- 1Biomolecules, Metagenomics, Endocrine and Tropical Disease Research Group (BMETDREG), Kampala International University, Western Campus, Ishaka-Bushenyi, Uganda
- 2Biopharmaceutics Unit, Department of Pharmacology and Toxicology, School of Pharmacy, Kampala International University, Ishaka-Bushenyi, Uganda
- 3Department of Pharmaceutics and Pharmaceutical Technology, Kampala International University, Western Campus, Ishaka-Bushenyi, Uganda
- 4Department of Microbiology and Cell Science, University of Florida, Gainesville, FL, United States
- 5School of Life Sciences, University of Warwick, Coventry, United Kingdom
Background and objective: Despite its critical role in individual and societal health, food hygiene remains underexplored. Antibiotic-resistant pathogenic bacteria in ready-to-eat (RTE) food threaten public health. This scoping review collected data on the epidemiological prevalence of RTE food-contaminated pathogens resistant to antimicrobial drugs and resistance genes in Africa.
Method: Using electronic databases, such as PubMed, Scopus, and Web of Science (WoS), handpicked from references, pre-reviewed published articles were retrieved and analyzed according to the PRISMA-ScR guidelines.
Results: The findings indicate 40 previewed published articles qualified for meta-synthesis in the scoping review with a population/case ratio of 11,653/5,338 (45.80%). The most frequently reported RTE foods were meat or beef/beef-soup, chicken or poultry products, salads, vegetable salads, and sandwiches, which harboured pathogens such as E. coli, Salmonella, and Staphylococcus. Antibiotic susceptibility tests revealed the use of 48 antibiotics to manage infections, following CLSI (Clinical and Laboratory Standards Institute) protocols. Moreover, 10 authors reported 54 resistance genes associated with pathogenic resistant bacteria. In addition, only 15 studies received funding or financial support.
Conclusion: These findings from several researchers indicate that RTE street foods in African and resource-limited nations harbour enteric pathogens and are a significant concern to the public health system and reservoir of the spread of antibiotic resistance. This underscores the necessity of implementing effective control strategies to address challenges and limit the spread of resistant bacteria in RTE foods. The antimicrobial resistance surveillance system in the region is a significant concern. Notably, Africa needs to strengthen the national and international regulatory bodies and a health surveillance system on antimicrobial resistance, particularly among developing nations.
Introduction
Ready-to-eat (RTE) foods are foods prepared for consumption, and they may be raw or cooked, served hot or chilled, and often eaten without any additional treatment or processing (Lund, 2019; Valiati et al., 2023). Most RTE foods in Africa include hamburgers, salads, macaroni salad, cooked/roasted meats, smoked fish, sandwiches, cheese, mushroom pasta, deli-style cheese, burritos, fajitas, freshly made sushi, desserts, and ice-creams such as doughnuts etc. (Lund, 2019; Valiati et al., 2023). The consumption of diverse RTE foods in public spaces has become prevalent globally. RTE foods are widely consumed in low- and middle-income countries (LMICs) because of their convenience, affordability, and palatability (Makinde et al., 2020). Given the essential significance of food in human life, it is imperative to uphold food safety to protect individuals from foodborne illnesses and other associated health risks (Mengistu and Tolera, 2020). At the same time, it could be a reservoir of antibiotic resistance (Igere et al., 2022b, 2024; Onohuean and Igere, 2022). Mainstream research has extensively focused on environmental sources (such as water and soil) and clinical surveillance as major reservoirs of bacteria antibiotic resistance (Igere et al., 2022a; Onohuean and Nwodo, 2023b; Regassa et al., 2023; Satán et al., 2023). There is sparse data on the distribution and prevalence of antibiotic resistance from RTE foods.
Furthermore, food safety involves preventing chemical, biological, pathogens, and other health hazards from contaminating food. However, improper handling and serving of RTE street food can cause contamination with pathogens such as Salmonella, Vibrio spp., Campylobacter, E. coli, Listeria monocytogenes, Toxoplasma gondii, Clostridium botulinum, Moulds and other foodborne pathogens including Cyclospora, Hepatitis A, and Cronobacter sakazakii (Beshiru et al., 2019; Igere et al., 2022a; Onohuean and Igere, 2022, 2023). The use of contaminated water, inadequate hygiene, and environmental sanitation also impacts the contamination of RTE food, resulting in typhoid fever and diarrhoea infections (Igere et al., 2023; Onohuean and Nwodo, 2023a). Therefore, RTE foods prepared with contaminated ingredients, water, and in an unsanitary setting can contain these bacteria, which significantly impacts public health.
Food contaminated with antibiotic-resistant pathogenic microorganisms poses a significant risk to public health. In addition to infecting people, they serve as potential reservoirs of antimicrobial resistance, facilitating the transfer of antibiotic-resistant components to both related and unrelated bacterial species (Odu and Akano, 2012; Beshiru et al., 2020; Onohuean and Igere, 2022). This has contributed to the global increase in antibiotic resistance among foodborne bacteria and infections in recent years. Regrettably, in many low-income African nations, most food vendors lack significant monitoring or licences by appropriate agencies or organizations overseen or accredited by relevant bodies or organizations to validate these RTE food safety. Again, these RTE food vendors could have compromised their products due to the environment and methods employed, increasing the likelihood that some are infected with bacterial infections. Although critical to health and productivity, food hygiene has been significantly overlooked in African research.
Antimicrobials, including antibiotics, are medications used to treat or prevent bacterial infections in humans and animals. Globally, antimicrobial resistance (AMR) in RTE foods has impacted product safety and quality. Countries with rigorous food safety standards, such as the European Union and the United States, impose stricter limitations on the use of antimicrobial agents in food production (Walia et al., 2019; Kättström et al., 2022; Taylor et al., 2022). In LMICs, where agricultural practices frequently lack control, the unrestrained use of antibiotics in animal production facilitates the emergence of resistant bacteria that may infiltrate the food chain (Samtiya et al., 2022). Due to their limited processing and frequent consumption without additional cooking, RTE foods present an increased risk of the spread of antimicrobial-resistant bacteria among consumers. Furthermore, the use of antimicrobials is affected by AMR, which constitutes a significant global public health and developmental threat. Bacterial AMR is thought to have contributed to 4.95 million fatalities worldwide in 2019 and been directly responsible for 1.27 million deaths (Murray et al., 2022; WHO, 2023).Resistance is a natural occurrence intensified by excessive or improper use (WHO, 2018; Onohuean et al., 2022a; Somda et al., 2023). Followed by mutations, gene transfer, and target modification, antimicrobial-sensitive organisms can develop microbial resistance to antimicrobials (Muteeb et al., 2023; Onohuean and Igere, 2023). Bacteria can increase antibiotic resistance through many processes, which differ according to species and origin. Thought resistance was common among clinical strains, it has become a widespread environmental and food bacterial isolate (Somda et al., 2021); one of the biggest public health issues is global.
Research has revealed a troubling degree of antibiotic resistance in the bacterial pathogens present in these foods, potentially resulting in profound health implications for consumers. The 11 AMR genes included tetracycline (tetA, tetB, tetM), aminoglycoside (aadA, aphA-1), sulphonamide (sulI, sulII), chloramphenicol (cmlA, floR), erythromycin (ermB), and disinfection resistance genes (qacE). TetA has been reported as the most prevalently identified gene in food samples, with a frequency of 100% across all samples (Xiong et al., 2019). Also, the study by Somda et al. (2023) identified tet(A), tet(B), tet(C), tet(K), blaTEM, catA1, catA2, cmlA, blaCTXM, qnrA, qnrB, qnrS, parC, and qepA4 genes as typical in foodborne pathogenic bacteria in West Africa.
The widespread prevalence of antibiotic-resistant pathogenic bacteria in RTE foods significantly contributes to human multidrug resistance. The food chain acts as a transmission route for resistant strains, facilitating the acquisition and of transfer resistance genes, which pose significant health risks. Evidence has shown that multidrug-resistant bacteria in food correlate with the growth of antibiotic-resistant illnesses in humans, thereby complicating treatment (Himanshu et al., 2022; Choy et al., 2024). This underscores the critical need for surveillance data to inform policymakers and stockholders about enhanced food safety protocols. The goal of this scoping review is to create a database for RTE food resistance to antimicrobial drugs in Africa. To identify the most incriminated pathogens, we assessed the evolution of antibiotic resistance in bacteria isolated from RTE food samples and determined the prevalence of antibiotic resistance genes in RTE food.
Methodology
Study design and article search strategies
This study adopted the protocol of Systematic Reviews and Meta-Analyses extension for Scoping Reviews (PRISMA-ScR) (Tricco et al., 2018) for the study selection process of pre-review published articles from African countries. Using specific keywords and wildcard* procedural details in Supplementary file. Research articles from PubMed, Scopus, and Web of Science (WoS), published in English between 2000 and 2024, were retrieved on 25 September 2024, and updated plus handpicked from references on 29 October 2024 at 11:05 p.m. The dataset was merged and normalized using the ScientoPy and fBasics R packages, duplicates were eliminated, and the final compilation was saved in CSV or Excel format (Rstudio Team, 2020; Onohuean et al., 2022b, 2022c).
Eligibility criteria
Articles that satisfied the specified inclusion criteria were included in the systematic review. Research articles conducted within the African nations between 2000 and 2024, a defined research methodology such as cross-sectional, survey, experimental or bacteriological research, conventional phenotypic method or genotypic primary Polymerase chain reaction (PCR) methods or molecular methods for antibiotic resistance genes or whole genome sequencing and MALDI-TOF mass spectrometry to identify the prevalence of antibiotic-resistant pathogenic bacteria of public health-relevant bacteria, such as Salmonella, Staphylococcus aureus, Vibrio spp., and E. coli, and resistance genes in RTE foods, articles published in English in language, studies conducted on various types of RTE foods in Africa nations qualified for inclusion. The excluded articles were review articles, studies on antibiotic resistance genes in artificially contaminated bacteria or machine learning studies, research theses, opinion pieces, book chapters, non-peer-reviewed works, clinical or environmental sample sources that are not RTE foods, and conference abstracts for which full articles were not readily available.
Outcome of interest
The outcome of interest based on the objective of this systematic review is to determine the prevalence of RTE food-contaminated pathogens (E. coli, Salmonella, Staphylococcus aureus, etc.), their resistance to antimicrobial drugs, and resistance genera within African nations.
Assessment of data quality
The Newcastle-Ottawa Scale (NOS), approved by the Agency for Healthcare Research and Quality (AHRQ), was used to evaluate the quality of the data included in this scoping review and analysis (Onohuean et al., 2022c). Using a star rating system, the quality of the studies was evaluated based on three categories: the selection of research groups received a maximum of 4 stars, variables include articles source or type of RTE foods, representativeness sample of community or population bacteria or contaminated pathogens, antimicrobial drugs, and/or resistance-genes. The comparability of groups received a maximum of 2 stars, variables assessed include methods and period, and the assessment of outcomes received a maximum of 3 stars, variables that clearly defined and reliably measured outcomes such as the present of RTE food-contaminated pathogens, antimicrobial drugs, and resistance-genes.
Data extraction
The two authors (HO and OH) independently analyzed the titles and abstracts of the retrieved datasets for potentially appropriate studies. Studies not conducted in Africa, as well as literature reviews, systematic reviews, conference papers, and opinion pieces, were excluded. The complete texts of the selected papers were meticulously analyzed, and the results aligned with the objectives of the scoping review, which was a meta-synthesis using a data extraction tool. The information, including title of articles, authors/year, countries, source (RTE foods), method, types of bacterial isolates, sample population, antibiotic/antimicrobial used, antibiotic susceptibility pattern, resistant genes, and funders, was obtained in the data extraction form.
Data analysis
The retrieved data were used for descriptive statistics. Subsequent analysis was conducted at several stages in Excel 2013 R version 4.1.0 software, and findings are presented in figures, graphical heatmaps, etc.
Results
Summary findings from the literature search
From the total of 668 articles identified in the searched different databases, 455 passed the initial screening, 198 were retrieved, 149 were eligible, and 40 published articles qualified for inclusion in the meta-synthesis in the scoping review (Figure 1). In the African region, the nation’s Nigeria (n = 18 articles), Ethiopia (n = 3 articles), and South Africa (n = 3 articles) were the countries most frequently reported on the national distribution of antibiotics recovered from RTE foods (Figure 2). However, only one study has been reported on mixed West African countries (Ghana, Nigeria, Benin, and Togo).
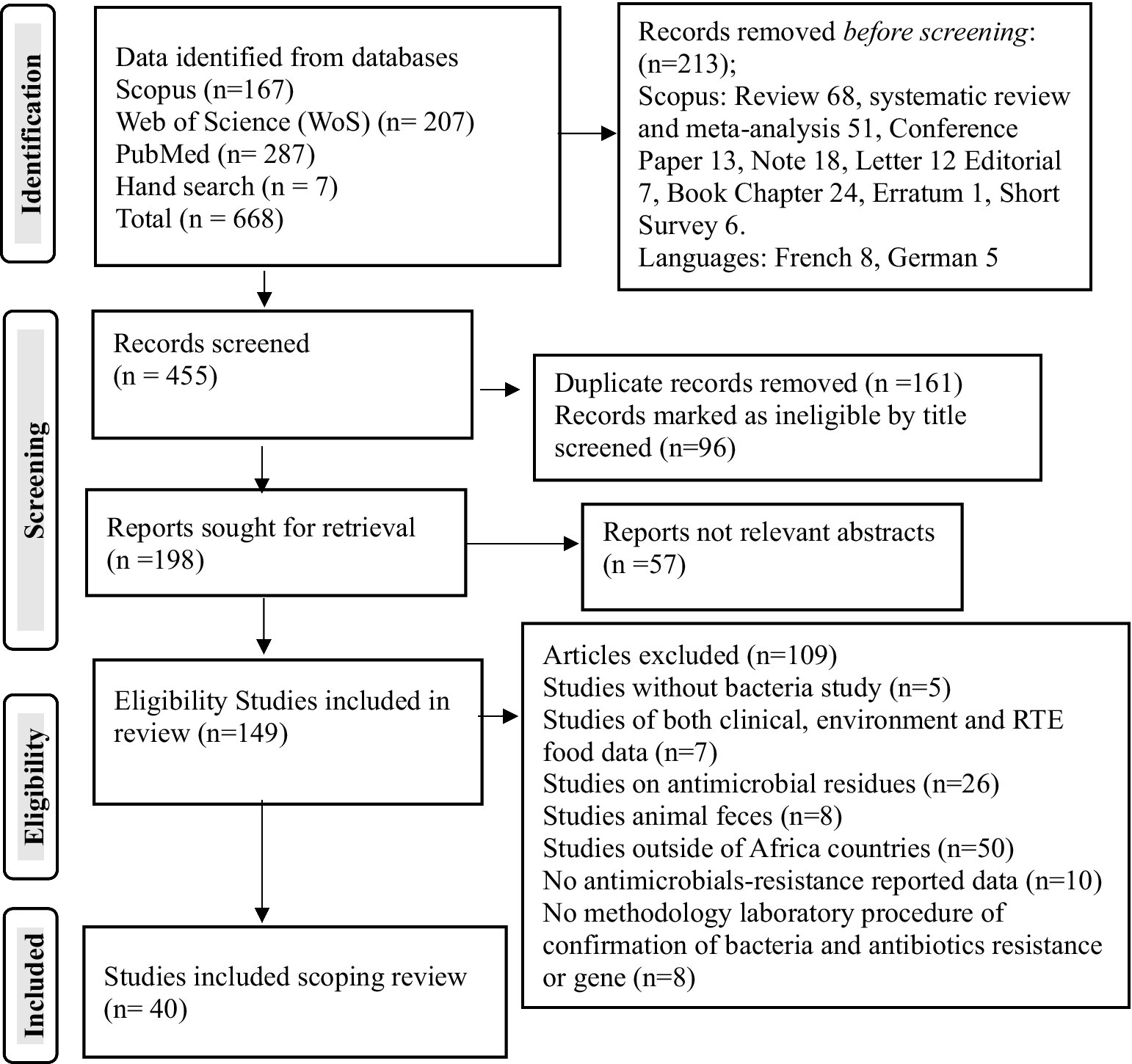
Figure 1. PRISMA study selection flowchart on antibiotic-resistant pathogenic bacteria, prevalence rates, and resistance genes in RTE foods in Africa.
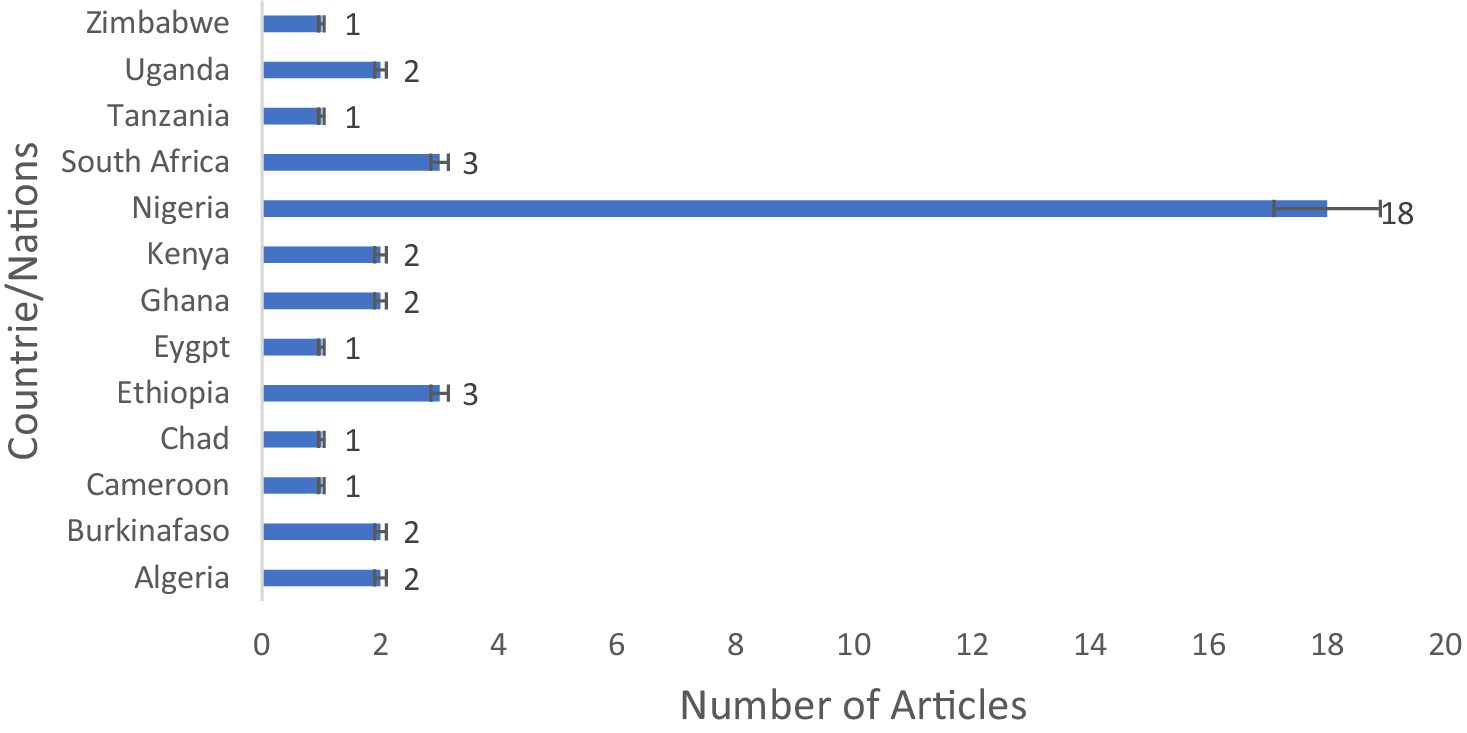
Figure 2. Study localities on antibiotic-resistant pathogenic bacteria, prevalence rates, and resistance genes in RTE foods in Africa.
Quality assessment
In Supplementary Table 1, we present the particular information on the evaluation questions listed according to a domain for each article and the quality evaluation ratings of the research that were included. On the other hand, the comparability of the NOS variables did not reward any of the research papers that were evaluated with any stars because no comparative data/studies were found in the included articles. However, the quality ratings of the included studies ranged from 8 to 10. A total of 12 studies were awarded 10 points out of the potential 10 points, 18 were awarded 9 points, and 10 were awarded 8 points.
Articles included
The articles used in this scoping review were Oladipo and Adejumobi (2010); Nyenje et al. (2012); Akinyem et al. (2013); Zige (2013); Aminu and Umeh (2014); Akinnibosun and Ojo (2015); Owoseni and Onilude (2016); Tesfaye et al. (2016); Yaici et al. (2017); Ananias and Roland (2017); Ebakota et al. (2018); Morshdy et al. (2018); Okoli et al. (2018); Tshipamba et al. (2018); Blessed (2018); Ndunguru and Ndossi (2020); Okafor-Elenwo and Imade (2020a, 2020b); Okubo et al. (2020); Asiegbu et al. (2020); Claudious et al. (2020); Fayemi et al. (2021); Izevbuwa and Okhuebor (2021); Makinde et al. (2021); Mayoré et al. (2021); Mekhloufi et al. (2021); Nikiema et al. (2021); Setsoafia Saba et al. (2021); Karikari et al. (2022); Mwove et al. (2022); Soubeiga et al. (2022); Esemu et al. (2023); Alelign et al. (2023); Ronald et al. (2023); Beshiru and Igbinosa (2023); Akinyele et al. (2024); Isic et al. (2024); Moges et al. (2024); Umar et al. (2024).
Population/cases of antibiotic-resistant pathogenic bacteria in RTE foods in African countries
In Africa, antibiotic-resistant pathogenic bacteria were found in 5,338 out of 11,653 (45.80%) samples of RTE foods. However, the sample size and cases by nations were Nigeria (59.05%, 128.9% cases), Ethiopia (6.87%, 14.99% cases), and South Africa (4.91%, 10.72% cases) were the countries most frequently reporting antibiotic-resistant bacteria from RTE foods (Table 1 and Figure 3). The, one study involving a combination of West African countries (Ghana, Nigeria, Benin, and Togo) reported fewer occurrences, accounting for only 0.37% of the total samples and 0.81% of the cases.
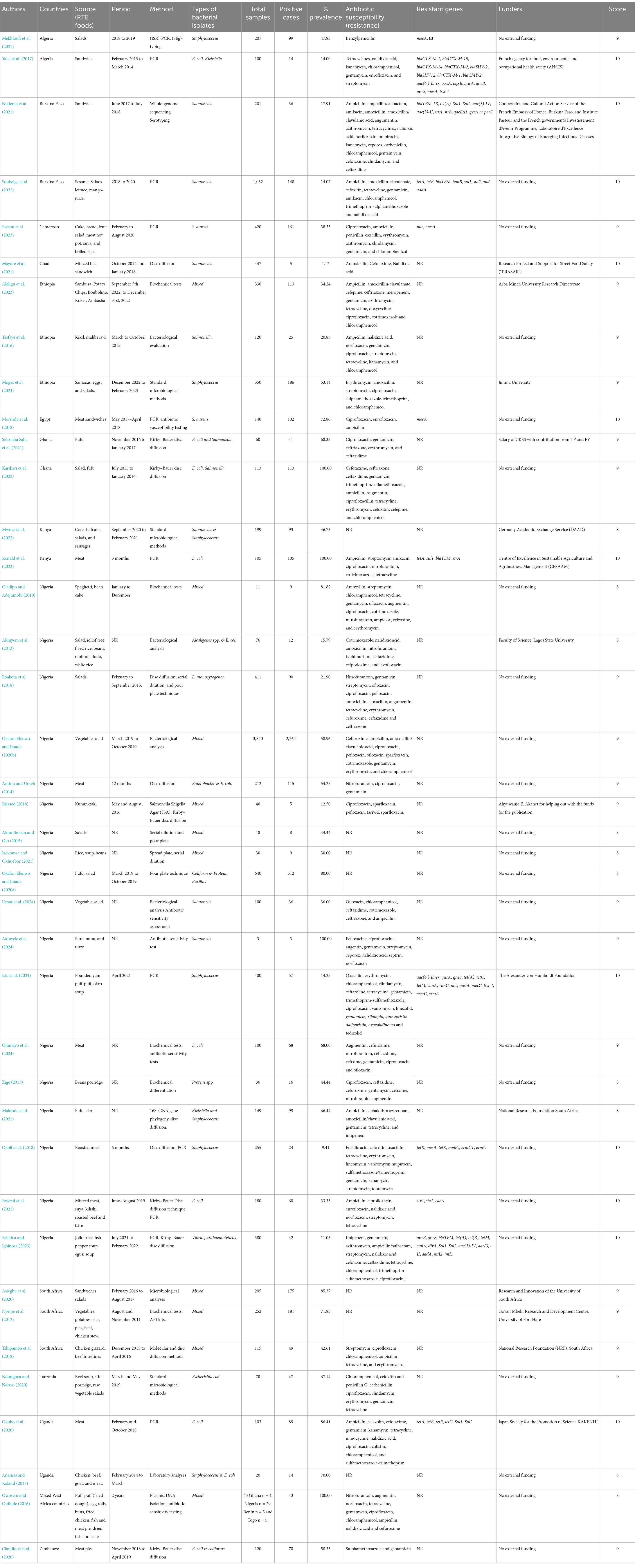
Table 1. Overall characteristics of the included studies on antibiotic-resistant pathogenic bacteria, prevalence rates, and resistance genes in RTE foods in Africa.
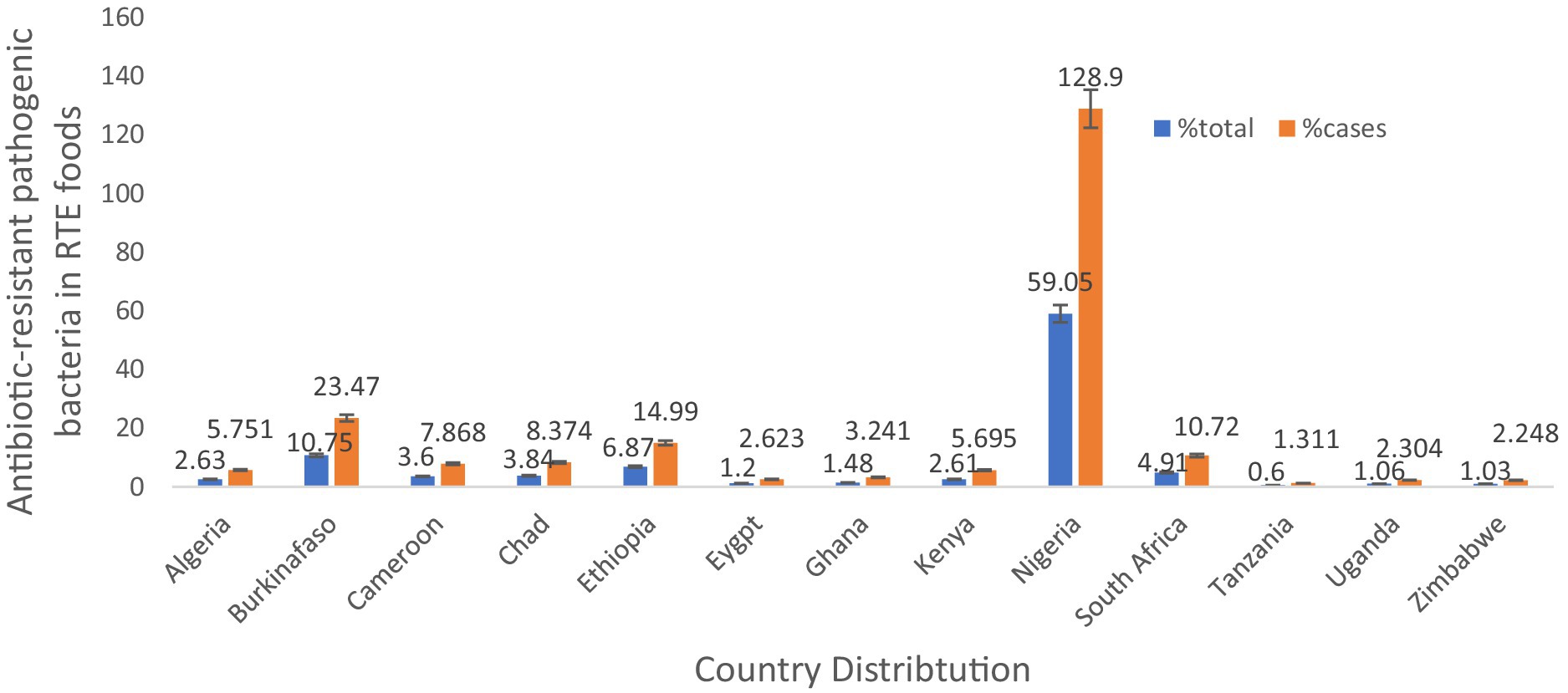
Figure 3. Country distribution of antibiotic-resistant pathogenic bacteria, prevalence rates in RTE foods in Africa.
Food types/pattern, period, and study method of antibiotic-resistant pathogenic bacteria in RTE foods
The primary authors reported different RTE foods; however, the most reported RTE foods were meat or beef/beef-soup, chicken or poultry products, salads, vegetable salads, sandwiches (Table 1). A total of 32 authors reported a study period ranging from 1 month to 2 years. All the included studies reported that the method applied comprised whole-genome sequencing, conventional PCR, serotyping, bacteriological analysis, or other standard microbiological laboratory techniques (Table 1).
Isolated antibiotic-resistant pathogenic bacteria in RTE foods
All the authors reported the isolated bacteria. Interestingly, 11 authors reported more than three bacterial isolates in their studies such as Yaici et al. (2017) (E. coli, K. pneumoniae, K. oxytoca), Alelign et al. (2023) (Staphylococcus aureus, Salmonella species, and E. coli), Oladipo and Adejumobi (2010) (Bacillus licheniformis, Aeromonas hydrophila, Enterobacter aerogenes, Bacillus cereus, Proteus mirabilis, Pseudomonas putida, Proteus vulgaris, Pseudomonas cholororaphi and Proteus morganii), Okafor-Elenwo and Imade (2020b) (Serratia, Citrobacter, Proteus, Staphylococcus and Bacillus, with Proteus), Blessed (2018) (C. freundii, E. coli, P. mirabilis, P. vulgaris), Akinnibosun and Ojo (2015) (E. coli, Pseudomonas aeroginosa, Staphylococcus aureus), Izevbuwa and Okhuebor (2021) (E. coli, Streptococcus spp., Staphylococcus aureus, Pseudomonas aeroginosa, Salmonella spp., Enterobacterspp), Asiegbu et al. (2020) (Enterobacteriaceae, L. monocytogenes, S. aureus), Tshipamba et al. (2018) (Staphylococcus aureus S. aureus, Enterococcus faecalis, Planomicrobium glaciei), Owoseni and Onilude (2016) (Citrobacter, Edwardsiella, Enterobacter, Escherichia coli, Klebsiella, Proteus, Salmonella, Serratia, and Shigella). Of all these different bacteria in any study, more than three were classified as mixed antibiotic-resistant pathogenic bacteria of public health significance. All other authors reported one or two antibiotic-resistant pathogenic bacteria in their studies, which are included in the scoping review in Figure 4 and Table 1.
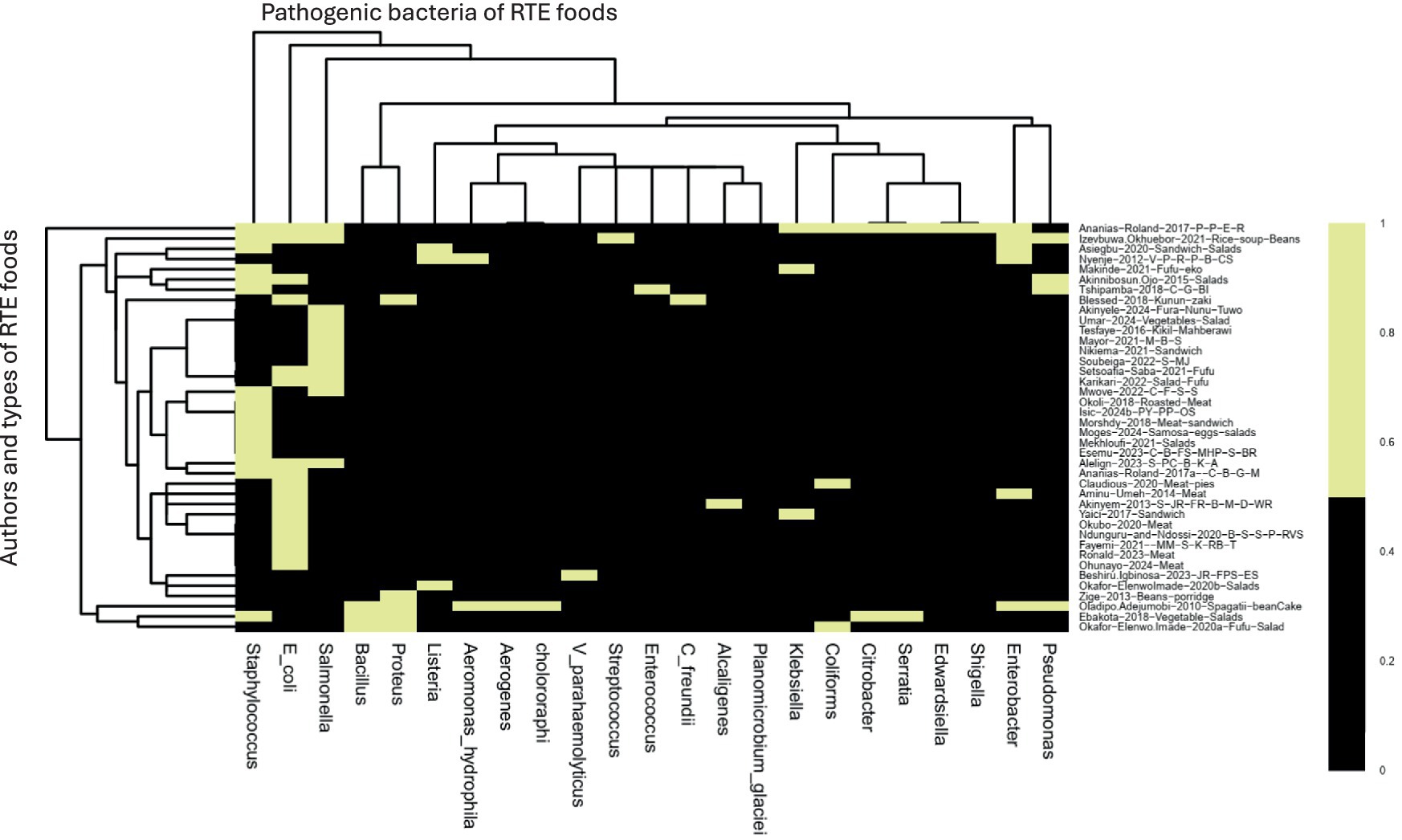
Figure 4. The heatmap analysis of the RTE foods samples on antibiotic-resistant pathogenic bacteria, prevalence rates, and resistance genes in RTE-foods. Black = author and antibiotic-resistant pathogenic bacteria reported. Yellow = not present. S_MJ = Salads, mango-juice. C_B_FS_MHP_S_BR = Cake, bread, fruit-salad, meat-hot-pot, suya, boiled-rice. M_B_S = Minced beef sandwich. S_PC_B_K_A = Sambusa, Potato-Chips, Bonbolino, Koker, Ambasha. C_F_S_S = Cereals, fruits, salads, sausages. S_JR_FR_B_M_D_WR = salad, jollof-rice, fried-rice, beans, moimoi, dodo, white-rice. PY_PP_OS = Pounded-Yam Puff-Puff, Okro-soup. MM_S_K_RB_T = Minced-meat, suya, kilishi, roasted-beef and tsire. JR_FPS_ES = Jollof-rice, fish-pepper-soup, Egusi-soup V_P_R_P_B_CS = Vegetables, potatoes, rice, pies, beef, chicken-stew. C_G_BI = Chicken gizzard, beef-intestines. B-S_S-P_RVS = Beef-soup, stiff-porridge, raw-vegetable-salads. C_B_G_M = chicken, beef, goat, meat. P-P_E_R = Puff-puff, egg rolls.
Antibiotic susceptibility patterns and resistance genes against antibiotic-resistant pathogenic bacteria in RTE foods
A total of 30 authors of the included studies reported the antibiotic susceptibility patterns of 48 antibiotics used to manage pathogenic bacterial infections following the CLSI protocols. The antibiotics reported are amoxicillin, amoxicillin-clavulanic acid, ampicillin, Augmentin, azithromycin, benzylpenicillin, carbenicillin, cefepime, cefotaxime, cefoxitin, cefpodoxime, ceftaroline, ceftazidime, cefixime, ceftriaxone, cephalothin, cefuroxime, chloramphenicol, ciprofloxacin, clindamycin, cloxacillin, cotrimoxazole, doxycycline, enrofloxacin, erythromycin, fusidic acid, gentamycin, imipenem, kanamycin, levofloxacin, linezolid, nalidixic acid, nitrofurantoin, norfloxacin, ofloxacin, oxacillin, pefloxacin, penicillin G., sparfloxacin, streptomycin, sulphamethoxazole, tedizolid, teicoplanin, tetracycline, trimethoprim, trimethoprim-sulfamethoxazole, typhimurium and vancomycin. A heatmap cluster analysis highlighted the antibiotics used for bacterial isolates in each study. The two colours in the heatmap denote susceptibility patterns, such as intermediate/dose-dependent and resistance, represented with black, whereas those studies that did not report any pattern were denoted with yellow of isolates (Figure 5A). The countries’ distribution of resistance bacteria isolates to the antibiotics recovered from the RTE food among the reported studies showed Nigeria samples to have high resistance to ampicillin (4,159), cefuroxime (4,150), chloramphenicol (2,108), cotrimoxazole (2,241), gentamycin (2,302), and branded amoxicillin/clavulanic acid (1,346). Studies in Ghana showed resistance to ceftazidime (85), ceftriaxone (78), and sulphametoxazole trimethoprim (73), and ampicillin (58) isolates. Studies in Ethiopia have reported isolates resistant to erythromycin (162), ampicillin (91) and amoxicillin (68), as shown in Figure 5B.
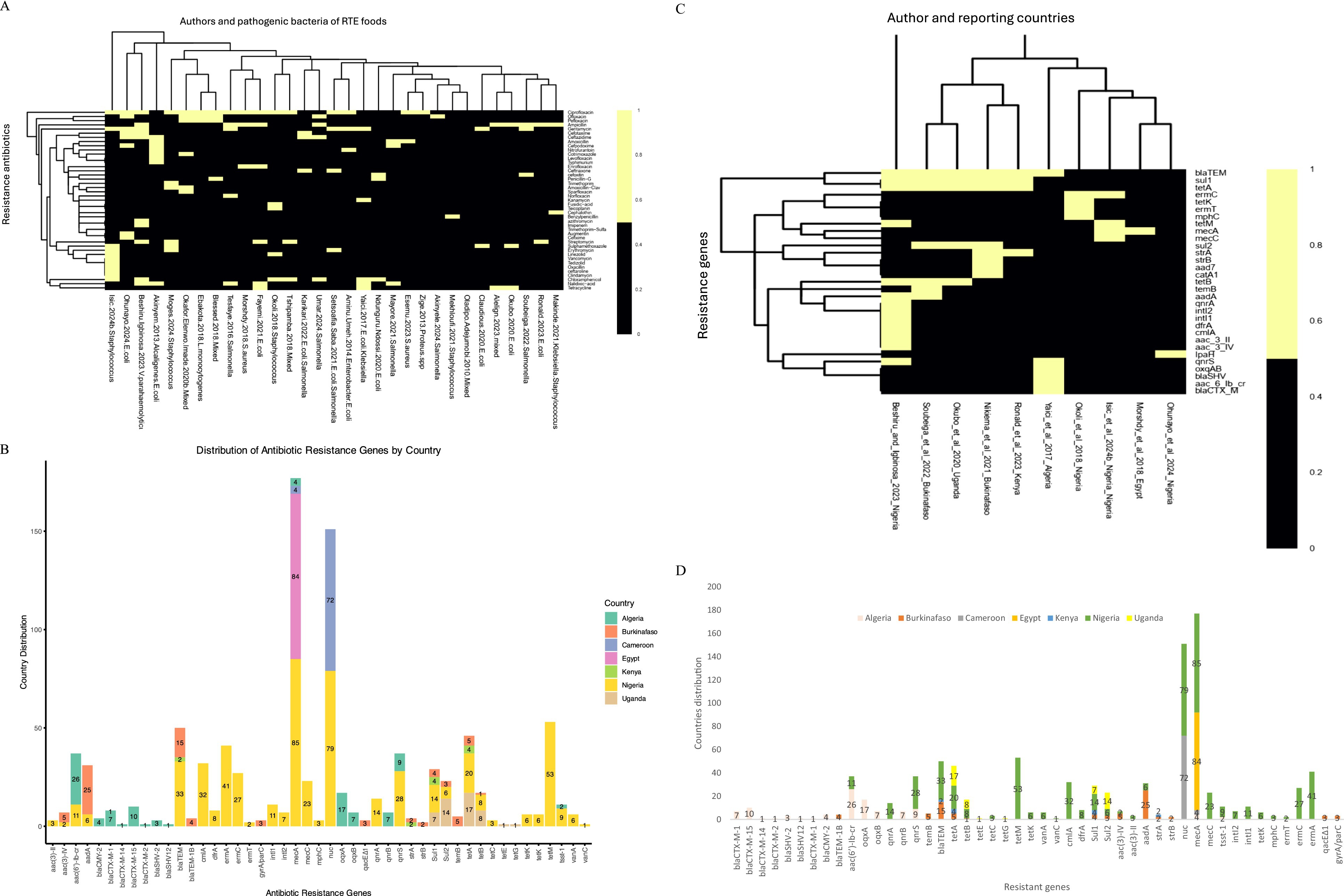
Figure 5. (A) The heatmap analysis of the susceptibility pattern on antibiotic-resistant pathogenic bacteria, prevalence rates, and resistance genes in RTE-foods. Black = intermediate/dose-dependent, and yellow = do not report any susceptibility pattern of isolates. (B) The countries distribution of resistance antibiotics on antibiotic-resistant pathogenic bacteria, prevalence rates, and resistance genes in RTE-foods. ampicillin/ST = ampicillin/sulbactam, amoxicillinCA = amoxicillin/clavulanic acid, QuinupristinD = Quinupristin-dalfopristin SulphametoxazoleT = Sulphametoxazole–trimethoprim. (C) The heatmap analysis of the resistant genes on antibiotic-resistant pathogenic bacteria, prevalence rates, and resistance genes in RTE-foods. Black = Present of the tested resistant genes, yellow = not Present. (D) The countries’ distribution of resistance genes on antibiotic-resistant pathogenic bacteria, prevalence rates, and resistance genes in RTE-foods.
Similarly, 10 authors reported resistance genes in seven nations in their studies, such as aac3II, aac3IV, aac6Ibcr, aad7, aadA, blaCTXM, blaSHV, blaTEM, catA1, cmlA, dfrA, ermC, ermT, intI1, intI2, IpaH, mecA, mecC, mphC, oxqAB, qnrA, qnrS, strA, strB, sul1, sul2, temB, tetA, tetB, tetK and tetM (Figure 5C). The majority of the resistance genes (blaCTX-M-1, blaCTX-M-15, blaCTX-M-14, blaCTX-M-2, blaSHV-2, blaSHV12, blaCTX-M-1, blaCMY-2, aac(6′)-Ib-cr, oqxA, oqxB, qnrB, qnrS) were reported in Algeria samples in Figure 5D and code for resistance to antibiotics such as beta-lactams (penicillins, cephalosporins, carbapenems), quinolones and glycopeptides (Table 2). In Nigeria, samples were observed to have several genes, namely, qnrA, qnrS, temB, blaTEM, tet(A), tet(B), tetM, cmlA, dfrA, Sul1, Sul2, aac(3)-IV, aac(3)-II, aadA, nuc, mecA, tsst-1, intI2, intI1, tetK, mphC, ermT, and ermC (Figure 5D) that are responsible for quinolones, penicillins, tetracyclines, chloramphenicol, aminoglycosides, sulphonamides, glycopeptides, nitrofurantoin, and macrolides (Table 2). In Burkina Faso, the studies show about seven resistance genes, temB, blaTEM, tet(A), tet(B), Sul1, Sul2 and aadA (Figure 5D) and that engenders resistance to beta-lactams (penicillins, cephalosporins, and carbapenems) and sulphonamides. In Cameroon, the included studies show two prevalence resistance genes: nuc and mecA, which implies resistance to oxacillin. In Kenya, blaTEM, tet(A), Sul1, and strA are the prevalent genes accountable for resistance to beta-lactams (penicillins, cephalosporins, and carbapenems) and sulphonamides. In Uganda, studies have shown the occurrence of tet(A), tet(B), tetE, tetG, Sul1, and Sul2 responsible for the resistance we find in tetracycline and sulphonamides. Egypt’s study shows sea and sec resistance genes for glycopeptides, as shown in Figure 5D and Table 2.
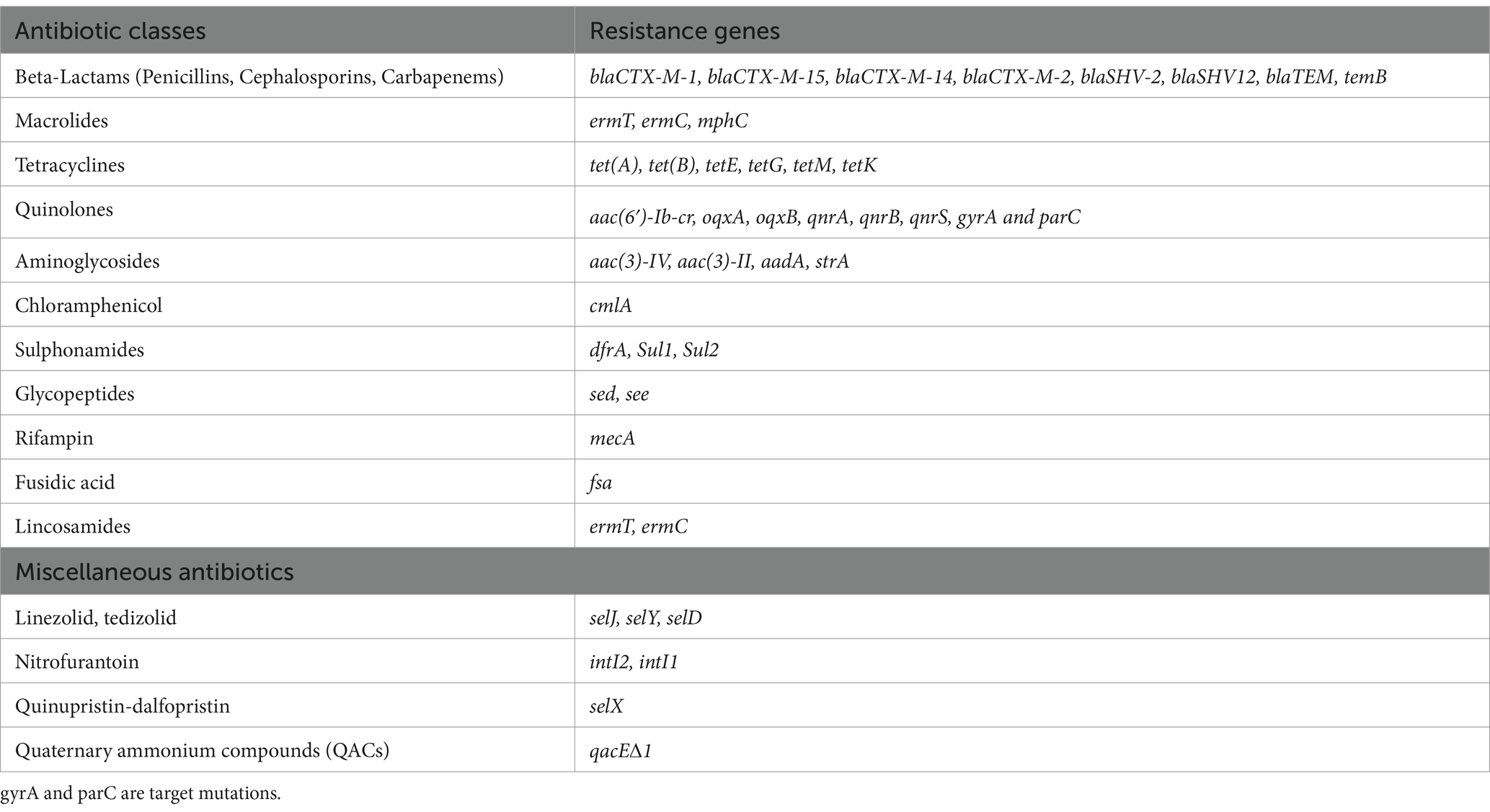
Table 2. The summary of resistance genes based on antibiotic classes in antibiotic-resistant pathogenic bacteria, prevalence rates, and resistance genes in RTE foods.
Funders of antibiotic-resistant pathogenic bacteria in RTE foods
Only 15 authors in the included studies received funding and support for research on antibiotic-resistant pathogenic bacteria in RTE foods. Yaici et al. (2017) by the French Agency for food, environmental and Occupational Health Safety (ANSES), Nikiema et al. (2021) by Cooperation and Cultural Action Service of the French Embassy of France, Burkina Faso and Institute Pasteur and the French government’s Investissement d’Avenir Programme, Laboratoire d’Excellence ‘Integrative Biology of Emerging Infectious Diseases, Mayoré et al. (2021) by Research Project and Support for Street Food Safety (“PRASAR”), Alelign et al. (2023) by Arba Minch University Research Directorate, Moges et al. (2024) by Jimma University, Setsoafia Saba et al. (2021) by Salary of CKSS with contribution from TP and EY, Mwove et al. (2022) by Germany Academic Exchange Service (DAAD), Ronald et al. (2023) by Centre of Excellence in Sustainable Agriculture and Agribusiness Management (CESAAM), Akinyem et al. (2013) by Faculty of Science, Lagos State University, Blessed (2018) by Ahyuwanie. E. Akanet for the publication funds, Isic et al. (2024) by The Alexander von Humboldt Foundation, Makinde et al. (2021) by National Research Foundation South Africa, Asiegbu et al. (2020) by Research and Innovation of the University of South Africa, Nyenje et al. (2012) by Govan Mbeki Research and Development Centre, University of Fort Hare, Tshipamba et al. (2018) by National Research Foundation (NRF), South Africa.
Discussion
Microbial contamination is a metric for the efficacy of food safety practices, recognized worldwide as a conduit for pathogen transmission. This scoping review indicated that all the included articles were presented with high population cases of antibiotic-resistant pathogenic bacteria in RTE food at varied degrees of contamination and are classified as unsatisfactory regarding microbiological quality. This findings from several researchers indicate that RTE street foods in the African and resource-limited nations harbour enteric pathogens and is a significant concern to public health system as well reservoir of spread of antibiotic resistance (Oladipo and Adejumobi, 2010; Nyenje et al., 2012; Akinyem et al., 2013; Zige, 2013; Aminu and Umeh, 2014; Akinnibosun and Ojo, 2015; Owoseni and Onilude, 2016; Tesfaye et al., 2016; Yaici et al., 2017; Ananias and Roland, 2017; Ebakota et al., 2018; Morshdy et al., 2018; Okoli et al., 2018; Tshipamba et al., 2018; Blessed, 2018; Ndunguru and Ndossi, 2020; Okafor-Elenwo and Imade, 2020a, 2020b; Okubo et al., 2020; Asiegbu et al., 2020; Claudious et al., 2020; Fayemi et al., 2021; Izevbuwa and Okhuebor, 2021; Makinde et al., 2021; Mayoré et al., 2021; Mekhloufi et al., 2021; Nikiema et al., 2021; Setsoafia Saba et al., 2021; Karikari et al., 2022; Mwove et al., 2022; Soubeiga et al., 2022; Esemu et al., 2023; Alelign et al., 2023; Ronald et al., 2023; Beshiru and Igbinosa, 2023; Akinyele et al., 2024; Isic et al., 2024; Moges et al., 2024; Umar et al., 2024). The notable progress in research concerning antibiotic-resistant pathogenic bacteria in RTE food could be influenced by the significance of the global threat of bacterial resistance and the emergence of infectious pathogens and outbreaks. The greater number of publication funds in Nigeria may be due to the greater number of universities and research institutions in Nigeria compared to other nations. Moreover, the level of educational attainment by the population surpasses that of other countries (Tanko et al., 2020). Other nations have very low research output on antibiotic-resistant pathogenic bacteria in RTE food, which could be elucidated by the fact that most African nations disseminate their research articles in local journals that are not listed or indexed in international databases.
This review underscores the public health risks posed by antibiotic-resistant bacteria in RTE foods, which contribute to foodborne illnesses in Africa. This specific focus on foodborne bacteria is associated with the emergence of resistance reported in environmental domains in recent years by various investigations. The bacteria present in these regions are identical to those associated with food linked to human activity, which may facilitate the development of multidrug-resistant bacteria. Different categories of food commodities have been recognized as highly consumed, as evidenced by the volume of publications concerning RTE food contamination. It can be asserted that food safety information in Africa is inadequate and disjointed, stemming from insufficient surveillance, documentation, and reporting. Furthermore, it reflects and agrees with the report of Paudyal et al. (2017) of poor or inefficient resource utilization, activity duplication, and a lack of synergy among countries in the African region. Our scoping review identified several primary categories of RTE food: chicken or poultry products, meat or beef/beef soup, and animal products and vegetables, including salads, sandwiches, and others. However, animal-derived food, such as poultry and meat products, has been widely identified as a source of illness and infections (Shange et al., 2019; Somda et al., 2023). The presence of enterobacteria in raw vegetable salads is due to their extensive distribution in soil, water, animal and human intestines, and plants. Some enterobacteria are naturally found in vegetable flora (Sagoo et al., 2003; Toe et al., 2017; Somda et al., 2023). At the same time, there are no EU or US criteria for Enterobacteriaceae in salad vegetables, as they naturally present in high quantities.
Epidemiological studies indicate that RTE foods are the primary sources of infectious diseases caused by Campylobacter, Yersinia, E. coli, Salmonella nontyphoidal, and several other pathogens, particularly in low-income nations in Africa. This scoping review indicated that E. coli, Salmonella spp., and Staphylococcus spp. were predominantly responsible for African food contamination during the study period. Most of the articles evaluated indicated that food contamination by microorganisms is associated with inadequate or violations of hygienic regulations in either handling or preparation. Moreover, pathogen-induced food contamination may be associated with the quality of raw materials, water, environment, and the existence of reservoirs and vectors in or next to food production or service locations (Igbinosa et al., 2017; Igere et al., 2022a; Onohuean and Igere, 2022; Onohuean and Nwodo, 2023a).
The public health system in the study region is generally threatened by the consumption of pathogens that contaminate RTE foods. This has been impacted by the increased use of antibiotics, particularly in populations at high risk of illness and life-threatening conditions. Aggravated by the impoverished settings of many African nations, antibiotics are indiscriminately used and sold in public spaces. All these variables significantly contribute to the proliferation of bacterial resistance to antibiotics. Furthermore, adverse economic conditions correlate with malnutrition, lack of access to safe drinking water, and poor hygiene; these are prevalent factors among populations in resource-limited countries that face an increased risk of infection and the transmission of resistant bacteria. In addition, antibiotic-resistant bacteria can infect humans through various situations: indirectly via the food chain through the ingestion of contaminated RTE foods or through direct contact with colonized or infected animals or biological materials such as blood, urine, faeces, saliva, semen, etc. (Cassini et al., 2019).
In this scoping review, E. coli, Salmonella, and Staphylococcus were frequently isolated resistance bacteria and were more implicated in foodborne infectious diseases, in agreement with previous findings (Adefisoye and Okoh, 2016; Igbinosa et al., 2017; Beshiru et al., 2020; Onohuean and Igere, 2022; Beshiru and Igbinosa, 2023). Several antibiotics are engaged in the management of related illnesses, thereby impacting the use of antibiotics and developing resistance to these bacteria. This indicates the overuse of antibiotics in agriculture, veterinary care, and humans. The various analyzed articles indicate the occurrence of genes including tetA, tetB, tetC, and tetK, which confer resistance to tetracycline, as well as blaTEM, catA1, cmlA, blaCTXM, and genes associated with quinolone resistance (qnrA, qnrB, qnrS, parC, and qep), similar to the findings by Igbinosa et al. (2017) and Kimera et al. (2020). However, few studies have reported resistance signatures or gene coding in isolated bacteria. This implies that analytical laboratories in many nations that were examined in this study lack sufficient technology, rendering them unable to identify specific genes associated with antibiotic resistance. Some experts assert that employing sophisticated techniques like whole genome sequencing may enhance the comprehension of the quantity, dissemination, and development of multidrug-resistant organisms.
The proliferation of resistance CTX-M in RTE foods is primarily attributed to human activities, as the majority of E. coli CTX-M, particularly the producer of the enzyme CTX-M-15, circulates clonally, which dominate the dominant faecal E. coli clones in humans, such as ST131, ST95, ST69, ST393, ST405, and ST10 (Sharma et al., 2020; Aworh et al., 2021). Another potential cause of increased antibiotic resistance in RTE food may be linked to resistance genes present in sewage, water or wastewater, soil and environmental indices (Igere et al., 2020, 2024; Onohuean et al., 2021), especially genes such as [aadA, blaOXA, blaOXA, erm (B), erm (F), mef (A), mph (E), sul1, sul3, clust, tet (39), tet (Q), tet (W)]. Interestingly, these wastewater or sewage systems are used for the direct irrigation of crops, particularly in the urban centres of numerous African cities, and are occasionally discharged into streams and rivers that humans and animals ingest in Africa. Studies from different African nations have indicated that Staphylococcus isolated from various sources (pigs, pig carcasses, chicken and handler’s carcasses) carry the mecA gene (Igbinosa et al., 2016; Okoli et al., 2018; Ouoba et al., 2019; Mamfe et al., 2021; Somda et al., 2023). Igbinosa et al. (2016) provided a comprehensive identification of Staphylococci in meat, which is frequently associated with inadequate hygienic measures during slaughtering, shipping, processing, storage, and retail by those engaged in the production process. However, the mecA gene encodes a penicillin-binding protein (PBP2a) that is linked to a markedly reduced affinity for beta-lactams. Its presence induces methicillin resistance in Staphylococcus (Igbinosa et al., 2016; Somda et al., 2023). qacEΔ1 is prevalent for the QAC resistance gene in Gram-negative bacteria and a deletion mutation of qacE (Hrovat et al., 2023).
Furthermore, the selection of antibiotics and patterns of antimicrobial consumption in some geographical locations in Africa are influenced by food, animal species, regional production patterns, types of farming systems (intensive or extensive), farming purposes (commercial, industrial, or domestic), coupled with the absence of a definitive legislative framework or policies regarding antibiotic use, as well as the size and socioeconomic status of the population, particularly among farmers, depicts the finding of the resistance genes. Resistance genes originating from agricultural environments are transferred to human diseases through lateral gene transfer.
In many African nations, antibiotics are readily available, like other over-the-counter drugs (OTC), coupled with self-prescription, resulting in overuse and abuse. Moreover, poverty compels most individuals in low-come settings to purchase cheap antibiotics to treat their ailments rather than incurring expenses from hospitals for proper diagnosis prior to antibiotic treatment. This scoping review indicates that only a limited number of antibiotics remain effective against various infections caused by pathogens. A multitude of reasons may elucidate these findings. Improper regulations for antibiotics, lack of antimicrobial awareness among the population, poor public health facilities in limited resources settings, inadequate and efficient health professionals, poor diagnostics and technological advances, self-health management, etc. The high cost of restricted antibiotics, which are typically regarded as a last resort, is used for critical cases of bacterial infections. Implementing measures to monitor the residual antibiotics that remain effective against these bacterial infections is imperative. As discussed above, the significant factors influencing AMR in Africa include the socioeconomic fallout, such as poverty, inadequate healthcare infrastructure, lack of education and awareness, availability of OTC antibiotics, urbanization, and population.
Our scoping analysis indicated that antibiotic-resistant pathogenic bacteria in RTE food research have not gained comprehensive financing and support from government entities, non-profit organizations, agencies, institutions, universities, commercial corporations, and pharmaceutical businesses. This has also impacted the progress in research and antibiotic epidemiological surveillance in poorly reported African nations. However, interrelationships among donors, funders, policymakers, and support teams are essential for progress, refining strategies, implementing initiatives, promoting stakeholder consultations, as advised by the African community, and ensuring accountability. Furthermore, it is essential to implement a proactive engagement strategy for stakeholders to mitigate the potential threat of bacterial resistance to antibiotics. The lessons from this contribution of this scoping review to the delinquency of multidrug resistance imply that the presence of antibiotic-resistant pathogenic bacteria in RTE foods considerably worsens the problem of multidrug resistance (MDR). The existence of these bacteria not only presents a direct health hazard but also enables the transmission of resistance genes to human infections, thereby complicating therapeutic alternatives. For instance, 43.5% of coagulase-negative staphylococci (CoNS) strains remained identified as multidrug-resistant (Chajęcka-Wierzchowska et al., 2023), and more than 95% of E. coli bacteria from RTE foods exhibited multidrug resistance, demonstrating elevated resistance levels to prevalent medicines such as tetracycline and ampicillin (Zhang et al., 2021; Onohuean and Igere, 2022). Of significant importance, the mobile genetic elements (MGEs) essential for the horizontal transfer of antibiotic resistance genes have been identified in Enterococcus faecium UC7251, which is isolated from RTE food and contains plasmids that enable the transfer of resistance genes (Belloso Daza et al., 2022). The existence of plasmid-mediated colistin resistance in E. coli underscores the capacity of these genes to disseminate swiftly from bacterial to human populations (Zhang et al., 2021).
AMR is associated with increased morbidity and mortality, with forecasts indicating up to 10 million fatalities per year by 2050 if current trends persist (Tang et al., 2023). Globally, AMR has become a critical global health issue, with increasing resistance to commonly used antibiotics threatening to undermine advances in medicine. Global trends in AMR reflect a rise in infections caused by resistant bacteria, driven by factors such as the overuse and misuse of antibiotics in healthcare and agriculture, poor sanitation, and inadequate infection control measures (WHO, 2023). Studies have shown that resistance is not confined to any region but is a global phenomenon affecting both high-income countries (e.g., the US and Europe) and low- and middle-income countries (LMICs) (Samtiya et al., 2022; Salam et al., 2023). In LMICs, the situation is often exacerbated by limited access to effective antibiotics, weak regulatory frameworks, and insufficient public health infrastructure (Salam et al., 2023). The RTE food industry has emerged as a significant pathway for the transmission of resistant pathogens, with studies suggesting that foodborne bacteria, particularly from animal products, can contribute to the spread of AMR (Conceição et al., 2023; Grudlewska-Buda et al., 2023; Ronald et al., 2023). Global surveillance data from WHO and the Centers for Disease Control and Prevention (CDC) indicate widespread resistance, especially to common pathogens like E. coli, Salmonella, and Staphylococcus aureus (WHO, 2020; Murray et al., 2022). This is compounded by the slow development of new antibiotics, creating an urgent need for more substantial prevention, monitoring, and treatment strategies across the food, healthcare, and environmental sectors. In addition, the financial ramifications of AMR are significant, with potential losses reaching trillions of dollars attributable to healthcare expenditures and diminished productivity (Ahmed et al., 2024), with a more significant consequence in low-income nations in their already poor and over-stressed medical health systems.
Going forward, a single health approach that involving an integrated interdisciplinary approach encompassing human, animal, and environmental health is crucial for efficient antimicrobial resistance management. Lessons learned from the four successful nations of Denmark, the United Kingdom (UK), the Netherlands, and Sweden on AMR mitigation strategies could strengthen and impact positive African outcomes. For instance, Denmark and Sweden’s strategy for AMR emphasized stringent rules governing antibiotic use in humans and animals (Levy, 2014; Wierup et al., 2021; Regeringskansliet, 2024; Wu, 2024). Denmark implemented policies to limit the use of antibiotics for growth promotion in animals, combined with surveillance programs to monitor antibiotic consumption and resistance patterns. The UK has an AMR policy that covers healthcare, agriculture, and the environment. The “UK Five-Year Action Plan for AMR” promotes antibiotic alternatives in agriculture, stewardship, and public awareness. This method has greatly reduced the number of human antibiotic prescriptions and associated resistance (Blake et al., 2022). Whereas, the Netherlands leverage on “One Health” approach to AMR by integrating human, animal, and environmental health sectors. By reducing antibiotic use in both human medicine and livestock, and by promoting alternative therapies, the country has reduced AMR, particularly in farm animals (Ooms et al., 2023). Therefore, stockholders in the African region must develop and implement short-, medium-, and long-term strategies that encompass one health approach. Furthermore, improving public awareness and education about AMR determinants and advocating for regulations on ethical antibiotic use are essential for addressing this problem. Other actionable recommendations for policymakers include establishing or improving national and global AMR surveillance networks to monitor AMR trends in humans, animals, and food products. Moreover, given the global nature of AMR, international cooperation is essential. Policymakers should collaborate through frameworks like the WHO’s Global Action Plan on AMR to ensure consistent policies and shared resources. Policymakers should incentivize public-private partnerships to accelerate innovation in combating resistant pathogens. Increased funding for developing vaccines, new antibiotics, alternative treatments (e.g., bacteriophages), and diagnostic tools is crucial.
Limitations of the study
The keywords for data collection may have constrained the research focus and omitted grey literatures that does not index the databases used, which may provide unique insights or contain emerging trends, novel findings, and data that could influence the interpretation of antibiotic resistance patterns. Again, grey literature often includes unpublished studies, government reports, conference proceedings, theses, and other non-peer-reviewed sources, which may contain valuable data not indexed in traditional databases. However, we are confident that the evidence synthesized from Scopus, WoS, PubMed, and Handpicked based on a literature reference search could provide an insightful picture of the research landscape on antimicrobial-resistance pathogens and resistance genes in RTE foods in Africa.
Also, the NOS rating system is subjective, leading to different reviewers having varied interpretations of the criteria. It fails to account for all aspects of bias, such as publication bias or bias due to selective reporting. However, the NOS is a simple, transparent, and reproducible method for assessing study quality and allows for flexibility across different study designs (case–control and cohort studies).
Conclusion
In this scoping review, some African nations were poorly represented in the antibiotic-resistant pathogenic bacteria survey following the article’s inclusion criteria and used databases. However, it increased the pathogens primarily responsible for foodborne infections, namely E. coli, Salmonella, and Staphylococcus. Educating food vendors and operators about proper hygiene practices can be a practical and cost-effective solution. The scoping analysis revealed that various forms of antibiotic resistance have been documented in food commodities across multiple African countries, with significant levels of resistance observed in infectious pathogens in diverse RTE foods. The regional antibiotic resistance surveillance system is a significant concern. Therefore, the use of antibiotics in agriculture, the food industry, and human health requires stringent regulations in Africa, particularly in resource-limited settings. This highlights the urgent need for effective control strategies to reduce the spread of resistant bacteria in RTE foods.
Data availability statement
The datasets presented in this study can be found in online repositories. The names of the repository/repositories and accession number(s) can be found in the article/Supplementary material.
Author contributions
HoO: Conceptualization, Data curation, Formal analysis, Funding acquisition, Investigation, Methodology, Project administration, Resources, Software, Supervision, Validation, Visualization, Writing – original draft, Writing – review & editing. HaO: Conceptualization, Data curation, Formal analysis, Funding acquisition, Investigation, Methodology, Project administration, Resources, Software, Supervision, Validation, Visualization, Writing – review & editing. FO: Data curation, Formal analysis, Investigation, Methodology, Project administration, Resources, Software, Supervision, Validation, Visualization, Writing – original draft, Writing – review & editing. SB: Data curation, Investigation, Methodology, Project administration, Resources, Software, Validation, Visualization, Writing – review & editing. OA: Data curation, Investigation, Methodology, Project administration, Resources, Software, Validation, Visualization, Writing – review & editing. AK: Data curation, Formal analysis, Investigation, Methodology, Project administration, Software, Validation, Visualization, Writing – review & editing.
Funding
The author(s) declare that no financial support was received for the research and/or publication of this article.
Conflict of interest
The authors declare that the research was conducted in the absence of any commercial or financial relationships that could be construed as a potential conflict of interest.
Generative AI statement
The authors declare that no Gen AI was used in the creation of this manuscript.
Publisher’s note
All claims expressed in this article are solely those of the authors and do not necessarily represent those of their affiliated organizations, or those of the publisher, the editors and the reviewers. Any product that may be evaluated in this article, or claim that may be made by its manufacturer, is not guaranteed or endorsed by the publisher.
Supplementary material
The Supplementary material for this article can be found online at: https://www.frontiersin.org/articles/10.3389/fmicb.2025.1525564/full#supplementary-material
References
Adefisoye, M. A., and Okoh, A. I. (2016). Identification and antimicrobial resistance prevalence of pathogenic Escherichia coli strains from treated wastewater effluents in Eastern Cape, South Africa. Microbiology 5, 143–151. doi: 10.1002/mbo3.319
Ahmed, S. K., Hussein, S., Qurbani, K., Ibrahim, R. H., Fareeq, A., Mahmood, K. A., et al. (2024). Antimicrobial resistance: Impacts, challenges, and future prospects. J. Med. Surg. Public Heal. 2:100081. doi: 10.1016/j.glmedi.2024.100081
Akinnibosun, F. I., and Ojo, K. N. (2015). Comparative study of ready-to-eat foods from road-side and eateries in Benin City, Nigeria. African J. Microbiol. Res 9, 929–933. doi: 10.5897/ajmr2015.7418
Akinyele, H. A., Oluwaseun, A. A., Tunde, A. K., and Oluwaseun, A. C. (2024). Detection and antibiogram of bacterial contaminants in some commonly consumed indigenous street vended foods in Kasua Central Market in Kaduna North, Kaduna State, Nigeria. Dutse J. Pure Appl. Sci. 10, 100–110. doi: 10.4314/dujopas.v10i1a.11
Akinyem, K. O., Fashola, M. O., Habib, N., and Akinwande, E. (2013). Vended foods in Lagos, Nigeria: A potential reservoir for the spread of emerging strains of drug resistant bacteria. Health (Irvine. Calif). 5, 675–680. doi: 10.4236/health.2013.54089
Alelign, D., Yihune, M., Bekele, M., Oumer, Y., Beyene, K., and Atnafu, K. (2023). Bacteriological quality and antimicrobial resistant patterns of foodborne pathogens isolated from commonly vended street foods in Arba Minch Town, Southern Ethiopia. Infect. Drug Resist 16, 2883–2899. doi: 10.2147/IDR.S411162
Aminu, R. F., and Umeh, E. U. (2014). Antibiotic susceptibility of enteric bacteria isolated from ready-to-eat meat balangu in Kogi State, Nigeria. African J. Microbiol. Res 8, 3536–3539. doi: 10.5897/ajmr2013.5884
Ananias, B., and Roland, K. (2017). Bacterial contamination of ready-to-eat meats vended in highway markets in Uganda. African J. Food Sci. 11, 160–170. doi: 10.5897/ajfs2016.1550
Asiegbu, C. V., Lebelo, S. L., and Tabit, F. T. (2020). Microbial quality of ready-to-eat street vended food groups sold in the Johannesburg metropolis, South Africa. J. Food Qual. Hazards Control. 7, 18–26. doi: 10.18502/JFQHC.7.1.2448
Aworh, M. K., Kwaga, J. K. P., Hendriksen, R. S., Okolocha, E. C., and Thakur, S. (2021). Genetic relatedness of multidrug resistant Escherichia coli isolated from humans, chickens and poultry environments. Antimicrob. Resist. Infect. Control 10:58. doi: 10.1186/s13756-021-00930-x
Belloso Daza, M. V., Milani, G., Cortimiglia, C., Pietta, E., Bassi, D., and Cocconcelli, P. S. (2022). Genomic insights of Enterococcus faecium UC7251, a multi-drug resistant strain from ready-to-eat food, highlight the risk of antimicrobial resistance in the food chain. Front. Microbiol. 13:894241. doi: 10.3389/fmicb.2022.894241
Beshiru, A., and Igbinosa, E. O. (2023). Surveillance of Vibrio parahaemolyticus pathogens recovered from ready-to-eat foods. Sci. Rep. 13:4186. doi: 10.1038/s41598-023-31359-4
Beshiru, A., Igbinosa, I. H., and Igbinosa, E. O. (2019). Prevalence of antimicrobial resistance and virulence gene elements of Salmonella serovars from ready-to-eat (RTE) shrimps. Front. Microbiol. 10:1613. doi: 10.3389/fmicb.2019.01613
Beshiru, A., Okareh, O. T., Okoh, A. I., and Igbinosa, E. O. (2020). Detection of antibiotic resistance and virulence genes of Vibrio strains isolated from ready-to-eat shrimps in Delta and Edo States, Nigeria. J. Appl. Microbiol. 129, 17–36. doi: 10.1111/jam.14590
Blake, L. J., Häsler, B., Bennani, H., Mateus, A. L. P., Eastmure, E., Mays, N., et al. (2022). The UK Antimicrobial Resistance Strategy 2013–18: A qualitative study of international and domestic policy and action related to livestock and the food chain. Front. Sustain. Food Syst. 6:819158. doi: 10.3389/fsufs.2022.819158
Blessed, K. Y. (2018). Quinolones resistant enteric bacteria isolated from hawked kunun-zaki in selected parts of chikun local government area, kaduna state, Nigeria. MOJ Toxicol. 4, 26–28. doi: 10.15406/mojt.2018.04.00085
Cassini, A., Högberg, L. D., Plachouras, D., Quattrocchi, A., Hoxha, A., Simonsen, G. S., et al. (2019). Attributable deaths and disability-adjusted life-years caused by infections with antibiotic-resistant bacteria in the EU and the European Economic Area in 2015: a population-level modelling analysis. Lancet Infect. Dis. 19, 56–66. doi: 10.1016/S1473-3099(18)30605-4
Chajęcka-Wierzchowska, W., Gajewska, J., Zadernowska, A., Randazzo, C. L., and Caggia, C. (2023). A comprehensive study on antibiotic resistance among coagulase-negative staphylococci (CoNS) strains isolated from ready-to-eat food served in bars and restaurants. Food Secur. 12:514. doi: 10.3390/foods12030514
Choy, S. K., Neumann, E. M., Romero-Barrios, P., and Tamber, S. (2024). Contribution of food to the human health burden of antimicrobial resistance. Foodborne Pathog. Dis. 21, 71–82. doi: 10.1089/fpd.2023.0099
Claudious, G., Jerikias, M., Tinashe, H., Gilbert, J., Delay, C., Katsande Peter, M. S., et al. (2020). Isolation and antibiotic susceptibility profiling of enteric bacteria from homemade and industrial made meat pies sold in harare CBD, Zimbabwe. Sch. Acad. J. Biosci. 8, 1–6. doi: 10.36347/sajb.2020.v08i01.001
Conceição, S., Queiroga, M. C., and Laranjo, M. (2023). Antimicrobial resistance in bacteria from meat and meat products: a one health perspective. Microorganisms. 11:2581. doi: 10.3390/microorganisms11102581
Ebakota, D. O., Abiodun, O. A., and Nosa, O. O. (2018). Prevalence of antibiotics resistant Listeria monocytogenes strains in Nigerian ready-to-eat foods. Food Saf. 6, 118–125. doi: 10.14252/foodsafetyfscj.2018002
Esemu, S. N., Njoh, S. T., Ndip, L. M., Keneh, N. K., Kfusi, J. A., and Njukeng, A. P. (2023). Ready-to-eat foods: a potential vehicle for the spread of coagulase-positive staphylococci and antimicrobial-resistant Staphylococcus aureus in Buea Municipality, South West Cameroon. Can. J. Infect. Dis. Med. Microbiol. 2023:9735319. doi: 10.1155/2023/9735319
Fayemi, O. E., Akanni, G. B., Elegbeleye, J. A., Aboaba, O. O., and Njage, P. M. (2021). Prevalence, characterization and antibiotic resistance of Shiga toxigenic Escherichia coli serogroups isolated from fresh beef and locally processed ready-to-eat meat products in Lagos, Nigeria. Int. J. Food Microbiol. 347:109191. doi: 10.1016/j.ijfoodmicro.2021.109191
Grudlewska-Buda, K., Bauza-Kaszewska, J., Wiktorczyk-Kapischke, N., Budzyńska, A., Gospodarek-Komkowska, E., and Skowron, K. (2023). Antibiotic resistance in selected emerging bacterial foodborne pathogens—an issue of concern? Antibiotics. 12:880. doi: 10.3390/antibiotics12050880
Himanshu, R., Prudencio, C., da Costa, A. C., Leal, E., Chang, C. M., and Pandey, R. P. (2022). Systematic surveillance and meta-analysis of antimicrobial resistance and food sources from China and the USA. Antibiotics. 11:1471. doi: 10.3390/antibiotics11111471
Hrovat, K., Zupančič, J. Č., Seme, K., and Avguštin, J. A. (2023). QAC resistance genes in ESBL-producing E. coli isolated from patients with lower respiratory tract infections in the central Slovenia region—A 21-year survey. Trop. Med. Infect. Dis. 8:273. doi: 10.3390/tropicalmed8050273
Igbinosa, E. O., Beshiru, A., Akporehe, L. U., Oviasogie, F. E., and Igbinosa, O. O. (2016). Prevalence of methicillin-resistant Staphylococcus aureus and other Staphylococcus species in raw meat samples intended for human consumption in Benin City, Nigeria: Implications for public health. Int. J. Environ. Res. Public Health. 13:949. doi: 10.3390/ijerph13100949
Igbinosa, I. H., Beshiru, A., and Igbinosa, E. O. (2017). Antibiotic resistance profile of Pseudomonas aeruginosa isolated from aquaculture and abattoir environments in urban communities. Asian Pacific J. Trop. Dis 7, 47–52. doi: 10.12980/apjtd.7.2017D6-363
Igere, B. E., Hope, O., and Oyama, G. (2022a). Occurrence of new delhi metallo-beta-lactamase 1 producing enterococcus species in oghara water nexus: an emerging environmental implications of resistance dynamics. Microbiol. insights 15:117863612211337. doi: 10.1177/11786361221133731
Igere, B. E., Okoh, A. I., and Nwodo, U. U. (2020). Wastewater treatment plants and release: The vase of Odin for emerging bacterial contaminants, resistance and determinant of environmental wellness. Emerg. Contam 6, 212–224. doi: 10.1016/j.emcon.2020.05.003
Igere, B. E., Onohuean, H., Iwu, D. C., and Igbinosa, E. O. (2023). Polymyxin sensitivity/resistance cosmopolitan status, epidemiology and prevalence among O1/O139 and non-O1/non-O139 Vibrio cholerae: a meta-analysis. Inf. Med. 2, 283–293. doi: 10.1016/j.imj.2023.11.004
Igere, B. E., Onohuean, H., and Nwodo, U. U. (2022b). Modern knowledge-scape possess petite influence on the factual persistence of resistance determinants (ARGs/MGEs): a map and assessment of discharged wastewater and water bodies. Heliyon 8:e12253. doi: 10.1016/j.heliyon.2022.e12253
Igere, B. E., Onohuean, H., and Odjadjare, E. E. O. (2024). Nucleic acids accumulation in environmental waste release; a scientific map of particulate nucleic acid yet unattended and a potential outbreak helix-spring. Int. J. Environ. Pollut. 1. doi: 10.1504/ijep.2024.10062258
Isic, B. O., Uwhuba, K. E., and Igere, B. E. (2024). Prevalence, characterization, and implications of methicillin-resistant Staphylococcus aureus (MRSA) in ready-to-eat foods from Delta, Nigeria: a concern for consumer safety. Sustainable Microbiol. 1, 1–13. doi: 10.1093/sumbio/qvae007
Izevbuwa, O., and Okhuebor, S. (2021). Microbiological assessment of ready to eat food from selected street vending food locations in Ikpoba-Okha local government area of Edo state. Bact. Emp. 4, 20–24. doi: 10.36547/be.2021.4.1.20-24
Karikari, A. B., Kpordze, S. W., Yamik, D. Y., and Saba, C. K. S. (2022). Ready-to-eat food as sources of extended-spectrum β-lactamase-producing salmonella and E. coli in Tamale, Ghana. Front. Trop. Dis. 3:834048. doi: 10.3389/fitd.2022.834048
Kättström, D., Beronius, A., Rudén, C., and Ågerstrand, M. (2022). Stricter regulation applies to antimicrobial substances when used as biocides compared to cosmetics under current EU legislation. Emerg. Contam 8, 229–242. doi: 10.1016/j.emcon.2022.04.003
Kimera, Z. I., Mshana, S. E., Rweyemamu, M. M., Mboera, L. E. G., and Matee, M. I. N. (2020). Antimicrobial use and resistance in food-producing animals and the environment: An African perspective. Antimicrob. Resist. Infect. Control 9:37. doi: 10.1186/s13756-020-0697-x
Levy, S. (2014). Reduced antibiotic use in livestock: How Denmark tackled resistance. Environ. Health Perspect. 122, A160–A165. doi: 10.1289/ehp.122-A160
Lund, B. M. (2019). Provision of microbiologically safe food for vulnerable people in hospitals, care homes and in the community. Food Control 96, 535–547. doi: 10.1016/j.foodcont.2018.09.032
Makinde, O. M., Adetunji, M. C., Ezeokoli, O. T., Odumosu, B. T., Ngoma, L., Mwanza, M., et al. (2021). Bacterial contaminants and their antibiotic susceptibility patterns in ready-to-eat foods vended in Ogun state, Nigeria. Lett. Appl. Microbiol. 72, 187–195. doi: 10.1111/lam.13407
Makinde, O. M., Ayeni, K. I., Sulyok, M., Krska, R., Adeleke, R. A., and Ezekiel, C. N. (2020). Microbiological safety of ready-to-eat foods in low- and middle-income countries: A comprehensive 10-year (2009 to 2018) review. Compr. Rev. Food Sci. Food Saf. 19, 703–732. doi: 10.1111/1541-4337.12533
Mamfe, L. M., Akwuobu, C. A., and Ngbede, E. O. (2021). Phenotypic detection, antimicrobial susceptibility and virulence profile of staphylococci in the pig production setting, Makurdi, Nigeria. Access Microbiol. 3:000293. doi: 10.1099/acmi.0.000293
Mayoré, A. D., Bessimbaye, N., Bodering, A., Evariste, B., Abdelsalam, T., and Nicolas, B. (2021). Resistance profile of Salmonella isolated from food sold in the streets of NDjamena, Chad. African J. Microbiol. Res. 15, 334–340. doi: 10.5897/ajmr2021.9475
Mekhloufi, O. A., Chieffi, D., Hammoudi, A., Bensefia, S. A., Fanelli, F., and Fusco, V. (2021). Prevalence, enterotoxigenic potential and antimicrobial resistance of staphylococcus aureus and methicillin-resistant Staphylococcus aureus (MRSA) isolated from Algerian ready to eat foods. Toxins (Basel). 13:835. doi: 10.3390/toxins13120835
Mengistu, D. A., and Tolera, S. T. (2020). Prevalence of microorganisms of public health significance in ready-to-eat foods sold in developing countries: systematic review and meta-analysis. Int. J. Food Sci. 2020, 1–9. doi: 10.1155/2020/8867250
Moges, M., Rodland, E. K., Legesse, T., and Argaw, A. (2024). Antibiotic resistance patterns of Staphylococcus aureus and Enterobacteriaceae isolated from street foods in selected towns of Ethiopia. BMC Infect. Dis. 24:367. doi: 10.1186/s12879-024-09266-4
Morshdy, A. E. M. A., Hussein, M. A., Tharwat, A. E., and Fakhry, B. A. (2018). Prevalence of enterotoxigenic and multi-drug-resistant Staphylococcus aureus in ready to eat meat sandwiches. Slov. Vet. Res. 55:357. doi: 10.26873/SVR-664-2018
Murray, C. J., Ikuta, K. S., Sharara, F., Swetschinski, L., Robles Aguilar, G., Gray, A., et al. (2022). Global burden of bacterial antimicrobial resistance in 2019: a systematic analysis. Lancet 399, 629–655. doi: 10.1016/S0140-6736(21)02724-0
Muteeb, G., Rehman, M. T., Shahwan, M., and Aatif, M. (2023). Origin of antibiotics and antibiotic resistance, and their impacts on drug development: a narrative review. Pharmaceuticals. 16:1615. doi: 10.3390/ph16111615
Mwove, J., Imathiu, S., Orina, I., and Karanja, P. (2022). Microbial quality and safety of ready-to-eat street-vended foods sold in selected locations in Kenya. J. Food Diet. Res. 1, 34–40. doi: 10.48165/jfdr.2021.1202
Ndunguru, J. N., and Ndossi, D. G. (2020). Bacteriological analysis of ready-to-eat foods from Morogoro Municipal Market. Tanzania Vet. J. 37, 17–24. doi: 10.4314/tvj.v37i1.4s
Nikiema, M. E. M., De La Gandara, M. P., Compaore, K. A. M., Ba, A. K., Soro, K. D., Nikiema, P. A., et al. (2021). Contamination of street food with multidrugresistant Salmonella, in Ouagadougou, Burkina Faso. PLoS One 16:e0253312. doi: 10.1371/journal.pone.0253312
Nyenje, M. E., Odjadjare, C. E., Tanih, N. F., Green, E., and Ndip, R. N. (2012). Foodborne pathogens recovered from ready-to-eat foods from roadside cafeterias and retail outlets in alice, eastern cape province, South Africa: Public health implications. Int. J. Environ. Res. Public Health 9, 2608–2619. doi: 10.3390/ijerph9082608
Odu, N. N., and Akano, U. M. (2012). The microbiological assessment of ready-to-eat-food (Shawarma) in Port Harcourt City, Nigeria. Nat. Sci.
Ohunayo, A. S., Molehin, O. R., Afolabi, A. T., Dauda, O. S., Ogundolie, F. A., and Alabi, O. S. (2024). Prevalence of multidrug-resistant and Entero-invasive E. coli isolates in ready-to-eat roasted beef sold on the streets of Ibadan metropolis. in 2024 International Conference on Science, Engineering and Business for Driving Sustainable Development Goals (SEB4SDG) (IEEE), 1–6. doi: 10.1109/SEB4SDG60871.2024.10629972
Okafor-Elenwo, E. J., and Imade, O. S. (2020a). Quantification of the probability of exposure of humans to pathogenic microbes present in some ready-to-eat foods served in Nigerian restaurants. Niger. J. Pure Appl. Sci 33, 3720–3727. doi: 10.48198/njpas/20.a06
Okafor-Elenwo, E. J., and Imade, O. S. (2020b). Ready-to-eat vegetable salads served in Nigerian restaurants: a potential source of multidrug-resistant bacteria. J. Appl. Microbiol. 129, 1402–1409. doi: 10.1111/jam.14693
Okoli, C. E., Njoga, E. O., Enem, S. I., Godwin, E. E., Nwanta, J. A., and Chah, K. F. (2018). Prevalence, toxigenic potential and antimicrobial susceptibility profile of Staphylococcus isolated from ready-to-eat meats. Vet. World 11, 1214–1221. doi: 10.14202/vetworld.2018.1214-1221
Okubo, T., Yossapol, M., Ikushima, S., Kakooza, S., Wampande, E. M., Asai, T., et al. (2020). Isolation and characterization of antimicrobial-resistant Escherichia coli from retail meats from roadside butcheries in Uganda. Foodborne Pathog. Dis. 17, 666–671. doi: 10.1089/fpd.2020.2796
Oladipo, I. C., and Adejumobi, O. D. (2010). Incidence of antibiotic resistance in some bacterial pathogens from street vended food in Ogbomoso, Nigeria. Pakistan J. Nutr 9, 1061–1068. doi: 10.3923/pjn.2010.1061.1068
Onohuean, H., Agwu, E., and Nwodo, U. U. (2022a). A global perspective of vibrio species and associated diseases: three-decade meta-synthesis of research advancement. Environ. Health Insights. 16:11786302221099406. doi: 10.1177/11786302221099406
Onohuean, H., Aigbogun, E. O., and Igere, B. E. (2022b). Meta-synthesis and science mapping analysis of HIV/HPV co-infection: a global perspective with emphasis on Africa. Glob. Health 18, 36–20. doi: 10.1186/s12992-022-00812-w
Onohuean, H., Akiyode, A. O., Akiyode, O., Igbinoba, S. I., and Alagbonsi, A. I. (2022c). Epidemiology of neurodegenerative diseases in the East African region: a meta-analysis. Front. Neurol. 13:1024004. doi: 10.3389/fneur.2022.1024004
Onohuean, H., and Igere, B. E. (2022). Occurrence, antibiotic susceptibility and genes encoding antibacterial resistance of Salmonella spp. and Escherichia coli from milk and meat sold in markets of Bushenyi District, Uganda. Microbiol. Insights 15:117863612210889. doi: 10.1177/11786361221088992
Onohuean, H., and Igere, B. E. (2023). Ethnopharmacology and phytochemistry of medicinal plants against enterocyte bacteria-linked infections and diarrhoea in some African countries: a systematic review. RPS Pharm. Pharmacol. Reports. 2:rqad04. doi: 10.1093/rpsppr/rqad041
Onohuean, H., and Nwodo, U. U. (2023a). Demographic dynamics of waterborne disease and perceived associated WASH factors in Bushenyi and Sheema districts of South-Western Uganda. Environ. Monit. Assess. 195:864. doi: 10.1007/s10661-023-11270-1
Onohuean, H., and Nwodo, U. U. (2023b). Polymorphism and mutational diversity of virulence (vcgCPI/vcgCPE) and resistance determinants (aac(3)-IIa, aacC2, strA, Sul 1, and 11) among human pathogenic Vibrio species recovered from surface waters in South-Western districts of Uganda. J. Genet. Eng. Biotechnol. 21:94. doi: 10.1186/s43141-023-00554-1
Onohuean, H., Okoh, A. I., and Nwodo, U. U. (2021). Antibiogram signatures of Vibrio species recovered from surface waters in South Western districts of Uganda: Implications for environmental pollution and infection control. Sci. Total Environ. 807:150706. doi: 10.1016/j.scitotenv.2021.150706
Ouoba, L. I. I., Vouidibio Mbozo, A. B., Anyogu, A., Obioha, P. I., Lingani-Sawadogo, H., Sutherland, J. P., et al. (2019). Environmental heterogeneity of Staphylococcus species from alkaline fermented foods and associated toxins and antimicrobial resistance genetic elements. Int. J. Food Microbiol. 311:108356. doi: 10.1016/j.ijfoodmicro.2019.108356
Owoseni, A., and Onilude, A. (2016). Incidence and plasmid profiles of multidrug resistant enterobacteria isolates from processed convenience foods in some countries of West Africa. Food Public Health 6, 44–51. doi: 10.5923/j.fph.20160602.03
Paudyal, N., Anihouvi, V., Hounhouigan, J., Matsheka, M. I., Sekwati-Monang, B., Amoa-Awua, W., et al. (2017). Prevalence of foodborne pathogens in food from selected African countries – a meta-analysis. Int. J. Food Microbiol. 249, 35–43. doi: 10.1016/j.ijfoodmicro.2017.03.002
Regassa, B. T., Tosisa, W., Eshetu, D., Beyene, D., Abdeta, A., Assefa, A., et al. (2023). Antimicrobial resistance profiles of bacterial isolates from clinical specimens referred to Ethiopian Public Health Institute: analysis of 5-year data. BMC Infect. Dis. 23:798. doi: 10.1186/s12879-023-08803-x
Regeringskansliet, R. O. (2024). Sweden’s efforts to combat antimicrobial resistance – so that we can continue to treat infections in the future. Available online at: https://www.government.se/government-policy/swedens-work-against-antimicrobial-resistance/swedens-efforts-to-combat-antimicrobial-resistance--so-that-we-can-continue-to-treat-infections-in-the-future/ (Accessed December 30, 2024).
Ronald, C., Matofari, J. W., and Nduko, J. M. (2023). Antimicrobial resistance of E. coli strains in ready-to-eat red meat products in Nakuru County, Kenya. The Microbe. 1:100022. doi: 10.1016/j.microb.2023.100022
Sagoo, S. K., Little, C. L., and Mitchell, R. T. (2003). Microbiological quality of open ready-to-eat salad vegetables: Effectiveness of food hygiene training of management. J. Food Prot. 66, 1581–1586. doi: 10.4315/0362-028X-66.9.1581
Salam, M. A., Al-Amin, M. Y., Salam, M. T., Pawar, J. S., Akhter, N., Rabaan, A. A., et al. (2023). Antimicrobial resistance: a growing serious threat for Global Public Health. Healthcare 11:1946. doi: 10.3390/healthcare11131946
Samtiya, M., Matthews, K. R., Dhewa, T., and Puniya, A. K. (2022). Antimicrobial resistance in the food chain: trends, mechanisms, pathways, and possible regulation strategies. Food Secur. 11:2966. doi: 10.3390/foods11192966
Satán, C., Satyanarayana, S., Shringarpure, K., Mendoza-Ticona, A., Palanivel, C., Jaramillo, K., et al. (2023). Epidemiology of antimicrobial resistance in bacteria isolated from inpatient and outpatient samples, Ecuador, 2018. Rev. Panam. Salud Publica/Pan Am. J. Public Heal 47:1. doi: 10.26633/RPSP.2023.14
Setsoafia Saba, C. K., Karikar, A. B., Yeleliere, E., Takyi, P., and Kpordze, S. W. (2021). Multidrug resistant Salmonella spp. and Escherichia coli from a popular Ready-to-Eat local food (Fufu) from commercial food vendors in Ghana. J. Food Saf. Hyg. 5, 214–219. doi: 10.18502/jfsh.v5i4.5702
Shange, N., Gouws, P., and Hoffman, L. C. (2019). Campylobacter and Arcobacter species in food-producing animals: prevalence at primary production and during slaughter. World J. Microbiol. Biotechnol. 35:146. doi: 10.1007/s11274-019-2722-x
Sharma, P., Gupta, S. K., Adenipekun, E. O., Barrett, J. B., Hiott, L. M., Woodley, T. A., et al. (2020). Genome analysis of multidrug-resistant Escherichia coli isolated from poultry in Nigeria. Foodborne Pathog. Dis. 17, 1–7. doi: 10.1089/fpd.2019.2659
Somda, N. S., Bonkoungou, I. J. O., Sambe-Ba, B., Drabo, M. S., Wane, A. A., Sawadogo-Lingani, H., et al. (2021). Diversity and antimicrobial drug resistance of non-typhoid Salmonella serotypes isolated in lettuce, irrigation water and clinical samples in Burkina Faso. J. Agric. Food Res. 5:100167. doi: 10.1016/j.jafr.2021.100167
Somda, N. S., Tankoano, A., Métuor-Dabiré, A., Kaboré, D., Bonkoungou, J. O. I., Kpoda, D. S., et al. (2023). A systematic review and meta-analysis of antibiotic resistance of foodborne pathogenic bacteria in West Africa between 2010 and 2020. J. Food Prot. 86:100061. doi: 10.1016/j.jfp.2023.100061
Soubeiga, A. P., Kpoda, D. S., Compaoré, M. K. A., Somda-Belemlougri, A., Kaseko, N., Rouamba, S. S., et al. (2022). Molecular characterization and the antimicrobial resistance profile of salmonella spp. isolated from ready-to-eat foods in Ouagadougou, Burkina Faso. Int. J. Microbiol. 2022, 1–10. doi: 10.1155/2022/9640828
Tang, K. W. K., Millar, B. C., and Moore, J. E. (2023). Antimicrobial resistance (AMR). Br. J. Biomed. Sci. 80:11387. doi: 10.3389/bjbs.2023.11387
Tanko, N., Bolaji, R. O., Olayinka, A. T., and Olayinka, B. O. (2020). A systematic review on the prevalence of extended-spectrum beta lactamase-producing gram-negative bacteria in Nigeria. J. Glob. Antimicrob. Resist. 22, 488–496. doi: 10.1016/j.jgar.2020.04.010
Taylor, D. D., Fenske, G. J., Pouzou, J. G., Costard, S., and Zagmutt, F. J. (2022). Codex alimentarius guidelines for risk analysis of foodborne antimicrobial resistance are incompatible with available surveillance data. J. Food Prot. 85, 1496–1505. doi: 10.4315/JFP-22-038
Tesfaye, W., Melese, A., Henok, S., and Yohannis, M. (2016). Prevalence and antimicrobial susceptibility profile of Salmonella species from ready-to-eat foods from catering establishments in Jigjiga City, Ethiopia. African J. Microbiol. Res 10, 1555–1560. doi: 10.5897/ajmr2016.7944
Toe, E., Dadié, A., Dako, E., and Loukou, G. (2017). Bacteriological quality and risk factors for contamination of raw mixed vegetable salads served in collective catering in Abidjan (Ivory Coast). Adv. Microbiol. 7, 405–419. doi: 10.4236/aim.2017.76033
Tricco, A. C., Lillie, E., Zarin, W., O’Brien, K. K., Colquhoun, H., Levac, D., et al. (2018). PRISMA extension for scoping reviews (PRISMA-ScR): checklist and explanation. Ann. Intern. Med. 169, 467–473. doi: 10.7326/M18-0850
Tshipamba, M. E., Lubanza, N., Adetunji, M. C., and Mwanza, M. (2018). Molecular characterization and antibiotic resistance of foodborne pathogens in street-vended ready-to-eat meat sold in South Africa. J. Food Prot. 81, 1963–1972. doi: 10.4315/0362-028X.JFP-18-069
Umar, A. T., Yarma, A. A., Bappah, R. H., Mofio, B. M., Bashir, M., and Rabiu, U. R. A. (2024). Risk factors and occurrence of Salmonella enterica in ready-to-eat vegetable salad sold in gombe metropolis, Gombe State, Nigeria. UMYU J. Microbiol. Res. 9, 279–286. doi: 10.47430/ujmr.2491.030
Valiati, B. S., Domingos, M. M., Lepaus, B. M., Faria-Silva, L., and de São José, J. F. B. (2023). “Emerging green technologies for decontamination of fresh produce” in Green Products in Food Safety. ed. B. Prakash, 267–291.
Walia, K., Sharma, M., Vijay, S., and Shome, B. R. (2019). Understanding policy dilemmas around antibiotic use in food animals & offering potential solutions. Indian J. Med. Res. 149, 107–118. doi: 10.4103/ijmr.IJMR_2_18
WHO (2023). Antimicrobial resistance. Available online at: https://www.who.int/news-room/fact-sheets/detail/antimicrobial-resistance (Accessed December 26, 2024).
Wierup, M., Wahlström, H., and Bengtsson, B. (2021). Successful prevention of antimicrobial resistance in animals—a retrospective country case study of sweden. Antibiotics. 10:129. doi: 10.3390/antibiotics10020129
Wu, Z. (2024). Approach choices for antimicrobial use reduction in European food animal production. EuroChoices 23, 36–42. doi: 10.1111/1746-692X.12424
Xiong, L., Sun, Y., Shi, L., and Yan, H. (2019). Characterization of antimicrobial resistance genes and class 1 integrase gene in raw meat and aquatic product, fresh vegetable and fruit, and swine manure in southern China. Food Control 104, 240–246. doi: 10.1016/j.foodcont.2019.05.004
Yaici, L., Haenni, M., Métayer, V., Saras, E., Mesbah Zekar, F., Ayad, M., et al. (2017). Spread of ESBL/AmpC-producing Escherichia coli and Klebsiella pneumoniae in the community through ready-to-eat sandwiches in Algeria. Int. J. Food Microbiol. 245, 66–72. doi: 10.1016/j.ijfoodmicro.2017.01.011
Zhang, S., Huang, Y., Yang, G., Lei, T., Chen, M., Ye, Q., et al. (2021). High prevalence of multidrug-resistant Escherichia coli and first detection of IncHI2/IncX4-plasmid carrying mcr-1 E. coli in retail ready-to-eat foods in China. Int. J. Food Microbiol. 355:109349. doi: 10.1016/j.ijfoodmicro.2021.109349
Keywords: prevalence, antibiotic-resistance, ready-to-eat (RTE), street-foods, Africa, public health
Citation: Onohuean H, Olot H, Onohuean FE, Bukke SPN, Akinsuyi OS and Kade A (2025) A scoping review of the prevalence of antimicrobial-resistant pathogens and signatures in ready-to-eat street foods in Africa: implications for public health. Front. Microbiol. 16:1525564. doi: 10.3389/fmicb.2025.1525564
Edited by:
Giovanna Suzzi, University of Teramo, ItalyReviewed by:
Abeni Beshiru, Federal Institute for Risk Assessment (BfR), GermanyAnthony Ayodeji Adegoke, University of Uyo, Nigeria
Alidehou Jerrold Agbankpe, Institut Pasteur de Guinée, Guinea
Copyright © 2025 Onohuean, Olot, Onohuean, Bukke, Akinsuyi and Kade. This is an open-access article distributed under the terms of the Creative Commons Attribution License (CC BY). The use, distribution or reproduction in other forums is permitted, provided the original author(s) and the copyright owner(s) are credited and that the original publication in this journal is cited, in accordance with accepted academic practice. No use, distribution or reproduction is permitted which does not comply with these terms.
*Correspondence: Hope Onohuean, b25vaHVlYW5AZ21haWwuY29t