- Service d’Hématologie Biologique, Hôpital Avicenne, Assistance Publique des Hôpitaux de Paris, Paris, France
In chronic lymphocytic leukemia (CLL), TP53 abnormalities are associated with reduced survival and resistance to chemoimmunotherapy (CIT). The recommended threshold to clinically report TP53 mutations is a matter of debate given that next-generation sequencing technologies can detect mutations with a limit of detection of approximately 1% with high confidence. However, the clinical impact of low-burden TP53 mutations with a variant allele frequency (VAF) of less than 10% remains unclear. Longitudinal analysis before and after fludarabine based on NGS sequencing demonstrated that low-burden TP53 mutations were present before the onset of treatment and expanded at relapse to become the predominant clone. Most studies evaluating the prognostic or predictive impact of low-burden TP53 mutations in untreated patients show that low-burden TP53 mutations have the same unfavorable prognostic impact as clonal defects. Moreover, studies designed to assess the predictive impact of low-burden TP53 mutations showed that TP53 mutations, irrespective of mutation burden, have an inferior impact on overall survival for CIT-treated patients. As low-burden and high-burden TP53 mutations have comparable clinical impacts, redefining the VAF threshold may have important implications for the clinical management of CLL.
Introduction
The heterogeneous clinical course of chronic lymphocytic leukemia has highlighted the need to define prognostic and predictive markers to improve the management of patients (1). On one hand, prognostic markers reflect the underlying biology and natural history of CLL and are informative for untreated patients or those requiring treatment (2, 3). On the other hand, predictive markers provide information on the likely benefits or contraindications of a given treatment. TP53 abnormalities, namely, both deletion of the 17p chromosome and mutations at TP53 loci, are one of the gold standards of high risk in CLL because these abnormalities indicate both an adverse prognosis and predict chemoresistance (4, 5). In the past decade, the therapeutic landscape of CLL has considerably improved, offering the possibility for patients with TP53 defects to benefit from targeted therapy with BcR pathway or bcl2 inhibitors (6–8). Although the first-line treatment strategy may differ among countries, assessment of TP53 status has become essential, as it serves as a contraindication for the use of chemoimmunotherapy (CIT) (9). Hence, in daily clinical practice, the use of TP53 status as a predictive marker is mandatory for treatment decisions before the addition of each new line of treatment (10, 11).
The implementation of NGS sequencing technologies with high sensitivity has facilitated the detection of TP53 mutations with the possibility of detecting variants with allelic fractions (VAFs) below the current conventional threshold of 10% published by the European Research Initiative on Chronic Lymphocytic Leukemia (ERIC) in 2018 (12), above which TP53 mutations should be clinically reported. Nevertheless, the clinical and biological relevance of these minor clones is debated.
The definition of minor clones and their biological and clinical significance have been discussed in numerous studies. However, contradictory results are often reported that might be in part attributed to different cohort compositions and variable low-burden threshold definitions. To clarify the clinical role of low-burden TP53 mutations in CLL, the prognostic and predictive impact of TP53 mutations were analyzed in different cohorts. The results and conclusions are discussed in this review.
What is a Low-Burden TP53 Mutation or minor Clone?
Del(17p) associated with TP53 mutations is the most common abnormality affecting the TP53 gene in CLL, accounting for approximately two-thirds of cases. The remaining cases either exclusively harbor TP53 gene mutation(s) or rarely a 17p deletion. Moreover, TP53 mutation can be accompanied by the mutation of the second allele or a copy number neutral loss of heterozygosity (13).
Historically, TP53 abnormalities were first analyzed by conventional karyotyping combined with Fluorescence In Situ Hybridization (FISH), which allowed the detection of cells carrying a deletion of chromosome 17p13.1 (TP53) with a sensitivity of >5% positive cells (14). Despite a relatively good sensitivity of detection, cytogenetic techniques failed to detect approximately 30–40% of patients carrying only mutations in the gene. Later, TP53 mutation screening relied on Sanger sequencing covering exons 4 to 9 of the gene with a sensitivity of approximately 10–20%. Hence, combining FISH analysis and sequencing substantially improved the detection of TP53 aberrations. The advent of NGS technologies next provided the opportunity to reduce the threshold of detection of TP53 mutations and to deeply examine the clonal heterogeneity of CLL. In a retrospective analysis of newly diagnosed patient samples, NGS sequencing could detect low-burden TP53 mutations previously identified as unmutated by Sanger sequencing due to their low abundance in the tumor cell population (15). Altogether, Sanger sequencing led to misclassification of approximately 6% of newly diagnosed and untreated patients harboring low-burden TP53 mutations with a VAF ranging from 0.3 to 11% (15–19). Of note, a fraction of patients harbored low-burden mutations associated with high-burden mutations, revealing the intratumoral heterogeneity of these mutations and the complexity of the TP53 clonal architecture.
The definition of minor clones often relies on the VAF threshold used to detect mutant alleles by Sanger sequencing, which is typically approximately 10–12%. This conventional threshold corresponds to the current recommendations published by ERIC in 2018, above which TP53 mutations should be clinically reported. Mutations with VAFs below the threshold are considered low allele frequency, whereas VAFs above the threshold are of a high allele frequency. This recommendation is still currently applied due to technical difficulties in detecting low-burden mutations. However, with the wide generalization and feasibility of NGS sequencing on a routine basis, the threshold to report TP53 mutations and hence to define minor clones is debated.
Indeed, below this arbitrary threshold of 10%, a wide range of TP53 variants can be detected by NGS sequencing with high confidence until reaching a limit of detection as low as 0.3% VAF (corresponding to three mutant alleles in a background of 1,000 wild-type alleles) while respecting specific procedures and quality criteria. First, CLL lymphocyte population purity greater than 80% reduces the possibility of dilution in nontumoral DNA that could underestimate a very low-burden mutation. Second, sufficient DNA corresponding to >6,000 diploid genomes and a third high target read depth is required to detect a very low-burden mutation with VAF<1% (20). Finally, robust bioinformatic workflows were developed to call true variants distinguished from background error noise. However, despite the very high confidence of TP53 variant detection by NGS sequencing, the limit of detection of these ultrasensitive technologies needs to be evaluated to distinguish true TP53 variants from background sequencing noise to avoid misdiagnosing TP53 unmutated patients as mutated. The sequencing background depends on sequencing technologies and library preparation, which differ in capture and amplicon-based processes (21, 22).
Clonal Evolution of Low-Burden TP53 Mutation After Chemotherapy
While TP53 abnormalities account for approximately 10% of naïve-treatment patients, these abnormalities are found in greater than 40% of patients with fludarabine-refractory CLL, which highlights the phenomenon of clonal evolution of TP53 mutation induced by chemotherapy (13). Despite the current recommendations that consider <10% of minor clones to be of uncertain significance, accumulating evidence based on longitudinal studies argues for the clinical relevance to report TP53 minor clones (15, 18, 20, 23–25). NGS sequencing of serial samples before and after treatment has allowed characterization of the dynamics of the minor clones under treatment and demonstrated their biological and clinical relevance.
Longitudinal retrospective studies based on NGS sequencing of fludarabine relapsed/refractory TP53 mutated patient samples showed that low-burden TP53 mutations were detected early in the disease course and before the onset of chemotherapy. These pre-treatment samples were initially screened using Sanger sequencing, and mutations were missed due to the lack of sensitivity of the technique. Interestingly, longitudinal analysis indicated that the acquisition of TP53 mutations clearly preceded karyotype evolution, which highlights the genetic instability related to the presence of a TP53 mutation and its likely role in the development of a complex karyotype (24). It is widely accepted that chemotherapy plays a key role in driving the selection of clones carrying TP53 mutations (26). Fludarabine is a purine analog that inhibits DNA synthesis in tumor cells. In the case of defects in the TP53 pathway, CLL cells lose their capacity to stop cell division and to trigger apoptosis in response to chemotherapy. As a result, the mutation induces a fitness effect by conferring a growth and survival advantage to the low-burden TP53 mutation, which expands under the selection pressure of chemotherapy (27). The fact that a given low-burden TP53 variant detected at the time of treatment initiation is found at relapse after a fludarabine-based regimen clearly demonstrates that these minor clones are not sequencing artifacts and highlights the need to redefine this threshold for optimal clinical practice.
Finally, relative stability in the TP53 variant allele frequency is observed in some patients as long as they are not treated with chemotherapy. This notion is particularly true for IGHV-mutated patients, which have a more indolent disease course and can show the persistence of the mutated clone for years (28–30). On the other hand, given the natural clonal evolution of the disease with time, TP53 minor clones can also be acquired during the disease course, independent of any pressure of selection induced by chemotherapy. This finding justifies early and iterative screening for TP53 abnormalities during follow-up and before each new line of treatment with a sensitive sequencing technique.
Impact of Targeted Agents on Low-Burden TP53 Mutations
Given that TP53-mutated patients can benefit from targeted therapies with improved remission duration, there is a need to evaluate the impact of these therapies on the evolution of the TP53-mutated clone. Data on the clonal evolution of low-burden TP53 mutations upon targeted treatment are limited (23, 31). Malcikova et al. showed that upon the use of BcR or bcl2 inhibitors as a second line of treatment, the percentage of VAF in the residual lymphocytosis remains stable, which reflects the efficacy of these treatments on the mutated clones (23). Indeed, BcR and bcl2 inhibitors target the BcR signaling pathway and apoptosis, respectively, and therefore overcome the p53 pathway. However, the persistence of TP53-mutated clones after treatment shows the failure to eradicate the disease (32). In some progressive patients treated with targeted therapies, the major TP53 mutated clone becomes minor. However, in these cases, mutations that confer resistance to ibrutinib (i.e., BTK mutation) or Venetoclax (i.e., BCL2 mutations) are frequently found. In another longitudinal study including treatment-naïve and relapsed/refractory patients treated with BcR inhibitors, the dynamics of TP53 mutated clones were complex. Most of the TP53 mutations decreased or were undetectable, but one-third remained stable with no differences noted between low- or high-VAF clones. A small proportion of TP53 mutations increased. After a prolonged follow-up of greater than 2 years, the overall stability of low-burden TP53 mutations was noted, supporting the notion of the lack of specific positive selection of TP53 mutations under conditions of ibrutinib treatment (31). Nevertheless, all these observations need to be confirmed in a cohort of patients treated with novel agents in the frontline setting. To date, this has not been explored within clinical studies, and data are preliminary, especially for bcl2 inhibitors.
Low- and High-Burden TP53 Mutations Have the Same Unfavorable Prognostic Impact
In most studies focusing on the clinical impact of TP53 minor clones, an arbitrary threshold of 10–12% VAF was chosen to define patients with low- or high-burden TP53-mutated clones. Most studies conducted in untreated patients (15, 18, 20) showed that low-burden TP53 mutations significantly reduced the OS compared to cases with unmutated TP53 genes. Moreover, the impact on OS was the same for patients harboring minor clones or high-burden TP53 mutations (Table 1). The clinical consequence of TP53 mutations was similar when patients with low VAF were stratified into subclasses <1%, between 1% and 5% or 5% and 10%. Shorter OS was also confirmed when separately considering patients with single or multiple mutations classified as high VAF or low VAF (15, 20).
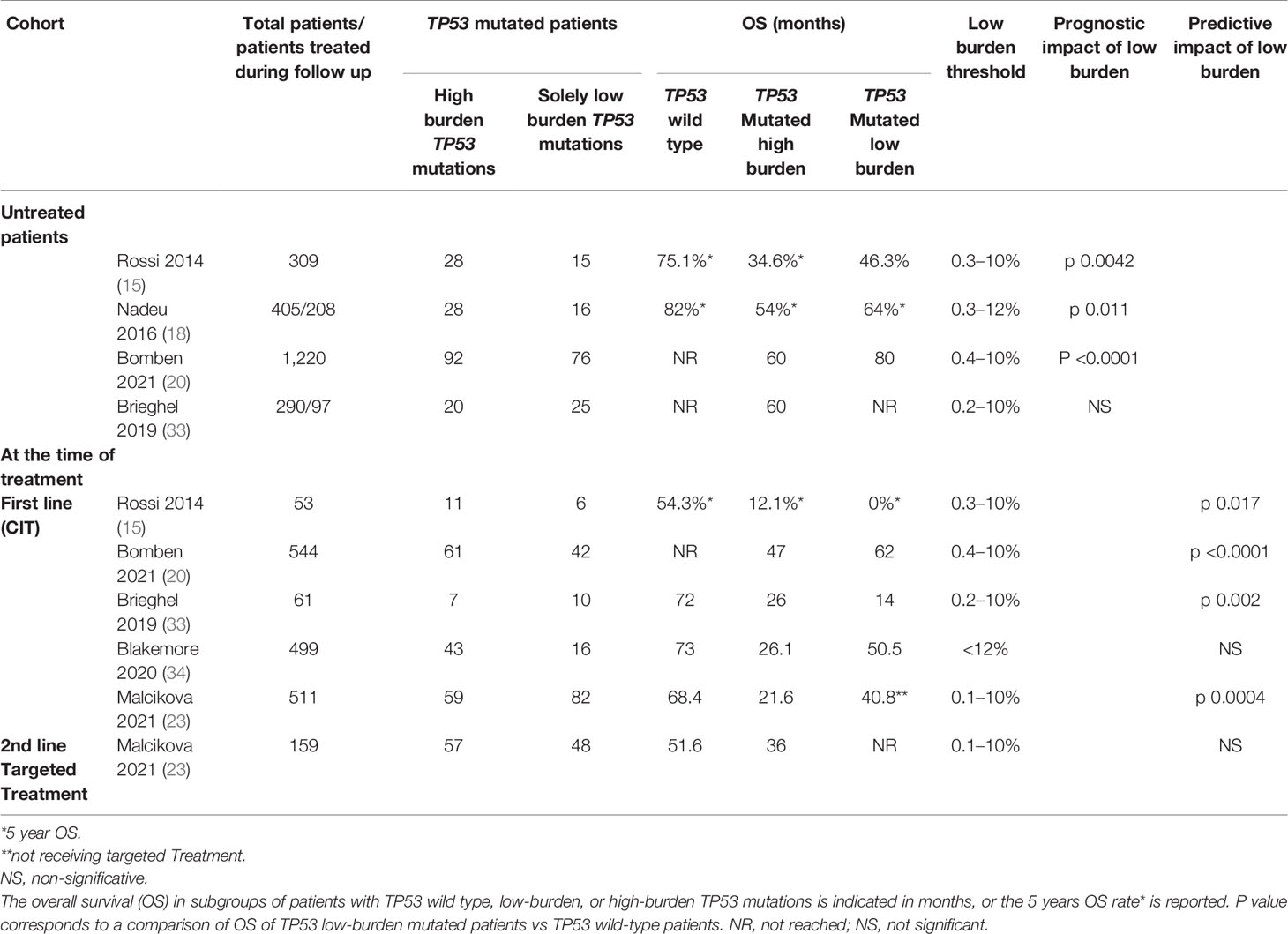
Table 1 Summary of the prognostic and predictive impact of TP53 mutations evaluated in 6 studies in CLL.
The presence of del(17p) and/or TP53 mutations are parameters of the CLL-International Prognostic Index (CLL-IPI), which combines five parameters (age, clinical stage, TP53, IGHV mutational status, serum β2-microglobulin) to predict survival and time-to-first-treatment (TTFT) in CLL patients. However, the value of the VAF threshold used to consider TP53 mutated considerably impacted this score. Indeed, revisited CLL-IPI combining both high- and low-VAF TP53 mutations significantly better discriminated high-risk patients than standard CLL-IPI, which exclusively considered high-VAF TP53 mutations (20, 23, 35). Therefore, minor clones should be considered to refine prognostication models.
Most studies evaluating the predictive impact of TP53 mutations showed significantly reduced survival in CIT-treated patients harboring either low- or high-burden TP53 mutations (15, 20, 23, 33). Clonal expansion is likely the main factor contributing to the inferior survival of CIT-treated patients with low-burden TP53 mutations, as demonstrated by longitudinal studies comparing pre- and post-treatment samples showing that the mutation burden consistently increases at relapse (18, 20, 23). Furthermore, the risk of TP53 mutation expansion beyond the current threshold of 10% in the first relapse was significantly higher for patients carrying mutations with VAF >1% than for those with VAF <1% (23). Additionally, very low clonal abundance cell populations (as low as 0.3%) are clinically relevant, as they are resistant to CIT, are positively selected and may become the dominant leukemic population at the time of relapse. Blakemore et al.’s (34) LRF CLL4 clinical trial could not demonstrate inferior survival associated with cases harboring <12% VAF TP53 mutations but rather an intermediate-risk group, revealing heterogeneity among studies based on the patients included, the duration of follow-up, and the thresholds used.
Therefore, these observations strengthen the need to redefine the clinically relevant threshold of VAF, which better discriminates TP53-mutated patients who will benefit from a targeted therapy (15, 26, 36–38).
The literature on the impact of TP53 minor clones on targeted therapies is less abundant. One study (23) showed that in a cohort of relapsed/refractory patients entering treatment with BcR and bcl2 inhibitors, OS in response to targeted treatment in TP53-mutated patients did not significantly differ from that of TP53 wild type patients irrespective of VAF.
Discussion
The main focus of this review was to demonstrate that low-burden TP53 mutations have an impact on CLL survival. This review analyzing different retrospective and prospective CLL cohorts highlights the need to detect TP53 mutations with highly sensitive NGS technology in a routine setting due to the clonal expansion of minor clones after CIT. NGS sequencing technology can detect low-burden TP53 mutations that are as low as 0.3% over the background noise using specific bioinformatics pipelines. The clinical relevance of these low-burden mutations is evaluated as prognostic or predictive markers, and most of the studies identified that cases bearing low-burden TP53 mutations (VAF <10%) experienced shorter OS similarly to cases with high-burden TP53 mutations (VAF >10%) compared to patients harboring wild type TP53. These concordant observations highlight the need to redefine the threshold used to identify TP53-mutated cases, as these findings may have important implications in the setting of CLL treatment.
Low-VAF mutations showed the same molecular characteristics and distribution as high-VAF mutations, confirming that they are not sequencing artifacts. Moreover, the pathogenicity of these mutations was confirmed using different databases (IARC TP53, UMD database) (39, 40). Accordingly, in longitudinal studies, sequential samples from CIT-treated patients showed that minor clones were positively selected and became dominant at relapse, confirming that these low-burden mutations that initially occur in a minority of cells are true mutations that expand under selective pressure (26).
Focusing on studies designed to assess overall survival (OS) between cases harboring the wild-type TP53 gene versus cases with low-burden TP53 variant (15, 18, 20, 35), the frequencies of TP53 mutation ranged from 10.6 to 27.5%, of which 26.8 to 45.2% cases exclusively harbored low-burden TP53 mutations depending on the threshold used to discriminate between low- and high-VAF TP53 mutations. Blakemore et al. failed to demonstrate a clinical impact of low-burden TP53 mutations but identified an intermediate-risk group. These findings were probably due to the choice of an arbitrary threshold of 12% for discriminating low- and high-burden TP53 mutations and a minimum VAF >1% (34).
The impact of TP53 mutations on OS also depended on the composition of the cohort with different proportions of patients carrying mutated IGHV or 17p deletion or variable times to diagnosis. Indeed, newly diagnosed patients often harbor mutated IGHV, and TP53 abnormalities may not have a negative impact on the indolent disease course (23, 28–30, 35). These observations suggest that TP53 mutation testing should be performed exclusively before treatment. Conversely, Brieghel et al. demonstrated that neither high nor low burden TP53 mutations at the time of CLL diagnosis influenced OS independently (35). Surprisingly, patients with 17p deletion had an inferior outcome, and only the subgroup of patients with high-burden TP53 mutations and unmutated IGHV demonstrated an inferior OS. This discrepancy may be explained by the composition of the cohort and the more indolent nature of the disease for the patients included. The frequency of 17p deletion was only 2.4%, whereas TP53 mutations without 17p deletions were more frequent (10.7%). Furthermore, the proportion of newly diagnosed TP53-mutated patients with unmutated IGHV genes was low (32%) as compared to 57% (18) and 35.5% (15).
Given that NGS technology can detect low-burden TP53 mutations at levels as low as 0.3%, should this limit of detection be used as a threshold to identified patients with TP53 mutations? One study further stratified patients based on a 5% VAF threshold and observed shortened survival only for mutations with 5–10% VAF but not for mutations with 1–5% VAF. Interestingly, the subgroup carrying mutations with <1% VAF showed significantly shortened OS. In addition, the risk of a rapid expansion of the clone to greater than 10% in the first relapse after CIT treatment was higher for patients carrying mutations with >1% VAF than for those with <1% VAF (23). These results suggest that a >1% VAF threshold could be clinically relevant.
Further standardization (41) and bioinformatics development (42) may be necessary to identify the background noise at each position of the TP53 gene to validate very low-burden mutations (as low as 0.3%).
Hence, there is a need to harmonize the methodologies used to detect minor clones and minimal requirements for the standardized assessment of such clones. An ERIC (European research initiative on CLL http://www.ericll.org/) multicenter study on the prognostic and predictive impact of low-burden TP53 mutations is in progress with three phases: 1) compare results among laboratories performing NGS analysis of TP53 mutations in CLL with a detection limit of ≤1% VAF, 2) assess the prognostic and predictive impact of low-VAF TP53 variants in patients entering first-line treatment, and 3) re-evaluate the cut-off for reporting of TP53 variants in CLL and, if needed, to update recommendations on minor TP53 variant detection, validation, and reporting. Forty-one laboratories participated in the 1st phase of the study and analyzed the same samples with low-VAF TP53 mutations. The collected results show that the 2% VAF cut-off could be reproducibly applied for the planned multicenter study on the clinical significance of low-VAF TP53 variants (43). The collection of clinical and biological data from a consecutive cohort of patients, namely, both wild-type and mutated TP53 CLL entering 1st-line therapy, is currently in progress to re-evaluate the cut-off for reporting TP53 variants.
Author Contributions
All authors listed have made a substantial, direct, and intellectual contribution to the work and approved it for publication.
Conflict of Interest
The authors declare that the research was conducted in the absence of any commercial or financial relationships that could be construed as a potential conflict of interest.
Publisher’s Note
All claims expressed in this article are solely those of the authors and do not necessarily represent those of their affiliated organizations, or those of the publisher, the editors and the reviewers. Any product that may be evaluated in this article, or claim that may be made by its manufacturer, is not guaranteed or endorsed by the publisher.
References
1. Parikh SA, Shanafelt TD. Prognostic Factors and Risk Stratification in Chronic Lymphocytic Leukemia. Semin Oncol (2016) 43:233–40. doi: 10.1053/j.seminoncol.2016.02.009
2. Minden M. Is It Time to Redefine Prognostic and Predictive in Oncology? J Clin Oncol (2016) 34:1702–3. doi: 10.1200/JCO.2015.66.3815
3. Ballman KV. Biomarker: Predictive or Prognostic? J Clin Oncol (2015) 33:3968–71. doi: 10.1200/JCO.2015.63.3651
4. Muller-Hermelink HK, Montserrat E, Catovsky D, Campo E, Harris NL, Stein H. Chronic Lymphocytic Leukemia/Small Lymphocytic Lymphoma. In: Swerdlow SH, Campo E, Harris NL, editors. World Health Organization Classification of Tumours, Pathology and Genetics of Tumours of Haematopoietic and LymphoidTissues. Lyon France: IARC (2008). p. 180–82.
5. Gonzalez D, Martinez P, Wade R, Hockley S, Oscier D, Matutes E, et al. Mutational Status of the TP53 Gene as a Predictor of Response and Survival in Patients With Chronic Lymphocytic Leukemia: Results From the LRF CLL4 Trial. J Clin Oncol (2011) 29:2223–9. doi: 10.1200/JCO.2010.32.0838
6. Stilgenbauer S, Eichhorst B, Schetelig J, Coutre S, Seymour JF, Munir T, et al. Venetoclax in Relapsed or Refractory Chronic Lymphocytic Leukaemia With 17p Deletion: A Multicentre, Open-Label, Phase 2 Study. Lancet Oncol (2016) 17:768–78. doi: 10.1016/S1470-2045(16)30019-5
7. O’Brien S, Jones JA, Coutre SE, Mato AR, Hillmen P, Tam C, et al. Ibrutinib for Patients With Relapsed or Refractory Chronic Lymphocytic Leukaemia With 17p Deletion (RESONATE-17): A Phase 2, Open-Label, Multicentre Study. Lancet Oncol (2016) 17:1409–18. doi: 10.1016/S1470-2045(16)30212-1
8. Brown JR, Byrd JC, Coutre SE, Benson DM, Flinn IW, Wagner-Johnston ND, et al. Idelalisib, an Inhibitor of Phosphatidylinositol 3-Kinase P110delta, for Relapsed/Refractory Chronic Lymphocytic Leukemia. Blood (2014) 123:3390–7. doi: 10.1182/blood-2013-11-535047
9. Quinquenel A, Aurran-Schleinitz T, Clavert A, Cymbalista F, Dartigeas C, Davi F, et al. Diagnosis and Treatment of Chronic Lymphocytic Leukemia: Recommendations of the French CLL Study Group (FILO). Hemasphere (2020) 4:e473. doi: 10.1097/HS9.0000000000000473
10. Gaidano G, Rossi D. The Mutational Landscape of Chronic Lymphocytic Leukemia and its Impact on Prognosis and Treatment. Hematol Am Soc Hematol Educ Program (2017) 2017:329–37. doi: 10.1182/asheducation-2017.1.329
11. Eichhorst B, Robak T, Montserrat E, Ghia P, Niemann CU, Kater AP, et al. Chronic Lymphocytic Leukaemia: ESMO Clinical Practice Guidelines for Diagnosis, Treatment and Follow-Up. Ann Oncol (2021) 32:23–33. doi: 10.1016/j.annonc.2020.09.019
12. Malcikova J, Tausch E, Rossi D, Sutton LA, Soussi T, Zenz T, et al. ERIC Recommendations for TP53 Mutation Analysis in Chronic Lymphocytic Leukemia-Update on Methodological Approaches and Results Interpretation. Leukemia (2018) 32:1070–80. doi: 10.1038/s41375-017-0007-7
13. Campo E, Cymbalista F, Ghia P, Jäger U, Pospisilova S, Rosenquist R, et al. TP53 Aberrations in Chronic Lymphocytic Leukemia: An Overview of the Clinical Implications of Improved Diagnostics. Haematologica (2018) 103:1956–68. doi: 10.3324/haematol.2018.187583
14. McGowan-Jordan J, Hastings RJ, Moore S eds. ISCN 2020: An International System for Human Cytogenomic Nomenclature. Basel: Karger (2020).
15. Rossi D, Khiabanian H, Spina V, Ciardullo C, Bruscaggin A, Fama R, et al. Clinical Impact of Small TP53 Mutated Subclones in Chronic Lymphocytic Leukemia. Blood (2014) 123:2139–47. doi: 10.1182/blood-2013-11-539726
16. Zenz T, Kröber A, Scherer K, Häbe S, Bühler A, Benner A, et al. Monoallelic TP53 Inactivation is Associated With Poor Prognosis in Chronic Lymphocytic Leukemia: Results From a Detailed Genetic Characterization With Long-Term Follow-Up. Blood (2008) 112:3322–9. doi: 10.1182/blood-2008-04-154070
17. Zenz T, Häbe S, Denzel T, Winkler D, Döhner H, Stilgenbauer S. How Little is Too Much? P53 Inactivation: From Laboratory Cutoff to Biological Basis of Chemotherapy Resistance. Leukemia (2008) 22:2257–8. doi: 10.1038/leu.2008.114
18. Nadeu F, Delgado J, Royo C, Baumann T, Stankovic T, Pinyol M, et al. Clinical Impact of Clonal and Subclonal TP53, SF3B1, BIRC3, NOTCH1, and ATM Mutations in Chronic Lymphocytic Leukemia. Blood (2016) 127:2122–30. doi: 10.1182/blood-2015-07-659144
19. Schuh A, Becq J, Humphray S, Alexa A, Burns A, Clifford R, et al. Monitoring Chronic Lymphocytic Leukemia Progression by Whole Genome Sequencing Reveals Heterogeneous Clonal Evolution Patterns. Blood (2012) 120:4191–6. doi: 10.1182/blood-2012-05-433540
20. Bomben R, Rossi FM, Vit F, Bittolo T, D’Agaro T, Zucchetto A, et al. TP53 Mutations With Low Variant Allele Frequency Predict Short Survival in Chronic Lymphocytic Leukemia. Clin Cancer Res (2021) 27:5566–75. doi: 10.1158/1078-0432.CCR-21-0701
21. Park G, Park JK, Shin SH, Jeon HJ, Kim NKD, Kim YJ, et al. Characterization of Background Noise in Capture-Based Targeted Sequencing Data. Genome Biol (2017) 18:136. doi: 10.1186/s13059-017-1275-2
22. Ma X, Shao Y, Tian L, Flasch DA, Mulder HL, Edmonson MN, et al. Analysis of Error Profiles in Deep Next-Generation Sequencing Data. Genome Biol (2019) 20:50. doi: 10.1186/s13059-019-1659-6
23. Malcikova J, Pavlova S, Kunt Vonkova B, Radova L, Plevova K, Kotaskova J, et al. Low-Burden TP53 Mutations in CLL: Clinical Impact and Clonal Evolution Within the Context of Different Treatment Options. Blood (2021) 138:2670–85. doi: 10.1182/blood.2020009530
24. Lazarian G, Tausch E, Eclache V, Sebaa A, Bianchi V, Letestu R, et al. TP53 Mutations are Early Events in Chronic Lymphocytic Leukemia Disease Progression and Precede Evolution to Complex Karyotypes. Int J Cancer (2016) 139:1759–63. doi: 10.1002/ijc.30222
25. Amin NA, Seymour E, Saiya-Cork K, Parkin B, Shedden K, Malek SN. A Quantitative Analysis of Subclonal and Clonal Gene Mutations Before and After Therapy in Chronic Lymphocytic Leukemia. Clin Cancer Res (2016) 22:4525–35. doi: 10.1158/1078-0432.CCR-15-3103
26. Landau DA, Carter SL, Stojanov P, McKenna A, Stevenson K, Lawrence MS, et al. Evolution and Impact of Subclonal Mutations in Chronic Lymphocytic Leukemia. Cell (2013) 152:714–26. doi: 10.1016/j.cell.2013.01.019
27. Dohner H, Fischer K, Bentz M, Hansen K, Benner A, Cabot G, et al. P53 Gene Deletion Predicts for Poor Survival and non-Response to Therapy With Purine Analogs in Chronic B-Cell Leukemias. Blood (1995) 85:1580–9. doi: 10.1182/blood.V85.6.1580.bloodjournal8561580
28. Best OG, Gardiner AC, Davis ZA, Tracy I, Ibbotson RE, Majid A, et al. A Subset of Binet Stage A CLL Patients With TP53 Abnormalities and Mutated IGHV Genes Have Stable Disease. Leukemia (2009) 23:212–4. doi: 10.1038/leu.2008.260
29. Sutton LA, Hadzidimitriou A, Baliakas P, Agathangelidis A, Langerak AW, Stilgenbauer S, et al. Immunoglobulin Genes in Chronic Lymphocytic Leukemia: Key to Understanding the Disease and Improving Risk Stratification. Haematologica (2017) 102:968–71. doi: 10.3324/haematol.2017.165605
30. Delgado J, Salaverria I, Baumann T, Martinez-Trillos A, Lee E, Jimenez L, et al. Genomic Complexity and IGHV Mutational Status are Key Predictors of Outcome of Chronic Lymphocytic Leukemia Patients With TP53 Disruption. Haematologica (2014) 99:e231–4. doi: 10.3324/haematol.2014.108365
31. Cafforio L, Raponi S, Cappelli LV, Ilari C, Soscia R, De Propris MS, et al. Treatment With Ibrutinib Does Not Induce a TP53 Clonal Evolution in Chronic Lymphocytic Leukemia. Haematologica (2021) 107:334–7. doi: 10.3324/haematol.2020.263715
32. Ahn IE, Tian X, Wiestner A. Ibrutinib for Chronic Lymphocytic Leukemia With TP53 Alterations. N Engl J Med (2020) 383:498–500. doi: 10.1056/NEJMc2005943
33. Brieghel C, Kinalis S, Yde CW, Schmidt AY, Jonson L, Andersen MA, et al. Deep Targeted Sequencing of TP53 in Chronic Lymphocytic Leukemia: Clinical Impact at Diagnosis and at Time of Treatment. Haematologica (2019) 104:789–96. doi: 10.3324/haematol.2018.195818
34. Blakemore SJ, Clifford R, Parker H, Antoniou P, Stec-Dziedzic E, Larrayoz M, et al. Clinical Significance of TP53, BIRC3, ATM and MAPK-ERK Genes in Chronic Lymphocytic Leukaemia: Data From the Randomised UK LRF CLL4 Trial. Leukemia (2020) 34:1760–74. doi: 10.1038/s41375-020-0723-2
35. Group I-L. An International Prognostic Index for Patients With Chronic Lymphocytic Leukaemia (CLL-IPI): A Meta-Analysis of Individual Patient Data. Lancet Oncol (2016) 17:779–90. doi: 10.1016/S1470-2045(16)30029-8
36. Edelmann J, Tausch E, Landau DA, Robrecht S, Bahlo J, Fischer K, et al. Frequent Evolution of Copy Number Alterations in CLL Following First-Line Treatment With FC(R) is Enriched With TP53 Alterations: Results From the CLL8 Trial. Leukemia (2017) 31:734–38. doi: 10.1038/leu.2016.317
37. Stilgenbauer S, Sander S, Bullinger L, Benner A, Leupolt E, Winkler D, et al. Clonal Evolution in Chronic Lymphocytic Leukemia: Acquisition of High-Risk Genomic Aberrations Associated With Unmutated VH, Resistance to Therapy, and Short Survival. Haematologica (2007) 92:1242–5. doi: 10.3324/haematol.10720
38. Malcikova J, Smardova J, Rocnova L, Tichy B, Kuglik P, Vranova V, et al. Monoallelic and Biallelic Inactivation of TP53 Gene in Chronic Lymphocytic Leukemia: Selection, Impact on Survival, and Response to DNA Damage. Blood (2009) 114:5307–14. doi: 10.1182/blood-2009-07-234708
39. Hamroun D, Kato S, Ishioka C, Claustres M, Beroud C, Soussi T. The UMD TP53 Database and Website: Update and Revisions. Hum Mutat (2006) 27:14–20. doi: 10.1002/humu.20269
40. Bouaoun L, Sonkin D, Ardin M, Hollstein M, Byrnes G, Zavadil J, et al. TP53 Variations in Human Cancers: New Lessons From the IARC TP53 Database and Genomics Data. Hum Mutat (2016) 37:865–76. doi: 10.1002/humu.23035
41. Petrackova A, Vasinek M, Sedlarikova L, Dyskova T, Schneiderova P, Novosad T, et al. Standardization of Sequencing Coverage Depth in NGS: Recommendation for Detection of Clonal and Subclonal Mutations in Cancer Diagnostics. Front Oncol (2019) 9:851. doi: 10.3389/fonc.2019.00851
42. Pecuchet N, Rozenholc Y, Zonta E, Pietrasz D, Didelot A, Combe P, et al. Analysis of Base-Position Error Rate of Next-Generation Sequencing to Detect Tumor Mutations in Circulating DNA. Clin Chem (2016) 62:1492–503. doi: 10.1373/clinchem.2016.258236
43. Pavlova S, Malcikova J, Radova L. Interlaboratory Comparison of NGS Methods for Detection of TP53 Variants < 10% VAF: The First Phase of an ERIC Multicenter Study on the Clinical Impact of Low-Frequent TP53 Variants in CLL. HemaSphere Abstract Book. 25th Congress of the European Hematology Association Virtual Edition, 11-14 June 2020. (2020) 4 S1:285.
Keywords: CLL (Chronic Lymphocytic Leukemia), TP53, NGS (next-generation sequencing), clinical impact, minor clone
Citation: Lazarian G, Cymbalista F and Baran-Marszak F (2022) Impact of Low-Burden TP53 Mutations in the Management of CLL. Front. Oncol. 12:841630. doi: 10.3389/fonc.2022.841630
Received: 22 December 2021; Accepted: 18 January 2022;
Published: 08 February 2022.
Edited by:
Jitka Malcikova, Masaryk University, CzechiaReviewed by:
Lydia Scarfò, Vita-Salute San Raffaele University, ItalyCopyright © 2022 Lazarian, Cymbalista and Baran-Marszak. This is an open-access article distributed under the terms of the Creative Commons Attribution License (CC BY). The use, distribution or reproduction in other forums is permitted, provided the original author(s) and the copyright owner(s) are credited and that the original publication in this journal is cited, in accordance with accepted academic practice. No use, distribution or reproduction is permitted which does not comply with these terms.
*Correspondence: Fanny Baran-Marszak, ZmFubnkuYmFyYW4tbWFyc3pha0BhcGhwLmZy