- 1Department of Medical Physics, School of Medicine, Ahvaz Jundishapur University of Medical Sciences, Ahvaz, Iran
- 2Cellular and Molecular Research Center, Ahvaz Jundishapur University of Medical Sciences, Ahvaz, Iran
Background and Objective: Recent studies have shown the potential of electroporation (EP) as a physical radiosensitizer for ionizing radiation (IR). The amount of sensitizing effect depends on some factors the most important of them is the time interval between the EP and IR. This experimental in vitro study aims to investigate the radiosensitizing effect of EP exposure prior to IR and also evaluate the effects of EP-IR time intervals on the amount of radiosensitizing effects.
Methods: Chinese hamster ovary (CHO) cell lines were cultured in vitro. The cells were divided into 10 groups including one untreated or control group, IR, and EP treatment alone groups, and seven combined EP-IR groups with 10, 20, 30, 40, 50, 60, and 70 min intervals. The dose enhancement factors (DEFs) for 6 MV X-rays IR were comparatively investigated between the groups using MTT assay.
Results: The EP significantly induced radiosensitizing effect and its amount depends on the time intervals. The viability rate of the cells in the combined EP-IR treatment groups for intervals of 10, 20, 30, 40, and 50 min was significantly lower than the IR alone group. The highest DEF (1.18) was observed 10 min time interval between EP and IR.
Conclusion: The radiosensitizing effects of EP persist long enough, 10–50 min, which allows safe application of EP as a radiosensitizer before IR in clinical setting.
Introduction
Cancer is the leading cause of human mortality worldwide (1). Radiation therapy (RT) is an important modality of cancer treatment (2, 3). In this method, the growth of tumor cells is controlled by bombardment with ionizing radiation, causing DNA damage by direct action or through formation of free radicals by indirect action (4, 5). However, RT can also induce unwanted damages to the normal surrounding tissues due to little discrimination between malignant and normal cells. In addition, the locoregional tumor progression following RT increases the mortality rates of this technique. Therefore, developing new improvements addressing these issues is necessary. Developing targeted drug delivery techniques along with efficient radiosensitizing agents can efficiently address these issues through enhancing targeted uptake of the anticancer agents into tumor cells and selective sensitizing tumor cells to ionizing radiation (IR) (6, 7). Electroporation (EP) is one of these modalities with promising outcomes as both targeted drug delivery system and radiosensitizing technique (8). EP increases uptake of antitumor agents in the tumor cells and their intracellular accumulation which result in radiosensitizing effects (9, 10). EP applies a single or repeated high voltage, short-duration electric pulse over the target cells inducing transient pores across membrane which in turn significantly increases the cell membrane permeability to ions and macromolecules (11–14). The induced pores are created rapidly approximately within 10−6 s and disappear within few seconds to several minutes after exposure to the electric field (12, 13).
EP is a highly effective technique to increase the cell membrane permeability by application of high voltage, short-duration electric pulses (9, 10). Indeed, when the cell membrane is exposed to an external electric field, a transmembrane potential is induced (12, 13, 15, 16). If the induced transmembrane potential is sufficiently high, dielectric breakdown leading to generate a phase transition that causes nano or micro-pores to form in lipid bilayers membrane (17, 18). During this step, the conductivity of membrane is increased rapidly (19). Therefore, the intracellular electric field increases, which may induce biochemical changes inside of the cell (20).
Some studies have shown EP, alone or in combination with other modalities, can induce radiosensitizing effects in different cancerous cells which consequently reduce the required total absorbed dose in radiotherapy (6, 7, 21).
Gabriel et al have reported that when the cells are exposed to electric pulses, the reactive oxygen species (ROS) is generated in the electropermeabilized part of the cell membrane. ROS can sensitize the cells to ionization radiation (22). Therefore, the radiation dose in the site of tumor can be selectively amplified by using EP prior to irradiation. Different studies have shown the radiosensitizing effects of EP, however, it is not clear how long do the effects persist. In this study we try to answer this question. The present study aims to investigate the effect of time intervals between EP and IR on the amount of radiosensitizing effects in vitro. In addition, in context of this study, we try to discuss the findings of our recent studies as well as current literature on the radiosensitizing effects and probable mechanisms of EP alone and in combination with other modalities in different cell lines. To the best of our knowledge this is the first time that the effects of time interval in different intervals (10–70 min) between EP and IR has been shown.
Methods
Cell Culture
Chinese hamster ovary (CHO) cell lines were purchased from National Cell Bank of Pasteur Institute of Iran (NCBI, C111). The cells were grown in the Roswell park memorial institute (RPMI) 1640 medium (Bio-Idea, Iran) supplemented with 10% Fetal bovine serum (FBS; Gibco), %1 penicillin/streptomycin (Bio-Idea, Iran). Cells were routinely subcultured twice a week using 0.25% trypsin-EDTA (Bio-Idea, Iran) and were maintained in T-75 flasks in CO2 incubator (RS Biotech Galaxy R) under standard condition (37°C, 5% CO2).
Electroporation Protocol
The cells from the exponential growth phase were harvested from monolayer cultures using trypsin-EDTA. Following the trypsination stage, the cells were centrifuged for 5 min at 1,500 rpm (Centrifuge Hettich, Protofix 32 A) and resuspended in RPMI 1640 medium at a concentration of 1 × 106 cells/ml. Finally, 30 μl cell suspension was added into Bio-Rad cuvettes with a 1-mm gap. The sample was exposed to one square electric pulse with electric field intensity 1200 V/cm and pulse duration 100 μs. EP of CHO cell line was carried out using a Bio-Rad Gene Pulser Xcell™ Electroporation system. After completion of delivering pulse, the sample was transferred to 96-well plate and the fresh medium was added to cells. In EP alone group, the 96-well plate was incubated for 24 h and then harvested for analysis. In contrast, in the EP+ IR groups, the 96-well plate was irradiated after different time intervals and then incubated for 24 h.
Ionizing Radiation of Sample
The IR treatments were carried out using 6 MV X-rays through Varian 2100 C/D linear accelerator (LINAC, Golestan Hospital, Ahvaz, Iran). The samples received a total dose of 2 Gy with a field size of 10 × 10 cm2 at source-to-surface distance (SSD) of 100 cm. To produce appropriate build up for the 6 MV beam, a Plexiglass sheet (water equivalent) with 1.5 cm thickness was placed on the top of the 96-well plate. In addition, to reach a sufficient generation of backscatter, 3 cm thickness of a Plexiglass sheet was utilized under the bottom of 96-well plate.
Experimental Groups
To determine whether EP can sensitize the cells to mega voltage IR and to obtain the optimal time interval between EP and irradiation, 10 experimental groups were designed in this study: untreated tumors, tumors treated with IR or EP alone, and seven groups with time intervals irradiated after 10, 20, 30, 40, 50, 60, and 70 min after EP (EP + IR). All of the measurements in the experimental groups were performed at least three times to increase the reliability and accuracy of the results and mean values were used for further analyses. The repetitions were biologically in which the experiments were performed in different dates from different cell cultures and resulting cell suspensions and the values were averaged.
MTT Assay and Determination of Cell Viability
The viability rate of cells in different groups as an indicator of cell response to irradiation was evaluated by MTT assay kit (Bio-Idea, Iran). In this colorimetric method, the mitochondrial dehydrogenase activity of proliferating cells reduce the MTT salt (3-[4,5-dimethylthiazol-2-yl]-2,5-diphenyltetrazolium bromide) to purple MTT formazan crystals. Therefore, upon the completion of treatments, cells were incubated 24 h at 37°C with 5% CO2 in atmosphere. Twenty four hours later, according to manufacturer's instructions, the culture medium was removed and 10 μl MTT solution (5 mg/ml) and 100 μl ready to use RPMI1640 culture media (without phenol red) were added to each well and plate was incubated for an additional 4 h. Then MTT was replaced by 50 μl dimethyl sulfoxide (DMSO) solution and the plate was shaken for 10 min by orbital shaker. The optical density (OD) of each well was assessed using spectrophotometer (Model 680, BIO-RAD) at a 570-nm test wavelength. The experiments were performed in triplicate, thus we used the average OD to obtain cell viability rate. This factor for each group was calculated the following formula: viability rate = (average OD570nm of treated group/average OD570nm of the untreated group) × 100%. In addition, the dose enhancement factor (DEF) was obtained by dividing the viability rate of EP + IR group to IR group.
Statistical Analysis
The normality of data was assessed by Kolmogorov-Smirnov test. The statistical differences between experimental groups were carried out by one-way ANOVA. If differences were significant, the post hoc test was applied to compare groups. All statistical analyses were performed with SigmaStat statistical software (Systat Software, Inc) and p-values ≤ 0.05 were considered statistically significant. The values are represented as Mean ± standard error of mean (SEM).
Results
The effect of time interval between EP and IR on radiosensitizing effect of EP was investigated in CHO cell lines. As shown in Figure 1 and Table 1, the viability rate decreased from 99.99% in untreated group to 76.73% in the cells treated with IR alone. When the cells were exposed to electric pulse alone, the viability rate reached 99.32% and had no significant difference with the untreated group (P = 0.976). However, combination of EP with IR increased the response of cells to treatment which was statistically significant. When EP was performed 10 min prior to irradiation, the lowest viability (65.10%) was observed (P = 0.0001).
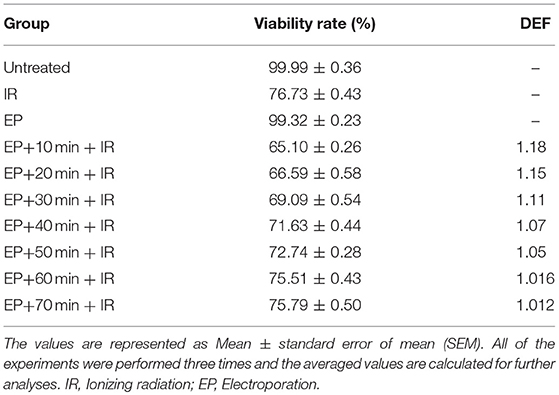
Table 1. Comparison of viability rates and dose enhancement factors of the CHO cells between the different experimental groups.
The dose enhancement factor (DEF) in this group was 1.18. By exposing of electric pulse 20 min before to irradiation, the survival rate, and DEF reached to 66.59% and 1.15, respectively. There was no significant difference between time interval of 10 and 20 min in the viability rate and DEF (P = 0.340). When the time interval was 30 min, the viability rate and DEF of 69.09% and 1.11 were achieved. By increasing the time interval from 40 to 50 min, survival was increased from 71.63 to 72.74% and DEF was decreased from 1.07 to 1.05. Treatment of cells with EP 60 min and 70 min prior to IR resulted in 75.51 and 75.79% viability rate, respectively that was similar to viability rate in the group received irradiation alone (P = 0.593 and P = 0.846, respectively). It seems, after 50 min of delivering electric pulse, the radiosensitizing effects induced by EP decreased to insignificant levels. Therefore, to obtain the highest cell response, it is essential to deliver electric pulse 10–50 min before irradiation.
Discussion
This study investigated the radiosensitizing effects of a single 100 μs pulse of EP to 6 MV X-rays IR in CHO cell lines in vitro. Moreover, the effects of time intervals between EP and IR on the amount of radiosensitizing effect were studied. Our findings showed that EP significantly increased the response rate in the CHO cells in vitro in a time dependent manner where the highest amount of radiosensitizing effect with DEF of 1.18 was observed for 10 min EP-IR interval that was significantly higher than IR alone group. This difference in the viability rate of the cells between the combined EP-IR and IR alone group remained significant for intervals of 20, 30, 40, and 50 min and then decreased. Our findings showed EP can be used 10 to 50 min before IR to induce significant radiosensitizing effects.
In normal physiological conditions, the electric conductivity of cytoplasm and extracellular medium is greater than the conductivity of the cell membrane. Therefore, under the exposure of an external electric field to the lipid membrane, the anode- and cathode-facing side, respectively becomes hyper-polarized and depolarized and a transmembrane potential is induced on the exposed cell (19, 23, 24). Reversible EP is the biophysical process that transiently increases the permeability of cell membrane through the application of short, intense electric pulses (25–27). EP is routinely employed to transport nonpermeant molecules such as DNA, dyes, proteins, and chemotherapeutic agents into the cell (28–30). This technique can modulate the intrinsic functional characterization of the target cell and increase the oxidative burst within the cell medium. Previously, this phenomenon has been observed in neutrophil (31), macrophage (32), and lymphocyte (33) cells. Gabriel et al reported that EP can induce oxidative jump and generate reactive-oxygen species (ROS) in CHO cells (22). The electro-induced oxidative jump can be appeared when the applied electric field intensity is higher than critical threshold value that is controlled by duration of pulse. To simultaneously trigger the electropermeabilization and electro-induced ROS production, this critical value is 0.44 V/cm (34). The electro-induced ROS generation is not homogenous and restricted to the electropermeabilized part of the cell membrane (35). Therefore, this technique has a potential to be combined by IR to increase lethal damage of irradiation. The efficiency of this combined modality (electro-radiotherapy) is determined by quality of radiation, electric pulse parameters, and time interval between EP and radiotherapy. In this present study, we focused on the effect of time interval between EP and radiotherapy. Our data are in agreement with previous studies investigating the radiosensitizing effects of EP alone or in combination with other modalities. West et al. (36) were probably the first group to report the interaction between EP and lethal damages of radiation. They investigated the effects of EP on the viability rate of the CHO cells in vitro at three time intervals between EP and Cs137- γ-radiation: immediately, 1 and 24 h. They reported that application of one exponential decaying electric pulse immediately prior to irradiation increased both α and β parameters of survival curve and the DEF to 1.19. However, EP applied 1 or 24 h before irradiation had no sensitizing effect on CHO cell line. Our study confirmed these results and also revealed that the optimal time interval to deliver electric pulse prior to radiotherapy is 10–50 min and applying electric pulse 60–70 min before radiotherapy has no effect on output of radiation treatment. Moreover, in our study EP could enhance the effect of radiation by factor of 1.18 (for time interval of 10 min). The findings of the Sersa group (21) revealed that EP can improve the effect of 220 KV radiation in tumor-bearing animals. The main purpose of their work was to investigate whether EP as a drug delivery system can increase the radiosensitizing effect of cisplatin as a chemotherapeutic drug, but they also observed that delivering of electric pulses prior to irradiation even in the absence of cisplatin, could enhance the response of cell to radiation. Kranjc et al reported the similar results where irradiation of LPB sarcoma cells that pretreated by electric pulses (without drug) enhanced cytotoxicity of radiation (EF = 1.25) (37).
The radiosensitizing effects of EP are not limited to CHO cell lines. Some studies have reported the radiosensitizing effects of EP in HT-29 cells which are highly radioresistant (7). In other in vitro study conducted by our group, we investigated the radiosensitizing effects EP, gold nanoparticles (GNPs) alone and combined EP-GNPs in the HT-29 and CHO cell lines in different conditions (7). Our results showed that EP could sensitize both HT-29 and CHO cell lines to 6 MV X-ray with a DEF of 1.36 and 1.28, respectively (1). The findings showed that EP and GNPs alone, and combined EP-GNPs significantly enhanced the response of cells to irradiation. Moreover, combined EP-GNPs showed synergistic radiosensitizing effect. However, the synergistic effect was observed just for HT-29 tumor cell lines. In other study by our group, we observed that the survival fraction of the HT-29 cells was significantly decreased by EP prior to radiotherapy. A single electric pulse of 100 μs increased the sensitivity of colorectal HT-29 cancer cell to megavoltage radiation by a factor of 1.36. The LD50 was decreased from 3.97 Gy in radiation alone group to 2.9 Gy in tumors treated with EP before irradiation which resulted in the sensitizer enhancement ratio of 1.36 (38).
Radiosensitizing effects of EP have been reported for different IR energies and also different types of the radiations ranging orthovoltage KV to MV X-rays. In this regard, West et al. have reported the radiosensitizing effects of EP to γ-radiation of the radioisotope sources of Cs137 (36) and Shil et al. to the γ-radiation of the radioisotope of Co60 (8). Moreover, the radiosensitizing effects of EP in lower energies of orthovoltage X-rays of 100-500 KV have been reported (21, 39, 40). However, nowadays, radioisotope sources and orthovoltage unit are gradually replaced by linear accelerators thus we used a LINAC as a radiation source to generate mega-voltage X-ray. That can be used to treatment of deep tumors.
Regarding the mechanisms of actions of radiosensitizing effects of EP, different studies have been conducted. Generation of oxidative jump at the electroporated sites of membrane and production of ROS is the probable mechanism of EP radiosensitization (34, 35). The level of generated ROS after EP was measured by Shil et al. in which they reported that the ROS level under the combined EP-irradiation group was significantly higher than irradiation alone group (8). Shil et al demonstrated that EP can significantly increase the generated ROS level in the Ehrlich Ascites Carcinoma (EAC) cells. In addition, for in vivo experiments, on the 7th day after treatment, the average tumor volume of electro-radiotherapy group was significantly (51%) smaller than this volume in control group (8).
Conclusion
In conclusion, the results of present study demonstrate that the best time of EP to induce radiosensitizing effect to megavoltage irradiation, is 10 min before IR. This radiosensitizing effect is lost after 60 min. In view of clinical applications, the 10–50 min is long enough to apply EP as a radiosensitizing approach to IR to increase the response rate in cancer treatment. However, further studies in different cell lines and also in vivo conditions are needed to reach a definitive conclusion and using the EP in clinical setting.
Author Contributions
ZR, AY, and VB contributed equally in notion, study design and implementing, and data collection and manuscript preparation.
Conflict of Interest Statement
The authors declare that the research was conducted in the absence of any commercial or financial relationships that could be construed as a potential conflict of interest.
Acknowledgments
We would like to thank the dean and staff of the Cellular and Molecular Research Center, Ahvaz Jundishapur University of Medical Sciences, Ahvaz, Iran for their support in conducting of this study. Authors also would like to thank Dr. M. J. Tahmasebi for his assistance in arranging for the use of LINAC facility in Golestan Hospital, Ahvaz, Iran.
References
1. Siegel RL, Miller KD, Jemal A. Cancer statistics, 2018. CA Cancer J Clin. (2018) 68:7–30. doi: 10.3322/caac.21442
2. Khan FM. The Physics of Radiation Therapy. Baltimore, MD; Philadelphia, PA: Lippincott Williams & Wilkins (2014).
3. Podgorsak ED. Radiation Oncology Physics : a Handbook for Teachers and Students. Vienna: International Atomic Energy Agency (2005).
4. Hall EJ, Giaccia AJ. Radiobiology for the Radiologist. Philadelphia, PA: Lippincott Williams & Wilkins (2006).
5. Dowd SB, Tilson ER. Practical Radiation Protection and Applied Radiobiology. London: W. B. Saunders (1999).
6. Garcia PA, Pancotto T, Rossmeisl JH Jr, Henao-Guerrero N, Gustafson NR, Daniel GB, et al. Non-thermal irreversible electroporation (N-TIRE) and adjuvant fractionated radiotherapeutic multimodal therapy for intracranial malignant glioma in a canine patient. Technol Cancer Res Treat. (2011) 10:73–83. doi: 10.7785/tcrt.2012.500181
7. Rezaee Z, Yadollahpour A, Bayati V, Dehbashi FN. Gold nanoparticles and electroporation impose both separate and synergistic radiosensitizing effects in HT-29 tumor cells: an in vitro study. Int J Nanomedicine. (2017) 12:1431–9. doi: 10.2147/IJN.S128996
8. Shil P, Sanghvi SH, Vidyasagar PB, Mishra KP. Enhancement of radiation cytotoxicity in murine cancer cells by electroporation: in vitro and in vivo studies. J Environ Pathol Toxicol Oncol. (2005) 24:291–8. doi: 10.1615/jenvironpatholtoxicoloncol.v24.i4.60
9. Ibey BL, Mixon DG, Payne JA, Bowman A, Sickendick K, Wilmink GJ, et al. Plasma membrane permeabilization by trains of ultrashort electric pulses. Bioelectrochemistry (2010) 79:114–21. doi: 10.1016/j.bioelechem.2010.01.001
10. Ibey BL, Pakhomov AG, Gregory BW, Khorokhorina VA, Roth CC, Rassokhin MA, et al. Selective cytotoxicity of intense nanosecond-duration electric pulses in mammalian cells. Biochim Biophys Acta - Gen Subj. (2010) 1800:1210–9. doi: 10.1016/j.bbagen.2010.07.008
11. Giardino R, Fini M, Bonazzi V, Cadossi R, Nicolini A, Carpi A. Electrochemotherapy a novel approach to the treatment of metastatic nodules on the skin and subcutaneous tissues. Biomed Pharmacother. (2006) 60:458–62. doi: 10.1016/j.biopha.2006.07.016
12. Neumann E, Sowers AE, Jordan CA. Electroporation and Electrofusion in Cell Biology. Boston, MA: Springer (1989).
13. Tsong TY. Electroporation of cell membranes. In: Neumann E, Sowers AE, Jordan CA, editors. Electroporation and Electrofusion in Cell Biology. Boston, MA: Springer (1989). p. 149–63. doi: 10.1007/978-1-4899-2528-2_9
14. Weaver JC, Chizmadzhev YA. Theory of electroporation: a review. Bioelectrochemistry Bioenerg. (1996) 41:135–60. doi: 10.1016/S0302-4598(96)05062-3
15. Weaver JC, Powell KT. Theory of electroporation. In: Neumann E, Sowers AE, Jordan CA, editors. Electroporation and Electrofusion in Cell Biology. Boston, MA: Springer (1989). p. 111–26. doi: 10.1007/978-1-4899-2528-2_7
16. Yadollahpour A, Rezaee Z. Electroporation as a new cancer treatment technique: a review on the mechanisms of action. Biomed Pharmacol J. (2014) 7:53–62. doi: 10.13005/bpj/452
17. Gaynor PT, Bodger PS. Ionisation of dielectric spheroid membranes: a balloon model of electroporation of biological cells. IEE Proc Sci Meas Technol. (1994) 141:190–6.
18. Partenskii Jordan. Negative capacitance and instability at electrified interfaces: lessons from the study of membrane capacitors. Condens Matter Phys. (2005) 8:397. doi: 10.5488/CMP.8.2.397
19. Weaver JC. Electroporation of biological membranes from multicellular to nano scales. IEEE Trans Dielectr Electr Insul. (2003) 10:754–68. doi: 10.1109/TDEI.2003.1237325
20. Prausnitz MR, Bose VG, Langer R, Weaver JC. Electroporation of mammalian skin: a mechanism to enhance transdermal drug delivery. Proc Natl Acad Sci USA. (1993) 90:10504–8. (Accessed 19 Jul 2018).
21. Serša G, Kranjc S, CemaŽar M. Improvement of combined modality therapy with cisplatin and radiation using electroporation of tumors. Int J Radiat Oncol. (2000) 46:1037–41. doi: 10.1016/S0360-3016(99)00464-2
22. Gabriel B, Teissie J. Generation of reactive-oxygen species induced by electropermeabilization of Chinese hamster ovary cells and their consequence on cell viability. Eur J Biochem. (1994) 223:25–33. doi: 10.1111/j.1432-1033.1994.tb18962.x
23. Haltiwanger S. Why electroporation is a useful technique for cancer treatments. Electroporation-Based Ther Cancer From Basics to Clin Appl. (2014) 3:103–25. doi: 10.1533/9781908818294.103
24. Weaver JC. Molecular basis for cell membrane electroporation. Ann NY Acad Sci. (1994) 720:141–52. (Accessed 19 Jul 2018).
25. Kee ST, Gehl J, Lee EW editors. Clinical Aspects of Electroporation. New York, NY: Springer (2011). doi: 10.1007/978-1-4419-8363-3
26. Orlowski S, Belehradek J, Paoletti C, Mir LM. Transient electropermeabilization of cells in culture. Increase of the cytotoxicity of anticancer drugs. Biochem Pharmacol. (1988) 37:4727–33. (Accessed 20 Jul 2018).
27. Salimi E. Nanosecond Pulse Electroporation of Biological Cells: the Effect of Membrane Dielectric Relaxation. (2011). Available online at: https://mspace.lib.umanitoba.ca/handle/1993/4460 (Accessed 20 Jul 2018).
28. Quaglino P, Mortera C, Osella-Abate S, Barberis M, Illengo M, Rissone M, et al. Electrochemotherapy with intravenous bleomycin in the local treatment of skin melanoma metastases. Ann Surg Oncol. (2008) 15:2215–22. doi: 10.1245/s10434-008-9976-0
29. Widera G, Austin M, Rabussay D, Goldbeck C, Barnett SW, Chen M, et al. Increased DNA vaccine delivery and immunogenicity by electroporation in vivo. J Immunol. (2000) 164:4635–40. doi: 10.4049/jimmunol.164.9.4635
30. Zhang G-H, Tan X-F, Shen D, Zhao S-Y, Shi Y-L, Jin C-K, et al. Gene expression and antitumor effect following im electroporation delivery of human interferon alpha 2 gene. Acta Pharmacol Sin. (2003) 24:891–6. (Accessed 20 Jul 2018).
31. Malinin VS, Sharov VS, Putvinsky AV, Osipov AN, Vladimirov YA. Chemiluminescent reactions of phagocytes induced by electroporation: the role of Ca2+ and Mg2+ ions. Bioelectrochemistry Bioenerg. (1989) 22:37–44. doi: 10.1016/0302-4598(89)85028-7
32. Annaberdyeva EM, Polnikov IG, Puchkova TV, Sharov VS, Putvinskii AV. [Induction of chemiluminescence of peritoneal exudate macrophages after exposure to an electric field]. Biulleten'eksperimental'noi Biol i meditsiny. (1987) 103:452–3.
33. Smith GK, Cleary SF. Effects of pulsed electric fields on mouse spleen lymphocytes in vitro. Biochim Biophys Acta - Mol Cell Res. (1983) 763:325–31. doi: 10.1016/0167-4889(83)90093-9
34. Bonnafous P, Vernhes M-C, Teissié J, Gabriel B. The generation of reactive-oxygen species associated with long-lasting pulse-induced electropermeabilisation of mammalian cells is based on a non-destructive alteration of the plasma membrane. Biochim Biophys Acta - Biomembr. (1999) 1461:123–34. doi: 10.1016/S0005-2736(99)00154-6
35. Gabriel B, Teissie J. Spatial compartmentation and time resolution of photooxidation of a cell membrane probe in electropermeabilized chinese hamster ovary cells. Eur J Biochem. (1995) 228:710–8. doi: 10.1111/j.1432-1033.1995.tb20314.x
36. West CML. A potential pitfall in the use of electroporation: cellular radiosensitization by pulsed high-voltage electric fields. Int J Radiat Biol. (1992) 61:329–34. doi: 10.1080/09553009214551011
37. Kranjc S, Cemazar M, Grosel A, Sentjurc M, Sersa G. Radiosensitising effect of electrochemotherapy with bleomycin in LPB sarcoma cells and tumors in mice. BMC Cancer (2005) 5:115. doi: 10.1186/1471-2407-5-115
38. Yadollahpour A, Rezaee Z, Bayati V, Tahmasebi Birgani MJ, Negad Dehbashi F. Radiotherapy enhancement with electroporation in human intestinal colon cancer HT-29 cells. Asian Pacific J Cancer Prev. (2018) 19:1259–62. doi: 10.22034/APJCP.2018.19.5.1259
39. Kranjc S, Tevz G, Kamensek U, Vidic S, Cemazar M, Sersa G. Radiosensitizing effect of electrochemotherapy in a fractionated radiation regimen in radiosensitive murine sarcoma and radioresistant adenocarcinoma tumor model. Radiat Res. (2009) 172:677–85. doi: 10.1667/RR1873.1
Keywords: electroporation, ionizing radiation, radiosensitizing effect, time interval, dose enhancement factor
Citation: Rezaee Z, Yadollahpour A and Bayati V (2018) Single Intense Microsecond Electric Pulse Induces Radiosensitization to Ionizing Radiation: Effects of Time Intervals Between Electric Pulse and Ionizing Irradiation. Front. Oncol. 8:418. doi: 10.3389/fonc.2018.00418
Received: 20 July 2018; Accepted: 10 September 2018;
Published: 27 September 2018.
Edited by:
Charles A. Kunos, National Cancer Institute (NIH), United StatesReviewed by:
Valdir Carlos Colussi, University Hospitals Cleveland Medical Center, United StatesMichael Wayne Epperly, University of Pittsburgh, United States
Copyright © 2018 Rezaee, Yadollahpour and Bayati. This is an open-access article distributed under the terms of the Creative Commons Attribution License (CC BY). The use, distribution or reproduction in other forums is permitted, provided the original author(s) and the copyright owner(s) are credited and that the original publication in this journal is cited, in accordance with accepted academic practice. No use, distribution or reproduction is permitted which does not comply with these terms.
*Correspondence: Ali Yadollahpour, yadollahpour.a@gmail.com