- 1Jiangxi Provincial Key Laboratory of Environmental Pollution Prevention and Control in Mining and Metallurgy, Jiangxi University of Science and Technology, Ganzhou, China
- 2Institute of Eco-Environment and Plant Protection, Shanghai Academy of Agricultural Sciences, Shanghai, China
Watermelon (Citrullus lanatus L.), a globally significant economic crop generating billions of dollars annually, faces severe production limitations due to persistent Fusarium wilt caused by continuous cropping. The disease emerges following watermelon cultivation, driven by the invasion of Fusarium oxysporum f. sp. niveum, the accumulation of allelochemicals in the rhizosphere, changes in soil properties, and disruptions to the soil microbial community. These factors interact complexly, influencing plant health and soil conditions. This review examines the causes and impacts of watermelon Fusarium wilt. It explores various control strategies, including developing resistant cultivars, adjusting planting systems and agricultural practices, soil fumigation, microbial inoculants, targeted fertilization, and reductive soil disinfection. Additionally, Future wilt control may leverage nanomaterial delivery systems for precisely targeted, environmentally sustainable fungicide applications in watermelon production. This review aims to establish a scientific foundation for preventing and controlling watermelon Fusarium wilt.
1 Introduction
1.1 A brief introduction of watermelon
Watermelon (Citrullus lanatus L.) ranks among the world’s most consumed fresh fruits, with global production reaching approximately 100 million tons annually according to the Food and Agriculture Organization of the United Nations (FAO, 2023). It is an annual dicotyledonous herb and a monoecious plant belonging to the gourd family Cucurbitaceae. Watermelon originated in southern Africa and has undergone roughly 4,000 years of domestication and breeding (Guo et al., 2019). Watermelons have been selectively improved into modern cultivated varieties known for their sweetness (Paris, 2015). In addition to its high water content (~92%) and provides multiple bioavailable nutrients, including carbohydrates (mainly fructose), proteins, vitamins, and essential minerals (Seymen et al., 2021; Karin and Melanie, 2009). This unique nutritional composition, particularly its high water content and electrolyte balance, makes it an ideal rehydration source. Given the projected increase in global temperatures and heatwave frequency under climate change scenarios, watermelon’s dual role as both a hydrating food and nutrient source suggests its agricultural importance will grow substantially in coming decades.
1.2 The continuous cropping obstacles of watermelon
Continuous cropping obstacles (CCOs) refer to the decline in crop yield or quality when the same crop or its related species are repeatedly grown on the same land (Wang K. et al., 2024). CCOs may arise due to three main factors: (i) shifts in microbial communities (Ma et al., 2023); (ii) allelopathic effects (Chen et al., 2023); and (iii) changes in nutrient availability (Zhang et al., 2024). As the global population grows and arable land becomes increasingly limited, CCOs have become a significant challenge in intensive, large-scale agricultural and horticultural systems (Tan et al., 2021). Watermelon production is particularly vulnerable to CCOs. Under continuous monoculture, watermelon is highly susceptible to these obstacles, exhibiting symptoms such as high seedling mortality, stunted growth, reduced vigor, wilting, plant death, and declines in both yield and quality (Zhang et al., 2022b). As a result, CCOs pose a major threat to the sustainable production of watermelon.
1.3 Remediation of watermelon CCOs
Over the past decades, various strategies have been explored to sustain watermelon production and mitigate CCOs. Most watermelon CCOs are caused by soilborne fungi, including Fusarium oxysporum f. sp. Niveum (FON), Sclerotium rolfsii, and Macrophomina phaseolina, which contribute to wilt disease in watermelon. The decade-long soil persistence of FON, mediated predominantly by its chlamydospores, represents a major obstacle for watermelon Fusarium wilt control (Zhang et al., 2015; McKeen and Wensley, 1961). The pathogen spreads through spores, which germinate and develop into hyphae. These hyphae penetrate vascular tissues through wounds, producing microconidia that facilitate reproduction. As the plant dies and decomposes, FON is released back into the soil, perpetuating the disease cycle (Rahman et al., 2021; Zhang et al., 2015). Chlamydospores formed by FON under stress are key to its decade-long soil persistence, which drives the recurrence and spread of wilt disease (Akhter et al., 2016; Hudson et al., 2021). FON can also spread through soil transfer, plant transplantation, seed sowing, and contaminated runoff. Once an area becomes contaminated, the risk of disease recurrence remains high (Rahman et al., 2021). Additionally, the presence of wilt disease alters soil microbial communities. Following infection, fungal diversity in the soil decreases, allowing FON to dominate the microbial ecosystem and intensifying the severity of wilt and other soilborne diseases (Xu et al., 2020).
Multiple management strategies have been developed to control pathogen proliferation through distinct mechanistic pathways: grafting resistant rootstocks induces systemic resistance, optimized crop rotation disrupts pathogen life cycles, intercropping with antagonistic species modifies rhizosphere microbiomes, while precision fertilization protocols and targeted soil fumigation techniques collectively alter physicochemical parameters critical for fungal survival (Ding et al., 2021; Li et al., 2019; Ling et al., 2015; Liu et al., 2023a; Lv and Yan, 2024; Yang et al., 2016).
1.4 Research status of watermelon wilt disease
To assess the research status of watermelon wilt disease, we conducted a literature search using the Web of Science platform. The search methodology is detailed in Supplementary Text S1. Using “wilt disease” as the search topic, we identified 10,590 publications from 2005 to 2024, among which only 2.93% (310/10,590) specifically addressed watermelon wilt disease, highlighting a critical research gap in this economically significant pathosystem (Figure 1). A co-occurrence network analysis of the keywords from these 310 articles showed that “watermelon Fusarium wilt” was the most frequently occurring keyword, indicating that watermelon Fusarium wilt is a central research focus in studies on wilt diseases affecting watermelon (Figure 2A). Further analysis of keyword bursts revealed that FON has been a primary subject in recent studies on watermelon wilt disease (Figure 2B).
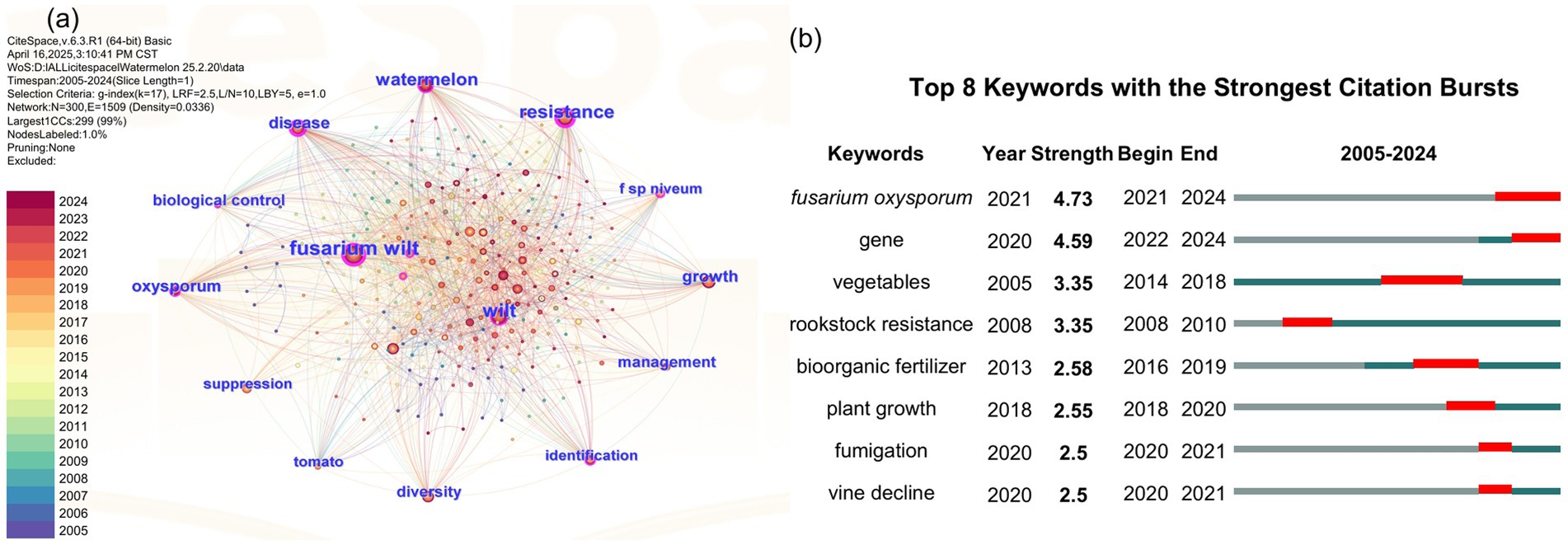
Figure 2. Co-occurrence network diagram (A) and the keyword bursts diagram (B) related to watermelon wilt disease.
Watermelon wilt caused by FON is the most severe soilborne disease affecting watermelon worldwide and can occur at all growth stages. However, the most critical period is from vine elongation to fruit setting, when the disease reaches its peak severity. The wilting of leaves (early stage) and vascular browning (late stage) result from the pathogen’s invasion process: root penetration, xylem colonization with toxin and enzyme production, and eventual vascular blockage causing permanent wilt (Zhang et al., 2005). Research on watermelon wilt disease continues to evolve. This review will focus on the tripartite determinants of Fusarium wilt pathogenesis: FON virulence, allelopathic interference, and abiotic stress potentiation. The review will also address the detrimental impacts of the disease, particularly plant damage coupled with yield and fruit quality reduction, and soil ecosystem disruption. Additionally, it will explore disease prevention and control strategies, including breeding resistant varieties, optimizing land management practices, and applying chemical or biological treatments.
2 Causes of watermelon wilt disease
The development of watermelon wilt disease is influenced by multiple factors resulting from interactions between plant roots, soil physicochemical properties, and microbial communities. These factors collectively contribute to disease onset and progression.
2.1 Direct pathogenesis: Fusarium oxysporum f. sp. niveum
Watermelon wilt disease is a soilborne disease primarily caused by pathogenic microorganisms inhabiting the rhizosphere. Upon infection, these pathogens produce phytotoxic compounds such as fusaric acid, lycomarasmin, and dehydrofusaric acid, which promote tissue colonization and block vascular conduits, thereby disrupting water transport and ultimately inducing plant wilting (Rahman et al., 2021; Michielse and Rep, 2009; Wang X. et al., 2024). Four distinct races of FON (race 0–3) have been identified based on their virulence and ability to infect different watermelon cultivars (Meru and McGregor, 2016). Race 0 is the least aggressive and only affects susceptible varieties lacking resistance genes, such as “Sugar Baby” and “Black Diamond.” Race 1 exhibits moderate pathogenicity and can infect some resistant cultivars, including “Charleston Gray.” Race 2 is highly virulent and can infect most watermelon varieties, causing severe wilt symptoms (Bruton et al., 2008). Race 3, first reported in 2006 and identified as a new race in 2009, represents the most aggressive strain of FON. As of 2018, no commercially available watermelon varieties have resisted race 3 (Amaradasa et al., 2018; Zhou et al., 2010).
Significant progress has been made in understanding the pathogenicity of FON. Recent studies indicate that various proteins, including protein kinase FonKin4, the pumilio protein family (PUF), and the ubiquitin–proteasome system (UPS), play essential roles in the ability of FON to infect and colonize host tissues (Gao et al., 2023; Noman et al., 2023b; Wang J. et al., 2024). Genes responsible for encoding these proteins have been identified as virulence factors. For example, FonSMT3, FonAOS1, FonUBC9, and FonMMS21 are key components of the SUMOylation pathway, which regulates critical cellular and biochemical processes. Deleting these genes has been shown to significantly reduce the pathogenicity of FON (Azizullah et al., 2023; Azizullah et al., 2024).
Additionally, mutations in genes such as FonNot2 have also been linked to decreased virulence (Dai et al., 2016). A complex network of multiple factors regulates the pathogenicity of FON. Further research is needed to elucidate the molecular mechanisms underlying FON infection in watermelon, which could aid in developing effective disease management strategies.
2.2 Effects of allelopathic chemicals
Allelopathic compounds, primarily secreted by plant roots and associated microorganisms, play a pivotal role in mediating interactions between plants and the rhizosphere microbiota (Supplementary Table S1). These chemicals, which include phenolic compounds, terpenes, flavonoids, polyacetylenes, and organic acids, can exert either stimulatory or inhibitory effects on neighboring organisms (Hao et al., 2010; Li et al., 2021; Polyak and Sukcharevich, 2019). In watermelon (Citrullus lanatus L.), allelopathic interactions have been increasingly recognized as a key factor influencing the onset and progression of Fusarium wilt, primarily caused by FON.
Watermelon roots predominantly exude phenolic acids as allelochemicals, including cinnamic acid, vanillic acid, coumaric acid, and ferulic acid (Wu et al., 2008a). These compounds have been demonstrated to act as autotoxins that inhibit seedling growth, with phytotoxic effects that may persist throughout the entire plant life cycle (Hao et al., 2007). Importantly, these autotoxins also influence the composition and stability of the rhizosphere microbial community. Disruption of microbial equilibrium can lead to reduced microbial antagonism against soilborne pathogens, thereby increasing the plant’s susceptibility to Fusarium wilt (Liu Q. et al., 2022).
Comparative studies across watermelon cultivars revealed significant differences in the types and relative proportions of phenolic acids. Wu et al. (2009) identified and classified 12 phenolic acids into antifungal and fungus-promoting groups, observing that the ratio of antifungal to fungus-promoting compounds was substantially lower in susceptible cultivars than in resistant ones. This finding suggests that a high abundance of fungus-promoting phenolic acids may compromise defense capacity in plants and contribute to disease development.
In addition to indirectly influencing FON through shifts in microbial dynamics, allelochemicals can also exert direct effects on the pathogen. Ferulic acid, at concentrations of 0.2 g·L−1, has been shown to stimulate hyphal growth, conidial germination, and mycotoxin production in FON, thereby exacerbating disease severity (Wu et al., 2010). Similarly, elevated levels (≥1.2 g·L−1) of specific amino acid have been found to promote FON growth and development (Liu et al., 2009). On the other hand, certain naturally occurring compounds may enhance plant resistance to Fusarium wilt. For instance, palmitic acid, a saturated fatty acid, has been shown to activate host defense mechanisms. Its application leads to increased reactive oxygen species (ROS) production and upregulation of defense-related enzymes, resulting in a significant reduction in FON abundance and enhanced early-stage resistance in watermelon seedlings (Kou et al., 2021; Ma et al., 2021; Li et al., 2022).
Taken together, these findings highlight the dual role of allelopathic compounds as both risk factors and potential modulators in Fusarium wilt pathogenesis. A deeper understanding of their biosynthesis, accumulation, and ecological impacts may offer novel insights for the development of sustainable disease management strategies.
2.3 Abiotic exacerbating factors
In addition to microbial pathogens and allelopathic compounds, abiotic environmental conditions significantly influence the onset and severity of watermelon wilt disease. These factors can either directly affect the physiological state of the host plant or indirectly alter the composition and functional stability of the rhizosphere microbiome, thereby modulating plant-pathogen interactions.
Soil physicochemical properties play a pivotal role in shaping disease dynamics. Soil pH, for instance, has been shown to affect both the growth of FON and the structure of microbial communities in the rhizosphere (hong et al., 2013; Lv et al., 2023). Suboptimal pH conditions, particularly acidification, can suppress beneficial microbial taxa while favoring pathogenic fungi (Das et al., 2022; Palmieri et al., 2023). Likewise, low levels of soil organic matter and poor aeration reduce the abundance and activity of antagonistic microorganisms that otherwise suppress FON proliferation (Zheng et al., 2024; Pommier, 2023). Soil compaction and poor drainage further exacerbate this imbalance by promoting anaerobic microenvironments, which impair root function and increase plant susceptibility to infection (Xu et al., 2025).
Nutrient availability and fertilization practices are also critical determinants of disease progression. Excessive nitrogen fertilization has been associated with increased disease incidence, likely due to enhanced pathogen growth and weakened host defenses (Sun et al., 2021). High nitrogen levels may stimulate the production of pathogen-derived phytotoxins such as fusaric acid and compromise systemic resistance in plants (Mur et al., 2017; Sun et al., 2020; Palmieri et al., 2023). In contrast, deficiencies in potassium and phosphorus, which are essential nutrients for root development and immune signaling, have been shown to compromise plant defense mechanisms and enhance susceptibility to soilborne pathogens (Dutta et al., 2024; Amtmann et al., 2008). Moreover, imbalanced nutrient regimes can modulate the production of root exudates, potentially altering the abundance and activity of pathogenic or beneficial microbes in the rhizosphere.
Environmental stressors such as extreme temperatures, drought, and flooding further intensify Fusarium wilt severity. Elevated soil temperatures not only enhance FON sporulation and virulence but also accelerate plant metabolic rates, often outpacing the activation of defense responses (Fawe et al., 1998; Venkatesh and Kang, 2019). Drought conditions disrupt root integrity and restrict the movement of defense-related phytohormones, while excessive soil moisture reduces oxygen availability, exacerbating root hypoxia and facilitating pathogen ingress (Jogawat et al., 2021; Gusain et al., 2024). These environmental fluctuations may also induce shifts in root exudate composition, indirectly promoting the accumulation of autotoxins or pathogen-favoring compounds in the rhizosphere (Zhalnina et al., 2018).
Collectively, these abiotic stressors interact in a complex and often synergistic manner with biotic factors, amplifying the risk of Fusarium wilt disease in watermelon. Integrated soil health management can suppress FON activity and reduce disease severity. Key strategies include adjusting pH, optimizing nutrients, improving drainage, and implementing crop rotations.
3 Harms of watermelon wilt disease
3.1 Plant damage and decline in yield and fruit quality
Once Fusarium wilt occurs in watermelon, it severely disrupts plant physiological functions, leading to significant reductions in both yield and fruit quality. Studies have shown that the pathogen FON exerts its pathogenicity primarily through the secretion of the secondary metabolite fusaric acid (FA). FA compromises the integrity of host cell membranes, induces excessive accumulation of mitochondrial reactive oxygen species (ROS), disrupts energy metabolism, and ultimately triggers programmed cell death (Xie et al., 2019). Additionally, FA significantly inhibits the biosynthesis of photosynthetic pigments in leaves, thereby reducing photosynthetic efficiency and causing leaf wilting and necrosis (Singh et al., 2017). As the initial site of infection, the root system also experiences substantial damage; FA suppresses root cell dehydrogenase activity, decreases membrane potential, and impairs water and nutrient uptake (Wu et al., 2008b). Moreover, FA-induced oxidative stress leads to lipid peroxidation, resulting in structural deterioration of leaf cells and further weakening the overall vitality of plants (Iqbal et al., 2023).
Such systemic damage directly compromises the yield formation process in watermelon. In continuous cropping systems, field incidence rates often reach 10–80% (Yang B. et al., 2024; Banerjee et al., 2025). Additionally, infected plants exhibit shortened growth periods, reduced fruit set, and impaired fruit development, which collectively intensify yield losses (Tong et al., 2025; Zheng et al., 2024; Pal et al., 2020). The soil persistence and accumulation ability of FON creates ongoing risks for watermelon production and complicates Fusarium wilt control.
3.2 Disruption of soil ecosystem
As the disease progresses, a cascade of physicochemical and biological alterations occurs within the rhizosphere. Notably, soil acidification becomes pronounced due to a gradual decline in pH, accompanied by elevated electrical conductivity and salinity, which together accelerate the process of soil salinization (Zheng et al., 2024). These changes not only compromise aggregate stability and reduce porosity but also degrade the overall structure of the soil, thereby impairing its ability to support healthy plant growth (Alekseeva et al., 2011; Pan et al., 2024). The resulting decrease in nutrient bioavailability often prompts growers to apply excessive amounts of chemical fertilizers in an attempt to sustain productivity. Overapplication of nitrogen-based fertilizers has been shown to suppress beneficial microbial taxa involved in key ecological functions, including antibiotic synthesis and nitrogen transformation (Fan et al., 2019; Zhang et al., 2022b). This imbalance disrupts microbial-mediated nutrient cycling and weakens soil disease resistance mechanisms. Moreover, excessive nitrogen inputs disturb the stoichiometric balance of carbon, nitrogen, and phosphorus in the rhizosphere, leading to nutrient imbalances that further constrain plant productivity (Cui et al., 2024).
Management practices involving the application of broad-spectrum fungicides, although aimed at disease suppression, often exert non-selective effects on soil microbiota. Such treatments can inhibit beneficial fungal populations and indirectly affect bacterial communities, particularly those involved in nitrogen cycling processes like ammonia oxidation (Carvalho, 2017; Luz et al., 2018; Meyer et al., 2024). Additionally, the potential for fungicide residues to accumulate in plant tissues and enter the food chain raises concerns about their broader ecological and human health implications (Lv et al., 2022). Collectively, these findings underscore the urgent need to adopt integrated soil and disease management strategies that prioritize ecological balance and long-term soil health.
4 Remediation measures of watermelon wilt disease
To minimize the yield and economic losses associated with watermelon production, the prevention and control of watermelon wilt disease have become a key research focus. Significant progress has been made in recent years. By managing interactions among plants, soil, and microorganisms, crop health and resistance to wilt disease can be significantly improved (Tong et al., 2024). Control strategies include breeding and cultivating highly resistant varieties, adjusting cropping systems, soil fumigation, applying microbial agents, optimizing fertilizer use, and reductive soil disinfestation.
4.1 Agroecological control measures
Agroecological strategies, including the development of resistant cultivars, Anti-stress grafting technique, and the optimization of cropping systems, offer sustainable solutions for managing Fusarium wilt in watermelon. The effectiveness of host resistance is closely related to both the watermelon genotype and the specific FON race (Supplementary Table S2). Several cultivars and germplasms such as “Charleston Gray,” “Longke No.13,” “PI 296341” and “PI 271769” have shown resistance to races 0, 1, or 2 (Wu et al., 2019; Bruton et al., 2008; Su et al., 2024; Wechter et al., 2012; Zhou and Everts, 2003), but highly resistant varieties remain limited, and no commercial cultivars are currently available for race 3 (Zhou et al., 2010; Song et al., 2017). Although conventional breeding methods and molecular techniques such as marker-assisted selection and QTL mapping have accelerated progress (Branham et al., 2019; Fall et al., 2018; Yang et al., 2009), developing cultivars with durable and broad-spectrum resistance remains challenging. By combining vigorous, stress-resistant rootstocks with high-yielding, high-quality scions, grafting not only enhances crop resistance to diseases but also improves tolerance to drought, salinity, and heavy metal stress. For instance, in watermelon grafting trials, using pumpkin as a rootstock significantly reduced the incidence of Fusarium wilt and increased the activity of antioxidant enzymes, thereby improving the plant’s adaptability to environmental stress (Mashilo et al., 2023).
Cropping system adjustments also play a key role in disease suppression. Crop rotation effectively suppresses Fusarium wilt through multiple mechanisms. Rice-watermelon rotation with intermittent flooding reduces FON populations (Ali et al., 2020). Wheat rotation significantly restructures soil microbial communities, enhancing beneficial taxa (e.g., increasing Podospora by 97%) while suppressing pathogens (e.g., reducing Fusarium by 89%), thereby improving soil health and disease suppression (Tian et al., 2023; Hu et al., 2025). Watermelon-garlic rotation increased yield by 67% and reduced wilt incidence by 50% (Yang et al., 2016), with garlic root exudates containing antimicrobial diallyl disulfide (DADS) identified as a key mechanism (Ren et al., 2018). Intercropping with wheat or aerobic rice can suppress pathogen growth through allelopathy and rhizosphere modification (Hao et al., 2010; Ren et al., 2008; Ren et al., 2016; Lv et al., 2018; Xu et al., 2015; Yu et al., 2019), though improper combinations may result in interspecies competition (Huang et al., 2017). Common intercropping combinations for watermelon include aerobic rice and wheat. Studies have shown that intercropping with aerobic rice suppresses pathogen spore production and modifies the microbial community in rhizosphere soil by influencing watermelon root exudates, thereby reducing disease severity (Hao et al., 2010; Ren et al., 2016). Other cultural practices, including delayed transplanting under higher temperatures (Keinath et al., 2019) and grafting onto resistant rootstocks (Ge et al., 2022; Ling et al., 2015; Toporek and Keinath, 2020), further contribute to disease mitigation. The integration of these approaches enhances system-level resilience and supports long-term, sustainable management of Fusarium wilt in watermelon.
4.2 Soil fumigation
Soil fumigation is a widely used method for controlling soilborne diseases (Sennett et al., 2022), including Fusarium wilt caused by FON. Fumigants evaporate and diffuse when applied to the soil, eliminating pathogens and beneficial microorganisms (Wang and Tang, 2024; Ge et al., 2021). Numerous studies have demonstrated the effectiveness of various fumigants in managing watermelon wilt disease. For example, ammonium bicarbonate (NH₄HCO₃) has been found to disrupt FON hyphae, causing fragmentation. Its effectiveness is further enhanced in acidic soils when combined with lime, increasing soil pH, improving nutrient availability, and boosting enzymatic activity (Li et al., 2019; Sun et al., 2015). Similarly, dazomet (C₅H₁₀N₂S₂) fumigation has increased soil phosphorus availability and enriched beneficial microorganisms, thereby enhancing watermelon resistance to FON infection (Zhu et al., 2020). Pic-Clor 60, a mixture of 1,3-dichloropropene (C₃H₄Cl₂) and chloropicrin (CCl₃NO₂), has been reported to reduce FON populations by over 90% while suppressing Meloidogyne spp. pathogens (Karki et al., 2022). However, the environmental persistence and non-target toxicity of synthetic fumigants necessitate alternative approaches (Tagele et al., 2021).
To address the limitations and environmental concerns associated with chemical fumigation, the development of safer and eco-friendly alternatives has gained increasing attention. Among these, biofumigation using cruciferous plants, particularly species from the Brassica genus, has shown promising potential due to their high glucosinolates content (Tagele et al., 2021). Although glucosinolates themselves have limited biocidal activity, their hydrolysis by myrosinase released upon tissue disruption produces isothiocyanates, which possess strong herbicidal and antimicrobial properties (Brennan et al., 2020; Zhang et al., 2019). Studies have shown that applying mustard plant extracts can reduce the incidence of Fusarium wilt in watermelon, enhance stress resistance, and promote seedling growth (Yuan et al., 2020). During biofumigation, the decomposition of Brassica tissues releases volatile compounds such as isothiocyanates and sulfur-containing substances, along with heat, which together inhibit pathogen growth (Liu et al., 2023b). Biofumigation combined with microbial inoculants improves soil nutrients, lowers pH, and reshapes fungal communities, mainly Ascomycota and Basidiomycota (95.14–96.17%), thereby creating a more favorable environment for watermelon growth (Chang et al., 2022). Taken together, these findings highlight the multifaceted benefits of biofumigation as a sustainable strategy for enhancing soil health and promoting watermelon productivity in environmentally responsible agricultural systems.
4.3 Application of microbial agents
The direct application of beneficial microbial agents to the soil is an effective strategy for mitigating watermelon wilt. Various microbial species, including Bacillus spp., Pseudomonas spp., Trichoderma spp., Streptomyces spp., and nonpathogenic Fusarium spp., have been utilized for pathogen control (Figure 3) (Faheem et al., 2015). These microorganisms promote plant health and growth through both direct and indirect mechanisms. Directly, they enhance plant development by improving nutrient availability, producing plant hormones, modifying enzyme activity, and generating antimicrobial compounds that inhibit or kill pathogens. Indirectly, certain microorganisms activate induced systemic resistance (ISR), strengthening plant immunity, making them more resistant to diseases and pests (Luo et al., 2023; Viaene et al., 2016).
Recent research has highlighted the potential of microbial-based technologies for controlling watermelon wilt. Noman et al. (2023a) developed biogenic manganese nanoparticles from Bacillus megaterium NOM14, which enhance various physiological processes in watermelon plants, thereby suppressing disease onset. Similarly, Trichoderma asperellum M45a has been shown to trigger defensive responses in watermelon roots against FON infection while increasing the diversity of beneficial rhizosphere bacteria (Zhang Y. et al., 2022). The solid fermentation products of Streptomyces ahygroscopicus strain 769 improve rhizosphere microbial community composition, creating a highly interconnected microbial network that enhances the expression of resistance-related and auxin-regulated genes in watermelon, ultimately reducing the incidence of FON infection (Ge et al., 2024). In addition, inactivated FON mycelium has significantly enhanced soil enzyme activity and systemic resistance in watermelon plants. This treatment also improves the abundance and diversity of beneficial rhizosphere microorganisms while substantially reducing FON populations and disease severity (Xie et al., 2021). However, microbial agents containing a single strain are not always effective. A combination of multiple strains may improve efficiency and stability. For example, a mixture of Pseudomonas fluorescens P4 and Bacillus amyloliquefaciens XY-13 has demonstrated antagonistic effects against 11 pathogenic fungi, including FON, with an inhibition rate of up to 78.17% against watermelon wilt (Yang D. et al., 2024). Similarly, a synthetic microbial consortium consisting of 16 core bacterial strains has been shown to mitigate watermelon wilt, with the synergistic interactions among these microorganisms playing a key role in disease suppression (Qiao et al., 2024).
4.4 Rational fertilization
Continuous cropping leads to the depletion and imbalance of soil nutrients, particularly in monoculture systems, which compromises plant resistance and increases susceptibility to soil-borne pathogens such as Fusarium oxysporum (Chen et al., 2018). Improper fertilization, especially the excessive and long-term application of chemical fertilizers, not only fails to restore soil health but also disrupts microbial communities. High concentrations of chemical inputs reduce microbial diversity, suppress beneficial taxa such as nitrogen-fixing and phosphate-solubilizing bacteria, and increase soil salinity and acidification (Bitew and Alemayehu, 2017). These changes create favorable conditions for the survival and proliferation of pathogenic fungi, thereby intensifying the risk of wilt disease (Fu et al., 2020; Zhang et al., 2022b).
In contrast, rational fertilization strategies, particularly those involving organic and bioorganic amendments, effectively alleviate soil nutrient stress and restore microbial balance. These inputs increase soil organic matter and improve the availability of key nutrients such as nitrogen, phosphorus, and potassium, which support plant development and metabolic resilience (Zhang et al., 2022a). More importantly, organic fertilization enhances the abundance and activity of beneficial microbial groups such as Bacillus, Pseudomonas, and Trichoderma, which are known for their biocontrol functions (Li et al., 2024). Additionally, organic fertilizers improve soil structure and buffer capacity, raising pH levels and reducing the accumulation of toxic ions (Liu et al., 2024). This creates a physicochemical environment less conducive to pathogen infection and colonization. The combined effects of microbial antagonism, improved nutrient dynamics, and enhanced root zone conditions contribute to a marked reduction in the incidence of watermelon wilt (Ling et al., 2014; Zhang et al., 2022b; Zhao et al., 2018). These findings underscore the mechanistic advantages of rational fertilization in promoting plant health and maintaining soil ecological stability.
4.5 Reductive soil disinfestation
Reductive soil disinfestation (RSD) is a soil treatment method that creates strong reducing conditions to eliminate aerobic pathogens. Also known as anaerobic soil disinfestation or biological soil disinfestation (Lopes et al., 2022), this technique was first developed by researchers in Japan (Shinmura, 2000) and the Netherlands (Blok et al., 2000). RSD typically consists of four key steps (Figure 4). First, easily degradable organic materials are evenly mixed into the soil. Second, the soil is flooded to saturation. Third, the soil is covered with a plastic mulch film for several weeks to maintain anaerobic conditions. Finally, the plastic film is removed, and excess water is drained to prepare the soil for planting (Ueki et al., 2018). The effectiveness of RSD is attributed to several mechanisms. Anaerobic and high-temperature conditions impose stress on pathogens. Anaerobic bacteria decompose organic matter, producing compounds harmful to pathogens (Momma et al., 2006). Additionally, the process leads to the release of iron and manganese ions, which further contribute to pathogen suppression (Momma et al., 2011). RSD also induces shifts in soil microbial communities, favoring the proliferation of beneficial microbes (Hewavitharana and Mazzola, 2016).
While these mechanisms collectively contribute to effective pathogen suppression, the transient nature of RSD-induced soil modifications poses challenges for long-term disease control, as observed in subsequent cropping cycles. Studies have shown that RSD significantly reduces bacterial diversity and alters microbial community composition. However, after watermelon replanting, bacterial diversity gradually recovers (Meng et al., 2019). Liu et al. (2018) showed that RSD treatment effectively suppresses FON growth while promoting beneficial soil microbiota. This not only prevents watermelon wilt but also enhances plant growth. However, after subsequent watermelon planting, the microbial community structure tends to revert to its original state, allowing FON populations to recover and regain pathogenicity. Researchers suggest that watermelon root exudates may play a role in driving this phenomenon. To extend the efficacy of RSD, some researchers have tested prolonged treatment durations at low temperatures (3.3°C–12.2°C), which have been shown to further improve soil microbial communities and physicochemical properties while suppressing soilborne pathogens (Liu L. et al., 2022). While RSD effectively controls watermelon wilt, the risk of disease recurrence in subsequent plantings remains. Further research is needed to better understand the mechanisms behind this process and to develop strategies for extending the duration of RSD treatment efficacy.
5 Conclusions and future perspectives
Watermelon wilt disease, caused by FON, represents a significant threat to global watermelon production, particularly in continuous cropping systems. The pathogenesis involves dynamic tripartite interactions among: (i) FON virulence factors, (ii) allelochemical-mediated rhizosphere feedbacks, and (iii) soil microbiome-physicochemical co-dysregulation, culminating in systemic host physiological disruption and yield depression.
Current management strategies, including resistant varieties, soil fumigation, microbial agents, and reductive soil disinfestation, have shown partial success but require optimization for improved efficacy and sustainability. Future research should focus on four key directions: First, advancing breeding programs through multi-omics approaches to develop durable resistant cultivars. Second, engineering precision microbiome solutions that simultaneously target pathogens, degrade allelochemicals, and enhance plant immunity. Third, exploring innovative nanotechnology applications for targeted delivery of antifungal compounds. Fourth, optimizing integrated soil health management practices tailored to regional conditions.
This comprehensive approach, balancing scientific innovation with practical application, will be crucial for ensuring the long-term sustainability of watermelon production while maintaining environmental integrity and meeting global food demands. Future studies should prioritize field validation of emerging technologies and economic feasibility assessments to facilitate widespread adoption.
Author contributions
YT: Investigation, Methodology, Writing – original draft, Writing – review & editing. HD: Investigation, Methodology, Writing – original draft, Writing – review & editing. JX: Methodology, Visualization, Writing – review & editing, Investigation. BZ: Visualization, Methodology, Writing – review & editing, Software. XiaoZ: Writing – review & editing, Software, Visualization, Investigation. YD: Conceptualization, Writing – review & editing. XianZ: Methodology, Conceptualization, Writing – review & editing, Writing – original draft. MC: Writing – review & editing, Methodology, Funding acquisition, Project administration, Supervision, Writing – original draft, Conceptualization, Resources.
Funding
The author(s) declare that financial support was received for the research and/or publication of this article. This work was funded by the National Key R&D Program of China [No. 2019YFC1805100], the National Natural Science Foundation of China [No. 51664025], the Jiangxi Provincial Natural Science Foundation [No. 20232ACB203026], the Science and Technology Project of Ganzhou City [No. 2023PNS27982]. Jiangxi Provincial Key Laboratory of Environmental Pollution Prevention and Control in Mining and Metallurgy [No.2023SSY01071], the National Agricultural Experimental Station for Agricultural Environment, Fengxian, China [No. NAES035AE03].
Conflict of interest
The authors declare that the research was conducted in the absence of any commercial or financial relationships that could be construed as a potential conflict of interest.
Generative AI statement
The authors declare that no Gen AI was used in the creation of this manuscript.
Publisher’s note
All claims expressed in this article are solely those of the authors and do not necessarily represent those of their affiliated organizations, or those of the publisher, the editors and the reviewers. Any product that may be evaluated in this article, or claim that may be made by its manufacturer, is not guaranteed or endorsed by the publisher.
Supplementary material
The Supplementary material for this article can be found online at: https://www.frontiersin.org/articles/10.3389/fmicb.2025.1601130/full#supplementary-material
References
Akhter, A., Hage-Ahmed, K., Soja, G., and Steinkellner, S. (2016). Potential of fusarium wilt-inducing chlamydospores, in vitro behaviour in root exudates and physiology of tomato in biochar and compost amended soil. Plant Soil 406, 425–440. doi: 10.1007/s11104-016-2948-4
Alekseeva, T., Alekseev, A., Xu, R., Zhao, A., and Kalinin, P. (2011). Effect of soil acidification induced by a tea plantation on chemical and mineralogical properties of Alfisols in eastern China. Environ. Geochem. Health 33, 137–148. doi: 10.1007/s10653-010-9327-5
Ali, A., Xue, Q., Chen, S., Ren, Y., Fu, Q., Shao, W., et al. (2020). Herbicides act as restrictive factors in rice-watermelon rotation. Sci. Hortic. 261:108974. doi: 10.1016/j.scienta.2019.108974
Amaradasa, B., Beckham, K., Dufault, N., Sanchez, T., Ertek, T., Iriarte, F., et al. (2018). First report of fusarium oxysporum f. sp. niveum race 3 causing wilt of watermelon in Florida, U.S.a. Plant Dis. 102:1029. doi: 10.1094/PDIS-10-17-1649-PDN
Amtmann, A., Troufflard, S., and Armengaud, P. (2008). The effect of potassium nutrition on pest and disease resistance in plants. Physiol. Plant. 133, 682–691. doi: 10.1111/j.1399-3054.2008.01075.x
Azizullah, M., Gao, Y., Wang, H., Xiong, X., Wang, J., Li, D., et al. (2024). The SUMOylation pathway regulates the pathogenicity of fusarium oxysporum f. sp. niveum in watermelon through stabilizing the pH regulator Fon Pal C via SUMOylation. Microbiol. Res. 281:127632. doi: 10.1016/j.micres.2024.127632
Azizullah,, Noman, M., Gao, Y., Wang, H., Xiong, X., Wang, J., et al. (2023). The SUMOylation pathway components are required for vegetative growth, asexual development, cytotoxic responses, and programmed cell death events in fusarium oxysporum f. sp. niveum. J. Fungi 9:94. doi: 10.3390/jof9010094
Banerjee, D., Surbhi, S., and Anirban, B. (2025). Synthesis and role of nanoparticles as immunomodulators against plant biotic stress: insights into fusarium wilt management. Physiol. Mol. Plant Pathol. 138:102658. doi: 10.1016/j.pmpp.2025.102658
Bitew, Y., and Alemayehu, M. (2017). Impact of crop production inputs on soil health: a review. Asian J. Plant Sci. 16, 109–131. doi: 10.3923/ajps.2017.109.131
Blok, W., Lamers, J., Termorshuizen, A., and Bollen, G. (2000). Control of soilborne plant pathogens by incorporating fresh organic amendments followed by tarping. Phytopathology 90, 253–259. doi: 10.1094/PHYTO.2000.90.3.253
Branham, S., Levi, A., and Wechter, W. (2019). QTL mapping identifies novel source of resistance to fusarium wilt race 1 in Citrullus amarus. Plant Dis. 103, 984–989. doi: 10.1094/PDIS-09-18-1677-RE
Brennan, R., Samantha, G., Robert, W., and Masoud, H. (2020). Biofumigation: an alternative strategy for the control of plant parasitic nematodes. J. Integr. Agric. 19, 1680–1690. doi: 10.1016/S2095-3119(19)62817-0
Bruton, B., Fish, W., and Langston, D. (2008). First report of fusarium wilt caused by fusarium oxysporum f. sp niveum race 2 in Georgia watermelons. Plant Dis. 92:983. doi: 10.1094/pdis-92-6-0983b
Carvalho, F. (2017). Pesticides, environment, and food safety. Food Energy Secur. 6, 48–60. doi: 10.1002/fes3.108
Chang, F., Zhang, G., Zhang, L., Lyu, B., Liu, Z., Fan, Q., et al. (2022). Effects of biological fumigation combined with microbial agents on fungi community structure in continuous watermelon cropping soil. Chin. J. Eco-Agric. 30, 248–257. doi: 10.12357/cjea.20210473
Chen, W., Teng, Y., Li, Z., Liu, W., Ren, W., Luo, Y., et al. (2018). Mechanisms by which organic fertilizer and effective microbes mitigate peanut continuous cropping yield constraints in a red soil of South China. Appl. Soil Ecol. 128, 23–34. doi: 10.1016/j.apsoil.2018.03.018
Chen, Y., Yang, L., Zhang, L., Li, J., Zheng, Y. L., Yang, W., et al. (2023). Autotoxins in continuous tobacco cropping soils and their management. Front. Plant Sci. 14:10. doi: 10.3389/fpls.2023.1106033
Cui, H., He, C., Zheng, W., Jiang, Z., and Yang, J. (2024). Effects of nitrogen addition on rhizosphere priming: the role of stoichiometric imbalance. Sci. Total Environ. 914:169731. doi: 10.1016/j.scitotenv.2023.169731
Dai, Y., Cao, Z., Huang, L., Liu, S., Shen, Z., Wang, Y., et al. (2016). CCR4-not complex subunit Not2 plays critical Rroles in vegetative growth, conidiation and virulence in watermelon fusarium wilt pathogen fusarium oxysporum f. sp. niveum. Front. Microbiol. 7:1449. doi: 10.3389/fmicb.2016.01449
Das, P., Singh, R., Nagpure, G., Mansoori, A., Singh, R., Ghazi, I., et al. (2022). Plant-soil-microbes: a tripartite interaction for nutrient acquisition and better plant growth for sustainable agricultural practices. Environ. Res. 214:113821. doi: 10.1016/j.envres.2022.113821
Ding, S., Zhou, D., Wei, H., Wu, S., and Xie, B. (2021). Alleviating soil degradation caused by watermelon continuous cropping obstacle: application of urban waste compost. Chemosphere 262:128387. doi: 10.1016/j.chemosphere.2020.128387
Dutta, A., Peter, M., and Ghazanfar, A. (2024). Balancing act: the dynamic relationship between nutrient availability and plant defence. Plant J. 120, 1724–1734. doi: 10.1111/tpj.17098
Faheem, M., Raza, W., Zhong, W., Nan, Z., Shen, Q., and Xu, Y. (2015). Evaluation of the biocontrol potential of Streptomyces goshikiensis YCXU against fusarium oxysporum f. sp. niveum. Biol. Control 81, 101–110. doi: 10.1016/j.biocontrol.2014.11.012
Fall, L., Clevenger, J., and McGregor, C. (2018). Assay development and marker validation for marker assisted selection of fusarium oxysporum f. sp. niveum race 1 in watermelon. Mol. Breed. 38:130. doi: 10.1007/s11032-018-0890-2
Fan, K., Delgado-Baquerizo, M., Guo, X., Wang, D., Wu, Y., Zhu, M., et al. (2019). Suppressed N fixation and diazotrophs after four decades of fertilization. Microbiome 7:143. doi: 10.1186/s40168-019-0757-8
FAO (2023). FAOSTAT. Crops and livestock products. Available online at: https://www.fao.org/faostat/en/#data/QCL (Accessed March 15, 2025).
Fawe, A., Abou-Zaid, M., Menzies, J., and Bélanger, R. (1998). Silicon-mediated accumulation of flavonoid phytoalexins in cucumber. Phytopathology 88, 396–401. doi: 10.1094/PHYTO.1998.88.5.396
Fu, L., Xiong, W., Dini-Andreote, F., Wang, B., Tao, C., Ruan, Y., et al. (2020). Changes in bulk soil affect the disease-suppressive rhizosphere microbiome against fusarium wilt disease. Front. Agricult. Sci. Eng. 7, 307–316. doi: 10.15302/j-fase-2020328
Gao, Y., Xiong, X., Wang, H., Bi, Y., Wang, J., Yan, Y., et al. (2023). Fusarium oxysporum f. sp. niveum Pumilio 1 regulates virulence on watermelon through interacting with the ARP2/3 complex and binding to an A-rich motif in the 3′ UTR of diverse transcripts. MBio 14, e00157–e00123. doi: 10.1128/mbio.00157-23
Ge, A., Li, Q., Liu, H., Zhang, Z., Lu, Y., Liang, Z., et al. (2024). Streptomyces-triggered coordination between rhizosphere microbiomes and plant transcriptome enables watermelon fusarium wilt resistance. Microb. Biotechnol. 17:e14435. doi: 10.1111/1751-7915.14435
Ge, A., Liang, Z., Han, L., Xiao, J., Zhang, Y., Zeng, Q., et al. (2022). Rootstock rescues watermelon from fusarium wilt disease by shaping protective root-associated microbiomes and metabolites in continuous cropping soils. Plant Soil 479, 423–442. doi: 10.1007/s11104-022-05532-z
Ge, A., Liang, Z., Xiao, J., Zhang, Y., Zeng, Q., Xiong, C., et al. (2021). Microbial assembly and association network in watermelon rhizosphere after soil fumigation for fusarium wilt control. Agric. Ecosyst. Environ. 312:107336. doi: 10.1016/j.agee.2021.107336
Guo, S., Zhao, S., Sun, H., Wang, X., Wu, S., Lin, T., et al. (2019). Resequencing of 414 cultivated and wild watermelon accessions identifies selection for fruit quality traits. Nat. Genet. 51, 1616–1623. doi: 10.1038/s41588-019-0518-4
Gusain, S., Khushbu, K., and Rohit, J. (2024). Physiological, hormonal and molecular dynamics of root system architectural response to drought stress signaling in crops. Rhizosphere 31:100922. doi: 10.1016/j.rhisph.2024.100922
Hao, W., Ren, L., Ran, W., and Shen, Q. (2010). Allelopathic effects of root exudates from watermelon and rice plants on fusarium oxysporum f.sp. niveum. Plant Soil 336, 485–497. doi: 10.1007/s11104-010-0505-0
Hao, Z., Wang, Q., Christie, P., and Li, X. (2007). Allelopathic potential of watermelon tissues and root exudates. Sci. Hortic. 112, 315–320. doi: 10.1016/j.scienta.2006.12.030
Hewavitharana, S., and Mazzola, M. (2016). Carbon source-dependent effects of anaerobic soil disinfestation on soil microbiome and suppression of Rhizoctonia solani AG-5 and Pratylenchus penetrans. Phytopathology 106, 1015–1028. doi: 10.1094/PHYTO-12-15-0329-R
Hong, C., Yao, Y., Wang, W., Zhu, F., Chen, X., and Xue, Z. (2013). The study on developing laws of soil physical and chemical properties and fusarium oxysporum under different years of watermelon continuous cropping with protected cultivation. Hans J. Agricult. Sci. 3, 25–28. doi: 10.12677/hjas.2013.32006
Hu, S., Shao, Q., Chen, Y., Kammenga, J., and Geisen, S. (2025). Increased diversification of crop rotation systems induces plant-beneficial rhizobiomes to facilitate crop performance. Appl. Soil Ecol. 205:105758. doi: 10.1016/j.apsoil.2024.105758
Huang, C., Liu, Q., Gou, F., Li, X., Zhang, C., van der Werf, W., et al. (2017). Plant growth patterns in a tripartite strip relay intercrop are shaped by asymmetric aboveground competition. Field Crop Res. 201, 41–51. doi: 10.1016/j.fcr.2016.10.021
Hudson, O., Fulton, J., Dong, A., Dufault, N., and Ali, M. (2021). Fusarium oxysporum f. sp. niveum molecular diagnostics past, present and future. Int. J. Mol. Sci. 22:9735. doi: 10.3390/ijms22189735
Iqbal, N., Zalán, C., Attila, Ö., and Péter, P. (2023). Fusaric acid-evoked oxidative stress affects plant defence system by inducing biochemical changes at subcellular level. Plant Cell Rep. 43:2. doi: 10.1007/s00299-023-03084-9
Jogawat, A., Yadav, B., Chhaya, N., Lakra, A. K., Singh, A., and Narayan, O. P. (2021). Crosstalk between phytohormones and secondary metabolites in the drought stress tolerance of crop plants: A review. Physiol. Plant. 172, 1106–1132. doi: 10.1111/ppl.13328
Karin, H., and Melanie, B. (2009). Watermelon contains 92% water but it also contains allergens! Int. Arch. Allergy Immunol. 149, 289–290. doi: 10.1159/000205573
Karki, K., Grant, J., Ribeiro, B., da Silva, A., Petkar, A., Hajihassani, A., et al. (2022). Evaluation of pic-clor 60 [choloropicrin pre-mixed with 1,3 dicholoropropene] and soil-applied fungicides for the fusarium wilt management in watermelon. Crop Prot. 154:105894. doi: 10.1016/j.cropro.2021.105894
Keinath, A., Coolong, T., Lanier, J., and Ji, P. (2019). Managing fusarium wilt of watermelon with delayed transplanting and cultivar resistance. Plant Dis. 103, 44–50. doi: 10.1094/PDIS-04-18-0709-RE
Kou, J., Rahman, M. K. U., Du, W., Yang, L., Li, D., Li, W., et al. (2021). Effects of exogenously applied palmitic acid on systemic resistance against fusarium oxysporum f. sp. niveum in watermelon (Citrullus lanatus (Thunb.) Matsum. et Nakai). Allelopathy J. 52, 213–224. doi: 10.26651/allelo.j/2021-52-2-1317
Li, M., Xiao, J., Lei, F., Zheng, K., Lu, W., Ma, J., et al. (2022). Effects of exogenous phthalic acid on seed germination, root physiological characteristics, and mineral element bbsorption of watermelon. Horticulturae 8:235. doi: 10.3390/horticulturae8030235
Li, W., Xin, Z., Xin, A., Li, L., Chen, L., Hua, L., et al. (2024). Enhancement of beneficial microbiomes in plant–soil continuums through organic fertilization: insights into the composition and multifunctionality. Soil Ecol. Lett. 6:230223. doi: 10.1007/s42832-023-0223-1
Li, B., Yin, Y., Kang, L., Feng, L., Liu, Y., Du, Z., et al. (2021). A review: application of allelochemicals in water ecological restoration——algal inhibition. Chemosphere 267:128869. doi: 10.1016/j.chemosphere.2020.128869
Li, H., Yuan, G., Zhu, C., Zhao, T., Zhang, R., Wang, X., et al. (2019). Soil fumigation with ammonium bicarbonate or metam sodium under high temperature alleviates continuous cropping-induced fusarium wilt in watermelon. Sci. Hortic. 246, 979–986. doi: 10.1016/j.scienta.2018.11.090
Ling, N., Deng, K., Song, Y., Wu, Y., Zhao, J., Raza, W., et al. (2014). Variation of rhizosphere bacterial community in watermelon continuous mono-cropping soil by long-term application of a novel bioorganic fertilizer. Microbiol. Res. 169, 570–578. doi: 10.1016/j.micres.2013.10.004
Ling, N., Song, Y., Raza, W., Huang, Q., Guo, S., and Shen, Q. (2015). The response of root-associated bacterial community to the grafting of watermelon. Plant Soil 391, 253–264. doi: 10.1007/s11104-015-2399-3
Liu, L., Chen, S., Zhao, J., Zhou, X., Wang, B., Li, Y., et al. (2018). Watermelon planting is capable to restructure the soil microbiome that regulated by reductive soil disinfestation. Appl. Soil Ecol. 129, 52–60. doi: 10.1016/j.apsoil.2018.05.004
Liu, Z., Fan, Q., Yao, Z., Chang, F., Zhang, L., and Zhang, G. (2023b). Effects of biofumigation combined with microbial agents on watermelon growth and fusarium wilt control. China Plant Protect. 43, 50–55. doi: 10.3969/j.issn.1672-6820.2023.04.009
Liu, Z., Ji, Z., Han, Y., and Sun, Y. (2023a). The mitigation effects of exogenous dopamine treatment on continuous cropping obstacles in watermelon. J. Soil Sci. Plant Nutr. 23, 4233–4249. doi: 10.1007/s42729-023-01344-x
Liu, Y., Lan, X., Hou, H., Ji, J., Liu, X., and Lv, Z. (2024). Multifaceted ability of organic fertilizers to improve crop productivity and abiotic stress tolerance: review and perspectives. Agronomy 14:1141. doi: 10.3390/agronomy14061141
Liu, B., Wu, F., Yang, Y., and Wang, X. (2009). Amino acids in watermelon root exudates and their effect on growth of fusarium oxysporum f.sp nevium. Allelopath. J. 23, 139–147.
Liu, L., Yan, Y., Ali, A., Zhao, J., Cai, Z., Dai, C., et al. (2022). Deciphering the fusarium-wilt control effect and succession driver of microbial communities managed under low-temperature conditions. Appl. Soil Ecol. 171:104334. doi: 10.1016/j.apsoil.2021.104334
Liu, Q., Zhang, L., Wang, L., Wu, Q., Li, K., and Guo, X. (2022). Autotoxin affects the rhizosphere microbial community structure by influencing the secretory characteristics of grapevine roots. Front. Microbiol. 13:18. doi: 10.3389/fmicb.2022.953424
Lopes, E., Canedo, E., Gomes, V., Vieira, B., Parreira, D., and Neves, W. (2022). Anaerobic soil disinfestation for the management of soilborne pathogens: a review. Appl. Soil Ecol. 174:104408. doi: 10.1016/j.apsoil.2022.104408
Luo, S., Wang, Z., and Xu, W. (2023). Bacillus velezensis WB invokes soil suppression of fusarium oxysporum f. sp. niveum by inducing particular taxa. Ann. Agric. Sci. 68, 159–170. doi: 10.1016/j.aoas.2023.12.005
Luz, A., Kassotis, C., Stapleton, H., and Meyer, J. (2018). The high-production volume fungicide pyraclostrobin induces triglyceride accumulation associated with mitochondrial dysfunction, and promotes adipocyte differentiation independent of PPARγ activation, in 3T3-L1 cells. Toxicology 393, 150–159. doi: 10.1016/j.tox.2017.11.010
Lv, H., Cao, H., Nawaz, M., Sohail, H., Huang, Y., Cheng, F., et al. (2018). Wheat intercropping enhances the resistance of watermelon to fusarium wilt. Front. Plant Sci. 9:696. doi: 10.3389/fpls.2018.00696
Lv, T., Cheng, Z., Qian, P., Hao, X., Hong, F., Meng, W., et al. (2023). Plant pathogenesis: toward multidimensional understanding of the microbiome. iMeta 2:e129. doi: 10.1002/imt2.129
Lv, L., Su, Y., Dong, B., Lu, W., Hu, J., and Liu, X. (2022). Dissipation residue behaviors and dietary risk assessment of boscalid and pyraclostrobin in watermelon by HPLC-MS/MS. Molecules 27:4410. doi: 10.3390/molecules27144410
Lv, H., and Yan, C. (2024). Effects of wheat intercropping on growth and occurrence of fusarium wilt in watermelon. PeerJ 12:e17587. doi: 10.7717/peerj.17587
Ma, K., Kou, J., Khashi, U., Rahman, M., Du, W., Liang, X., et al. (2021). Palmitic acid mediated change of rhizosphere and alleviation of fusarium wilt disease in watermelon. Saudi J. Biol. Sci. 28, 3616–3623. doi: 10.1016/j.sjbs.2021.03.040
Ma, Z., Li, P., Yang, C., Feng, Z., Feng, H., Zhang, Y., et al. (2023). Soil bacterial community response to continuous cropping of cotton. Front. Microbiol. 14:10. doi: 10.3389/fmicb.2023.1125564
Mashilo, J., Hussein, S., Rodrigo, I., and Richard, M. (2023). A meta-analysis on rootstock-induced effects in grafted watermelon (Citrullus lanatus var. lanatus). Sci. Hortic. 319:112158. doi: 10.1016/j.scienta.2023.112158
McKeen, D., and Wensley, N. (1961). Longevity of fusarium oxysporum in soil tube culture. Science 134, 1528–1529. doi: 10.1126/science.134.3489.1528
Meng, T., Ren, G., Wang, G., and Ma, Y. (2019). Impacts on soil microbial characteristics and their restorability with different soil disinfestation approaches in intensively cropped greenhouse soils. Appl. Microbiol. Biotechnol. 103, 6369–6383. doi: 10.1007/s00253-019-09964-z
Meru, G., and McGregor, C. (2016). Genotyping by sequencing for SNP discovery and genetic mapping of resistance to race 1 of fusarium oxysporum in watermelon. Sci. Hortic. 209, 31–40. doi: 10.1016/j.scienta.2016.06.005
Meyer, C., Jeanbille, M., Breuil, M. C., Bru, D., Höfer, K., Screpanti, C., et al. (2024). Soil microbial community fragmentation reveals indirect effects of fungicide exposure mediated by biotic interactions between microorganisms. J. Hazard. Mater. 470:134231. doi: 10.1016/j.jhazmat.2024.134231
Michielse, C., and Rep, M. (2009). Pathogen profile update: Fusarium oxysporum. Mol. Plant Pathol. 10, 311–324. doi: 10.1111/j.1364-3703.2009.00538.x
Momma, N., Kobara, Y., and Momma, M. (2011). Fe2+ and Mn2+, potential agents to induce suppression of fusarium oxysporum for biological soil disinfestation. J. Gen. Plant Pathol. 77, 331–335. doi: 10.1007/s10327-011-0336-8
Momma, N., Yamamoto, K., Simandi, P., and Shishido, M. (2006). Role of organic acids in the mechanisms of biological soil disinfestation (BSD). J. Gen. Plant Pathol. 72, 247–252. doi: 10.1007/s10327-006-0274-z
Mur, L., Simpson, C., Kumari, A., Gupta, A., and Gupta, K. (2017). Moving nitrogen to the Centre of plant defence against pathogens. Ann. Bot. 119, mcw179–mcw709. doi: 10.1093/aob/mcw179
Noman, M., Ahmed, T., Ijaz, U., Shahid, M., Nazir, M., Azizullah White, J., et al. (2023a). Bio-functionalized manganese nanoparticles suppress fusarium wilt in watermelon (Citrullus lanatus L.) by infection disruption, host defense response potentiation, and soil microbial community modulation. Small 19:e2205687. doi: 10.1002/smll.202205687
Noman, M., Azizullah Ahmed, T., Gao, Y., Wang, H., Xiong, X., Wang, J., et al. (2023b). Degradation of α-subunits, Doa1 and Doa4, are critical for growth, development, programmed cell death events, stress responses, and pathogenicity in the watermelon fusarium wilt fungus fusarium oxysporum f. sp. niveum. J. Agric. Food Chem. 71, 11667–11679. doi: 10.1021/acs.jafc.3c01785
Pal, S., Eguru, S., Shankara, H., Subbaraman, S., Pitchaimuthu, M., and Keshava, R. (2020). Assessment of fusarium wilt resistant Citrullus sp. rootstocks for yield and quality traits of grafted watermelon. Sci. Hortic. 272:109497. doi: 10.1016/j.scienta.2020.109497
Palmieri, D., Segorbe, D., López-Berges, M., Curtis, F., Lima, G., Pietro, A., et al. (2023). Alkaline pH, low iron availability, poor nitrogen sources and CWI MAPK signaling are associated with increased fusaric acid production in fusarium oxysporum. Toxins 15:50. doi: 10.3390/toxins15010050
Pan, J., Xue, X., Huang, C., You, Q., Guo, P., Yang, R., et al. (2024). Effect of salinization on soil properties and mechanisms beneficial to microorganisms in salinized soil remediation–a review. Res. Cold Arid Reg. 16, 121–128. doi: 10.1016/j.rcar.2024.07.001
Paris, H. (2015). Origin and emergence of the sweet dessert watermelon, Citrullus lanatus. Ann. Bot. 116, 133–148. doi: 10.1093/aob/mcv077
Polyak, Y., and Sukcharevich, V. I. (2019). Allelopathic interactions between plants and microorganisms in soil ecosystems. Biol. Bull. Rev. 9, 562–574. doi: 10.1134/S2079086419060033
Pommier, T. (2023). Diverse organic carbon activates soil microbiome functioning via niche modulation. Soil Ecol. Lett. 5:230180. doi: 10.1007/s42832-023-0180-8
Qiao, Y., Wang, Z., Sun, H., Guo, H., Song, Y., Zhang, H., et al. (2024). Synthetic community derived from grafted watermelon rhizosphere provides protection for ungrafted watermelon against fusarium oxysporum via microbial synergistic effects. Microbiome 12:101. doi: 10.1186/s40168-024-01814-z
Rahman, M., Ahmad, K., Bashir Kutawa, A., Siddiqui, Y., Saad, N., Geok Hun, T., et al. (2021). Biology, diversity, detection and management of fusarium oxysporum f. sp. niveum causing vascular wilt disease of watermelon (Citrullus lanatus): a review. Agronomy 11:1310. doi: 10.3390/agronomy11071310
Ren, K., Hayat, S., Qi, X., Liu, T., and Cheng, Z. (2018). The garlic allelochemical DADS influences cucumber root growth involved in regulating hormone levels and modulating cell cycling. J. Plant Physiol. 230, 51–60. doi: 10.1016/j.jplph.2018.08.007
Ren, L., Huo, H., Zhang, F., Hao, W., Xiao, L., Dong, C., et al. (2016). The components of rice and watermelon root exudates and their effects on pathogenic fungus and watermelon defense. Plant Signal. Behav. 11:e1187357. doi: 10.1080/15592324.2016.1187357
Ren, L., Su, S., Yang, X., Xu, Y., Huang, Q., and Shen, Q. (2008). Intercropping with aerobic rice suppressed fusarium wilt in watermelon. Soil Biol. Biochem. 40, 834–844. doi: 10.1016/j.soilbio.2007.11.003
Sennett, L., Burton, D., Goyer, C., and Zebarth, B. (2022). Chemical fumigation alters soil carbon and nitrogen dynamics in soils amended with substrates of contrasting carbon availability. Geoderma 419:115878. doi: 10.1016/j.geoderma.2022.115878
Seymen, M., Yavuz, D., Ercan, M., Akbulut, M., Çoklar, H., Kurtar, E. S., et al. (2021). Effect of wild watermelon rootstocks and water stress on chemical properties of watermelon fruit. Hortic. Environ. Biotechnol. 62, 411–422. doi: 10.1007/s13580-020-00329-4
Shinmura, A. (2000). Causal agent and control of root rot of welsh onion. PSJ Soil-Borne Disease Workshop Report 20, 133–143.
Singh, V., Singh, H., and Upadhyay, R. (2017). Role of fusaric acid in the development of ‘fusarium wilt’ symptoms in tomato: physiological, biochemical and proteomic perspectives. Plant Physiol. Biochem. 118, 320–332. doi: 10.1016/j.plaphy.2017.06.028
Song, R., Yang, H., Li, C., Zhu, L., and Gu, W. (2017). Germplasm improvement and utilization for small-fruit watermelon with early maturity, good quality, and resistance to disease. Acta Agric. Shanghai 33, 96–100. doi: 10.15955/j.issn1000-3924.2017.03.18
Su, Y., Wang, Z., Ren, K., and Gu, Y. (2024). A new watermelon F1 hybrid—‘Longke no.13’. China Veget. 1, 135–137+155. doi: 10.19928/j.cnki.1000-6346.2024.0055
Sun, Y., Li, Y., Li, Y., Wang, M., Mur, L., Shen, Q., et al. (2021). Nitrate mediated resistance against fusarium infection in cucumber plants acts via photorespiration. Plant Cell Environ. 44, 3412–3431. doi: 10.1111/pce.14140
Sun, Y., Min, W., Mur, L., Shen, Q., and Guo, S. (2020). Unravelling the roles of nitrogen nutrition in plant disease defences. Int. J. Mol. Sci. 21:572. doi: 10.3390/ijms21020572
Sun, L., Song, S., Fu, L., Deng, X., Wang, D., Liang, X., et al. (2015). Exploring a soil fumigation strategy based on ammonium bicarbonate to control fusarium wilts of cucurbits. Crop Prot. 70, 53–60. doi: 10.1016/j.cropro.2015.01.004
Tagele, S., Kim, R., and Shin, J. (2021). Interactions between Brassica biofumigants and soil microbiota: causes and impacts. J. Agric. Food Chem. 69, 11538–11553. doi: 10.1021/acs.jafc.1c03776
Tan, G., Liu, Y., Peng, S., Yin, H., Meng, D., Tao, J., et al. (2021). Soil potentials to resist continuous cropping obstacle: three field cases. Environ. Res. 200:111319. doi: 10.1016/j.envres.2021.111319
Tian, M., Liang, J., Liu, S., Yu, R., and Zhang, X. (2023). Effects of watermelon cropping management on soil bacteria and fungi biodiversity. Agriculture 13:1010. doi: 10.3390/agriculture13051010
Tong, Y., Zheng, X., Hu, Y., Wu, J., Liu, H., Deng, Y., et al. (2024). Root exudate-mediated plant–microbiome interactions determine plant health during disease infection. Agric. Ecosyst. Environ. 370:109056. doi: 10.1016/j.agee.2024.109056
Tong, Y., Zheng, X., Liu, H., Zhang, H., Deng, Y., Chen, M., et al. (2025). Bio-organic fertilizer enhances soil mineral solubilization, microbial community stability, and fruit quality in an 8-year watermelon continuous cropping system. Biol. Fertil. Soils 61, 747–760. doi: 10.1007/s00374-025-01892-7
Toporek, S., and Keinath, A. (2020). Evaluating cucurbit rootstocks to prevent disease caused by Pythium aphanidermatum and P. Myriotylum on watermelon. Plant Dis. 104, 3019–3025. doi: 10.1094/PDIS-03-20-0474-RE
Ueki, A., Kaku, N., and Ueki, K. (2018). Role of anaerobic bacteria in biological soil disinfestation for elimination of soil-borne plant pathogens in agriculture. Appl. Microbiol. Biotechnol. 102, 6309–6318. doi: 10.1007/s00253-018-9119-x
Venkatesh, J., and Kang, B. (2019). Current views on temperature-modulated R gene-mediated plant defense responses and tradeoffs between plant growth and immunity. Curr. Opin. Plant Biol. 50, 9–17. doi: 10.1016/j.pbi.2019.02.002
Viaene, T., Langendries, S., Beirinckx, S., Maes, M., and Goormachtig, S. (2016). Streptomyces as a plant's best friend? FEMS Microbiol. Ecol. 92:fiw119. doi: 10.1093/femsec/fiw119
Wang, J., Gao, Y., Xiong, X., Yan, Y., Lou, J., Noman, M., et al. (2024). The Ser/Thr protein kinase FonKin4-poly(ADP-ribose) polymerase FonPARP1 phosphorylation cascade is required for the pathogenicity of watermelon fusarium wilt fungus fusarium oxysporum f. sp. niveum. Front. Microbiol. 15:1397688. doi: 10.3389/fmicb.2024.1397688
Wang, K., Lu, Q., Dou, Z., Chi, Z., Cui, D., Ma, J., et al. (2024). A review of research progress on continuous cropping obstacles. Front. Agricult. Sci. Eng. 01–270. doi: 10.15302/j-fase-2024543
Wang, Y., and Tang, D. W. S. (2024). Soil chemical fumigation alters soil phosphorus cycling: effects and potential mechanisms. Front. Plant Sci. 15:9. doi: 10.3389/fpls.2024.1289270
Wang, X., Zhu, Z., Zhang, C., Song, J., Wang, Q., Luan, F., et al. (2024). Evaluation of differential miRNA expression between fusarium wilt-resistant and -susceptible watermelon varieties. Sci. Hortic. 332:113189. doi: 10.1016/j.scienta.2024.113189
Wechter, W., Kousik, C., McMillan, M., and Levi, A. (2012). Identification of resistance to fusarium oxysporum f. sp niveum race 2 in Citrullus lanatus var. citroides plant introductions. HortScience 47, 334–338. doi: 10.21273/hortsci.47.3.334
Wu, H., Liu, D., Ling, N., Bao, W., Ying, R., and Shen, Q. (2009). Influence of root exudates of watermelon on fusarium oxysporum f. sp. niveum. Soil Sci. Soc. Am. J. 73, 1150–1156. doi: 10.2136/sssaj2008.0266
Wu, H., Luo, J., Raza, W., Liu, Y., Gu, M., Chen, G., et al. (2010). Effect of exogenously added ferulic acid on in vitro fusarium oxysporum f. sp. niveum. Sci. Hortic. 124, 448–453. doi: 10.1016/j.scienta.2010.02.007
Wu, H., Raza, W., Fan, J., Sun, Y., Bao, W., and Shen, Q. (2008a). Cinnamic acid inhibits growth but stimulates production of pathogenesis factors by in vitro cultures of fusarium oxysporum f.sp. niveum. J. Agric. Food Chem. 56, 1316–1321. doi: 10.1021/jf0726482
Wu, S., Wang, X., Reddy, U., Sun, H., Bao, K., Gao, L., et al. (2019). Genome of 'Charleston Gray', the principal American watermelon cultivar, and genetic characterization of 1,365 accessions in the US National Plant Germplasm System watermelon collection. Plant Biotechnol. J. 17, 2246–2258. doi: 10.1111/pbi.13136
Wu, H., Yin, X., Liu, D., Ling, N., Bao, W., Ying, R., et al. (2008b). Effect of fungal fusaric acid on the root and leaf physiology of watermelon (Citrullus lanatus) seedlings. Plant Soil 308, 255–266. doi: 10.1007/s11104-008-9627-z
Xie, X., Huang, C., Cai, Z., Chen, Y., and Dai, C. (2019). Targeted acquisition of fusarium oxysporum f. sp. niveum toxin-deficient mutant and its effects on watermelon fusarium wilt. J. Agric. Food Chem. 67, 8536–8547. doi: 10.1021/acs.jafc.9b02172
Xie, X., Huang, C., Jiang, H., Zhao, Y., and Dai, C. (2021). Inactivated pathogenic mycelia as a biocontrol agent against fusarium wilt and its effects on continuously cropped watermelon. Biocontrol Sci. Tech. 31, 817–833. doi: 10.1080/09583157.2021.1892591
Xu, J., Long, Z., Sun, B., Zhang, F., Shen, J., and Jin, K. (2025). Optimizing root phenotypes for compacted soils: enhancing root-soil-microbe interactions. Plant Cell Environ. doi: 10.1111/pce.15462
Xu, L., Nicolaisen, M., Larsen, J., Zeng, R., Gao, S., and Dai, F. (2020). Pathogen infection and host-resistance interactively affect root-associated fungal communities in watermelon. Front. Microbiol. 11:605622. doi: 10.3389/fmicb.2020.605622
Xu, W., Wang, Z., and Wu, F. (2015). Companion cropping with wheat increases resistance to fusarium wilt in watermelon and the roles of root exudates in watermelon root growth. Physiol. Mol. Plant Pathol. 90, 12–20. doi: 10.1016/j.pmpp.2015.02.003
Yang, H., Gu, W., Song, R., Yu, J., and Dai, F. (2009). Breeding and evaluation of new superior early-maturing watermelon germplasms with disease resistance. Acta Agric. Shanghai 25, 35–38. doi: 10.3969/j.issn.1000-3924.2009.03.009
Yang, R., Mo, Y., Liu, C., Wang, Y., Ma, J., Zhang, Y., et al. (2016). The effects of cattle manure and garlic rotation on soil under continuous cropping of watermelon (Citrullus lanatus L.). PLoS One 11:e0156515. doi: 10.1371/journal.pone.0156515
Yang, B., Yang, J., Wang, G., Dong, W., Xu, P., Zheng, Y., et al. (2024). Tacrolimus analogue produced by Bacillus amyloliquefaciens HSSN09 suppresses watermelon fusarium wilt by antagonizing FON. Biol. Control 189:105447. doi: 10.1016/j.biocontrol.2024.105447
Yang, D., Zhang, X., Li, Z., Chu, R., Shah, S., Wang, X., et al. (2024). Antagonistic effect of Bacillus and Pseudomonas combinations against fusarium oxysporum and their effect on disease resistance and growth promotion in watermelon. J. Appl. Microbiol. 135:lxae074. doi: 10.1093/jambio/lxae074
Yu, H., Chen, S., Zhang, X., Zhou, X., and Wu, F. (2019). Rhizosphere bacterial community in watermelon-wheat intercropping was more stable than in watermelon monoculture system under fusarium oxysporum f. sp. niveum invasion. Plant Soil 445, 369–381. doi: 10.1007/s11104-019-04321-5
Yuan, R., Ma, X., Liu, L., Zhang, R., and Fan, S. (2020). Inhibitory effects of mustard extract on watermelon fusarium wilt and its influence on protective enzyme activities in plant leaves. Northern Horticult. 3, 36–43. doi: 10.11937/bfyy.20192016
Zhalnina, K., Katherine, B., Zhao, H., Nasim, M., Ulisses, N., Shi, S., et al. (2018). Dynamic root exudate chemistry and microbial substrate preferences drive patterns in rhizosphere microbial community assembly. Nat. Microbiol. 3, 470–480. doi: 10.1038/s41564-018-0129-3
Zhang, P., Xia, L., Sun, Y., and Gao, S. (2024). Soil nutrients and enzyme activities based on millet continuous cropping obstacles. Sci. Rep. 14:8. doi: 10.1038/s41598-024-68278-x
Zhang, Y., Xiao, J., Yang, K., Wang, Y., Tian, Y., and Liang, Z. (2022). Transcriptomic and metabonomic insights into the biocontrol mechanism of Trichoderma asperellum M45a against watermelon fusarium wilt. PLoS One 17:e0272702. doi: 10.1371/journal.pone.0272702
Zhang, M., Xu, J., Liu, G., Yao, X., Li, P., and Yang, X. (2015). Characterization of the watermelon seedling infection process by fusarium oxysporum f. sp. niveum. Plant Pathol. 64, 1076–1084. doi: 10.1111/ppa.12355
Zhang, D., Yan, D., Fang, W., Huang, B., Wang, X., Wang, X., et al. (2019). Chloropicrin alternated with biofumigation increases crop yield and modifies soil bacterial and fungal communities in strawberry production. Sci. Total Environ. 675, 615–622. doi: 10.1016/j.scitotenv.2019.04.222
Zhang, H., Yang, H., Zhang, X., Sun, J., Dong, L., Han, H., et al. (2022a). Bama pig manure organic fertilizer regulates the watermelon rhizosphere bacterial community to inhibit the occurrence of fusarium wilt under continuous cropping conditions. Curr. Microbiol. 79:364. doi: 10.1007/s00284-022-03056-4
Zhang, Z., Zhang, J., Wang, Y., and Zheng, X. (2005). Molecular detection of fusarium oxysporum f. sp. niveum and Mycosphaerella melonis in infected plant tissues and soil. FEMS Microbiol. Lett. 249, 39–47. doi: 10.1016/j.femsle.2005.05.057
Zhang, H., Zheng, X., Wang, X., Xiang, W., Xiao, M., Wei, L., et al. (2022b). Effect of fertilization regimes on continuous cropping growth constraints in watermelon is associated with abundance of key ecological clusters in the rhizosphere. Agric. Ecosyst. Environ. 339:108135. doi: 10.1016/j.agee.2022.108135
Zhao, J., Wang, Y., Liang, H., Huang, J., Chen, Z., and Nie, Y. (2018). The rhizosphere microbial community response to a bio-organic fertilizer: finding the mechanisms behind the suppression of watermelon fusarium wilt disease. Acta Physiol. Plant. 40:17. doi: 10.1007/s11738-017-2581-8
Zheng, X., Wei, L., Lv, W., Zhang, H., Zhang, Y., Zhang, H., et al. (2024). Long-term bioorganic and organic fertilization improved soil quality and multifunctionality under continuous cropping in watermelon. Agric. Ecosyst. Environ. 359:108721. doi: 10.1016/j.agee.2023.108721
Zhou, X., and Everts, K. (2003). Races and inoculum density of fusarium oxysporum f. sp. niveum in commercial watermelon fields in Maryland and Delaware. Plant Dis. 87, 692–698. doi: 10.1094/PDIS.2003.87.6.692
Zhou, X., Everts, K., and Bruton, B. (2010). Race 3, a new and highly virulent race of fusarium oxysporum f. sp. niveum causing fusarium wilt in watermelon. Plant Dis. 94, 92–98. doi: 10.1094/PDIS-94-1-0092
Keywords: Fusarium oxysporum f. sp. niveum, continuous cropping obstacles, soil microbiome, biofumigation, microbial antagonism
Citation: Tong Y, Du H, Xiao J, Zhou B, Zheng X, Deng Y, Zheng X and Chen M (2025) Watermelon wilt disease: causes, harms, and control measures. Front. Microbiol. 16:1601130. doi: 10.3389/fmicb.2025.1601130
Edited by:
Williamson Gustave, University of The Bahamas, BahamasReviewed by:
Jia Liu, Jiangxi Academy of Agricultural Sciences (CAAS), ChinaTin Mar Lynn, Ministry of Education, Myanmar
An-Hui Ge, Chinese Academy of Sciences (CAS), China
Copyright © 2025 Tong, Du, Xiao, Zhou, Zheng, Deng, Zheng and Chen. This is an open-access article distributed under the terms of the Creative Commons Attribution License (CC BY). The use, distribution or reproduction in other forums is permitted, provided the original author(s) and the copyright owner(s) are credited and that the original publication in this journal is cited, in accordance with accepted academic practice. No use, distribution or reproduction is permitted which does not comply with these terms.
*Correspondence: Xianqing Zheng, enhxZnhmQDE2My5jb20=; Ming Chen, Y21Aanh1c3QuZWR1LmNu
†These authors have contributed equally to this work