- 1Department of Regenerative Medicine, School of Pharmaceutical Sciences, Jilin University, ChangChun, China
- 2Department of Thoracic Surgery, The First Hospital of Jilin University, Changchun, China
- 3School of Biosciences and Biopharmaceutics, Guangdong Pharmaceutical University, Guangzhou, China
Exosomes are lipid membrane bilayer-encapsulated vesicles secreted by cells into the extracellular space. They carry abundant inclusions (such as nucleic acids, proteins, and lipids) that play pivotal roles in intercellular communication. Tumor stem cells are capable of self-renewal and are crucial for survival, proliferation, drug resistance, metastasis, and recurrence of tumors. The miRNAs (microRNAs) in exosomes have various functions, such as participating in inflammatory response, cell migration, proliferation, apoptosis, autophagy, and epithelial-mesenchymal transition. Tumor stem cells secrete exosomes that act as important messengers involved in various tumor processes and several studies provide increasing evidence supporting the importance of these exosomes in tumor recurrence and metastasis. This review primarily focuses on the production and secretion of exosomes from tumors and tumor stem cells and their effects on cancer progression. Cancer stem cancer derived exosome play an important massager in the tumor microenvironment. It also emphasizes on the study of tumor stem cell exosomes in the light of cancer metastasis and recurrence aiming to provide valuable insights and novel perspectives, which could be beneficial for developing effective diagnostic and treatment strategies.
Exosomes
Exosomes are extracellular vesicles (EVs) with a double-layer membrane structure without replication ability that all cells can secrete and release externally (1). In vivo, exosomes exist in body fluids such as blood, urine, cerebrospinal fluid, pleural fluid, abdominal fluid, saliva, amniotic fluid, breast milk, and semen, and can also be isolated from cell supernatants derived from in vitro cell cultures. Exosomes were previously regarded as worthless waste, and recent studies have shown that they play a pivotal role in cell interaction (2, 3). Exosomes can transfer genetic material from donor cells to recipient cells, and are also crucial in cell-to-cell communication, especially remote cell information interaction (4–7). The contents carried by exosomes contain diverse components. Cells can influence another cell through the action of exosomes; in some cases, exosomes enable cells to transfer their genetic material to another cell (8, 9). Exosomes carry a variety of components, including proteins, nucleic acids, amino acids, and metabolites, which may be related to the components and functions of their secretory cells. They are naturally secreted under physiological or pathological conditions (3, 10–12). In physiology, related research on stem cells, tissue regeneration, bone reconstruction, angiogenesis, and other fields has been reported (13–15). In pathology, especially in tumor research, the application of tumor-derived exosomes has been intensively investigated (10, 13, 16, 17). Exosomes play an important role in carcinogenesis. They have been found to participate in many important cancer processes and have the potential to be used as cancer markers. They also drive the key multi-stage tumor process, which provides important therapeutic targets (18–21). In vitro studies have shown that exosomes secreted by tumors can induce high cell proliferation (22–24), invasion, metastasis (25, 26), acquired drug resistance (27), angiogenesis (28–31), and the establishment of the pre-metastasis niche (32–34). In addition, exosomes have been used in clinics as therapeutic drugs and biomarkers (35–37). Tumor-derived exosomes play an important role in multiple stages of cancer progression. Cancer stem cells (CSCs) are a subpopulation of tumor cells that contribute to resistance to chemotherapy drugs, metastasis, and recurrence in the development of tumors. Studying the exosomes secreted by CSCs and their anti-tumor clinical applications is of far-reaching significance. This article reviews the role of exosomes secreted by tumor cells in tumorigenesis and development, providing insight into their potential clinical applications.
Discovery of Exosomes
Exosomes were first discovered in 1983 in vesicles secreted by immature red blood cells of sheep (38, 39). Later, it was discovered that all types of cells can secrete exosomes and can be detected in body fluids. Exosomes have received widespread attention for their important functions, such as changing the extracellular microenvironment and participating in immunity (34). Exosomes contain mRNAs and miRNAs that can mediate cell-to-cell communication (5). Exosomes play an important role in tumor progression as messengers in cellular interactions, homeostasis of the tumor microenvironment, and formation of metastatic foci. Exosomes play an important part in tumor microenvironment as an important messenger with genetic information and proteins during cancer progression.
Formation of Exosomes
Exosomes are nanometer-sized (diameter = 30–150 nm, average 100 nm) membranous EVs formed by endocytosis (40). They have a double-layer membrane structure and a saucer-like morphology, abundant contents (including nucleic acids, proteins, lipids, etc.), and the ability to participate in information transfer among cells.
Exosomes originate from cells budding inward, forming an early membrane-endosome structure. Early endosomes mature into late endosomes and then accumulate in the lumen to form multivesicular bodies (MVBs), which can also be called multivesicular endosomes (MVEs), which contain many intraluminal vesicles (ILVs). After cells form MVEs, they may be degraded by fusion with auto-phagosomes or lysosomes, or they may be fused with the plasma membrane to release the substances in them. These ILVs are the final exosome formed (40). Exosomes belong to a subgroup of EVs, which are composed of vesicles of different sizes, mainly formed by cells through budding into early endosomes and MVEs to form ILVs, and are released through the fusion of MVEs with the plasma membrane (7, 8, 37).
The composition of exosomes differs depending on the cell source, indicating that tumor-derived exosomes may have specific markers (41). Compared with cell membranes, the phospholipid bilayer of exosomes is rich in lipids, such as cholesterol, ceramide, phosphatidylcholine, phosphatidyl ethanol sphingomyelin, and glycosphingolipid (42). During MVE formation, the surface of exosomes carries various proteins, such as transmembrane proteins, fusion proteins, receptor EGFR, adhesion molecules, integrin, histocompatibility complexes MHC I and II, cytokine protein ESCRT complex protein, and lipid raft-associated protein (43). Different exosomes have conserved components. The most common exosomal marker proteins are integrins and adhesion molecules, mainly CD9, CD63, CD81, MHC, TSG101, and HSC70, which can be used for the identification and sorting of exosomes (9, 37, 44). The main nucleic acids carried by exosomes include miRNA, mRNA, lncRNA, gDNA, and mtDNA (5, 45).
The process of the substances carried by exosomes is not random. The composition is determined by a specific mechanism and can change in response to the tumor microenvironment and stress conditions, thereby affecting the recipient cells (46–51). After secretion, exosomes interact with the recipient cells through different mechanisms, and the receptors or ligands contained in the recipient membrane can activate or stimulate multiple signaling pathways; they can also transfer the contents to the recipient cells through internalization. Exosomes can also be taken up by recipient cells through mechanisms such as phagocytosis and pinocytosis (52). The following mechanisms of exosomes bioactive molecules what influence target calls have been shown: (1) direct stimulate target cells by surface-binding ligands; (2) transfer activated receptors to recipient cells; (3) epigenetic reprogram recipient cells via delivery of functional proteins, RNAs and lipids (53). Through these mechanisms, exosomes can alter gene expression and protein translation modification (11).
Isolation and Identification of Exosomes
It is very important to isolate pure exosomes to study their mechanism of action and application in biomedical science. Exosomes have been successfully isolated by techniques such as super centrifugation, ultrafiltration, chromatography, polymer-based precipitation, and affinity capture of antibody-coupled magnetic beads. In the field of exosome research, there is no unified, simple, feasible, and high-purity separation method. At present, no extraction method can guarantee the content, purity, and biological activity of exosomes at the same time. The appropriate method is chosen based on the topic and experiment. The overspeed centrifugal method is widely used. The advantages and disadvantages of several methods for obtaining exosomes are shown in Table 1.
The appropriate method is chosen based on the topic and experiment. The overspeed centrifugal method is widely used. Isolation exosome instruments apply to characterization techniques are shown in Table 2.
Cancer Stem Cells
CSCs, also known as tumor-initiating cells, are cells in tumor tissues that have the ability to self-renew and produce heterogeneous tumor cells (54, 55). CSCs were first discovered in leukemia in 1997, were subsequently isolated from a variety of solid tumors, and presumed to be the clonal core of the tumor, playing an important role in multiple stages of tumor progression (56, 57). CSCs have the ability of retro-differentiation, self-replenishment, and self-renewal in tumor tissues, and have potential migration and drug resistance characteristics. They are considered triggers of carcinogenesis that promote cancer progression, spread, metastasis, drug resistance, and recurrence (56, 58, 59). Under the regulation of the tumor microenvironment (TME), the tumor stem cell population changes dynamically, and this change may achieve tumor activity through communication between the tumor and the CSCs. As an important messenger in the TME, exosomes secreted by tumor stem cells play a pivotal role in the dynamic regulation of tumor stem cell populations.
Origin of Cancer Stem Cells
CSCs are a subpopulation of cells in tumors. After xenotransplantation into immunodeficient animal hosts in in vivo experiments, this subpopulation has higher tumorigenicity than tumor cells at a lower cell concentration (56). This indicates that this subgroup of cells has a higher dividing ability, and their daughter cells are self-renewing and highly tumorigenic (60). Subgroups of such cells have also been found in solid tumors (61, 62). Cancer stem cell subgroups are characterized by low content (0.01–2%) and high tumorigenicity (54). A relatively small number of cancer stem cells with self-renewal ability can produce a large number of progeny cells that maintain limited proliferation ability (63). The small population of tumor cells with tumorigenicity expresses some specific antigen markers on the surface that are not expressed in a large population of cells. There are differences between different malignant tumors, and even between individual tumor cells of the same histology (54). Therefore, in the same TME, CSCs and tumor cells are at different stages of differentiation.
There are several hypotheses about the origin of cancer stem cells: (1) some genes of adult stem cells are mutated, their genome stability is reduced, and their cancer-causing mutation induction and self-renewal ability are excessively enhanced, so that they evolve into CSCs (64–66); (2) some genes of targeted progenitor cells have mutations, which enhance their limited self-renewal ability, and eventually evolve into CSCs (67); (3) differentiated non-stem cells use certain regulatory mechanisms, such as endothelial-mesenchymal transition (EMT), obtain the characteristics of stem cells and, as gene mutations occur, they are finally transformed into CSCs (64, 68, 69); (4) inflammatory cytokines may induce the formation of CSCs (70). The origin of CSCs is a multi-factor induction and multistep outcome. There is now a general consensus that differences exist between different malignant tumors and even between individual tumors of the same tissue. The phenotype of CSCs is complex; CSCs in tumors have the same genes, but there are multiple CSC subgroups in each tumor, and each CSC has its own unique biological characteristics (71). Tumor-forming CSCs play a significant role in tumor recurrence during the multi-step carcinogenesis of tumors. Therefore, the signal changes of CSCs in multi-stage tumor progression, especially in the recurrence stage after metastasis, are worthy of in-depth study.
Characteristics of Cancer Stem Cells
CSCs account for a very low proportion of solid tumor cells, and it is difficult to detect and identify them histologically (72). At present, there are many methods for their identification; serum-free culture into balls, labeling specific surface markers (73), in vivo limiting-dilution tumorigenicity assays in immunocompromised mice (74), etc. However, it is still very difficult to create a definitive classification of CSC subgroups (60). Therefore, the sorting of CSC subpopulations is based on the combination of tumor surface-specific antigen sorting and other functional analyses (such as functional recognition) and other screening conditions to establish CSC subpopulations. Cancer cells display diverse phenotypes within a certain period of time. Cell surface markers have been used in a variety of tumors to separate different tumor cell subgroups with different biological characteristics. Commonly used surface antigen markers, such as CD133 antigen, have been used for lung cancer (75–79), brain cancer (80), colon cancer (81, 82), and prostate cancer (83). The isolated cells have strong tumorigenic ability and stem cell characteristics, such as stem cell/progenitor cell markers of normal tissue (84), kidney stem cells (85), prostate stem cells (86), fetal neural stem cells (87), etc. The isolation of CSC subgroups is conducive to accurately studying signal transduction changes.
There are many forms of intratumorally heterogeneous tumor subgroups, showing obvious inter- and intra-tumor diversity (88). The existence of CSC subsets drives tumorigenesis and tumor metastasis (58). The results of exome and whole-genome sequencing indicate that most tumors have at least two driver mutations (89). Heterogeneous tumor cells produced by differentiation and proliferation can be transferred to a variety of tissues, causing the destruction and abnormal functions of normal tissues and organs. These abnormalities may lead to the appearance of different subgroups within the tumor, which have different combinations of dominant genetic susceptibility in different TMEs. In the multi-step process of tumor development and recurrence, signal transduction plays an important role in adaption to the TME in heterogeneous CSC subsets with superior susceptibility combinations. Therefore, studying CSCs in tumors can further elucidate the genesis of their heterogeneity and metastasis, which provides insight and inspiration for the design of novel intervention drugs targeting tumor suppressor signals.
Clinical Characteristics of CSCs
In-depth CSC research has shown that CSCs participate in metastatic formation, natural resistance to chemotherapy and radiotherapy, and cancer recurrence.
In clinical settings, the limitations of traditional anti-cancer therapies have been attributed to the targeting of the large non-CSC populations in tumors, rather than eliminating the rare subpopulations of CSCs (90–92). The difference between CSCs and non-CSCs could be mainly attributed to the process of EMT (93). EMT changes the heritable phenotype of cancer cells by employing epigenetic modifications rather than by introducing new genetic changes. As the EMT program is activated, cancer cells lose many epithelial cell characteristics, including epithelial cell connections and apical-basal polarity, and acquire mesenchymal characteristics, such as elongated, fibroblast-like morphology and increased migration and invasion ability (94). In some cancers, only tumor cells in the CSC-enriched subpopulation exhibit EMT-activated characteristics (95, 96). It is worth noting that in some experimental cancer models, the forced induction of EMT by epithelial tumor cells increases their ability to initiate tumors (95, 97, 98). These CSCs activate a genetically determined morphogenesis program. Normal CSCs reside in the CSC niche, maintain their stem cell status, and control their self-renewal and differentiation. The TME is also involved in metastasis through EMT, which leads to the spread and metastasis of the tumor (99).
Molecular targeted therapy for acquired drug resistance remains a major challenge in cancer treatment. Drug resistance may be the key to improving the effectiveness of targeted therapies for cancer patients. The dormant or slowly circulating subgroups of tumors keep being viable under treatment. These cells do not exhibit typical resistance-driven changes. The drug-resistant phenotype is temporary and can be reversed after drug removal. Tumors can relapse if treatment is stopped or acquired drug resistance is caused by continued treatment (100). CSCs are important factors in tumor drug resistance. Their mechanism of action includes pumping drugs out of the cell through the high expression of ABC transporters, high expression of anti-apoptotic genes, abnormal DNA damage repair mechanism, increased telomerase activity, self-renewal ability, and general quiescent phase, making drugs resistant to targeting the proliferation cycle (101). An important characteristic of CSCs is the activation of the EMT, during which activated tumor cells are resistant to many types of therapeutic drugs (102–104). Exosomes, as messengers of drug resistance in cancer stem cells under treatment, play a central role in the information transfer of the EMT effect of CSCs.
In the cascade of tumor invasion and metastasis, the most complicated process occurs after the metastatic tumor cells reach the parenchymal tissue, proliferate and form clones in the new environment, and finally form tumor masses. As the new tissue environment cannot provide the migrating tumor cells with the microenvironment of the primary tumor, metastatic tumor cells lacking various growth factors usually die quickly or survive for a period of time as micro-metastases in the form of single cells or small clusters of cells. Considering the tumor-initiating ability of CSCs, active CSCs are prone to form multiple micro-metastases in the body, which are difficult to detect in clinical settings and have the potential to develop or re-develop into new tumor mass precursors. These micro-metastases, which are widely distributed in the patient’s tissues, eventually cause tumor recurrence. In the cascade of tumor invasion and metastasis, early steps result in a very high success rates, but the success rate of colonization, that is, metastasis and recurrence, is very low. The invasion–metastasis cascade involves the migration of tumor cells from the primary tumor to potential sites of metastasis. This phenomenon depends on a series of intricately orchestrated and distinct physiological steps. In the initial step, the EMT occurs in situ and the tumor cells break through the basement membrane. Subsequently, these cells enter the blood or the lymphatic circulation and are transported to the distal parts of the body. The tumor cells then stay and penetrate the capillary/lymphatic walls to form occult micro-metastases at the metastatic sites. Some micro-metastases may gain the ability to form micro-clones at the metastatic sites as tumor cells can clone the metastatic foci, they often acquire the ability to form tiny clones at the metastatic sites, thereby, facilitating metastases. In this way the entire process is eventually amplified.
Therefore, when the metastatic cells and their cloned cells form a detectable mass, micro-metastases have already spread across multiple tissues in the body. Although the tumor cells in these micro-metastases have been in a state of growth quiescence for a long time, the isolated tumor cells still have the ability to proliferate in vitro and form new tumors (105). As these newly disseminated tumor cells have the same origin, the micro-metastases formed by them have similar genetic backgrounds. Each cell of the secondary micro-metastases has the ability to form clones. These clonal metastatic cells in micro-metastases drive the micro-metastases to quickly grow into clinically detectable metastases and ultimately lead to tumor recurrence. In-depth research on the mechanism of CSCs in the recurrence process is expected to produce new treatment strategies aimed at eradicating CSCs and may lead to a better prognosis.
The Role of CSC Exosomes in Cancer Metastasis
As carriers, exosomes play an important role in mediating cell communication and material exchange between CSCs and tumor cells and other cells in the microenvironment, regulating processes such as tumor growth metastasis, drug resistance, EMT, angiogenesis, and immune escape, by transporting tumor-related mRNA, miRNA, proteins, etc. In the TME, exosomes secreted by tumor cells are different from those secreted by normal tissue cells (46). Tumors are complex tissues that rely on communication between different cell types, and CSCs can secrete exosomes, participate in the construction of the TME, and maintain the self-renewal of CSCs and the biological behavior of the tumor. Exosomes secreted by tumor cells increase in the TEM, which is typically acidic (the pH shifts from 7.4 to 6.5, which is typical for tumors), which is associated with the formation of malignant tumor phenotypes (106). As information carriers, exosomes play an important role in cell communication locally and remotely, participate in the mutual transformation between non-CSCs and CSCs, and maintain the dynamic balance of CSCs. These exosomes mediate metastasis to organs by regulating the pre-metastatic microenvironment through different pathways, contains the induction of phenotypic changes and cell differentiation, incorporation of different supporting mesenchymal cells, upregulation of pro-inflammatory gene expression, and induction of an immunosuppressive state. However, exosomes may mediate the re-awakening of dormant ecotopes instead of pre-metastatic ecotone formation (107). Growing evidence indicates that stable microvasculature constitutes a dormant niche, whereas sprouting neovasculature sparks micrometastatic outgrowth (108). Exosomes, especially secreted by CSCs play an important role in cancer progression, and the carried miRNA is considered an important molecular marker for tumor diagnosis and prognosis, especially in lung cancer patients (109). Targeting signal pathways regulated by exosomes could act on CSCs to inhibit the occurrence and development of tumors, which has become a hot topic in recent years.
The Messenger Role of CSC Exosomes in the TEM
CSCs are non-quiescent and dynamically changing solid cell populations. The actual process of CSCs driving tumor progression is complicated; as the tumor progresses, the tumor genome becomes unstable, and the rate at which each generation of cells acquires mutations continues to increase. Therefore, there are genetically heterogeneous subclones in each tumor cell cluster. Accumulated genetic heterogeneity in a specific cell cluster results in different subclones carrying different genetic phenotypic changes. The heterogeneity of CSCs determines the homeostasis of CSCs in a given TEM. The interaction between CSCs and the microenvironment is a key factor in tumor development. Considering the dynamic conversion mechanism of CSCs and non-CSCs as the starting point for research is of great significance to the information transmission of CSCs (110).
CSCs need signal feedback from the TEM to maintain their stemness, regulate the balance between self-renewal and differentiation, and avoid being depleted. Cell communication and substance exchanges among CSCs, tumor cells, and other cells in the TEM are vital for maintaining their dynamic balance. CSCs can influence the surrounding stromal cells through cell-to-cell contact or paracrine signaling molecules. These signals not only recruit cells to the microenvironment, but also change the function and activity of stromal cells. Some differentiated tumor cells (non-CSCs) have lost the characteristics of stem cells and can regain their stem phenotype through dedifferentiation or reprogramming. CSCs and non-CSCs are in a dynamic balance between differentiation and dedifferentiation. This information exchange between cells and the extracellular matrix maintains the stemness of CSCs and promotes tumor survival and development. The interaction between CSCs and their microenvironment is conducive to the maintenance of homeostasis. Exosomes, as information transmitters between cells in the microenvironment of CSCs, play an irreplaceable messenger role.
Tumor Metastasis and EMT
The first step in the localized invasion of tumor cell metastasis is the phenotypic transformation of primary tumor cells. To obtain mobility and invasion capabilities, tumor cells lose their epithelial cell phenotype and undergo EMT. EMT refers to epithelial cells that have lost intercellular adhesion and lack motility differentiation characteristics, thus have the characteristics of mesenchymal cells. This process is considered to be the basis of morphogenesis and is involved in the formation of tissues and organs during animal embryogenesis (111). A similar process occurs during wound healing, indicating that EMT is also important in adulthood (94). Tumor cells migrate and become motile through EMT, which promotes their invasion (112). Epithelial cells undergo continuous cell activities during the EMT process, including loss of top-bottom polarity structure, destruction of cell-to-cell connections, cytoskeleton remodeling, changing cell morphology, and eventually showing mesenchymal and invasive phenotypes, thereby causing increased cell motility and degradation of the extracellular matrix (113). Most malignant tumors are epithelial tumors. The malignant tumor cells that undergo EMT will gain enhanced migration and invasion abilities, invade the surrounding extracellular matrix, and eventually metastasize to distant sites. EMT plays an important role in the invasive phenotype of colon cancer, thyroid cancer, and breast cancer (114). Once the tumor cells begin to undergo EMT, their phenotype changes and they acquire mobility and the ability to invade adjacent cells, which can promote tumor metastasis. The EMT effect under physiological and pathological conditions not only changes cell morphology and mobility, but also changes the cell gene expression profile. In human tumors, the progression of sarcoma carcinosarcomas is related to EMT; most cancers have partial EMT features and express both epithelial and mesenchymal markers (115). Xenotransplantation experiments in vivo have shown that EMT is associated with tumor metastasis in vivo (116). EMT-TFs are not only involved in migration and invasion, but also in inhibiting cellular senescence and apoptosis as well as attenuating cell cycle progression and resistance to radiotherapy and chemotherapy (104). The EMT effect of tumor pathogenesis has been increasingly reported to be related to the local infiltration and metastasis of tumor lesions (111).
EMT cells have the ability to resist apoptosis, chemotherapy, and immunotherapy. EMT induces immune tolerance and causes malignant tumor cells to evade immune surveillance. An increasing number of studies have shown that EMT is related to CSC characteristics (103). In the pathogenesis of cancer, tumor cells receive signals transmitted by exosomes secreted by other tumor cells, and induce changes in their phenotype, so that they can acquire the ability of cell proliferation, migration, and invasion. The nuclear driver of EMT has growth inhibition and stimulation functions in different microenvironments. EMT participates in multiple signaling pathways involving multiple related proteins and their transcription. Non-coding RNA, differential splicing, translation, and post-translational control mainly participate in the regulation of EMT (94). Exosomes are rich in small non-coding RNAs or miRNAs, which are now widely regarded as effective modifiers of gene expression, enabling cells to respond quickly to new environments, and are directly or indirectly related to EMT-related miRNAs (117). The miR-200 family has been shown to be downregulated in normal human and mouse mammary stem and progenitor cells (118). EMT is associated with the loss of p53 function. miRNAs are important mediators of p53 to regulate EMT and are related to the expression of the tumor stem cell phenotype of tumor cells and affect tumor proliferation and invasion (119, 120). Therefore, p53 transactivates miR‐200c through direct binding to the miR‐200c promoter. Loss of p53 cells leads to decreased expression of miRNA and activates the EMT programme, accompanied by stemness properties cells increased (121). Tumor cells secrete exosomes containing the miR-200 family, which play an important role in the regulation of the tumor microenvironment in tumor metastasis. As messengers of information exchange between cells, tumor stem cell exosomes are loaded with these non-coding RNAs, which act on the inhibition or expression of EMT-TF and reflecting the important role of EMT in the cancer process, which has research significance. Exosomes take up miRNAs in cancers plays important function in TEM. The cell interaction of exosome miRNA in cancers function shown in Table 3.
EMT is closely associated with cancer progression (112, 127). While epithelial cells undergo EMT, the dynamic changes in cell morphology from the epithelial to mesenchymal phenotype are covered, and epithelial cells transform into migratory and invasive cells. When tumor cells have an EMT effect, not only does the morphology change, but the gene expression profile also changes. Cadherin and vimentin are EMT-TFs, and the stimulating signal pathways are stimulated by transcriptional reprogramming and thus transformed into a regulatory network (128). The expression of epithelial cell markers (E-cadherin and cytokeratin) is inhibited, and the expression of a mesenchymal cytoskeleton intermediate fiber component (vimentin) is induced, which is a prerequisite for the progression of malignant tumors. The cytoplasmic domain of cadherin interacts with a catenin-based complex, which binds to the actin cytoskeleton to regulate adhesion-dependent signal transduction. Loss of function may lead to cancer progression through increased proliferation, invasion, and/or metastasis (94). Tumor-derived exosomes are involved in the EMT regulation process, play a role in intercellular signal transduction in the tumor microenvironment, and regulate recipient cells (129–131).
Tumor Recurrence and Mesenchymal-Epithelial Transformation (MET)
EMT is an abnormal trans-differentiation program induced by exogenous transcription factors (transforming growth factor, fibroblast growth factor), key signaling pathways (the Hh, notch, or NF-κB signaling pathways), and cytokines in the TME. Extracellular signal by exosome mediated stimulation promotes the overexpression of EMT transcription factors, which trigger the EMT by suppressing epithelial phenotypes, enhancing mesenchymal properties, and inducing the degradation of the basement membrane and extracellular matrix (132). The phenotype induced by EMT is reversible, causing the tumor to acquire a highly malignant growth state. When the tumor cells have completed the process of invasion and metastasis, they have to go through the process of MET to restore the epithelial-like cell phenotype. This reversible process indicates that EMT is usually triggered by microenvironmental signals in which tumor cells are located. Exosome changes its messages to guide tumor cells changing. Tumor cells at the pre-aggressive edge of the primary tumor can obtain heterogeneous signals from the adjacent reactive matrix, which is gradually formed during tumorigenesis and tumor development. Once the tumor cells leave the primary tumor and migrate to the distal end, the interstitial microenvironment that they are located in stops to release EMT-induced signals continuously, and some tumor cells undergo MET conversion and restore the phenotype of their primary tumor. Tumor cells that restore the epithelial phenotype clone a large number of them after interacting with the cells of the tumor microenvironment by exosomes, and finally the tumor recurs after the metastasis is complete.
Except for partial activation, the EMT of tumor cells is usually reversibly activated, and cancer cells can be restored to the epithelial state through the MET during tumor progression. During cancer invasion, tumor cells undergo a dynamic transition between epithelial mesenchymalization and mesenchymal epithelialization (133). Tumor stem cell-secreted exosomes play an important role as messengers in the dynamic transition between EMT and MET in tumor cells. Cancer cells can flexibly activate EMT and MET during cancer cell invasion. The MET is synergistically induced by a variety of microenvironmental signals, which can lead to a decrease in the expression of the epithelial cell marker E-cadherin and an increase in the expression of mesenchymal cell proteins, leading to the loss of adhesion junctions and epithelial polarity in epithelial cells and the acquired invasive mesenchymal phenotype. This is the reverse process of transformation of epithelial cells into mesenchymal cells. In addition, apart from morphological changes, some cancer cells also induce the initial state of the CSC during the EMT process. The reversibility and plasticity of MET and epithelial mesenchymal stem cells are crucial. The reverse process in embryogenesis and cancer progression (MET) is critical for the final developmental cell differentiation and post-metastatic clonal growth (128, 134). Signal transmission by exosomes between tumors in the microenvironment plays an important role in the occurrence and development of tumors. The cancer cells especially CSCs-derived exosome mediated invasion and metastasis by EMT and MET during cancer progression are shown in Figure 1.
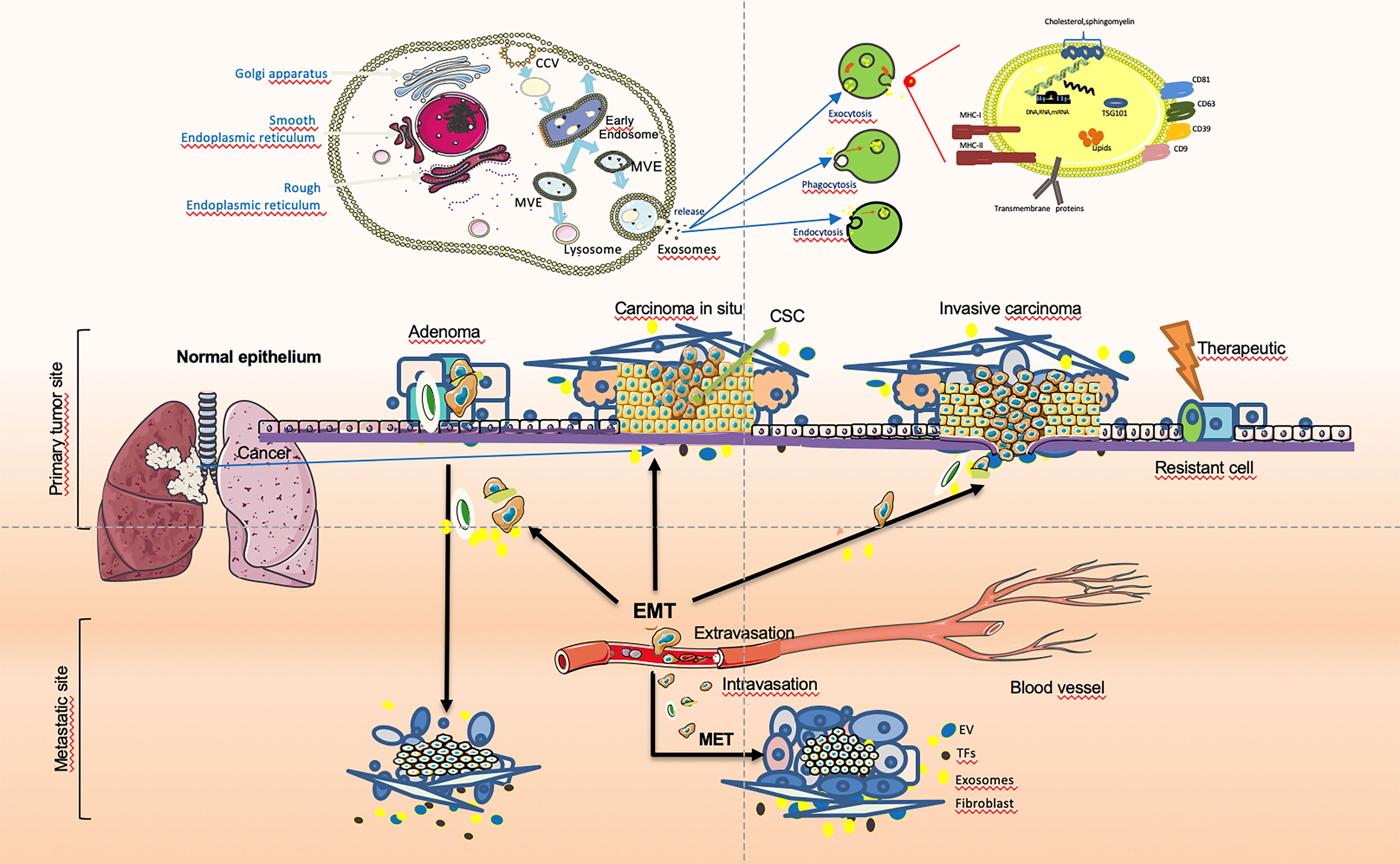
Figure 1 Cancer cells especially cancer stem cells(CSCs)-derived exosome-mediated EMT during cancer progression. In tumor cells, epithelial to mesenchymal transition (EMT) and transcription factors (EMT-TFs), especially tumor-derived exosomes, assign stem cell (SC) characteristics to dedifferentiated tumor cells that are called cancer stem cells (CSCs). The dissemination of tumor cells in the primary tumor and migration after the breakdown of the basement membrane (BM) can be achieved That cells initiates invasion when integrated necessary genetic aberrations and simulated by regarding signals from exosome origins at the tumor. Based on this, providing signals and maintaining the mesenchymal state of metastatic cells may be a positive contribution of EMT.EMT features may promote resistance in the period of anti-tumor therapy, leading to recurrence and poor prognosis. The imbalance between relevant regulatory networks and activated oncogenic approaches at different cancer stages may determine the extent of EMT. In particular CSC in tumor cells-secreted exosomes play an important role as messengers in the dynamic transition between EMT and MET in tumor cells. Cancer cells can activate transfer between EMT and MET while cancer cell invasion.
CSC Exosomes Regulate the Dynamic Balance of the Tumor Niche
The differences between CSCs and non-CSCs can be attributed to the biological process of EMT (135). EMT activation requires intercellular signal transmission in normal cells and tumor cells. Signal transmission in the extracellular matrix is mainly carried out in the form of secretions, which are released by various types of cells and interact with nearby epithelial cells, leading to the intracellular signal cascade transmission of epithelial cells. These pathways lead to the expression of transcripts that coordinate the EMT expression and regulate the expression of various target genes, which finally cause the expression of mesenchymal cell characteristics.
The EMT process contributes to the progression of many cancer types (94, 136, 137). Cancer cells lose epithelial characteristics, gain invasive characteristics and stem cell-like characteristics, and also have epithelial cells that acquire stem-like properties (95). Epithelial cells may enter the stem cell state, mesenchymal state, or both states dynamically, depending on the EMT-TF process. Normal stem cells and CSCs produced in epithelial tissues usually show a mixture of epithelial and mesenchymal features, indicating that the process can only be partially promoted. MET enables cells to restore their ability to grow cohesively, thereby enabling them to colonize the site of secondary tumors. Therefore, MET is necessary for the late stage of tumor cell metastasis and colonization (138). EMT occurs in a gradual manner, showing intermediate morphological and epigenetic characteristics between epithelial and mesenchymal cells (139). However, cells in the mixed epithelial/mesenchymal phenotype tend to display the characteristics of epithelial cells and mesenchymal cells, and these hybrid epithelial/mesenchymal phenotypes are related to the collective cell migration of cancer cells (140). Exosomes secreted by CSCs play an important messenger role in this process and participate in the regulation of this transformation. The exosomes derived from cancer cells mediated invasion and metastasis play an important role during cancer progression are shown in Figure 2.
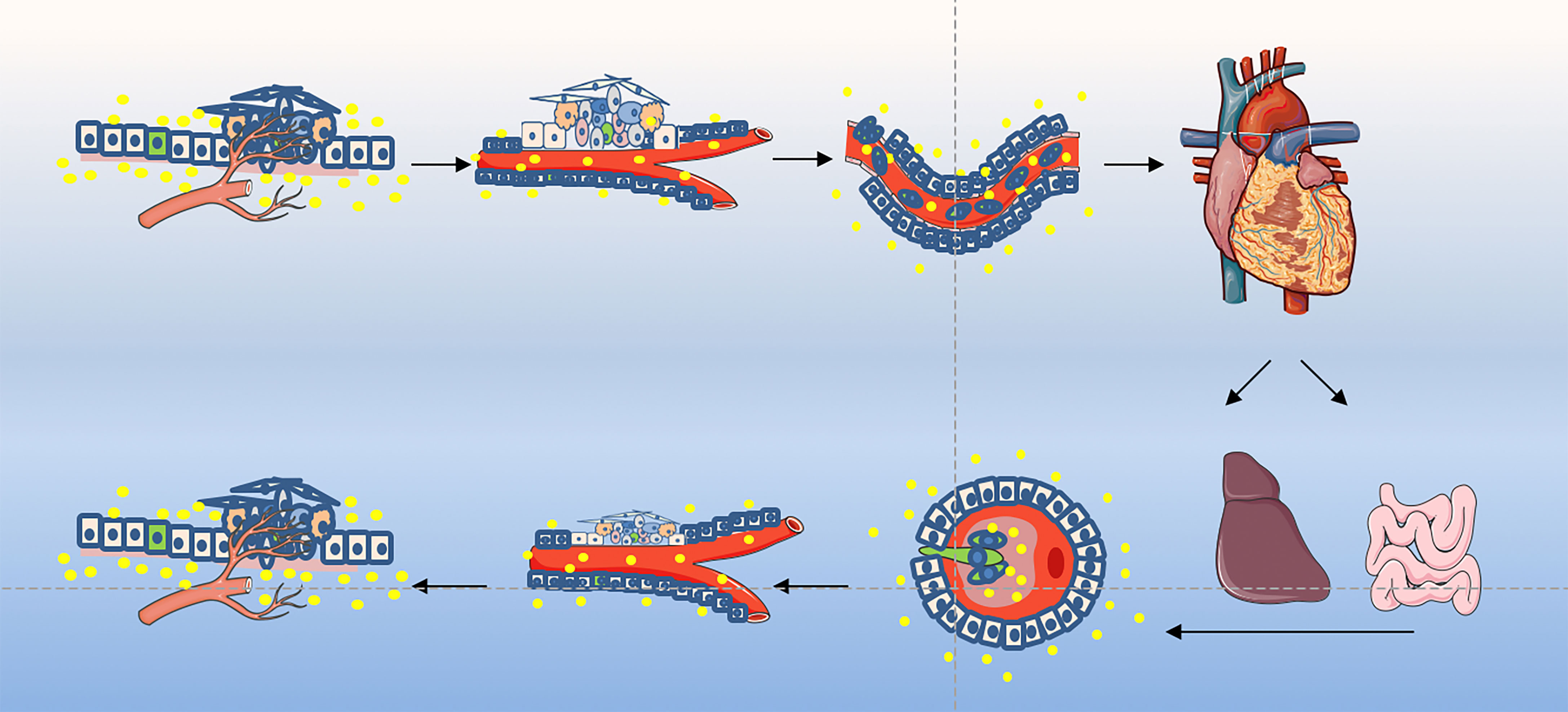
Figure 2 Exosome play an important role in tumor invasion–metastasis cascade. This depiction of the invasion–metastasis cascade ascribes distinct steps to the overall process. The initial step in local invasion is for the in situ cancer cells to break through the basement membrane. They may then infiltrate into the lymphatic vessels or microvasculature. The latter may then transport these cancer cells through the systemic circulation to distant anatomic sites, where they may become trapped and subsequently exude, forming dormant micrometastases. Some micrometastases may eventually acquire the ability to colonize the tissue in which they land, allowing them to form macroscopic metastases. The final step - colonization - appears to be the least efficient of all. The little of successfully completing all steps of this cascade process explains why the likelihood of any individual cancer cell leaving the primary tumor and becoming the founder of a distant macroscopic metastasis is low. More suggestions proposed an alternative description involving two main stages: the first enables the physical dissemination of cancer cells from the core of the primary tumor to the parenchyma of a distant tissue, while the second—colonization—depends on the adaptation of disseminated cancer cells to the microenvironment of this tissue. Exosomes as an essential part taking into the messages during the whole process.
Application Prospects of CSC Exosomes in Cancer
The biological behavior of cancer cell invasion and metastasis is the same as that of other biological phenotypes, which are triggered by gene function. The propensity for metastasis is related to the expression patterns of specific genes in human breast cancer. Most cells in the primary tumor show this characteristic gene expression profile (141). In other words, during the multi-stage tumor progression, the primary tumor continues to accumulate metastatic potential, and the tumor as a whole shows a characteristic expression pattern of metastasis.
In recent years there has been an increasing number of studies on the biological function of exosomes. Exosomes originate from various types of cellular secretion and are present in almost all body fluids. As a vehicle for intercellular communication, they can play an essential role in the physiological and pathological course of disease. As exosomes can be encapsulated in various contents, including lipids, proteins and RNA, they can signal and drive metastasis to specific recipient cells or tissues and organs. These exosomes, which are released into the tumor microenvironment, are received by the recipient cells, thereby inducing intracellular signaling and altered recipient cell physiology. Exosomes can occur organ-specific and direct organogenic metastasis of different metastatic tumors before tumor cells, especially tumor stem cells, reach the target organ. Exosomes are particularly important for supporting the formation of distal organogenic pre-metastatic niches. Cancer is the most common type of human tumor. The malignant epithelial cells in the tumor need to undergo EMT to acquire the ability to invade and metastasize. By blocking the expression of key regulatory factors, EMT plays an important role in the genesis of tumor EMT, MET, CSCs, and tumor metastasis to promote tumor proliferation, invasion, adhesion, angiogenesis, and acquired drug resistance, thereby affecting the process of tumor development. Metastatic dormancy is a challenge in tumor research; for instance, in breast cancer, metastases are found decades after the removal of the primary tumor, due to the long incubation period and multiple micro-metastases occurring.
Exosomes secreted by tumor stem cells are regulated through the tumor stem cell-tumor microenvironment-target tissue interface, potentially serving as metastasis markers and promising as entry points for blocking tumor metastasis.
The study of tumor stem cell exosomes facilitates the in-depth understanding of the mechanism of metastasis, which will hopefully lead to new treatment avenues. CSC exosomes, which are important in the promotion of CSCs and tumor foci, the effect of primary tumors and EMT, and the recurrence of metastases and cancer, have important research value for exploring cancer progression and tumor treatment. The personalized and pinpoint treatment of anti-tumors further raises the requirement to diagnose the mechanisms of tumor metastasis and recurrence. The close relationship between exosomes and metastasis is predicted to be a diagnostic marker for tumor recurrence; targeting the signal transduction of exosomes for interventional blockade will likely be useful for clinical application of therapeutic new therapies; the targeting ability of exosomes can also be utilized to target the contents of exosomes as drug carriers for research, which is expected to target drug delivery for effective treatment of recurrent tumors. The in-depth study of the effect of tumor stem cell exosomes on the regulation of tumor metastasis and recurrence in the process of cancer has far-reaching significance for the precise diagnosis of anti-tumor phased treatment, prognosis judgment, and other fields.
Author Contributions
KL conceptualized and wrote the article. XG, BK, DW, and YW revised the article. YW revised and finalized the article. All authors contributed to the article and approved the submitted version.
Funding
Project supported by Jilin Province Science and Technology Support Program [Grant number 20200404121YY]; Education Department of Jilin Province [Grant number JJKH20201122KJ]; Guangzhou Science and Technology Planning Project, China (201904010443).
Conflict of Interest
The authors declare that the research was conducted in the absence of any commercial or financial relationships that could be construed as a potential conflict of interest.
Publisher’s Note
All claims expressed in this article are solely those of the authors and do not necessarily represent those of their affiliated organizations, or those of the publisher, the editors and the reviewers. Any product that may be evaluated in this article, or claim that may be made by its manufacturer, is not guaranteed or endorsed by the publisher.
Abbreviations
CSCs, cancer stem cells; EMT, endothelial-mesenchymal transition; EVs, extracellular vesicles; ILVs, intraluminal vesicles; MET, mesenchymal-epithelial transformation; miRNAs, microRNAs; MVBs, multivesicular bodies; MVEs, multivesicular endosomes; TME, tumor microenvironment.
References
1. Théry C, Witwer KW, Aikawa E, Alcaraz MJ, Anderson JD, Andriantsitohaina R, et al. Minimal Information for Studies of Extracellular Vesicles 2018 (MISEV2018): A Position Statement of the International Society for Extracellular Vesicles and Update of the MISEV2014 Guidelines. J Extracell Vesicles (2018) 7(1):1535750. doi: 10.1080/20013078.2018.1535750
2. Cocucci E, Meldolesi J. Ectosomes and Exosomes: Shedding the Confusion Between Extracellular Vesicles. Trends Cell Biol (2015) 25(6):364–72. doi: 10.1016/j.tcb.2015.01.004
3. Kalra H, Drummen GPC, Mathivanan S. Focus on Extracellular Vesicles: Introducing the Next Small Big Thing. Int J Mol Sci (2016) 17(2):170. doi: 10.3390/ijms17020170
4. Lötvall J, Hill AF, Hochberg F, Buzás EI, Vizio DD, Gardiner C, et al. Minimal Experimental Requirements for Definition of Extracellular Vesicles and Their Functions: A Position Statement From the International Society for Extracellular Vesicles. J Extracell Vesicles (2014) 3(1):26913. doi: 10.3402/jev.v3.26913
5. Valadi H, Ekström K, Bossios A, Sjöstrand M, Lee JJ, Lötvall JO. Exosome-Mediated Transfer of mRNAs and microRNAs Is a Novel Mechanism of Genetic Exchange Between Cells. Nat Cell Biol (2007) 9(6):654–9. doi: 10.1038/ncb1596
6. Théry C, Zitvogel L, Amigorena S. Exosomes: Composition, Biogenesis and Function. Nat Rev Immunol (2002) 2(8):569–79. doi: 10.1038/nri855
7. Colombo M, Raposo G, Théry C. Biogenesis, Secretion, and Intercellular Interactions of Exosomes and Other Extracellular Vesicles. Annu Rev Cell Dev Biol (2014) 30:255–89. doi: 10.1146/annurev-cellbio-101512-122326
8. Raposo G, Stoorvogel W. Extracellular Vesicles: Exosomes, Microvesicles, and Friends. J Cell Biol (2013) 200(4):373–83. doi: 10.1083/jcb.201211138
9. El Andaloussi S, Mäger I, Breakefield XO, Wood MJA. Extracellular Vesicles: Biology and Emerging Therapeutic Opportunities. Nat Rev Drug Discov (2013) 12(5):347–57. doi: 10.1038/nrd3978
10. Xu R, Rai A, Chen M, Suwakulsiri W, Greening DW, Simpson RJ. Extracellular Vesicles in Cancer — Implications for Future Improvements in Cancer Care. Nat Rev Clin Oncol (2018) 15(10):617–38. doi: 10.1038/s41571-018-0036-9
11. Mathivanan S, Ji H, Simpson RJ. Exosomes: Extracellular Organelles Important in Intercellular Communication. J Proteomics (2010) 73(10):1907–20. doi: 10.1016/j.jprot.2010.06.006
12. Anderson HC, Mulhall D, Garimella R. Role of Extracellular Membrane Vesicles in the Pathogenesis of Various Diseases, Including Cancer, Renal Diseases, Atherosclerosis, and Arthritis. Lab Investig (2010) 90(11):1549–57. doi: 10.1038/labinvest.2010.152
13. Sharma A. Role of Stem Cell Derived Exosomes in Tumor Biology. Int J Cancer (2018) 142(6):1086–92. doi: 10.1002/ijc.31089
14. Bjørge IM, Kim SY, Mano JF, Kalionis B, Chrzanowski W. Extracellular Vesicles, Exosomes and Shedding Vesicles in Regenerative Medicine-A New Paradigm for Tissue Repair. Biomater Sci (2018) 6(1):60–78. doi: 10.1039/c7bm00479f
15. Xian X, Gong Q, Li C, Guo B, Jiang H. Exosomes With Highly Angiogenic Potential for Possible Use in Pulp Regeneration. J Endod (2018) 44(5):751–8. doi: 10.1016/j.joen.2017.12.024
16. Bastos N, Ruivo CF, da Silva S, Melo SA. Exosomes in Cancer: Use Them or Target Them? Semin Cell Dev Biol (2018) 78:13–21. doi: 10.1016/j.semcdb.2017.08.009
17. Fitts CA, Ji N, Li Y, Tan C. Exploiting Exosomes in Cancer Liquid Biopsies and Drug Delivery. Adv Healthc Mater (2019) 8(6):1–8. doi: 10.1002/adhm.201801268
18. Hanahan D, Weinberg RA. Hallmarks of Cancer: The Next Generation. Cell (2011) 144(5):646–74. doi: 10.1016/j.cell.2011.02.013
19. Pan Z, Cai J, Lin J, Zhou H, Peng J, Liang J, et al. A Novel Protein Encoded by Circfndc3b Inhibits Tumor Progression and EMT Through Regulating Snail in Colon Cancer. Mol Cancer (2020) 19(1):1–15. doi: 10.1186/s12943-020-01179-5
20. Kamerkar S, Lebleu VS, Sugimoto H, Yang S, Ruivo CF, Melo SA, et al. Exosomes Facilitate Therapeutic Targeting of Oncogenic KRAS in Pancreatic Cancer. Nature (2017) 546(7659):498–503. doi: 10.1038/nature22341
21. Li L, Cao B, Liang X, Lu S, Luo H, Wang Z, et al. Microenvironmental Oxygen Pressure Orchestrates an Anti- and Pro-Tumoral γδ T Cell Equilibrium via Tumor-Derived Exosomes. Oncogene (2019) 38(15):2830–43. doi: 10.1038/s41388-018-0627-z
22. Li XJ, Ren ZJ, Tang JH, Yu Q. Exosomal MicroRNA MiR-1246 Promotes Cell Proliferation, Invasion and Drug Resistance by Targeting CCNG2 in Breast Cancer. Cell Physiol Biochem (2017) 44(5):1741–8. doi: 10.1159/000485780
23. Yang H, Fu H, Wang B, Zhang X, Mao J, Li X, et al. Exosomal miR-423-5p Targets SUFU to Promote Cancer Growth and Metastasis and Serves as a Novel Marker for Gastric Cancer. Mol Carcinog (2018) 57(9):1223–36. doi: 10.1002/mc.22838
24. Qu J, Qu X, Zhao M, Teng Y, Zhang Y, Hou K, et al. Gastric Cancer Exosomes Promote Tumour Cell Proliferation Through PI3K/Akt and MAPK/ERK Activation. Dig Liver Dis (2009) 41(12):875–80. doi: 10.1016/j.dld.2009.04.006
25. Fang T, Lv H, Lv G, Li T, Wang C, Han Q, et al. Tumor-Derived Exosomal miR-1247-3p Induces Cancer-Associated Fibroblast Activation to Foster Lung Metastasis of Liver Cancer. Nat Commun (2018) 9(1):1–14. doi: 10.1038/s41467-017-02583-0
26. Yu Y, Abudula M, Li C, Chen Z, Zhang Y, Chen Y. Icotinib-Resistant HCC827 Cells Produce Exosomes With mRNA MET Oncogenes and Mediate the Migration and Invasion of NSCLC. Respir Res (2019) 20(1):1–16. doi: 10.1186/s12931-019-1202-z
27. Mikamori M, Yamada D, Eguchi H, Hasegawa S, Kishimoto T, Tomimaru Y, et al. MicroRNA-155 Controls Exosome Synthesis and Promotes Gemcitabine Resistance in Pancreatic Ductal Adenocarcinoma. Sci Rep (2017) 7:1–14. doi: 10.1038/srep42339
28. Chiba M, Kubota S, Sato K, Monzen S. Exosomes Released From Pancreatic Cancer Cells Enhance Angiogenic Activities via Dynamin-Dependent Endocytosis in Endothelial Cells In Vitro. Sci Rep (2018) 8(1):1–9. doi: 10.1038/s41598-018-30446-1
29. Zeng Z, Li Y, Pan Y, Lan X, Song F, Sun J, et al. Cancer-Derived Exosomal miR-25-3p Promotes Pre-Metastatic Niche Formation by Inducing Vascular Permeability and Angiogenesis. Nat Commun (2018) 9(1):5395. doi: 10.1038/s41467-018-07810-w
30. Webber JP, Spary LK, Sanders AJ, Chowdhury R, Jiang WG, Steadman R, et al. Differentiation of Tumour-Promoting Stromal Myofibroblasts by Cancer Exosomes. Oncogene (2015) 34(3):319–33. doi: 10.1038/onc.2013.560
31. Todorova D, Simoncini S, Lacroix R, Sabatier F, Dignat-George F. Extracellular Vesicles in Angiogenesis. Circ Res (2017) 120(10):1658–73. doi: 10.1161/CIRCRESAHA.117.309681
32. Meehan K, Vella LJ. The Contribution of Tumour-Derived Exosomes to the Hallmarks of Cancer. Crit Rev Clin Lab Sci (2016) 53(2):121–31. doi: 10.3109/10408363.2015.1092496
33. Peinado H, Zhang H, Matei IR, Costa-Silva B, Hoshino A, Rodrigues G, et al. Pre-Metastatic Niches: Organ-Specific Homes for Metastases. Nat Rev Cancer (2017) 17(5):302–17. doi: 10.1038/nrc.2017.6
34. Raposo G, Nijman HW, Stoorvogel W, Liejendekker R, Harding CV, Melief CJ, et al. B Lymphocytes Secrete Antigen-Presentingvesicles. J Exp Med (1996) 183(3):1161–72. doi: 10.1084/jem.183.3.1161
35. He C, Zheng S, Luo Y, Wang B. Exosome Theranostics: Biology and Translational Medicine. Theranostics (2018) 8(1):237–55. doi: 10.7150/thno.21945
36. Sheridan C. Exosome Cancer Diagnostic Reaches Market. Nat Biotechnol (2016) 34(4):359–60. doi: 10.1038/nbt0416-359
37. Shao H, Im H, Castro CM, Breakefield X, Weissleder R, Lee H. New Technologies for Analysis of Extracellular Vesicles. Chem Rev (2018) 118(4):1917–50. doi: 10.1021/acs.chemrev.7b00534
38. Johnstone RM, Adam M, Pan BT. The Fate of the Transferrin Receptor During Maturation of Sheep Reticulocytes In Vitro. Can J Biochem Cell Biol (1984) 62(11):1246–54. doi: 10.1139/o84-159
39. Johnstone RM, Adam M, Hammond JR, Orr L, Turbide C. Vesicle Formation During Reticulocyte Maturation. Association of Plasma Membrane Activities With Released Vesicles (Exosomes). J Biol Chem (1987) 262(19):9412–20. doi: 10.1016/s0021-9258(18)48095-7
40. Kalluri R, LeBleu VS. The Biology, Function, and Biomedical Applications of Exosomes. Sci (80-) (2020) 367(6478):6977. doi: 10.1126/science.aau6977
41. Zhang HG, Grizzle WE. Exosomes: A Novel Pathway of Local and Distant Intercellular Communication That Facilitates the Growth and Metastasis of Neoplastic Lesions. Am J Pathol (2014) 184(1):28–41. doi: 10.1016/j.ajpath.2013.09.027
42. Subra C, Laulagnier K, Perret B, Record M. Exosome Lipidomics Unravels Lipid Sorting at the Level of Multivesicular Bodies. Biochimie (2007) 89(2):205–12. doi: 10.1016/j.biochi.2006.10.014
43. Kreimer S, Belov AM, Ghiran I, Murthy SK, Frank DA, Ivanov AR. Mass-Spectrometry-Based Molecular Characterization of Extracellular Vesicles: Lipidomics and Proteomics. J Proteome Res (2015) 14(6):2367–84. doi: 10.1021/pr501279t
44. Borges FT, Reis LA, Schor N. Extracellular Vesicles: Structure, Function, and Potential Clinical Uses in Renal Diseases. Braz J Med Biol Res (2013) 46(10):824–30. doi: 10.1590/1414-431X20132964
45. Shah R, Patel T, Freedman JE. Circulating Extracellular Vesicles in Human Disease. N Engl J Med (2018) 379(10):958–66. doi: 10.1056/nejmra1704286
46. Milane L, Singh A, Mattheolabakis G, Suresh M, Amiji MM. Exosome Mediated Communication Within the Tumor Microenvironment. J Control Rel (2015) 219:278–94. doi: 10.1016/j.jconrel.2015.06.029
47. Zhao H, Yang L, Baddour J, Achreja A, Bernard V, Moss T, et al. Tumor Microenvironment Derived Exosomes Pleiotropically Modulate Cancer Cell Metabolism. Elife (2016) 5:1–27. doi: 10.7554/eLife.10250
48. Villarroya-Beltri C, Baixauli F, Gutiérrez-Vázquez C, Sánchez-Madrid F, Mittelbrunn M. Sorting It Out: Regulation of Exosome Loading. Semin Cancer Biol (2014) 28:3–13. doi: 10.1016/j.semcancer.2014.04.009
49. Record M, Carayon K, Poirot M, Silvente-Poirot S. Exosomes as New Vesicular Lipid Transporters Involved in Cell-Cell Communication and Various Pathophysiologies. Biochim Biophys Acta - Mol Cell Biol Lipids (2014) 1841(1):108–20. doi: 10.1016/j.bbalip.2013.10.004
50. Villarroya-Beltri C, Gutiérrez-Vázquez C, Sánchez-Cabo F, Perez-Hernandez D, Vazquez J, Martin-Cofreces N, et al. Sumoylated Hnrnpa2b1 Controls the Sorting of miRNAs Into Exosomes Through Binding to Specific Motifs. Nat Commun (2013) 4:1–10. doi: 10.1038/ncomms3980
51. Chevillet JR, Kang Q, Ruf IK, Briggsa HA, Vojtechc LN, Hughes SM, et al. Quantitative and Stoichiometric Analysis of the microRNA Content of Exosomes. Proc Natl Acad Sci USA (2014) 111(41):14888–93. doi: 10.1073/pnas.1408301111
52. Mathieu M, Martin-Jaular L, Lavieu G, Théry C. Specificities of Secretion and Uptake of Exosomes and Other Extracellular Vesicles for Cell-to-Cell Communication. Nat Cell Biol (2019) 21(1):9–17. doi: 10.1038/s41556-018-0250-9
53. Zhang Y, Liu Y, Liu H, Tang WH. Exosomes: Biogenesis, Biologic Function and Clinical Potential. Cell Biosci (2019) 9(1):1–18. doi: 10.1186/s13578-019-0282-2
54. Clara JA, Monge C, Yang Y, Takebe N. Targeting Signalling Pathways and the Immune Microenvironment of Cancer Stem Cells — A Clinical Update. Nat Rev Clin Oncol (2020) 17(4):204–32. doi: 10.1038/s41571-019-0293-2
55. Ayob AZ, Ramasamy TS. Cancer Stem Cells as Key Drivers of Tumour Progression. J BioMed Sci (2018) 25(1):1–18. doi: 10.1186/s12929-018-0426-4
56. Lapidot T, Sirard C, Vormoor J, Murdoch B, Hoang T, Caceres-Cortes J, et al. A Cell Initiating Human Acute Myeloid Leukaemia After Transplantation Into SCID Mice. Nature (1994) 367(6464):645–8. doi: 10.1038/367645a0
57. Vermeulen L, Sprick MR, Kemper K, Stassi G, Medema JP. Cancer Stem Cells - Old Concepts, New Insights. Cell Death Differ (2008) 15(6):947–58. doi: 10.1038/cdd.2008.20
58. Konrad CV, Murali R, Varghese BA, Nair R. The Role of Cancer Stem Cells in Tumor Heterogeneity and Resistance to Therapy. Can J Physiol Pharmacol (2017) 95(1):1–15. doi: 10.1139/cjpp-2016-0079
59. Peitzsch C, Tyutyunnykova A, Pantel K, Dubrovska A. Cancer Stem Cells: The Root of Tumor Recurrence and Metastases. Semin Cancer Biol (2017) 44:10–24. doi: 10.1016/j.semcancer.2017.02.011
60. Zhou BBS, Zhang H, Damelin M, Geles KG, Grindley JC, Dirks PB. Tumour-Initiating Cells: Challenges and Opportunities for Anticancer Drug Discovery. Nat Rev Drug Discov (2009) 8(10):806–23. doi: 10.1038/nrd2137
61. Singh SK, Clarke ID, Terasaki M, Bonn VE, Hawkins C, Squire J, et al. Identification of a Cancer Stem Cell in Human Brain Tumors. Cancer Res (2003) 63(18):5821–8.
62. Hemmati HD, Nakano I, Lazareff JA, Michael MS, Daniel HG, Marianne BF, et al. Cancerous Stem Cells can Arise From Pediatric Brain Tumors. Proc Natl Acad Sci USA (2003) 100(25):15178–83. doi: 10.1073/pnas.2036535100
63. Al-Hajj M, Wicha MS, Benito-Hernandez A, Morrison SJ, Clarke MF. Prospective Identification of Tumorigenic Breast Cancer Cells. Proc Natl Acad Sci USA (2003) 100(7). doi: 10.1073/pnas.0530291100
64. Pattabiraman DR, Weinberg RA. Tackling the Cancer Stem Cells-What Challenges Do They Pose? Nat Rev Drug Discov (2014) 13(7):497–512. doi: 10.1038/nrd4253
65. Merlos-Suárez A, Barriga FM, Jung P, Iglesias M, Céspedes MV, Rossell D, et al. The Intestinal Stem Cell Signature Identifies Colorectal Cancer Stem Cells and Predicts Disease Relapse. Cell Stem Cell (2011) 8(5):511–24. doi: 10.1016/j.stem.2011.02.020
66. Kaveh K, Kohandel M, Sivaloganathan S. Replicator Dynamics of Cancer Stem Cell: Selection in the Presence of Differentiation and Plasticity. Math Biosci (2016) 272:64–75. doi: 10.1016/j.mbs.2015.11.012
67. Zhao Z, Zuber J, Diaz-Flores E, Lintault L, Kogan SC, Shannon K, et al. P53 Loss Promotes Acute Myeloid Leukemia By Enabling Aberrant Self-Renewal. Genes Dev (2010) 24(13):1389–402. doi: 10.1101/gad.1940710
68. Espinoza I, Miele L. Deadly Crosstalk: Notch Signaling at the Intersection of EMT and Cancer Stem Cells. Cancer Lett (2013) 341(1):41–5. doi: 10.1016/j.canlet.2013.08.027
69. Chaffer CL, Brueckmann I, Scheel C, Kaestli AJ, Wiggins PA, Rodrigues LO, et al. Normal and Neoplastic Nonstem Cells can Spontaneously Convert to a Stem-Like State. Proc Natl Acad Sci USA (2011) 108(19):7950–5. doi: 10.1073/pnas.1102454108
70. Ge Y, Gomez NC, Adam RC, Nikolova M, Yang H, Verma A, et al. Stem Cell Lineage Infidelity Drives Wound Repair and Cancer. Cell (2017) 169(4):636–650.e14. doi: 10.1016/j.cell.2017.03.042
71. Visvader JE, Lindeman GJ. Cancer Stem Cells: Current Status and Evolving Complexities. Cell Stem Cell (2012) 10(6):717–28. doi: 10.1016/j.stem.2012.05.007
72. Ishizawa K, Rasheed ZA, Karisch R, Wang Q, Kowalski J, Susky E, et al. Tumor-Initiating Cells Are Rare in Many Human Tumors. Cell Stem Cell (2010) 7(3):279–82. doi: 10.1016/j.stem.2010.08.009
73. Schulenburg A, Blatt K, Cerny-Reiterer S, Sadovnik I, Herrmann H, Marian B, et al. Cancer Stem Cells in Basic Science and in Translational Oncology: Can We Translate Into Clinical Application? J Hematol Oncol (2015) 8(1):1–21. doi: 10.1186/s13045-015-0113-9
74. Sachs N, Clevers H. Organoid Cultures for the Analysis of Cancer Phenotypes. Curr Opin Genet Dev (2014) 24(1):68–73. doi: 10.1016/j.gde.2013.11.012
75. Desai A, Webb B, Gerson SL. CD133+ Cells Contribute to Radioresistance via Altered Regulation of DNA Repair Genes in Human Lung Cancer Cells. Radiother Oncol (2014) 110(3):538–45. doi: 10.1016/j.radonc.2013.10.040
76. Eramo A, Lotti F, Sette G, Pilozzi E, Biffoni M, Virgilio AD, et al. Identification and Expansion of the Tumorigenic Lung Cancer Stem Cell Population. Cell Death Differ (2008) 15(3):504–14. doi: 10.1038/sj.cdd.4402283
77. Prabavathy D, Swarnalatha Y, Ramadoss N. Lung Cancer Stem Cells-Origin, Characteristics and Therapy. Stem Cell Investig (2018) 5(2):1–9. doi: 10.21037/sci.2018.02.01
78. Prabavathy D, Ramadoss N. Heterogeneity of Small Cell Lung Cancer Stem Cells. Adv Exp Med Biol (2019) 1139:41–57. doi: 10.1007/978-3-030-14366-4_3
79. Bertolini G, Roz L, Perego P, Tortoreto M, Fontanella E, Gatti L, et al. Highly Tumorigenic Lung Cancer CD133-Positive Cells Display Stem-Like Features and Are Spared by Cisplatin Treatment Giulia. Proc Natl Acad Sci USA (2009) 106(38):16281–86. doi: 10.1073/pnas.0905653106
80. Singh SK, Hawkins C, Clarke ID, Squire JA, Bayani J, Hide T, et al. Identification of Human Brain Tumour Initiating Cells. Nature (2004) 432:396–401. doi: 10.1038/nature03031.1
81. Ricci-Vitiani L, Lombardi DG, Pilozzi E, Biffoni M, Todaro M, Peschle C, et al. Identification and Expansion of Human Colon-Cancer-Initiating Cells. Nature (2007) 445(7123):111–5. doi: 10.1038/nature05384
82. O’Brien CA, Pollett A, Gallinger S, Dick JE. A Human Colon Cancer Cell Capable of Initiating Tumour Growth in Immunodeficient Mice. Nature (2007) 445(7123):106–10. doi: 10.1038/nature05372
83. Norman JM, Anne TC, Steven B, Maxfield S, Paul B, Katy H, et al. Prospective Identification of Tumorigenic Prostate Cancer Stem Cells. Cancer Res (2005) 65(9Suppl):591. doi: 10.1158/0008-5472
84. Bühring HJ, Seiffert M, Bock TA, Scheding S, Thiel A, Scheffold A, et al. Expression of Novel Surface Antigens on Early Hematopoietic Cells. Ann NY Acad Sci (1999) 872(0). doi: 10.1111/j.1749-6632.1999.tb08450.x
85. Bussolati B, Bruno S, Grange C, Buttiglieri S, Deregibus MC, Cantino D, et al. Isolation of Renal Progenitor Cells From Adult Human Kidney. Am J Pathol (2005) 166(2):545–55. doi: 10.1016/S0002-9440(10)62276-6
86. Richardson GD, Robson CN, Lang SH, Neal DE, Maitland NJ, Collins AT. CD133, a Novel Marker for Human Prostatic Epithelial Stem Cells. J Cell Sci (2004) 117(16):3539–45. doi: 10.1242/jcs.01222
87. Uchida N, Buck DW, He D, Reitsma MJ, Masek M, Phan TV, et al. Direct Isolation of Human Central Nervous System Stem Cells. Proc Natl Acad Sci USA (2000) 97(26):14720–5. doi: 10.1073/pnas.97.26.14720
88. Valent P, Bonnet D, De Maria R, Lapidot T, Copland M, Melo JV, et al. Cancer Stem Cell Definitions and Terminology: The Devil Is in the Details. Nat Rev Cancer (2012) 12(11):767–75. doi: 10.1038/nrc3368
89. Stephens PJ, Tarpey PS, Davies H, Van Loo P, Greenman C, Wedge DC, et al. The Landscape of Cancer Genes and Mutational Processes in Breast Cancer. Nature (2012) 486(7403):400–4. doi: 10.1038/nature11017
90. Clarke MF, Dick JE, Dirks PB, Eaves CJ, Jamieson CHM, Jones DL, et al. Cancer Stem Cells - Perspectives on Current Status and Future Directions: AACR Workshop on Cancer Stem Cells. Cancer Res (2006) 66(19):9339–44. doi: 10.1158/0008-5472.CAN-06-3126
91. Dean M, Fojo T, Bates S. Tumour Stem Cells and Drug Resistance. Nat Rev Cancer (2005) 5(4):275–84. doi: 10.1038/nrc1590
92. Eyler CE, Rich JN. Survival of the Fittest: Cancer Stem Cells in Therapeutic Resistance and Angiogenesis. J Clin Oncol (2008) 26(17):2839–45. doi: 10.1200/JCO.2007.15.1829
93. Medema JP. Cancer Stem Cells: The Challenges Ahead. Nat Cell Biol (2013) 15(4):338–44. doi: 10.1038/ncb2717
94. Craene B, Berx G. Regulatory Networks Defining EMT During Cancer Initiation and Progression. Nat Rev Cancer (2013) 13(2):97–110. doi: 10.1038/nrc3447
95. Mani SA, Guo W, Liao MJ, Eaton EN, Ayyanan A, Zhou AY, et al. The Epithelial-Mesenchymal Transition Generates Cells With Properties of Stem Cells. Cell (2008) 133(4):704–15. doi: 10.1016/j.cell.2008.03.027
96. Mulholland DJ, Kobayashi N, Ruscetti M, Zhi A, Tran LM, Huang J, et al. Pten Loss and RAS/MAPK Activation Cooperate to Promote EMT and Metastasis Initiated From Prostate Cancer Stem/Progenitor Cells. Cancer Res (2012) 72(7):1878–89. doi: 10.1158/0008-5472.CAN-11-3132
97. Morel AP, Lièvre M, Thomas C, Hinkal G, Ansieau S, Puisieux A. Generation of Breast Cancer Stem Cells Through Epithelial-Mesenchymal Transition. PloS One (2008) 3(8):e2888. doi: 10.1371/journal.pone.0002888
98. Wellner U, Schubert J, Burk UC, Schmalhofer O, Zhu F, Sonntag A, et al. The EMT-Activator ZEB1 Promotes Tumorigenicity by Repressing Stemness-Inhibiting microRNAs. Nat Cell Biol (2009) 11(12):1487–95. doi: 10.1038/ncb1998
99. Borovski T, De Sousa E Melo F, Vermeulen L, Medema JP. Cancer Stem Cell Niche: The Place to be. Cancer Res (2011) 71(3):634–9. doi: 10.1158/0008-5472.CAN-10-3220
100. Cabanos HF, Hata AN. Emerging Insights Into Targeted Therapy-Tolerant Persister Cells in Cancer. Cancers (2021) 13(11):2666. doi: 10.3390/cancers13112666
101. Wang H, Gong P, Chen T, Gao S, Wu Z, Wang X, et al. Colorectal Cancer Stem Cell States Uncovered by Simultaneous Single-Cell Analysis of Transcriptome and Telomeres. Adv Sci (2021) 8(8):2004320. doi: 10.1002/advs.202004320
102. Singh A, Settleman JEMT. Cancer Stem Cells and Drug Resistance: An Emerging Axis of Evil in the War on Cancer. Oncogene (2010) 29(34):4741–51. doi: 10.1038/onc.2010.215
103. Phi LTH, Sari IN, Yang YG, Lee SH, Jun N, Kim KS, et al. Cancer Stem Cells (CSCs) in Drug Resistance and Their Therapeutic Implications in Cancer Treatment. Stem Cells Int (2018) 2018. doi: 10.1155/2018/5416923
104. Mejlvang J, Kriajevska M, Vandewalle C, Chernova T, Sayan AE, Berx G, et al. Direct Repression of Cyclin D1 by SIP1 Attenuates Cell Cycle Progression in Cells Undergoing an Epithelial Mesenchymal Transition. Mol Biol Cell (2007) 18:3250–63. doi: 10.1091/mbc.e07-05-0406
105. Naumov GN, MacDonald IC, Weinmeister PM, Kerkvliet N, Nadkarni KV, Wilson SM, et al. Persistence of Solitary Mammary Carcinoma Cells in a Secondary Site: A Possible Contributor to Dormancy. Cancer Res (2002) 62(7):2162–68.
106. Logozzi M, Spugnini E, Mizzoni D, Di Raimo R, Fais S. Extracellular Acidity and Increased Exosome Release as Key Phenotypes of Malignant Tumors. Cancer Metastasis Rev (2019) 38(1-2):93–101. doi: 10.1007/s10555-019-09783-8
107. Rezaie J, Ahmadi M, Ravanbakhsh R, Mojarad B, Mahbubfam S, Shaban SA, et al. Tumor-Derived Extracellular Vesicles: The Metastatic Organotropism Drivers. Life Sci (2021) 289:120216. doi: 10.1016/j.lfs.2021.120216
108. Ghajar CM, Peinado H, Mori H, Matei IR, Evason KJ, Brazier H, et al. The Perivascular Niche Regulates Breast Tumour Dormancy. Nat Cell Biol (2013) 15(7):807–17. doi: 10.1038/ncb2767
109. Aramini B, Masciale V, Haider KH. Defining Lung Cancer Stem Cells Exosomal Payload of miRNAs in Clinical Perspective. World J Stem Cells (2020) 12(6):406–21. doi: 10.4252/wjsc.v12.i6.406
110. Yang G, Quan Y, Wang W, Fu Q, Wu J, Mei T, et al. Dynamic Equilibrium Between Cancer Stem Cells and Non-Stem Cancer Cells in Human SW620 and MCF-7 Cancer Cell Populations. Br J Cancer (2012) 106(9):1512–9. doi: 10.1038/bjc.2012.126
111. Nieto MA. The Ins and Outs of the Epithelial to Mesenchymal Transition in Health and Disease. Annu Rev Cell Dev Biol (2011) 27:347–76. doi: 10.1146/annurev-cellbio-092910-154036
112. Their JP. Epithelial-Mesenchymal Transitions in Tumor Progression. Nat Rev Cancer (2002) 2(6):442–54. doi: 10.1038/nrc822
113. Lucio DF, Ricardo G, Juan FM, Hilda V, Francisco V, Lucio D. Adult Stem Cells and Repair Through Granulation Tissue. Front Biosci (Landmark edition) (2009) 14(4). doi: 10.1006/bbrc.1997.7466
114. Li H, Courtois ET, Sengupta D, Tan Y, Chen KH, Goh JJL, et al. Reference Component Analysis of Single-Cell Transcriptomes Elucidates Cellular Heterogeneity in Human Colorectal Tumors. Nat Publ Gr (2017) 49(5). doi: 10.1038/ng.3818
115. Sarrió D, Rodriguez-Pinilla SM, Hardisson D, Cano A, Moreno-Bueno G, Palacios J. Epithelial-Mesenchymal Transition in Breast Cancer Relates to the Basal-Like Phenotype. Cancer Res (2008) 68(4):989–97. doi: 10.1158/0008-5472.CAN-07-2017
116. Trimboli AJ, Fukino K, De Bruin A, Wei G, Shen L, Tanner SM, et al. Direct Evidence for Epithelial-Mesenchymal Transitions in Breast Cancer. Cancer Res (2008) 68(3):937–45. doi: 10.1158/0008-5472.CAN-07-2148
117. Zhang J, Ma L. MicroRNA Control of Epithelial-Mesenchymal Transition and Metastasis. Cancer Metastasis Rev (2012) 31(3-4):653–62. doi: 10.1007/s10555-012-9368-6
118. Shimono Y, Zabala M, Cho RW, Lobo N, Dalerba P, Qian D, et al. Downregulation of miRNA-200c Links Breast Cancer Stem Cells With Normal Stem Cells. Cell (2009) 138(3):592–603. doi: 10.1016/j.cell.2009.07.011
119. Siemens H, Jackstadt R, Hünten S, Kaller M, Menssen A, Götz U, et al. miR-34 and SNAIL Form a Double-Negative Feedback Loop to Regulate Epithelial-Mesenchymal Transitions. Cell Cycle (2011) 10(24):4256–71. doi: 10.4161/cc.10.24.18552
120. Kim T, Veronese A, Pichiorri F, Lee TJ, Jeon Y, Volinia S, et al. P53 Regulates Epithelial-Mesenchymal Transition Through microRNAs Targeting ZEB1 and ZEB2. J Exp Med (2011) 208(5):875–83. doi: 10.1084/jem.20110235
121. Holzner S, Senfter D, Stadler S, Staribacher A, Nguyen CH, Gaggl A, et al. Colorectal Cancer Cell-Derived MicroRNA200 Modulates the Resistance of Adjacent Blood Endothelial Barriers In Vitro. Oncol Rep (2016) 36(5):3065–71. doi: 10.3892/or.2016.5114
122. Wang W, Hong G, Wang S, Gao W, Wang P. Tumor-Derived Exosomal miRNA-141 Promote Angiogenesis and Malignant Progression of Lung Cancer by Targeting Growth Arrest-Specific Homeobox Gene (GAX). Bioengineered (2021) 12(1):821–31. doi: 10.1080/21655979.2021.1886771
123. Fang JH, Zhang ZJ, Shang LR, Luo YW, Lin YF, Yuan YF, et al. Hepatoma Cell-Secreted Exosomal microRNA-103 Increases Vascular Permeability and Promotes Metastasis by Targeting Junction Proteins. Hepatology (2018) 68(4):1459–75. doi: 10.1002/hep.29920
124. Wang L, Yang G, Zhao D, Wang J, Bai Y, Peng Q, et al. CD103-Positive CSC Exosome Promotes EMT of Clear Cell Renal Cell Carcinoma: Role of Remote MiR-19b-3p. Mol Cancer (2019) 18(1):1–15. doi: 10.1186/s12943-019-0997-z
125. Wang L, He J, Hu H, Tu L, Sun Z, Liu Y, et al. Lung CSC-Derived Exosomal miR-210-3p Contributes to a Pro-Metastatic Phenotype in Lung Cancer by Targeting FGFRL1. J Cell Mol Med (2020) 24(11):6324–39. doi: 10.1111/jcmm.15274
126. Sánchez CA, Andahur EI, Valenzuela R, Castellón EA, Fullá JA, Ramos CG, et al. Exosomes From Bulk and Stem Cells From Human Prostate Cancer Have a Differential microRNA Content That Contributes Cooperatively Over Local and Pre-Metastatic Niche. Oncotarget (2016) 7(4):3993–4008. doi: 10.18632/oncotarget.6540
127. Savagner P, Yamada KM, Thiery JP. The Zinc-Finger Protein Slug Causes Desmosome Dissociation, an Initial and Necessary Step for Growth Factor-Induced Epithelial-Mesenchymal Transition. J Cell Biol (1997) 137(6):1403–19. doi: 10.1083/jcb.137.6.1403
128. Thiery JP, Acloque H, Huang RYJ, Nieto MA. Epithelial-Mesenchymal Transitions in Development and Disease. Cell (2009) 139(5):871–90. doi: 10.1016/j.cell.2009.11.007
129. Blackwell RH, Foreman KE, Gupta GN. The Role of Cancer-Derived Exosomes in Tumorigenicity & Epithelial-to-Mesenchymal Transition. Cancers (Basel) (2017) 9(8):12–7. doi: 10.3390/cancers9080105
130. Syn N, Wang L, Sethi G, Thiery JP, Goh BC. Exosome-Mediated Metastasis: From Epithelial-Mesenchymal Transition to Escape From Immunosurveillance. Trends Pharmacol Sci (2016) 37(7):606–17. doi: 10.1016/j.tips.2016.04.006
131. Chen R, Xu X, Qian Z, Zhang C, Niu Y, Wang Z, et al. The Biological Functions and Clinical Applications of Exosomes in Lung Cancer. Cell Mol Life Sci (2019) 76(23):4613–33. doi: 10.1007/s00018-019-03233-y
132. Doherty MR, Smigiel JM, Junk DJ, Jackson MW. Cancer Stem Cell Plasticity Drives Therapeutic Resistance. Cancers (Basel) (2016) 8(1):1–13. doi: 10.3390/cancers8010008
133. Cheung KJ, Gabrielson E, Werb Z, Ewald AJ. Collective Invasion in Breast Cancer Requires a Conserved Basal Epithelial Program. Cell (2013) 155(7):1639–51. doi: 10.1016/j.cell.2013.11.029
134. Brabletz T. To Differentiate or Not-Routes Towards Metastasis. Nat Rev Cancer (2012) 12(6):425–36. doi: 10.1038/nrc3265
135. Shibue T, Weinberg RA. EMT. CSCs, and Drug Resistance: The Mechanistic Link and Clinical Implications. Nat Rev Clin Oncol (2017) 14(10):611–29. doi: 10.1038/nrclinonc.2017.44
136. Puhr M, Hoefer J, Schäfer G, Erb HH, Oh SJ, Klocker H, et al. Epithelial-To-Mesenchymal Transition Leads to Docetaxel Resistance in Prostate Cancer and Is Mediated by Reduced Expression of miR-200c and miR-205. Am J Pathol (2012) 181(6):2188–201. doi: 10.1016/j.ajpath.2012.08.011
137. Rhim AD, Mirek ET, Aiello NM, Maitra A, Bailey JM, McAllister F, et al. EMT and Dissemination Precede Pancreatic Tumor Formation. Cell (2012) 148(1-2):349–61. doi: 10.1016/j.cell.2011.11.025
138. Williams ED, Gao D, Redfern A, Thompson EW. Controversies Around Epithelial–Mesenchymal Plasticity in Cancer Metastasis. Nat Rev Cancer (2019) 19(12):716–32. doi: 10.1038/s41568-019-0213-x
139. Pastushenko I, Blanpain C. EMT Transition States During Tumor Progression and Metastasis. Trends Cell Biol (2019) 29(3):212–26. doi: 10.1016/j.tcb.2018.12.001
140. Jolly MK, Boareto M, Huang B, Jia D, Lu M, Ben-Jacob E, et al. Implications of the Hybrid Epithelial/Mesenchymal Phenotype in Metastasis. Front Oncol (2015) 5:155(JUN). doi: 10.3389/fonc.2015.00155
Keywords: cancer stem cells, exosomes, metastasis, tumor, tumor microenvironment
Citation: Liu K, Gao X, Kang B, Liu Y, Wang D and Wang Y (2022) The Role of Tumor Stem Cell Exosomes in Cancer Invasion and Metastasis. Front. Oncol. 12:836548. doi: 10.3389/fonc.2022.836548
Received: 15 December 2021; Accepted: 07 February 2022;
Published: 08 March 2022.
Edited by:
Pasquale Simeone, University of Studies G. d’Annunzio Chieti and Pescara, ItalyReviewed by:
Jafar Rezaie, Urmia University of Medical Sciences, IranMohammad Saeid Hejazi, Tabriz University of Medical Sciences, Iran
Copyright © 2022 Liu, Gao, Kang, Liu, Wang and Wang. This is an open-access article distributed under the terms of the Creative Commons Attribution License (CC BY). The use, distribution or reproduction in other forums is permitted, provided the original author(s) and the copyright owner(s) are credited and that the original publication in this journal is cited, in accordance with accepted academic practice. No use, distribution or reproduction is permitted which does not comply with these terms.
*Correspondence: Yi Wang, d2FuZ3lpQGpsdS5lZHUuY24=