- 1Department of Radiation Oncology, Erasmus MC Cancer Institute, Rotterdam, Netherlands
- 2Department of Obstetrics and Gynaecology, Erasmus MC Cancer Institute, Rotterdam, Netherlands
- 3Department of Electrical Engineering, Eindhoven University of Technology, Eindhoven, Netherlands
- 4Department of Radiation oncology, University Medical Centre Maastricht (MAASTRO), Maastricht, Netherlands
Background: Addition of deep hyperthermia to radiotherapy results in improved local control (LC) and overall survival compared to radiotherapy alone in cervical carcinoma patients. Based on preclinical data, the time interval between radiotherapy, and hyperthermia is expected to influence treatment outcome. Clinical studies addressing the effect of time interval are sparse. The repercussions for clinical applications are substantial, as the time between radiotherapy and hyperthermia should be kept as short as possible. In this study, we therefore investigated the effect of the time interval between radiotherapy and hyperthermia on treatment outcome.
Methods: We analyzed all primary cervical carcinoma patients treated between 1996 and 2016 with thermoradiotherapy at our institute. Data on patients, tumors and treatments were collected, including the thermal dose parameters TRISE and CEM43T90. Follow-up data on tumor status and survival as well as late toxicity were collected. Data was analyzed using Cox proportional hazards analysis and Kaplan Meier analysis.
Results: 400 patients were included. Kaplan Meier and univariate Cox analysis showed no effect of the time interval (range 30–230 min) on any clinical outcome measure. Besides known prognostic factors, thermal dose parameters TRISE and CEM43T90 had a significant effect on LC. In multivariate analysis, the thermal dose parameter TRISE (HR 0.649; 95% CI 0.501–0.840) and the use of image guided brachytherapy (HR 0.432; 95% CI 0.214–0.972), but not the time interval, were significant predictors of LC and disease specific survival.
Conclusions: The time interval between radiotherapy and hyperthermia, up to 4 h, has no effect on clinical outcome. These results are re-ensuring for our current practice of delivering hyperthermia within maximal 4 h after radiotherapy.
Introduction
Cervical carcinoma is the sixth most common cancer in women in The Netherlands and the fourth most common cancer in women worldwide(1). Local control (LC) is a prerequisite for definitive cure and contributes highly to patient survival (2). For women with locally advanced cervical cancer, radiotherapy combined with cisplatin-based chemotherapy is the standard treatment (3). In patients unfit for chemotherapy or patients with large FIGO IIB or higher FIGO stage disease, radiotherapy can also be combined with hyperthermia -also called thermoradiotherapy-, instead of chemotherapy. In the latter patients, the addition of hyperthermia to radiotherapy results in comparable outcome compared to chemoradiotherapy in terms of LC and overall survival (OS), while treatment-related toxicity is not increased (4–7). A direct clinical comparison between thermo- and chemoradiotherapy is lacking to date. Nevertheless, a recent network meta-analysis by Datta et al showed that both thermo- as well as chemoradiotherapy were the modalities with the best comprehensive impact on clinical endpoints in cervical cancer patients(8).
At our institute patients are treated standard with thermoradiotherapy in case of a locally advanced (FIGO IIB and higher) tumor or when patients are unfit for or refuse Cisplatin chemotherapy. Induction chemotherapy is indicated in case the tumor is 6 cm or more, lymph node metastases are 2 cm or larger and in case of positive para-aortic lymph nodes. If induction chemotherapy is applied, local treatment consists of thermoradiotherapy, which is given sequentially to the chemotherapy.
Hyperthermia, defined as an elevation of tissue temperature in the range of 40–44 degrees Celsius, is a potent sensitizer of radiotherapy (9, 10). The biological mechanisms of hyperthermia in combination with radiotherapy are diverse and include increased oxygenation, induction of direct cell death and immune stimulation (11, 12). In addition, hyperthermia inhibits the repair of DNA double strand breaks, which are the main inducers of tumor cell death following ionizing radiation (13, 14).
The sensitizing effect of hyperthermia in radiotherapy is determined by the thermal dose, which is the net result of the temperature rise in the target area and the duration of heating (15, 16). Two clinical thermal dose parameters are often investigated are: the CEM43T90 and the linearized TRISE (15). We have previously shown that CEM43T90 and TRISE are independent predictors for LC and Disease Specific Survival (DSS) in a large cohort of cervical cancer patients (15).
For practical reasons and a pre-clinically observed improved therapeutic ratio, hyperthermia is usually applied following external beam radiotherapy treatment (17–19). The effect of the time interval between radiotherapy and hyperthermia on clinical outcome is subject of active investigation. Preclinical evidence suggests a synergistic effect of hyperthermia with radiation when administered within 4 h, with an enhanced effect using shorter time intervals (17, 20). However, the size and relevance of this effect in the clinical setting has not been investigated thoroughly (21, 22). At our institution the hyperthermia treatment is applied within 4 h after the external beam fraction. The waiting time within these 4 h, though, is completely random to date, depending on machine and personnel availability.
Recently, a retrospective analysis showed a significant association between a short time interval and a poor LC and OS (23). Confirmation of this relation would have great impact: all centers treating cervical cancer patients with thermoradiotherapy should reduce the time interval. Therefore, the aim of our study was to analyze the effect of time interval between radiotherapy and hyperthermia on patient outcome in larger cohort of patients with cervical carcinoma cancer.
Methods
Patient Population
The research protocol for this investigation was approved by the medical ethics committee of Erasmus MC Cancer Institute, Rotterdam, the Netherlands (MEC-2018-1081). Patients included were diagnosed with primary cervical cancer and treated with curative intent using thermoradiotherapy at our institute between August 1996 and December 2016. Excluded were patients receiving concurrent chemotherapy, fewer than four of the five intended hyperthermia sessions and patients receiving radiotherapy at other institutes because of a lack of data on follow-up. All patients had a histologically confirmed cervical carcinoma and were staged by the International Federation of Gynecology and Obstetrics (FIGO) clinical staging system, including investigation under general anesthesia with cystoscopy and lymph node staging using CT and/or MRI and/or PET-CT. Indications for primary thermoradiotherapy at Erasmus MC Cancer Institute are locally advanced tumors: “lateral” FIGO IIB (>50% parametrial invasion), IIIA, IIIB, and IVA, patients unfit or refusing platinum-based chemotherapy and patients receiving induction chemotherapy. Indications for induction chemotherapy include primary tumor size of 6 cm or larger, pelvic lymph node metastases of 2 cm or larger or para-aortic lymph node metastases (24). Induction chemotherapy consisted of 6-weekly cycles of cisplatin/taxol chemotherapy, with clinical assessment after 3 and 6 cycles.
Radiotherapy Treatment
Radiotherapy treatment consisted of daily EBRT 23 × 2 Gy for pelvic fields or 28 × 1.8 Gy if the para-aortal region was included in the field. EBRT was delivered using 3D conformal techniques until 2011, after which intensity modulated radiotherapy (IMRT) was gradually introduced. From 2014 onwards VMAT was the preferred technique, because of better conformity and faster treatment times. From 2011 onwards a plan-of-the day protocol was introduced (25). A brachytherapy boost was delivered using a high-dose rate iridium source using 2D planning to point A, with a dose of 2 × 8.5 Gy. From the end of 2012 onwards, image (MRI) guided brachytherapy (IGBT) was applied with a combined interstitial and intracavitary approach, delivering 3 or 4 fractions of 7 Gy or higher to the high-risk CTV (26).
Hyperthermia Treatment
Hyperthermia (HT) was delivered after radiation therapy, a total of five times during the 5–6 weeks of EBRT. The BSD-2000 system was used (Pyrexar Medical Corporation, Salt Lake City, Utah, USA) for all HT treatments, with the Sigma-60 or Sigma-eye applicator selection depending on the patients' size. Intraluminal thermometry was performed by placing thermometers in rectum, bladder and vagina for all patients. Until July 2012 thermometry was performed by using Bowman probes (Pyrexar Medical Corporation, Salt Lake City, Utah, USA) combined with thermal mapping every 5 min during treatment with a step size of 1 cm and a maximum length of 14 cm. Hereafter temperature measurement was continuous using multi-sensor fiber optic temperature probes (FISO Technologies Inc., Québec, QC, Canada) (4–8 sensor distance between sensors 2 cm). Both systems fulfill the quality assurance guidelines of European Society for Hyperthermic Oncology, accuracy of ±0.2°C (27, 28). Of 90 min scheduled treatment time, the first 30 min are used to warm up to intraluminal temperatures of ≥40°C. Then treatment was continued for 60 min aiming for maximum intraluminal temperatures depending on patient tolerance.
Collection of Patient and Follow-Up Data
The following patient characteristics were extracted from the patient's files: age at diagnosis, histology, FIGO stage and lymph node status. Local FIGO stage was noted. Seven patients presenting with limited distant metastases, but treated with curative intent, were recorded as FIGO IVB. Induction chemotherapy was noted. Radiotherapy and HT treatment characteristics were extracted from treatment planning and other recording systems.
Pelvic recurrence, distant recurrence, survival status, date and cause of death, as well as late toxicity data were extracted and/or retrieved from patient records, referring hospitals, general physicians and the civil registry. Late toxicity, occurring and/or persisting after 6 months following treatment, was scored according to CTCAE v4.0. Only high-grade (equal or higher than grade 3) toxicities were extracted, as these events are clinically most relevant and most reliably extracted retrospectively.
Hyperthermia Treatment Parameters
Hyperthermia treatment characteristics collected were the number of hyperthermia sessions, CEM43T90, TRISE and treatment duration. The CEM43T90 is the mean cumulative equivalent minutes of T90 (temperature reached in at least 90% of measurement locations) at 43°C. TRISE is a thermal dose parameter based on the temperature exceeded by 50% of measurement sites and duration of heating (15, 29).
The time interval between EBRT and HT was defined as the time (in minutes) between the first beam-on of the radiotherapy treatment and the start of the heating (power on HT device). As multiple HT treatments are delivered over the course of treatment, the mean time interval between HT and EBRT treatment over all hyperthermia treatments was calculated. In patients in which more than 50% of the time intervals could not be reconstructed, the time interval was noted as missing.
Statistical Analysis
LC, disease free survival (DFS), DSS and OS were calculated from start date of radiotherapy until event. LC was noted as “failed” when a physician diagnosed a local recurrence either clinically or with imaging (CT/MRI). For DFS, an event was defined as the occurrence of either local or distant recurrence. Patients were censored for local or distant control after the last visit of any physician specifically examining for recurrent disease. For DSS and OS, patients without an event were censored on the day the civil registry was consulted. LC, DFS, DSS, OS were analyzed using the Kaplan–Meier method and statistical differences between groups were determined using the log-rank test. A p-value of ≤ 0.05 was considered statistically significant. For KM analyses per time period, the cohort was divided into 5-year periods. Differences in continuous factors between groups were analyzed using the Mann-Whitney U test. Differences in categorical factors between groups were analyzed using the Chi-Square of Fisher's exact test. Hazard ratio (HR) and 95% confidence interval (CI) for various covariates were obtained by univariate Cox proportional hazards analyses. For categorical values, all categories were compared to the index category. In case of small numbers of patients per category of a categorical variable, combinations were made where statistically possible and clinically reasonable to prevent too small subcategories in the analyses. Covariates were taken into multivariable analysis based on clinical experience and a p-value ≤ 0.20 in univariate analysis. A backward selection procedure was applied with p < 0.05 as a threshold to find the combination of factors that have independent prognostic value. All analyses were performed using IBM SPSS statistics version 24.0 software package (SPSS Inc., Chicago, IL, USA).
Results
General Characteristics of the Cohort
Four hundred patients were included in the analysis. General characteristics of the entire cohort are listed in Table 1. 40 (10.0%) patients had an adenocarcinoma and 342 (85.5%) had a squamous cell carcinoma. Eighteen patients (4.5%) had another histology, but were primarily diagnosed as having a cervix uterus origin. The median time interval between start of radiotherapy and start of hyperthermia was 74 min (interquartile range (IQR) 62–94 min) (Table 1). Median follow-up for local and distant recurrence was 57 months (IQR 31–81 months). Median follow-up for survival was 106 months (IQR 52–160 months). There were 113 cases with a local recurrence out of 175 cases with any recurrence. There were 151 disease specific deaths out of 218 total deaths.
One time interval could not be reconstructed in 10.0% and two time intervals in 2.0% of patients. In these cases, the mean time interval was calculated using the available interval times. In 8 cases more than 50% of the potential time intervals could not be reconstructed. Temperature data were system-lost in 27 cases. Hence, 373 cases were included in the analysis of thermal dose and 392 for the time interval.
Kaplan-Meier Analysis of the Effect of Time Interval and Thermal Dose
To determine the effect of time interval and thermal dose on clinical outcome, we divided the cohort over the median of the time interval (74 min), TRISE (3.46°C) and CEM43T90 (3.40 min). For the time interval no effect was observed for any of the outcome measures (Figure 1). Figure 1 shows that a higher thermal dose, expressed as TRISE, corresponded to improved outcome for all outcome measures. This effect was statistically significant for LC and DFS. For the thermal dose parameter CEM43T90, KM curves for LC and DFS showed no significant differences, see Supplementary Figure 1.
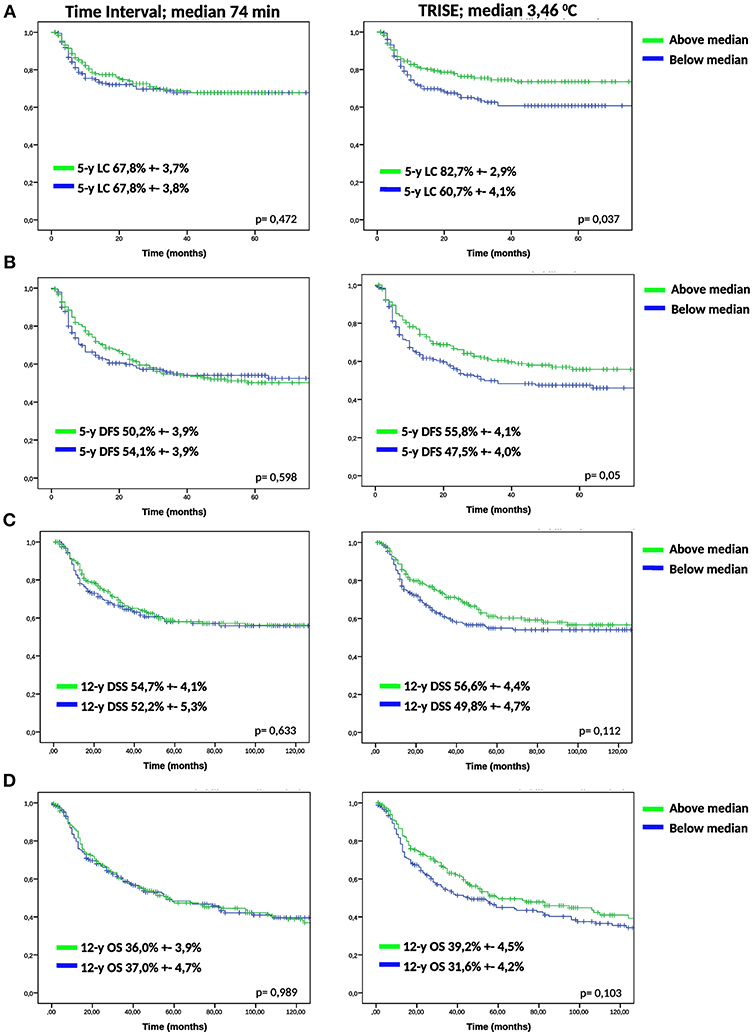
Figure 1. KM analysis of low and high time interval and TRISE. KM-curves for low and high time interval and TRISE for LC (A), DFS (B), DSS (C), and OS (D) were compared using log-rank test.
We compared the groups higher and lower than median time interval and TRISE for equal distribution of other prognostic factors (Table 2). Patients in the high TRISE group were significantly older and had more HT treatments (Table 2). In the low time interval group significantly more patients were diagnosed with para-aortic lymphadenopathy (20.8 vs. 10.2%) compared to the high time interval group, more patients were treated with induction chemotherapy (34.4 vs. 17.0%, p ≤ 0,001) and more with VMAT (16.7 vs. 5.0%, p = 0.001) and IGBT (20.8 vs. 13.0%, p = 0.038). These differences are explained by the length of the time interval, which became shorter over time (Supplementary Figure 2). KM analyses performed in four different time periods showed no differences in LC between high and low time interval patient groups (Supplementary Figure 3), as well as in DFS, DSS and OS (data not shown). In order to determine the effect of very short or very long time intervals, cases were divided over the quartiles of the time interval. Again no differences were observed for LC, DFS, DSS and OS between the four time interval groups (Figure 2).
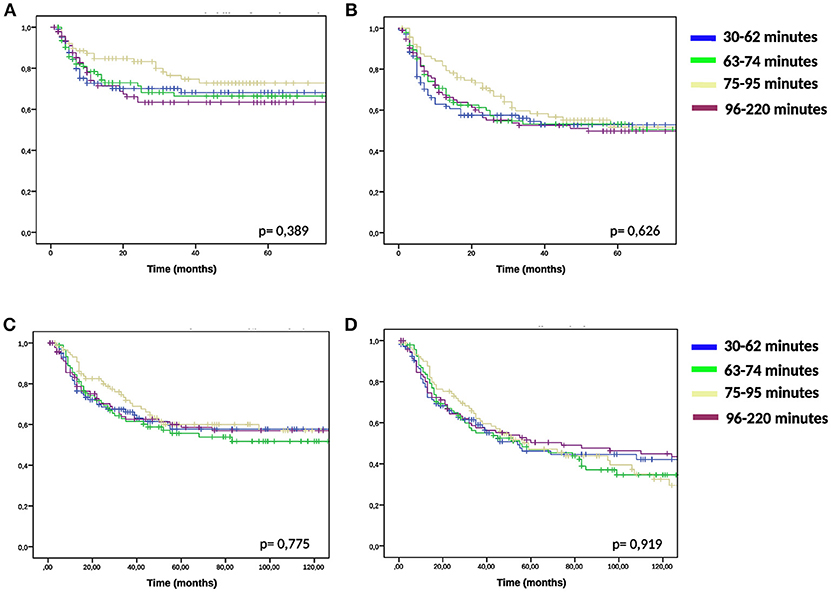
Figure 2. KM analysis of quartile time interval groups. KM-curves for quartiles of the time interval and for LC(A), DFS (B), DSS (C), and OS (D) were compared using log-rank test.
Univariate and Multivariate Cox Proportional Hazard Analysis
Using univariate Cox proportional hazards regression analysis the influence of known prognostic factors, time interval and thermal dose on LC, DFS, DSS, and OS was determined (Table 3). The time interval showed no effect on LC, DFS, DSS, and OS with Hazard Ratios of 1. Histology (squamous cell vs. adenocarcinoma; HR 0.48; 95% CI 0.29–0.81), FIGO stage (FIGO IIIA + IIIB vs. FIGO IB; HR 2.72; 95% CI 1.37–5.39), CEM43T90 (HR 0.93; 95% CI 0.87–0.99), TRISE (HR 0.67; 95% CI 0.52–0.86) and IGBT (HR 0.41; 95% CI 0.21–0.82) showed a significant effect on LC in univariate analysis. FIGO stage, TRISE, and IGBT also showed a significant effect on DFS, DSS, and OS.
Multivariate Cox analyses were performed (Table 3). As CEM43T90 and TRISE are both indicators of thermal dose, these factors were introduced separately into the analyses. Histology (squamous cell vs. adenocarcinoma; HR 0.45; 95% CI 0.26–0.78), FIGO stage (FIGO IIIA + IIIB vs. FIGO IB; HR 2.80; 95% CI 1.34–5.84), lymphadenopathy (iliac vs. negative; HR 1.80; 95% CI 1.16–2.81), CEM43T90 (HR 0.92; 95% CI 0.85–0.99), TRISE (HR 0.65; 95% CI 0.50–0.84), and IGBT (HR 0.43; 95% CI 0.21–0.97) showed a significant effect on LC (Table 3). Note that having another histology than adeno- or squamous cell carcinoma did not have a significant effect on any of the clinical endpoints (Table 3). For DSS, independent prognostic factors were FIGO stage, lymphadenopathy, TRISE, and IGBT. Time interval was added to every analysis, but was always removed because of non-significance.
Effect of Time Interval and Thermal Dose on Late Toxicity
To determine the effect of time interval and thermal dose on toxicity, grade 3 or higher late toxicity was analyzed. The incidence of late toxicity did not differ between low or high TRISE (10.2 vs. 11.2%, respectively, p = 0.751) or low or high time interval patients (10.4 vs. 10.5%, respectively, p = 0.978) (Table 4).
Discussion
From this large retrospective cohort study, we conclude that a time interval between radiotherapy and hyperthermia up to 4 h has no effect on clinical outcome. Other known prognostic factors; use of IGBT and the thermal dose did have an effect on clinical outcome.
Hyperthermia is a potent sensitizer of radiotherapy in cancer treatment (6, 11, 17, 18, 30–33). Based on preclinical data, it could be hypothesized that a shorter time interval between radiotherapy and hyperthermia results in improved clinical outcome. This is largely based on the -relatively recent- insight that hyperthermia inhibits DNA repair in combination with the observation that most DNA damage after radiotherapy is repaired within 2–6 h (13, 20, 23). Several preclinical studies have analyzed the influence of the time interval (17, 20, 34). Li et al showed enhanced cell kill efficacy upon a shorter time interval (34). Overgaard et al. using a murine model, showed that at a time interval of 4 h, the normal tissue damage is minimized compared to the tumor damage (17). This study also showed that a shorter time interval increased tumor cell killing, but at the cost of increased normal tissue toxicity. The drawback of these two studies is that the target temperatures used, exceed those generally measured in the clinic. More recently, two cervical carcinoma cell lines were treated with hyperthermia and radiotherapy at different time intervals and target temperatures (20). There was a small increase in cell killing at shorter time intervals, but the effect was less apparent than in previous studies (17, 34). Finally, preclinical data generated at our institute indicate no enhanced effect of a shorter time interval on tumor cell kill (personal communication R. Kanaar, Dept. of Molecular Genetics).
There are few clinical studies which have investigated the clinical relevance of the time interval between radiotherapy and hyperthermia (21, 22). Arcangeli et al showed that skin toxicity was reduced with an interval of 4 h, compared to simultaneous application (21). Lindholm et al compared short and long time intervals in patients with superficial tumors (22). In both studies patient numbers were too small to draw firm conclusions. More recently, in a cohort of 58 primary cervical carcinoma patients, a significant association between a lower than median time interval and improved LC and OS was observed (23). To explain for these different findings, firstly patient characteristics may be different between the two cohorts. In the cohort of Van Leeuwen et al. patients are only indicated for thermoradiotherapy when chemoradiation was not possible due to renal function impairment, co-morbidity or age. Therefore, these patients are potentially more frail compared to the patients in our cohort. Second, it is possible that thermal dose was higher compared to our cohort, leading to a more pronounced biological effect of the time interval in the cohort of Van Leeuwen. Finally, in the study by Van leeuwen, following dichotomization of the time interval for KM analysis, the dichotomized time interval was also used in Cox proportional hazard analysis. Instead, we used the continuous value of the time interval in Cox analysis, as this is more informative and less sensitive to false positive results (35). Future clinical studies, preferably prospective, should provide a more definitive answer to this relevant clinical issue.
Besides the abovementioned inhibition of DNA repair, hyperthermia can also sensitize tumor cells to radiotherapy through various other mechanisms. These mechanisms include increased oxygenation, induction of direct tumor cell death and immune stimulation (12). Little is known about the exact biological and physiological mechanisms that constitute hyperthermia's proven clinical effectiveness. Increased perfusion and thus increased oxygenation can be expected at temperatures ≥39°C and may hold for many hours after treatment (36, 37). The direct hyperthermia-induced cytotoxicity seems to come in play at temperatures of ≥40°C. This effect is short-lived after treatment but results in spatial cooperation as it mainly effects cancer cells that are relatively insensitive to the effects of radiotherapy and chemotherapy (19, 38, 39). Studies investigating the effect of local or loco-regional hyperthermia on the immune system have not yet looked into the time or temperature dependency to our knowledge (40). The relative contribution of these effects to the clinical effect of hyperthermia is unknown. Our finding of a null-effect of the time interval could be interpreted as that DNA repair inhibition is relatively unimportant for the clinical hyperthermia effect. But it could also imply that in patients, it takes longer before the DNA repair inhibition effect disappears, compared to in vitro studies.
A limitation of our study is the retrospective setup, with the potential for confounding, data misinterpretation and data loss. The latter could be relevant for the collection of the late toxicity data in our study, as it was not recorded in a standardized manner at Erasmus MC Cancer Institute during the time of inclusion. Therefore, we chose not to include Grade 1–2 late toxicities as these might have been recorded incompletely. Grade 3 or higher toxicities are more likely to be recorded as by definition, a medical intervention is required. As potential miss of toxicity is not related to the studied factors (TRISE, time interval), we do not expect the statistical analysis to be influenced by this potential miss of data.
Conclusions
In contrast to thermal dose, there is no effect of the time interval between radiotherapy and hyperthermia on clinical outcome in primary cervical carcinoma patients. The clinical consequence of this study is that our current recommendation to apply hyperthermia within 4 h after radiation was and remains valid for cervical carcinoma patients treated with thermoradiotherapy. These finding are also relevant for the, mostly centralized, clinical hyperthermia units, as patients can be re-assured that their outcome is not affected by travel times up to 4 h.
Data Availability
The datasets generated for this study are available on request to the corresponding author.
Author Contributions
MK: drafting the article, data analysis, data extraction; HM: data extraction, data analysis, critically revising the article; JvH: data extraction, critically revising the article; AA: data extraction; JM: critically revising the article; HvD: critically revising the article; MP: critically revising the article, data extraction, data analysis; EO: data analysis, critically revising the article; RV: critically revising the article; LL: critically revising the article; GvR: critically revising the article; MF: data extraction, data analysis, critically revising the article.
Funding
This study was supported by the Dutch Cancer Society (grant DDHK 2013-6072).
Conflict of Interest Statement
GvR reported conflict of interests outside the submitted work: Dr. G. Sennewald Medizintechnik GmbH (Support conference participation), Pyrexar Medical (Research MR-thermometry), Sensius BV (Consultant (unpaid)). MP reported conflict of interests outside the submitted work: Sensius BV (Consultant (unpaid)).
The remaining authors declare that the research was conducted in the absence of any commercial or financial relationships that could be construed as a potential conflict of interest.
Supplementary Material
The Supplementary Material for this article can be found online at: https://www.frontiersin.org/articles/10.3389/fonc.2019.00134/full#supplementary-material
Supplementary Figure 1. KM analysis of low and high CEM43T90 groups. KM-curves for low and high CEM43T90 for LC (A) and DFS (B) were compared using log-rank test.
Supplementary Figure 2. Development of the time interval in time. Shown are the different time periods used in subsequent KM-analysis.
Supplementary Figure 3. KM analysis of low and high time interval per time period. KM-curves for low and high time interval for LC were compared using log-rank test.
References
1. Ferlay J, Soerjomataram I, Dikshit R, Eser S, Mathers C, Rebelo M, et al. Cancer incidence and mortality worldwide: sources, methods and major patterns in GLOBOCAN 2012. Int J Cancer. (2015) 136:E359–386. doi: 10.1002/ijc.29210
2. Sturdza A, Potter R, Fokdal LU, Haie-Meder C, Tan LT, Mazeron R, et al. Image guided brachytherapy in locally advanced cervical cancer: Improved pelvic control and survival in RetroEMBRACE, a multicenter cohort study. Radiother Oncol. (2016) 120:428–33. doi: 10.1016/j.radonc.2016.03.011
3. Chemoradiotherapy for Cervical Cancer Meta-Analysis C. Reducing uncertainties about the effects of chemoradiotherapy for cervical cancer: a systematic review and meta-analysis of individual patient data from 18 randomized trials. J Clin Oncol. (2008) 26:5802–12. doi: 10.1200/JCO.2008.16.4368
4. van der Zee J, Koper PC, Lutgens LC, Burger CW. Point-counterpoint: what is the optimal trial design to test hyperthermia for carcinoma of the cervix? Point: addition of hyperthermia or cisplatin to radiotherapy for patients with cervical cancer; two promising combinations–no definite conclusions. Int J Hyperthermia. (2002) 18:19–24. doi: 10.1080/02656730110083738
5. Franckena M, Lutgens LC, Koper PC, Kleynen CE, van der Steen-Banasik EM, Jobsen JJ, et al. Radiotherapy and hyperthermia for treatment of primary locally advanced cervix cancer: results in 378 patients. Int J Radiat Oncol Biol Phys. (2009) 73:242–50. doi: 10.1016/j.ijrobp.2008.03.072
6. Lutgens L, van der Zee J, Pijls-Johannesma M, De Haas-Kock DF, Buijsen J, Mastrigt GA, et al. Combined use of hyperthermia and radiation therapy for treating locally advanced cervical carcinoma. Cochr Database Syst Rev. (2010) 20:CD006377. doi: 10.1002/14651858.CD006377.pub2
7. Lutgens LC, Koper PC, Jobsen JJ, van der Steen-Banasik EM, Creutzberg CL, van den Berg HA, et al. Radiation therapy combined with hyperthermia versus cisplatin for locally advanced cervical cancer: results of the randomized RADCHOC trial. Radiother Oncol. (2016) 120:378–82. doi: 10.1016/j.radonc.2016.02.010
8. Datta NR, Stutz E, Gomez S, Bodis S. Efficacy and safety evaluation of the various therapeutic options in locally advanced cervix cancer: a systematic review and network meta-analysis of randomized clinical trials. Int J Radiat Oncol Biol Phys. (2019) 103:411–37. doi: 10.1016/j.ijrobp.2018.09.037
9. van der Zee J, Vujaskovic Z, Kondo M, Sugahara T. The Kadota Fund International Forum 2004–clinical group consensus. Int J Hyperthermia. (2008) 24:111–22. doi: 10.1080/02656730801895058
10. Datta NR, Ordonez SG, Gaipl US, Paulides MM, Crezee H, Gellermann J, et al. Local hyperthermia combined with radiotherapy and-/or chemotherapy: recent advances and promises for the future. Cancer Treat Rev. (2015) 41:742–53. doi: 10.1016/j.ctrv.2015.05.009
11. Vujaskovic Z, Song CW. Physiological mechanisms underlying heat-induced radiosensitization. Int J Hyperthermia. (2004) 20:163–74. doi: 10.1080/02656730310001619514
12. van den Tempel N, Horsman MR, Kanaar R. Improving efficacy of hyperthermia in oncology by exploiting biological mechanisms. Int J Hyperthermia. (2016) 32:446–54. doi: 10.3109/02656736.2016.1157216
13. Krawczyk PM, Eppink B, Essers J, Stap J, Rodermond H, Odijk H, et al. Mild hyperthermia inhibits homologous recombination, induces BRCA2 degradation, and sensitizes cancer cells to poly (ADP-ribose) polymerase-1 inhibition. Proc Natl Acad Sci USA. (2011) 108:9851–6. doi: 10.1073/pnas.1101053108
14. Oei AL, Vriend LE, Crezee J, Franken NA, Krawczyk PM. Effects of hyperthermia on DNA repair pathways: one treatment to inhibit them all. Radiat Oncol. (2015) 10:165. doi: 10.1186/s13014-015-0462-0
15. Franckena M, Fatehi D, de Bruijne M, Canters RA, van Norden Y, Mens JW, et al. Hyperthermia dose-effect relationship in 420 patients with cervical cancer treated with combined radiotherapy and hyperthermia. Eur J Cancer. (2009a) 45:1969–78. doi: 10.1016/j.ejca.2009.03.009
16. van den Tempel N, Laffeber C, Odijk H, van Cappellen WA, van Rhoon GC, Franckena M, et al. The effect of thermal dose on hyperthermia-mediated inhibition of DNA repair through homologous recombination. Oncotarget. (2017) 8:44593–604. doi: 10.18632/oncotarget.17861
17. Overgaard J. Simultaneous and sequential hyperthermia and radiation treatment of an experimental tumor and its surrounding normal tissue in vivo. Int J Radiat Oncol Biol Phys. (1980) 6:1507–17. doi: 10.1016/0360-3016(80)90008-5
18. van der Zee J, Gonzalez Gonzalez D, van Rhoon GC, van Dijk JD, van Putten WL, Hart AA. Comparison of radiotherapy alone with radiotherapy plus hyperthermia in locally advanced pelvic tumours: a prospective, randomised, multicentre trial. Dutch Deep Hyperthermia Group Lancet. (2000) 355:1119–25. doi: 10.1016/S0140-6736(00)02059-6
19. Kampinga HH. Cell biological effects of hyperthermia alone or combined with radiation or drugs: a short introduction to newcomers in the field. Int J Hyperthermia. (2006) 22:191–6. doi: 10.1080/02656730500532028
20. van Leeuwen CM, Oei AL, Ten Cate R, Franken NAP, Bel A, Stalpers LJA, et al. Measurement and analysis of the impact of time-interval, temperature and radiation dose on tumour cell survival and its application in thermoradiotherapy plan evaluation. Int J Hyperthermia. (2018) 34:30–8. doi: 10.1080/02656736.2017.1320812
21. Arcangeli G, Nervi C, Cividalli A, Lovisolo GA. Problem of sequence and fractionation in the clinical application of combined heat and radiation. Cancer Res. (1984) 44 (Suppl. 10):4857s−63s.
22. Lindholm CE, Kjellen E, Nilsson P, Hertzman S. Microwave-induced hyperthermia and radiotherapy in human superficial tumours: clinical results with a comparative study of combined treatment versus radiotherapy alone. Int J Hyperthermia. (1987) 3:393–411. doi: 10.3109/02656738709140410
23. van Leeuwen CM, Oei AL, Chin K, Crezee J, Bel A, Westermann AM, et al. A short time interval between radiotherapy and hyperthermia reduces in-field recurrence and mortality in women with advanced cervical cancer. Radiat Oncol. (2017) 12:75. doi: 10.1186/s13014-017-0813-0
24. Heijkoop ST, Franckena M, Thomeer MG, Boere IA, Van Montfort C, Van Doorn HC. Neoadjuvant chemotherapy followed by radiotherapy and concurrent hyperthermia in patients with advanced-stage cervical cancer: a retrospective study. Int J Hyperthermia. (2012) 28:554–61. doi: 10.3109/02656736.2012.674622
25. Heijkoop ST, Langerak TR, Quint S, Bondar L, Mens JW, Heijmen BJ, et al. Clinical implementation of an online adaptive plan-of-the-day protocol for nonrigid motion management in locally advanced cervical cancer IMRT. Int J Radiat Oncol Biol Phys. (2014) 90:673–9. doi: 10.1016/j.ijrobp.2014.06.046
26. Potter R, Kirisits C, Fidarova EF, Dimopoulos JC, Berger D, Tanderup K, et al. Present status and future of high-precision image guided adaptive brachytherapy for cervix carcinoma. Acta Oncol. (2008) 47:1325–36. doi: 10.1080/02841860802282794
27. Bruggmoser G, Bauchowitz S, Canters R, Crezee H, Ehmann M, Gellermann J, et al. Quality assurance for clinical studies in regional deep hyperthermia. Strahlenther Onkol. (2011) 187:605–10. doi: 10.1007/s00066-011-1145-x
28. Bruggmoser G, Bauchowitz S, Canters R, Crezee H, Ehmann M, Gellermann J, et al. Guideline for the clinical application, documentation and analysis of clinical studies for regional deep hyperthermia: quality management in regional deep hyperthermia. Strahlenther Onkol. (2012) 188(Suppl. 2):198–211. doi: 10.1007/s00066-012-0176-2
29. Fatehi D, de Bruijne M, van der Zee J, van Rhoon GC. RHyThM, a tool for analysis of PDOS formatted hyperthermia treatment data generated by the BSD2000/3D system. Int J Hyperthermia. (2006) 22:173–84. doi: 10.1080/02656730600597459
30. Datta NR, Bose AK, Kapoor HK, Gupta S. Head and neck cancers: results of thermoradiotherapy versus radiotherapy. Int J Hyperthermia. (1990) 6:479–86. doi: 10.3109/02656739009140944
31. Overgaard J, Gonzalez Gonzalez D, Hulshof MC, Arcangeli G, Dahl O, Mella O, et al. Randomised trial of hyperthermia as adjuvant to radiotherapy for recurrent or metastatic malignant melanoma. Eur Soc Hyperthermic Oncol Lancet. (1995) 345:540–3. doi: 10.1016/S0140-6736(95)90463-8
32. Harima Y, Nagata K, Harima K, Ostapenko VV, Tanaka Y, Sawada S. A randomized clinical trial of radiation therapy versus thermoradiotherapy in stage IIIB cervical carcinoma. Int J Hyperthermia. (2001) 17:97–105. doi: 10.1080/02656730010001333
33. Franckena M, Stalpers LJ, Koper PC, Wiggenraad RG, Hoogenraad WJ, van Dijk JD, et al. Long-term improvement in treatment outcome after radiotherapy and hyperthermia in locoregionally advanced cervix cancer: an update of the Dutch Deep Hyperthermia Trial. Int J Radiat Oncol Biol Phys. (2008) 70:1176–82. doi: 10.1016/j.ijrobp.2007.07.2348
34. Li GC, Kal HB. Effect of hyperthermia on the radiation response of two mammalian cell lines. Eur J Cancer. (1977) 13:65–9. doi: 10.1016/0014-2964(77)90231-6
35. Royston P, Altman DG, Sauerbrei W. Dichotomizing continuous predictors in multiple regression: a bad idea. Stat Med. (2006) 25:127–41. doi: 10.1002/sim.2331
36. Dewhirst MW, Vujaskovic Z, Jones E, Thrall D. Re-setting the biologic rationale for thermal therapy. Int J Hyperthermia. (2005) 21:779–90. doi: 10.1080/02656730500271668
37. van Rhoon GC. Is CEM43 still a relevant thermal dose parameter for hyperthermia treatment monitoring? Int J Hyperthermia. (2016) 32:50–62. doi: 10.3109/02656736.2015.1114153
38. Overgaard J. The current and potential role of hyperthermia in radiotherapy. Int J Radiat Oncol Biol Phys. (1989) 16:535–49. doi: 10.1016/0360-3016(89)90470-7
39. Horsman MR, Overgaard J. Hyperthermia: a potent enhancer of radiotherapy. Clin Oncol. (2007) 19:418–26. doi: 10.1016/j.clon.2007.03.015
Keywords: hyperthermia, cervical cancer, radiotherapy, fractionation, clinical practical procedures, schedulability
Citation: Kroesen M, Mulder HT, van Holthe JML, Aangeenbrug AA, Mens JWM, van Doorn HC, Paulides MM, Oomen-de Hoop E, Vernhout RM, Lutgens LC, van Rhoon GC and Franckena M (2019) The Effect of the Time Interval Between Radiation and Hyperthermia on Clinical Outcome in 400 Locally Advanced Cervical Carcinoma Patients. Front. Oncol. 9:134. doi: 10.3389/fonc.2019.00134
Received: 09 November 2018; Accepted: 14 February 2019;
Published: 08 March 2019.
Edited by:
Charles A. Kunos, Investigational Drug Branch, National Cancer Institute (NIH), United StatesReviewed by:
Sarah M. Temkin, Virginia Commonwealth University, United StatesJyoti Mayadev, University of California, San Diego, United States
Copyright © 2019 Kroesen, Mulder, van Holthe, Aangeenbrug, Mens, van Doorn, Paulides, Oomen-de Hoop, Vernhout, Lutgens, van Rhoon and Franckena. This is an open-access article distributed under the terms of the Creative Commons Attribution License (CC BY). The use, distribution or reproduction in other forums is permitted, provided the original author(s) and the copyright owner(s) are credited and that the original publication in this journal is cited, in accordance with accepted academic practice. No use, distribution or reproduction is permitted which does not comply with these terms.
*Correspondence: M. Franckena, bS5mcmFuY2tlbmFAZXJhc211c21jLm5s
†These authors have contributed equally to this work