- 1Consejo Nacional de Humanidades, Ciencias y Tecnologías, Mexico City, Mexico
- 2Instituto de Ciencias del Mar y Limnología, Universidad Nacional Autonoma de Mexico, Mexico City, Mexico
The intricate relationship between prokaryotic vitamin B12 (cobalamin) producers and metazoans in deep-sea ecosystems, particularly within ferromanganese crusts and polymetallic nodules, is critical for understanding oceanic biogeochemical cycling of cobalt. Microbial communities are key regulators of essential biogeochemical cycles, with cobalt serving as a vital component in the synthesis of cobalamin, a metallocofactor indispensable for numerous metabolic processes. We analyzed the significance of cobalamin biosynthetic pathways confined to prokaryotes and emphasized the ecological importance of auxotrophic organisms that rely on exogenous sources of vitamin B12. Additionally, we recognize recent research regarding the spatial distribution of dissolved cobalt and its consequential effects on cobalamin production and bioavailability, indicating the scarcity of cobalt and cobalamin in marine environments. We propose that cobalt-rich environments may foster unique interactions between prokaryotic and eukaryotic organisms, potentially altering the food web dynamics owing to the localized abundance of this element. By investigating the roles of cobalt and cobalamin in nutrient cycling and interspecies interactions, we outlined key criteria for future research on deep-sea microbial communities and their contributions to the cobalt biogeochemical cycle.
1 Introduction
Microbial communities represent most of the ocean’s diversity and are of fundamental importance in maintaining the functionality and stability of global ocean ecosystems. Microorganisms, while not strictly essential for survival, play a pivotal role in regulating marine C, N, P, and S biogeochemical cycles. However, trace elements (<0.1 μM) are also required for growth and are interlinked with the global biogeochemical cycles of macro and microelements (Giovannelli, 2023). One such example is cobalt (Co), as cobalt and iron cycles are co-regulated by phytoplankton, as they require both nutrients for optimal growth, and the availability of one can influence the utilization of the other (Chmiel et al., 2022).
In addition, Co is used for numerous metabolic functions, including non-corrin Co-containing enzymes such as methionine aminopeptidase, prolidase, nitrile hydratase, glucose isomerase, methylmalonyl-CoA carboxytransferase, aldehyde decarbonylase, lysine-2,3-aminomutase, and bromoperoxidase (Okamoto and Eltis, 2011).
We focused on cobalt as a central constituent of the corrin cofactor cobalamin or vitamin B12 present in early life as methanogenesis (Buan, 2018) and globally as phytoplankton, particularly cyanobacteria, which have an absolute requirement for cobalamin (Chmiel et al., 2022). Cobalamin has been demonstrated to be of crucial importance in trophic networks from several environments, including marine systems. Consequently, Co can limit certain metabolic pathways, potentially restricting the metabolic niches of cobalamin synthesis and auxotrophy, and incentivizing ecological relationships linked to the acquisition of vitamin B12, such as predation and symbiosis.
The sensitivity of trophic networks to Co has been studied in Co-depleted systems with nutrient amendments. In this regard, we propose to study sites naturally characterized by a high abundance of cobalt. We specifically refer to ferromanganese nodules and cobalt-rich crusts, which are mineral deposits found on the deep ocean floor that are enriched in metals, such as manganese, iron, copper, nickel, and cobalt. These mineral concretions are recognized as important habitats that support a unique deep-sea biodiversity (Rabone et al., 2023).
We review the importance of understanding the sources and delivery pathways of vitamin B12 in marine ecosystems, particularly those linked to polymetallic nodules and cobalt crusts, considering that the bioavailability of cobalt may regulate its use in different life forms. This allowed us to gain new insights into the biogeochemical cycling of Co in deep-sea ecosystems.
2 Cobalamin: structure, biosynthesis and ecological significance
2.1 Cobalamin is a corrinoid with cobalt
Coenzyme B12 is the only vitamin that contains metal ions; therefore, it is an organometallic cofactor. It also has the most complex structure and largest formula weight, with a chemical structure characterized by a tetrapyrrole with a central Co atom (Figure 1). Cobalamin is derived from the same porphyrin precursor as heme and chlorophyll and the F420 coenzyme. It has the most complex structure of any biological cofactor (Bryant et al., 2020) and contains a tetrapyrrole corrin ring surrounding a central Co atom with an oxidation state of 3+. The fifth coordinated position of Co is occupied by a dimethyl-benzimidazole nucleotide loop, and the sixth catalytic upper ligand position is occupied by either a methyl group or deoxyadenosine, leading to methylcobalamin (MeCbl) or deoxyadenosylcobalamin (AdoCbl), respectively (Doxey et al., 2015; Figure 1).
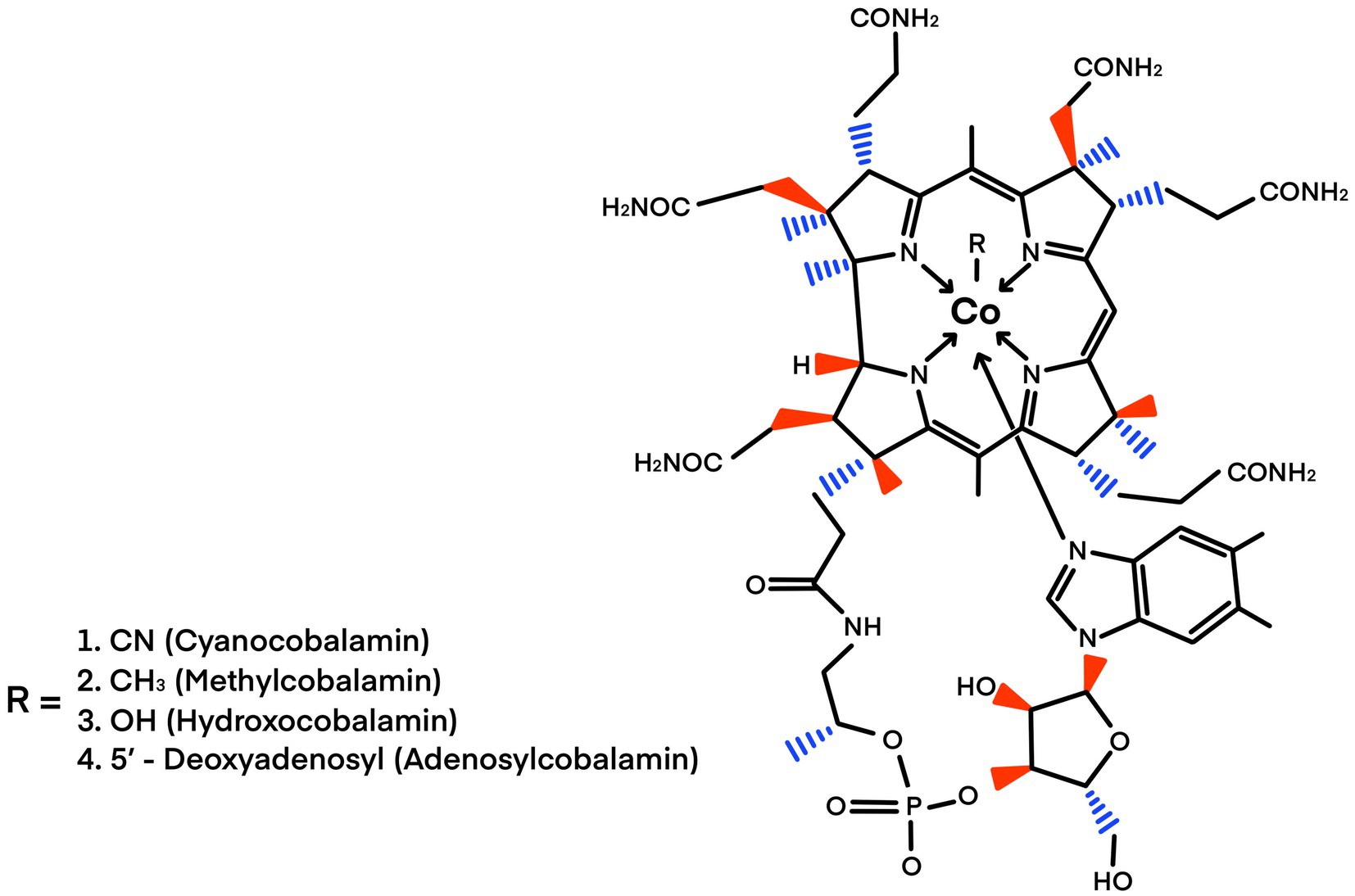
Figure 1. Structure of cobalamin. The cobalt ion is coordinated equally to the four pyrrolic nitrogen atoms of the corrin macrocycle. Cobalamines differ in their sixth ligand (R −). The sixth ligand differentiates between the cofactors cyanocobalamin, methylcobalamin, hydroxocobalamin, and adenosylcobalamin. Modified from Buckel (2007). Credit design: L. Montoya and C. F. Franco-Rodríguez.
The main driving force for the utilization of Co is the chemistry of this transition metal. The essential ability of Co is to form metal-carbon bonds, which is facilitated by its powerful nucleophilicity. Three cobalt oxidation states participate in the functioning of the B12 coenzyme: Co+, Co2+, and Co3+ (Okamoto and Eltis, 2011), each in a coordinated manner and a spatial arrangement facilitated by conformational rearrangements in the corrin ring (Osman et al., 2021).
2.2 Biosynthetic pathways for cobalamin
There are essentially two alternative cobalamin biosynthetic routes in bacteria and archaea, aerobic and anaerobic. These routes differ primarily in the contraction stage of the macrocycle: (a) the late insertion pathway is an aerobic pathway because cobalt is inserted into the tetrapyrrole macrocycle after ring contraction, where molecular oxygen is a prerequisite; and (b) the early insertion pathway can operate under anaerobic conditions, where cobalt is chelated before ring contraction (Scott and Roessner, 2002). Despite the differences in the timing of cobalt insertion and the mechanism of ring contraction, many other enzymes associated with methylation and amidations operate in the same order along the pathway (Osman et al., 2021).
In natural ecosystems, B12 biosynthesis is energetically expensive and imposes a high metabolic burden on the B12 producers. The cobalamin biosynthetic pathway (aerobic or anaerobic) is one of the most complex pathways in nature, requiring approximately 20 enzymes for complete de novo synthesis, starting with cobalt (Co2+) (Warren et al., 2002). Cofactors are often imported by cobalamin producers, a strategy that is energetically more favorable than biosynthesis (Nijland et al., 2022).
2.3 Metabolic processes dependent on cobalamin
Coenzyme B12 or cobalamin-dependent enzymes are mainly included in the following groups: (a) transferases (E.C. 2.x.x.x), such as methylcobalamin (MeCbl)-dependent transferases and (b) isomerases (E.C. 5.x.x.x), such as adenosylcobalamin (AdoCbl) deoxyadenosyl cobalamin-dependent isomerase (Table 1). These catalysts are present in both prokaryotes and eukaryotes and reductive dehalogenases (E.C. 1.x.x.x) are found only in organohalide-respiring bacteria (Giedyk et al., 2015).
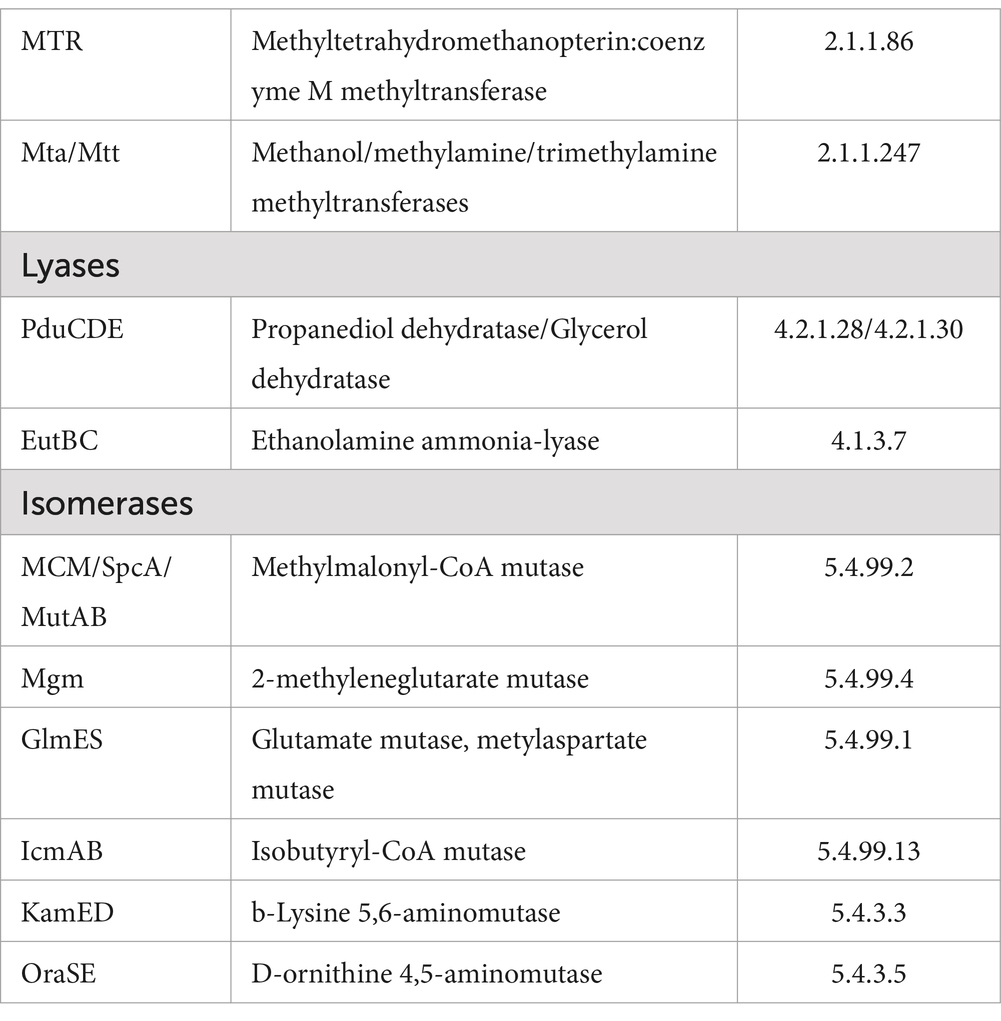
Table 1. Metabolism processes linked to cobalamin are denoted as cobalamin-dependent enzymes (Buckel, 2007; Degnan et al., 2014).
MeCbl serves as the intermediate carrier of activated methyl groups. During the catalytic cycle, the coenzyme shuttles between MeCbl and the highly nucleophilic cobalamin form (Giedyk et al., 2015). Examples of MeCbl-dependent enzymes include methionine synthase, which is a cytosolic methionine synthase (formation of methionine) (Balabanova et al., 2021) that reacts with cobalamin-dependent methionine synthesis and DNA synthesis through the conversion of ribonucleotides to deoxyribonucleotides and tRNA biosynthesis (Banerjee and Ragsdale, 2003).
AdoCbl functions as a source of carbon-based free radicals that are unmasked by homolysis of the cobalt-carbon bond of the coenzyme. Free radicals are subsequently used to remove non-acidic hydrogen atoms from substrates to facilitate a variety of reactions involving cleavage of carbon–carbon, carbon–oxygen, and carbon–nitrogen bonds. Most of these reactions involve the migration of hydroxy-, amino-, and carbon-containing groups. In addition, one class of ribonucleotide reductases uses AdoCbl (Giedyk et al., 2015).
2.4 Ecological interactions between cobalamin producers and auxotrophs in marine ecosystems
The natural forms of vitamin B12, MeCbl, and AdoCbl are synthesized only by prokaryotes via aerobic and anaerobic pathways (Balabanova et al., 2021). Cyanobacteria and key taxa within α- and γ-Proteobacteria (Sañudo-Wilhelmy et al., 2014), Actinobacteria, and Bacteroidetes Thaumarchaeota (Doxey et al., 2015; Shelton et al., 2019) are included among the cobalamin producers. The involvement of archaea in cobalamin production is poorly understood; thus, the insight into the cobalamin biosynthesis have mainly focused on bacteria (Table 2). The ability to synthesize cobalamin has neither been inherited by eukaryotes nor has it been subjected to lateral gene transfer (Croft et al., 2005). In eukaryotes, vitamin B12 is acquired in two ways:
a. Directly from a metabolite pool released by prokaryotes, either through cell lysis of the producers or via passive transport (Romine et al., 2017), benefiting cobalamin-dependent eukaryotic consumers, such as the diatom Thalassiosira pseudonana (Sultana et al., 2023). Several experimental amendments support this, showing that dissolved cobalamin in the ocean stimulates the growth of eukaryotic phytoplankton (Bonnet et al., 2013) and heterotrophic protists (Wienhausen et al., 2022).
b. Indirectly through interactions with prokaryotes, such as mutualism, symbiosis, or predation (Joglar et al., 2021). Among marine cultures, symbiosis and mutualism are the most studied interactions. For example, symbiosis has been explored in co-cultures such as Ostreococcus tauri and the alphaproteobacterium Dinoroseobacter shibae (Cooper et al., 2018). Additionally, it has been hypothesized that marine sponges obtain cobalamin through symbiotic relationships with associated microbes (Degnan et al., 2014).
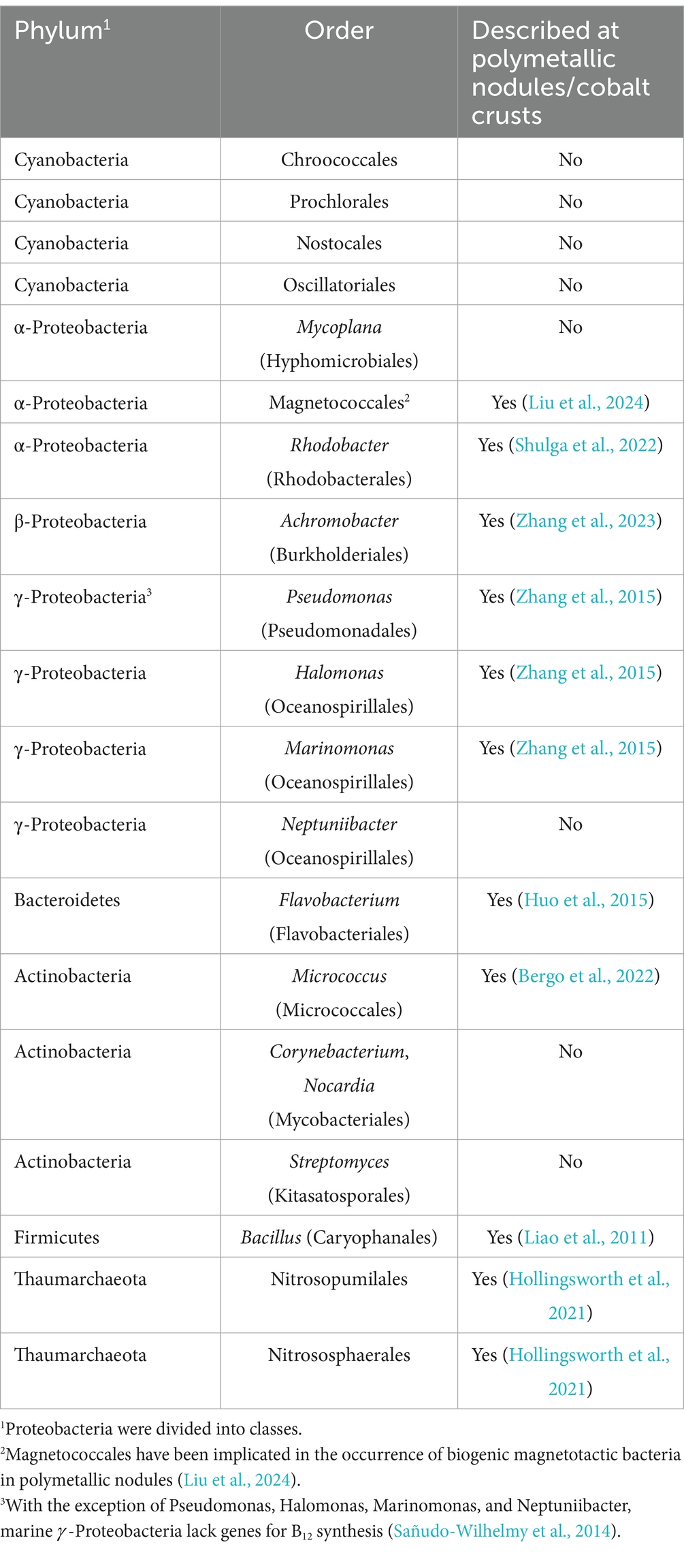
Table 2. Cobalamin producers in marine ecosystems (Sañudo-Wilhelmy et al., 2014; Wang J. et al., 2024; Wang L. et al., 2024).
These mechanisms of eukaryotic acquisition of cobalamin from external sources have shaped ecosystems. In all ecosystems, auxotrophs are organisms that depend on cobalamin, but lack the ability to synthesize these cofactors, including both prokaryotes and eukaryotes (Nijland et al., 2022). Examples of auxotrophs are organohalide-respiring bacteria and several eukaryotic lineages, such as vertebrates, most protists, and invertebrates, but not plants, insects, or fungi (Degnan et al., 2014). Intriguingly, higher plants have no cobalamin-dependent enzymes and so do not utilize or synthesize cobalamin (Croft et al., 2006); in comparison, nearly half of marine species are cobalamin auxotrophs (Croft et al., 2005). Eukaryotic auxotrophy has evolved several times, resulting in close and intricate ecological relationships. For example, algae can be influenced by their symbionts, which supply fixed carbon in return for vitamin B12 (Grant et al., 2014).
As one of the highly limited nutrients and growth factors controlled by a minority of microbes, B12 can be considered as “hard currency” in the global ocean ecosystem (Zhou et al., 2023). Therefore, cobamide sharing creates a network of cobamide-dependent interactions, providing a useful system for studying mechanisms that influence community composition and function (Sokolovskaya et al., 2020). Evidence of this shaping of B vitamins in the photic zone, including vitamin B12, has been studied in the Mediterranean Sea and the Eastern Atlantic Ocean, exploring picoplankton and suggesting that cobalamin is crucial for determining the structure and function of microbial ecosystems (Suffridge et al., 2018).
3 Cobalt and cobalamin in the water column
3.1 Distribution and dynamics of cobalt
Cobalt (Co) is required for the de novo synthesis of vitamin B12 (Panzeca et al., 2008), as this trace metal serves as the central coordinating ion within the molecule. However, the total dissolved Co concentrations (mainly Co2+ and Co3+) are relatively low, ranging from 3 to 120 picomolar (pM) in the open ocean (Saito and Moffett, 2002), and only a small fraction of this Co pool is thought to exist in the bioavailable dissolved form as Co2+ (Panzeca et al., 2009). Generally, dissolved metals, including Co, increase with depth because of the release associated with the decomposition of organic matter (Gerringa et al., 2021). In comparison, at the surface, dissolved cobalt exhibits low concentrations and high variability, whereas in the ocean interior, cobalt concentrations increase from the surface to intermediate waters and decline in the deep ocean (Tagliabue et al., 2018). Considering the low cobalt concentrations and chemical speciation, the total dissolved cobalt pool of redox state 2+, suitable for B12 synthesis, is very low, within the femtomolar (fM) range (Panzeca et al., 2008). This low Co (II) content is consistent with the proportional distribution of dissolved B12 in the North Atlantic Ocean according to the abundance of total dissolved Co availability (Panzeca et al., 2009). A similar conclusion was reported by Hawco et al. (2020), who found that Co could limit the growth of the cyanobacterium, Prochlorococcus, in the Pacific Ocean.
3.2 Cobalamin availability
Vitamin B12 is an energetically costly metabolite, which may explain why less than 40% of prokaryotes encode the genes required for its complete biosynthesis (Shelton et al., 2019). In the open ocean, vitamin B12 concentrations range from sub-picomolar to picomolar (Sañudo-Wilhelmy et al., 2012). This distribution is influenced by Co availability (Moore et al., 2013), which plays a role in shaping the ecological structure. Sañudo-Wilhelmy et al. (2006) conducted field-based vitamin B12 amendment experiments and observed an increase in phytoplankton biomass, leading to the recognition of this metallocofactor as a limiting factor in some marine ecosystems (Wang L. et al., 2024). Additionally, cobalt has been identified as playing a secondary role in biogeochemical interactions, such as phosphorus, whereas nitrogen and iron remain the primary limiting nutrients (Valiela, 2015).
The concentration of vitamin B12 in seawater varies significantly across marine regions. For instance, in coastal areas, cobalamin levels range from undetectable to 30 pM (Sañudo-Wilhelmy et al., 2012). Additionally, it has been reported that coastal waters generally contain higher concentrations of vitamin B12 compared to open ocean waters (Sañudo-Wilhelmy et al., 2012; Heal et al., 2017). A similar trend was observed when comparing water depths, with lower vitamin B12 concentrations found in deeper waters (100–200 m) than in surface and subsurface waters (0–100 m) (Bonnet et al., 2013).
Cobalamin de novo synthesis in surface waters has been linked to heterotrophic Proteobacteria bacteria, mainly Rhodobacterales (Gómez-Consarnau et al., 2018), and to photoautotrophic Cyanobacteria synthesizing pseudocobalamin with a peak concentration within the euphotic zone (Heal et al., 2017). These cobalamin providers are related to different biogeographic settings, including the Mediterranean Sea and Eastern Atlantic Ocean (Suffridge et al., 2018), Northwest Atlantic Ocean (Soto et al., 2023), subtropical, equatorial, polar frontal Pacific Ocean (Wienhausen et al., 2022), and North Pacific Ocean (Heal et al., 2017).
Cobalamin compounds (cyanocobalamin, methylcobalamin, and hydroxycobalamin; Figure 1) are labile after aerobic light exposure, even in various aqueous solutions (Vaid et al., 2018). The photosensitivity of cobalamin is attributed to the dissociation of covalent cobalt-carbon bonds upon exposure to light, resulting in its photolabile characteristics (Vaid et al., 2018; Bannon et al., 2024). Consequently, enzymatic reactions involving cobalamin must be conducted under dim light (Giedyk et al., 2015).
4 Polymetallic nodules and cobalt crusts: key study sites in cobalt biogeochemistry
4.1 Generalities of polymetallic nodules and cobalt crusts
Polymetallic nodules and cobalt-rich crusts are classified in the same paragenetic group (Hazen and Morrison, 2022). Their formation involves complex interactions among geological, chemical, and biological processes that occurr over millions of years (Wang and Müller, 2009). Nodules develop through three main types of precipitation: hydrogenesis refers to the growth of nodules through the direct precipitation of metals from the water column, whereas diagenesis involves the growth of nodules through the precipitation of metals from the sediment pore water. Mixed-type nodules contain layers formed by both hydrogenesis and diagenesis (Benites et al., 2018; Hein et al., 2020). Hydrogenetic nodules form on the sediment surface in well-oxygenated environments where sedimentation rates are low because of the direct supply and precipitation of metallic elements from seawater and diagenetically unenriched sediment pore water (Sujith and Gonsalves, 2021). These nodules are mainly composed of manganese, iron, and trace metals such as nickel (Ni), copper (Cu), cobalt, molybdenum (Mo), and rare earth elements (REE) (Mukhopadhyay et al., 2008). Cobalt crusts are found globally on the ocean floor, generally adjacent to the oxygen minimum zone (OMZ), where less oxidizing conditions prevail (Verlaan and Cronan, 2022). The exposed rock surfaces of the seamounts and ridges are the most concentrated areas. Cobalt-rich crusts occur at shallower depths (<2,500 m) in the deep sea, whereas nodules are formed at much deeper depths (up to 5,500 m) (Verlaan and Cronan, 2022). Initially, it was hypothesized that cobalt precipitates formed solely through inorganic precipitation; however, increasing evidence suggests the involvement of certain bacteria found within nodules and crusts that can oxidize both Mn and Co (Moffett and Ho, 1996; Murray et al., 2007).
4.2 Cobalt enrichment in deep-sea minerals
Both polymetallic nodules and cobalt-rich crusts are enriched in cobalt, Ni, Cd, Zn, and REE, relative to their lower concentrations in seawater (Hawco et al., 2018; Hein et al., 2020). Oxidized cobalt (Co3+) precipitates in the water column and eventually precipitates via hydrogenesis. Cobalt enrichment in nodules can be up to 100-fold higher than its elemental abundance in Earth’s crust. Cobalt (II) in seawater can undergo surface oxidation to Co (III) via adsorption onto iron and manganese oxyhydroxides (Koschinsky and Hein, 2003; Wang et al., 2009) in minerals, such as birnessite (Wu et al., 2019). Factors such as sedimentation, oxygen concentration, temperature, and growth rate influence Co enrichment in crusts. Slower rates lead to greater Co accumulation, resulting in an average Co content of up to 0.77% in the crust (Verlaan and Cronan, 2022). These factors explain the higher Co concentration in cobalt crusts than in nodules (Mukhopadhyay et al., 2008). Hydrogenetic-type nodules are characterized by higher Co/Mn ratios (Hein et al., 2020). In cobalt-rich crusts, the Co content ranges from 0.3 to 1.2% (Halbach et al., 2017). Nodules from the Clarion-Clipperton Zone (CCZ) have higher Co concentrations, with an average content of 0.21%, compared to other locations (Hein et al., 2020). The Co oxidation state predominantly exists as +3 and a minor fraction as +2 in these deposits (Wegorzewski et al., 2020).
4.3 Cobalt crusts: a reliable system for comparative studies
Experiments on cobalt limitation or co-limitation of cobalamin availability have shown that B12 synthesis can be restricted by cobalt concentrations in certain ocean regions. For example, amendment experiments conducted in waters with low dissolved cobalt (approximately 20 pM) resulted in a two-fold increase in B12 production compared to unamended controls (Panzeca et al., 2008). Furthermore, in amendment experiments conducted in the South Atlantic gyre, the addition of cobalt after nitrogen and iron limitation was alleviated, leading to a significant increase in phytoplankton growth (Browning et al., 2017). It has been proposed that cobalamin availability is regulated not only by photodegradation, alteration, and the supply/demand ratio but also by cobalt availability (Bannon et al., 2022). This suggests that, in surface waters, cobalt and light frequently modulate and limit cobalamin availability, as phytoplankton, especially cyanobacteria, are the main sources of this metallocofactor (Heal et al., 2017). However, this scenario is not equivalent to an increase in depth, considering that anoxic ecosystems favor cobalt dissolution, leading to a long residence time for cobalt (Tagliabue et al., 2018).
Therefore, different conditions of cobalamin availability, such as light, phytoplankton distribution, and oxygen concentration, provoke distinctive ecological networks in the deep sea. In this regard, sites enriched in ferromanganese (Fe-Mn) nodules and cobalt crusts are useful for resolving these ecological questions and testing hypotheses. As mentioned earlier, these deposits are broadly characterized by high levels of Mn oxides, where cobalt is incorporated into the octahedral sheets of phyllomanganates, particularly vernadite (δ-MnO2) (Wegorzewski et al., 2020). A relevant question arises regarding whether cobalamin synthesizers in these regions play a role in supporting local auxotrophic organisms, or whether and to what extent the communities depend on surface sources, such as cyanobacteria, given that previous studies have shown phytodetritus contributes to particulate organic carbon in sediments within the Clarion Clipperton Zone (Cecchetto et al., 2023).
4.4 Thaumarchaeota as potential key players in cobalamin biosynthesis
Thaumarchaeota, formerly known as Crenarchaeota, is a phylum that is frequently found in the deeper waters of the ocean (Heal et al., 2017; Qin et al., 2020). Their distribution is likely due to the sensitivity of ammonia oxidation to light (Merbt et al., 2012). Ammonia-oxidizing archaea (AOA) or Thaumarchaeota strains are typically isolated under dark conditions (Stieglmeier et al., 2014). Indeed, the initial steps of both ammonia-oxidizing pathways, archaeal and bacterial (AOA and AOB, respectively), are inhibited by light (Merbt et al., 2012). In this sense, ammonia-oxidizing archaea are abundant in sediments (coastal and estuarine) (Francis et al., 2005). These archaea are the major cobalamin sources in the oxygen-deficient zone (ODZ) of coastal productive ecosystems (Heal et al., 2017), and as suggested by their genomic potential, they could potentially inhabit bathypelagic habitats (Doxey et al., 2015). Interestingly, Thaumarchaeota, formerly known as Crenarchaeota, is a significant source of vitamin B12 in these environments (Heal et al., 2017).
Isolation of the non-cyanobacterial and cobalamin synthesizers Nitrosopumilus spp. (Thaumarchaeota), Sulfitobacter sp. SA11, and Ruegeria pomeroyi DSS-3 (α-Proteobacteria) from deep water support the hypothesis of vertical niche differentiation (Heal et al., 2017). This is particularly noteworthy given that cobalamin itself is photosensitive (Bannon et al., 2024), suggesting an interplay between the light environment and cobalamin dynamics in the ocean. The growth of Thaumarchaeota, also known as AOA, can be promoted by ammonia provided by auxotrophs (Heal et al., 2017; Hollibaugh, 2017). Therefore, in mesophotic ecosystems, only selected cobalamin auxotrophic hosts (bacteria and eukaryotes) provide this essential nutrient for AOA. This contrasts with Cyanobacteria, which have a distinct euphotic ecological niche.
The presence of the cobalamin biosynthetic pathway in an ecosystem implies a level of sustainability at which auxotrophic organisms can thrive only in communities that provide this essential vitamin. In this context, we hypothesized that certain taxa characteristic of these aquatic environments play key roles in cobalamin biosynthesis. In this regard, we highlight the abundance of Thaumarchaeota associated with these concretions, as recognized by the clone library (Shulse et al., 2017) and metagenomic approaches (Zhang et al., 2023). Notably, analysis of bacterial and archaeal 16S rRNA gene sequences from deep-sea polymetallic nodules and sediment extracted from the South Pacific Gyre revealed that the phylum Thaumarchaeota was more abundant in the nodules than in the surrounding sediments (Shiraishi et al., 2016). Based on this, we suggest that members of this archaeal phylum may serve as a source of cobalamin for both prokaryotes and eukaryotes. Furthermore, considering the high cobalt content in cobalt crusts and some polymetallic nodules, it is plausible that, in these habitats, a cobalt-cobalamin connection exists along the food web and involves prokaryotes and metazoans (Figure 2).
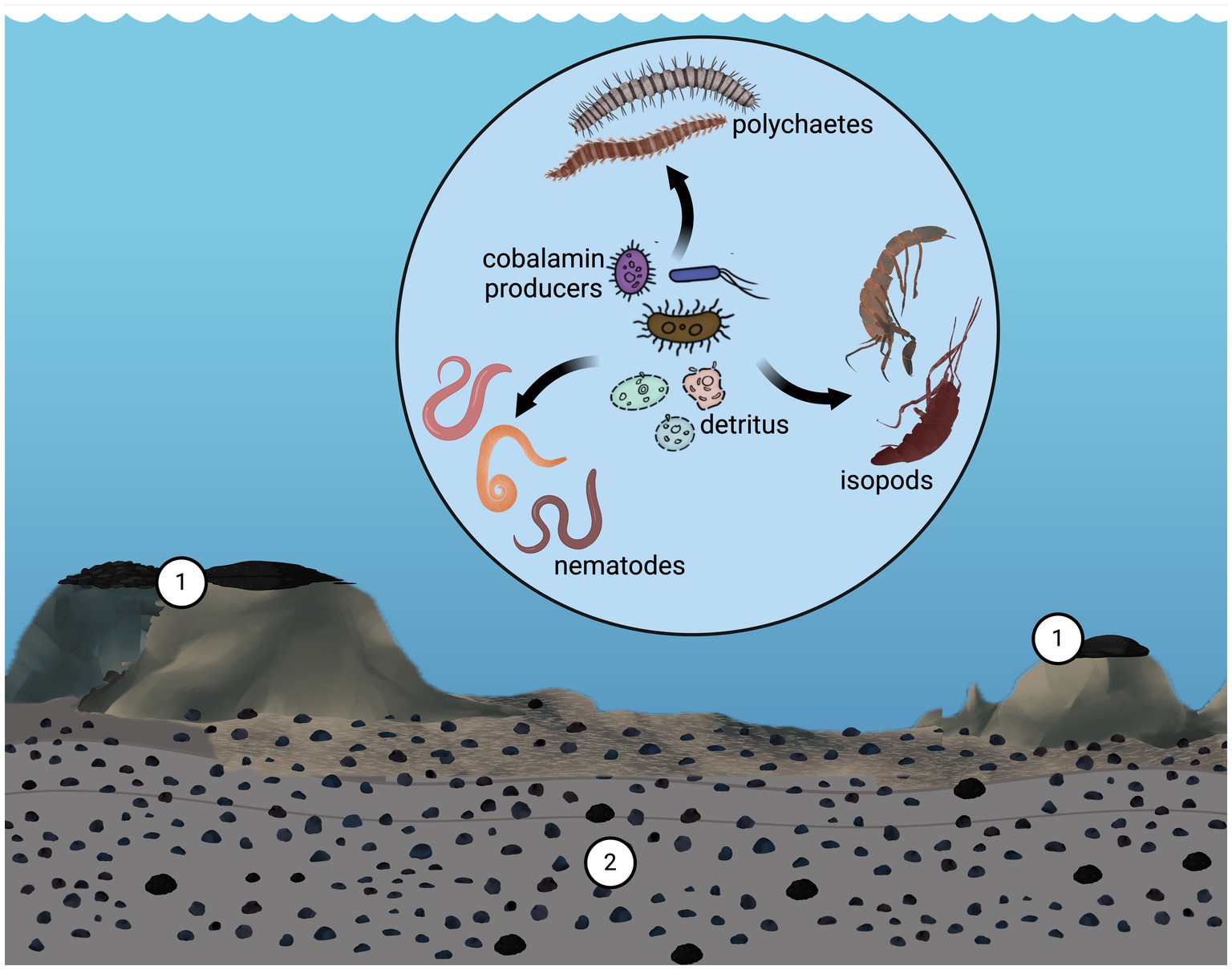
Figure 2. Scheme illustrates the proposed interrelationship between cobalt found in cobalt crusts (1) and polymetallic nodules (2), and cobalamin and trophic interactions within the community in these systems, mediated through synthesizers (prokaryotes) and bacterivores. Credit design: L. Montoya and C. F. Franco-Rodríguez.
Therefore, cobalamin-producing prokaryotes, including Thaumarchaeota, play key roles in linking cobalt and cobalamin. Furthermore, the availability of cobalt, a critical element in the organometallic metabolite cobalamin, may explain the sustainability of auxotrophs in these deep-sea regions. To examine this claim, it is essential to study organisms, such as Thaumarchaeota and other cobalamin producers, as they provide this vital metabolite to auxotrophic bacteria and metazoans. This study aimed to explore the relative abundance of these prokaryotes, and the genetic repertoire associated with cobalamin synthesis in the metazoan microbiome thriving on cobalt crusts and polymetallic nodules and compare them with other deep-sea regions. The relationship between metazoans and their cobalamin-producing symbionts or mutualists may be particularly relevant for bacterivorous meiofauna.
4.5 Proposed ecological networks driven by cobalt and cobalamin availability
Prokaryotic B12 sources in some unicellular eukaryotes and metazoans may occur through symbiosis, predation, or commensalism. Within the nodule and cobalt crust communities, some prokaryotes, such as α- and γ-Proteobacteria and Thaumarchaeota, may benefit to other populations with essential vitamin B12. An ecological example to argue this is the commensalism between the auxotrophic algal species Porphyridium purpureum, which obtains cobalamin from marine Halomonas sp. In return, algae provide γ-Proteobacteria with carbon sources (Croft et al., 2005) and and also acquire cobalamin through particulate organic carbon (POC) and dissolved organic carbon (DOC) (Moran et al., 2022). The implications of cobalt availability modulation on cobalamin synthesis have been demonstrated in surface water samples from the North Atlantic Ocean (Panzeca et al., 2008). Therefore, we propose that contrary to the cobalt limitation prevailing in the North Atlantic Ocean, as reported by Panzeca et al. (2008), cobalt crusts and polymetallic nodules support an abundance of cobalamin synthesizers in the absence of light.
Polymetallic nodules provide crucial hard surfaces for deep-sea benthic life with organisms such as stalked and encrusting sponges (Stratmann et al., 2021). An example is Plenaster craigi, an abundant sponge frequently attached to nodules that relies on particulate organic matter, bacteria, and other microbes (Taboada et al., 2018). Some annelids like the polychaeta Neanthes goodayi reside inside polymetallic nodules highlight the importance of the mineral itself as microhabitat (Drennan et al., 2021; Neal et al., 2022). In this sense, nodules and crusts represent a cobalt micro-oasis to sessile life that is likely to provide metal content that is beneficial to the synthesis of cobalamine. The experimental extraction of nodules from the Clarion-Clipperton Fracture Zone (CCZ) in the central Pacific has recorded impacts on biodiversity loss and changes in deep-sea water-sediment processes (Bonifácio et al., 2024; Stratmann, 2023; Stratmann et al., 2021). Bacterivores, such as Bryozoa, Cnidaria, Platyhelminthes, and Porifera, are among the most affected ecological groups (Stratmann et al., 2021). Other studies have experimentally focused on Nematoda and found that this taxon does not recover after extraction (Miljutin et al., 2011). These taxa, together with the rotifers and polychaetes present in nodules and crusts, represent a trophic link between bacteria and larger fauna (Stratmann, 2023; Uhlenkott et al., 2023). In this context, we propose a role for cobalt in the loss of taxa induced by nodule extraction. This proposal arises from keeping in mind that model species for some of the phyla described in the CCZ metazoan organisms acquire vitamin B12 from bacteria directly by ingestion or commensalism, as cobalamin-producing bacteria are abundant resident gut microbes (Degnan et al., 2014), such as α-, γ-Proteobacteria and Thaumarchaeota (Shulse et al., 2017). Nematodes are noteworthy in this context as they are the most abundant meiobenthic taxon in the ecosystems of nodule-bearing deep-sea sediments (Hauquier et al., 2019). Experimental work with the laboratory nematode Caenorhabditis elegans highlights cobalamin limitation, resulting in reduced fertility and longevity (Degnan et al., 2014) and predatory behavior, such as Pristionchus pacificus, where vitamin B12 is an important inducer (Lo and Sommer, 2022). Additional experimental work has shown symbiotic synthesis of cobalamin in a microbial co-culture of the bacteria Colwellia sp. and Roseovarius sp. (Wienhausen et al., 2024). Shallow water Aplysina aerophoba sponges show B12 synthesis dependency on Poribacteria (Siegl et al., 2011). Similar ecological interactions can occur in the mesophotic water columns and seafloor ecosystems.
5 Future research questions for understanding cobalt-cobalamin dynamics in deep-sea ecosystems
The ecological significance of cobalamin (vitamin B12) in other ecosystems remains largely unexplored. Future field studies should clarify the physiological requirements of organisms for cobalamin, particularly the minimum concentration required for growth and survival in the absence of light and oxygen. Laboratory evidence demonstrating the effects of B vitamins, specifically cobalamin, on microplankton has shed light on how marine bacteria fulfill the cobalamin requirements of auxotrophic organisms. For example, key research, such as the co-culture of Ostreococcus tauri with the bacterium Dinoroseobacter shibae (Cooper et al., 2018), provides a foundation for analyzing cobalt-rich crust prokaryotic and eukaryotic isolates. In addition, the co-culture approach employed by Wienhausen et al. (2024) further enhances research on prokaryote-prokaryote interactions, revealing a closer ecological connection where a symbiosis of two bacteria (Colwellia sp. and Roseovarius sp.) is required for complete cobalamin synthesis.
It is crucial to determine whether metazoans living in the absence of light selectively or preferentially ingest cobalamin directly or rely on symbiotic microbes (endo- or exosymbionts) to synthesize and provide cobalamin. Furthermore, investigating whether cobalamin-dependent metabolism offers an advantage to organisms living in environments with high concentrations of cobalt despite the absence of light or oxygen is essential for understanding the occurrence of microbial species that use cobalt and their adaptations.
The potential influence of cobalt availability on deep-sea metazoan distribution owing to cobalamin requirements highlights the urgent need for further research on the ecological implications of cobalt. In this regard, cobalt crusts and nodule habitats are sites for study. Advancements in genomics and metagenomics, particularly regarding the microbiome of metazoans, will be instrumental in characterizing their potential role as vitamin B12 auxotrophs. A valuable strategy for understanding the importance of cobalamin in ocean microbial communities and their integration along the food web will lead to new discoveries (Wang L. et al., 2024).
However, the chemical profile of Co in areas containing Co crusts and polymetallic nodules remains poorly characterized. A thorough investigation of cobalt distribution, particularly its redox speciation, is needed, as is research on abiotic and biotic cobalt oxidation from Co2+ to Co3+ within these systems, where microorganisms interact with the global cobalt biogeochemical cycle (Swanner et al., 2014). Some approaches already used in this context have involved cobalt amendment experiments (Bannon et al., 2022) and characterization of cobalamin-dependent enzymes (Hawco et al., 2020). This review provides a synthesis for future research determining whether cobalt limits or co-limits ecosystems in the absence of light and/or oxygen.
Studies focused on this topic will not only provide a clearer understanding of this subset of prokaryotes in the global ocean but will also shed light on its function (Zhou et al., 2023). As stated by Moore et al. (2013) and Giovannelli (2023), the distribution and availability of trace elements in the environment are required to understand the diversity of life on the planet.
Data availability statement
The original contributions presented in the study are included in the article/supplementary material, further inquiries can be directed to the corresponding authors.
Author contributions
LM: Conceptualization, Formal analysis, Investigation, Methodology, Visualization, Writing – original draft, Writing – review & editing. EE-B: Formal analysis, Funding acquisition, Project administration, Resources, Supervision, Validation, Writing – review & editing.
Funding
The author(s) declare that financial support was received for the research and/or publication of this article. LM and EE-B acknowledge Instituto de Ciencias del Mar y Limnología, UNAM, Mexico for full coverage of the article processing fee through the yearly budget of EE-B. LM received support from the Consejo Nacional de Humanidades, Ciencias y Tecnologías, Mexico, under grant number 3838403.
Acknowledgments
Credit design for Figures 1, 2: L. Montoya and C. F. Franco-Rodríguez.
Conflict of interest
The authors declare that the research was conducted in the absence of any commercial or financial relationships that could be construed as a potential conflict of interest.
Generative AI statement
The authors declare that no Gen AI was used in the creation of this manuscript.
Publisher’s note
All claims expressed in this article are solely those of the authors and do not necessarily represent those of their affiliated organizations, or those of the publisher, the editors and the reviewers. Any product that may be evaluated in this article, or claim that may be made by its manufacturer, is not guaranteed or endorsed by the publisher.
References
Balabanova, L., Averianova, L., Marchenok, M., Son, O., and Tekutyeva, L. (2021). Microbial and genetic resources for cobalamin (Vitamin B12) biosynthesis: from ecosystems to industrial biotechnology. Int. J. Mol. Sci. 22:4522. doi: 10.3390/ijms22094522
Banerjee, R., and Ragsdale, S. W. (2003). The many faces of vitamin B12: catalysis by cobalamin-dependent enzymes. Annu. Rev. Biochem. 72, 209–247. doi: 10.1146/annurev.biochem.72.121801.161828
Bannon, C. C., Mudge, E. M., and Bertrand, E. M. (2024). Shedding light on cobalamin photodegradation in the ocean. Limnol. Oceanogr. Lett. 9, 135–144. doi: 10.1002/lol2.10371
Bannon, C., Rapp, I., and Bertrand, E. M. (2022). Community interaction co-limitation: nutrient limitation in a marine microbial community context. Front. Microbiol. 13:846890. doi: 10.3389/fmicb.2022.846890
Benites, M., Millo, C., Hein, J., Nath, B. N., Murton, B., Galante, D., et al. (2018). Integrated geochemical and morphological data provide insights into the genesis of ferromanganese nodules. Fortschr. Mineral. 8:488. doi: 10.3390/min8110488
Bergo, N. M., Torres-Ballesteros, A., Signori, C. N., Benites, M., Jovane, L., Murton, B. J., et al. (2022). Spatial patterns of microbial diversity in Fe-Mn deposits and associated sediments in the Atlantic and Pacific oceans. Sci. Total Environ. 837:155792. doi: 10.1016/j.scitotenv.2022.155792
Bonifácio, P., Kaiser, S., Washburn, T. W., Smith, C. R., Vink, A., and Arbizu, P. M. (2024). Biodiversity of the clarion-Clipperton fracture zone: a worm perspective. Mar. Biodivers. 54:5. doi: 10.1007/s12526-023-01396-3
Bonnet, S., Tovar-Sanchez, A., Panzeca, C., Ortega-Retuerta, E., Duarte, C., and Sanudo-Wilhelmy, S. (2013). Geographical gradients of dissolved vitamin B12 in the Mediterranean Sea. Front. Microbiol. 4:126. doi: 10.3389/fmicb.2013.00126
Browning, T. J., Achterberg, E. P., Rapp, I., Engel, A., Bertrand, E. M., Tagliabue, A., et al. (2017). Nutrient co-limitation at the boundary of an oceanic gyre. Nature 551, 242–246. doi: 10.1038/nature24063
Bryant, D. A., Hunter, C. N., and Warren, M. J. (2020). Biosynthesis of the modified tetrapyrroles—the pigments of life. J. Biol. Chem. 295, 6888–6925. doi: 10.1074/jbc.REV120.006194
Buan, N. R. (2018). Methanogens: pushing the boundaries of biology. Emerg. Top. Life Sci. 2, 629–646. doi: 10.1042/ETLS20180031
Buckel, W. (2007). “Cobalamin coenzymes and vitamin B12” in eLS. ed. M. Maccarrone (Chichester: John Wiley & Sons Ltd.).
Cecchetto, M. M., Moser, A., Smith, C. R., Van Oevelen, D., and Sweetman, A. K. (2023). Abyssal seafloor response to fresh phytodetrital input in three areas of particular environmental interest (APEIs) in the western clarion-Clipperton zone (CCZ). Deep-Sea Res. I Oceanogr. Res. Pap. 195:103970. doi: 10.1016/j.dsr.2023.103970
Chmiel, R., Lanning, N., Laubach, A., Lee, J. M., Fitzsimmons, J., Hatta, M., et al. (2022). Major processes of the dissolved cobalt cycle in the north and equatorial Pacific Ocean. Biogeosciences 19, 2365–2395. doi: 10.5194/bg-19-2365-2022
Cooper, M. B., Kazamia, E., Helliwell, K. E., Kudahl, U. J., Sayer, A., Wheeler, G. L., et al. (2018). Cross-exchange of B-vitamins underpins a mutualistic interaction between Ostreococcus tauri and Dinoroseobacter shibae. ISME J. 13, 334–345. doi: 10.1038/s41396-018-0274-y
Croft, M. T., Lawrence, A. D., Raux-Deery, E., Warren, M. J., and Smith, A. G. (2005). Algae acquire vitamin B12 through a symbiotic relationship with bacteria. Nature 438, 90–93. doi: 10.1038/nature04056
Croft, M. T., Warren, M. J., and Smith, A. G. (2006). Algae need their vitamins. Eukaryot. Cell 5, 1175–1183. doi: 10.1128/EC.00097-06
Degnan, P. H., Taga, M. E., and Goodman, A. L. (2014). Vitamin B12 as a modulator of gut microbial ecology. Cell Metab. 20, 769–778. doi: 10.1016/j.cmet.2014.10.002
Doxey, A. C., Kurtz, D. A., Lynch, M. D. J., Sauder, L. A., and Neufeld, J. D. (2015). Aquatic metagenomes implicate Thaumarchaeota in global cobalamin production. ISME J. 9, 461–471. doi: 10.1038/ismej.2014.142
Drennan, R., Wiklund, H., Rabone, M., Georgieva, M. N., Dahlgren, T. G., and Glover, A. G. (2021). Neanthes goodayi sp. nov. (Annelida, Nereididae), a remarkable new annelid species living inside deep-sea polymetallic nodules. Eur. J. Taxon. 760, 160–185. doi: 10.5852/ejt.2021.760.1447
Francis, C. A., Roberts, K. J., Beman, J. M., Santoro, A. E., and Oakley, B. B. (2005). Ubiquity and diversity of ammonia-oxidizing archaea in water columns and sediments of the ocean. Proc. Natl. Acad. Sci. 102, 14683–14688. doi: 10.1073/pnas.0506625102
Gerringa, L. J. A., Rijkenberg, M. J. A., Slagter, H. A., Laan, P., Paffrath, R., Bauch, D., et al. (2021). Dissolved Cd, Co, Cu, Fe, Mn, Ni, and Zn in the Arctic Ocean. J. Geophys. Res. Oceans 126:e2021JC017323. doi: 10.1029/2021JC017323
Giedyk, M., Goliszewska, K., and Gryko, D. (2015). Vitamin B12 catalysed reactions. Chem. Soc. Rev. 44, 3391–3404. doi: 10.1039/C5CS00165J
Giovannelli, D. (2023). Trace metal availability and the evolution of biogeochemistry. Nat. Rev. Earth Environ. 4, 597–598. doi: 10.1038/s43017-023-00477-y
Gómez-Consarnau, L., Sachdeva, R., Gifford, S. M., Cutter, L. S., Fuhrman, J. A., Sañudo-Wilhelmy, S. A., et al. (2018). Mosaic patterns of B-vitamin synthesis and utilization in a natural marine microbial community. Environ. Microbiol. 20, 2809–2823. doi: 10.1111/1462-2920.14133
Grant, M. A. A., Kazamia, E., Cicuta, P., and Smith, A. G. (2014). Direct exchange of vitamin B12 is demonstrated by modelling the growth dynamics of algal–bacterial co-cultures. ISME J. 8, 1418–1427. doi: 10.1038/ismej.2014.9
Halbach, P. E., Jahn, A., and Cherkashov, G. (2017). “Marine co-rich ferromanganese crust deposits: description and formation, occurrences and distribution, estimated world-wide resources” in Deep-Sea mining: Resource potential, technical and environmental considerations. ed. R. Sharma (Cham: Springer International Publishing), 65–141.
Hauquier, F., Macheriotou, L., Bezerra, T. N., Egho, G., Martínez Arbizu, P., and Vanreusel, A. (2019). Distribution of free-living marine nematodes in the clarion–Clipperton zone: implications for future deep-sea mining scenarios. Biogeosciences 16, 3475–3489. doi: 10.5194/bg-16-3475-2019
Hawco, N. J., Lam, P. J., Lee, J.-M., Ohnemus, D. C., Noble, A. E., Wyatt, N. J., et al. (2018). Cobalt scavenging in the mesopelagic ocean and its influence on global mass balance: synthesizing water column and sedimentary fluxes. Mar. Chem. 201, 151–166. doi: 10.1016/j.marchem.2017.09.001
Hawco, N. J., Mcilvin, M. M., Bundy, R. M., Tagliabue, A., Goepfert, T. J., Moran, D. M., et al. (2020). Minimal cobalt metabolism in the marine cyanobacterium Prochlorococcus. Proc. Natl. Acad. Sci. 117, 15740–15747. doi: 10.1073/pnas.2001393117
Hazen, R. M., and Morrison, S. M. (2022). On the paragenetic modes of minerals: a mineral evolution perspective. Am. Mineral. 107, 1262–1287. doi: 10.2138/am-2022-8099
Heal, K. R., Qin, W., Ribalet, F., Bertagnolli, A. D., Coyote-Maestas, W., Hmelo, L. R., et al. (2017). Two distinct pools of B12 analogs reveal community interdependencies in the ocean. Proc. Natl. Acad. Sci. 114, 364–369. doi: 10.1073/pnas.1608462114
Hein, J. R., Koschinsky, A., and Kuhn, T. (2020). Deep-ocean polymetallic nodules as a resource for critical materials. Nat. Rev. Earth Environ. 1, 158–169. doi: 10.1038/s43017-020-0027-0
Hollibaugh, J. T. (2017). Oxygen and the activity and distribution of marine Thaumarchaeota. Environ. Microbiol. Rep. 9, 186–188. doi: 10.1111/1758-2229.12534
Hollingsworth, A. L., Jones, D. O. B., and Young, C. R. (2021). Spatial vriability of abyssal nitrifying microbes in the north-eastern clarion-clipperton zone. Front. Mar. Sci. 8:663420. doi: 10.3389/fmars.2021.663420
Huo, Y., Cheng, H., Post, A. F., Wang, C., Jiang, X., Pan, J., et al. (2015). Ecological functions of uncultured microorganisms in the cobalt-rich ferromanganese crust of a seamount in the Central Pacific are elucidated by fosmid sequencing. Acta Oceanol. Sin. 34, 92–113. doi: 10.1007/s13131-015-0650-7
Joglar, V., Pontiller, B., Martínez-García, S., Fuentes-Lema, A., Pérez-Lorenzo, M., Lundin, D., et al. (2021). Microbial plankton community structure and function responses to vitamin B12 and B12 amendments in an uwelling system. Appl. Environ. Microbiol. 87:e01525-21. doi: 10.1128/AEM.01525-21
Koschinsky, A., and Hein, J. R. (2003). Uptake of elements from seawater by ferromanganese crusts: solid-phase associations and seawater speciation. Mar. Geol. 198, 331–351. doi: 10.1016/S0025-3227(03)00122-1
Liao, L., Xu, X.-W., Jiang, X.-W., Wang, C.-S., Zhang, D.-S., Ni, J.-Y., et al. (2011). Microbial diversity in deep-sea sediment from the cobalt-rich crust deposit region in the Pacific Ocean. FEMS Microbiol. Ecol. 78, 565–585. doi: 10.1111/j.1574-6941.2011.01186.x
Liu, Y., Liu, S., Piedrahita, V. A., Liu, P., He, S., Pan, H., et al. (2024). Insights into a correlation between magnetotactic bacteria and polymetallic nodule distribution in the Eastern Central Pacific Ocean. J. Geophys. Res. 129:e2024JB029062. doi: 10.1029/2024JB029062
Lo, W.-S., and Sommer, R. J. (2022). Vitamin B12 and predatory behavior in nematodes. Vitam. Horm. 119, 471–89. doi: 10.1016/bs.vh.2022.01.006
Merbt, S. N., Stahl, D. A., Casamayor, E. O., Martí, E., Nicol, G. W., and Prosser, J. I. (2012). Differential photoinhibition of bacterial and archaeal ammonia oxidation. FEMS Microbiol. Lett. 327, 41–46. doi: 10.1111/j.1574-6968.2011.02457.x
Miljutin, D. M., Miljutina, M. A., Arbizu, P. M., and Galéron, J. (2011). Deep-sea nematode assemblage has not recovered 26 years after experimental mining of polymetallic nodules (clarion-Clipperton fracture zone, tropical eastern Pacific). Deep-Sea Res. I Oceanogr. Res. Pap. 58, 885–897. doi: 10.1016/j.dsr.2011.06.003
Moffett, J. W., and Ho, J. (1996). Oxidation of cobalt and manganese in seawater via a common microbially catalyzed pathway. Geochim. Cosmochim. Acta 60, 3415–3424. doi: 10.1016/0016-7037(96)00176-7
Moore, C. M., Mills, M. M., Arrigo, K. R., Berman-Frank, I., Bopp, L., Boyd, P. W., et al. (2013). Processes and patterns of oceanic nutrient limitation. Nat. Geosci. 6, 701–710. doi: 10.1038/ngeo1765
Moran, M. A., Kujawinski, E. B., Schroer, W. F., Amin, S. A., Bates, N. R., Bertrand, E. M., et al. (2022). Microbial metabolites in the marine carbon cycle. Nat. Microbiol. 7, 508–523. doi: 10.1038/s41564-022-01090-3
Mukhopadhyay, R., Ghosh, A. K., and Iyer, S. D. (2008). “Ferromanganese deposits” in Handbook of exploration and environmental geochemistry. eds. R. Mukhopadhyay, A. K. Ghosh, and S. D. Iyer (Netherlands: Elsevier Science BV), 155–224.
Murray, K. J., Webb, S. M., Bargar, J. R., and Tebo, B. M. (2007). Indirect oxidation of co(II) in the presence of the marine Mn(II)-oxidizing bacterium Bacillus sp. strain SG-1. Appl. Environ. Microbiol. 73, 6905–6909. doi: 10.1128/AEM.00971-07
Neal, L., Wiklund, H., Rabone, M., Dahlgren, T. G., and Glover, A. G. (2022). Abyssal fauna of polymetallic nodule exploration areas, eastern clarion-Clipperton zone, Central Pacific Ocean: Annelida: Spionidae and Poecilochaetidae. Mar. Biodivers. 52:51. doi: 10.1007/s12526-022-01277-1
Nijland, M., Martínez Felices, J. M., Slotboom, D. J., and Thangaratnarajah, C. (2022). Membrane transport of cobalamin. Vitam. Horm. 119, 121–148. doi: 10.1016/bs.vh.2022.01.008
Okamoto, S., and Eltis, L. D. (2011). The biological occurrence and trafficking of cobalt. Metallomics 3, 963–970. doi: 10.1039/c1mt00056j
Osman, D., Cooke, A., Young, T. R., Deery, E., Robinson, N. J., and Warren, M. J. (2021). The requirement for cobalt in vitamin B12: a paradigm for protein metalation. Biochim. Biophys. Acta 1868:118896. doi: 10.1016/j.bbamcr.2020.118896
Panzeca, C., Beck, A. J., Leblanc, K., Taylor, G. T., Hutchins, D. A., and Sañudo-Wilhelmy, S. A. (2008). Potential cobalt limitation of vitamin B12 synthesis in the North Atlantic Ocean. Glob. Biogeochem. Cycles 22:GB2029. doi: 10.1029/2007GB003124
Panzeca, C., Beck, A. J., Tovar-Sanchez, A., Segovia-Zavala, J., Taylor, G. T., Gobler, C. J., et al. (2009). Distributions of dissolved vitamin B12 and Co in coastal and open-ocean environments. Estuar. Coast. Shelf Sci. 85, 223–230. doi: 10.1016/j.ecss.2009.08.016
Qin, W., Zheng, Y., Zhao, F., Wang, Y., Urakawa, H., Martens-Habbena, W., et al. (2020). Alternative strategies of nutrient acquisition and energy conservation map to the biogeography of marine ammonia-oxidizing archaea. ISME J. 14, 2595–2609. doi: 10.1038/s41396-020-0710-7
Rabone, M., Wiethase, J. H., Simon-Lledó, E., Emery, A. M., Jones, D. O. B., Dahlgren, T. G., et al. (2023). How many metazoan species live in the world’s largest mineral exploration region? Curr. Biol. 33, 2383–2396.e5. doi: 10.1016/j.cub.2023.04.052
Romine, M. F., Rodionov, D. A., Maezato, Y., Osterman, A. L., and Nelson, W. C. (2017). Underlying mechanisms for syntrophic metabolism of essential enzyme cofactors in microbial communities. ISME J. 11, 1434–1446. doi: 10.1038/ismej.2017.2
Saito, M. A., and Moffett, J. W. (2002). Temporal and spatial variability of cobalt in the Atlantic Ocean. Geochim. Cosmochim. Acta 66, 1943–1953. doi: 10.1016/S0016-7037(02)00829-3
Sañudo-Wilhelmy, S. A., Cutter, L. S., Durazo, R., Smail, E. A., Gómez-Consarnau, L., Webb, E. A., et al. (2012). Multiple B-vitamin depletion in large areas of the coastal ocean. Proc. Natl. Acad. Sci. 109, 14041–14045. doi: 10.1073/pnas.1208755109
Sañudo-Wilhelmy, S. A., Gobler, C. J., Okbamichael, M., and Taylor, G. T. (2006). Regulation of phytoplankton dynamics by vitamin B12. Geophys. Res. Lett. 33:L04604. doi: 10.1029/2005GL025046
Sañudo-Wilhelmy, S. A., Gómez-Consarnau, L., Suffridge, C., and Webb, E. A. (2014). The role of B vitamins in marine biogeochemistry. Annu. Rev. Mar. Sci. 6, 339–367. doi: 10.1146/annurev-marine-120710-100912
Scott, A. I., and Roessner, C. A. (2002). Biosynthesis of cobalamin (vitamin B12). Biochem. Soc. Trans. 30, 613–620. doi: 10.1042/bst0300613
Shelton, A. N., Seth, E. C., Mok, K. C., Han, A. W., Jackson, S. N., Haft, D. R., et al. (2019). Uneven distribution of cobamide biosynthesis and dependence in bacteria predicted by comparative genomics. ISME J. 13, 789–804. doi: 10.1038/s41396-018-0304-9
Shiraishi, F., Mitsunobu, S., Suzuki, K., Hoshino, T., Morono, Y., and Inagaki, F. (2016). Dense microbial community on a ferromanganese nodule from the ultra-oligotrophic South Pacific gyre: implications for biogeochemical cycles. Earth Planet. Sci. Lett. 447, 10–20. doi: 10.1016/j.epsl.2016.04.021
Shulga, N., Abramov, S., Klyukina, A., Ryazantsev, K., and Gavrilov, S. (2022). Fast-growing Arctic Fe–Mn deposits from the Kara Sea as the refuges for cosmopolitan marine microorganisms. Sci. Rep. 12:21967. doi: 10.1038/s41598-022-23449-6
Shulse, C. N., Maillot, B., Smith, C. R., and Church, M. J. (2017). Polymetallic nodules, sediments, and deep waters in the equatorial North Pacific exhibit highly diverse and distinct bacterial, archaeal, and microeukaryotic communities. Microbiol. Open 6:e00428. doi: 10.1002/mbo3.428
Siegl, A., Kamke, J., Hochmuth, T., Piel, J., Richter, M., Liang, C., et al. (2011). Single-cell genomics reveals the lifestyle of Poribacteria, a candidate phylum symbiotically associated with marine sponges. ISME J. 5, 61–70. doi: 10.1038/ismej.2010.95
Sokolovskaya, O. M., Shelton, A. N., and Taga, M. E. (2020). Sharing vitamins: cobamides unveil microbial interactions. Science 369:eaba0165. doi: 10.1126/science.aba0165
Soto, M. A., Desai, D., Bannon, C., Laroche, J., and Bertrand, E. M. (2023). Cobalamin producers and prokaryotic consumers in the Northwest Atlantic. Environ. Microbiol. 25, 1300–1313. doi: 10.1111/1462-2920.16363
Stieglmeier, M., Klingl, A., Alves, R. J. E., Rittmann, S. K.-M. R., Melcher, M., Leisch, N., et al. (2014). Nitrososphaera viennensis gen. nov., sp. nov., an aerobic and mesophilic, ammonia-oxidizing archaeon from soil and a member of the archaeal phylum Thaumarchaeota. Int. J. Syst. Evol. Microbiol. 64, 2738–2752. doi: 10.1099/ijs.0.063172-0
Stratmann, T. (2023). Role of polymetallic-nodule dependent fauna on carbon cycling in the eastern clarion-Clipperton fracture zone (Pacific). Front. Marine Sci. 10:1151442. doi: 10.3389/fmars.2023.1151442
Stratmann, T., Soetaert, K., Kersken, D., and Van Oevelen, D. (2021). Polymetallic nodules are essential for food-web integrity of a prospective deep-seabed mining area in Pacific abyssal plains. Sci. Rep. 11:12238. doi: 10.1038/s41598-021-91703-4
Suffridge, C. P., Gómez-Consarnau, L., Monteverde, D. R., Cutter, L., Arístegui, J., Alvarez-Salgado, X. A., et al. (2018). B vitamins and their congeners as potential drivers of microbial community composition in an oligotrophic marine ecosystem. J. Geophys. Res. Biogeo. 123, 2890–2907. doi: 10.1029/2018JG004554
Sujith, P. P., and Gonsalves, M. J. B. D. (2021). Ferromanganese oxide deposits: geochemical and microbiological perspectives of interactions of cobalt and nickel. Ore Geol. Rev. 139:104458. doi: 10.1016/j.oregeorev.2021.104458
Sultana, S., Bruns, S., Wilkes, H., Simon, M., and Wienhausen, G. (2023). Vitamin B12 is not shared by all marine prototrophic bacteria with their environment. ISME J. 17, 836–845. doi: 10.1038/s41396-023-01391-3
Swanner, E. D., Planavsky, N. J., Lalonde, S. V., Robbins, L. J., Bekker, A., Rouxel, O. J., et al. (2014). Cobalt and marine redox evolution. Earth Planet. Sci. Lett. 390, 253–263. doi: 10.1016/j.epsl.2014.01.001
Taboada, S., Riesgo, A., Wiklund, H., Paterson, G. L. J., Koutsouveli, V., Santodomingo, N., et al. (2018). Implications of population connectivity studies for the design of marine protected areas in the deep sea: an example of a demosponge from the clarion-Clipperton zone. Mol. Ecol. 27, 4657–4679. doi: 10.1111/mec.14888
Tagliabue, A., Hawco, N. J., Bundy, R. M., Landing, W. M., Milne, A., Morton, P. L., et al. (2018). The role of external inputs and internal cycling in shaping the global ocean cobalt distribution: insights from the first cobalt biogeochemical model. Glob. Biogeochem. Cycles 32, 594–616. doi: 10.1002/2017GB005830
Uhlenkott, K., Meyn, K., Vink, A., and Martínez Arbizu, P. (2023). A review of megafauna diversity and abundance in an exploration area for polymetallic nodules in the eastern part of the clarion Clipperton fracture zone (North East Pacific), and implications for potential future deep-sea mining in this area. Mar. Biodivers. 53:22. doi: 10.1007/s12526-022-01326-9
Vaid, F. H. M., Zahid, S., Faiyaz, A., Qadeer, K., Gul, W., Anwar, Z., et al. (2018). Photolysis of methylcobalamin in aqueous solution: a kinetic study. J. Photochem. Photobiol. A Chem. 362, 40–48. doi: 10.1016/j.jphotochem.2018.05.011
Valiela, I. (2015). “Controls of primary production” in Marine ecological processes. ed. I. Valiela (New York, NY: Springer New York), 61–112.
Verlaan, P. A., and Cronan, D. S. (2022). Origin and variability of resource-grade marine ferromanganese nodules and crusts in the Pacific Ocean: a review of biogeochemical and physical controls. Geochemistry 82:125741. doi: 10.1016/j.chemer.2021.125741
Wang, X., and Müller, W. E. (2009). Marine biominerals: Perspectives and challenges for polymetallic nodules and crusts. Trends Biotechnol. 27, 375–383. doi: 10.1016/j.tibtech.2009.03.004
Wang, X.-H., Schloßmacher, U., Natalio, F., Schröder, H. C., Wolf, S. E., Tremel, W., et al. (2009). Evidence for biogenic processes during formation of ferromanganese crusts from the Pacific Ocean: implications of biologically induced mineralization. Micron 40, 526–535. doi: 10.1016/j.micron.2009.04.005
Wang, L., Zhao, H., Bi, R., Chen, X., Lyu, Z., and Liu, W. (2024). Roles and sources of B vitamins in the marine ecosystem. Rev. Fish Biol. Fish. 34, 111–130. doi: 10.1007/s11160-023-09818-y
Wang, J., Zhu, Y.-G., Tiedje, J. M., and Ge, Y. (2024). Global biogeography and ecological implications of cobamide-producing prokaryotes. ISME J. 18:wrae009. doi: 10.1093/ismejo/wrae009
Warren, M. J., Raux, E., Schubert, H. L., and Escalante-Semerena, J. C. (2002). The biosynthesis of adenosylcobalamin (vitamin B12). Nat. Prod. Rep. 19, 390–412. doi: 10.1039/b108967f
Wegorzewski, A. V., Grangeon, S., Webb, S. M., Heller, C., and Kuhn, T. (2020). Mineralogical transformations in polymetallic nodules and the change of Ni, Cu and Co crystal-chemistry upon burial in sediments. Geochim. Cosmochim. Acta 282, 19–37. doi: 10.1016/j.gca.2020.04.012
Wienhausen, G., Dlugosch, L., Jarling, R., Wilkes, H., Giebel, H.-A., and Simon, M. (2022). Availability of vitamin B12 and its lower ligand intermediate α-ribazole impact prokaryotic and protist communities in oceanic systems. ISME J. 16, 2002–2014. doi: 10.1038/s41396-022-01250-7
Wienhausen, G., Moraru, C., Bruns, S., Tran, D. Q., Sultana, S., Wilkes, H., et al. (2024). Ligand cross-feeding resolves bacterial vitamin B12 auxotrophies. Nature 629, 886–892. doi: 10.1038/s41586-024-07396-y
Wu, Z., Peacock, C. L., Lanson, B., Yin, H., Zheng, L., Chen, Z., et al. (2019). Transformation of co-containing birnessite to todorokite: effect of co on the transformation and implications for co mobility. Geochim. Cosmochim. Acta 246, 21–40. doi: 10.1016/j.gca.2018.11.001
Zhang, D., Li, X., Wu, Y., Xu, X., Liu, Y., Shi, B., et al. (2023). Microbe-driven elemental cycling enables microbial adaptation to deep-sea ferromanganese nodule sediment fields. Microbiome 11:160. doi: 10.1186/s40168-023-01601-2
Zhang, D.-C., Liu, Y.-X., and Li, X.-Z. (2015). Characterization of bacterial diversity associated with deep sea ferromanganese nodules from the South China Sea. J. Microbiol. 53, 598–605. doi: 10.1007/s12275-015-5217-y
Keywords: food web, meiofauna, prokaryotes, biogeochemical cycling, auxotrophy
Citation: Montoya L and Escobar-Briones E (2025) Unveiling the significance of prokaryotic composition from ferromanganese crusts regarding the interlink between cobalt and vitamin B12 in deep-sea ecosystems. Front. Microbiol. 16:1524057. doi: 10.3389/fmicb.2025.1524057
Edited by:
Alberto Robador, University of Southern California, United StatesReviewed by:
Luigi Jovane, University of São Paulo, BrazilCopyright © 2025 Montoya and Escobar-Briones. This is an open-access article distributed under the terms of the Creative Commons Attribution License (CC BY). The use, distribution or reproduction in other forums is permitted, provided the original author(s) and the copyright owner(s) are credited and that the original publication in this journal is cited, in accordance with accepted academic practice. No use, distribution or reproduction is permitted which does not comply with these terms.
*Correspondence: Elva Escobar-Briones, ZXNjb2JyaUBjbWFybC51bmFtLm14