- Human Metabolomics, North-West University, Potchefstroom, South Africa
Vitamins and minerals (micronutraceuticals) maintain good health. However, the specific effects of these micronutraceuticals on brain health are often overlooked, or not even known. In this review, an overview of the direct and indirect effects of micronutraceuticals on brain energy metabolism (neuroenergetics) and neuronal health is provided. Thereafter, a holistic summary of the existing studies that have shown the impact of micronutraceuticals on neurodegenerative diseases. Lastly, this review concludes by identifying several research gaps that remain and provides suggestions for future research on these hot topics.
1 Introduction
The term “nutraceutical,” first coined in 1989, refers to dietary foods with medicinal and health benefits (Andlauer and Fürst, 2002). In the context of this review, the term “micronutraceuticals” will be used to describe all micronutrients that are inexplicably intertwined with nutraceuticals. These micronutraceuticals are necessary for the functionality of nutraceuticals and include vitamins (A, B, C, D, and E) and minerals (Ca, Cu, Fe, Zn, Mg, etc.). Vitamins encompass a vast array of polar (water-soluble) and non-polar (fat-soluble) chemical compounds that are classified as either non-essential (can be synthesized in our bodies) or essential (can only be obtained from exogenous sources—dietary). While the focus of this review is on vitamins, several minerals are also mentioned as cofactors of important metabolic reactions. The significance of minerals in brain health should not be undermined and deserves equivalent attention to vitamins, but perhaps in another review. Of the vitamins discussed here, most fall into the B group because there is a paucity of information on the other vitamins.
We should have a well-balanced diet to obtain all the vitamins that are needed for healthy living. Even if our diet is well balanced, once we reach middle age and progress to the age of the “elderly,” vitamin supplements are strongly recommended to maintain healthy neurological function (O’Leary et al., 2012). There is increasing scientific evidence that vitamin supplementation can reduce cognitive decline associated with advanced aging (Aisen et al., 2008; O’Leary et al., 2012). Moreover, neuropathological diseases often arise because of perturbed brain energy metabolism (neuroenergetics) (Mason, 2017) and/or physiological changes that impact neuronal health. The roles of micronutraceuticals (“the little guys”) in neuroenergetics and neuronal health are often overlooked.
Hence, the aim of this review is to: (1) give an overview of the role of micronutraceuticals in neuroenergetics; (2) describe the physiological association these micronutraceuticals have with maintaining healthy neuronal cells; (3) relate some of the major neurodegenerative diseases to altered micronutraceuticals; and lastly, (4) identify research gaps and directives for future research on this topic.
2 Micronutraceuticals in neuroenergetics
Homeostatic neuroenergetics (that is, adequate brain energy metabolism) are closely linked to normal neuronal function and brain health. However, homeostasis of the brain is quickly lost when the metabolic pathways associated with neuroenergetics are perturbed (Mason, 2017; Falkowska et al., 2015). Quite often, allostatic overload and/or pathology caused by altered neuroenergetics can be linked to hypovitaminosis—a deficiency in one or more vitamins, as shown in this review.
The brain is the highest energy-consuming organ in the human body, consuming 25% of circulating glucose under normal conditions (Pellerin, 2010). In the brain, primary energy metabolism is predominantly glucocentric—relying mostly on glucose, albeit with shifting paradigms (Mason, 2017). The catabolism of glucose through primary energy metabolic pathways involved in neuroenergetics [glycolysis, Krebs cycle, and oxidative phosphorylation (OXPHOS)] is illustrated in Figure 1. In the case of inborn errors of metabolism (IEMs), any genetic disorder that results in a defective enzyme involved in any of these energy metabolic pathways can lead to serious consequences, often death at an early age, if left untreated. It is also important to note that many enzymes involved in neuroenergetics are dependent on coenzymes and/or cofactors (micronutraceuticals) for their normal functions. Hence, hypovitaminosis can result in metabolic conditions that subtly mimic secondary forms of IEMs.
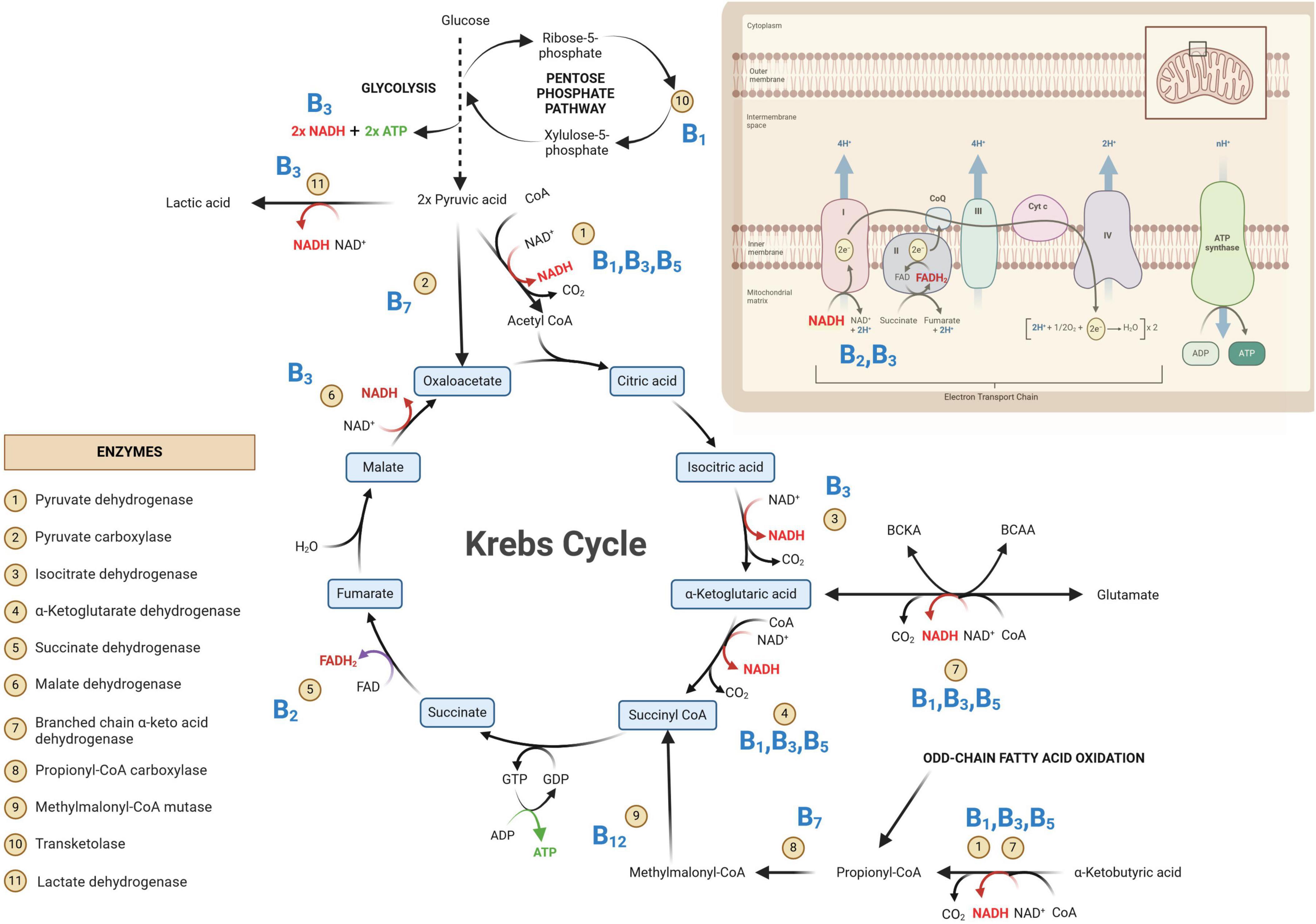
Figure 1. Primary metabolic pathways involved in neuroenergetics [glycolysis, Krebs cycle and oxidative phosphorylation (OXPHOS)]. Some of the key enzymes are indicated and their associated B vitamins (given in bold blue text) are required for homeostasis of neuroenergetics. NAD, oxidized nicotinamide adenine dinucleotide; NADH, oxidized nicotinamide adenine dinucleotide; FAD, oxidized flavin adenine dinucleotide; FADH2, reduced flavin adenine dinucleotide; GDP, guanosine diphosphate; GTP, guanosine triphosphate; ADP, adenine diphosphate; ATP, adenine triphosphate; CoA, coenzyme A; CO2, carbon dioxide.
In Figure 1, the glycolysis metabolic pathway is condensed to that of the primary substrate glucose and the end-product pyruvate. The net yield of glycolysis from one glucose molecule is two units of adenosine triphosphate (ATP; the energy currency of the cells) and two units of reduced nicotinamide adenine dinucleotide (NADH). The emphasis in Figure 1 is on the Krebs cycle—the “heart” of metabolism, which will be discussed in greater detail. The OXPHOS system is also depicted in Figure 1, indicating the entry points of the all-important reduced coenzymes [NADH and reduced flavin adenine dinucleotide (FADH2)] into the electron transport chain. Of note within Figure 1 are the eleven indicated enzymes and vitamins (shown in bold blue text in Figure 1) associated with these enzymes. What immediately stands out in Figure 1 is that all these vitamins are B-group vitamins. Another point of interest in Figure 1 is that seven of the eleven listed enzymes are dehydrogenases.
Dehydrogenases are oxidoreductase enzymes that catalyze either oxidative or reductive metabolic reactions, depending on the direction of the metabolic reaction. Each dehydrogenase enzyme requires a coenzyme that facilitates the transfer of electrons and hydrogen atoms (H+). The coenzymes that function with dehydrogenase are typically NAD+→ NADH + H+ and, to a lesser extent, FAD+→ FADH2 + H+. If we look at the chemical structure of these coenzymes (Figure 2), it starts to become clear why vitamins (in particular B2 and B3) are important. In Figure 2, it is shown that vitamin B3 (niacin/nicotinic acid) is converted to its amide form nicotinamide, which is then incorporated into the structure of NAD. Similarly, for FAD, vitamin B2 (riboflavin) forms part of the chemical structure of FAD (shown in Figure 2). Hence, deficiencies in vitamins B2 and B3 can lead to insufficient levels of the coenzymes FAD and NAD, respectively. Insufficient NAD and FAD will result in decreased production of their reduced forms (NADH and FADH2) and a noticeable decrease in OXPHOS, leading to impaired neuroenergetics. Additionally, increased NAD/NADH and FAD/FADH2 ratios are biochemical indicators of oxidative stress, which will be discussed in the following sections. Another important coenzyme that is needed for normal neuroenergetics is coenzyme A (CoA). The metabolic reactions of pyruvic acid → acetyl-CoA, α-ketoglutaric acid → succinyl-CoA, and α-ketobutyric acid → propionyl-CoA require the presence of CoA. Figure 2 shows the chemical structure of CoA, and it is clearly indicated that the core component of this coenzyme is vitamin B5 (pantothenic acid). Hence, vitamin B5 deficiency will result in CoA deficiency and a subsequent perturbation in neuroenergetics. Lastly, vitamin B1 (thiamine) is also required for the proper functioning of dehydrogenases. Pyruvate dehydrogenase (Krebs cycle), α-ketoglutarate dehydrogenase (Krebs cycle), and branched chain α-keto acid dehydrogenase (involvement of glutamate and branched-chain amino acids in Krebs cycle), as well as transketolase (pentose phosphate pathway), are enzymes that are thiamine-dependent and are important for neuroenergetics (see Figure 1). Because these four enzymatic reactions involve the creation of reducing power (NADH), thiamine thus fights oxidative stress (i.e., it has anti-oxidative properties), making thiamine neuroprotective (discussed in more detail in the next section). Downregulation of pyruvate dehydrogenase and α-ketoglutarate dehydrogenase leads to an interruption of the Krebs cycle, resulting in reduced ATP production in the brain (i.e., reduced neuroenergetics). This loss of ATP also results in calcium overflow in the brain, leading to neuronal apoptosis. It should also be noted that another important mineral in neuroenergetics is magnesium because ATP must bind to magnesium for it to be biologically active. Hence, magnesium deficiency has a global effect on neuroenergetics.
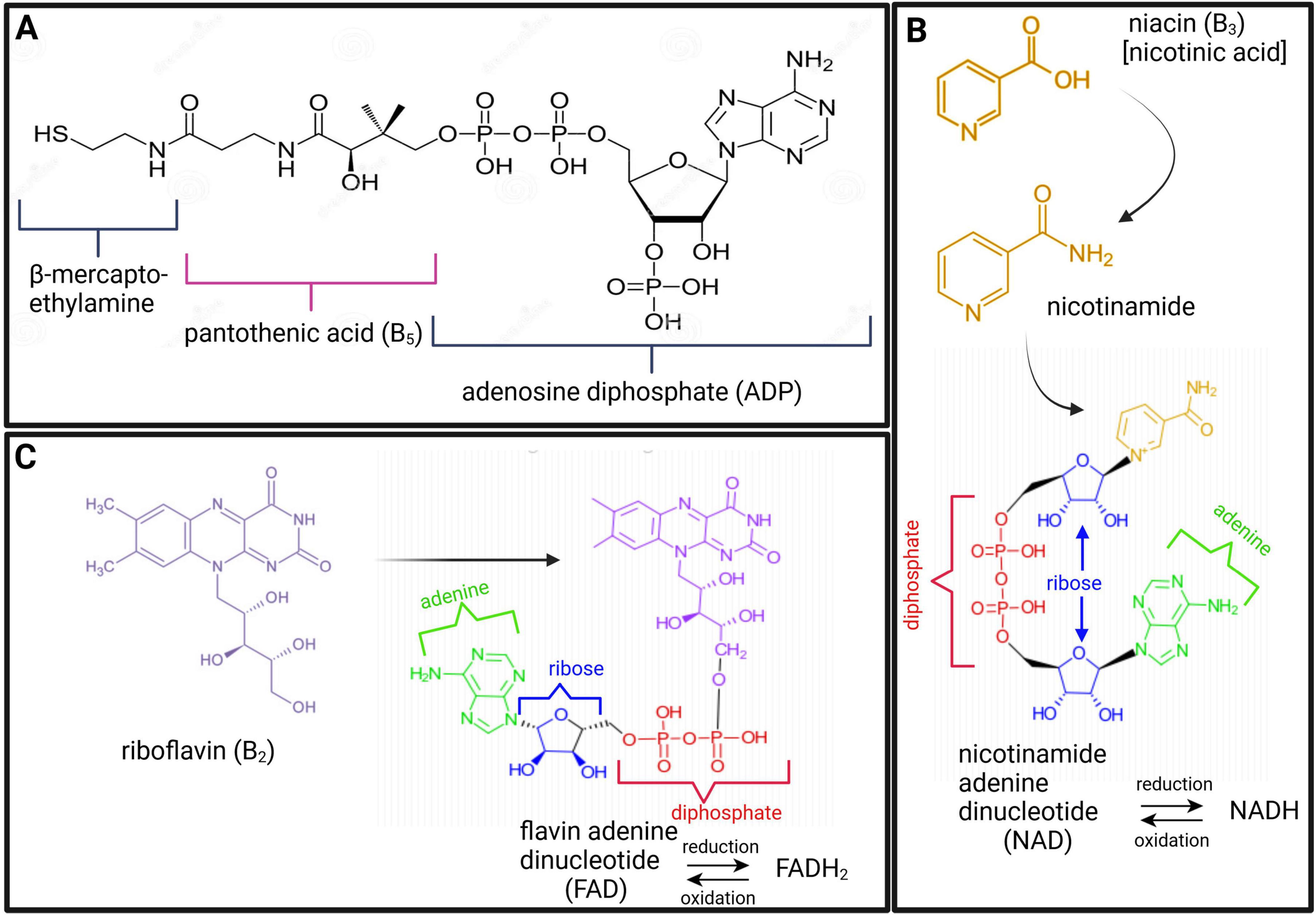
Figure 2. Chemical structures of coenzyme A [CoA; (A)], nicotinamide adenine dinucleotide [NAD; (B)], and flavin adenine dinucleotide [FAD; (C)], highlighting the constituents of vitamins B5 (maroon), B3 (gold), and B2 (purple), respectively.
Two of the eleven enzymes in Figure 1 are carboxylases (pyruvate carboxylase and propionyl-CoA carboxylase). Carboxylases catalyze decarboxylation reactions—removal of carboxyl groups and release of carbon dioxide. These carboxylases are dependent on vitamin B7 (biotin). Hence, vitamin B7 deficiency leads to reduced activity of pyruvate carboxylase (pyruvate → oxaloacetate) and propionyl-CoA carboxylase (propionyl-CoA → methylmalonyl-CoA). Both of these metabolic reactions are also part of the primary energy pathway (Figure 1); hence, a biotin deficiency can result in perturbed neuroenergetics. Lastly, vitamin B12 (cobalamine) is required for the proper functioning of methylmalonyl-CoA mutase, for propionyl-CoA, which is produced by odd-chain fatty acid oxidation, to be incorporated into the Krebs cycle. It should be noted that the other end-product of fatty acid oxidation – acetyl-CoA, is also incorporated into the Krebs cycle for energy production, reinforcing the statement that the Krebs cycle is the “heart” of neuroenergetics.
Thus, as described above and illustrated in Figures 1, 2, vitamins B1, B2, B3, B5, B7, and B12, as well as magnesium, are all necessary micronutraceuticals for the homeostasis of neuroenergetics. A deficiency in one or a combination of these micronutraceuticals will result in allostatic overload. If this allostatic overload is not alleviated (i.e., if this micronutraceutical deficiency is not corrected), the health of the neurons will begin to deteriorate, causing neurodegeneration.
3 Micronutraceuticals and neuronal health
Perturbed neuroenergetics are sufficient to cause neuronal dysfunction, or even neuronal death; however, beyond neuroenergetics, micronutraceuticals have direct involvement in various biological functions that affect the health of neuronal cells (summarized in Table 1), as discussed here.
Firstly, many micronutraceuticals have anti-oxidative effects. One way in which these micronutraceuticals function as anti-oxidants is that they directly or indirectly neutralize free radicals and excitotoxic compounds in the brain. A good example is the excitotoxic metabolite glutamate, which is released into the synapses of neurons when presynaptic neurons polarize and can initiate the action potential of postsynaptic neurons (Hübel et al., 2017). Vitamins B1, B6, C, and E, as well as magnesium, are directly involved in glutamate clearance and have a neuroprotective role (see Table 1). Another way that micronutraceuticals are anti-oxidative agents is that they help maintain the redox cycle of other anti-oxidants, such as the glutathione cycle (see Table 1—vitamins B2, B6, B12, and D). Hence, micronutraceuticals regulate oxidants in the brain, protecting neurons.
Secondly, the anti-inflammatory effects of micronutraceuticals further highlight their neuroprotective effects, which go hand-in-hand with their anti-oxidant abilities. Vitamins B2, B3, C, D, and E (Table 1) have been shown to exhibit anti-inflammatory roles by mitigating oxidative stress and regulating microglial activation (modulating the release of cytokines/chemokines). Additional neuroprotective roles of micronutraceuticals arise through the maintenance of calcium homeostasis (by vitamin D), protection against uncontrolled calcium ion influx (by magnesium), and regeneration of neuroprotective vitamin E (by vitamin C). Indeed, micronutraceuticals represent a unique weapon against the “neurotoxic triad” of excitotoxicity, oxidative stress, and neuroinflammation (Holton, 2021).
Thirdly, the physical structure of neurons (biogenesis, growth, and maintenance) is also dependent on micronutraceuticals. Myelin—the sheath surrounding the axons of neurons—requires vitamins B1, B5, and B6 (see Table 1) for its synthesis and maintenance. Vitamins B1 and C are involved in axonal growth and regeneration (see Table 1). Iron is necessary for neuron dendrite growth and branching (Bastian et al., 2016; Brunette et al., 2010). Vitamin B12 plays an all-around role as it is a major component required for the growth, differentiation, development, and repair of neurons (Reynolds, 2006). Okada et al., 2010) demonstrated that methylcobalamine is the most effective analog of vitamin B12 for promoting neurite outgrowth and neuronal survival through the methylation cycle and that methylcobalamine also promotes nerve regeneration and functional recovery in a rat model of sciatic nerve injury. Of note, vitamins B1, B6, and B12 are often termed “neurotropic B vitamins” due to their joint role in neuronal health and repair (Calderón-Ospina and Nava-Mesa, 2020; Paez-Hurtado et al., 2023). Additionally, since vitamins B9 and B12 are also closely linked with each other, vitamin B9 can also be considered neurotropic.
Lastly, micronutraceuticals are required for normal neuronal function. Nerve conduction and stimulation via gated ion channels are supported by the presence of vitamin B1 (see Table 1). Vitamins B1 and D also have neuro-modulatory and immune-modulatory functions, respectively. Furthermore, neurons function through neurotransmitters, and micronutraceuticals have been linked to the synthesis and functioning of specific neurotransmitters (e.g., acetylcholine, dopamine, gamma-aminobutyric acid, noradrenaline, and serotonin—see Table 1). For example, vitamin B6 is needed for the functioning of aromatic L-amino acid decarboxylase, an enzyme required for the decarboxylation of L-3,4-dihydroxyphenylalanine (L-DOPA) to dopamine and 5-hydroxytryptophan (5-HTP) to serotonin. Hence, vitamin B6 is important for normal neuronal function. Vitamins B6, B9, and B12 are typically discussed together as their complementary roles are inextricably linked (Kennedy, 2016). One very good example of this is the homocysteine-methionine cycle and one-carbon metabolism. For more information, the reader is referred to the following papers that cover this specific topic in detail: Araújo et al., 2015; Calderón-Ospina and Nava-Mesa, 2020; Kennedy, 2016; Lauer et al., 2022; Mitchell et al., 2014; Obeid et al., 2007; Reynolds, 2006; Sechi et al., 2016; Smith, 2008; Vogel et al., 2009 Zhang et al., 2009.
4 Neuropathologies linked to micronutraceutical deficiencies
It has long been taught at medical schools that deficiencies of certain B vitamins, e.g., B1 (beriberi), B3 (pellagra) and B12, contribute to causes of dementia and other neurological problems. Table 2 presents the results of various studies that identified micronutraceuticals as playing an important role in the pathogenesis and progression of neurodegenerative diseases. Although Table 2 is by no means exhaustive nor comprehensive, it is quite evident that Alzheimer’s disease (AD) is one of the more researched neurodegenerative diseases in terms of micronutraceuticals (Mielech et al., 2020). Missing from Table 2 are psychiatric disorders and other neurological problems, such as headaches, migraines, epilepsy, neuroinfectious diseases, and various rarer genetic diseases that present with major neurological complications. In terms of psychiatric disorders, depression is one the most common psychiatric problems in our society, but some studies have shown benefits by supplementation with vitamins B1 (Amerikanou et al., 2023; Rouhani et al., 2023), B6 (Almeida et al., 2014), B9 (Almeida et al., 2014, 2015; Obeid et al., 2007; Petridou et al., 2016), B12 (Almeida et al., 2014, 2015; Moorthy et al., 2012; Obeid et al., 2007; Petridou et al., 2016), and D (Guzek et al., 2023). Bipolar disorder and schizophrenia, two other psychiatric disorders, have been shown to improve with supplementation with vitamin D (Ashton et al., 2021; Gabriel et al., 2023; İmre et al., 2023; Marazziti et al., 2023; Späth et al., 2023) and vitamin B9 (Obeid et al., 2007), respectively. Therefore, these studies show that micronutraceuticals should be incorporated into the treatment of psychiatric disorders.
In migraine research, two particular micronutraceuticals have been investigated: riboflavin (Licina et al., 2023; Marashly and Bohlega, 2017; Nambiar et al., 2011; Plantone et al., 2021; Schoenen et al., 1998; Thompson and Saluja, 2017; Yamanaka et al., 2021) and magnesium (Domitrz and Cegielska, 2022; Licina et al., 2023; Maier et al., 2020). Various forms of epilepsy, which are receiving increasing amounts of research, respond to supplementation with vitamins B6 (Fox and Tullidge, 1946; Gospe, 2002; Kim and Cho, 2019), and B9 (Obeid et al., 2007), and D and E (Kim and Cho, 2019). Another well-researched neurological disorder is Wernicke-Korsakoff syndrome, which is linked specifically to vitamin B1 deficiency and alcoholism (Licina et al., 2023; Marashly and Bohlega, 2017; Nambiar et al., 2011; Plantone et al., 2021; Schoenen et al., 1998; Thompson and Saluja, 2017; Yamanaka et al., 2021; Isenberg-Grzeda et al., 2012; Krzysztoforska et al., 2023; Sechi and Serra, 2007). However, exploring all possible neurological disorders associated with micronutraceuticals is far too broad and beyond the scope of this review. Instead, Table 2 presents the major neurodegenerative diseases.
While minerals are not discussed in detail in this review, Table 2 shows that AD has been linked to the perturbation of several minerals. One mineral in particular—iron—has been observed to accumulate in the plagues of AD cases and to be neurotoxic; whereas, in Parkinson’s disease (PD) cases, iron is deficient (Table 2). As shown in Table 2, iron has not been directly linked to other major neurodegenerative conditions; however, it should be noted that iron is used in various mitochondrial enzyme components. Hence, a deficiency of iron (anemia) leads to perturbed neuroenergetics. Furthermore, various forms of anemia are often linked to a lack of bioavailability of zinc and vitamins A, B6, B9, B12, C, and E (Bhadra and Deb, 2020; Fishman et al., 2000). Hence, excessive or insufficient iron is associated with the pathogenesis and progression of neurodegenerative conditions.
Further inspection of Table 2 reveals that vitamins B2, B3, B5, and A are somewhat neglected in research on neurodegenerative diseases. In their 2014 review, Ashoori and Saedisomeolia (2014) identified vitamin B2 as a neglected micronutraceutical in neuronal research. Similarly, Miller and Dulay declared in their 2008 study that “the function of niacin in the brain has not yet been studied” (Miller and Dulay, 2008). However, since 2014, studies using a mouse model (Zhao et al., 2018) and a yeast model (Chen et al., 2020) have shown that vitamin B2 ameliorates AD. In 2023, Kodam et al. (2023) used an integrated multi-omics approach (transcriptomics, proteomics, and metabolomics) to identify deregulated neuroenergetics in patients with AD modulated by impaired metabolism involving vitamins B2, B5, and B6. In 2014 and 2015, studies on PD by the research team of Wakade, Chong, and Morgan reported the role of niacin in the management of symptoms of PD (Wakade and Chong, 2014; Wakade et al., 2015). Thus, the roles of the coenzymes NAD | NADH, FAD | FADH2, and FMN | FMNH2 in neurodegenerative diseases require more attention. In fact, recent research strategies have “gone back to basics” and are (re)examining the role of neuroenergetics in neurological disease. Two such AD studies, one by Xu et al. (2020) and another by Sang et al. (2022), took a closer look at the ubiquitous compound CoA and found a widespread deficiency in vitamin B5 in AD patients. A 2019 study on deficient neuroenergetics in HD also identified vitamin B5 deficiency (Patassini et al., 2019). Finally, in their in-depth and comprehensive systematic review and meta-analysis of AD, da Silva et al. (2014) found nine AD studies that measured vitamin A; however, the role of vitamin A in AD is still inconclusive, with only four studies reporting significantly decreased levels, whereas nine studies reported no changes. Hence, the role of these neglected vitamins (B2, B3, B5, and A) in neurodegenerative diseases should be a focal point for future research.
The most researched micronutraceuticals in neurodegenerative diseases are neurotropic B vitamins (B6 | B9 | B12), as well as vitamins D and E (see the studies listed in Table 2). Onaolapo and Onaolapo (2023) described the relationship between parkinsonism, vitamin B6, and the phosphorylated form of B6 (pyridoxal phosphate) as quite complex. As described in the previous section, all these vitamins play a significant role in maintaining good neuronal health; hence, it is no surprise that these vitamins are the focus of research on neurodegenerative diseases.
As a point of note, it must be noted that several studies have reported no correlation between vitamin supplementation and the alleviation of symptoms of neurodegenerative diseases (Kennedy, 2016). This begs the question: Why? Why are there conflicting outcomes regarding the efficacy of micronutraceuticals against neurodegenerative diseases and brain health in these studies? With this question in mind and the hot topics identified in this review, herein lies the motivation for the last section of this review: identifying the research gaps that exist in the literature and articulating the directives needed for future research in this scientific field.
5 Research gaps and directives for future research
Based on this review of the literature on the role of micronutraceuticals in neuronal health, it is evident that there are several gaps in the existing knowledge. These research gaps and suggestions for future studies are articulated here.
1) Many studies examining normal brain function have focused only on a small subset of the B vitamins (B6 | B9 | B12) (Kennedy, 2016). Other B vitamins and other micronutraceuticals are largely ignored. Instead of focusing on a small subset of micronutraceuticals in brain health, future research should investigate the potential effects of acute and chronic administration of a full range of micronutraceuticals and their interactions.
2) Studies that examine neurological diseases often neglect vitamins such as B2, B3, and B5. The role of these neglected micronutraceuticals in terms of the allostasis of neuroenergetics during the early onset, progression, and establishment of neurological diseases needs to be examined more closely.
3) The exact mechanisms of action of B vitamins in neurological diseases are unclear (Calderón-Ospina and Nava-Mesa, 2020). Future clinical studies that directly compare the effects of B vitamins in patients with neuropathology are needed to test their synergistic effects.
4) It is clinically difficult to isolate the effect of a single micronutraceutical on brain development because studies typically investigate the combined effects of multiple micronutrients (Tardy et al., 2020). Robust empirical data are needed to test the theoretical hypotheses regarding the effects of individual micronutraceuticals on brain development and function. Additionally, more validated experimental models and clinical data on (relatively ignored) younger and older populations are needed.
5) More studies are needed to identify biomarkers that can be used to assess the effects of micronutraceutical deficiencies (Sechi et al., 2016). New and improved methods are needed for the early detection of dysregulated/deficient micronutraceutical levels, before the onset of neurological symptoms, to prompt corrective treatment.
6) There are several conflicting studies on the therapeutic potential of micronutraceuticals in neurodegenerative diseases. More validation studies are needed to determine whether vitamin supplementation improves neurodegenerative diseases and to determine the optimal dosage and route of micronutraceutical administration.
7) Lack of application of micronutraceuticals laboratory findings in neurodegenerative diseases to clinical practice. Future clinical trials must be designed effectively and objectively to evaluate the progression, or lack thereof, of neurodegenerative diseases in patients receiving individual or combined vitamin supplementation.
8) There is a paucity of clinical studies on the role of micronutraceuticals in the pathogenesis and potential treatment of neurological disorders (Lahoda Brodska et al., 2023; Rai et al., 2021). Clinical studies are needed to elucidate the mechanism(s) by which micronutraceuticals affect the (anti)inflammatory and (anti)oxidative responses in the brain (i.e., evaluate the efficacy and protective role of micronutraceuticals in neurological disorders).
9) Explore the interaction between micronutraceuticals and environmental and lifestyle factors (such as exercise, stress, sleep, etc.) in neurological health to provide more comprehensive, personalized health recommendations.
With the abovementioned nine points in mind, research should be driven to garner mechanistic insights into the role of micronutraceuticals in neuronal health, validate their potential in ameliorating neurodegenerative diseases, and drive their application to personalized medicine (Badaeva et al., 2023).
6 Concluding remarks
This review highlights the role of micronutraceuticals in neuroenergetics, normal neuronal functioning, and health; and provides a summary of some of the neurological consequences of perturbed micronutraceuticals. The focus of this review was on neurons; however, neurons do not function independently but rely on support from glial cells (e.g., astrocytes and oligodendrocytes) to assist in neuronal function. Additional reviews are needed that cover the topic of neuronal support. It must also be stated that this review is by no means an exhaustive nor comprehensive overview of the literature. Specifically, one-carbon metabolism and the role of B vitamins in the homocysteine-methionine cycle and methylation, albeit important metabolic functions, were not discussed in this review. Other related topics not covered in this review include: various forms of brain cancer (gliomas), physical (traumatic) brain injury, neuroinfectious diseases (e.g., meningitis, encephalitis), psychiatric conditions, and cognitive decline, sensory disorders, and neurodiverse conditions (such as autism). Instead, the novelty offered by this review is the collective insights into existing research gaps and the provision of directives for future research.
We are at an inspiring stage in scientific research on the brain and its disorders. Various studies that have involved controlled interventions in large cohorts of participants have begun to demonstrate the mechanistic functions of micronutraceuticals in the brain. Based on the knowledge presented in this review, it is clear that the onset and/or severity of the increasing number of neurodegenerative diseases that occur in our society can be ameliorated by personalized medicine, whereby micronutraceuticals are actively monitored and adjusted accordingly via dietary supplementation. More research, driven by the research gaps and directives presented in this review, is needed to validate the roles of micronutraceuticals in neurodegenerative diseases.
Author contributions
SM: Conceptualization, Data curation, Formal Analysis, Funding acquisition, Investigation, Methodology, Project administration, Resources, Software, Supervision, Validation, Visualization, Writing – original draft, Writing – review and editing.
Funding
The author(s) declare that no financial support was received for the research and/or publication of this article.
Conflict of interest
The author declares that the research was conducted in the absence of any commercial or financial relationships that could be construed as a potential conflict of interest.
Publisher’s note
All claims expressed in this article are solely those of the authors and do not necessarily represent those of their affiliated organizations, or those of the publisher, the editors and the reviewers. Any product that may be evaluated in this article, or claim that may be made by its manufacturer, is not guaranteed or endorsed by the publisher.
References
Abeyawardhane, D., and Lucas, H. (2019). Iron redox chemistry and implications in the Parkinson’s disease brain. Oxid. Med. Cell. Longev. 2019:4609702. doi: 10.1155/2019/4609702
Aisen, P., Schneider, L., Sano, M., Diaz-Arrastia, R., van Dyck, C., Weiner, M., et al. (2008). High-dose B vitamin supplementation and cognitive decline in Alzheimer disease: A randomized controlled trial. JAMA 300, 1774–1783. doi: 10.1001/jama.300.15.1774
Almeida, O., Ford, A., and Flicker, L. (2015). Systematic review and meta-Analysis of randomized placebo-controlled trials of folate and vitamin B12 for depression. Int. Psychogeriatr. 27, 727–737. doi: 10.1017/S1041610215000046
Almeida, O., Ford, A., Hirani, V., Singh, V., vanBockxmeer, F., McCaul, K., et al. (2014). B vitamins to enhance treatment response to antidepressants in middle-aged and older adults: Results from the B-VITAGE randomised, double-blind, placebo-controlled trial. Br. J. Psychiatry 205, 450–457. doi: 10.1192/bjp.bp.114.145177
Amerikanou, C., Gioxari, A., Kleftaki, S., Valsamidou, E., Zeaki, A., and Kaliora, A. (2023). Mental health component scale is positively associated with riboflavin intake in people with central obesity. Nutrients 15:4464. doi: 10.3390/nu15204464
Andlauer, W., and Fürst, P. (2002). Nutraceuticals: A piece of history, present status and outlook. Food Res. Int. 35, 171–176. doi: 10.1016/S0963-9969(01)00179-X
Annweiler, C., Llewellyn, D., and Beauchet, O. (2013). Low serum vitamin D concentrations in Alzheimer’s disease: A systematic review and meta-analysis. J. Alzheimers Dis. 33, 659–674. doi: 10.3233/JAD-2012-121432
Araújo, J., Martel, F., Borges, N., Araújo, J., and Keating, E. (2015). Folates and aging: Role in mild cognitive impairment, dementia and depression. Ageing Res. Rev. 22, 9–19. doi: 10.1016/j.arr.2015.04.005
Arslan, J., Jamshed, H., and Qureshi, H. (2020). Early detection and prevention of Alzheimer’s disease: Role of oxidative markers and natural antioxidants. Front. Aging Neurosci. 12:231. doi: 10.3389/fnagi.2020.00231
Ashoori, M., and Saedisomeolia, A. (2014). Riboflavin (vitamin B2) and oxidative stress: A review. Br. J. Nutr. 111, 1985–1991. doi: 10.1017/S0007114514000178
Ashton, M., Kavanagh, B., Marx, W., Berk, M., Sarris, J., Ng, C., et al. (2021). A systematic review of nutraceuticals for the treatment of bipolar disorder. Can. J. Psychiatry 66, 262–273. doi: 10.1177/0706743720961734
Bâ, A. (2005). Functional vulnerability of developing central nervous system to maternal thiamine deficiencies in the rat. Dev. Psychobiol. 47, 408–414. doi: 10.1002/dev.20105
Bâ, A. (2008). Metabolic and structural role of thiamine in nervous tissues. Cell. Mol Neurobiol. 28, 923–931. doi: 10.1007/s10571-008-9297-7
Badaeva, A., Danilov, A., Clayton, P., Moskalev, A., Karasev, A., Tarasevich, A., et al. (2023). Perspectives on neuronutrition in prevention and treatment of neurological disorders. Nutrients 15:2505. doi: 10.3390/nu15112505
Bagur, M., Murcia, M., Jiménez-Monreal, A., Tur, J., Bibiloni, M., Alonso, G., et al. (2017). Influence of diet in multiple sclerosis: A systematic review. Adv. Nutr. 8, 463–472. doi: 10.3945/an.116.014191
Balion, C., Griffith, L., Strifler, L., Henderson, M., Patterson, C., Heckman, G., et al. (2012). Vitamin D, cognition, and dementia: A systematic review and meta-analysis. Neurology 79, 1397–1405. doi: 10.1212/WNL.0b013e31826c197f
Bastian, T., von Hohenberg, W., Mickelson, D., Lanier, L., and Georgieff, M. (2016). Iron deficiency impairs developing hippocampal neuron gene expression, energy metabolism, and dendrite complexity. Dev. Neurosci. 38, 264–276. doi: 10.1159/000448514
Beltramo, E., Berrone, E., Tarallo, S., and Porta, M. (2008). Effects of thiamine and benfotiamine on intracellular glucose metabolism and relevance in the prevention of diabetic complications. Acta Diabetol. 45, 131–141. doi: 10.1007/s00592-008-0042-y
Bender, D. (1999). Optimum nutrition: Thiamin, biotin and pantothenate. Proc. Nutr. Soc. 58, 427–433. doi: 10.1017/s0029665199000567
Betti, M., Minelli, A., Ambrogini, P., Ciuffoli, S., Viola, V., Galli, F., et al. (2011). Dietary supplementation with α-tocopherol reduces neuroinflammation and neuronal degeneration in the rat brain after kainic acid-induced status epilepticus. Free Radic. Res. 45, 1136–1142. doi: 10.3109/10715762.2011.597750
Bhadra, P., and Deb, A. (2020). A review on nutritional anemia. Indian J. Nat. Sci. 10, 18466–18474.
Brown, J., Bianco, J., McGrath, J., and Eyles, D. (2003). 1,25-dihydroxyvitamin D3 induces nerve growth factor, promotes neurite outgrowth and inhibits mitosis in embryonic rat hippocampal neurons. Neurosci. Lett. 343, 139–143. doi: 10.1016/s0304-3940(03)00303-3
Brunette, K., Tran, P., Wobken, J., Carlson, E., and Georgieff, M. (2010). Gestational and neonatal iron deficiency alters apical dendrite structure of CA1 pyramidal neurons in adult rat hippocampus. Dev. Neurosci. 32, 238–248. doi: 10.1159/000314341
Bulk, M., Abdelmoula, W., Geut, H., Wiarda, W., Ronen, I., Dijkstra, J., et al. (2020). Quantitative MRI and laser ablation-inductively coupled plasma-mass spectrometry imaging of iron in the frontal cortex of healthy controls and Alzheimer’s disease patients. Neuroimage 215:116808. doi: 10.1016/j.neuroimage.2020.116808
Bush, A., Pettingell, W., Multhaup, G., Paradis, M., Vonsattel, J. P., Gusella, J. F., et al. (1994). Rapid induction of Alzheimer A beta amyloid formation by zinc. Science 265, 1464–1467. doi: 10.1126/science.8073293
Calderón-Ospina, C., and Nava-Mesa, M. O. (2020). B Vitamins in the nervous system: Current knowledge of the biochemical modes of action and synergies of thiamine, pyridoxine, and cobalamin. CNS Neurosci. Ther. 26, 5–13. doi: 10.1111/cns.13207
Chabas, J., Alluin, O., Rao, G., Garcia, S., Lavaut, M., Risso, J., et al. (2008). Vitamin D2 potentiates axon regeneration. J. Neurotrauma 25, 1247–1256. doi: 10.1089/neu.2008.0593
Chai, B., Gao, F., Wu, R., Dong, T., Gu, C., Lin, Q., et al. (2019). Vitamin D deficiency as a risk factor for dementia and Alzheimer’s disease: An updated meta-analysis. BMC Neurol. 19:284. doi: 10.1186/s12883-019-1500-6
Chan, W., Almasieh, M., Catrinescu, M., and Levin, L. (2018). Cobalamin-associated superoxide scavenging in neuronal cells is a potential mechanism for vitamin B12-deprivation optic neuropathy. Am. J. Pathol. 188, 160–172. doi: 10.1016/j.ajpath.2017.08.032
Chen, X., Ji, B., Hao, X., Li, X., Eisele, F., Nyström, T., et al. (2020). FMN reduces Amyloid-β toxicity in yeast by regulating redox status and cellular metabolism. Nat. Commun. 11:867. doi: 10.1038/s41467-020-14525-4
Cilliers, K. (2021). Trace element alterations in Alzheimer’s disease: A review. Clin. Anat. 34, 766–773. doi: 10.1002/ca.23727
Clarke, R., Smith, A., Jobst, K., Refsum, H., Sutton, L., and Ueland, P. (1998). Folate, vitamin B12, and serum total homocysteine levels in confirmed Alzheimer disease. Arch. Neurol. 55, 1449–1455. doi: 10.1001/archneur.55.11.1449
Coimbra, C., and Junqueira, V. (2003). High doses of riboflavin and the elimination of dietary red meat promote the recovery of some motor functions in Parkinson’s disease patients. Braz. J. Med. Biol. Res. 36, 1409–1417. doi: 10.1590/s0100-879x2003001000019
da Silva, S. L., Vellas, B., Elemans, S., Luchsinger, J., Kamphuis, P., Yaffe, K., et al. (2014). Plasma nutrient status of patients with Alzheimer’s disease: Systematic review and meta-analysis. Alzheimers Dement. 10, 485–502. doi: 10.1016/j.jalz.2013.05.1771
Dakshinamurti, K., Sharma, S., and Geiger, J. (2003). Neuroprotective actions of pyridoxine. Biochim. Biophys. Acta 1647, 225–229. doi: 10.1016/s1570-9639(03)00054-2
Dakshinamurti, S., and Dakshinamurti, K. (2013). “Vitamin B6,” in Handbook of Vitamins, 5th Edn, eds J. Zempleni, J. W. Suttie, and J. F. Gregory (Boca Raton, FL: CRC Press).
De Jager, C., Oulhaj, A., Jacoby, R., Refsum, H., and Smith, A. (2012). Cognitive and clinical outcomes of homocysteine-lowering B-vitamin treatment in mild cognitive impairment: A randomized controlled trial. Int. J. Geriatr. Psychiatry 27, 592–600. doi: 10.1002/gps.2758
De Marchi, F., Saraceno, M., Sarnelli, M., Virgilio, E., Cantello, R., and Mazzini, L. (2023). Potential role of vitamin D levels in amyotrophic lateral sclerosis cognitive impairment. Neurol. Sci. 44, 2795–2802. doi: 10.1007/s10072-023-06751-7
Domitrz, I., and Cegielska, J. (2022). Magnesium as an important factor in the pathogenesis and treatment of migraine-from theory to practice. Nutrients 14:1089. doi: 10.3390/nu14051089
Dos Santos, E., Busanello, E., Miglioranza, A., Zanatta, A., Barchak, A., Vargas, C., et al. (2009). Evidence that folic acid deficiency is a major determinant of hyperhomocysteinemia in Parkinson’s disease. Metab. Brain Dis. 24, 257–269. doi: 10.1007/s11011-009-9139-4
Douaud, G., Refsum, H., de Jager, C., Jacoby, R., Nichols, T., Smith, S., et al. (2013). Preventing Alzheimer’s disease-related gray matter atrophy by B-vitamin treatment. Proc. Natl. Acad. Sci. U S A. 110, 9523–9528. doi: 10.1073/pnas.1301816110
Dursun, E., and Gezen-Ak, D. (2019). Vitamin D basis of Alzheimer’s disease: From genetics to biomarkers. Hormones 18, 7–15. doi: 10.1007/s42000-018-0086-5
Esler, W., Stimson, E., Jennings, J., Ghilardi, J., Mantyh, P., and Maggio, J. (1996). Zinc-induced aggregation of human and rat beta-amyloid peptides in vitro. J. Neurochem. 66, 723–732. doi: 10.1046/j.1471-4159.1996.66020723.x
Evans, E., Piccio, L., and Cross, A. (2018). Use of vitamins and dietary supplements by patients with multiple sclerosis: A review. JAMA Neurol. 75, 1013–1021. doi: 10.1001/jamaneurol.2018.0611
Evatt, M., DeLong, M., Kumari, M., Auinger, P., McDermott, M., Tangpricha, V., et al. (2011). High prevalence of hypovitaminosis D status in patients with early Parkinson disease. Arch. Neurol. 68, 314–319. doi: 10.1001/archneurol.2011.30
Eyles, D., Burne, T., and McGrath, J. (2013). Vitamin D, effects on brain development, adult brain function and the links between low levels of vitamin D and neuropsychiatric disease. Front. Neuroendocrinol. 34:47–64. doi: 10.1016/j.yfrne.2012.07.001
Falkowska, A., Gutowska, I., Goschorska, M., Nowacki, P., Chlubek, D., and Baranowska-Bosiacka, I. (2015). Energy metabolism of the brain, including the cooperation between astrocytes and neurons, especially in the context of glycogen metabolism. Int. J. Mol. Sci. 16, 25959–25981. doi: 10.3390/ijms161125939
Fei, H., Qian, C., Wu, X., Wei, Y., Huang, J., and Wei, L. (2022). Role of micronutrients in Alzheimer’s disease: Review of available evidence. World J. Clin. Cases 10, 7631–7641. doi: 10.12998/wjcc.v10.i22.7631
Fishman, S., Christian, P., and West, K. (2000). The role of vitamins in the prevention and control of anaemia. Public Health Nutr. 3, 125–150. doi: 10.1017/s1368980000000173
Fox, J., and Tullidge, G. (1946). Pyridoxine (vitamin B6) in epilepsy; A clinical trial. Lancet 2:345. doi: 10.1016/s0140-6736(46)90842-2
Gabriel, F., Oliveira, M., Bruna, D. M., Berk, M., Brietzke, E., Jacka, F. N., et al. (2023). Nutrition and bipolar disorder: A systematic review. Nutr. Neurosci. 26, 637–651. doi: 10.1080/1028415X.2022.2077031
García-Krauss, A., Ferrada, L., Astuya, A., Salazar, K., Cisternas, P., Martínez, F., et al. (2016). Dehydroascorbic acid promotes cell death in neurons under oxidative stress: A protective role for astrocytes. Mol. Neurobiol. 53, 5847–5863. doi: 10.1007/s12035-015-9497-3
Garcion, E., Wion-Barbot, N., Montero-Menei, C., Berger, F., and Wion, D. (2002). New clues about vitamin D functions in the nervous system. Trends Endocrinol. Metab. 13, 100–105. doi: 10.1016/s1043-2760(01)00547-1
Gasperi, V., Sibilano, M., Savini, I., and Catani, M. (2019). Niacin in the central nervous system: An update of biological aspects and clinical applications. Int. J. Mol. Sci. 20:974. doi: 10.3390/ijms20040974
Geng, M., Saito, H., and Katsuki, H. (1995). The effects of thiamine and oxythiamine on the survival of cultured brain neurons. Jpn. J. Pharmacol. 68, 349–352. doi: 10.1254/jjp.68.349
Gibson, G., Hirsch, J., Fonzetti, P., Jordan, B., Cirio, R., and Elder, J. (2016). Vitamin B1 (thiamine) and dementia. Ann. N. Y. Acad. Sci. 1367, 21–30. doi: 10.1111/nyas.13031
Gospe, S. (2002). Pyridoxine-dependent seizures: Findings from recent studies pose new questions. Pediatr. Neurol. 26, 181–185. doi: 10.1016/s0887-8994(01)00407-6
Guzek, D., Kołota, A., Lachowicz, K., Skolmowska, D., Stachoń, M., and Gła̧bska, D. (2023). Effect of vitamin D supplementation on depression in adults: A systematic review of randomized controlled trials (RCTs). Nutrients 15:951. doi: 10.3390/nu15040951
Halubiec, P., Leończyk, M., Staszewski, F., Łazarczyk, A., Jaworek, A., and Wojas-Pelc, A. (2021). Pathophysiology and clinical management of pellagra - A review. Folia Med Cracov. 61, 125–137. doi: 10.24425/fmc.2021.138956
Harrison, F., Green, R., Dawes, S., and May, J. (2010). Vitamin C distribution and retention in the mouse brain. Brain Res. 1348, 181–186. doi: 10.1016/j.brainres.2010.05.090
Hathorn, T., Snyder-Keller, A., and Messer, A. (2011). Nicotinamide improves motor deficits and upregulates PGC-1α and BDNF gene expression in a mouse model of Huntington’s disease. Neurobiol. Dis. 41, 43–50. doi: 10.1016/j.nbd.2010.08.017
Hegyi, J., Schwartz, R., and Hegyi, V. (2004). Pellagra: Dermatitis, dementia, and diarrhea. Int. J. Dermatol. 43, 1–5. doi: 10.1111/j.1365-4632.2004.01959.x
Hirsch, J., and Parrott, J. (2012). New considerations on the neuromodulatory role of thiamine. Pharmacology 89, 111–116. doi: 10.1159/000336339
Holton, K. (2021). Micronutrients may be a unique weapon against the neurotoxic triad of excitotoxicity, oxidative stress and neuroinflammation: A perspective. Front. Neurosci. 15:726457. doi: 10.3389/fnins.2021.726457
Hübel, N., Hosseini-Zare, M., Žiburkus, J., and Ullah, G. (2017). The role of glutamate in neuronal ion homeostasis: A case study of spreading depolarization. PLoS Comput. Biol. 13:e1005804. doi: 10.1371/journal.pcbi.1005804
Ibi, M., Sawada, H., Nakanishi, M., Kume, T., Katsuki, H., Kaneko, S., et al. (2001). Protective effects of 1 alpha,25-(OH)(2)D(3) against the neurotoxicity of glutamate and reactive oxygen species in mesencephalic culture. Neuropharmacology 40, 761–771. doi: 10.1016/s0028-3908(01)00009-0
Icer, M., Arslan, N., and Gezmen-Karadağ, M. (2021). Effects of vitamin E on neurodegenerative diseases: An update. Acta Neurobiol. Exp. 81, 21–33. doi: 10.21307/ane-2021-003
İmre, O., Karaağaç, M., and Caglayan, C. (2023). Does decreased Vitamin D level trigger bipolar manic attacks? Behav. Sci. 13:779. doi: 10.3390/bs13090779
Isenberg-Grzeda, E., Kutner, H., and Nicolson, S. (2012). Wernicke-Korsakoff-syndrome: Under-recognized and under-treated. Psychosomatics 53, 507–516. doi: 10.1016/j.psym.2012.04.008
Ismail, N., Kureishy, N., Church, S., Scholefield, M., Unwin, R., Xu, J., et al. (2020). Vitamin B5 (d-pantothenic acid) localizes in myelinated structures of the rat brain: Potential role for cerebral vitamin B5 stores in local myelin homeostasis. Biochem. Biophys. Res. Commun. 522, 220–225. doi: 10.1016/j.bbrc.2019.11.052
James, E., Dobson, R., Kuhle, J., Baker, D., Giovannoni, G., and Ramagopalan, S. (2013). The effect of vitamin D-related interventions on multiple sclerosis relapses: A meta-analysis. Mult. Scler. 19, 1571–1579. doi: 10.1177/1352458513489756
Kang, J., Park, M., Lee, E., Jung, J., and Kim, T. (2022). The role of Vitamin D in Alzheimer’s disease: A transcriptional regulator of amyloidopathy and gliopathy. Biomedicines 10:1824. doi: 10.3390/biomedicines10081824
Kennedy, D. O. (2016). B vitamins and the brain: Mechanisms, dose and efficacy–A review. Nutrients 8:68. doi: 10.3390/nu8020068
Kim, J., and Cho, K. (2019). Functional nutrients for epilepsy. Nutrients 11:1309. doi: 10.3390/nu11061309
Kodam, P., Sai Swaroop, R., Pradhan, S., Sivaramakrishnan, V., and Vadrevu, R. (2023). Integrated multi-omics analysis of Alzheimer’s disease shows molecular signatures associated with disease progression and potential therapeutic targets. Sci. Rep. 13:3695. doi: 10.1038/s41598-023-30892-6
Kola, A., Nencioni, F., and Valensin, D. (2023). Bioinorganic chemistry of micronutrients related to Alzheimer’s and Parkinson’s diseases. Molecules 28:5467. doi: 10.3390/molecules28145467
Krzysztoforska, K., Piechal, A., Wojnar, E., Blecharz-Klin, K., Pyrzanowska, J., Joniec-Maciejak, I., et al. (2023). Protocatechuic acid prevents some of the memory-related behavioural and neurotransmitter changes in a pyrithiamine-induced thiamine deficiency model of wernicke-korsakoff syndrome in rats. Nutrients 15:625. doi: 10.3390/nu15030625
Lahoda Brodska, H., Klempir, J., Zavora, J., and Kohout, P. (2023). The role of micronutrients in neurological disorders. Nutrients 15:4129. doi: 10.3390/nu15194129
Lauer, A., Grimm, H., Apel, B., Golobrodska, N., Kruse, L., Ratanski, E., et al. (2022). Mechanistic link between vitamin B12 and Alzheimer’s disease. Biomolecules 12:129. doi: 10.3390/biom12010129
Li, X., Huang, J., and May, J. (2003). Ascorbic acid spares alpha-tocopherol and decreases lipid peroxidation in neuronal cells. Biochem. Biophys. Res. Commun. 305, 656–661. doi: 10.1016/s0006-291x(03)00836-2
Licina, E., Radojicic, A., Jeremic, M., Tomic, A., and Mijajlovic, M. (2023). Non-pharmacological treatment of primary headaches-a focused review. Brain Sci. 13:1432. doi: 10.3390/brainsci13101432
Littlejohns, T., Henley, W., Lang, I., Annweiler, C., Beauchet, O., Chaves, P., et al. (2014). Vitamin D and the risk of dementia and Alzheimer disease. Neurology 83, 920–928. doi: 10.1212/WNL.0000000000000755
Lu’o’ng, K. V. Q., and Nguyễn, L. T. H. (2011). Role of thiamine in Alzheimer’s disease. Am. J. Alzheimer’s Dis. Other Dement. 26, 588–598.
Luo, X., Ou, R., Dutta, R., Tian, Y., Xiong, H., and Shang, H. (2018). Association between serum vitamin d levels and parkinson’s disease: A systematic review and meta-analysis. Front. Neurol. 9:909. doi: 10.3389/fneur.2018.00909
Lv, Z., Qi, H., Wang, L., Fan, X., Han, F., Wang, H., et al. (2014). Vitamin D status and Parkinson’s disease: A systematic review and meta-analysis. Neurol. Sci. 35, 1723–1730. doi: 10.1007/s10072-014-1821-6
Madsen, S., DiGiacomo, P., Zeng, Y., Goubran, M., Chen, Y., Rutt, B., et al. (2020). Correlative microscopy to localize and characterize iron deposition in Alzheimer’s disease. J. Alzheimers Dis. Rep. 4, 525–536. doi: 10.3233/ADR-200234
Maier, J., Pickering, G., Giacomoni, E., Cazzaniga, A., and Pellegrino, P. (2020). Headaches and magnesium: Mechanisms, bioavailability, therapeutic efficacy and potential advantage of magnesium pidolate. Nutrients 12:2660. doi: 10.3390/nu12092660
Mantyh, P., Ghilardi, J., Rogers, S., DeMaster, E., Allen, C., Stimson, E., et al. (1993). Aluminum, iron, and zinc ions promote aggregation of physiological concentrations of beta-amyloid peptide. J. Neurochem. 61, 1171–1174. doi: 10.1111/j.1471-4159.1993.tb03639.x
Manzanares, W., and Hardy, G. (2010). Vitamin B12: The forgotten micronutrient for critical care. Curr. Opin. Clin. Nutr. Metab. Care 13, 662–668. doi: 10.1097/MCO.0b013e32833dfaec
Manzanos, I., Martino, P., Audisio, E., and Bonet, J. (2022). Vitamin D: Between the brightness of the sun and the darkness of depression. Rev. Colomb. Psiquiatr. 51, 199–205. doi: 10.1016/j.rcpeng.2020.08.002
Marashly, E., and Bohlega, S. (2017). Riboflavin has neuroprotective potential: Focus on Parkinson’s disease and migraine. Front. Neurol. 8:333. doi: 10.3389/fneur.2017.00333
Marazziti, D., Mangiapane, P., Carbone, M., Morana, F., Arone, A., Massa, L., et al. (2023). Decreased levels of vitamin D in bipolar patients. Life 13:883. doi: 10.3390/life13040883
Martin, P. R. (2001). Molecular mechanisms of thiamine utilization. Curr. Mol. Med. 1, 197–207. doi: 10.2174/1566524013363870
Mason, S. (2017). Lactate shuttles in neuroenergetics-homeostasis, Allostasis and Beyond. Front. Neurosci. 11:43. doi: 10.3389/fnins.2017.00043
McCaddon, A. (2013). Vitamin B12 in neurology and ageing; clinical and genetic aspects. Biochimie 95, 1066–1076. doi: 10.1016/j.biochi.2012.11.017
McCaddon, A., Regland, B., Hudson, P., and Davies, G. (2002). Functional vitamin B(12) deficiency and Alzheimer disease. Neurology 58, 1395–1399. doi: 10.1212/wnl.58.9.1395
Mielech, A., Puścion-Jakubik, A., Markiewicz-Żukowska, R., and Socha, K. (2020). Vitamins in Alzheimer’s disease-review of the latest reports. Nutrients 12:3458. doi: 10.3390/nu12113458
Miller, C., and Dulay, J. (2008). The high-affinity niacin receptor HM74A is decreased in the anterior cingulate cortex of individuals with schizophrenia. Brain Res. Bull. 77, 33–41. doi: 10.1016/j.brainresbull.2008.03.015
Mitchell, E., Conus, N., and Kaput, J. (2014). B vitamin polymorphisms and behavior: Evidence of associations with neurodevelopment, depression, schizophrenia, bipolar disorder and cognitive decline. Neurosci. Biobehav. Rev. 47, 307–320. doi: 10.1016/j.neubiorev.2014.08.006
Miyaue, N., Kubo, M., and Nagai, M. (2022). Ascorbic acid can alleviate the degradation of levodopa and carbidopa induced by magnesium oxide. Brain Behav. 12:e2672. doi: 10.1002/brb3.2672
Moorthy, D., Peter, I., Scott, T., Parnell, L., Lai, C., Crott, J., et al. (2012). Status of vitamins B-12 and B-6 but not of folate, homocysteine, and the methylenetetrahydrofolate reductase C677T polymorphism are associated with impaired cognition and depression in adults. J. Nutr. 142, 1554–1560. doi: 10.3945/jn.112.161828
Moreira, E., Brasch, N., and Yun, J. (2011). Vitamin B12 protects against superoxide-induced cell injury in human aortic endothelial cells. Free Radic. Biol. Med. 51, 876–883. doi: 10.1016/j.freeradbiomed.2011.05.034
Moretti, R., and Peinkhofer, C. (2019). B vitamins and fatty acids: What do they share with small vessel disease-related dementia? Int. J. Mol. Sci. 20:5797. doi: 10.3390/ijms20225797
Moynier, F., Borgne, M., Lahoud, E., Mahan, B., Mouton-Liger, F., Hugon, J., et al. (2020). Copper and zinc isotopic excursions in the human brain affected by Alzheimer’s disease. Alzheimers Dement. 12:e12112. doi: 10.1002/dad2.12112
Munger, K., Levin, L., Hollis, B., Howard, N., and Ascherio, A. (2006). Serum 25-hydroxyvitamin D levels and risk of multiple sclerosis. JAMA 296, 2832–2838. doi: 10.1001/jama.296.23.2832
Murakami, K., Miyake, Y., Sasaki, S., Tanaka, K., Fukushima, W., Kiyohara, C., et al. (2010). Dietary intake of folate, vitamin B6, vitamin B12 and riboflavin and risk of Parkinson’s disease: A case-control study in Japan. Br. J. Nutr. 104, 757–764. doi: 10.1017/S0007114510001005
Murata, T., Dietrich, H., Horiuchi, T., Hongo, K., and Dacey, R. (2016). Mechanisms of magnesium-induced vasodilation in cerebral penetrating arterioles. Neurosci. Res. 107, 57–62. doi: 10.1016/j.neures.2015.12.005
Murdaca, G., Banchero, S., Tonacci, A., Nencioni, A., Monacelli, F., and Gangemi, S. (2021). Vitamin D and folate as predictors of MMSE in Alzheimer’s disease: A machine learning analysis. Diagnostics (Basel) 11:940. doi: 10.3390/diagnostics11060940
Nagayama, H., Hamamoto, M., Ueda, M., Nito, C., Yamaguchi, H., and Katayama, Y. (2004). The effect of ascorbic acid on the pharmacokinetics of levodopa in elderly patients with Parkinson disease. Clin. Neuropharmacol. 27, 270–273. doi: 10.1097/01.wnf.0000150865.21759.bc
Naghashpour, M., Jafarirad, S., Amani, R., Sarkaki, A., and Saedisomeolia, A. (2017). Update on riboflavin and multiple sclerosis: A systematic review. Iran. J. Basic Med. Sci. 20, 958–966. doi: 10.22038/IJBMS.2017.9257
Nambiar, N., Aiyappa, C., and Srinivasa, R. (2011). Oral riboflavin versus oral propranolol in migraine prophylaxis: An open label randomized controlled trial. Neurol. Asia 16, 223–229.
Narne, P., Pandey, V., and Phanithi, P. (2017). Interplay between mitochondrial metabolism and oxidative stress in ischemic stroke: An epigenetic connection. Mol. Cell. Neurosci. 82, 176–194. doi: 10.1016/j.mcn.2017.05.008
Nemazannikova, N., Mikkelsen, K., Stojanovska, L., Blatch, G., and Apostolopoulos, V. (2018). Is there a link between Vitamin B and multiple sclerosis? Med. Chem. 14, 170–180. doi: 10.2174/1573406413666170906123857
Neveu, I., Naveilhan, P., Jehan, F., Baudet, C., Wion, D., De Luca, H., et al. (1994). 1,25-dihydroxyvitamin D3 regulates the synthesis of nerve growth factor in primary cultures of glial cells. Brain Res. Mol. Brain Res. 24, 70–76. doi: 10.1016/0169-328x(94)90119-8
Obeid, R., McCaddon, A., and Herrmann, W. (2007). The role of hyperhomocysteinemia and B-vitamin deficiency in neurological and psychiatric diseases. Clin. Chem. Lab. Med. 45, 1590–1606. doi: 10.1515/CCLM.2007.356
Okada, K., Tanaka, H., Temporin, K., Okamoto, M., Kuroda, Y., Moritomo, H., et al. (2010). Methylcobalamin increases Erk1/2 and Akt activities through the methylation cycle and promotes nerve regeneration in a rat sciatic nerve injury model. Exp. Neurol. 222, 191–203. doi: 10.1016/j.expneurol.2009.12.017
O’Keeffe, S., Tormey, W., Glasgow, R., and Lavan, J. (1994). Thiamine deficiency in hospitalized elderly patients. Gerontology 40, 18–24. doi: 10.1159/000213570
O’Leary, F., Allman-Farinelli, M., and Samman, S. (2012). Vitamin B12 status, cognitive decline and dementia: A systematic review of prospective cohort studies. Br. J. Nutr. 108, 1948–1961. doi: 10.1017/S0007114512004175
Onaolapo, O. J., and Onaolapo, A. Y. (2023). “B vitamins: Pyridoxal phosphate and parkinsonism,” in Vitamins and Minerals in Neurological Disorders, eds C. R. Martin, V. Patel, and V. R. Preedy (Cambridge, MA: Academic Press), 527–542.
Osakada, F., Hashino, A., Kume, T., Katsuki, H., Kaneko, S., and Akaike, A. (2003). Neuroprotective effects of alpha-tocopherol on oxidative stress in rat striatal cultures. Eur. J. Pharmacol. 465, 15–22. doi: 10.1016/s0014-2999(03)01495-x
Paez-Hurtado, A. M., Cortes-Albornoz, M. C., Rodríguez-Gomez, D. A., Calderón-Ospina, C. A., and Nava-Mesa, M. O. (2023). “B vitamins on the nervous system: A focus on peripheral neuropathy,” in Vitamins and Minerals in Neurological Disorders, eds C. R. Martin, V. Patel, and V. R. Preedy (Cambridge, MA: Academic Press), 643–657.
Patassini, S., Begley, P., Xu, J., Church, S., Kureishy, N., Reid, S., et al. (2019). Cerebral Vitamin B5 (D-Pantothenic Acid) deficiency as a potential cause of metabolic perturbation and neurodegeneration in Huntington’s disease. Metabolites 9:113. doi: 10.3390/metabo9060113
Pellerin, L. (2010). Food for thought: The importance of glucose and other energy substrates for sustaining brain function under varying levels of activity. Diabetes Metab. 36, S59–S63. doi: 10.1016/S1262-3636(10)70469-9
Petridou, E., Kousoulis, A., Michelakos, T., Papathoma, P., Dessypris, N., Papadopoulos, F., et al. (2016). Folate and B12 serum levels in association with depression in the aged: A systematic review and meta-analysis. Aging Ment. Health 20, 965–973. doi: 10.1080/13607863.2015.1049115
Peyser, C., Folstein, M., Chase, G., Starkstein, S., Brandt, J., Cockrell, J., et al. (1995). Trial of d-alpha-tocopherol in Huntington’s disease. Am. J. Psychiatry 152, 1771–1775. doi: 10.1176/ajp.152.12.1771
Pierrot-Deseilligny, C. (2009). Clinical implications of a possible role of vitamin D in multiple sclerosis. J. Neurol. 256, 1468–1479. doi: 10.1007/s00415-009-5139-x
Pignolo, A., Mastrilli, S., Davì, C., Arnao, V., Aridon, P., Dos Santos Mendes, F. A., et al. (2022). Vitamin D and Parkinson’s disease. Nutrients 14:1220. doi: 10.3390/nu14061220
Plantone, D., Pardini, M., and Rinaldi, G. (2021). Riboflavin in neurological diseases: A narrative review. Clin. Drug Investig. 41, 513–527. doi: 10.1007/s40261-021-01038-1
Quadri, P., Fragiacomo, C., Pezzati, R., Zanda, E., Forloni, G., Tettamanti, M., et al. (2004). Homocysteine, folate, and vitamin B-12 in mild cognitive impairment, Alzheimer disease, and vascular dementia. Am. J. Clin. Nutr. 80, 114–122. doi: 10.1093/ajcn/80.1.114
Rai, S., Singh, P., Steinbusch, H., Vamanu, E., Ashraf, G., and Singh, M. (2021). The role of vitamins in neurodegenerative disease: An update. Biomedicines 9:1284. doi: 10.3390/biomedicines9101284
Reynolds, E. (2006). Vitamin B12, folic acid, and the nervous system. Lancet Neurol. 5, 949–960. doi: 10.1016/S1474-4422(06)70598-1
Rimmelzwaan, L., van Schoor, N., Lips, P., Berendse, H., and Eekhoff, E. (2016). Systematic review of the relationship between vitamin D and Parkinson’s disease. J. Parkinsons Dis. 6, 29–37. doi: 10.3233/JPD-150615
Rouhani, P., Amoushahi, M., Keshteli, A., Saneei, P., Afshar, H., Esmaillzadeh, A., et al. (2023). Dietary riboflavin intake in relation to psychological disorders in Iranian adults: An observational study. Sci. Rep. 13:5152. doi: 10.1038/s41598-023-32309-w
Rucker, R. B., and Bauerly, K. (2013). “Pantothenic acid,” in Handbook of Vitamins, 5th Edn, eds J. Zempleni, J. W. Suttie, and J. F. Gregory (Boca Raton, FL: CRC Press).
Salemi, G., Gueli, M., Vitale, F., Battaglieri, F., Guglielmini, E., Ragonese, P., et al. (2010). Blood lipids, homocysteine, stress factors, and vitamins in clinically stable multiple sclerosis patients. Lipids Health Dis. 9:19. doi: 10.1186/1476-511X-9-19
Sang, C., Philbert, S., Hartland, D., Unwin, R., Dowsey, A., Xu, J., et al. (2022). Coenzyme A-dependent tricarboxylic acid cycle enzymes are decreased in Alzheimer’s disease consistent with cerebral pantothenate deficiency. Front. Aging Neurosci. 14:893159. doi: 10.3389/fnagi.2022.893159
Sasanian, N., Bernson, D., Horvath, I., Wittung-Stafshede, P., and Esbjörner, E. (2020). Redox-dependent copper ion modulation of Amyloid-β (1-42) aggregation in vitro. Biomolecules 10:924. doi: 10.3390/biom10060924
Schoenen, J., Jacquy, J., and Lenaerts, M. (1998). Effectiveness of high-dose riboflavin in migraine prophylaxis. A randomized controlled trial. Neurology 50, 466–470. doi: 10.1212/wnl.50.2.466
Sechi, G., and Serra, A. (2007). Wernicke’s encephalopathy: New clinical settings and recent advances in diagnosis and management. Lancet Neurol. 6, 442–455. doi: 10.1016/S1474-4422(07)70104-7
Sechi, G., Sechi, E., Fois, C., and Kumar, N. (2016). Advances in clinical determinants and neurological manifestations of B vitamin deficiency in adults. Nutr. Rev. 74, 281–300. doi: 10.1093/nutrit/nuv107
Selhub, J., Bagley, L., Miller, J., and Rosenberg, I. H. (2000). B vitamins, homocysteine, and neurocognitive function in the elderly. Am. J. Clin. Nutr. 71, 614S–620S. doi: 10.1093/ajcn/71.2.614s
Seshadri, S., Beiser, A., Selhub, J., Jacques, P., Rosenberg, I., D’Agostino, R., et al. (2002). Plasma homocysteine as a risk factor for dementia and Alzheimer’s disease. N. Engl. J. Med. 346, 476–483. doi: 10.1056/NEJMoa011613
Singleton, C., and Martin, P. (2001). Molecular mechanisms of thiamine utilization. Curr. Mol. Med. 1, 197–207. doi: 10.2174/1566524013363870
Smith, A. (2008). The worldwide challenge of the dementias: A role for B vitamins and homocysteine? Food Nutr. Bull. 29, S143–S172. doi: 10.1177/15648265080292S119
Smith, D., Cappai, R., and Barnham, K. (2007). The redox chemistry of the Alzheimer’s disease amyloid beta peptide. Biochim. Biophys. Acta 1768, 1976–1990. doi: 10.1016/j.bbamem.2007.02.002
Smolders, J., Torkildsen, Ø, Camu, W., and Holmøy, T. (2019). An update on Vitamin D and disease activity in multiple sclerosis. CNS Drugs 33, 1187–1199. doi: 10.1007/s40263-019-00674-8
Socha, K., Klimiuk, K., Naliwajko, S., Soroczyńska, J., Puścion-Jakubik, A., Markiewicz-Żukowska, R., et al. (2021). Dietary habits, selenium, copper, zinc and total antioxidant status in serum in relation to cognitive functions of patients with Alzheimer’s disease. Nutrients 13:287. doi: 10.3390/nu13020287
Sommer, I., Griebler, U., Kien, C., Auer, S., Klerings, I., Hammer, R., et al. (2017). Vitamin D deficiency as a risk factor for dementia: A systematic review and meta-analysis. BMC Geriatr. 17:16. doi: 10.1186/s12877-016-0405-0
Sorolla, M., Rodríguez-Colman, M., Tamarit, J., Ortega, Z., Lucas, J., Ferrer, I., et al. (2010). Protein oxidation in Huntington disease affects energy production and vitamin B6 metabolism. Free Radic. Biol. Med. 49, 612–621. doi: 10.1016/j.freeradbiomed.2010.05.016
Späth, Z., Tmava-Berisha, A., Fellendorf, F., Stross, T., Maget, A., Platzer, M., et al. (2023). Vitamin D status in bipolar disorder. Nutrients 15:4752. doi: 10.3390/nu15224752
Spector, R., and Johanson, C. (2007). Vitamin transport and homeostasis in mammalian brain: Focus on Vitamins B and E. J. Neurochem. 103, 425–438. doi: 10.1111/j.1471-4159.2007.04773.x
Spinneker, A., Sola, R., Lemmen, V., Castillo, M., Pietrzik, K., and González-Gross, M. (2007). Vitamin B6 status, deficiency and its consequences–an overview. Nutr. Hosp. 22, 7–24.
Sudha, K., Rao, A., Rao, S., and Rao, A. (2003). Free radical toxicity and antioxidants in Parkinson’s disease. Neurol. India 51, 60–62.
Tardy, A., Pouteau, E., Marquez, D., Yilmaz, C., and Scholey, A. (2020). Vitamins and minerals for energy, fatigue and cognition: A narrative review of the biochemical and clinical evidence. Nutrients 12:228. doi: 10.3390/nu12010228
Thompson, D., and Saluja, H. (2017). Prophylaxis of migraine headaches with riboflavin: A systematic review. J. Clin. Pharm. Ther. 42, 394–403. doi: 10.1111/jcpt.12548
Tomé, A., Feng, D., and Freitas, R. (2010). The effects of alpha-tocopherol on hippocampal oxidative stress prior to in pilocarpine-induced seizures. Neurochem. Res. 35, 580–587. doi: 10.1007/s11064-009-0102-x
Vaur, P., Brugg, B., Mericskay, M., Li, Z., Schmidt, M., Vivien, D., et al. (2017). Nicotinamide riboside, a form of vitamin B3, protects against excitotoxicity-induced axonal degeneration. FASEB J. 31, 5440–5452. doi: 10.1096/fj.201700221RR
Vicente-Zurdo, D., Romero-Sánchez, I., Rosales-Conrado, N., León-González, M., and Madrid, Y. (2020). Ability of selenium species to inhibit metal-induced Aβ aggregation involved in the development of Alzheimer’s disease. Anal. Bioanal. Chem. 412, 6485–6497. doi: 10.1007/s00216-020-02644-2
Vogel, T., Dali-Youcef, N., Kaltenbach, G., and Andrès, E. (2009). Homocysteine, vitamin B12, folate and cognitive functions: A systematic and critical review of the literature. Int. J. Clin. Pract. 63, 1061–1067. doi: 10.1111/j.1742-1241.2009.02026.x
Wakade, C., and Chong, R. (2014). A novel treatment target for Parkinson’s disease. J. Neurol. Sci. 347, 34–38. doi: 10.1016/j.jns.2014.10.024
Wakade, C., Chong, R., Bradley, E., and Morgan, J. (2015). Low-dose niacin supplementation modulates GPR109A, niacin index and ameliorates Parkinson’s disease symptoms without side effects. Clin. Case Rep. 3, 635–637. doi: 10.1002/ccr3.232
Wang, T., Xu, S. F., Fan, Y. G., Li, L. B., and Guo, C. (2019). Iron pathophysiology in Alzheimer’s diseases. Brain Iron Metab. CNS Dis. 1173, 67–104. doi: 10.1007/978-981-13-9589-5_5
Watt, N., Whitehouse, I., and Hooper, N. (2011). The role of zinc in Alzheimer’s disease. Int J Alzheimers Dis. 2011:971021. doi: 10.4061/2011/971021
Wendołowicz, A., Stefańska, E., and Ostrowska, L. (2018). Influence of selected dietary components on the functioning of the human nervous system. Rocz. Panstw. Zakl Hig. 69, 15–21.
Xie, Y., Feng, H., Peng, S., Xiao, J., and Zhang, J. (2017). Association of plasma homocysteine, vitamin B12 and folate levels with cognitive function in Parkinson’s disease: A meta-analysis. Neurosci. Lett. 636, 190–195. doi: 10.1016/j.neulet.2016.11.007
Xu, J., Patassini, S., Begley, P., Church, S., Waldvogel, H., Faull, R., et al. (2020). Cerebral deficiency of vitamin B5 (d-pantothenic acid; pantothenate) as a potentially-reversible cause of neurodegeneration and dementia in sporadic Alzheimer’s disease. Biochem. Biophys. Res. Commun. 527, 676–681. doi: 10.1016/j.bbrc.2020.05.015
Yamanaka, G., Suzuki, S., Morishita, N., Takeshita, M., Kanou, K., Takamatsu, T., et al. (2021). Experimental and clinical evidence of the effectiveness of riboflavin on migraines. Nutrients 13:2612. doi: 10.3390/nu13082612
Zhang, C., Wei, W., Liu, Y., Peng, J., Tian, Q., Liu, G., et al. (2009). Hyperhomocysteinemia increases beta-amyloid by enhancing expression of gamma-secretase and phosphorylation of amyloid precursor protein in rat brain. Am. J. Pathol. 174, 1481–1491. doi: 10.2353/ajpath.2009.081036
Zhao, R., Wang, H., Qiao, C., and Zhao, K. (2018). Vitamin B2 blocks development of Alzheimer’s disease in APP/PS1 transgenic mice via anti-oxidative mechanism. Trop. J. Pharm. Res. 17, 1049–1054. doi: 10.4314/tjpr.v17i6.10
Zhou, D., Sun, Y., Qian, Z., Wang, Z., Zhang, D., Li, Z., et al. (2023). Long-term dietary folic acid supplementation attenuated aging-induced hippocampus atrophy and promoted glucose uptake in 25-month-old rats with cognitive decline. J. Nutr. Biochem. 117:109328. doi: 10.1016/j.jnutbio.2023.109328
Keywords: vitamins, neuroenergetics, brain, energy metabolism, neurons, neurodegenerative disease
Citation: Mason S (2025) Say hello to my little friend… micronutraceuticals in neuroenergetics, neuronal health, and neurodegenerative diseases. Front. Neurosci. 19:1498655. doi: 10.3389/fnins.2025.1498655
Received: 19 September 2024; Accepted: 01 April 2025;
Published: 23 April 2025.
Edited by:
Avital Schurr, University of Louisville, United StatesReviewed by:
Nafisa M. Jadavji, University of Arizona, United StatesJacqueline P. Robbins, University of Oxford, United Kingdom
Copyright © 2025 Mason. This is an open-access article distributed under the terms of the Creative Commons Attribution License (CC BY). The use, distribution or reproduction in other forums is permitted, provided the original author(s) and the copyright owner(s) are credited and that the original publication in this journal is cited, in accordance with accepted academic practice. No use, distribution or reproduction is permitted which does not comply with these terms.
*Correspondence: Shayne Mason, bm1yLm53dUBnbWFpbC5jb20=