic granular and suspended activated sludge processes, specifically with sulfamethoxazole (Kang et al., 2018). The shortcoming could be improved by supplementing the sludge with bacteria capable of better remediation or even mixing treatments and complementing CAS with mycoremediation with macromycetes has been proven to be very effective for antibiotics.
T. versicolor, in flask experiments, degraded the antibiotic ofloxacin (10 mg/L) with 80% efficiency. When upscaled to 10 L fluidized air-pulse bioreactors, ofloxacin spiked into hospital waste was removed by 98.5% under sterile conditions and 99% under nonsterile conditions (Gros et al., 2014).
Buchicchio et al. (2016) reported the elimination of 55% clarithromycin (0.03 μg/L) by edible mushroom P. ostreatus and 57% by the micromycete T. harzianum. In flask experiments, P. ostreatus could also eliminate oxytetracycline (50 and 100 mg/L) after 14 days (Migliore et al., 2012). The antifungal drugs bifonazole and clotrimazole were also bioaccumulated and eliminated by the mycelia of the edible fungus Lentinus edodes (Kryczyk-Poprawa et al., 2019). In flask experiments, the cephalosporin antibiotic cefuroxime axetil was entirely eradicated by both the edible mushrooms Imleria badia and L. edodes within seven days at all concentrations tested (400, 1,000, 1,600 mg/L) (Dąbrowska et al., 2018).
Leptosphaerulina sp. removed oxacillin (16 mg/L, in 6 days), cloxacillin (17.5 mg/L, in 7 days) and dicloxacillin (19 mg/L, in 8 days) from water in flask experiments by the action of laccase and peroxidase. With synthetic hospital waste, oxacillin was reduced by 60% within two days and wholly eradicated after six days by the Leptosphaerulina sp. (Copete-Pertuz et al., 2018).
In a comparative study investigating the degradation efficiencies of five ligninolytic fungi, the polypore Irpex lacteus degraded the fluoroquinolone antibiotic flumequine, ciprofloxacin and ofloxacin effectively within six days (Èvanèarová et al., 2013; Čvanv̌arová et al., 2015). I. lacteus also removed the residual antibacterial activity of norfloxacin and ofloxacin via the action of manganese peroxidase (Čvanv̌arová et al., 2015).
Ahumada-Rudolph et al. (2021) evaluated fifty fungal isolates from sediments of salmon hatcheries for their oxytetracycline remediation abilities. The filamentous fungi Penicillium commune, Epicoccum nigrum, T. harzianum, Aspergillus terreus, and Beauveria bassiana were identified as having the best remediation rates amounting to a maximum of 78% removal of a 250 mg/L oxytetracycline concentration in flask experiments (Ahumada-Rudolph et al., 2021). P. oxalicum RJJ-2 has also been studied in the degradation of erythromycin and degraded 84.88% erythromycin after 96-h incubation used as the sole carbon source producing different metabolites (Ren et al., 2021).
The studies on the efficiency to remove antibiotics reported to date have focused on the efficiency under set conditions. However, in a WWTP, environmental conditions and even the water’s parameter would fluctuate from time to time. How this could affect the remediation efficiency and fungal longevity over time is unknown. Nevertheless, this information could be essential in evaluating this technique’s applicability in the field. It is importante to note the relevance of the use of fungi in removing antibiotics since bacteria can adquire rapidly antibiotic resistance genes during bioremediation and contribute to the widespread of ARGs.
Endocrine Disruptors
The fate of estrogenic hormones treated via activated sludge systems in full-scale WWTPs was reviewed by Hamid and Eskicioglu (2012). Activated sludge systems with nutrient removal achieved more than 90% degradation in most studies (Hamid and Eskicioglu, 2012).
Degradation of testosterone and 17α-ethinylestradiol (EE2) by the fungus L. edodes was reported by Muszyńska et al. (2018), with no testosterone or 17α-ethynylestradiol detected after 21 days (Muszyńska et al., 2018). Interestingly, the white-rot fungus P. ostreatus HK 35, in the presence of the natural water microbiota of a WWTP, degraded up to 90% of 17β-estradiol (E2) within 12 days in various bioreactor sizes and under different regimes (Křesinová et al., 2018). The micromycete Trichoderma citrinoviride AJAC3 degraded 99.6% 17 β-estradiol (E2) (at a starting concentration of 200 mg/L) after four days attributed to the secretion of ligninolytic enzymes (Chatterjee and Abraham, 2019). A study investigating the efficiency of mycoremediation to remove 17 β-estradiol (E2) from poultry litter found that the polypore Pycnoporus sp. SYBC-L3 could remove up to 78.4% via solid-state cultivation supplemented with citric acid and lignocellulosic biomasses to boost laccase activity (Liu et al., 2016), an approach that could be tested for increasing remediation from wastewaters.
Even though the hormone remediation percentage reported with mycoremediation is, in some cases, higher than the CAS studies reviewed by Hamid and Eskicioglu (2012), a comparison is not possible since the studies on the fungal efficiency were performed in the laboratory in comparison to the CAS studies completed on-site at WWTPs. In addition to excluding several variables that could impact the remediation efficiency, these studies have established the remediation efficiencies for individual compounds. In wastewater effluent, a mixture of not only PhACs are present, and the synergistic effect of all these compounds could affect the efficiencies reported (Chatterjee and Abraham, 2019).
Bioabsorption is another approach to PhAC remediation with fungi. L. edodes and Agaricus bisporus (champignon) stalks removal 100% of 17α-ethinylestradiol (EE2) in 20 and 30 min, respectively via absorption, whereas Shiitake substrate absorbed 80% (de Jesus Menk et al., 2019).
Despite the high hormone remediation percentages achieved with fungi described above, few studies have been published on this topic in the last decade, and renewed investigations would greatly benefit the development of this technique to elevate the environmental impacts of hormones released untreated from WWTPs.
Mixed Effluents
Cruz-Morató et al. (2013) studied the degradation of pharmaceuticals in hospital effluent by T. versicolor. By employing fluidized bed bioreactor in fed-batch mode, T. versicolor could eliminate ibuprofen (2.34 mg/L), acetaminophen (1.56 mg/L), ketoprofen (0.08 mg/L), propranolol (0.06 mg/L), and azithromycin (4.31 mg/L). By running the fluidized bed reactor in continuous mode, the efficiency was increased, and the fungus was able to completely remove acetaminophen (109 mg/L), naproxen (1.62 mg/L), ibuprofen (35.5 mg/L), diclofenac (0.477 mg/L), codeine (0.606 mg/L), trimethoprim (0.853 mg/L), and sulfamethoxazole 1.41 mg/L 100%, and partially remove several other drugs. However, salicylic acid, tetracycline, and carbamazepine were not degraded (Cruz-Morató et al., 2013, 2014). T. versicolor was also investigated for its performance to remediate PhACs from veterinary hospital wastewater; however, only 66% removal efficiency was achieved in a non-sterile batch bioreactor (Badia-Fabregat et al., 2016).
P. oxalicum XD.3.1 has also been used in batch bench-scale bioreactors to test the remediation efficiency with real hospital effluents. Within 24 h, P. oxalicum was able to reduce the majority of the PhAC present in the effluent, including ketoprofen, naproxen and paracetamol. Interestingly, P. oxalicum also affected the native microbiota, including opportunistic pathogens (Olicón-Hernández et al., 2021). In fluidized bed bioreactor studies, including hospital wastewater spiked with 10 mg/L each diclofenac, ketoprofen, and atenolol, P. ostreatus completely remediated diclofenac in 24 h and 50% of the ketoprofen in 5 days. However, atenolol was not removed (Palli et al., 2017). These studies demonstrated the complexity of degrading PhAC in mixed matrix effluents, which could drastically reduce the remediation efficiency. Therefore, more studies should be conducted at a larger scale employing real effluents to develop mycoremediation using fungi.
Currently, mycoremediation studies on other emerging PhACs, such as anticancer and antiretrovirals, are lacking. Testing fungal species capable of degrading pharmaceuticals at a laboratory scale is ongoing; however, it is difficult to predict how biological organisms would cope in a treatment facility exposed to chemical mixtures over long periods. Thus, recognizing the potential of mycoremediation for the treatment of pharmaceuticals demonstrated to date, studies regarding functioning and long-term applicability in practical terms to evaluate the feasibility of mycoremediation fully are still lacking. However, limitations such as partial degradation of pharmaceuticals and reduced efficiency at lower PhAC concentrations have been identified but could be overcome by using consortia or optimizing enzyme extraction and isolation to reduce costs.
The exact mechanism of degradation for each fungal type and PhACs is still vague due to its complexity and all the counterparts involved (Dąbrowska et al., 2018). However, the degradation seems to include activities of the intracellular enzymatic system such as the cytochrome P450 system, mainly in fungi lacking ligninolityc enzymes, and the extracellular enzymatic system, including lignin peroxidase, manganese peroxidase, laccase, versatile peroxidase as well as hydroxyl and free radical, in the case of lignin degrading enzymes producers (Dąbrowska et al., 2018; Barh et al., 2019). Nevertheless, elimination is reported to produce no toxic byproducts (Copete-Pertuz et al., 2018), therefore necessitating further studies into mycoremediation optimization for an add-on in WWTPs and elucidating the mechanism of action.
Isolated Fungal Enzymes
The use of isolated fungal enzymes could also overcome some limitations associated with mycoremediation. Fungal enzymes, specifically the ligninolytic enzymes, have been recognized for their abilities to transform a broad range of recalcitrant PhACs. However, difficulties in growing fungi on a large scale, together with the long incubation processes, extensive growth phase, and spore formation, have prompted the exploration of extracted crude and isolated enzymes (Stadlmair et al., 2018). Though, to date, the main limiting factor has been the high cost of the enzyme purification procedure.
Commercially available laccases from T. versicolor efficiently degraded diclofenac, trimethoprim, carbamazepine and sulfamethoxazole as individual drugs, but the remediation efficiency decreased when applied to mixtures of the drugs (Alharbi et al., 2019). Kang et al. (2021) isolated laccases from Bjerkandera spp., which could efficiently remediate acetaminophen under a range of pH conditions (Kang et al., 2021). In a study employing immobilized laccases from Trametes hirsuta, Hachi et al. (2017) reported better remediation efficiencies for carbamazepine and acetaminophen (40 and 70%) in single compared to in mixtures (5 and 25%) (Hachi et al., 2017).
Using laccases (2,000 U/L) isolated from Myceliophthora thermophile, 94.1 and 95.5% of estrone E1 and 17β-estradiol E2 could be degraded within 8 h in the presence of a natural mediator in a fed-batch bioreactor. In an enzymatic membrane reactor (EMR) with a stir-tank configuration, this percentage was increased to 95% for E1 and near total E2 degradation (Lloret et al., 2010). This indicates that the bioreactor type significantly impacts the remediation efficiency regarding isolated enzymes. In a study by Becker et al. (2017), immobilized laccase from T. versicolor and M. thermophila could degrade 83 and 87%, respectively, of estrogenic compounds (E1 estrone; E2 17β-estradiol; EE2 17α-ethinylestradiol) in mixtures with other endocrine-disrupting compounds within 6h (Becker et al., 2017). Golveia et al. (2018) reported 96.5% remediation of 10 mg/L 17-α-ethinylestradiol by Pycnoporus sanguineus laccase (1,642 U/mL) after 8 h (Golveia et al., 2018). It would be noted that 1% (v/w) was added to the fungal culture to promote optimal laccase production concentration before extraction.
Utilizing isolated enzymes has the advantages of reducing the remediation time by avoiding the lag phase of fungal growth, reducing sludge production, and facilitating process control (Jebapriya and Gnanadoss, 2013). Apart from the high cost as a disadvantage, a study by Nguyen et al. (2014) demonstrated another drawback of using isolated enzymes (Nguyen et al., 2014). In a direct comparison, whole-cell culture degraded trace organic compounds with higher efficiency, which is said to be facilitated by biosorption and the activity of both intracellular and mycelium associated enzymes.
Conclusion
The environmental impact of pharmaceuticals and their proper elimination from wastewaters have gained interest in recent years, mostly due to the intrinsic characteristics of these compounds, their massive use, and the negative effects on the environment and humans. Although they are medicinal substances developed to aid in the well-being of organisms, their indiscriminate use can lead to irreversible environmental problems. Therefore, it is important to create legislation according to the current standards of using substances and eco-friendly trends. More versatile and efficient systems for eliminating PhACs such as mycoremediation are being developed to lessen or avoid the problems associated with pharmaceutical pollution in the environment. However, these promising techniques are still at a laboratory scale and data regarding the application in WWTPs are still lacking. Even though new techniques for the remediation of PhAC are being developed and optimized, relative to the development of new drugs, implementing these techniques into practice is slow. New promising approaches for this purpose, such as genetic engineering, are still in their infancy. Thus, the new editing tool, such as CRISPR-Cas9, could help to introduce metabolic genes focused on target recalcitrant compounds. Much more studies are still necessary to deal with the problem of PhACs.
Author Contributions
ME, EA, DRO-H: conceptualization. MO and ME: literature search and data analysis and original draft preparation. MO, ME, DRO-H, JG-L, and EA: critical revision of the work. All authors contributed to the article and approved the submitted version.
Funding
MO received a Ph.D. grant from the Junta de Castilla y León (Spain). Open Access Funding was provided by the University of Helsinki.
Conflict of Interest
The authors declare that the research was conducted in the absence of any commercial or financial relationships that could be construed as a potential conflict of interest.
Publisher’s Note
All claims expressed in this article are solely those of the authors and do not necessarily represent those of their affiliated organizations, or those of the publisher, the editors and the reviewers. Any product that may be evaluated in this article, or claim that may be made by its manufacturer, is not guaranteed or endorsed by the publisher.
Acknowledgments
DRO-H thanks National Council of Science and Technology (CONACyT) and Secretariat of Research and Postgraduate Studies of the IPN project 20220492. We gratefully acknowledge the Spanish Ministry for Economy and Competitiveness within the context of the research projects CTM2017-84332-R (MINECO/AEI/FEDER/UE).
Supplementary Material
The Supplementary Material for this article can be found online at: https://www.frontiersin.org/articles/10.3389/fmicb.2022.869332/full#supplementary-material
References
Abafe, O. A., Späth, J., Fick, J., Jansson, S., Buckley, C., Stark, A., et al. (2018). LC-MS/MS determination of antiretroviral drugs in influents and effluents from wastewater treatment plants in KwaZulu-Natal, South Africa. Chemosphere 200, 660–670. doi: 10.1016/j.chemosphere.2018.02.105
Agunbiade, F. O., and Moodley, B. (2016). Occurrence and distribution pattern of acidic pharmaceuticals in surface water, wastewater, and sediment of the Msunduzi River, Kwazulu-Natal, South Africa. Environ. Toxicol. Chem. 35, 36–46. doi: 10.1002/etc.3144
Ahmed, I., Iqbal, H. M. N., and Dhama, K. (2017). Enzyme-based biodegradation of hazardous pollutants—An overview. J. Exp. Biol. Agric. Sci. 5, 402–411. doi: 10.18006/2017.5(4).402.411
Ahumada-Rudolph, R., Novoa, V., Becerra, J., Cespedes, C., and Cabrera-Pardo, J. R. (2021). Mycoremediation of oxytetracycline by marine fungi mycelium isolated from salmon farming areas in the south of Chile. Food Chem. Toxicol. 152:112198. doi: 10.1016/j.fct.2021.112198
Akhbarizadeh, R., Russo, G., Rossi, S., Golianova, K., Moore, F., Guida, M., et al. (2021). Emerging endocrine disruptors in two edible fish from the Persian Gulf: occurrence, congener profile, and human health risk assessment. Mar. Pollut. Bull. 166:112241. doi: 10.1016/j.marpolbul.2021.112241
Akhtar, N., and Mannan, M. A. (2020). Mycoremediation: expunging environmental pollutants. Biotechnol. Rep. 26:e00452. doi: 10.1016/j.btre.2020.e00452
Alharbi, S. K., Nghiem, L. D., van de Merwe, J. P., Leusch, F. D. L., Asif, M. B., Hai, F. I., et al. (2019). Degradation of diclofenac, trimethoprim, carbamazepine, and sulfamethoxazole by laccase from Trametes versicolor: transformation products and toxicity of treated effluent. Biocatal. Biotransformation 37, 399–408. doi: 10.1080/10242422.2019.1580268
Ali, A. M., Rønning, H. T., Alarif, W., Kallenborn, R., and Al-Lihaibi, S. S. (2017). Occurrence of pharmaceuticals and personal care products in effluent-dominated Saudi Arabian coastal waters of the Red Sea. Chemosphere 175, 505–513. doi: 10.1016/j.chemosphere.2017.02.095
Aminot, Y., Litrico, X., Chambolle, M., Arnaud, C., Pardon, P., and Budzindki, H. (2015). Development and application of a multi-residue method for the determination of 53 pharmaceuticals in water, sediment, and suspended solids using liquid chromatography-tandem mass spectrometry. Anal. Bioanal. Chem. 407, 8585–8604. doi: 10.1007/s00216-015-9017-3
Amos, G. C. A., Ploumakis, S., Zhang, L., Hawkey, P. M., Gaze, W. H., and Wellington, E. M. H. (2018). The widespread dissemination of integrons throughout bacterial communities in a riverine system. ISME J. 12, 681–691. doi: 10.1038/s41396-017-0030-8
Andrade-Eiroa, A., Canle, M., Leroy-Cancellieri, V., and Cerdà, V. (2016). Solid-phase extraction of organic compounds: a critical review (Part I). TrAC Trends Anal. Chem. 80, 641–654. doi: 10.1016/j.trac.2015.08.015
Ashe, B., Nguyen, L. N., Hai, F. I., Lee, D.-J., van de Merwe, J. P., Leusch, F. D. L., et al. (2016). Impacts of redox-mediator type on trace organic contaminants degradation by laccase: degradation efficiency, laccase stability and effluent toxicity. Int. Biodeterior. Biodegradation 113, 169–176. doi: 10.1016/j.ibiod.2016.04.027
Ashton, D., Hilton, M., and Thomas, K. V. (2004). Investigating the environmental transport of human pharmaceuticals to streams in the United Kingdom. Sci. Total Environ. 333, 167–184. doi: 10.1016/j.scitotenv.2004.04.062
Asif, M. B., Hai, F. I., Singh, L., Price, W. E., and Nghiem, L. D. (2017). Degradation of pharmaceuticals and personal care products by white-rot fungi—a critical review. Curr. Pollut. Rep. 3, 88–103. doi: 10.1007/s40726-017-0049-5
aus der Beek, T., Weber, F.-A., Bergmann, A., Hickmann, S., Ebert, I., Hein, A., et al. (2016). Pharmaceuticals in the environment—global occurrences and perspectives. Environ. Toxicol. Chem. 35, 823–835. doi: 10.1002/etc.3339
Azanu, D., Styrishave, B., Darko, G., Weisser, J. J., and Abaidoo, R. C. (2018). Occurrence and risk assessment of antibiotics in water and lettuce in Ghana. Sci. Total Environ. 622–623, 293–305. doi: 10.1016/j.scitotenv.2017.11.287
Azubuike, C. C., Chikere, C. B., and Okpokwasili, G. C. (2016). Bioremediation techniques–classification based on site of application: principles, advantages, limitations and prospects. World J. Microbiol. Biotechnol. 32:180. doi: 10.1007/s11274-016-2137-x
Azuma, T., Otomo, K., Kunitou, M., Shimizu, M., Hosomaru, K., Mikata, S., et al. (2019). Environmental fate of pharmaceutical compounds and antimicrobial-resistant bacteria in hospital effluents, and contributions to pollutant loads in the surface waters in Japan. Sci. Total Environ. 657, 476–484. doi: 10.1016/j.scitotenv.2018.11.433
Badia-Fabregat, M., Lucas, D., Pereira, M. A., Alves, M., Pennanen, T., Fritze, H., et al. (2016). Continuous fungal treatment of non-sterile veterinary hospital effluent: pharmaceuticals removal and microbial community assessment. Appl. Microbiol. Biotechnol. 100, 2401–2415. doi: 10.1007/s00253-015-7105-0
Balakrishna, K., Rath, A., Praveenkumarreddy, Y., Guruge, K. S., and Subedi, B. (2017). A review of the occurrence of pharmaceuticals and personal care products in Indian water bodies. Ecotoxicol. Environ. Saf. 137, 113–120. doi: 10.1016/j.ecoenv.2016.11.014
Barber, L. B., Loyo-Rosales, J. E., Rice, C. P., Minarik, T. A., and Oskouie, A. K. (2015). Endocrine disrupting alkylphenolic chemicals and other contaminants in wastewater treatment plant effluents, urban streams, and fish in the Great Lakes and Upper Mississippi River Regions. Sci. Total Environ. 517, 195–206. doi: 10.1016/j.scitotenv.2015.02.035
Barh, A., Kumari, B., Sharma, S., Annepu, S. K., Kumar, A., Kamal, S., et al. (2019). “Chapter 1 - mushroom mycoremediation: kinetics and mechanism,” in Smart Bioremediation Technologies, ed. P. Bhatt (Cambridge, MA: Academic Press), 1–22. doi: 10.1016/B978-0-12-818307-6.00001-9
Barrios-Estrada, C., de Jesús Rostro-Alanis, M., Muñoz-Gutiérrez, B. D., Iqbal, H. M. N., Kannan, S., and Parra-Saldívar, R. (2018). Emergent contaminants: endocrine disruptors and their laccase-assisted degradation – a review. Sci. Total Environ. 612, 1516–1531. doi: 10.1016/j.scitotenv.2017.09.013
Bártíková, H., Podlipná, R., and Skálová, L. (2016). Veterinary drugs in the environment and their toxicity to plants. Chemosphere 144, 2290–2301. doi: 10.1016/j.chemosphere.2015.10.137
Bartolo, N. S., Azzopardi, L. M., and Serracino-Inglott, A. (2021). Pharmaceuticals and the environment. Early Hum. Dev. 155:105218. doi: 10.1016/j.earlhumdev.2020.105218
Becker, D., Rodriguez-Mozaz, S., Insa, S., Schoevaart, R., Barceló, D., de Cazes, M., et al. (2017). Removal of endocrine disrupting chemicals in wastewater by enzymatic treatment with fungal laccases. Org. Process Res. Dev. 21, 480–491. doi: 10.1021/acs.oprd.6b00361
Ben Maamar, M., Lesné, L., Hennig, K., Desdoits-Lethimonier, C., Kilcoyne, K. R., Coiffec, I., et al. (2017). Ibuprofen results in alterations of human fetal testis development. Sci. Rep. 7:44184. doi: 10.1038/srep44184
Bengtsson-Palme, J., and Larsson, D. G. J. (2016). Concentrations of antibiotics predicted to select for resistant bacteria: proposed limits for environmental regulation. Environ. Int. 86, 140–149. doi: 10.1016/j.envint.2015.10.015
Bernabeu-Martínez, M. A., Ramos Merino, M., Santos Gago, J. M., Álvarez Sabucedo, L. M., Wanden-Berghe, C., and Sanz-Valero, J. (2018). Guidelines for safe handling of hazardous drugs: a systematic review. PLoS One 13:e0197172. doi: 10.1371/journal.pone.0197172
Bilal, M., Mehmood, S., Rasheed, T., and Iqbal, H. M. N. (2020). Antibiotics traces in the aquatic environment: persistence and adverse environmental impact. Curr. Opin. Environ. Sci. Heal. 13, 68–74. doi: 10.1016/j.coesh.2019.11.005
Bis, M., Montusiewicz, A., Piotrowicz, A., and Łagód, G. (2019). Modeling of wastewater treatment processes in membrane bioreactors compared to conventional activated sludge systems. Processes 7:285. doi: 10.3390/pr7050285
Bittner, L., Teixido, E., Seiwert, B., Escher, B. I., and Klüver, N. (2018). Influence of pH on the uptake and toxicity of β-blockers in embryos of zebrafish, Danio rerio. Aquat. Toxicol. 201, 129–137. doi: 10.1016/j.aquatox.2018.05.020
Blanco, G., Junza, A., and Barrón, D. (2017). Occurrence of veterinary pharmaceuticals in golden eagle nestlings: unnoticed scavenging on livestock carcasses and other potential exposure routes. Sci. Total Environ. 586, 355–361. doi: 10.1016/j.scitotenv.2017.02.023
Bondarczuk, K., and Piotrowska-Seget, Z. (2019). Microbial diversity and antibiotic resistance in a final effluent-receiving lake. Sci. Total Environ. 650, 2951–2961. doi: 10.1016/j.scitotenv.2018.10.050
Borecka, M., Siedlewicz, G. Haliński, Ł. P., Sikora, K., Pazdro, K., Stepnowski, P., et al. (2015). Contamination of the southern Baltic Sea waters by the residues of selected pharmaceuticals: method development and field studies. Mar. Pollut. Bull. 94, 62–71. doi: 10.1016/j.marpolbul.2015.03.008
Borgeat, A., Ofner, C., Saporito, A., Farshad, M., and Aguirre, J. (2018). The effect of nonsteroidal anti-inflammatory drugs on bone healing in humans: a qualitative, systematic review. J. Clin. Anesth. 49, 92–100. doi: 10.1016/j.jclinane.2018.06.020
Boulard, L., Dierkes, G., and Ternes, T. (2018). Utilization of large volume zwitterionic hydrophilic interaction liquid chromatography for the analysis of polar pharmaceuticals in aqueous environmental samples: benefits and limitations. J. Chromatogr. A 1535, 27–43. doi: 10.1016/j.chroma.2017.12.023
Braeuer, S., Borovička, J., Kameník, J., Prall, E., Stijve, T., and Goessler, W. (2020). Is arsenic responsible for the toxicity of the hyperaccumulating mushroom Sarcosphaera coronaria? Sci. Total Environ. 736:139524. doi: 10.1016/j.scitotenv.2020.139524
Brain, R. A., Hanson, M. L., Solomon, K. R., and Brooks, B. W. (2008). Aquatic plants exposed to pharmaceuticals: effects and risks. Rev. Environ. Contam. Toxicol. 192, 67–115. doi: 10.1007/978-0-387-71724-1_3
Branchet, P. Ariza Castro, N., Fenet, H., Gomez, E., Courant, F., Sebag, D., et al. (2019). Anthropic impacts on Sub-Saharan urban water resources through their pharmaceutical contamination (Yaoundé, Center Region, Cameroon). Sci. Total Environ. 660, 886–898. doi: 10.1016/j.scitotenv.2018.12.256
Braun, J. M. (2017). Early-life exposure to EDCs: role in childhood obesity and neurodevelopment. Nat. Rev. Endocrinol. 13, 161–173. doi: 10.1038/nrendo.2016.186
Brausch, J. M., Connors, K. A., Brooks, B. W., and Rand, G. M. (2012). Human pharmaceuticals in the aquatic environment: a review of recent toxicological studies and considerations for toxicity testing. Rev. Environ. Contam. Toxicol. 218, 1–99. doi: 10.1007/978-1-4614-3137-4_1
Brown, K. D., Kulis, J., Thomson, B., Chapman, T. H., and Mawhinney, D. B. (2006). Occurrence of antibiotics in hospital, residential, and dairy effluent, municipal wastewater, and the Rio Grande in New Mexico. Sci. Total Environ. 366, 772–783. doi: 10.1016/j.scitotenv.2005.10.007
Buchicchio, A., Bianco, G., Sofo, A., Masi, S., and Caniani, D. (2016). Biodegradation of carbamazepine and clarithromycin by Trichoderma harzianum and Pleurotus ostreatus investigated by liquid chromatography – high-resolution tandem mass spectrometry (FTICR MS-IRMPD). Sci. Total Environ. 55, 733–739. doi: 10.1016/j.scitotenv.2016.03.119
Buelow, E., Bayjanov, J. R., Majoor, E., Willems, R. J. L., Bonten, M. J. M., Schmitt, H., et al. (2018). Limited influence of hospital wastewater on the microbiome and resistome of wastewater in a community sewerage system. FEMS Microbiol. Ecol. 94:fiy087. doi: 10.1093/femsec/fiy087
Cacace, D., Fatta-Kassinos, D., Manaia, C. M., Cytryn, E., Kreuzinger, N., Rizzo, L., et al. (2019). Antibiotic resistance genes in treated wastewater and in the receiving water bodies: a pan-European survey of urban settings. Water Res. 162, 320–330. doi: 10.1016/j.watres.2019.06.039
Cardoso, O., Porcher, J.-M., and Sanchez, W. (2014). Factory-discharged pharmaceuticals could be a relevant source of aquatic environment contamination: review of evidence and need for knowledge. Chemosphere 115, 20–30. doi: 10.1016/j.chemosphere.2014.02.004
Carvalho, I. T., and Santos, L. (2016). Antibiotics in the aquatic environments: a review of the European scenario. Environ. Int. 94, 736–757. doi: 10.1016/j.envint.2016.06.025
Chari, R. V. J. (2008). Targeted cancer therapy: conferring specificity to cytotoxic drugs. Acc. Chem. Res. 41, 98–107. doi: 10.1021/ar700108g
Chatterjee, A., and Abraham, J. (2019). Mycoremediation of 17 β-Estradiol using Trichoderma citrinoviride strain AJAC3 along with enzyme studies. Environ. Prog. Sustain. Energy 38:13142. doi: 10.1002/ep.13142
Chaturvedi, P., Giri, B. S., Shukla, P., and Gupta, P. (2021a). Recent advancement in remediation of synthetic organic antibiotics from environmental matrices: challenges and perspective. Bioresour. Technol. 319:124161. doi: 10.1016/j.biortech.2020.124161
Chaturvedi, P., Shukla, P., Giri, B. S., Chowdhary, P., Chandra, R., Gupta, P., et al. (2021b). Prevalence and hazardous impact of pharmaceutical and personal care products and antibiotics in environment: a review on emerging contaminants. Environ. Res. 194:110664. doi: 10.1016/j.envres.2020.110664
Clara, M., Strenn, B., Gans, O., Martinez, E., Kreuzinger, N., and Kroiss, H. (2005). Removal of selected pharmaceuticals, fragrances and endocrine disrupting compounds in a membrane bioreactor and conventional wastewater treatment plants. Water Res. 39, 4797–4807. doi: 10.1016/j.watres.2005.09.015
Comber, S., Gardner, M., Sörme, P., Leverett, D., and Ellor, B. (2018). Active pharmaceutical ingredients entering the aquatic environment from wastewater treatment works: a cause for concern? Sci. Total Environ. 613–614, 538–547. doi: 10.1016/j.scitotenv.2017.09.101
Copete-Pertuz, L. S., Plácido, J., Serna-Galvis, E. A., Torres-Palma, R. A., and Mora, A. (2018). Elimination of isoxazolyl-penicillins antibiotics in waters by the ligninolytic native colombian strain Leptosphaerulina sp. considerations on biodegradation process and antimicrobial activity removal. Sci. Total Environ. 630, 1195–1204. doi: 10.1016/j.scitotenv.2018.02.244
Courtheyn, D., Le Bizec, B., Brambilla, G., De Brabander, H. F., Cobbaert, E., Van de Wiele, M., et al. (2002). Recent developments in the use and abuse of growth promoters. Anal. Chim. Acta 473, 71–82. doi: 10.1016/S0003-2670(02)00753-5
Crini, G., and Lichtfouse, E. (2019). Advantages and disadvantages of techniques used for wastewater treatment. Environ. Chem. Lett. 17, 145–155. doi: 10.1007/s10311-018-0785-9
Cristóvão, M. B. Bento-Silva, A., Bronze, M. R., Crespo, J. G., and Pereira, V. J. (2021). Detection of anticancer drugs in wastewater effluents: grab versus passive sampling. Sci. Total Environ. 786:147477. doi: 10.1016/j.scitotenv.2021.147477
Cristóvão, M. B., Torrejais, J., Janssens, R., Luis, P., Van der Bruggen, B., Dubey, K. K., et al. (2019). Treatment of anticancer drugs in hospital and wastewater effluents using nanofiltration. Sep. Purif. Technol. 224, 273–280. doi: 10.1016/j.seppur.2019.05.016
Cruz-Morató, C., Ferrando-Climent, L., Rodriguez-Mozaz, S., Barceló, D., Marco-Urrea, E., Vicent, T., et al. (2013). Degradation of pharmaceuticals in non-sterile urban wastewater by Trametes versicolor in a fluidized bed bioreactor. Water Res. 47, 5200–5210. doi: 10.1016/j.watres.2013.06.007
Cruz-Morató, C., Lucas, D., Llorca, M., Rodriguez-Mozaz, S., Gorga, M., Petrovic, M., et al. (2014). Hospital wastewater treatment by fungal bioreactor: removal efficiency for pharmaceuticals and endocrine disruptor compounds. Sci. Total Environ. 493, 365–376. doi: 10.1016/j.scitotenv.2014.05.117
Cuthbert, R., Parry-Jones, J., Green, R. E., and Pain, D. J. (2007). NSAIDs and scavenging birds: potential impacts beyond Asia’s critically endangered vultures. Biol. Lett. 3, 91–94. doi: 10.1098/rsbl.2006.0554
Čvanv̌arová, M., Moeder, M., Filipová, A., and Cajthaml, T. (2015). Biotransformation of fluoroquinolone antibiotics by ligninolytic fungi – metabolites, enzymes and residual antibacterial activity. Chemosphere 136, 311–320. doi: 10.1016/j.chemosphere.2014.12.012
Èvanèarová, M., Moeder, M., Filipová, A., Reemtsma, T., and Cajthaml, T. (2013). Biotransformation of the antibiotic agent flumequine by ligninolytic fungi and residual antibacterial activity of the transformation mixtures. Environ. Sci. Technol. 47, 14128–14136. doi: 10.1021/es403470s
Dąbrowska, M., Muszyńska, B., Starek, M., Żmudzki, P., and Opoka, W. (2018). Degradation pathway of cephalosporin antibiotics by in vitro cultures of Lentinula edodes and Imleria badia. Int. Biodeterior. Biodegrad. 127, 104–112. doi: 10.1016/j.ibiod.2017.11.014
Danner, M.-C., Robertson, A., Behrends, V., and Reiss, J. (2019). Antibiotic pollution in surface fresh waters: occurrence and effects. Sci. Total Environ. 664, 793–804. doi: 10.1016/j.scitotenv.2019.01.406
Daouk, S., Chèvre, N., Vernaz, N., Bonnabry, P., Dayer, P., Daali, Y., et al. (2015). Prioritization methodology for the monitoring of active pharmaceutical ingredients in hospital effluents. J. Environ. Manage. 160, 324–332. doi: 10.1016/j.jenvman.2015.06.037
David, A., and Pancharatna, K. (2009). Developmental anomalies induced by a non-selective COX inhibitor (ibuprofen) in zebrafish (Danio rerio). Environ. Toxicol. Pharmacol. 27, 390–395. doi: 10.1016/j.etap.2009.01.002
De Gusseme, B., Vanhaecke, L., Verstraete, W., and Boon, N. (2011). Degradation of acetaminophen by Delftia tsuruhatensis and Pseudomonas aeruginosa in a membrane bioreactor. Water Res. 45, 1829–1837. doi: 10.1016/j.watres.2010.11.040
Deo, R. P. (2014). Pharmaceuticals in the surface water of the USA: a review. Curr. Environ. Heal. Rep. 1, 113–122. doi: 10.1007/s40572-014-0015-y
Dhangar, K., and Kumar, M. (2020). Tricks and tracks in removal of emerging contaminants from the wastewater through hybrid treatment systems: a review. Sci. Total Environ. 738:140320. doi: 10.1016/j.scitotenv.2020.140320
Domaradzka, D., Guzik, U., and Wojcieszyńska, D. (2015). Biodegradation and biotransformation of polycyclic non-steroidal anti-inflammatory drugs. Rev. Environ. Sci. Biotechnol. 14, 229–239. doi: 10.1007/s11157-015-9364-8
Ebele, A. J., Oluseyi, T., Drage, D. S., and Harrad, S.Abou-Elwafa Abdallah, M. (2020). Occurrence, seasonal variation and human exposure to pharmaceuticals and personal care products in surface water, groundwater and drinking water in Lagos State, Nigeria. Emerg. Contam. 6, 124–132. doi: 10.1016/j.emcon.2020.02.004
Edwards, S. J., and Kjellerup, B. V. (2013). Applications of biofilms in bioremediation and biotransformation of persistent organic pollutants, pharmaceuticals/personal care products, and heavy metals. Appl. Microbiol. Biotechnol. 97, 9909–9921. doi: 10.1007/s00253-013-5216-z
Ekpeghere, K. I., Lee, J.-W., Kim, H.-Y., Shin, S.-K., and Oh, J.-E. (2017). Determination and characterization of pharmaceuticals in sludge from municipal and livestock wastewater treatment plants. Chemosphere 168, 1211–1221. doi: 10.1016/j.chemosphere.2016.10.077
Ekpeghere, K. I., Sim, W.-J., Lee, H.-J., and Oh, J.-E. (2018). Occurrence and distribution of carbamazepine, nicotine, estrogenic compounds, and their transformation products in wastewater from various treatment plants and the aquatic environment. Sci. Total Environ. 640–641, 1015–1023. doi: 10.1016/j.scitotenv.2018.05.218
Esterhuizen-Londt, M., Hendel, A.-L., and Pflugmacher, S. (2017). Mycoremediation of diclofenac using Mucor hiemalis. Toxicol. Environ. Chem. 99, 795–808. doi: 10.1080/02772248.2017.1296444
Esterhuizen-Londt, M., Schwartz, K., Balsano, E., Kühn, S., and Pflugmacher, S. (2016a). LC–MS/MS method development for quantitative analysis of acetaminophen uptake by the aquatic fungus Mucor hiemalis. Ecotoxicol. Environ. Saf. 128, 230–235. doi: 10.1016/j.ecoenv.2016.02.029
Esterhuizen-Londt, M., Schwartz, K., and Pflugmacher, S. (2016b). Using aquatic fungi for pharmaceutical bioremediation: uptake of acetaminophen by Mucor hiemalis does not result in an enzymatic oxidative stress response. Fungal Biol. 120, 1249–1257. doi: 10.1016/j.funbio.2016.07.009
Esterhuizen, M., Behnam Sani, S., Wang, L., Kim, Y. J., and Pflugmacher, S. (2021). Mycoremediation of acetaminophen: culture parameter optimization to improve efficacy. Chemosphere 263:128117. doi: 10.1016/j.chemosphere.2020.128117
Fang, T.-H., Nan, F.-H., Chin, T.-S., and Feng, H.-M. (2012). The occurrence and distribution of pharmaceutical compounds in the effluents of a major sewage treatment plant in Northern Taiwan and the receiving coastal waters. Mar. Pollut. Bull. 64, 1435–1444. doi: 10.1016/j.marpolbul.2012.04.008
Fekadu, S., Alemayehu, E., Dewil, R., and Van der Bruggen, B. (2019). Pharmaceuticals in freshwater aquatic environments: a comparison of the African and European challenge. Sci. Total Environ. 654, 324–337. doi: 10.1016/j.scitotenv.2018.11.072
Ferlay, J., Steliarova-Foucher, E., Lortet-Tieulent, J., Rosso, S., Coebergh, J. W. W., Comber, H., et al. (2013). Cancer incidence and mortality patterns in Europe: estimates for 40 countries in 2012. Eur. J. Cancer 49, 1374–1403. doi: 10.1016/j.ejca.2012.12.027
Ferrari, B., Mons, R., Vollat, B., Fraysse, B., Paxēaus, N., Lo, G. R., et al. (2004). Environmental risk assessment of six human pharmaceuticals: are the current environmental risk assessment procedures sufficient for the protection of the aquatic environment? Environ. Toxicol. Chem. 23, 1344–1354. doi: 10.1897/03-246
Ferreira, T. C. R., Esterhuizen-Londt, M., Zaiat, M., and Pflugmacher, S. (2018). Fate of enrofloxacin in lake sediment: biodegradation, transformation product identification, and ecotoxicological implications. Soil Sediment Contam. Int. J. 27, 357–368. doi: 10.1080/15320383.2018.1478798
Fisher, I. J., Phillips, P. J., Colella, K. M., Fisher, S. C., Tagliaferri, T., Foreman, W. T., et al. (2016). The impact of onsite wastewater disposal systems on groundwater in areas inundated by Hurricane Sandy in New York and New Jersey. Mar. Pollut. Bull. 107, 509–517. doi: 10.1016/j.marpolbul.2016.04.038
Flippin, J. L., Huggett, D., and Foran, C. M. (2007). Changes in the timing of reproduction following chronic exposure to ibuprofen in Japanese medaka, Oryzias latipes. Aquat. Toxicol. 81, 73–78. doi: 10.1016/j.aquatox.2006.11.002
Fonseca, V. F., Duarte, I. A., Duarte, B., Freitas, A., Pouca, A. S. V., Barbosa, J., et al. (2021). Environmental risk assessment and bioaccumulation of pharmaceuticals in a large urbanized estuary. Sci. Total Environ. 783:147021. doi: 10.1016/j.scitotenv.2021.147021
Forte, M., Di Lorenzo, M., Carrizzo, A., Valiante, S., Vecchione, C., Laforgia, V., et al. (2016). Nonylphenol effects on human prostate non tumorigenic cells. Toxicology 357–358, 21–32. doi: 10.1016/j.tox.2016.05.024
Forte, M., Di Lorenzo, M., Iachetta, G., Mita, D. G., Laforgia, V., and De Falco, M. (2019). Nonylphenol acts on prostate adenocarcinoma cells via estrogen molecular pathways. Ecotoxicol. Environ. Saf. 180, 412–419. doi: 10.1016/j.ecoenv.2019.05.035
Freeman, C. N., Scriver, L., Neudorf, K. D., Truelstrup Hansen, L., Jamieson, R. C., and Yost, C. K. (2018). Antimicrobial resistance gene surveillance in the receiving waters of an upgraded wastewater treatment plant. FACETS 3, 128–138. doi: 10.1139/facets-2017-0085
Furlong, E. T., Batt, A. L., Glassmeyer, S. T., Noriega, M. C., Kolpin, D. W., Mash, H., et al. (2017). Nationwide reconnaissance of contaminants of emerging concern in source and treated drinking waters of the United States: pharmaceuticals. Sci. Total Environ. 579, 1629–1642. doi: 10.1016/j.scitotenv.2016.03.128
Gajski, G., Gerić, M., Negura, B., Novak, M., Nunić, J., Bajrektarević, D., et al. (2016). Genotoxic potential of selected cytostatic drugs in human and zebrafish cells. Environ. Sci. Pollut. Res. 23, 14739–14750. doi: 10.1007/s11356-015-4592-6
Gani, K. M., Hlongwa, N., Abunama, T., Kumari, S., and Bux, F. (2021). Emerging contaminants in South African water environment- a critical review of their occurrence, sources and ecotoxicological risks. Chemosphere 269:128737. doi: 10.1016/j.chemosphere.2020.128737
García, J., García-Galán, M. J., Day, J. W., Boopathy, R., White, J. R., Wallace, S., et al. (2020). A review of emerging organic contaminants (EOCs), antibiotic resistant bacteria (ARB), and antibiotic resistance genes (ARGs) in the environment: increasing removal with wetlands and reducing environmental impacts. Bioresour. Technol. 307:123228. doi: 10.1016/j.biortech.2020.123228
Giebułtowicz, J., Tyski, S., Wolinowska, R., Grzybowska, W. Zarȩba, T., Drobniewska, A., et al. (2018). Occurrence of antimicrobial agents, drug-resistant bacteria, and genes in the sewage-impacted Vistula River (Poland). Environ. Sci. Pollut. Res. 25, 5788–5807. doi: 10.1007/s11356-017-0861-x
Gil, A., García, A. M., Fernández, M., Vicente, M. A., González-Rodríguez, B., Rives, V., et al. (2017). Effect of dopants on the structure of titanium oxide used as a photocatalyst for the removal of emergent contaminants. J. Ind. Eng. Chem. 53, 183–191. doi: 10.1016/j.jiec.2017.04.024
Gillings, M. R., Gaze, W. H., Pruden, A., Smalla, K., Tiedje, J. M., and Zhu, Y.-G. (2015). Using the class 1 integron-integrase gene as a proxy for anthropogenic pollution. ISME J. 9, 1269–1279. doi: 10.1038/ismej.2014.226
Godlewska, K., Jakubus, A., Stepnowski, P., and Paszkiewicz, M. (2021). Impact of environmental factors on the sampling rate of β-blockers and sulfonamides from water by a carbon nanotube-passive sampler. J. Environ. Sci. 101, 413–427. doi: 10.1016/j.jes.2020.08.034
Godoy, A. A., Oliveira, ÁC., Silva, J. G. M., Azevedo, C. C. J., Domingues, I., Nogueira, A. J. A., et al. (2019). Single and mixture toxicity of four pharmaceuticals of environmental concern to aquatic organisms, including a behavioral assessment. Chemosphere 235, 373–382. doi: 10.1016/j.chemosphere.2019.06.200
Golveia, J. C. S., Santiago, M. F., Sales, P. T. F., Sartoratto, A., Ponezi, A. N., Thomaz, D. V., et al. (2018). Cupuaçu (Theobroma grandiflorum) residue and its potential application in the bioremediation of 17-A -ethinylestradiol as a Pycnoporus sanguineus laccase inducer. Prep. Biochem. Biotechnol. 48, 541–548. doi: 10.1080/10826068.2018.1466161
González-Alonso, S., Merino, L. M., Esteban, S., López de Alda, M., Barceló, D., Durán, J. J., et al. (2017). Occurrence of pharmaceutical, recreational and psychotropic drug residues in surface water on the northern Antarctic Peninsula region. Environ. Pollut. 229, 241–254. doi: 10.1016/j.envpol.2017.05.060
González-Pleiter, M., Gonzalo, S., Rodea-Palomares, I., Leganés, F., Rosal, R., Boltes, K., et al. (2013). Toxicity of five antibiotics and their mixtures towards photosynthetic aquatic organisms: implications for environmental risk assessment. Water Res. 47, 2050–2064. doi: 10.1016/j.watres.2013.01.020
Gonzalez-Rey, M., and Bebianno, M. J. (2014). Effects of non-steroidal anti-inflammatory drug (NSAID) diclofenac exposure in mussel Mytilus galloprovincialis. Aquat. Toxicol. 148, 221–230. doi: 10.1016/j.aquatox.2014.01.011
Gore, A. C., Crews, D., Doan, L. L. La Merrill, M., Patisaul, H., and Zota, A. (2014). Introduction to Endocrine Disrupting Chemicals (EDCs). A Guide for Public Interest Organizations and Policy-Makers. Washington, DC: Endocrine Society, 21–22.
Gothwal, R., and Shashidhar, T. (2015). Antibiotic pollution in the environment: a review. CLEAN – Soil Air Water 43, 479–489. doi: 10.1002/clen.201300989
Grassi, M., Kaykioglu, G., Belgiorno, V., and Lofrano, G. (2012). “Removal of emerging contaminants from water and wastewater by adsorption process,” in Emerging Compounds Removal From Wastewater: Natural and Solar Based Treatments, ed. G. Lofrano (Dordrecht: Springer Netherlands), 15–37. doi: 10.1007/978-94-007-3916-1_2
Gravel, A., Wilson, J. M., Pedro, D. F. N., and Vijayan, M. M. (2009). Non-steroidal anti-inflammatory drugs disturb the osmoregulatory, metabolic and cortisol responses associated with seawater exposure in rainbow trout. Comp. Biochem. Physiol. Part C Toxicol. Pharmacol. 149, 481–490. doi: 10.1016/j.cbpc.2008.11.002
Grenni, P., Ancona, V., and Barra Caracciolo, A. (2018). Ecological effects of antibiotics on natural ecosystems: a review. Microchem. J. 136, 25–39. doi: 10.1016/j.microc.2017.02.006
Gröger, T. M., Käfer, U., and Zimmermann, R. (2020). Gas chromatography in combination with fast high-resolution time-of-flight mass spectrometry: technical overview and perspectives for data visualization. TrAC Trends Anal. Chem. 122:115677. doi: 10.1016/j.trac.2019.115677
Gros, M., Cruz-Morato, C., Marco-Urrea, E., Longrée, P., Singer, H., Sarrà, M., et al. (2014). Biodegradation of the X-ray contrast agent iopromide and the fluoroquinolone antibiotic ofloxacin by the white rot fungus Trametes versicolor in hospital wastewaters and identification of degradation products. Water Res. 60, 228–241. doi: 10.1016/j.watres.2014.04.042
Gros, M., Marti, E., Balcázar, J. L., Boy-Roura, M., Busquets, A., Colón, J., et al. (2019). Fate of pharmaceuticals and antibiotic resistance genes in a full-scale on-farm livestock waste treatment plant. J. Hazard. Mater. 378:120716. doi: 10.1016/j.jhazmat.2019.05.109
Gros, M., Petrović, M., Ginebreda, A., and Barceló, D. (2010). Removal of pharmaceuticals during wastewater treatment and environmental risk assessment using hazard indexes. Environ. Int. 36, 15–26. doi: 10.1016/j.envint.2009.09.002
Hachi, M., Chergui, A., Yeddou, A. R., Selatnia, A., and Cabana, H. (2017). Removal of acetaminophen and carbamazepine in single and binary systems with immobilized laccase from Trametes hirsuta. Biocatal. Biotransform. 35, 51–62. doi: 10.1080/10242422.2017.1280032
Hamid, H., and Eskicioglu, C. (2012). Fate of estrogenic hormones in wastewater and sludge treatment: a review of properties and analytical detection techniques in sludge matrix. Water Res. 46, 5813–5833. doi: 10.1016/j.watres.2012.08.002
Hanna, N., Sun, P., Sun, Q., Li, X., Yang, X., Ji, X., et al. (2018). Presence of antibiotic residues in various environmental compartments of Shandong province in eastern China: its potential for resistance development and ecological and human risk. Environ. Int. 114, 131–142. doi: 10.1016/j.envint.2018.02.003
Harrabi, M. Varela Della Giustina, S., and Aloulou, F. Rodriguez-Mozaz, S. Barceló, D., and Elleuch, B. (2018). Analysis of multiclass antibiotic residues in urban wastewater in Tunisia. Environ. Nanotechnol. Monit. Manage. 10, 163–170. doi: 10.1016/j.enmm.2018.05.006
Hawkins, T. (2010). Understanding and managing the adverse effects of antiretroviral therapy. Antiviral Res. 85, 201–209. doi: 10.1016/j.antiviral.2009.10.016
Heath, E., Filipiè, M., Kosjek, T., and Isidori, M. (2016). Fate and effects of the residues of anticancer drugs in the environment. Environ. Sci. Pollut. Res. 23, 14687–14691. doi: 10.1007/s11356-016-7069-3
Heindel, J. J., Newbold, R., and Schug, T. T. (2015). Endocrine disruptors and obesity. Nat. Rev. Endocrinol. 11, 653–661. doi: 10.1038/nrendo.2015.163
Hendricks, R., and Pool, E. J. (2012). The effectiveness of sewage treatment processes to remove faecal pathogens and antibiotic residues. J. Environ. Sci. Health A 47, 289–297. doi: 10.1080/10934529.2012.637432
Hoeger, B., Köllner, B., Dietrich, D. R., and Hitzfeld, B. (2005). Water-borne diclofenac affects kidney and gill integrity and selected immune parameters in brown trout (Salmo trutta f. fario). Aquat. Toxicol. 75, 53–64. doi: 10.1016/j.aquatox.2005.07.006
Hu, Y., Jiang, L., Sun, X., Wu, J., Ma, L., Zhou, Y., et al. (2021). Risk assessment of antibiotic resistance genes in the drinking water system. Sci. Total Environ. 800:149650. doi: 10.1016/j.scitotenv.2021.149650
Huggett, D. B., Brooks, B. W., Peterson, B., Foran, C. M., and Schlenk, D. (2002). Toxicity of select beta adrenergic receptor-blocking pharmaceuticals (b-blockers) on aquatic organisms. Arch. Environ. Contam. Toxicol. 43, 229–235. doi: 10.1007/s00244-002-1182-7
Hurtado-Gonzalez, P., Anderson, R. A., Macdonald, J., van den Driesche, S., Kilcoyne, K., Jørgensen, A., et al. (2021). Effects of exposure to acetaminophen and ibuprofen on fetal germ cell development in both sexes in rodent and human using multiple experimental systems. Environ. Health Perspect. 126:47006. doi: 10.1289/EHP2307
Jäger, T., Hembach, N., Elpers, C., Wieland, A., Alexander, J., Hiller, C., et al. (2018). Reduction of antibiotic resistant bacteria during conventional and advanced wastewater treatment, and the disseminated loads released to the environment. Front. Microbiol. 9:2599. doi: 10.3389/fmicb.2018.02599
Jebapriya, G. R., and Gnanadoss, J. J. (2013). Bioremediation of textile dye using white rot fungi: a review. Int. J. Curr. Res. Rev. 5:1.
Jelic, A., Cruz-Morató, C., Marco-Urrea, E., Sarrà, M., Perez, S., Vicent, T., et al. (2012). Degradation of carbamazepine by Trametes versicolor in an air pulsed fluidized bed bioreactor and identification of intermediates. Water Res. 46, 955–964. doi: 10.1016/j.watres.2011.11.063
Jiao, S., and Lu, Y. (2020). Abundant fungi adapt to broader environmental gradients than rare fungi in agricultural fields. Glob. Chang. Biol. 26, 4506–4520. doi: 10.1111/gcb.15130
Jin, H., Yang, D., Wu, P., and Zhao, M. (2022). Environmental occurrence and ecological risks of psychoactive substances. Environ. Int. 158:106970. doi: 10.1016/j.envint.2021.106970
Johnson, A. C., Jürgens, M. D., Williams, R. J., Kümmerer, K., Kortenkamp, A., and Sumpter, J. P. (2008). Do cytotoxic chemotherapy drugs discharged into rivers pose a risk to the environment and human health? An overview and UK case study. J. Hydrol. 348, 167–175. doi: 10.1016/j.jhydrol.2007.09.054
Kairigo, P., Ngumba, E., Sundberg, L.-R., Gachanja, A., and Tuhkanen, T. (2020). Occurrence of antibiotics and risk of antibiotic resistance evolution in selected Kenyan wastewaters, surface waters and sediments. Sci. Total Environ. 720:137580. doi: 10.1016/j.scitotenv.2020.137580
Kaloudas, D., Pavlova, N., and Penchovsky, R. (2021). Phycoremediation of wastewater by microalgae: a review. Environ. Chem. Lett. 19, 2905–2920. doi: 10.1007/s10311-021-01203-0
Kang, A. J., Brown, A. K., Wong, C. S., and Yuan, Q. (2018). Removal of antibiotic sulfamethoxazole by anoxic/anaerobic/oxic granular and suspended activated sludge processes. Bioresour. Technol. 251, 151–157. doi: 10.1016/j.biortech.2017.12.021
Kang, B. R., Kim, S. Y., Kang, M., and Lee, T. K. (2021). Removal of pharmaceuticals and personal care products using native fungal enzymes extracted during the ligninolytic process. Environ. Res. 195:110878. doi: 10.1016/j.envres.2021.110878
Karkman, A., Do, T. T., Walsh, F., and Virta, M. P. J. (2018). Antibiotic-resistance genes in waste water. Trends Microbiol. 26, 220–228. doi: 10.1016/j.tim.2017.09.005
Kebede, T. G., Dube, S., and Nindi, M. M. (2018). Removal of non-steroidal anti-inflammatory drugs (NSAIDs) and carbamazepine from wastewater using water-soluble protein extracted from Moringa stenopetala seeds. J. Environ. Chem. Eng. 6, 3095–3103. doi: 10.1016/j.jece.2018.04.066
Kim, J.-W., Jang, H.-S., Kim, J.-G., Ishibashi, H., Hirano, M., Nasu, K., et al. (2009). Occurrence of pharmaceutical and personal care products (PPCPs) in surface water from Mankyung River, South Korea. J. Health Sci. 55, 249–258. doi: 10.1248/jhs.55.249
Kim, Y., Jung, J., Kim, M., Park, J., Boxall, A. B. A., and Choi, K. (2008). Prioritizing veterinary pharmaceuticals for aquatic environment in Korea. Environ. Toxicol. Pharmacol. 26, 167–176. doi: 10.1016/j.etap.2008.03.006
Kollef, M. H., Bassetti, M., Francois, B., Burnham, J., Dimopoulos, G., Garnacho-Montero, J., et al. (2017). The intensive care medicine research agenda on multidrug-resistant bacteria, antibiotics, and stewardship. Intensive Care Med. 43, 1187–1197. doi: 10.1007/s00134-017-4682-7
Kołodziejska, M., Maszkowska, J., Białk-Bielińska, A., Steudte, S., Kumirska, J., Stepnowski, P., et al. (2013). Aquatic toxicity of four veterinary drugs commonly applied in fish farming and animal husbandry. Chemosphere 92, 1253–1259. doi: 10.1016/j.chemosphere.2013.04.057
K’oreje, K. O., Demeestere, K. De Wispelaere, P., Vergeynst, L., Dewulf, J., and Van Langenhove, H. (2012). From multi-residue screening to target analysis of pharmaceuticals in water: development of a new approach based on magnetic sector mass spectrometry and application in the Nairobi River basin, Kenya. Sci. Total Environ. 437, 153–164. doi: 10.1016/j.scitotenv.2012.07.052
K’oreje, K. O., Kandie, F. J., Vergeynst, L., Abira, M. A. Van Langenhove, H., Okoth, M., et al. (2018). Occurrence, fate and removal of pharmaceuticals, personal care products and pesticides in wastewater stabilization ponds and receiving rivers in the Nzoia Basin, Kenya. Sci. Total Environ. 637–638, 336–348. doi: 10.1016/j.scitotenv.2018.04.331
Kovács, R., Csenki, Z., Bakos, K., Urbányi, B., Horváth, Á, Garaj-Vrhovac, V., et al. (2015). Assessment of toxicity and genotoxicity of low doses of 5-fluorouracil in zebrafish (Danio rerio) two-generation study. Water Res. 77, 201–212. doi: 10.1016/j.watres.2015.03.025
Kovalova, L., Siegrist, H., Singer, H., Wittmer, A., and McArdell, C. S. (2012). Hospital wastewater treatment by membrane bioreactor: performance and efficiency for organic micropollutant elimination. Environ. Sci. Technol. 46, 1536–1545. doi: 10.1021/es203495d
Křesinová, Z., Linhartová, L., Filipová, A., Ezechiáń, M., Mańín, P., and Cajthaml, T. (2018). Biodegradation of endocrine disruptors in urban wastewater using Pleurotus ostreatus bioreactor. N. Biotechnol. 43, 53–61. doi: 10.1016/j.nbt.2017.05.004
Kryczyk-Poprawa, A., Żmudzki, P., Maślanka, A., Piotrowska, J., Opoka, W., and Muszyńska, B. (2019). Mycoremediation of azole antifungal agents using in vitro cultures of Lentinula edodes. 3 Biotech 9:207.
Kümmerer, K. (2009). Antibiotics in the aquatic environment – a review – Part I. Chemosphere 75, 417–434. doi: 10.1016/j.chemosphere.2008.11.086
Kümmerer, K., Al-Ahmad, A., Bertram, B., and Wießler, M. (2000). Biodegradability of antineoplastic compounds in screening tests: influence of glucosidation and of stereochemistry. Chemosphere 40, 767–773. doi: 10.1016/S0045-6535(99)00451-8
Kurade, M. B., Ha, Y.-H., Xiong, J.-Q., Govindwar, S. P., Jang, M., and Jeon, B.-H. (2021). Phytoremediation as a green biotechnology tool for emerging environmental pollution: a step forward towards sustainable rehabilitation of the environment. Chem. Eng. J. 415:129040. doi: 10.1016/j.cej.2021.129040
Letsinger, S., Kay, P., Rodríguez-Mozaz, S., Villagrassa, M., Barceló, D., and Rotchell, J. M. (2019). Spatial and temporal occurrence of pharmaceuticals in UK estuaries. Sci. Total Environ. 678, 74–84. doi: 10.1016/j.scitotenv.2019.04.182
Li, C., Wei, Y., Zhang, S., and Tan, W. (2020). Advanced methods to analyze steroid estrogens in environmental samples. Environ. Chem. Lett. 18, 543–559. doi: 10.1007/s10311-019-00961-2
Lian, L., Yao, B., Hou, S., Fang, J., Yan, S., and Song, W. (2017). Kinetic study of hydroxyl and sulfate radical-mediated oxidation of pharmaceuticals in wastewater effluents. Environ. Sci. Technol. 51, 2954–2962. doi: 10.1021/acs.est.6b05536
Lin, A. Y.-C., and Tsai, Y.-T. (2009). Occurrence of pharmaceuticals in Taiwan’s surface waters: impact of waste streams from hospitals and pharmaceutical production facilities. Sci. Total Environ. 407, 3793–3802. doi: 10.1016/j.scitotenv.2009.03.009
Lin, A. Y.-C., Yu, T.-H., and Lin, C.-F. (2008). Pharmaceutical contamination in residential, industrial, and agricultural waste streams: risk to aqueous environments in Taiwan. Chemosphere 74, 131–141. doi: 10.1016/j.chemosphere.2008.08.027
Lindberg, R., Jarnheimer, P. -Å, Olsen, B., Johansson, M., and Tysklind, M. (2004). Determination of antibiotic substances in hospital sewage water using solid phase extraction and liquid chromatography/mass spectrometry and group analogue internal standards. Chemosphere 57, 1479–1488. doi: 10.1016/j.chemosphere.2004.09.015
Lishman, L., Smyth, S. A., Sarafin, K., Kleywegt, S., Toito, J., Peart, T., et al. (2006). Occurrence and reductions of pharmaceuticals and personal care products and estrogens by municipal wastewater treatment plants in Ontario, Canada. Sci. Total Environ. 367, 544–558. doi: 10.1016/j.scitotenv.2006.03.021
Lister, A. L., and Van Der Kraak, G. J. (2009). Regulation of prostaglandin synthesis in ovaries of sexually-mature zebrafish (Danio rerio). Mol. Reprod. Dev. 76, 1064–1075. doi: 10.1002/mrd.21072
Liu, J., Luo, Q., and Huang, Q. (2016). Removal of 17 β-estradiol from poultry litter via solid state cultivation of lignolytic fungi. J. Clean. Prod. 139, 1400–1407. doi: 10.1016/j.jclepro.2016.09.020
Liu, L., Su, J.-Q., Guo, Y., Wilkinson, D. M., Liu, Z., Zhu, Y.-G., et al. (2018). Large-scale biogeographical patterns of bacterial antibiotic resistome in the waterbodies of China. Environ. Int. 117, 292–299. doi: 10.1016/j.envint.2018.05.023
Lloret, L., Eibes, G., Lú-Chau, T. A., Moreira, M. T., Feijoo, G., and Lema, J. M. (2010). Laccase-catalyzed degradation of anti-inflammatories and estrogens. Biochem. Eng. J. 51, 124–131. doi: 10.1016/j.bej.2010.06.005
López-Serna, R., Jurado, A., Vázquez-Suñé, E., Carrera, J., Petrović, M., and Barceló, D. (2013). Occurrence of 95 pharmaceuticals and transformation products in urban groundwaters underlying the metropolis of Barcelona, Spain. Environ. Pollut. 174, 305–315. doi: 10.1016/j.envpol.2012.11.022
Madikizela, L. M., Ncube, S., and Chimuka, L. (2020). Analysis, occurrence and removal of pharmaceuticals in African water resources: a current status. J. Environ. Manage. 253:109741. doi: 10.1016/j.jenvman.2019.109741
Mamta, S., Bhushan, S., Rana, M. S., Raychaudhuri, S., Simsek, H., and Prajapati, S. K. (2020). “15 - Algae- and bacteria-driven technologies for pharmaceutical remediation in wastewater,” in Removal of Toxic Pollutants Through Microbiological and Tertiary Treatment, ed. T. T. Shah (Amsterdam: Elsevier), 373–408. doi: 10.1016/B978-0-12-821014-7.00015-0
Marcoux, M.-A., Matias, M., Olivier, F., and Keck, G. (2013). Review and prospect of emerging contaminants in waste – Key issues and challenges linked to their presence in waste treatment schemes: general aspects and focus on nanoparticles. Waste Manag. 33, 2147–2156. doi: 10.1016/j.wasman.2013.06.022
Masoner, J. R., Kolpin, D. W., Furlong, E. T., Cozzarelli, I. M., Gray, J. L., and Schwab, E. A. (2014). Contaminants of emerging concern in fresh leachate from landfills in the conterminous United States. Environ. Sci. Process. Impacts 16, 2335–2354. doi: 10.1039/C4EM00124A
Marotta, V., Russo, G., Gambardella, C., Grasso, M., La Sala, D., Chiofalo, M. G., et al. (2019). Human exposure to bisphenol AF and diethylhexylphthalate increases susceptibility to develop differentiated thyroid cancer in patients with thyroid nodules. Chemosphere 218, 885–894. doi: 10.1016/j.chemosphere.2018.11.084
Matongo, S., Birungi, G., Moodley, B., and Ndungu, P. (2015). Occurrence of selected pharmaceuticals in water and sediment of Umgeni River, KwaZulu-Natal, South Africa. Environ. Sci. Pollut. Res. 22, 10298–10308. doi: 10.1007/s11356-015-4217-0
de Jesus Menk, J., do Nascimento, A. I. S., Leite, F. G., de Oliveira, R. A., Jozala, A. F., et al. (2019). Biosorption of pharmaceutical products by mushroom stem waste. Chemosphere 237:124515. doi: 10.1016/j.chemosphere.2019.124515
Migliore, L., Fiori, M., Spadoni, A., and Galli, E. (2012). Biodegradation of oxytetracycline by Pleurotus ostreatus mycelium: a mycoremediation technique. J. Hazard. Mater. 21, 227–232. doi: 10.1016/j.jhazmat.2012.02.056
Minguez, L., Pedelucq, J., Farcy, E., Ballandonne, C., Budzinski, H., and Halm-Lemeille, M.-P. (2016). Toxicities of 48 pharmaceuticals and their freshwater and marine environmental assessment in northwestern France. Environ. Sci. Pollut. Res. 23, 4992–5001. doi: 10.1007/s11356-014-3662-5
Mlunguza, N. Y., Ncube, S., Mahlambi, P. N., Chimuka, L., and Madikizela, L. M. (2020). Determination of selected antiretroviral drugs in wastewater, surface water and aquatic plants using hollow fibre liquid phase microextraction and liquid chromatography - tandem mass spectrometry. J. Hazard. Mater. 382:121067. doi: 10.1016/j.jhazmat.2019.121067
Morelli, K. M., Brown, L. B., and Warren, G. L. (2017). Effect of NSAIDs on recovery from acute skeletal muscle injury: a systematic review and meta-analysis. Am. J. Sports Med. 46, 224–233. doi: 10.1177/0363546517697957
Mosekiemang, T. T., Stander, M. A., and de Villiers, A. (2019). Simultaneous quantification of commonly prescribed antiretroviral drugs and their selected metabolites in aqueous environmental samples by direct injection and solid phase extraction liquid chromatography - tandem mass spectrometry. Chemosphere 220, 983–992. doi: 10.1016/j.chemosphere.2018.12.205
Mtolo, S. P., Mahlambi, P. N., and Madikizela, L. M. (2019). Synthesis and application of a molecularly imprinted polymer in selective solid-phase extraction of efavirenz from water. Water Sci. Technol. 79, 356–365. doi: 10.2166/wst.2019.054
Mukhtar, A., Manzoor, M., Gul, I., Zafar, R., Jamil, H. I., Niazi, A. K., et al. (2020). Phytotoxicity of different antibiotics to rice and stress alleviation upon application of organic amendments. Chemosphere 258:127353. doi: 10.1016/j.chemosphere.2020.127353
Mupatsi, N. (2020). Observed and potential environmental impacts of COVID-19 in Africa. Preprints 2020080442. doi: 10.20944/preprints202008.0442.v1
Muszyńska, B., Dąbrowska, M., Starek, M., Żmudzki, P., Lazur, J., Pytko-Polończyk, J., et al. (2019). Lentinula edodes Mycelium as effective agent for piroxicam mycoremediation. Front. Microbiol. 10:313. doi: 10.3389/fmicb.2019.00313
Muszyńska, B., Żmudzki, P., Lazur, J., Kała, K., Sułkowska-Ziaja, K., and Opoka, W. (2018). Analysis of the biodegradation of synthetic testosterone and 17α-ethynylestradiol using the edible mushroom Lentinula edodes. 3 Biotech 8:424. doi: 10.1007/s13205-018-1458-x
Nadal, A., Quesada, I., Tudurí, E., Nogueiras, R., and Alonso-Magdalena, P. (2017). Endocrine-disrupting chemicals and the regulation of energy balance. Nat. Rev. Endocrinol. 13, 536–546. doi: 10.1038/nrendo.2017.51
Nadimpalli, M. L., Marks, S. J., Montealegre, M. C., Gilman, R. H., Pajuelo, M. J., Saito, M., et al. (2020). Urban informal settlements as hotspots of antimicrobial resistance and the need to curb environmental transmission. Nat. Microbiol. 5, 787–795. doi: 10.1038/s41564-020-0722-0
Nakada, N., Tanishima, T., Shinohara, H., Kiri, K., and Takada, H. (2006). Pharmaceutical chemicals and endocrine disrupters in municipal wastewater in Tokyo and their removal during activated sludge treatment. Water Res. 40, 3297–3303. doi: 10.1016/j.watres.2006.06.039
Nannou, C., Ofrydopoulou, A., Evgenidou, E., Heath, D., Heath, E., and Lambropoulou, D. (2020). Antiviral drugs in aquatic environment and wastewater treatment plants: a review on occurrence, fate, removal and ecotoxicity. Sci. Total Environ. 699:134322. doi: 10.1016/j.scitotenv.2019.134322
Nantaba, F., Wasswa, J., Kylin, H., Palm, W.-U., Bouwman, H., and Kümmerer, K. (2020). Occurrence, distribution, and ecotoxicological risk assessment of selected pharmaceutical compounds in water from Lake Victoria, Uganda. Chemosphere 239:124642. doi: 10.1016/j.chemosphere.2019.124642
Nassour, C., Barton, S. J., Nabhani-Gebara, S., Saab, Y., and Barker, J. (2020). Occurrence of anticancer drugs in the aquatic environment: a systematic review. Environ. Sci. Pollut. Res. 27, 1339–1347. doi: 10.1007/s11356-019-07045-2
Ncube, S., Madikizela, L. M., Chimuka, L., and Nindi, M. M. (2018). Environmental fate and ecotoxicological effects of antiretrovirals: a current global status and future perspectives. Water Res. 145, 231–247. doi: 10.1016/j.watres.2018.08.017
Negreira, N. de Alda, M. L.Barceló, D. (2014). Cytostatic drugs and metabolites in municipal and hospital wastewaters in Spain: filtration, occurrence, and environmental risk. Sci. Total Environ. 497–498, 68–77. doi: 10.1016/j.scitotenv.2014.07.101
Ngumba, E., Gachanja, A., Nyirenda, J., Maldonado, J., and Tuhkanen, T. (2020). Occurrence of antibiotics and antiretroviral drugs in source-separated urine, groundwater, surface water and wastewater in the peri-urban area of Chunga in Lusaka, Zambia. Water SA 46, 278–284. doi: 10.17159/wsa/2020.v46.i2.8243
Ngumba, E., Gachanja, A., and Tuhkanen, T. (2016). Occurrence of selected antibiotics and antiretroviral drugs in Nairobi River Basin, Kenya. Sci. Total Environ. 539, 206–213. doi: 10.1016/j.scitotenv.2015.08.139
Nguyen, L. N., Hai, F. I., Yang, S., Kang, J., Leusch, F. D. L., Roddick, F., et al. (2013). Removal of trace organic contaminants by an MBR comprising a mixed culture of bacteria and white-rot fungi. Bioresour. Technol. 148, 234–241. doi: 10.1016/j.biortech.2013.08.142
Nguyen, L. N., Hai, F. I., Yang, S., Kang, J., Leusch, F. D. L., Roddick, F., et al. (2014). Removal of pharmaceuticals, steroid hormones, phytoestrogens, UV-filters, industrial chemicals and pesticides by Trametes versicolor: role of biosorption and biodegradation. Int. Biodeterior. Biodegrad. 88, 169–175. doi: 10.1016/j.ibiod.2013.12.017
Nie, X.-P., Liu, B.-Y., Yu, H.-J., Liu, W.-Q., and Yang, Y.-F. (2013). Toxic effects of erythromycin, ciprofloxacin and sulfamethoxazole exposure to the antioxidant system in Pseudokirchneriella subcapitata. Environ. Pollut. 172, 23–32. doi: 10.1016/j.envpol.2012.08.013
Ogwugwa, V. H., Oyetibo, G. O., and Amund, O. O. (2021). Taxonomic profiling of bacteria and fungi in freshwater sewer receiving hospital wastewater. Environ. Res. 192:110319. doi: 10.1016/j.envres.2020.110319
Ojemaye, C. Y., and Petrik, L. (2018). Pharmaceuticals in the marine environment: a review. Environ. Rev. 27, 151–165. doi: 10.1139/er-2018-0054
Olaitan, O. J., Okunuga, Y. O., Kasim, L. S., Chimezie, A., and Oderinde, O. (2017). Determination of selected antimalarial pharmaceuticals in water from two hospital environments in Abeokuta Ogun state-Nigeria using SPE-LC. Afr. J. Sci. Nat. 3, 50–56.
Olicón-Hernández, D. R., Camacho-Morales, R. L., Pozo, C., González-López, J., and Aranda, E. (2019). Evaluation of diclofenac biodegradation by the ascomycete fungus Penicillium oxalicum at flask and bench bioreactor scales. Sci. Total Environ. 662, 607–614. doi: 10.1016/j.scitotenv.2019.01.248
Olicón-Hernández, D. R., Gómez-Silván, C., Pozo, C., Andersen, G. L., González-Lopez, J., and Aranda, E. (2021). Penicillium oxalicum XD-3.1 removes pharmaceutical compounds from hospital wastewater and outcompetes native bacterial and fungal communities in fluidised batch bioreactors. Int. Biodeterior. Biodegrad. 158:105179. doi: 10.1016/j.ibiod.2021.105179
Olicón-Hernández, D. R., González-López, J., and Aranda, E. (2017). Overview on the biochemical potential of filamentous fungi to degrade pharmaceutical compounds. Front. Microbiol. 8:1792. doi: 10.3389/fmicb.2017.01792
Olicón-Hernández, D. R., Ortúzar, M., Pozo, C., González-López, J., and Aranda, E. (2020). Metabolic capability of penicillium oxalicum to transform high concentrations of anti-inflammatory and analgesic drugs. Appl. Sci. 10:2479. doi: 10.3390/app10072479
Palanisamy, V., Gajendiran, V., and Mani, K. (2021). Meta-analysis to identify the core microbiome in diverse wastewater. Int. J. Environ. Sci. Technol. 1–18. doi: 10.1007/s13762-021-03349-4
Palli, L., Castellet-Rovira, F., Pérez-Trujillo, M., Caniani, D., Sarrà-Adroguer, M., and Gori, R. (2017). Preliminary evaluation of Pleurotus ostreatus for the removal of selected pharmaceuticals from hospital wastewater. Biotechnol. Prog. 33, 1529–1537. doi: 10.1002/btpr.2520
Pan, M., and Chu, L. M. (2017). Fate of antibiotics in soil and their uptake by edible crops. Sci. Total Environ. 599–600, 500–512. doi: 10.1016/j.scitotenv.2017.04.214
Park, H., and Choi, I.-G. (2020). Genomic and transcriptomic perspectives on mycoremediation of polycyclic aromatic hydrocarbons. Appl. Microbiol. Biotechnol. 104, 6919–6928. doi: 10.1007/s00253-020-10746-1
Parolini, M. (2020). Toxicity of the non-steroidal anti-inflammatory drugs (NSAIDs) acetylsalicylic acid, paracetamol, diclofenac, ibuprofen and naproxen towards freshwater invertebrates: a review. Sci. Total Environ. 740:140043. doi: 10.1016/j.scitotenv.2020.140043
Peng, X., Yu, Y., Tang, C., Tan, J., Huang, Q., and Wang, Z. (2008). Occurrence of steroid estrogens, endocrine-disrupting phenols, and acid pharmaceutical residues in urban riverine water of the Pearl River Delta, South China. Sci. Total Environ. 397, 158–166. doi: 10.1016/j.scitotenv.2008.02.059
Peng, X., Zhang, K., Tang, C., Huang, Q., Yu, Y., and Cui, J. (2011). Distribution pattern, behavior, and fate of antibacterials in urban aquatic environments in South China. J. Environ. Monit. 13, 446–454. doi: 10.1039/C0EM00394H
Pereira, C. D. S., Maranho, L. A., Cortez, F. S., Pusceddu, F. H., Santos, A. R., Ribeiro, D. A., et al. (2016). Occurrence of pharmaceuticals and cocaine in a Brazilian coastal zone. Sci. Total Environ. 548–549, 148–154. doi: 10.1016/j.scitotenv.2016.01.051
Phillips, P. J., Smith, S. G., Kolpin, D. W., Zaugg, S. D., Buxton, H. T., Furlong, E. T., et al. (2010). Pharmaceutical formulation facilities as sources of opioids and other pharmaceuticals to wastewater treatment plant effluents. Environ. Sci. Technol. 44, 4910–4916. doi: 10.1021/es100356f
Pivetta, R. C., Rodrigues-Silva, C., Ribeiro, A. R., and Rath, S. (2020). Tracking the occurrence of psychotropic pharmaceuticals in Brazilian wastewater treatment plants and surface water, with assessment of environmental risks. Sci. Total Environ. 727:138661. doi: 10.1016/j.scitotenv.2020.138661
Prasse, C., Schlüsener, M. P., Schulz, R., and Ternes, T. A. (2010). Antiviral drugs in wastewater and surface waters: a new pharmaceutical class of environmental relevance? Environ. Sci. Technol. 44, 1728–1735. doi: 10.1021/es903216p
Ramírez-Morales, D., Masís-Mora, M., Beita-Sandí, W., Montiel-Mora, J. R., Fernández-Fernández, E., Méndez-Rivera, M., et al. (2021). Pharmaceuticals in farms and surrounding surface water bodies: hazard and ecotoxicity in a swine production area in Costa Rica. Chemosphere 272:129574. doi: 10.1016/j.chemosphere.2021.129574
Ramírez-Morales, D., Masís-Mora, M., Montiel-Mora, J. R., Cambronero-Heinrichs, J. C., Briceño-Guevara, S., Rojas-Sánchez, C. E., et al. (2020). Occurrence of pharmaceuticals, hazard assessment and ecotoxicological evaluation of wastewater treatment plants in Costa Rica. Sci. Total Environ. 746:141200. doi: 10.1016/j.scitotenv.2020.141200
Rao, P. H., Kumar, R. R., and Mohan, N. (2019). “Phycoremediation: role of algae in waste management,” in Environmental Contaminants: Ecological Implications and Management, ed. R. N. Bharagava (Berlin: Springer), 49–82. doi: 10.1007/978-981-13-7904-8_3
Rathi, B. S., Kumar, P. S., and Show, P.-L. (2021). A review on effective removal of emerging contaminants from aquatic systems: current trends and scope for further research. J. Hazard. Mater. 409:124413. doi: 10.1016/j.jhazmat.2020.124413
Reddy, K., Renuka, N., Kumari, S., and Bux, F. (2021). Algae-mediated processes for the treatment of antiretroviral drugs in wastewater: prospects and challenges. Chemosphere 280:130674. doi: 10.1016/j.chemosphere.2021.130674
Reis-Santos, P., Pais, M., Duarte, B., Caçador, I., Freitas, A., Vila Pouca, A. S., et al. (2018). Screening of human and veterinary pharmaceuticals in estuarine waters: a baseline assessment for the Tejo estuary. Mar. Pollut. Bull. 135, 1079–1084. doi: 10.1016/j.marpolbul.2018.08.036
Ren, J., Wang, Z., Deng, L., Niu, D., Huhetaoli, Li, Z., et al. (2021). Degradation of erythromycin by a novel fungus, Penicillium oxalicum RJJ-2, and the degradation pathway. Waste Biomass Valorization 12, 4513–4523. doi: 10.1007/s12649-021-01343-y
Reyes, N. J. D. G., Geronimo, F. K. F., Yano, K. A. V., Guerra, H. B., and Kim, L.-H. (2021). Pharmaceutical and personal care products in different matrices: occurrence, pathways, and treatment processes. Water 13:1159. doi: 10.3390/w13091159
Rimayi, C., Odusanya, D., Weiss, J. M., de Boer, J., and Chimuka, L. (2018). Contaminants of emerging concern in the Hartbeespoort Dam catchment and the uMngeni River estuary 2016 pollution incident, South Africa. Sci. Total Environ. 627, 1008–1017. doi: 10.1016/j.scitotenv.2018.01.263
Rivera-Jaimes, J. A., Postigo, C., Melgoza-Alemán, R. M., Aceña, J., Barceló, D., and López de Alda, M. (2018). Study of pharmaceuticals in surface and wastewater from Cuernavaca, Morelos, Mexico: occurrence and environmental risk assessment. Sci. Total Environ. 613–614, 1263–1274. doi: 10.1016/j.scitotenv.2017.09.134
Roberts, P. H., and Thomas, K. V. (2006). The occurrence of selected pharmaceuticals in wastewater effluent and surface waters of the lower Tyne catchment. Sci. Total Environ. 356, 143–153. doi: 10.1016/j.scitotenv.2005.04.031
Rodarte-Morales, A. I., Feijoo, G., Moreira, M. T., and Lema, J. M. (2012a). Biotransformation of three pharmaceutical active compounds by the fungus Phanerochaete chrysosporium in a fed batch stirred reactor under air and oxygen supply. Biodegradation 23, 145–156. doi: 10.1007/s10532-011-9494-9
Rodarte-Morales, A. I., Feijoo, G., Moreira, M. T., and Lema, J. M. (2012b). Operation of stirred tank reactors (STRs) and fixed-bed reactors (FBRs) with free and immobilized Phanerochaete chrysosporium for the continuous removal of pharmaceutical compounds. Biochem. Eng. J. 66, 38–45. doi: 10.1016/j.bej.2012.04.011
Rodriguez-Mozaz, S. Vaz-Moreira, I. Varela Della Giustina, S., Llorca, M. Barceló, D., Schubert, S., et al. (2020). Antibiotic residues in final effluents of European wastewater treatment plants and their impact on the aquatic environment. Environ. Int. 140:105733. doi: 10.1016/j.envint.2020.105733
Rouches, E., Herpoël-Gimbert, I., Steyer, J. P., and Carrere, H. (2016). Improvement of anaerobic degradation by white-rot fungi pretreatment of lignocellulosic biomass: a review. Renew. Sustain. Energy Rev. 59, 179–198. doi: 10.1016/j.rser.2015.12.317
Rowney, N. C., Johnson, A. C., and Williams, R. J. (2009). Cytotoxic drugs in drinking water: a prediction and risk assessment exercise for the thames catchment in the United Kingdom. Environ. Toxicol. Chem. 28, 2733–2743. doi: 10.1897/09-067.1
Ruan, Y., Lin, H., Zhang, X., Wu, R., Zhang, K., Leung, K. M. Y., et al. (2020). Enantiomer-specific bioaccumulation and distribution of chiral pharmaceuticals in a subtropical marine food web. J. Hazard. Mater. 394:122589. doi: 10.1016/j.jhazmat.2020.122589
Russo, D., Siciliano, A., Guida, M., Andreozzi, R., Reis, N. M., Li Puma, G., et al. (2018). Removal of antiretroviral drugs stavudine and zidovudine in water under UV254 and UV254/H2O2 processes: quantum yields, kinetics and ecotoxicology assessment. J. Hazard. Mater. 349, 195–204. doi: 10.1016/j.jhazmat.2018.01.052
Santos, J. L., Aparicio, I., and Alonso, E. (2007). Occurrence and risk assessment of pharmaceutically active compounds in wastewater treatment plants. a case study: Seville city (Spain). Environ. Int. 33, 596–601. doi: 10.1016/j.envint.2006.09.014
Santos, L. H. M. L., Araújo, A. N., Fachini, A., Pena, A., Delerue-Matos, C., and Montenegro, M. C. B. S. M. (2010). Ecotoxicological aspects related to the presence of pharmaceuticals in the aquatic environment. J. Hazard. Mater. 175, 45–95. doi: 10.1016/j.jhazmat.2009.10.100
Santos, L. H. M. L. M., Rodríguez-Mozaz, S., and Barceló, D. (2021). Microplastics as vectors of pharmaceuticals in aquatic organisms – an overview of their environmental implications. Case Stud. Chem. Environ. Eng. 3: 100079. doi: 10.1016/j.cscee.2021.100079
Schlüsener, M. P., Hardenbicker, P., Nilson, E., Schulz, M., Viergutz, C., and Ternes, T. A. (2015). Occurrence of venlafaxine, other antidepressants and selected metabolites in the Rhine catchment in the face of climate change. Environ. Pollut. 196, 247–256. doi: 10.1016/j.envpol.2014.09.019
Schwaiger, J., Ferling, H., Mallow, U., Wintermayr, H., and Negele, R. D. (2004). Toxic effects of the non-steroidal anti-inflammatory drug diclofenac: part I: histopathological alterations and bioaccumulation in rainbow trout. Aquat. Toxicol. 68, 141–150. doi: 10.1016/j.aquatox.2004.03.014
Segura, P. A., Takada, H., Correa, J. A. El Saadi, K., Koike, T. Onwona-Agyeman, S., et al. (2015). Global occurrence of anti-infectives in contaminated surface waters: impact of income inequality between countries. Environ. Int. 80, 89–97. doi: 10.1016/j.envint.2015.04.001
Shah, A., and Shah, M. (2020). Characterisation and bioremediation of wastewater: a review exploring bioremediation as a sustainable technique for pharmaceutical wastewater. Groundw. Sustain. Dev. 11:100383. doi: 10.1016/j.gsd.2020.100383
Shao, B., Liu, Z., Zeng, G., Liu, Y., Yang, X., Zhou, C., et al. (2019). Immobilization of laccase on hollow mesoporous carbon nanospheres: noteworthy immobilization, excellent stability and efficacious for antibiotic contaminants removal. J. Hazard. Mater. 362, 318–326. doi: 10.1016/j.jhazmat.2018.08.069
Sim, W.-J., Lee, J.-W., Lee, E.-S., Shin, S.-K., Hwang, S.-R., and Oh, J.-E. (2011). Occurrence and distribution of pharmaceuticals in wastewater from households, livestock farms, hospitals and pharmaceutical manufactures. Chemosphere 82, 179–186. doi: 10.1016/j.chemosphere.2010.10.026
Stadlmair, L. F., Letzel, T., Drewes, J. E., and Grassmann, J. (2018). Enzymes in removal of pharmaceuticals from wastewater: a critical review of challenges, applications and screening methods for their selection. Chemosphere 205, 649–661. doi: 10.1016/j.chemosphere.2018.04.142
Sui, Q., Cao, X., Lu, S., Zhao, W., Qiu, Z., and Yu, G. (2015). Occurrence, sources and fate of pharmaceuticals and personal care products in the groundwater: a review. Emerg. Contam. 1, 14–24. doi: 10.1016/j.emcon.2015.07.001
Svobodníková, L., Kummerová, M., Zezulka, Š, Babula, P., and Sendecká, K. (2020). Root response in Pisum sativum under naproxen stress: morpho-anatomical, cytological, and biochemical traits. Chemosphere 258:127411. doi: 10.1016/j.chemosphere.2020.127411
Świacka, K., Michnowska, A., Maculewicz, J., Caban, M., and Smolarz, K. (2021). Toxic effects of NSAIDs in non-target species: a review from the perspective of the aquatic environment. Environ. Pollut. 273:115891. doi: 10.1016/j.envpol.2020.115891
Tahrani, L. Van Loco, J., Anthonissen, R., Verschaeve, L. Ben Mansour, H., and Reyns, T. (2017). Identification and risk assessment of human and veterinary antibiotics in the wastewater treatment plants and the adjacent sea in Tunisia. Water Sci. Technol. 76, 3000–3021. doi: 10.2166/wst.2017.465
Tan, B. L. L., Hawker, D. W. Müller, J. F., Leusch, F. D. L., Tremblay, L. A., and Chapman, H. F. (2007). Modelling of the fate of selected endocrine disruptors in a municipal wastewater treatment plant in South East Queensland, Australia. Chemosphere 69, 644–654. doi: 10.1016/j.chemosphere.2007.02.057
Tanoue, R., Margiotta-Casaluci, L., Huerta, B., Runnalls, T. J., Eguchi, A., Nomiyama, K., et al. (2019). Protecting the environment from psychoactive drugs: problems for regulators illustrated by the possible effects of tramadol on fish behaviour. Sci. Total Environ. 664, 915–926. doi: 10.1016/j.scitotenv.2019.02.090
Ternes, T. A. (1998). Occurrence of drugs in German sewage treatment plants and rivers. Water Res. 32, 3245–3260. doi: 10.1016/S0043-1354(98)00099-2
Ternes, T. (2001). “Pharmaceuticals and metabolites as contaminants of the aquatic environment,” in Pharmaceuticals and Care Products in the Environment ACS Symposium Series, ed. C. G. Daughton (Washington, DC: American Chemical Society), 2–39. doi: 10.1021/bk-2001-0791.ch002
Thibaut, R., Schnell, S., and Porte, C. (2006). The interference of pharmaceuticals with endogenous and xenobiotic metabolizing enzymes in carp liver: an in-vitro study. Environ. Sci. Technol. 40, 5154–5160. doi: 10.1021/es0607483
Tijani, J. O., Fatoba, O. O., Babajide, O. O., and Petrik, L. F. (2016). Pharmaceuticals, endocrine disruptors, personal care products, nanomaterials and perfluorinated pollutants: a review. Environ. Chem. Lett. 14, 27–49. doi: 10.1007/s10311-015-0537-z
Tińma, M., Nnidarńič-Plazl, P., Ńelo, G., Tolj, I., Ńperanda, M., Bucić-Kojić, A., et al. (2021). Trametes versicolor in lignocellulose-based bioeconomy: state of the art, challenges and opportunities. Bioresour. Technol. 330:124997. doi: 10.1016/j.biortech.2021.124997
Tixier, C., Singer, H. P., and Oellers, S.Müller, S. R. (2003). Occurrence and fate of carbamazepine, clofibric acid, diclofenac, ibuprofen, ketoprofen, and naproxen in surface waters. Environ. Sci. Technol. 37, 1061–1068. doi: 10.1021/es025834r
Tompsett, A. (2020). The lazarus drug: the impact of antiretroviral therapy on economic growth. J. Dev. Econ. 143:102409. doi: 10.1016/j.jdeveco.2019.102409
Tran, N. H., Urase, T., and Ta, T. T. (2014). A preliminary study on the occurrence of pharmaceutically active compounds in hospital wastewater and surface water in Hanoi, Vietnam. Clean Soil Air Water 42, 267–275. doi: 10.1002/clen.201300021
Triebskorn, R., Casper, H., Heyd, A., Eikemper, R., Köhler, H.-R., and Schwaiger, J. (2004). Toxic effects of the non-steroidal anti-inflammatory drug diclofenac: Part II. Cytological effects in liver, kidney, gills and intestine of rainbow trout (Oncorhynchus mykiss). Aquat. Toxicol. 68, 151–166. doi: 10.1016/j.aquatox.2004.03.015
Valcárcel, Y., González Alonso, S., Rodríguez-Gil, J. L., Gil, A., and Catalá, M. (2011). Detection of pharmaceutically active compounds in the rivers and tap water of the Madrid Region (Spain) and potential ecotoxicological risk. Chemosphere 84, 1336–1348. doi: 10.1016/j.chemosphere.2011.05.014
Valdez-Carrillo, M., Abrell, L., Ramírez-Hernández, J., Reyes-López, J. A., and Carreón-Diazconti, C. (2020). Pharmaceuticals as emerging contaminants in the aquatic environment of Latin America: a review. Environ. Sci. Pollut. Res. 27, 44863–44891. doi: 10.1007/s11356-020-10842-9
Vasiliadou, I. A., Molina, R., Pariente, M. I., Christoforidis, K. C., Martinez, F., and Melero, J. A. (2019). Understanding the role of mediators in the efficiency of advanced oxidation processes using white-rot fungi. Chem. Eng. J. 359, 1427–1435. doi: 10.1016/j.cej.2018.11.035
Vaudreuil, M.-A., Vo Duy, S., Munoz, G., and Furtos, A.Sauvé, S. (2020). A framework for the analysis of polar anticancer drugs in wastewater: on-line extraction coupled to HILIC or reverse phase LC-MS/MS. Talanta 220:121407. doi: 10.1016/j.talanta.2020.121407
Vergeynst, L., Haeck, A. De Wispelaere, P. Van Langenhove, H., and Demeestere, K. (2015). Multi-residue analysis of pharmaceuticals in wastewater by liquid chromatography-magnetic sector mass spectrometry: method quality assessment and application in a Belgian case study. Chemosphere 119, S2–S8. doi: 10.1016/j.chemosphere.2014.03.069
Verlicchi, P., Galletti, A., Petrovic, M., and Barceló, D. (2010). Hospital effluents as a source of emerging pollutants: an overview of micropollutants and sustainable treatment options. J. Hydrol. 389, 416–428. doi: 10.1016/j.jhydrol.2010.06.005
Vidal-Dorsch, D. E., Bay, S. M., Maruya, K., Snyder, S. A., Trenholm, R. A., and Vanderford, B. J. (2012). Contaminants of emerging concern in municipal wastewater effluents and marine receiving water. Environ. Toxicol. Chem. 31, 2674–2682. doi: 10.1002/etc.2004
Vieira, W. T., de Farias, M. B., Spaolonzi, M. P., da Silva, M. G. C., and Vieira, M. G. A. (2020). Removal of endocrine disruptors in waters by adsorption, membrane filtration and biodegradation. A review. Environ. Chem. Lett. 18, 1113–1143. doi: 10.1007/s10311-020-01000-1
Vilvert, E., Contardo-Jara, V., Esterhuizen-Londt, M., and Pflugmacher, S. (2017). The effect of oxytetracycline on physiological and enzymatic defense responses in aquatic plant species Egeria densa, Azolla caroliniana, and Taxiphyllum barbieri. Toxicol. Environ. Chem. 99, 104–116. doi: 10.1080/02772248.2016.1165817
Wang, S., Ma, X., Liu, Y., Yi, X., Du, G., and Li, J. (2020). Fate of antibiotics, antibiotic-resistant bacteria, and cell-free antibiotic-resistant genes in full-scale membrane bioreactor wastewater treatment plants. Bioresour. Technol. 302:122825. doi: 10.1016/j.biortech.2020.122825
Wang, Z., Du, Y., Yang, C., Liu, X., Zhang, J., Li, E., et al. (2017). Occurrence and ecological hazard assessment of selected antibiotics in the surface waters in and around Lake Honghu, China. Sci. Total Environ. 609, 1423–1432. doi: 10.1016/j.scitotenv.2017.08.009
Wijaya, L., Alyemeni, M., Ahmad, P., Alfarhan, A., Barcelo, D., El-Sheikh, M. A., et al. (2020). Ecotoxicological effects of ibuprofen on plant growth of Vigna unguiculata L. Plants 9:1473. doi: 10.3390/plants9111473
Willyard, C. (2017). The drug-resistant bacteria that pose the greatest health threats. Nature 543:15. doi: 10.1038/nature.2017.21550
Wojcieszyńska, D., Domaradzka, D., Hupert-Kocurek, K., and Guzik, U. (2014). Bacterial degradation of naproxen – undisclosed pollutant in the environment. J. Environ. Manage. 145, 157–161. doi: 10.1016/j.jenvman.2014.06.023
Wollenberger, L., Halling-Sørensen, B., and Kusk, K. O. (2000). Acute and chronic toxicity of veterinary antibiotics to Daphnia magna. Chemosphere 40, 723–730. doi: 10.1016/S0045-6535(99)00443-9
Wu, J., Qian, X., Yang, Z., and Zhang, L. (2010). Study on the matrix effect in the determination of selected pharmaceutical residues in seawater by solid-phase extraction and ultra-high-performance liquid chromatography-electrospray ionization low-energy collision-induced dissociation tandem mass spectrometry. J. Chromatogr. A 1217, 1471–1475. doi: 10.1016/j.chroma.2009.12.074
Wu, S., Zhang, L., and Chen, J. (2012). Paracetamol in the environment and its degradation by microorganisms. Appl. Microbiol. Biotechnol. 96, 875–884. doi: 10.1007/s00253-012-4414-4
Xia, L., Zheng, L., and Zhou, J. L. (2017). Effects of ibuprofen, diclofenac and paracetamol on hatch and motor behavior in developing zebrafish (Danio rerio). Chemosphere 182, 416–425. doi: 10.1016/j.chemosphere.2017.05.054
Xu, S., Yao, J., Ainiwaer, M., Hong, Y., and Zhang, Y. (2018). Analysis of bacterial community structure of activated sludge from wastewater treatment plants in winter. Biomed Res. Int. 2018:8278970. doi: 10.1155/2018/8278970
Yadav, A., Rene, E. R., Mandal, M. K., and Dubey, K. K. (2021). Threat and sustainable technological solution for antineoplastic drugs pollution: review on a persisting global issue. Chemosphere 263:128285. doi: 10.1016/j.chemosphere.2020.128285
Yan, J., Lin, W., Gao, Z., and Ren, Y. (2021). Use of selected NSAIDs in Guangzhou and other cities in the world as identified by wastewater analysis. Chemosphere 279:130529. doi: 10.1016/j.chemosphere.2021.130529
Yang, S., Hai, F. I., Nghiem, L. D., Nguyen, L. N., Roddick, F., and Price, W. E. (2013). Removal of bisphenol A and diclofenac by a novel fungal membrane bioreactor operated under non-sterile conditions. Int. Biodeterior. Biodegrad. 85, 483–490. doi: 10.1016/j.ibiod.2013.03.012
Yang, Y., Fu, J., Peng, H., Hou, L., Liu, M., and Zhou, J. L. (2011). Occurrence and phase distribution of selected pharmaceuticals in the Yangtze Estuary and its coastal zone. J. Hazard. Mater. 190, 588–596. doi: 10.1016/j.jhazmat.2011.03.092
Yu, J. T., Bouwer, E. J., and Coelhan, M. (2006). Occurrence and biodegradability studies of selected pharmaceuticals and personal care products in sewage effluent. Agric. Water Manage. 86, 72–80. doi: 10.1016/j.agwat.2006.06.015
Yu, Y., Wu, L., and Chang, A. C. (2013). Seasonal variation of endocrine disrupting compounds, pharmaceuticals and personal care products in wastewater treatment plants. Sci. Total Environ. 442, 310–316. doi: 10.1016/j.scitotenv.2012.10.001
Zafar, R., Bashir, S., Nabi, D., and Arshad, M. (2021). Occurrence and quantification of prevalent antibiotics in wastewater samples from Rawalpindi and Islamabad, Pakistan. Sci. Total Environ. 764:142596. doi: 10.1016/j.scitotenv.2020.142596
Zainab, S. M., Junaid, M., Xu, N., and Malik, R. N. (2020). Antibiotics and antibiotic resistant genes (ARGs) in groundwater: a global review on dissemination, sources, interactions, environmental and human health risks. Water Res. 187:116455. doi: 10.1016/j.watres.2020.116455
Zhang, Q.-Q., Ying, G.-G., Pan, C.-G., Liu, Y.-S., and Zhao, J.-L. (2015). Comprehensive evaluation of antibiotics emission and fate in the river basins of china: source analysis, multimedia modeling, and linkage to bacterial resistance. Environ. Sci. Technol. 49, 6772–6782. doi: 10.1021/acs.est.5b00729
Zhang, Y., Duan, L., Wang, B., Liu, C. S., Jia, Y., Zhai, N., et al. (2020). Efficient multiresidue determination method for 168 pharmaceuticals and metabolites: optimization and application to raw wastewater, wastewater effluent, and surface water in Beijing, China. Environ. Pollut. 261:114113. doi: 10.1016/j.envpol.2020.114113
Zhang, Y., and Geißen, S.-U. (2012). Elimination of carbamazepine in a non-sterile fungal bioreactor. Bioresour. Technol. 112, 221–227. doi: 10.1016/j.biortech.2012.02.073
Keywords: pharmaceutical active compounds, bioremediation, wastewater, mycoremediation, emerging contaminants, pharmaceutical pollution
Citation: Ortúzar M, Esterhuizen M, Olicón-Hernández DR, González-López J and Aranda E (2022) Pharmaceutical Pollution in Aquatic Environments: A Concise Review of Environmental Impacts and Bioremediation Systems. Front. Microbiol. 13:869332. doi: 10.3389/fmicb.2022.869332
Received: 04 February 2022; Accepted: 30 March 2022;
Published: 26 April 2022.
Edited by:
Muhammad Bilal, Huaiyin Institute of Technology, ChinaReviewed by:
Sandeep Kumar Singh, Centre of Bio-Medical Research (CBMR), IndiaM. Kamaraj, Addis Ababa Science and Technology University, Ethiopia
Copyright © 2022 Ortúzar, Esterhuizen, Olicón-Hernández, González-López and Aranda. This is an open-access article distributed under the terms of the Creative Commons Attribution License (CC BY). The use, distribution or reproduction in other forums is permitted, provided the original author(s) and the copyright owner(s) are credited and that the original publication in this journal is cited, in accordance with accepted academic practice. No use, distribution or reproduction is permitted which does not comply with these terms.
*Correspondence: Maranda Esterhuizen, bWFyYW5kYS5lc3Rlcmh1aXplbkBoZWxzaW5raS5maQ==
†These authors have contributed equally to this work