- 1Precision Breast Cancer Institute, University of Cambridge, Cambridge, United Kingdom
- 2Department of Oncology, Cambridge University Hospitals NHS Foundation Trust, Cambridge, United Kingdom
- 3Cancer Research UK Cambridge Centre, Cancer Research UK Cambridge Institute, University of Cambridge, Cambridge, United Kingdom
Personalised approaches to the management of all solid tumours are increasing rapidly, along with wider accessibility for clinicians. Advances in tumour characterisation and targeted therapies have placed triple-negative breast cancers (TNBC) at the forefront of this approach. TNBC is a highly heterogeneous disease with various histopathological features and is driven by distinct molecular alterations. The ability to tailor individualised and effective treatments for each patient is of particular importance in this group due to the high risk of distant recurrence and death. The mainstay of treatment across all subtypes of TNBC has historically been cytotoxic chemotherapy, which is often associated with off-target tissue toxicity and drug resistance. Neoadjuvant chemotherapy is commonly used as it allows close monitoring of early treatment response and provides valuable prognostic information. Patients who achieve a complete pathological response after neoadjuvant chemotherapy are known to have significantly improved long-term outcomes. Conversely, poor responders face a higher risk of relapse and death. The identification of those subgroups that are more likely to benefit from breakthroughs in the personalised approach is a challenge of the current era where several targeted therapies are available. This review presents an overview of contemporary practice, and promising future trends in the management of early TNBC. Platinum chemotherapy, DNA damage response (DDR) inhibitors, immune checkpoint inhibitors, inhibitors of the PI3K-AKT-mTOR, and androgen receptor (AR) pathways are some of the increasingly studied therapies which will be reviewed. We will also discuss the growing evidence for less-developed agents and predictive biomarkers that are likely to contribute to the forthcoming advances in this field. Finally, we will propose a framework for the personalised management of TNBC based upon the integration of clinico-pathological and molecular features to ensure that long-term outcomes are optimised.
1. Introduction
Breast cancer (BC) is the most common cancer affecting women and is the leading cause of cancer-related death in women worldwide (1). Triple-negative breast cancer (TNBC), a highly heterogeneous subtype, represents approximately 15% of all breast cancers (2). TNBC behaves aggressively, has a poorer prognosis, and a higher risk of distant relapse and death relative to other BC subtypes (2). Genomic and transcriptomic data have enhanced our ability to understand the TNBC taxonomy and have enabled the identification of new therapeutic targets. The development of new therapeutic options and optimisation of personalised management strategies is critical in improving outcomes for affected patients.
This review provides an overview of contemporary practice in the treatment of early-stage TNBC and highlights promising future directions. We will discuss the growing evidence for newer therapies predicted to contribute to forthcoming advances in this field, and propose a framework for the personalisedmanagement of TNBC based upon the integration of clinical and molecular features.
2. Diagnosis and Clinical Presentation
TNBC is characterised by the absence of oestrogen (ER) and progesterone (PR) receptor expression, in addition to the absence of HER2 amplification as measured by immunohistochemistry or fluorescence in situ hybridisation. TNBC is disproportionately seen in younger women, as well as in Hispanic and African American populations (3). Disease-free intervals following primary treatment of early-stage (I–III) TNBCs are often short. The recurrence rate is 25%, with the highest risk of recurrence in the first three years after diagnosis and a median time to relapse after surgery of 18.8 months (4). Metastatic TNBC (mTNBC) exhibits a more aggressive phenotype than other BC subtypes, as demonstrated by a shorter chemotherapy response duration, and a shorter overall survival (OS) (median 13.3 months) (5).
3. TNBC Heterogeneity
TNBC is a heterogeneous disease with significant inter- and intra-tumour heterogeneity (6–8). Multiple efforts have focused on adequately addressing this biological complexity to enable the tailoring of therapeutic options to individual tumour characteristics.
3.1. Histological Subtypes
The current clinical definition of TNBC encompasses multiple histological subtypes. Approximately 85% of TNBCs are morphologically defined as invasive carcinoma of no special type (IC-NST). The remaining TNBCs are less common tumours of a special type, which are collectively associated with a poor prognosis (9). Individual special types display distinct pathological and molecular characteristics and prognoses. Tumours of indolent course include adenoid cystic, secretory and tubular carcinomas. Medullary histology is associated with a good prognosis and high response rates to chemotherapy, whereas metaplastic tumours show differentiation towards squamous epithelium with mesenchymal components and are frequently chemoresistant (10). An accurate histological examination marks the first step towards the identification of key mechanistic features that could be exploited for direct treatment (Table 1).
3.2. Molecular Subtypes
Numerous efforts to build upon the molecular classification of TNBCs have been proposed (Table 2). Here we review the most recognised classifiers that utilise genomic and transcriptomic data and summarise their predictive value when tested in early TNBC clinical cohorts. Many other classification approaches have been proposed (Table S1), with the absence of clinical evidence for treatment response limiting their use.
3.2.1. Intrinsic Subtypes
Breast cancers can be classified into six intrinsic molecular subtypes by gene expression (GE) profiling (17, 24) as follows: Luminal A, Luminal B, Her2 enriched, normal-like, basal-like, and Claudin low. Each subtype is identified within the TNBC group as defined by immunohistochemistry. Basal-like tumours are most frequent (50–75%). However, they are not exclusive to the TNBC phenotype (24). The claudin-low subtype represents 25–40% of TNBC and was more recently introduced (25).
Basal-like tumours are characterised by the presence of cytokeratins typically expressed by the basal layer of the skin, widespread genomic instability, high proliferation markers, loss of function of BRCA1, and dysregulation of MYC and RB1 pathways (24). Claudin-low tumours have several features in common with basal-like tumours but are uniquely characterised by low levels of cell adhesion proteins, the enrichment of mesenchymal traits and stem cell features (26). Luminal tumours overexpress a ‘luminal signature’ containing ESR1, GATA3, FOXA1, XBP1, and MYB. Her2 amplification concomitantly with overexpression of HER2-amplicon-associated genes defines the Her2 enriched subtype (24).
Intrinsic subtypes provide independent predictive information regarding the response to neoadjuvant chemotherapy (NACT) when considering all subtypes of breast cancer, although not consistently for the TNBC cohort when viewed in isolation. Claudin-low tumours are associated with lower pathological complete response (pCR) rates compared to basal-like subtypes (27). In a subgroup analysis of the BrighTNess trial, pCR rates were higher for basal-like vs. non-basal tumours (52.3% vs 35.4%, p=0.003) (27). In contrast, no difference in pCR rate was observed with the addition of carboplatin for patients with basal-like TNBC vs non-basal TNBC in the CALGB40603 study (28). These results illustrate that the predictive value often linked to the basal-like subtypes has not always been reproduced in the early setting of TNBC, making intrinsic subtypes less reliable biomarkers of response within this group.
3.2.2. Lehmann/Pietenpol Subtypes
Lehmann et al. selected clustering analyses to identify six TNBC subtypes displaying unique GE patterns and ontologies. Each subtype is characterised by the activation of specific signalling pathways that lead to a selective response to targeted therapies in vivo (18). Additional histopathological quantification and laser-capture microdissection prompted a refined classification with only four tumour-specific subtypes (TNBCtype-4). The original immunomodulatory and mesenchymal stem-like subtypes were deemed to originate from infiltrating lymphocytes and tumour-associated stromal cells, therefore excluding the impact of these elements from the classification. The new approach demonstrated differences in clinical baseline characteristics and both local and distant disease progression (29). Basal-like 1 (BL1) revealed increased markers of proliferation and elevated expression of the DNA damage response (DDR) genes. Basal-like 2 (BL2) is characterised by features of basal/myoepithelial origin and activation of growth factor pathways such as EGF, NGF, MET, Wnt/β-catenin, and IGF1R. The mesenchymal (M) subtype displays activation of pathways involved in epithelial–mesenchymal transition (EMT), cellular differentiation, and growth pathways. Luminal androgen receptor (LAR) tumours are characterised by high expression of androgen receptor (AR) and downstream AR targets, and enrichment of pathways involved in steroid synthesis, porphyrin metabolism, and androgen/oestrogen metabolism (18).
A retrospective analysis from the validation cohort of the TNBC subtype classification presented by Masuda et al. showed that the likelihood of pCR with NACT was subtype dependent. BL1 had the highest pCR rate; BL2 and LAR had the lowest. TNBC subtypes demonstrated improved pCR predictions compared to intrinsic subtypes (basal-like vs. non-basal) (30). In a retrospective analysis of clinically annotated microarray datasets of BC patients, TNBC type-4 subtyping was not associated with significant differences in pCR in the TNBC subgroup. However, the overall incidence of pCR for the subtypes demonstrates trends similar to those observed in previous studies. BL1 displayed the greatest pCR rate (41%), and LAR and BL2 displayed the lowest (29 and 18%, respectively). BL1 patients had significantly higher pCR rates compared with other subtypes (49% vs. 31%, p = 0.04) (29). Santonja et al. explored the performance of Lehmann subtypes and their association with pCR in 125 TNBC patients treated with neoadjuvant anthracyclines and/or taxanes with and without carboplatin, and their results agreed with previous reports (31). The pCR rate for carboplatin containing regimens was highest for BL1 tumours (80% vs 23%, p = 0.027). LAR tumours had the lowest pCR rate for all treatments (14.3% vs 42.7%, p = 0.045).
3.2.3. Burstein Subtypes
Burstein and colleagues applied non-negative matrix factorisation clustering to identify four distinct TNBC subtypes characterised by key molecular features and prognosis: LAR, mesenchymal, basal-like immunosuppressed (BLIS), and basal-like immune-activated (BLIA). BLIS and BLIA had the best and worst clinical outcomes, respectively. LAR and mesenchymal subtypes revealed significant overlap with Lehmman’s classification. Burstein’s subtypes based on immune signalling (BLIA, BLIS) revealed a combination of BL1 and BL2 subtypes (19).
3.2.4. FUSCC Classification
Liu et al. developed a classification system based on the transcriptome profiles of both messenger RNAs and long non-coding RNAs to divide TNBC into four distinct clusters. Cluster A: immunomodulatory subtype, Cluster B: luminal androgen receptor subtype (LAR), Cluster C: mesenchymal-like subtype, and Cluster D: basal-like and immune-suppressed (BLIS) subtype. No significant difference in prognosis was found between the four subtypes. Tumours classified as the BLIS subtype experienced poorer relapse-free survival (RFS) compared with all other subtypes (20, 32). Further classification of BLIS tumours based on their homologous recombination deficiency (HRD) status (33) showed that high-HRD BLIS TNBCs and low-HRD BLIS TNBCs exhibited distinctive genomic characteristics and prognoses. Patients with tumours defined as low-HRD had a worse prognosis than those in the high-HRD subgroup (5-year RFS of 73 and 95%, respectively, p = 0.002) (32).
3.2.5. Integrative Clusters
Combining GE and DNA copy number analysis within the METABRIC dataset further expanded the taxonomy of breast cancer (22). Eleven Integrative Clusters (IntClust) with distinctive copy number profiles and clinical outcomes were identified. TNBCs are most frequently classified as IntClust 4ER- or IntClust 10. Rueda et al. showed that patients with tumours classified as IntClust 10 (n = 222) have a low probability of late relapse (five years after diagnosis), while those classified as IntClust4ER- (n = 73) show a persistent and increasing risk of relapse or cancer-related death after 5 years. Classification by immunohistochemistry or intrinsic subtypes did not show this risk (21). The predictive value of IntClust to define response to NACT is yet to be fully established.
3.2.6. Prado-Vasquez Classification
Prado-Vasquez et al. developed a probabilistic graphical model to classify the cellular components of tumours into four groups based on the ‘stem cell hypothesis,’ defined based on the grade of development of the cells from which they derived luminal (LAR), basal, claudin-high (CLDN-high), and claudin-low (CLDN-low). The sparse k-means method was used to define high or low immune activity and to classify the tumour as immune metanode positive or negative. Immune metanode activity was prognostic overall, and particularly in the luminal group defined by the cellular classification and TNBC type4-LAR (23).
Combining molecular knowledge with patient management is an increasingly accepted technique across tumour types. In early TNBC, lack of reproducibility and the absence of a unified approach have led to the continuous use of unselected clinical strategies that remain insufficient. Stable commonalities among the classification methods of molecular subtyping in TNBC suggest the presence of clear biological groups suitable for personalised therapeutic interventions. For instance, luminal-like and mesenchymal tumours are consistently identified across the methods with decent overlap and reproducible outcome data. Moreover, most methods include a measurement of the interaction between tumours and the immune response, highlighting the importance of considering this element as a key component of the TNBC taxonomy. Overall, these efforts provide the basis for understand how the molecular complexity of TNBC influences outcomes. Considering the treatment response due to dynamic network interaction, rather than focusing on individual static components, is likely to have more predictive power. But even with reproducible and reliable classification, delivering this in a clinical timeframe suitable for neoadjuvant therapy decision-making remains a challenge.
Summary Box 1—Biological and clinical features of TNBC
–TNBC is characterised by the absence of ER, PR and HER2 expression and is associated with high early response rates to treatment and poor prognosis.
–TNBC is a heterogeneous disease with a high level of inter and intra tumour heterogeneity.
–Multiple TNBC classifications that split TNBC tumours based on unique molecular features have been described but have yet to be incorporated into routine clinical practice.
4. Overall Approach to the Treatment of Early Stage TNBC
Therapeutic options for early TNBC have traditionally been limited to cytotoxic chemotherapy, surgery, and radiotherapy. Significant advances in basic and clinical research have led to tangible improvements in the current therapeutic arsenal. The FDA has now approved pembrolizumab immunotherapy for use along with chemotherapy for high-risk early-stage TNBC following survival data from the KEYNOTE-522 trial (34). This has established immunotherapy as a new standard of care in the United States, and it is anticipated to reach clinical practise in other countries in the near future. Similarly, the recent FDA approval of Olaparib for the adjuvant treatment of high-risk germline BRCA (gBRCA) carriers following results of the OlympiA trial is expected to reshape clinical practice (35). These encouraging developments highlight the importance of a personalised treatment approach and focus attention on the unresolved challenges of appropriate patient selection and derived toxicity.
Closing the gap between preclinical advances and the clinical setting remains a prolonged and challenging process.
4.1. (Neo)adjuvant Chemotherapy
The effect of polychemotherapy compared with no chemotherapy across all BC subtypes was assessed as part of the 2012 Early Breast Cancer Trialists’ Collaborative Group (EBCTCG) meta-analysis of 32,000 patients. This resulted in a ~50% reduction in 2-year recurrence and a 20–25% decrease in BC (36). Chemotherapy is particularly important in managing TNBC as these tumours demonstrate a better response compared to other subtypes of BC and the importance of achieving and optimising the early treatment response in these tumours is well recognised.
4.1.1. Anthracyclines
Anthracyclines target cell proliferation pathways by interacting with DNA gyrase and leading to DNA double-strand breaks (DSBs). The ABC trials proved that the addition of an anthracycline to taxane and cyclophosphamide improved patient outcomes, with the greatest benefit in high-risk patients, those with lymph node involvement or hormone-negative disease (37). More recently, a large meta-analysis by Braybrooke et al. found an 18% reduction in the 10-year recurrence risk with the addition of anthracycline to taxane chemotherapy, as compared to taxane alone (38). There are multiple anthracycline-taxane-based regimens now in use, with evidence to support one “optimal” standard of care regimen for TNBC lacking (39).
Anthracycline-free chemotherapy regimens are considered when cardiotoxicity is a concern, and routine use of such regimens for treatment de-escalation is an area of increasing interest (40) (see Table 3). Evidence regarding efficacy as a standard treatment for TNBC is conflicting, although a recent meta-analysis has established anthracycline-free chemotherapy to be acceptable for lower risk, early-stage HER2-negative BC (39).
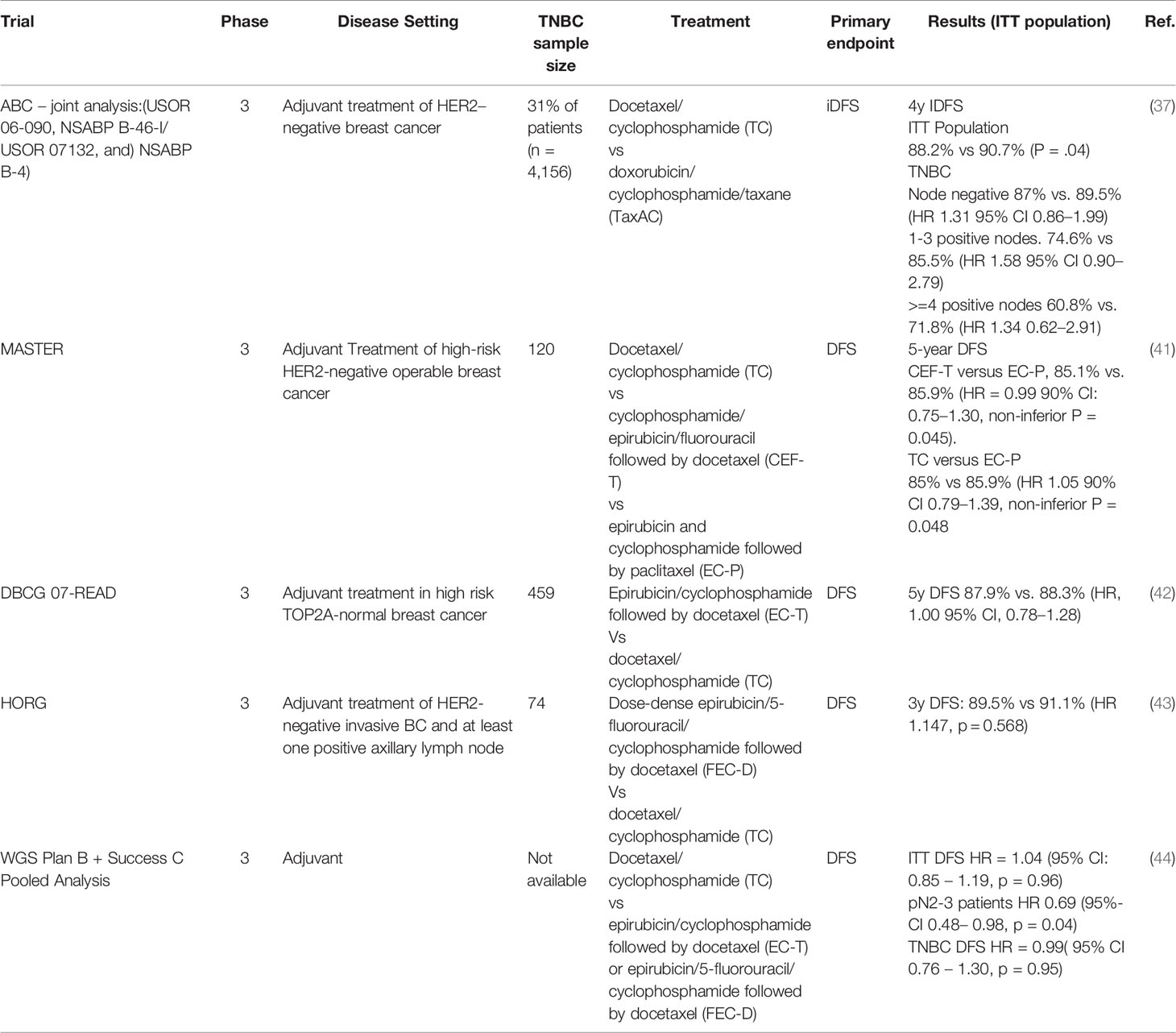
Table 3 Major clinical trials evaluating adjuvant anthracycline-free chemotherapy regimens for patients. with stage I-III TNBC.
4.1.2. Microtubule Targeting Agents
Taxanes inhibit cell division by stabilising microtubules, preventing depolymerisation, spindle formation, and progression through the cell cycle. Paclitaxel and docetaxel are regularly used to treat early-stage TNBC. An EBCTCG meta-analysis showed that the addition of taxane to anthracycline resulted in a proportional reduction in mortality rates of 15–20% (36). The European Cooperative Trial in Operable Breast Cancer (ECTO) also demonstrated significant improvements in RFS and distant RFS (45). Although this evidence is not unique to TNBC, these studies provide the strongest evidence to support taxane use in this cohort. BL1 and BL2 tumours appear to derive an increased benefit from this drug class (46).
There are several novel alternatives to traditional taxanes under investigation. Nab-paclitaxel is a solvent-free albumin-bound nanoparticle formulation of paclitaxel. It potentially enables higher intra-tumoural taxane concentrations, better efficacy, and improved tolerability. The GeparSepto (47) and ETNA trials (48) showed conflicting results with a significant difference in pCR rates seen only in GeparSepto (Table S2), which may reflect the relative dose intensities used.
Epothilones are promising alternatives to taxanes in development. These novel potent microtubule stabilisers can bypass common resistance mechanisms seen with taxanes, such as drug efflux pumps and β-tubulin. In the early setting, the phase 3 TITAN trial has shown similar efficacy and reduced rates of peripheral neuropathy, dose modifications, and discontinuation with Ixabepilone comparised with paclitaxel (49).
4.1.3. Platinum Salts
The clinical activity of platinum agents has been significantly associated with a DDR vulnerability in both sporadic and gBRCA-associated TNBC. Carboplatin is increasingly used in neoadjuvant regimens, improving both pCR and long-term outcomes (50). Please see section DNA Damage Response (DDR).
4.1.4. Capecitabine
Capecitabine is an oral prodrug of the antimetabolite 5-fluorouracil. Capecitabine is not currently recommended in clinical guidelines for the neoadjuvant or adjuvant treatment of TNBC, though it is selectively used as a post-neoadjuvant treatment for residual TNBC. Insights for use in the adjuvant setting are accumulating (Table 4), but in most cases, studies have not incorporated the molecular features of the TNBC cohort into the planned analysis for response assessment. The recent phase 3 CBCSG-010 trial for unselected patients with TNBC with concomitant use of capecitabine 1,000 mg/m2 and standard anthracycline-taxane adjuvant chemotherapy (ACT) established a significant 5-year disease free survival (DFS) benefit (53). This is supported by the FINXX trial (54) and the Ye et al. meta-analysis, which demonstrated improved DFS and OS with a tolerable increase in toxicity (56).
4.2. Bone Modifying Agents
Adjuvant bisphosphonates are recommended for breast cancer patients with low-oestrogen status at high risk of relapse to decrease skeletal metastases and improve OS and DFS, as evidenced by the AZURE trial and the EBCTCG meta-analysis, both of which included patients of all BC subtypes (57, 58). While most of the evidence for bone modifying agents in TNBC comes from studies of patients receiving ACT, benefit is also probably derived in the neoadjuvant setting (59). A subgroup analysis of patients receiving neoadjuvant ZA alongside NACT in the AZURE trial led to improved pCR rates (60). The role of RANK-L remains under investigation. The D-CARE trial of adjuvant denosumab showed no improvement in bone metastasis free survival, invasive disease free survival (iDFS), or OS in high-risk early breast cancer. This suggests that the mechanisms by which bisphosphonates act against the metastatic potential of BC cells are broader and more sustained than the known effects on bone cell function (61).
Summary Box 2—Standard of care treatments in TNBC
−Sequential anthracycline-taxane based regimens are considered standard of care.
−Anthracycline-free chemotherapies are considered for lower risk tumours or in patients where cardiotoxicity is a concern.
−Taxane-free chemotherapy or use of an alternative microtubule stabiliser is considered in patients with peripheral neuropathy or taxane hypersensitivity reactions.
−Bisphosphonates are recommended for the treatment of operable breast cancer of all subtypes in patients with low oestrogen states, whether natural or induced. They should particularly be considered in patients at high risk of relapse or treatment-related bone loss.
4.3. Treatment Schedule
4.3.1. Neoadjuvant vs. Adjuvant Chemotherapy
Chemotherapy can be delivered in an adjuvant or neoadjuvant setting with no significant difference in long-term outcomes, as illustrated by the NSABP B-18, EORTC 10902, and IBBGS trials (62–64). More recently, an EBCTCG meta-analysis demonstrated no significant difference in distant recurrence or death between NACT and ACT but a more frequent local recurrence rate (65). A TNBC-specific meta-analysis suggested that NACT is associated with a comparable DFS but worse OS than ACT (66), perhaps explained by patients with higher disease burden being more likely to receive NACT. In this meta-analysis, patients who achieved pCR had superior OS and DFS compared to those treated with ACT. This evidence does not support the suggestion that NACT promotes cancer cell dissemination (67).
Advantages of NACT include downstaging tumours, resulting in increased rates of breast-conserving surgery and associated improved cosmesis and reductions in postoperative lymphoedema. Additionally, it allows the assessment of treatment response, provides valuable prognostic information (68), guide choice of post-surgical treatment and allows for ineffective treatment to be ceased to avoid unnecessary toxicity. NACT also provides an ideal platform for translational research, assessment of biomarkers, and genetic testing (69).
The same anthracycline/taxane-based regimens are typically used in NACT and ACT. Whether the scheduling of these combinations has any effect on efficacy has been the subject of extensive research. It is been shown that using taxanes and anthracyclines sequentially increases efficacy and decreases toxicity (70). Some evidence suggest that administration of taxane chemotherapy before anthracyclines is associated with improved pCR rates (71).
4.3.2. Dose-Dense and Metronomic Chemotherapy
There has been an increasing interest in personalising the treatment timetables to take patient and tumour characteristics into account. Dose-dense NACT is now a widely accepted treatment strategy for high-risk TNBC in order to prevent cancer cell repopulation (72). It has been consistently shown to improve the rates of pCR, breast-conserving surgery, and recurrence in hormone-low BC (73, 74). Although this regimen has not translated into a significant survival benefit (74), this approach should be considered in selected patients with a high disease burden. Dose-dense ACT improves DFS and OS rates in patients with low hormone receptor levels, although this is accompanied by increased toxicity and patients need to be selected carefully (75).
At the other end of the spectrum, metronomic chemotherapy is given at a minimum biologically effective dose either continuously or with minimal extended breaks from treatment to reduce severe toxicity. It is thought to have angiogenic, stroma-targeting, and immunostimulatory effects (76). It has been investigated as a single approach and is being used in combination to intensify standard chemotherapy. It may have a role as a maintenance therapy for high-risk patients or for use by patients who would not otherwise be able to tolerate the adverse effects of standard treatments. The SYSUCC-001 study showed significant improvement in 5-year DFS with 1 year of maintenance capecitabine (55). The IBCSG 22-00 trial confirmed a 7.9% reduction in the absolute risk of relapse in patients with node-positive TNBC (77) after 1 year of low-dose capecitabine and methotrexate maintenance treatment, although no improvement in DFS was observed.
4.4. Assessing Response to NACT
Residual Cancer Burden (RCB) classifies tumour response to chemotherapy using a numeric score based on four characteristics of surgical outcome: primary tumour bed dimensions; cellularity fraction of invasive cancer; size of largest metastasis; and number of positive lymph nodes (68). Four prognostic categories were established (Table 5). It has been shown that NACT achieves a pCR in slightly over a third of patients with TNBC, and these patients enjoy excellent long-term survival outcomes (78, 79). Higher rates of pCR following NACT are seen in TNBC as compared to other subtypes, despite the high rate of disease relapse in this cohort. This is believed to derive from poor outcomes in patients with residual chemotherapy-resistant disease (80). RCB after NACT can accurately predict both event-free survival (EFS) and DFS and is commonly used as a surrogate outcome in clinical trials (79).
Liquid biopsies for circulating tumour DNA (ctDNA) measurement are a promising dynamic approach to assess residual disease and predict treatment response in real-time (81). Fragments of DNA released by apoptosed or necrosed tumour cells can be longitudinally measured in the blood samples of patients. Detection of high ctDNA levels at the time of surgery has been associated with reduced DFS and OS rates, and clearance of ctDNA during NACT has been associated with improved outcomes across all BC subtypes (82). Clinical trials that incorporate this approach for patient selection are imminent.
4.5. Post Neoadjuvant Treatments
Patients with residual disease after surgery are often considered for further systemic therapy. Current treatment options in this setting following NACT include capecitabine and poly ADP-ribose polymerase inhibitors (PARPi) for gBRCA carriers.
The Create-X trial demonstrated that six to eight cycles of capecitabine improved 5-year DFS and OS compared to no further therapy, especially in the TNBC cohort (51). In contrast, the GEICAM/2003-11_CIBOMA/2004-01 trial failed to show a statistically significant increase in DFS with the use of eight cycles of adjuvant capecitabine. Of note, a pre-planned analysis of this study showed that the non-basal TNBC cohort derived the most benefit from receiving capecitabine (52). Significant differences in study populations limit direct comparisons between these two studies. Create-X enrolled an Asian population who are highly efficient metabolizers of fluoropyrimidines, all of whom had high-risk pathologically-assessed residual disease. In contrast, GEICAM/CIBOMA accrued patients from Europe and South America, only 80% of whom had residual disease. Meta-analyses on the topic have concluded upon an overall improvement in DFS and OS with capecitabine (83) and opinions from the St. Gallen International Conference found 87% of experts would offer capecitabine to patients with residual TNBC in the post-neoadjuvant setting (84). Differences in outcomes at a population level and issues with toxicity have led to capecitabine being offered on a case-specific basis rather than as a standard of care (85). The GEICAM/CIBOMA data indicate that more detailed investigation is needed into exactly which TNBC sub-types would benefit from capecitabine.
The OlympiA trial recruited 1,836 patients with HER2-negative cancers, 82% classified as TNBC, and showed that 52 weeks of adjuvant olaparib was associated with a significant DFS improvement in patients with gBRCA1/2 mutations (3-year iDFS of 85.9% for olaparib vs 77.1% for placebo) (35). A 32% reduction in the risk of death versus placebo (HR = 0.68; 95% CI 0.50–0.91; p = 0.0091) led to the recent FDA approval for olaparib in this setting.
The optimal treatment for residual disease after NACT remains a matter of debate, particularly for gBRCA carriers with high-risk TNBC. A direct comparison between adjuvant olaparib and capecitabine is unavailable. The theoretical advantage for olaparib use includes targeting a known tumour susceptibility in a selected population, leading to an improved response and improved tolerability compared to standard cytotoxics. Interestingly, a phase 2 trial that assessed the value of molecularly targeted postneoadjuvant treatment vs choice of clinician in TNBC patients with residual disease did not demonstrate the superiority of this approach (86). Despite the limitations with regard to the primary outcome, an example was set for biomarker-driven clinical trials and the use of ctDNA in optimising the selection of biomarker-treatment partners. Patient preference and financial issues clearly need to be considered in this setting.
Summary Box 3—Key concepts in the current treatment of TNBC
−Chemotherapy can be given in the adjuvant or neoadjuvant setting and the same regimens are typically used. Long-term survival outcomes are similar.
−Advantages of NACT include a rapid evaluation of tumour response, prognostication using RCB scoring, and improved surgical outcomes.
−RCB is strongly associated with long-term outcomes in TNBC.
−Patients with TNBC who are at increased risk of relapse after chemotherapy in the neoadjuvant setting benefit from adjuvant capecitabine. Patients in the gBRCA subgroup benefit from PARP inhibitors.
−Sequential liquid biopsies to assess ctDNA levels represent a possibility for monitoring treatment response in real-time.
5. Targetable Molecular Pathways
Much progress has been made in defining and treating TNBC according to aberrations on the molecular level, although the derivation and use of biomarkers to select patients for specific treatments has been somewhat lacking. To make further progress, the identification of predictive biomarkers must be a central focus of our research and, once secured, used to guide and to select patients most likely to derive benefit from targeted treatments.
Tables S3–S5 summarise ongoing trials contributing to the use of molecularly targeted treatments for early TNBC.
5.1. DNA Damage Response (DDR)
TNBCs are frequently deficient in DDR pathways and exhibit high chromosomal instability (7, 87). The repair of DNA double-strand breaks (DSBs) relies on the homologous recombination (HR) pathway. Dysfunctional activity of genes involved in this process compromises the ability of cells to mend DSBs, thereby inducing Homologous Recombination Deficiency (HRD) (88).
HRD can occur via numerous mechanisms, all resulting in similar phenotypic and genotypic features to those of BRCA mutant tumours, an observation termed ‘BRCA-ness’. Phenotypic and molecular similarities between BRCA-associated BC and sporadic TNBC have led to the application of similar therapeutic interventions in both groups. In patients with BRCA mutations and BRCAness features, a compromised DDR pathway facilitates increased sensitivity to drugs such as platinum and PARPi, based on the concept of synthetic lethality (89).
Approximately 10–20% of TNBC harbour gBRCA mutations, and 70% of gBRCA1 and 16% of gBRCA2-associated tumours are classified as TNBC (90). Somatic BRCA mutations are uncommon in sporadic TNBCs (6, 24, 32). BRCA1 and 2 mutations, and hypermethylation of the BRCA1 promoter, only account for some TNBCs that exhibit functional evidence of HRD. In total, around 40% of BCs are identifiable as HRD in the absence of these changes (91). Dysfunctional BRCA pathways are frequently enabled by other mechanisms; for example, RAD51 and PALB2 mutations can confer a BRCA-ness phenotype (91).
5.1.1. Therapeutic Approaches
5.1.1.1. Platinum Agents
The cytotoxic activity of platinum is mediated by the formation of platinum–DNA adducts that interfere with DNA replication and transcription, activating DNA-damage recognition and repair, cell-cycle arrest, and apoptosis.
Platinum-containing regimens have not been regarded as the standard of care for treating TNBC in most guidelines to date. Several trials have investigated the addition of platinum agents to standard chemotherapy for this subgroup based on the potential-increased susceptibility of TNBC to DNA-damaging compounds (30) (Table 6). Improved pCR rates with the addition of carboplatin have been a consistent finding, with confirmed EFS benefit in two large randomised studies, GeparSixto and BrighTNess (92–95, 101–103). These results have led to the inclusion of carboplatin within neoadjuvant regimens for high-risk TNBC in the American Society of Clinical Oncology guidelines.
Combining carboplatin with anthracycline/taxane NACT increases haematological and gastrointestinal toxicity, which in turn has implications for patient selection. Predictive biomarkers to identify those patients deriving the most benefit from the addition of platinum, for example, gBRCA mutations, have been investigated. Single-agent cisplatin has shown conflicting results for BRCA carriers (96). The PARTNER (NCT03150576) trial includes a cohort of gBRCA-mutated patients (100) and will help elucidate the effect of platinum and PARPi in this subgroup.
There is currently no routine indication for platinum agents in the post-neoadjuvant setting. The EA1131 study (NCT02445391) was closed early as neither cisplatin nor carboplatin was able to demonstrate non-inferiority or superiority over capecitabine, and toxicity rates were higher (97).
5.1.1.2. PARP Inhibition
Poly ADP-ribose polymerase (PARP) activity is crucial for maintaining the correct fork speed and fidelity of DNA synthesis. PARP1 is involved in the response to single-strand DNA (ssDNA) damage and maintains genome integrity via base excision repair. PARP1 is also a critical early event for DNA DSB repair activation and regulation of resection (104). PARP inhibition causes replication stress, induces ssDNA breaks and affects the normal regulation of p53 and its downstream effectors (105). In tumours that have deficiencies in the HR pathway, the accumulation of DSBs originating from primary ssDNA breaks leads to cell cycle arrest and death (106).
Robust evidence now supports the efficacy of single-agent PARPi in BC patients with gBRCA mutations who have received prior chemotherapy (107, 108). A variety of PARPi and combinations have now been explored in both patients with gBRCA mutations and sporadic (non-BRCA) TNBC in the early setting.
Evidence to date for the use of olaparib is promising, both as monotherapy and in combination with chemotherapy, immunotherapy, or radiotherapy. In the neoadjuvant setting, olaparib was given as monotherapy in 32 patients with unselected TNBC for up to 10 weeks before chemotherapy (109) with an overall objective response rate of 56.3% vs 51.9% among patients not harbouring gBRCA1/2 or germline PALB2 mutations. A numerical enrichment of somatic HR mutations and BRCA1 methylation in the responding group suggests favourable activity of olaparib here. Other trials in the neoadjuvant setting combine olaparib with chemotherapy. GeparOLA included patients with HER2-negative BC and HRD, received paclitaxel with olaparib or carboplatin followed by epirubicin and cyclophosphamide (110). No formal testing between the arms was planned but increased benefit from olaparib was observed in young (<40 years) and HR-positive patients. In the TNBC subgroup, the pCR rate was 56.0% with olaparib and 59.3% with carboplatin. PARTNER is a phase 3 trial that assesses the addition of olaparib to neoadjuvant platinum-based chemotherapy in the treatment of TNBC and gBRCA-derived tumours. Preliminary safety results show that the combination of olaparib and platinum has an acceptable and manageable toxicity profile (111). In the I-SPY2 trial, research arm patients received olaparib and Durvalumab with paclitaxel, then doxorubicin and cyclophosphamide (112), which increased pCR in the TNBC group (27–47%). Immune-rich tumours had greater sensitivity to this treatment. The adjuvant phase 1 RadioPARP trial for patients with inflammatory, locoregionally advanced or mTNBC, or patients with residual disease after surgery for TNBC, sought to evaluate safety and dosing for olaparib in combination with radiotherapy (113). Olaparib was escalated to the maximum target dose of 200 mg twice daily with no dose-limiting toxicity.
Talazoparib has been reviewed in the neoadjuvant setting as monotherapy and along with chemotherapy. TALA was a pilot study that recruited 20 patients with operable BC and a BRCA mutation to receive talazoparib monotherapy for 6 months (114). Despite the small sample size, this trial showed an encouraging pCR rate of 53% and RCB-0/I of 63%, with a manageable safety profile. In the I-SPY2 trial, talazoparib combined with irinotecan for HER2-negative patients had limited activity beyond that seen with standard treatment (115).
Veliparib has also been evaluated in the neoadjuvant setting in the I-SPY2 trial (116). The addition of veliparib to carboplatin-containing chemotherapy increased the pCR rate in the TNBC group from 26 to 51%. This combination was further assessed in the phase 3 BrighTNess trial in 634 patients with TNBC (94), where no additional benefit for veliparib above that achieved by adding carboplatin, regardless of BRCA mutation status, was found. A key limitation of this study is the low dose of veliparib, less than half of that used in the BROCADE-3 study in the advanced disease setting (117). Veliparib has been combined with radiotherapy for inflammatory or locoregionally recurrent TNBC, which results in significant local toxicity (118).
Both talazoparib and olaparib are effective as monotherapies in patients carrying gBRCA mutations. Given the low dose of velaparib used in the BrighTNess trial, and considering individual PARPi differences in PARP trapping capacity, the potential summative benefit from the addition of platinum to PARPi cannot be excluded. This encourages further investigation into the role of other PARPi such as olaparib and talazoparib and the great potential for combination therapy, as demonstrated by the ongoing trials in Table S3.
5.1.1.3. Other DDR Agents
The ATR inhibitor Ceralasertib (AZD6738) is being investigated as monotherapy in chemotherapy-resistant TNBC as part of a pre-surgical window of opportunity and post-surgical biomarker study (NCT03740893, PHOENIX), reviewing the change in mean proliferation index between baseline and post-treatment. PARTNERING is a phase 2 sub-study for the PARTNER trial that offers durvalumab along with AZD6738 to patients with evidence of residual disease after completion of NACT and before surgery. WEE 1 inhibitors have not yet been reviewed in the early TNBC setting.
Table S3 summarises the major incomplete clinical trials involving DDR agents in patients with stage I–III TNBC.
Summary Box 4—DNA damage response: treatment strategies
−There is strong evidence to support the addition of platinum agents to NACT to improve patient outcomes, especially in high-risk and gBRCA carriers.
−Improvements in pCR and EFS rates with platinum chemotherapy combinations need to be balanced against additive chemotherapy toxicities.
−PARP inhibition causes replication stress, induces ssDNA breaks and affects the normal regulation of p53 and its downstream effectors.
−Encouraging evidence supports the efficacy of single agent PARPi in BC patients with gBRCA mutations who have no prior chemotherapy exposure.
−The group of patients with TNBC most likely to benefit from PARP inhibition in the neoadjuvant setting is yet to be established.
−Olaparib improves DFS in gBRCA carriers with high-risk HER2 negative disease following neoadjuvant or adjuvant chemotherapy.
5.1.2. Predictive Biomarkers of DDR Agents Response
5.1.2.1. BRCA Mutations
The predictive value of both gBRCA and somatic BRCA mutations for response to platinum and PARPi has been validated in large clinical trials that included patients with ovarian and metastatic BC (108, 114). The role of BRCA status as an independent predictive biomarker for the TNBC population in the neoadjuvant setting is still unclear, with studies showing conflicting results. In a secondary analysis of the GeparSixto trial (n = 50) (119), gBRCA mutations were predictive of higher pCR rates and carboplatin did not increase this further. In the CALGB 40603 trial, pCR rates in patients with gBRCA mutations were similar to the overall population, and this outcome was not altered by the addition of carboplatin (120).
BRCA1/2 mutation carriers with the TNBC subtype in the I-SPY 2 trial were significantly more likely to achieve a pCR than non-BRCA TNBC (predicted pCR of 75% vs. 29%) (121) and a greater response was seen for patients with a BRCA-ness signature (116). Subgroup analysis of the BrighTNess trial did not show a difference in pCR rate based on BRCA status (93). However, in the GeparOcto trial (122) gBRCA mutation carriers gained greater benefit from platinum (68.1% vs 45.7%, p = 0.005), particularly in the TNBC subgroup (74.3% vs 47%, p = 0.005).
In the PETREMAC trial, in which patients received olaparib monotherapy before chemotherapy, pathogenic mutations (germline or somatic) in the HR pathways and/or BRCA1 promoter methylation were associated with olaparib and its overall response (OR) of 88.9% (109). Although pCR rates in the GeparOLA trial for gBRCA1/2 carriers were significantly higher than those in non-carriers (62.7% vs 41.3%, P = 0.047), exploratory analysis revealed no difference between treatment arms if somatic or germline BRCA1/2 mutations were detected (110).
5.1.2.2. HRD by Gene Set Analysis and Functional Assays
Several attempts to simplify and systematically identify common molecular changes associated with defective HR have been published. The evaluation of DNA damage repair-related genes by either gene expression or by the presence of mutations has shown a positive association with response. Confirmation of the predictive value of these individual efforts has not always been accomplished given the underlying heterogeneity of some of these variations (Table 7).
5.1.2.3. HRD by Genomic Scars and Mutational Signatures
The detection of mutational signatures that uniquely identify patterns of defective HR repair has been the subject of several studies. Vollebergh et al. assessed whether array comparative genomic hybridisation patterns could predict the benefit of intensified carboplatin-based chemotherapy (126). An HRD score defined by an unweighted sum of loss of heterozygosity, telomeric allelic imbalance, large-scale transition, and BRCA1/2 mutations has been tested in TNBC treated with platinum, and used to aid patient selection in PARPi trials (128, 129). In the absence of gBRCA mutations, a high HRD score was associated with higher pCR rates irrespective of the use of carboplatin. The microhomology-mediated indels, HRD index, single base substitution signature 3, rearrangement signatures 3 and 5, and genomic instability markers of HRD are aggregated into the HRDetect score (91). The prognostic value of HRDetect has been demonstrated in two retrospective clinical cohorts, and further evaluation of its predictive power in randomised clinical trials is awaited.
HRD has yet to be used to guide the clinical management of TNBC despite its theoretical significance. The absence of a standardised definition of HRD beyond gBRCA mutation and the lack of prospective clinical trial data currently limits its clinical utility.
5.1.2.4. Tumour Mutational Burden
More tumour mutations could be correlated with an enhanced response to drugs causing DNA damage. For example, somatic hypermutation was shown to be an independent factor for estimating the risk of platinum sensitivity in high-grade serous ovarian cancer (OR = 3.616, p = 0.002) (132). A higher tumour mutational burden (TMB) has been observed in BCs that harbour DDR gene mutations (133), although the correlation with response to platinum is not yet established. In the PETREMAC trial, no difference in TMB was observed between responders and non-responders, or BRCA carriers versus non-carriers (109).
Summary Box 5—DNA damage response: Biomarkers
−The role of BRCA status as an independent predictive biomarker among the TNBC population in the neoadjuvant setting is unclear.
−Overall, alterations in DNA damage repair-related genes by either gene expression or presence of mutations has shown a positive association with response to NACT and/or PARPi.
−Mutational signatures predictive of BRCA1/BRCA2 deficiency or a `BRCA-ness status` have shown a trend to positive association with response to platinum chemotherapy. However, these results are signature specific and should be considered preliminary. Data from randomised clinical trials that prospectively assess the value of these biomarkers is awaited.
−Higher TMB has been observed in BC tumours that harbour DDR gene mutations. Correlation with response to platinum agents is not yet established.
5.2. Immune Response
Although BC is largely considered an immune-quiescent cancer type (134), increasing evidence suggests that a range of tumour immunogenicity is present. TNBC is characterised by increased immune activation and wide immune heterogeneity compared to other BC subtypes (135).
5.2.1. Therapeutic Approaches
Tumours evade detection and eradication by the immune system through the dysregulation of pathways controlled by immune checkpoints. Immunotherapy harnesses the immune system of the patient to target malignant cells using immune checkpoint inhibitors (ICI), chimeric antigen receptor T cells, or cancer vaccines. ICIs release the immune system from tumour-induced inhibitory signals, allowing an effective anti-tumour response. They include monoclonal antibodies (mAbs) against cytotoxic T lymphocyte-associated antigen-4 (CTLA-4), programmed cell death-1 (PD-1), and programmed cell death ligand-1 (PD-L1).
5.2.1.1. Monoclonal Antibodies Against PD-1
Pembrolizumab is the most well-established and successful anti-PD-1 ICI in operable TNBC. The addition of pembrolizumab to NACT has shown increases in pCR rates across several RCTs, including the KEYNOTE-173 and I-SPY 2 trials (136, 137). These successes led to the landmark phase 3 KEYNOTE-522 trial, which has culminated in the FDA approval for use of pembrolizumab in high-risk early-stage TNBC, the first regulatory approval for an immunotherapy agent in this setting. Pembrolizumab is now considered a standard of care treatment in the United States for patients fitting trial eligibility criteria.
KEYNOTE-522 evaluated neoadjuvant pembrolizumab along with carboplatin/paclitaxel and anthracycline-based NACT, and then adjuvantly as monotherapy, in high-risk early TNBC. The pCR rate improved by 7.5% (95% CI: 1.6 to 13.4%) with the addition of pembrolizumab, and after a median follow-up of 39.1 months, 36-month EFS improved from 77 to 85% (HR: 0.63; 95% CI, 0.48 to 0.82; P <0.001). OS data remains immature at the time of analysis (34). High-risk patients derived the greatest benefit, with higher absolute improvements in pCR in stage III and node-positive disease. There are some limitations to this study. With this trial design, it is not possible to elucidate the relative contributions of the neoadjuvant and adjuvant treatment phases to these EFS results. Concern has been raised at the rate of serious adverse events (77% incidence of grade ≥3 events in the immunotherapy group) and immunotherapy-related adverse effects (irAE) (affecting 33.5% of patients on this trial) due to their protracted nature. It is therefore imperative to detect predictive biomarkers to facilitate the selection of patients likely to derive the most benefit from immunotherapy and treatment de-escalation strategies. No predictive biomarkers were identified in this trial. Improvement in pCR rate was seen regardless of PD-L1 status (138). Patients on the pembrolizumab arm that achieved pCR derived a modest survival benefit (approximately 2%), as compared to 10% in the cohort of patients with residual disease at surgery. This suggests that the value of adjuvant pembrolizumab as a monotherapy may be small in the group that achieved pCR. Removal of the adjuvant portion of treatment based on response to surgery could represent a potential treatment de-escalation strategy that requires further exploration.
5.2.1.2. Monoclonal Antibodies Against PD-L1
Atezolizumab, durvalumab, and avelumab are the most established anti-PD-L1 ICIrs being investigated in operable TNBC, although results from trials have been inconsistent. The pCR rate improved from 41 to 58% with the addition of atezolizumab to anthracycline/taxane-based chemotherapy in Impassion031 (139). Secondary endpoints (EFS, DFS, and OS) are expected later this year. However, this trial is not powered to show survival differences. The phase 3 NeoTRIPaPDL1 trial failed to show a significant pCR advantage with the addition of atezolizumab to neoadjuvant carboplatin and nab-paclitaxel (140), although EFS was the primary endpoint and this data is not yet available. These incongruent results are likely to reflect the higher-risk patient population in NeoTRIPaPDL1 and the difference in the chemotherapy backbone. Results from the TONIC trial suggest anthracycline chemotherapy, used in Impassion031, leads to a potentiation of the effects of immunotherapy (141). These insights should inform the choice of chemotherapy backbone in the design of future immunotherapy trials.
GeparNUEVO assessed Durvalumab in addition to anthracycline/taxane-based NACT. This showed a non-significant 9% improvement in pCR rate. Improvements in 3-year iDFS and 3-year OS were also seen, though this trial was not powered to definitively assess long-term survival differences. An underpowered subgroup analysis showed a particular benefit in patients who received durvalumab alone for two weeks prior to NACT, suggesting immunological interactions with priming in this window phase (142, 143). While the small patient cohort included in GeparNUEVO has resulted in statistically non-significant pCR and iDFS benefits, the results are similar to those from KEYNOTE-522. This is despite lacking a platinum agent and an adjuvant treatment phase. These represent potential treatment de-escalation avenues that could benefit from further exploration. Discrepancy between the magnitud of benefit for pCR rate and survival seen across both trials suggest the value of pCR as a marker for long term survival in immunotherapy trials requires further exploration. Published and ongoing trials of ICI have been summarised in Tables 8, 9.
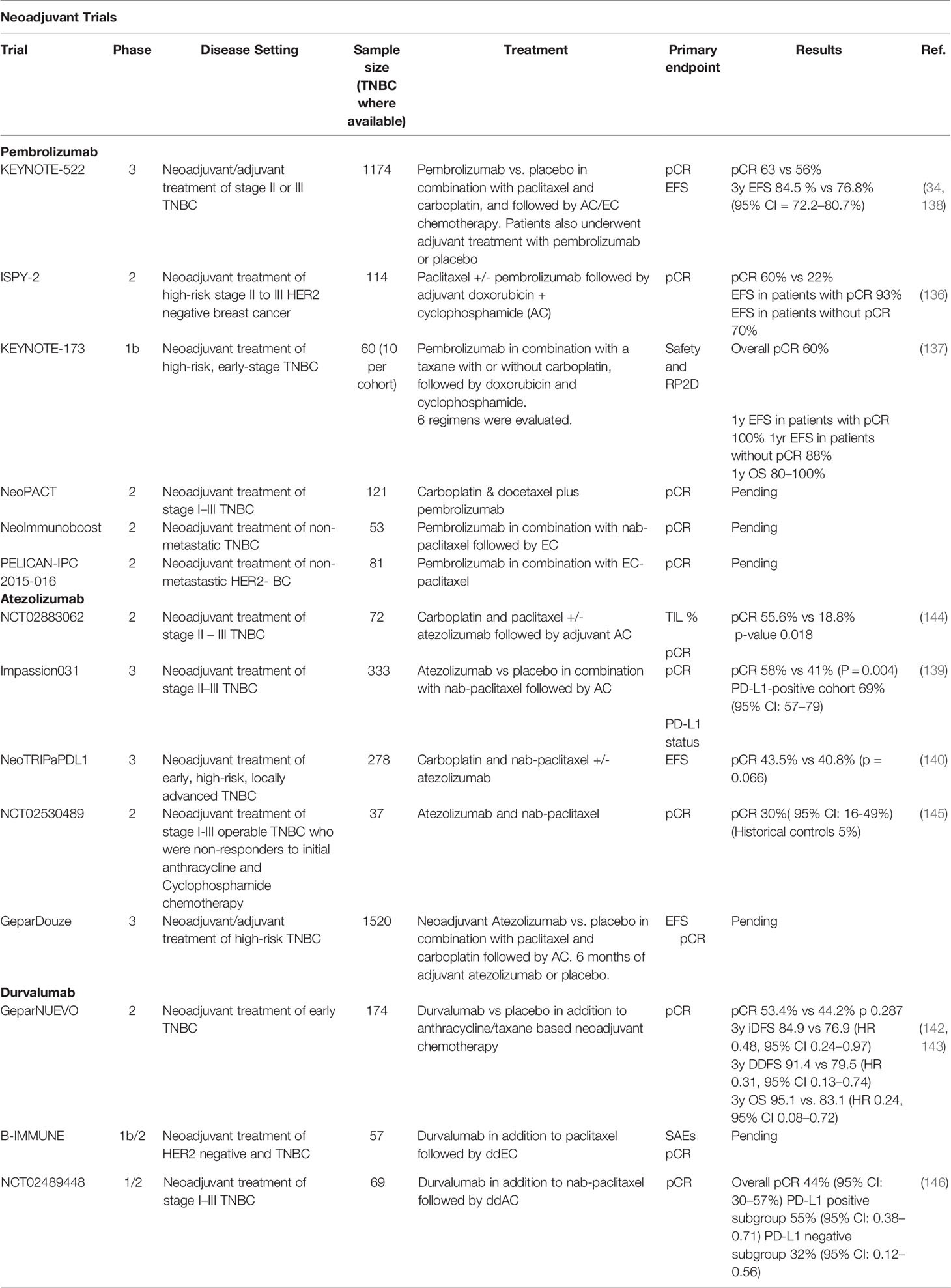
Table 8 Major neoadjuvant trials that include immune checkpoint inhibitors in patients with stage I–III TNBC.
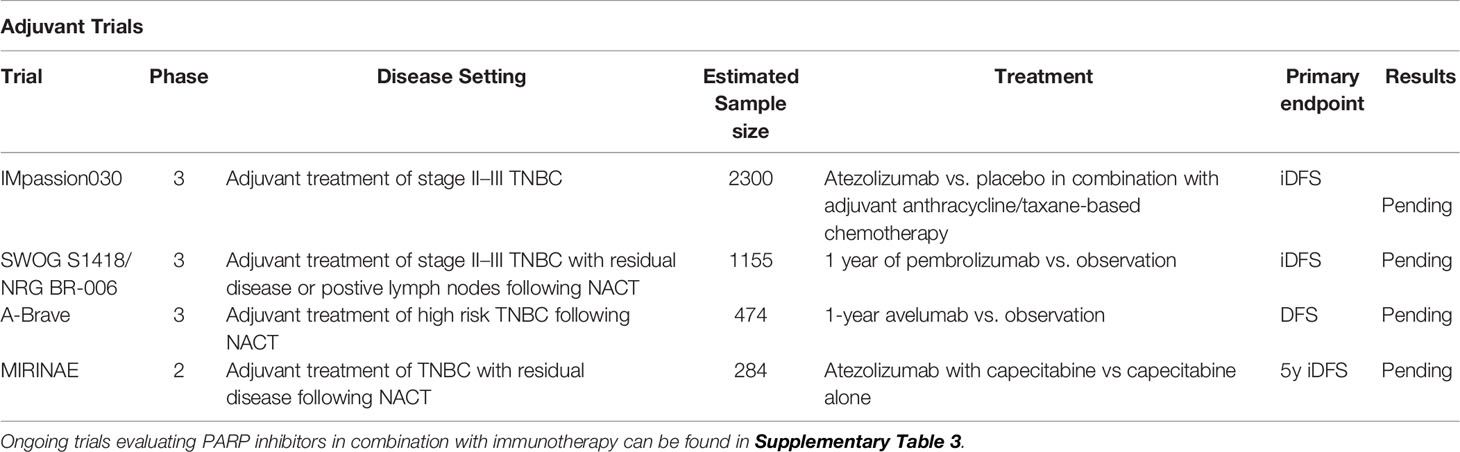
Table 9 Major adjuvant trials that include immune checkpoint inhibitors in patients with stage I–III TNBC.
The use of ICIs in TNBC is an area of active research, although it is at an early stage, and long-term outcome data remain immature for the majority of the neoadjuvant trials. Concern regarding the use of pCR as a primary endpoint upon which to grant regulatory approval for neoadjuvant pembrolizumab was cited by the FDA, and long-term survival data is of particular interest (147). There is a paucity of data available to guide use of pembrolizumab in the adjuvant or post-neoadjuvant setting, particularly in combination with agents such as capecitabine or olaparib, used in more contemporary practice. This represents a challenge when adopting pembrolizumab as the standard of care treatment, and the results of trials investigating these issues are highly anticipated.
5.2.1.3. Cancer Vaccines
Cancer vaccines use tumour associated antigens to stimulate CD4+ and CD8+ T cells, inducing the immune system of the patient to target cancer cells that were previously successfully evading immune suppression. They have yet to show success in late-stage clinical trials or to receive regulatory approval for TNBC. Clinical trials evaluating cancer vaccines in non-metastatic TNBCs are listed in Table S4.
Summary Box 6—Immune response: Treatment strategies
−Immunotherapy is of particular interest in TNBC due to the higher degree of immune activation seen in comparison to other BC types.
−TNBC is a heterogeneous disease that exhibits various degrees of immunogenicity.
−Several early stage BC trials have established PD-1 and PD-L1 ICIs as a promising treatment option in combination with chemotherapy.
−Pembrolizumab has been granted FDA approval in the neoadjuvant setting for high-risk early-stage TNBC in combination with chemotherapy and to continue as monotherapy in the adjuvant setting (KEYNOTE-522).
5.2.2. Predictive Biomarkers of ICI Response
5.2.2.1. PD-L1
PD-L1 expression is higher in TNBCs compared with non-TNBCs (135) and quantification is currently performed using five distinct FDA-approved companion diagnostic tests across tumour types. The variety in assays, scoring systems, and cut-off values renders the interpretation of its predictive value challenging (148). Increased pCR rate in PD-L1+ early-stage TNBC is seen, but rather confusingly, ICI benefit independent of PD-L1 status has been consistently described (138, 139, 143). In the GeparNUEVO trial, pCR rate was increased in PD-L1+ tumours in all therapy groups, but PD-L1 did not predict ICI response (143). Similar results were observed in the KEYNOTE-522 and Impassion 031 trials.
5.2.2.2. Tumour Mutational Burden
High tumour mutational burden precipitates enhanced immunogenicity by increasing the number of tumour antigenic peptides or neoantigens that can be recognised by T cells (149). Based on this hypothesis, high TMB has been correlated with an increased response to ICI (150, 151) independently of PD-L1 expression (152). The FDA granted accelerated approval of pembrolizumab as monotherapy for advanced tumours that exhibit high TMB (defined as ≥10 mut/Mb) in 2020 (153). More recently, it has been shown that the association of TMB with response to ICI relies on a positive correlation between CD8+ T-cell level and neoantigen load and differs across tumour types (154).
Due to limited data availability and differences among TMB quantification methods, the role of mutational load as an independent predictive biomarker of ICI response is yet to be defined in TNBC. In the GeparNUEVO trial, TMB was higher in patients with pCR (median 1.87 versus 1.39 mut/MB), and both continuous TMB and the immune GE profile independently predicted pCR (155). In comparison, no difference in pCR rate was observed in patients with high TMB who received ICI compared with other targeted therapies in the ARTEMIS trial (NCT02276443) (156).
5.2.2.3. Tumour Infiltrating Lymphocytes (TILs)
Both intra-tumoural TILs (iTILS) and stromal TILs (sTILs) have prognostic and predictive roles for treating early TNBC and have also been evaluated in this setting as biomarkers of immunotherapy response. In the GeparNUEVO trial (142), sTILs before therapy predicted a higher pCR rate overall and in both therapy groups, but were not predictive of durvalumab response. The increase in iTILs in post-window samples compared with pre-therapeutic samples was predictive of pCR, yet the treatment interaction test did not reach significance (P = 0.085). High TILs were significantly associated with the olaparib response in the PETREMACT trial (109). Criscitiello et al. used the LASSO penalised regression model to develop a 4-gene signature to predict high and low TILs after NACT. A high TIL signature was associated with improved long-term outcomes independent of pCR (157). Overall, increased TILs are associated with a more favourable response to NACT and improved long-term outcomes (158, 159).
5.2.2.4. Immune Signatures
GE immune signatures have been extensively used to describe profiles of immune infiltration and immune cell types that impact on the prognosis of many tumour types, including TNBC (160–163). Few studies have tested the value of GE immune signatures in the prediction of chemotherapy response in the early setting of TNBC. In the SWOG 9313 trial, Sharma et al. (164) evaluated the performance of a DNA damage immune response signature and sTILs as prognostic markers in patients with TNBC treated with adjuvant doxorubicin and cyclophosphamide. DDIR was associated with improved OS and DFS and was moderately correlated with sTILs density (≥20% v, <20%). Using network analysis, Lv et al. identified CXCL9 and CXCL13 as prognostic biomarkers in TNBC. Further testing in two neoadjuvant data sets confirmed its predictive value in the response to chemotherapy (165). An exploratory analysis of the GeparNUEVO trial revealed that predefined TIL and IFN-gamma signatures were associated with an increased pCR rate, without specificity for durvalumab response. The expression of six genes required for immune cell function was significantly correlated with pCR and showed a positive test for interaction with durvalumab plus NACT (166). Further evaluation of the interactions between the tumour and the immune system, as well as its architectural heterogeneity, will provide a more accurate estimation of the individual predictive potential to be derived from immune signatures.
5.2.2.5. Microsatellite Instability Status
Pembrolizumab monotherapy received FDA approval in 2017 for treating advanced mismatch repair deficient solid tumours (167). Although only a small proportion of breast cancers are defined as microsatellite instable, (168) tumours with defects in the mismatch repair pathways are known to have highly upregulated expression of multiple immune checkpoints and increased sensitivity to ICI (169). The introduction of new strategies to facilitate the identification of this biomarker in a low-frequency cohort like TNBC remains a challenge.
Summary Box 7—Immune response: Biomarkers
–Response to ICIs appears to be independent of PD-L1 status in early TNBC.
–High TMB has been correlated with an increased likelihood of response to ICI, particularly in tumours where CD8+ T-cell levels are positively correlated with neoantigen load.
–The role of mutational load as an independent predictive biomarker of ICI response is yet to be defined in TNBC.
–Increased TILs are associated with a more favourable response to NACT and long-term outcomes.
–Modest positive association of GE immune signatures with ICI response has been reported.
–The interaction between TMB and GE immune signatures has been shown to be a promising independent predictor of pCR.
–The dynamics of immune activation after treatment are strongly associated with long term outcome, independently of response rate.
–Tumours with defects in the mismatch repair pathways are known to have highly upregulated expression of multiple immune checkpoints and increased sensitivity to ICI.
5.3. PIK3CA/AKT1/PTEN Pathway
Dysregulation of the PI3K/AKT/mTOR pathway is often observed in TNBC (24, 32), and remains a promising target for the future treatment of this BC subtype. Pathway activation is predominantly via PIK3CA mutations (~9–18%), loss of PTEN (~35%), or INPP4B (~30%), and amplification of PIK3CA (~43%). The frequency of PI3K/AKT/mTOR pathway activation and its spectrum varies by TNBC subtype (24, 32), and is strongly associated with the LAR subtype across classifiers.
5.3.1. Alpha-Specific PI3K Inhibitors
In unselected TNBC, response to PIK3CA inhibitors remains low. The BELLE-4 study evaluated the efficacy of buparlisib in the locally advanced setting for patients with HER2-negative BC along with paclitaxel versus placebo and observed no benefit from PIK3CA inhibition. Worse outcomes were observed in the TNBC cohort treated with the PIK3CA inhibitor, and the lack of benefit was independent of PIK3CA mutation or PTEN loss by immunohistochemistry (170). Shorter treatment duration in the buparlisib arm due to adverse events and longer progression-free survival (PFS) in the placebo arm than anticipated are possible explanations for the worse outcomes in this subgroup. The global lack of activity is possibly due to inadequate patient selection and the absence of an accurate biomarker. Parallel pathway activation could also explain a resistance mechanism that requires addressing.
5.3.2. AKT Inhibitors
Ipatasertib was reviewed in the neoadjuvant setting along with paclitaxel for TNBC patients in the FAIRLANE trial (171). Adding ipatasertib did not significantly increase the pCR rate compared with paclitaxel alone, and this effect was independent of PIK3CA/AKT1/PTEN or PTEN low status. A complete clinical response was absent in the placebo-treated group in patients with tumours defined as the LAR subtype, but was observed in 50% of those treated with ipatasertib. This difference was not evident in pCR rates. Elevated immune scores were more strongly associated with improved outcomes in paclitaxel-treated compared with ipatasertib-treated patients, highlighting the key interaction with the immune system. All ipatasertib-treated patients with low immune scores and complete clinical response had PIK3CA/AKT1/PTEN-altered tumours. MK2206 has been trialled in the neoadjuvant setting in the I-SPY2 trial for stage 2–3 BC of any subtype (172). Patients received paclitaxel chemotherapy with or without MK2206, then AC. pCR for the TNBC group was 40.2% with MK2206 vs. 22.4% without. Following assessment of biomarkers in the AKT pathway in the TNBC subgroup, higher levels of phosphorylated AKT and its substrates were paradoxically associated with a reduced response to MK-2206.
5.3.3. MTOR Inhibitors
Everolimus has been reviewed in the neoadjuvant setting for patients with TNBC along with cisplatin and paclitaxel (173), and along with docetaxel, 5-fluorouracil, epirubicin, and cyclophosphamide (174). No improvement in the response rate has been demonstrated.
The exact contribution of drugs targeting the PIK3CA/AKT1/PTEN pathway in early TNBC has not yet been defined. The complexity of the immune microenvironment and parallel molecular alterations can obscure accurate estimation of clinical benefit if they are not in some way accounted for. It is important to try these therapies in a way that reduces these confounders and separates the TNBC subtypes to determine their individual responses. Current approaches include combining alpelisib with nab-paclitaxel in the neoadjuvant setting (NCT04216472) for anthracycline refractory TNBC with PIK3CA or PTEN alterations, with exploratory objectives to assess biomarkers of response and resistance to alpelisib and nab-paclitaxel combination.
Table S5 summarises ongoing trials that target this pathway in early TNBC.
5.4. AR Pathway
AR expression is found in approximately 10–35% of TNBCs as detected by immunohistochemistry (175, 176). The LAR molecular subtype derived from GE accounts for 20–40% of TNBC and is characterised by the activation of AR, ER, prolactin, and ErbB4 signalling. Tumours defined as the LAR subtype typically contain a higher number of PIK3CA mutations, and the pCR rate following NACT is significantly lower compared to other subtypes. (18–20).
There is a paucity of data for drugs targeting the AR pathway in the early TNBC setting. Enzalutamide has been trialled as monotherapy (177), and along with PIK3CAi in the advanced setting with modest benefit (178). Other AR pathway targeted drugs, for example, abiraterone and bicalutamide, have been reviewed in the advanced setting with modest results (179, 180). Although the overall benefit remains limited, it is unclear if this derives from inadequate patient selection or analogous pathway activation. Results from four trials in the early TNBC setting are highly anticipated.
Table S5 summarises ongoing trials that target this pathway in early TNBC.
5.5. Receptor Tyrosine Kinase Family
5.5.1. HER2
Approximately 35% of TNBCs as defined by immunohistochemistry could be classified as HER2-low (181). Somatic ERBB2 mutations occur in approximately 3% of TNBC (6), and a subset of TNBC tumours are classified as HER2-enriched by gene expression. This biological heterogeneity has expanded therapeutic opportunities in this population of patients. In an exploratory analysis of a cohort of the I-SPY2 trial, activation of HER2-EGFR was identified as a positive predictor of pCR in 49 TNBC patients treated with a pan-HER inhibitor (182). A significant correlation between the response to HER2 inhibition and HER2 pathway activation has been demonstrated in TNBC cell lines (183).
Neratinib has been investigated in the neoadjuvant setting for high-risk clinical stage II or III BC. The pCR rate overall in the I-SPY 2 trial was 37.5% in the neratinib arm, and among patients demonstrating the phosphorylation of HER2 or EGFR (i.e., biomarker-positive for EGFR Y1173 or ERBB2 Y1248), it rose to 63% (184). Encouraging results in the HER2-low–expressing refractory BC setting with Trastuzumab Deruxtecan (OR 37%) (185) and Trastuzumab Duocarmazine (OR 40%) (186) now require translation into the early setting. These trials illustrate the importance of identifying patients categorised as TNBC who are more accurately defined as HER2 low (Table S5).
5.5.2. VEGF
VEGF promotes angiogenesis, invasion, and increases vascular permeability and is an essential element in TNBC formation, progression, and metastasis. VEGF-A expression is higher in TNBC compared with other BC subtypes (187), and enhanced angiogenic potential is associated with poor prognosis in BC (188). Targeting of VEGF has been extensively tested in TNBC, but no clear predictive biomarkers of treatment response have been identified.
Trials targeting VEGF in the neoadjuvant TNBC setting have shown disappointing results to date, with no difference in DFS or OS. The addition of bevacizumab significantly increased the rate of pCR among patients with Her2-negative disease in some studies (103, 189–191). The ARTemis and GeparQuinto trials reported increased benefits primarily in the TNBC subgroup. In the adjuvant setting, the BEATRICE trial added bevacizumab to anthracycline and/or taxane-based chemotherapy (192), and no difference in iDFS or OS between treatment groups was found. The underlying reason for the lack of treatment effects with these drugs is poorly understood. It is possible that a fundamental flaw in either the drug or the signalling pathway is being overlooked. Attempts to overcome drug resistance using novel agents and combinations are ongoing (Table S5).
5.5.3. FGFR
The fibroblast growth factor receptor family includes FGFR1–4. Signalling through this pathway regulates cell survival, proliferation, and differentiation. Genes that encode for these receptors are amplified in ~10% of BC (24). Although FGFR1 is the most frequent genomic alteration in all subtypes of BC, amplification and overexpression of FGFR2 are more frequently observed among TNBCs (~4%). Basal BC with elevated MET and FGFR1 signatures is associated with poor relapse-free survival (193). The interplay between MET and FGFR regulates cancer stem cells in mesenchymal subtypes (194).
Trial data in this setting is limited to a few studies that do not select for TNBC but in which some response to this target has been seen. It seems likely that the correct biomarker has not yet been identified. Ongoing trials for this target in the neoadjuvant setting include a window of opportunity trial combining lenvatinib and pembrolizumab (NCT04427293).
5.5.4. EGFR
EGFR dysregulation is frequently reported in TNBC (195) and enrichment for this pathway signalling is predominantly observed in BL2 tumours (196). In contrast to EGFR mutations, EGFR amplification is a relatively frequent event (11% vs 23%, respectively) (24, 197) and is considered an independent prognostic factor for poor disease-free survival (198). Several attempts to target this pathway with tyrosine kinase inhibitors and mAbs in the context of mTNBC have been pursued without success. A limited number of trials have used these therapies in the early setting.
Trials are underway in the locallyCetuximab has been trialled along with neoadjuvant docetaxel in a pilot phase two study, including stage II–IIIA TNBC (199). The pCR rate was 24% [95% CI: 7.3–40.7] and the pre-therapy ratio between CD8+ and FOXP3+ TILs equal or higher than 2.75 was predictive of pCR (43% versus 0%). In addition, panitumumab and the EGFR/HER2 inhibitor lapatinib failed to demonstrate additional benefit in the advanced setting independent of EGFR activation (200, 201). The paucity of accurate biomarkers predictive of sensitive patients has led to unsatisfactory outcomes and limited clinical utility despite increasing evidence for EGFR as the driver of tumorigenesis in some TNBC.
Table S5 summarises ongoing trials that target these pathways in early TNBC.
5.6. Other Oncogenic Targets
Inter-chromosomal rearrangements causing NTRK gene fusions can result in constitutive activation of TRK proteins, which act as oncogenic drivers through activation of cellular growth pathways. Results from early phase trials that included advanced NTRK fusion-positive solid tumours support the use of larotrectinib and entrectinib in this subgroup (202, 203). NTRK gene fusions occur at low frequency (~0.3%) among all solid tumours (203). However, a high prevalence is observed in a subgroup of TNBC (16). The ETV6-NTRK3 gene fusion is frequently found in human secretory breast carcinoma (16), and although the vast majority of these breast tumours are treated with local treatments, targeting TRK signalling remains an option for cases of locally advanced disease.
Trop-2/TACSTD2 is a calcium signal transducer with extracellular, transmembrane, and intracellular domains, and is overexpressed in many epithelial cancers, including TNBC. It stimulates cancer cell growth and is implicated in various metabolic pathways. TROP-2 has also been found in stem cells of various tissues, particularly in basal cells (204). Sacituzumab govitecan, a humanised mAb that targets TROP2, has shown a PFS and OS benefit in mTNBC (205). Trials are upcoming in the neoadjuvant setting (NeoSTAR, NCT04230109), and recruiting in the adjuvant setting (GBG102-SASCIA NCT04595565 as monotherapy and ASPRIA NCT04434040 along with immunotherapy) for patients with residual invasive disease after NACT.
Dysregulation of the NOTCH pathway leads to aberrant self-renewal and transformation of mammary cancer stem cells, resulting in tumourigenesis (206). Inhibition of NOTCH signalling is an attractive strategy for treating TNBC given its role in promoting EMT and cancer stem cell maintenance (207). Preclinical and clinical studies involving γ-secretase inhibitors and mAbs against NOTCH receptors have explored its potential utility with encouraging results, but toxicity has been limiting (208). The subgroup of TNBCs achieving the best response to the targeting of this pathway remains undefined.
Activation of RAS/MAPK signalling is more frequent in TNBCs compared to other BC subtypes, and it is typically associated with shorter survival (209, 210). Although canonical aberrations in the RAS, RAF, MEK, or ERK genes are not found frequently in TNBC, amplification or mutations in these genes are described in approximately 6% of BC overall (24). Other mechanisms for RAS/MAK activation have also been described (211). MEK inhibitors have been trialled in unselected mTNBCs with modest results (212, 213). Trials are underway in the locally advanced setting that select for hyperactivation of ERK (NCT04494958) and RAS pathway mutations (NCT05111561).
Dysregulation of the JAK/STAT3, cyclinD–CDK4/6–INK4–Rb–E2F, TGF-β, and WNT/B-catenin pathways appears critical in TNBC development and progression. Clinical testing of the inhibition of these pathways in TNBC is still immature.
Table S5 summarises ongoing trials that target the above pathways in early TNBC.
Summary Box 8—Other pathways: Treatment strategies
–Targeted therapies should be guided by a biomarker to best determine efficacy in the TNBC population most likely to derive benefit.
–Dysregulation of the PI3K/AKT/mTOR pathway is often observed in TNBC. Efforts to target this pathway have inconsistently shown a modest benefit.
–Targeting AR has shown some clinical benefit, and several trials are ongoing to further evaluate this. A standardised method to determine AR pathway activation is lacking.
–Overall benefit of targeting the EGFR, VEGF, and FGFR pathways remains modest. The lack of predictive biomarkers that identify sensitive patients has limited the clinical utility of these drugs.
–Treatment directed towards HER2-low TNBC has provided new therapeutic opportunities in a proportion of patients, with encouraging results from trials to date.
–Sacituzumab govitecan, a humanised mAb that targets TROP2, has shown a PFS and OS benefit in mTNBC. It remains to be seen if this success can be translated into the early TNBC setting.
Discussion
Improved understanding of tumour genomics, transcriptomics, epigenetics, and their interaction with the tumour microenvironment has allowed a greater insight into the true diversity of TNBCs. Additionally, numerous advances in both preclinical and clinical research have directed the treatment of TNBC towards a more personalised approach. Despite the introduction of an increasing number of novel strategies in the clinical setting, approximately one-third of patients diagnosed with early stage disease will have limited response to primary treatment and face a poor long-term outcome. The underlying complexity of TNBC and the challenges in translating experimental science into the clinic could explain why current management approaches remain insufficient. The current therapeutic landscape for early TNBC is severely limited compared to the large number of compounds in development. Figure 1 shows the spectrum of agents with known or potential activity in TNBC. Only a small proportion of these reach patient care, and the pace at which these agents enter the early BC setting remains frustratingly slow. Immunotherapy and DDR agents lead the field with encouraging results.
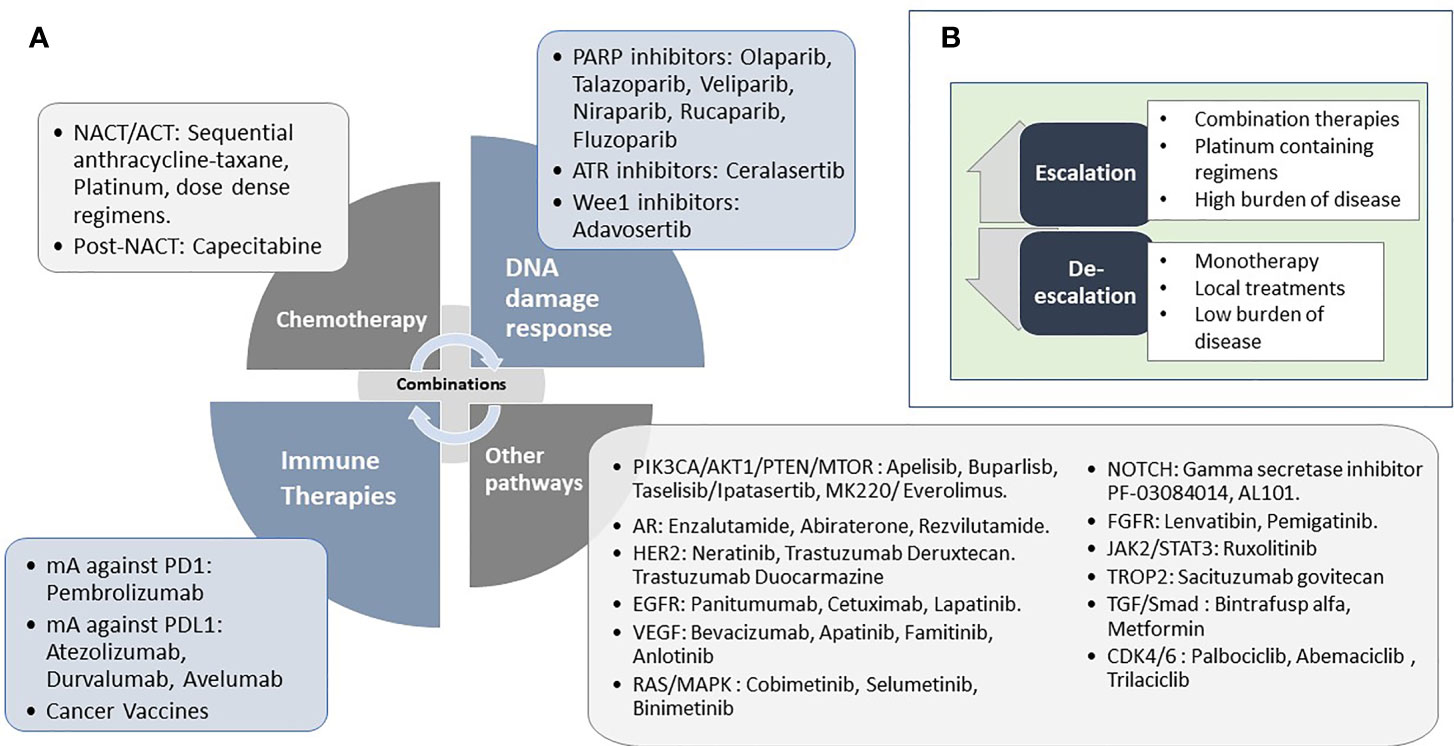
Figure 1 Current therapeutics strategies in early TNBC. (A). Treatment spectrum (B). Treatment modalities for escalation and de-escalation.
Predictive biomarkers are not routinely used in the clinical management of early sporadic TNBC. The use of gBRCA mutations to select patients who could benefit from platinum-based chemotherapy and PARPi demonstrates how a molecular alteration can aid patient selection for treatment. As yet, there is no definitive evidence to either support or refute the use of PARPi in the non-gBRCA TNBC population. An ongoing neoadjuvant study (NCT03150576) that includes both sporadic TNBC and BRCA-associated tumours will help elucidate the value of gBRCA mutations in predicting the response to the addition of PARPi to platinum-based chemotherapy (100). Furthermore, no biomarker was predicted for benefit from Pembrolizumab in the KEYNOTE-522 trial, despite the encouraging response rates shown. The expected role of PD-L1 as a biomarker of response has not been proven in the early setting (138, 139). Substantial differences between the clonal architecture and the microenvironment of primary and metastatic tumours (214, 215) suggest that the role of a given biomarker should be evaluated separately in both early and advanced settings.
A single biomarker strategy is unlikely to be successful for such a heterogeneous disease, considering the large number of treatment strategies already tested and the increasing evidence of molecular complexity in TBNC. Figure 2 illustrates the variety of molecular components currently being explored as potential biomarkers of response and resistance. Several interactions across components also contribute to the challenge. As an example, to adequately characterise the relationship between host immunity and tumour, a single determination of the extent of immune activation is expected to be insufficient. Understanding how the immune response modulates the intrinsic genomic architecture of the tumour and the spatial and cellular distribution of immune cells in response to treatment appears to be crucial. Similarly, multiple pathway signalling, a common finding in TNBC tumours, could result in the activation of compensatory feedback loops that explain some mechanisms of tumour evasion and resistance when a single pathway inhibition is applied (216). An integrative approach including tumour architecture, microenvironment, and pathway activation is more likely to succeed. A pragmatic example of how an immune-molecular profile directed approach could be implemented is shown in Figure 3. Tumours could be classed as ‘hot’ (high immune activation) or ‘cold’ (low immune activation) as well as ‘high-burden’ (high mutational/clonal burden) or ‘low-burden’ (low mutational/clonal burden). Hot-high burden tumours are frequently highly proliferative and more likely to exhibit high chromosomal instability. Increased response to cytotoxic and immunotherapy agents is anticipated in this subgroup. The hot-low burden group represents a subgroup in which clonal selection has been enforced by an active immune system. This good-prognosis subgroup is likely to require less intensive therapies with treatments focused on targeting key drivers. In sharp contrast, cold tumours require more comprehensive approaches that often include treatment escalation strategies. It is possible that due to quiescent mechanisms of tumourigenesis, cold tumours remain invisible to the immune system. Therefore, sequential strategies that aim to enhance the effect of the immune system are essential in this group. In cold-low burden tumours, targeted pathway inactivation followed by immune checkpoint inhibition could potentially result in an augmented immune response, achieving long-lasting control of the disease. Cold-high burden tumours constitute a poor prognosis group with patent mechanisms of immune evasion. Sequential strategies that include immunotherapy followed by either chemotherapy, pathway-specific targeted agents, or radiotherapy-targeted agent combinations are plausible options.
Response to NACT, measured as the amount of residual disease found at surgery, has recently been used as a primary endpoint to test novel agents in the early setting. RCB is widely considered a prognostic factor and is frequently used as a surrogate endpoint for long-term outcomes, particularly in this BC subgroup. Although it is clear from recent meta-analyses that RCB is a better endpoint than pCR, the identification of the molecular characteristics that explain why some tumours do not follow the predicted outcomes (recurrences after excellent responses or long-lasting EFS after residual disease) continues to be a challenge. Robust evidence supports the association between RCB score and long-term outcome in patients twho have received NACT (79). Conversely, evidence for the predictive value of RCB in the context of targeted therapy is limited and requires further investigation. Multiple other methodologies to aid the identification of patients with higher disease relapse risk are currently being explored. The post neoadjuvant and adjuvant settings are an excellent opportunity to evaluate the contribution of dynamic biomarkers (e.g., RCB, TILs) to enable an accurate selection of patients who may benefit from escalating treatment strategies. Pre- and post-treatment assessment of ctDNA and TME plus integration of traditional transcriptomic and genomic signatures or classifications are some of the more promising approaches. Alternatively, innovative adaptive trial designs that enable early response assessment and facilitate an early change in management could minimise overtreatment and appropriately de-escalate or escalate therapy when appropriate.
Several molecular predictors of response that incorporate various ‘omic’ data to aid clinical decisions have been developed. Limited clinical impact has been derived due to lack of reproducibility, lengthy timeline of results, and expense. The real-time delivery of genomic and transcriptomic results will facilitate the implementation of adaptive trial designs and permit the investigation of novel and existing biomarkers. There are multiple pan-cancer studies assessing the implementation of genomics and transcriptomics into clinical care, for example, the UK 100,000 Genomes Project (217), the Dutch national Centre for Personalised Cancer Treatment (CPCT) study (218), and the Personalised Onco-Genomics (POG) Programme (219). The Personalised Breast Cancer Programme (PBCP) (220) is a tumour-specific precision medicine project that implements whole-genome sequencing data into the real-time treatment of early and advanced breast cancer patients. This programme ensures the delivery of high-quality annotated genomic data to patients and clinicians while promoting hypothesis testing and tumour-specific analysis. These large-scale sequencing studies will add considerably to our understanding and enable better optimisation of trial design, response prediction, and biomarker discovery. These efforts, combined with the promising potential of novel agents and treatment combinations, provide us with the exciting prospect of a tailored treatment pathway for each patient diagnosed with early-stage TNBC.
The ultimate aim is that every patient diagnosed with early-stage TNBC has a bespoke treatment pathway developed that fits their TNBC. The individualised use of preclinical models such as patient-derived organoids or xenografts (221), and the implementation of advanced radiodiagnostic techniques (222) are pivotal to achieving this goal. This type of integrated approach requires open and clear communication and collaboration between basic scientists, clinicians, and other scientific disciplines, for example, bioinformatics, mathematics, and physics, which will maximise the chance of success and ultimately enhance patient benefit.
In conclusion, advances in tumour characterisation, real-time biomarker/genomic testing, trial design, and drug development provide the foundation for an era of precision treatment in early TNBC. The development of complex strategies that integrate multi-modal data to derive individualised care plans, should consider the holistic needs of each patient to achieve a truly personalised approach.
Author Contributions
KP, LD, RL, and JA each contributed to the design, literature review, writing, and editing of the manuscript. All authors listed have made a substantial, direct, and intellectual contribution to the work and approved it for publication.
Funding
We would like to thank and acknowledge our funders, the Cancer Research UK (CRUK) (CRUK Cambridge Centre Grant Funding: RDZD141, RDZD192); Addenbrookes Charitable Trust (98100) and the Mark Foundation for Cancer Research (RDZD/174). This research was also supported by the NIHR Cambridge Biomedical Research Centre (BRC-1215-20014). The views expressed are those of the authors and not necessarily those of the NIHR or the Department of Health and Social Care.
Acknowledgments
The authors want to thank the CRUK Precision Breast Cancer Institute, Cambridge and the Cambridge Cancer Trials Centre Womens’ Team for their support for our work in Triple Negative Breast Cancer. We also acknowledge the CRUK Cambridge Centre for its core support. We would like to thank the patients, families and carers who inspire us to work on TNBC.
Conflict of Interest
The authors declare that the research was conducted in the absence of any commercial or financial relationships that could be construed as a potential conflict of interest.
Publisher’s Note
All claims expressed in this article are solely those of the authors and do not necessarily represent those of their affiliated organizations, or those of the publisher, the editors and the reviewers. Any product that may be evaluated in this article, or claim that may be made by its manufacturer, is not guaranteed or endorsed by the publisher.
Supplementary Material
The Supplementary Material for this article can be found online at: https://www.frontiersin.org/articles/10.3389/fonc.2022.866889/full#supplementary-material
Abbreviations
AR, Androgen receptor; BC, Breast cancer; BL1, Basal-like 1; BL2, Basal-like 2; BLIA, Basal-like immune activated; BLIS, Basal-like immunosuppressed; ctDNA, Circulating tumour DNA; DDR, DNA damage response; DFS, Disease free survival; DSBs, Double strand breaks; EBCTCG, Early Breast Cancer Trailist’s Collaborative Group; EFS, Event free survival; EMT, Epithelial-mesenchymal transition; ER, Oestrogen receptor; gBRCA, Germline BRCA; GE, Gene expression; HR, Homologous recombination; HRD, Homologous recombination deficiency; ICI, Immune checkpoint inhibitors; iDFS, Invasive disease free survival; IntClust, Integrative Cluster; iTILs, Intratumoural TILs; LAR, Luminal androgen receptor; M, Mesenchymal -Lehmann subtype; mAbs, Monoclonal antibodies; mTNBC, Metastatic triple negative breast cancer; NACT, Neoadjuvant chemotherapy; OR, Overall response; OS, Overall survival; PARP, Poly ADP-ribose polymerase; PARPi, Poly ADP-ribose polymerase inhibitors; pCR, Pathological complete response; PD-1, Programmed cell death 1; PD-L1, Programmed cell death ligand-1; PFS, Progression free survival; PR, Progesterone receptor; RCB, Residual Cancer Burden; RFS, Relapse-free survival; ssDNA, Single strand DNA; sTILs, Stromal TILs; TILs, Tumour infiltrating lymphocytes; TMB, Tumour mutational burden; TNBC, Triple negative breast cancer.
Glossary
References
1. Wild CP, Stewart WE eds. World Cancer Report: Cancer Research for Cancer Prevention. Lyon, France: International Agency for Research on Cancer (2020).
2. Penault-Llorca F, Viale G. Pathological and Molecular Diagnosis of Triple-Negative Breast Cancer: A Clinical Perspective. Ann Oncol (2012) 23:VI19–VI22. doi: 10.1093/annonc/mds190
3. Bauer KR, Brown M, Cress RD, Parise CA, Caggiano V. Descriptive Analysis of Oestrogen Receptor (ER)-Negative, Progesterone Receptor (PR)-Negative, and HER2-Negative Invasive Breast Cancer, the So-Called Triple-Negative Phenotype. Cancer (2007) 109:1721–28. doi: 10.1002/cncr.22618
4. Steward L, Conant L, Gao F, Margenthaler JA. Predictive Factors and Patterns of Recurrence in Patients With Triple Negative Breast Cancer. Annals of Surgical. Oncology (2014) 21:2165–71. doi: 10.1245/s10434-014-3546-4
5. Dent R, Trudeau M, Pritchard KI, Hanna WM, Kahn HK, Sawka CA, et al. Triple-Negative Breast Cancer: Clinical Features and Patterns of Recurrence. Clin Cancer Res (2007) 13:4429–34. doi: 10.1158/1078-0432.CCR-06-3045
6. Pereira B, Chin S-F, Rueda OM, Vollan H-KM, Provenzano E, Bardwell HA, et al. The Somatic Mutation Profiles of 2,433 Breast Cancers Refine Their Genomic and Transcriptomic Landscapes. Nat Commun (2016) 7:11479. doi: 10.1038/ncomms11479
7. Shah SP, Roth A, Goya R, Oloumi A, Ha G, Zhao Y, et al. The Clonal and Mutational Evolution Spectrum of Primary Triple-Negative Breast Cancers. Nature (2012) 486:395–9. doi: 10.1038/nature10933
8. Bianchini G, Balko JM, Mayer IA, Sanders ME, Gianni L. Triple-Negative Breast Cancer: Challenges and Opportunities of a Heterogeneous Disease. Nat Rev Clin Oncol (2016) 13:674–90. doi: 10.1038/nrclinonc.2016.66
9. Balkenhol MCA, Vreuls W, Wauters CAP, Mol SJJ, van der Laak JAWM, Bult P. Histological Subtypes in Triple Negative Breast Cancer are Associated With Specific Information on Survival. Ann Diagn Pathol (2020) 46:151490. doi: 10.1016/J.AN§NDIAGPATH.2020.151490
10. Pareja F, Geyer FC, Marchiò C, Burke KA, Weigelt B, Reis-Filho JS. Triple-Negative Breast Cancer: The Importance of Molecular and Histologic Subtyping, and Recognition of Low-Grade Variants. NPJ Breast Cancer (2016) 2:1–11. doi: 10.1038/npjbcancer.2016.36
11. Bergeron A, MacGrogan G, Bertaut A, Ladoire S, Arveux P, Desmoulins I, et al. Triple-Negative Breast Lobular Carcinoma: A Luminal Androgen Receptor Carcinoma With Specific ESRRA Mutations. Modern Pathol (2021) 34:1282–96. doi: 10.1038/s41379-021-00742-9
12. Ng CKY, Piscuoglio S, Geyer FC, Burke KA, Pareja F, Eberle CA, et al. The Landscape of Somatic Genetic Alterations in Metaplastic Breast Carcinomas. Clin Cancer Res (2017) 23:3859–70. doi: 10.1158/1078-0432.CCR-16-2857
13. Bertucci F, Finetti P, Cervera N, Charafe-Jauffret E, Mamessier E, Adélaïde J, et al. Gene Expression Profiling Shows Medullary Breast Cancer is a Subgroup of Basal Breast Cancers. Cancer Res (2006) 66:4636–44. doi: 10.1158/0008-5472.CAN-06-0031
14. Sun X, Zuo K, Yao Q, Zhou S, Shui R, Xu X, et al. Invasive Apocrine Carcinoma of the Breast: Clinicopathologic Features and Comprehensive Genomic Profiling of 18 Pure Triple-Negative Apocrine Carcinomas. Modern Pathol (2020) 33:2473–82. doi: 10.1038/s41379-020-0589-x
15. Martelotto LG, De Filippo MR, Ng CK, Natrajan R, Fuhrmann L, Cyrta J, et al. Genomic Landscape of Adenoid Cystic Carcinoma of the Breast. J Pathol (2015) 237:179–89. doi: 10.1002/path.4573
16. Tognon C, Knezevich SR, Huntsman D, Roskelley CD, Melnyk N, Mathers JA, et al. Expression of the ETV6-NTRK3 Gene Fusion as a Primary Event in Human Secretory Breast Carcinoma. Cancer Cell (2002) 2:367–76. doi: 10.1016/S1535-6108(02)00180-0
17. Perou CM, Sørlie T, Eisen MB, van de Rijn M, Jeffrey SS, Rees CA, et al. Molecular Portraits of Human Breast Tumours. Nature (2000) 406:747–52. doi: 10.1038/35021093
18. Lehmann BD, Pietenpol JA. Identification and Use of Biomarkers in Treatment Strategies for Triple-Negative Breast Cancer Subtypes. J Pathol (2014) 232:142–50. doi: 10.1002/path.4280
19. Burstein MD, Tsimelzon A, Poage GM, Covington KR, Contreras A, Fuqua SAW, et al. Comprehensive Genomic Analysis Identifies Novel Subtypes and Targets of Triple-Negative Breast Cancer. Clin Cancer Res (2015) 21:1688–98. doi: 10.1158/1078-0432.CCR-14-0432
20. Liu Y-R, Jiang Y-Z, Xu X-E, Yu K-D, Jin X, Hu X, et al. Comprehensive Transcriptome Analysis Identifies Novel Molecular Subtypes and Subtype-Specific RNAs of Triple-Negative Breast Cancer. Breast Cancer Res (2016) 18. doi: 10.1186/s13058-016-0690-8
21. Rueda OM, Sammut S-J, Seoane JA, Chin S-F, Caswell-Jin JL, Callari M, et al. Dynamics of Breast-Cancer Relapse Reveal Late-Recurring ER-Positive Genomic Subgroups. Nature (2019) 567:399–404. doi: 10.1038/s41586-019-1007-8
22. Curtis C, Shah SP, Chin S-FF, Turashvili G, Rueda OM, Dunning MJ, et al. The Genomic and Transcriptomic Architecture of 2,000 Breast Tumours Reveals Novel Subgroups. Nature (2012) 486:346–52. doi: 10.1038/nature10983
23. Prado-Vázquez G, Gámez-Pozo A, Trilla-Fuertes L, Arevalillo JM, Zapater-Moros A, Ferrer-Gómez M, et al. A Novel Approach to Triple-Negative Breast Cancer Molecular Classification Reveals a Luminal Immune-Positive Subgroup With Good Prognoses. Sci Rep (2019) 9:1538. doi: 10.1038/s41598-018-38364-y
24. Cancer Genome Atlas Network. Comprehensive Molecular Portraits of Human Breast Tumours. Nature (2012) 490:61–70. doi: 10.1038/nature11412
25. Prat A, Parker JS, Karginova O, Fan C, Livasy C, Herschkowitz JI, et al. Phenotypic and Molecular Characterization of the Claudin-Low Intrinsic Subtype of Breast Cancer. Breast Cancer Res (2010) 12:R68. doi: 10.1186/bcr2635
26. Pommier RM, Sanlaville A, Tonon L, Kielbassa J, Thomas E, Ferrari A, et al. Comprehensive Characterization of Claudin-Low Breast Tumours Reflects the Impact of the Cell-of-Origin on Cancer Evolution. Nat Commun (2020) 11:3431. doi: 10.1038/s41467-020-17249-7
27. Metzger Filho O, Stover DG, Asad S, Ansell PJ, Watson M, Loibl S, et al. Immunophenotype and Proliferation to Predict for Response to Neoadjuvant Chemotherapy in TNBC: Results From BrighTNess Phase III Study. J Clin Oncol (2019) 37:510–0. doi: 10.1200/JCO.2019.37.15_suppl.510
28. Sikov WM, Barry WT, Hoadley KA, Pitcher BN, Singh B, Tolaney SM, et al. Abstract S4-05: Impact of Intrinsic Subtype by PAM50 and Other Gene Signatures on Pathologic Complete Response (pCR) Rates in Triple-Negative Breast Cancer (TNBC) After Neoadjuvant Chemotherapy (NACT) +/- Carboplatin (Cb) or Bevacizumab (Bev): CALGB 40603. In General Session Abstracts (American Association for Cancer Research), S4-05-S4-05. Cancer Res (2015) 75(9_Supplement). doi: 10.1158/1538-7445.SABCS14-S4-05
29. Lehmann BD, Jovanović B, Chen X, Estrada M, Johnson KN, Shyr Y, et al. Refinement of Triple-Negative Breast Cancer Molecular Subtypes: Implications for Neoadjuvant Chemotherapy Selection. PLoS One (2016) 11:e0157368. doi: 10.1371/journal.pone.0157368
30. Masuda H, Baggerly KA, Wang Y, Zhang Y, Gonzalez-Angulo AM, Meric-Bernstam F, et al. Differential Response to Neoadjuvant Chemotherapy Among 7 Triple-Negative Breast Cancer Molecular Subtypes. Clin Cancer Res (2013) 19:5533–40. doi: 10.1158/1078-0432.CCR-13-0799
31. Santonja A, Sánchez-Muñoz A, Lluch A, Chica-Parrado MR, Albanell J, Chacón JI, et al. Triple Negative Breast Cancer Subtypes and Pathologic Complete Response Rate to Neoadjuvant Chemotherapy. Oncotarget (2018) 9:26406–16. doi: 10.18632/oncotarget.25413
32. Jiang Y-Z, Ma D, Suo C, Shi J, Xue M, Hu X, et al. Genomic and Transcriptomic Landscape of Triple-Negative Breast Cancers: Subtypes and Treatment Strategies. Cancer Cell (2019) 35:428–40.e5. doi: 10.1016/j.ccell.2019.02.001
33. Telli ML, Timms KM, Reid J, Hennessy B, Mills GB, Jensen KC, et al. Homologous Recombination Deficiency (HRD) Score Predicts Response to Platinum-Containing Neoadjuvant Chemotherapy in Patients With Triple-Negative Breast Cancer. Clin Cancer Res (2016) 22:3764–73. doi: 10.1158/1078-0432.CCR-15-2477
34. Schmid P, Cortes J, Dent R, Pusztai L, McArthur H, Kümmel S, et al. Event-Free Survival With Pembrolizumab in Early Triple-Negative Breast Cancer. New Engl J Med (2022) 386:556–67. doi: 10.1056/NEJMOA2112651/SUPPL_FILE/NEJMOA2112651_DATA-SHARING.PDF
35. Tutt ANJ, Garber JE, Kaufman B, Viale G, Fumagalli D, Rastogi P, et al. Adjuvant Olaparib for Patients with BRCA1 - or BRCA2 -Mutated Breast Cancer. New Engl J Med (2021) 384(25):2394–405. doi: 10.1056/nejmoa2105215
36. Early Breast Cancer Trialists’ Collaborative Group (EBCTCG). Comparisons Between Different Polychemotherapy Regimens for Early Breast Cancer: Meta-Analyses of Long-Term Outcome Among 100 000 Women in 123 Randomised Trials. Lancet (2012) 379(9814). doi: 10.1016/S0140-6736(11)61625-5
37. Blum JL, Flynn PJ, Yothers G, Asmar L, Geyer CE, Jacobs SA, et al. Anthracyclines in Early Breast Cancer: The ABC Trials—USOR 06-090, NSABP B-46-I/USOR 07132, and NSABP B-49 (NRG Oncology). J Clin Oncol (2017) 35:2647–55. doi: 10.1200/JCO.2016.71.4147
38. Braybrooke J, Bradley R, Gray R, Hills R, Liu Z, Pan H, et al. Abstract GS2-06. Taxane With Anthracycline Versus Taxane Without Anthracycline: An Individual Patient-Level Meta-Analysis of 16,500 Women With Early-Stage Breast Cancer in 13 Randomised Trials. Cancer Res (2021) 82(4_Supplement). doi: 10.1158/1538-7445.SABCS21-GS2-06
39. John P, Osani MC, Kodali A, Buchsbaum R, Bannuru RR, Erban JK. Comparative Effectiveness of Adjuvant Chemotherapy in Early-Stage Breast Cancer: A Network Meta-Analysis. Clin Breast Cancer (2021) 21(1):e22–37. doi: 10.1016/j.clbc.2020.07.005
40. Hurvitz SA, McAndrew NP, Bardia A, Press MF, Pegram M, Crown JP, et al. A careful reassessment of anthracycline use in curable breast cancer. NPJ Breast Cancer (2021) 7:1. doi: 10.1038/s41523-021-00342-5
41. Yu K-D, Liu X-Y, Chen L, Mo M, Wu J, Liu G-Y, et al. Anthracycline-Free or Short-Term Regimen as Adjuvant Chemotherapy for Operable Breast Cancer: A Phase III Randomised non-Inferiority Trial. Lancet Regional Health - Western Pac (2021) 11:100158. doi: 10.1016/j.lanwpc.2021.100158
42. Ejlertsen B, Tuxen MK, Jakobsen EH, Jensen M-B, Knoop AS, Højris I, et al. Adjuvant Cyclophosphamide and Docetaxel With or Without Epirubicin for Early TOP2A -Normal Breast Cancer: DBCG 07-READ, an Open-Label, Phase III, Randomised Trial. J Clin Oncol (2017) 35:2639–46. doi: 10.1200/JCO.2017.72.3494
43. Mavroudis D, Matikas A, Malamos N, Papakotoulas P, Kakolyris S, Boukovinas I, et al. Dose-Dense FEC Followed by Docetaxel Versus Docetaxel Plus Cyclophosphamide as Adjuvant Chemotherapy in Women With HER2-Negative, Axillary Lymph Node-Positive Early Breast Cancer: A Multicenter Randomised Study by the Hellenic Oncology Research Group (HORG). Ann Oncol (2016) 27:1873–8. doi: 10.1093/annonc/mdw274
44. Janni W, Nitz U, Rack BK, Gluz O, Schneeweiss A, Kates RE, et al. Pooled Analysis of Two Randomised Phase III Trials (PlanB/SuccessC) Comparing Six Cycles of Docetaxel and Cyclophosphamide to Sequential Anthracycline Taxane Chemotherapy in Patients With Intermediate and High Risk HER2-Negative Early Breast Cancer (N=5,923). J Clin Oncol (2018) 36:522–2. doi: 10.1200/JCO.2018.36.15_suppl.522
45. Gianni L, Baselga J, Eiermann W, Guillem Porta V, Semiglazov V, Zambetti L, et al. European Cooperative Trial in Operable Breast Cancer (ECTO): Improved Freedom From Progression (FFP) From Adding Paclitaxel (T) to Doxorubicin (A) Followed by Cyclophosphamide Methotrexate and Fluorouracil (CMF). J Clin Oncol ASCO (2005) 16):513. doi: 10.1200/jco.2005.23.16_suppl.513
46. Yin L, Duan JJ, Bian XW, Yu SC. Triple-Negative Breast Cancer Molecular Subtyping and Treatment Progress. Breast Cancer Res (2020) 22(1):61. doi: 10.1186/s13058-020-01296-5
47. Untch M, Jackisch C, Schneeweiss A, Conrad B, Aktas B, Denkert C, et al. Nab-Paclitaxel Versus Solvent-Based Paclitaxel in Neoadjuvant Chemotherapy for Early Breast Cancer (GeparSepto—GBG 69): A Randomised, Phase 3 Trial. Lancet Oncol (2016) 17:345–56. doi: 10.1016/S1470-2045(15)00542-2
48. Gianni L, Mansutti M, Anton A, Calvo L, Bisagni G, Bermejo B, et al. Comparing Neoadjuvant Nab-Paclitaxel vs Paclitaxel Both Followed by Anthracycline Regimens in Women With ERBB2/HER2 -Negative Breast Cancer—The Evaluating Treatment With Neoadjuvant Abraxane (ETNA) Trial. JAMA Oncol (2018) 4:302. doi: 10.1001/jamaoncol.2017.4612
49. Yardley DA, Arrowsmith ER, Daniel BR, Eakle J, Brufsky A, Drosick DR, et al. TITAN: Phase III Study of Doxorubicin/Cyclophosphamide Followed by Ixabepilone or Paclitaxel in Early-Stage Triple-Negative Breast Cancer. Breast Cancer Res Treat (2017) 164:649–58. doi: 10.1007/s10549-017-4285-6
50. Poggio F, Bruzzone M, Ceppi M, Pondé NF, La Valle G, Del Mastro L, et al. Platinum-Based Neoadjuvant Chemotherapy in Triple-Negative Breast Cancer: A Systematic Review and Meta-Analysis. Ann Oncol (2018) 7:1497–1508. doi: 10.1093/annonc/mdy127
51. Masuda N, Lee S-J, Ohtani S, Im Y-H, Lee E-S, Yokota I, et al. Adjuvant Capecitabine for Breast Cancer After Preoperative Chemotherapy. New Engl J Med (2017) 376(22):2147–59. doi: 10.1056/NEJMoa1612645
52. Lluch A, Barrios CH, Torrecillas L, Ruiz-Borrego M, Bines J, Segalla J, et al. Phase III Trial of Adjuvant Capecitabine After Standard Neo-/Adjuvant Chemotherapy in Patients With Early Triple-Negative Breast Cancer (GEICAM/2003-11_CIBOMA/2004-01). J Clin Oncol (2020) 38(3):203–13. doi: 10.1200/JCO.19.00904
53. Li J, Yu K, Pang D, Wang C, Jiang J, Yang S, et al. Adjuvant Capecitabine With Docetaxel and Cyclophosphamide Plus Epirubicin for Triple-Negative Breast Cancer (CBCSG010): An Open-Label, Randomized, Multicenter, Phase III Trial. J Clin Oncol (2020) 38(16):1774–84. doi: 10.1200/JCO.19.02474
54. Joensuu H, Kellokumpu-Lehtinen P, Huovinen R, Jukkola-Vuorinen A, Tanner M, Kokko R, et al. Adjuvant Capecitabine in Combination With Docetaxel, Epirubicin, and Cyclophosphamide for Early Breast Cancer: The Randomized Clinical FinXX Trial. JAMA Oncol (2017) 3(6):793–800. doi: 10.1001/jamaoncol.2016.6120
55. Wang X, Wang S-S, Huang H, Cai L, Zhao L, Peng R-J, et al. Effect of Capecitabine Maintenance Therapy Using Lower Dosage and Higher Frequency vs Observation on Disease-Free Survival Among Patients With Early-Stage Triple-Negative Breast Cancer Who Had Received Standard Treatment. JAMA (2021) 325(1):50. doi: 10.1001/jama.2020.23370
56. Ye F, Bian L, Wen J, Yu P, Li N, Xie X, et al. Additional Capecitabine Use in Early-Stage Triple Negative Breast Cancer Patients Receiving Standard Chemotherapy: A New Era? A meta-analysis of randomized controlled trials. BMC Cancer (2022) 22:261. doi: 10.1186/s12885-022-09326-5
57. Early Breast Cancer Trialists’ Collaborative Group (2015). Adjuvant Bisphosphonate Treatment in Early Breast Cancer: Meta-Analyses of Individual Patient Data From Randomised Trials. Lancet (2015) 386:1353–61. doi: 10.1016/S0140-6736(15)60908-4
58. Coleman RE, Collinson M, Gregory W, Marshall H, Bell R, Dodwell D, et al. Benefits and Risks of Adjuvant Treatment With Zoledronic Acid in Stage II/III Breast Cancer. 10 years follow-up of the AZURE randomised clinical trial (BIG 01/04). J Bone Oncol (2018) 13:123–35. doi: 10.1016/j.jbo.2018.09.008
59. von Minckwitz G, Rezai M, Tesch H, Huober J, Gerber B, Zahm DM, et al. Zoledronate for Patients With Invasive Residual Disease After Anthracyclines-Taxane-Based Chemotherapy for Early Breast Cancer – The Phase III NeoAdjuvant Trial Add-oN (NaTaN) Study (GBG 36/ABCSG 29). Eur J Cancer (2016) 64:12–21. doi: 10.1016/J.EJCA.2016.05.015
60. Coleman RE, Winter MC, Cameron D, Bell R, Dodwell D, Keane MM, et al. The Effects of Adding Zoledronic Acid to Neoadjuvant Chemotherapy on Tumour Response: Exploratory Evidence for Direct Anti-Tumour Activity in Breast Cancer. Br J Cancer (2010) 102:1099–105. doi: 10.1038/sj.bjc.6605604
61. Coleman R, Finkelstein DM, Barrios C, Martin M, Iwata H, Hegg R, et al. Adjuvant Denosumab in Early Breast Cancer (D-CARE): An International, Multicentre, Randomised, Controlled, Phase 3 Trial. Lancet Oncol (2020) 21:60–72. doi: 10.1016/S1470-2045(19)30687-4
62. van der Hage JA, van de Velde CJH, Julien J-P, Tubiana-Hulin M, Vandervelden C, Duchateau L. Preoperative Chemotherapy in Primary Operable Breast Cancer: Results From the European Organization for Research and Treatment of Cancer Trial 10902. J Clin Oncol (2001) 19:4224–37. doi: 10.1200/JCO.2001.19.22.4224
63. Wolmark N, Wang J, Mamounas E, Bryant J, Fisher B. Preoperative Chemotherapy in Patients With Operable Breast Cancer: Nine-Year Results From National Surgical Adjuvant Breast and Bowel Project B-18. JNCI Monogr (2001) 2001(30):96–102. doi: 10.1093/oxfordjournals.jncimonographs.a003469
64. Mauriac L, MacGrogan G, Avril A, Durand M, Floquet A, Debled M, et al. Neoadjuvant Chemotherapy for Operable Breast Carcinoma Larger Than 3 Cm: A Unicentre Randomised Trial With a 124-Month Median Follow-Up. Ann Oncol (1999) 10:47–52. doi: 10.1023/A:1008337009350
65. Early Breast Cancer Trialists’ Collaborative Group (EBCTCG). Long-Term Outcomes for Neoadjuvant Versus Adjuvant Chemotherapy in Early Breast Cancer: Meta-Analysis of Individual Patient Data From Ten Randomised Trials. Lancet Oncol (2018) 19:27–39. doi: 10.1016/S1470-2045(17)30777-5
66. Xia L-Y, Hu Q-L, Zhang J, Xu W-Y, Li X-S. Survival Outcomes of Neoadjuvant Versus Adjuvant Chemotherapy in Triple-Negative Breast Cancer: A Meta-Analysis of 36,480 Cases. World J Surg Oncol (2020) 18:129. doi: 10.1186/s12957-020-01907-7
67. Karagiannis GS, Pastoriza JM, Wang Y, Harney AS, Entenberg D, Pignatelli J, et al. Neoadjuvant Chemotherapy Induces Breast Cancer Metastasis Through a TMEM-Mediated Mechanism. Sci Transl Med (2017) 9(397):eaan0026. doi: 10.1126/scitranslmed.aan0026
68. Symmans WF, Wei C, Gould R, Yu X, Zhang Y, Liu M, et al. Long-Term Prognostic Risk After Neoadjuvant Chemotherapy Associated With Residual Cancer Burden and Breast Cancer Subtype. J Clin Oncol (2017) 35:1049–60. doi: 10.1200/JCO.2015.63.1010
69. Thompson AM, Moulder-Thompson SL. Neoadjuvant Treatment of Breast Cancer. Ann Oncol (2012) 23:X231–36. doi: 10.1093/annonc/mds324
70. Shao N, Wang S, Yao C, Xu X, Zhang Y, Zhang Y, et al. Sequential Versus Concurrent Anthracyclines and Taxanes as Adjuvant Chemotherapy of Early Breast Cancer: A Meta-Analysis of Phase III Randomised Control Trials. Breast (2012) 21:389–93. doi: 10.1016/j.breast.2012.03.011
71. Earl HM, Vallier A-L, Hiller L, Fenwick N, Young J, Iddawela M, et al. Effects of the Addition of Gemcitabine, and Paclitaxel-First Sequencing, in Neoadjuvant Sequential Epirubicin, Cyclophosphamide, and Paclitaxel for Women With High-Risk Early Breast Cancer (Neo-Tango): An Open-Label, 2×2 Factorial Randomised Phase 3 Trial. Lancet Oncol (2014) 15:201–12. doi: 10.1016/S1470-2045(13)70554-0
72. Amir E, Ocana A, Freedman O, Clemons M, Seruga B. Dose-Dense Treatment for Triple-Negative Breast Cancer. Nat Rev Clin Oncol (2010) 7:190–91. doi: 10.1038/nrclinonc.2009.23
73. Bonilla L, Ben-Aharon I, Vidal L, Gafter-Gvili A, Leibovici L, Stemmer SM. Dose-Dense Chemotherapy in Nonmetastatic Breast Cancer: A Systematic Review and Meta-Analysis of Randomised Controlled Trials. JNCI J Natl Cancer Inst (2010) 102:1845–54. doi: 10.1093/jnci/djq409
74. Ding Y, Ding K, Yang H, He X, Mo W, Ding X. Does Dose-Dense Neoadjuvant Chemotherapy Have Clinically Significant Prognostic Value in Breast Cancer?: A Meta-Analysis of 3,724 Patients. PLoS One (2020) 15:e0234058. doi: 10.1371/journal.pone.0234058
75. Gray R, Bradley R, Braybrooke J, Liu Z, Peto R, Davies L, et al. Increasing the Dose Intensity of Chemotherapy by More Frequent Administration or Sequential Scheduling: A Patient-Level Meta-Analysis of 37 298 Women With Early Breast Cancer in 26 Randomised Trials. Lancet (2019) 393:1440–52. doi: 10.1016/S0140-6736(18)33137-4/ATTACHMENT/4A5A797F-D8DD-4E81-B17F-E8F9CE7116FF/MMC1.PDF
76. Hanahan D, Bergers G, Bergsland E. Less is More, Regularly: Metronomic Dosing of Cytotoxic Drugs can Target Tumour Angiogenesis in Mice. J Clin Invest (2000) 105(8):1045. doi: 10.1172/JCI9872
77. Colleoni M, Gray KP, Gelber S, Láng I, Thürlimann B, Gianni L, et al. Low-Dose Oral Cyclophosphamide and Methotrexate Maintenance for Hormone Receptor–Negative Early Breast Cancer: International Breast Cancer Study Group Trial 22-00. J Clin Oncol (2016) 34:3400–8. doi: 10.1200/JCO.2015.65.6595
78. Huang M, O’Shaughnessy J, Zhao J, Haiderali A, Cortés J, Ramsey SD, et al. Association of Pathologic Complete Response With Long-Term Survival Outcomes in Triple-Negative Breast Cancer: A Meta-Analysis. Cancer Res (2020) 80:5427–34. doi: 10.1158/0008-5472.CAN-20-1792
79. Yau C, Osdoit M, Noordaa ,Mvd, Shad S, Wei J, de Croze D, et al. Residual Cancer Burden After Neoadjuvant Chemotherapy and Long-Term Survival Outcomes in Breast Cancer: A Multicentre Pooled Analysis of 5161 Patients. Lancet Oncol (2022) 23(1):149–60. doi: 10.1016/S1470-2045(21)00589-1
80. Carey LA, Dees EC, Sawyer L, Gatti L, Moore DT, Collichio F, et al. The Triple Negative Paradox: Primary Tumour Chemosensitivity of Breast Cancer Subtypes. Clin Cancer Res (2007) 13:2329–34. doi: 10.1158/1078-0432.CCR-06-1109
81. Cavallone L, Aguilar-Mahecha A, Lafleur J, Brousse S, Aldamry M, Roseshter T, et al. Prognostic and Predictive Value of Circulating Tumour DNA During Neoadjuvant Chemotherapy for Triple Negative Breast Cancer. Sci Rep (2020) 10:14704. doi: 10.1038/s41598-020-71236-y
82. Magbanua MJM, Swigart LB, Wu HT, Hirst GL, Yau C, Wolf DM, et al. Circulating Tumor DNA in Neoadjuvant-Treated Breast Cancer Reflects Response and Survival. Ann Oncol (2021) 32:229–39. doi: 10.1016/J.ANNONC.2020.11.007/ATTACHMENT/E73EC62B-DC11-49A9-942F-DBA96CD2C123/MMC1.DOCX
83. Huo X, Li J, Zhao F, Ren D, Ahmad R, Yuan X, et al. The Role of Capecitabine-Based Neoadjuvant and Adjuvant Chemotherapy in Early-Stage Triple-Negative Breast Cancer: A Systematic Review and Meta-Analysis. BMC Cancer (2021) 21(1):78. doi: 10.1186/s12885-021-07791-y
84. Burstein HJ, Curigliano G, Thürlimann B, Weber WP, Poortmans P, Regan MM, et al. Customizing Local and Systemic Therapies for Women With Early Breast Cancer: The St. Gallen International Consensus Guidelines for Treatment of Early Breast Cancer 2021. Ann Oncol (2021) 32(10):P1216–35. doi: 10.1016/j.annonc.2021.06.023
85. Beyerlin K, Jimenez R, Zangardi M, Fell GG, Edmonds C, Johnson A, et al. The Adjuvant Use of Capecitabine for Residual Disease Following Pre-Operative Chemotherapy for Breast Cancer: Challenges Applying CREATE-X to a US Population. J Oncol Pharm Pract (2020) 27(8):1883–90. doi: 10.1177/1078155220971751
86. Schneider BP, Jiang G, Ballinger TJ, Shen F, Chitambar C, Nanda R, et al. BRE12-158: A Postneoadjuvant, Randomized Phase II Trial of Personalized Therapy Versus Treatment of Physician’s Choice for Patients With Residual Triple-Negative Breast Cancer. J Clin Oncol (2022) 40:345–55. doi: 10.1200/JCO.21.01657
87. Birkbak NJ, Eklund AC, Li Q, McClelland SE, Endesfelder D, Tan P, et al. Paradoxical Relationship Between Chromosomal Instability and Survival Outcome in Cancer. Cancer Res (2011) 71(10):3447–52. doi: 10.1158/0008-5472.CAN-10-3667
88. den Brok WD, Schrader KA, Sun S, Tinker A, Zhao EY, Aparicio S, et al. Homologous Recombination Deficiency in Breast Cancer: A Clinical Review. JCO Precis Oncol (2017) 1:1–13. doi: 10.1200/PO.16.00031
89. Kaelin WG. The Concept of Synthetic Lethality in the Context of Anticancer Therapy. Nat Rev Cancer (2005) 5:689–98. doi: 10.1038/nrc1691
90. Evans DG, Howell A, Ward D, Lalloo F, Jones JL, Eccles DM. Prevalence of BRCA1 and BRCA2 Mutations in Triple Negative Breast Cancer. J Med Genet (2011) 48(8):520–22. doi: 10.1136/jmedgenet-2011-100006
91. Davies H, Glodzik D, Morganella S, Yates LR, Staaf J, Zou X, et al. HRDetect is a Predictor of BRCA1 and BRCA2 Deficiency Based on Mutational Signatures. Nat Med (2017) 23(4):517–25. doi: 10.1038/nm.4292
92. Sikov WM, Berry DA, Perou CM, Singh B, Cirrincione CT, Tolaney SM, et al. Impact of the Addition of Carboplatin and/or Bevacizumab to Neoadjuvant Once-Per-Week Paclitaxel Followed by Dose-Dense Doxorubicin and Cyclophosphamide on Pathologic Complete Response Rates in Stage II to III Triple-Negative Breast Cancer: CALGB 40603 (Alliance). J Clin Oncol (2015) 33(1):13–21. doi: 10.1200/JCO.2014.57.0572
93. Loibl S, O’Shaughnessy J, Untch M, Sikov WM, Rugo HS, McKee MD, et al. Addition of the PARP Inhibitor Veliparib Plus Carboplatin or Carboplatin Alone to Standard Neoadjuvant Chemotherapy in Triple-Negative Breast Cancer (BrighTNess): A Randomised, Phase 3 Trial. Lancet Oncol (2018) 19(4):497–509. doi: 10.1016/S1470-2045(18)30111-6
94. von Minckwitz G, Schneeweiss A, Loibl S, Salat C, Denkert C, Rezai M, et al. Neoadjuvant Carboplatin in Patients With Triple-Negative and HER2-Positive Early Breast Cancer (GeparSixto; GBG 66): A Randomised Phase 2 Trial. Lancet Oncol (2014) 15(7):747–56. doi: 10.1016/S1470-2045(14)70160-3
95. Byrski T, Huzarski AT, Dent AR, Gronwald AJ, Zuziak AD, Cybulski AC, et al. Response to Neoadjuvant Therapy With Cisplatin in BRCA1-Positive Breast Cancer Patients. Breast Cancer Res Treat (2009) 115:359–63. doi: 10.1007/s10549-008-0128-9
96. Tung N, Arun B, Hacker MR, Hofstatter E, Toppmeyer DL, Isakoff SJ, et al. TBCRC 031: Randomised Phase II Study of Neoadjuvant Cisplatin Versus Doxorubicin-Cyclophosphamide in Germline BRCA Carriers With HER2-Negative Breast Cancer (the INFORM Trial). J Clin Oncol (2020) 38(14):1539–48. doi: 10.1200/JCO.19.03292
97. Mayer IA, Zhao F, Arteaga CL, Symmans WF, Park BH, Burnette BL, et al. Randomised Phase III Postoperative Trial of Platinum-Based Chemotherapy Versus Capecitabine in Patients With Residual Triple-Negative Breast Cancer Following Neoadjuvant Chemotherapy: ECOG-ACRIN Ea1131. J Clin Oncol (2021) 39(23):2539–51. doi: 10.1200/JCO.21.0097675859012345666768690123
98. Yu K-D, Ye F-G, He M, Fan L, Ma D, Mo M, et al. Effect of Adjuvant Paclitaxel and Carboplatin on Survival in Women With Triple-Negative Breast Cancer. JAMA Oncol (2020) 6(9):1390. doi: 10.1001/jamaoncol.2020.2965
99. Cheng YC, O'Regan R, Chaudhary LN, Kamaraju S, Einhorn H, Sampene E, et al. Abstract PS13-51: A Phase II Study of Neoadjuvant Weekly Carboplatin/Paclitaxel Followed by Dose-Dense Doxorubicin/Cyclophosphamide (DD AC)in Patients With Triple Negative Breast Cancer (TNBC): Wisconsin Oncology Network (WON) Study. Cancer Res (2021) 81(4):PS13–51. doi: 10.1158/1538-7445.SABCS20-PS13-5
100. Abraham J, Vallier A-L, Qian W, Machin A, Grybowicz L, Thomas S, et al. PARTNER: Randomised, Phase II/III Trial to Evaluate the Safety and Efficacy of the Addition of Olaparib to Platinum-Based Neoadjuvant Chemotherapy in Triple Negative and/or Germline BRCA Mutated Breast Cancer Patients. J Clin Oncol (2018) 36(15):TPS605–5. doi: 10.1200/JCO.2018.36.15_suppl.TPS605
101. Kern P, Kalisch A, von Minckwitz G, Pütter C, Kolberg H-C, Pott D, et al. Neoadjuvant, Anthracycline-Free Chemotherapy With Carboplatin and Docetaxel in Triple-Negative, Early-Stage Breast Cancer: A Multicentric Analysis of Rates of Pathologic Complete Response and Survival. J Chemother (2016) 28(3):210–7. doi: 10.1179/1973947815Y.0000000061
102. Roy V, Pockaj BA, Allred JB, Apsey H, Northfelt DW, Nikcevich D, et al. A Phase II Trial of Docetaxel and Carboplatin Administered Every 2 Weeks as Preoperative Therapy for Stage II or III Breast Cancer. Am J Clin Oncol (2013) 36(6):540–4. doi: 10.1097/COC.0b013e318256f619
103. Ando M, Yamauchi H, Aogi K, Shimizu S, Iwata H, Masuda N, et al. Randomised Phase II Study of Weekly Paclitaxel With and Without Carboplatin Followed by Cyclophosphamide/Epirubicin/5-Fluorouracil as Neoadjuvant Chemotherapy for Stage II/IIIA Breast Cancer Without HER2 Overexpression. Breast Cancer Res Treat (2014) 145(2):401–9. doi: 10.1007/S10549-014-2947-1
104. Caron M-C, Sharma AK, O’Sullivan J, Myler LR, Ferreira MT, Rodrigue A, et al. Poly(ADP-Ribose) Polymerase-1 Antagonizes DNA Resection at Double-Strand Breaks. Nat Commun (2019) 10(1):2954. doi: 10.1038/s41467-019-10741-9
105. Maya-Mendoza A, Moudry P, Merchut-Maya JM, Lee M, Strauss R, Bartek J. High Speed of Fork Progression Induces DNA Replication Stress and Genomic Instability. Nature (2018) 559(7713):279–84. doi: 10.1038/s41586-018-0261-5
106. Farmer H, McCabe N, Lord CJ, Tutt ANJ, Johnson DA, Richardson TB, et al. Targeting the DNA Repair Defect in BRCA Mutant Cells as a Therapeutic Strategy. Nature (2005) 434(7035):917–21. doi: 10.1038/nature03445
107. Litton JK, Rugo HS, Ettl J, Hurvitz SA, Gonçalves A, Lee K-H, et al. Talazoparib in Patients With Advanced Breast Cancer and a Germline BRCA Mutation. New Engl J Med (2018) 379(8):753–63. doi: 10.1056/NEJMoa1802905
108. Robson M, Im S-A, Senkus E, Xu B, Domchek SM, Masuda N, et al. Olaparib for Metastatic Breast Cancer in Patients With a Germline BRCA Mutation. New Engl J Med (2017) 377(6):523–33. doi: 10.1056/NEJMoa1706450
109. Eikesdal HP, Yndestad S, Elzawahry A, Llop-Guevara A, Gilje B, Blix ES, et al. Olaparib Monotherapy as Primary Treatment in Unselected Triple Negative Breast Cancer. Ann Oncol (2021) 32(2):240–9. doi: 10.1016/j.annonc.2020.11.009
110. Fasching PA, Link T, Hauke J, Seither F, Jackisch C, Klare P, et al. Neoadjuvant Paclitaxel/Olaparib in Comparison to Paclitaxel/Carboplatinum in Patients With HER2-Negative Breast Cancer and Homologous Recombination Deficiency (GeparOLA Study). Ann Oncol (2020) 32(1):P49–57. doi: 10.1016/j.annonc.2020.10.471. 5.
111. Alba KP, McMurtry E, Vallier A-L, Grybowicz L, Copson E, Armstrong A, et al. Abstract P3-10-05: Preliminary Safety Data From Stage 1 and 2 of the Phase II/III PARTNER Trial: Addition of Olaparib to Platinum-Based Neoadjuvant Chemotherapy in Triple Negative and/or Germline BRCA Mutated Breast Cancer Patients. Poster Session Abstracts (2020) 80(4_Supplement):P3–10–05–P3–10–05. doi: 10.1158/1538-7445.SABCS19-P3-10-05
112. Pusztai L, Yau C, Wolf DM, Han HS, Du L, Wallace AM, et al. Durvalumab With Olaparib and Paclitaxel for High-Risk HER2-Negative Stage II/III Breast Cancer: Results From the Adaptively Randomised I-SPY2 Trial. Cancer Cell (2021) 39(7):989–98.e5. doi: 10.1016/j.ccell.2021.05.009
113. Loap P, Loirat D, Berger F, Ricci F, Vincent-Salomon A, Ezzili C, et al. Combination of Olaparib and Radiation Therapy for Triple Negative Breast Cancer: Preliminary Results of the RADIOPARP Phase 1 Trial. Int J Radiat Oncol Biol Phys (2021) 109(2):436–40. doi: 10.1016/j.ijrobp.2020.09.032
114. Litton JK, Scoggins ME, Hess KR, Adrada BE, Murthy RK, Damodaran S, et al. Neoadjuvant Talazoparib for Patients With Operable Breast Cancer With a Germline BRCA Pathogenic Variant. J Clin Oncol (2020) 38(5):388–94. doi: 10.1200/JCO.19.01304
115. Schwab R, Clark AS, Yau C, Hylton N, Li W, Wolfe D, et al. Abstract CT136: Evaluation of Talazoparib in Combination With Irinotecan in Early Stage, High-Risk HER2 Negative Breast Cancer: Results From the I-SPY 2 TRIAL. Clin Trials (2019) 79(13_Supplement):CT136–6. doi: 10.1158/1538-7445.AM2019-CT136
116. Severson TM, Wolf DM, Yau C, Peeters J, Wehkam D, Schouten PC, et al. The BRCA1ness Signature is Associated Significantly With Response to PARP Inhibitor Treatment Versus Control in the I-SPY 2 Randomised Neoadjuvant Setting. Breast Cancer Res (2017) 19(1):1–9. doi: 10.1186/s13058-017-0861-2
117. Diéras V, Han HS, Kaufman B, Wildiers PH, Friedlander PM, Ayoub J-P, et al. Veliparib With Carboplatin and Paclitaxel in BRCA-Mutated Advanced Breast Cancer (BROCADE3): A Randomised, Double-Blind, Placebo-Controlled, Phase 3 Trial. Lancet Oncol (2020) 21(10):1269–82. doi: 10.1016/S1470-2045(20)30447-2
118. Jagsi R, Griffith KA, Bellon JR, Woodward WA, Horton JK, Ho A, et al. Concurrent Veliparib With Chest Wall and Nodal Radiotherapy in Patients With Inflammatory or Locoregionally Recurrent Breast Cancer: The TBCRC 024 Phase I Multicenter Study. J Clin Oncol (2018) 36(13):1317–22. doi: 10.1200/JCO.2017.77.2665
119. Hahnen E, Lederer B, Hauke J, Loibl S, Kröber S, Schneeweiss A, et al. Germline Mutation Status, Pathological Complete Response, and Disease-Free Survival in Triple-Negative Breast Cancer. JAMA Oncol (2017) 3(10):1378. doi: 10.1001/jamaoncol.2017.1007
120. Hoadley KA, Powell BC, Kanavy D, Marron D, Mose LE, Hyslop T, et al. Abstract P4-05-03: Mutational Analysis of Triple-Negative Breast Cancer (TNBC): CALGB 40603 (Alliance). Poster Session Abstracts (2020) 80(4_Supplement):P4–05–03–P4–05–03. doi: 10.1158/1538-7445.SABCS19-P4-05-03
121. Wolf DM, Yau C, Sanil A, Glas A, Petricoin E, Wulfkuhle J, et al. DNA Repair Deficiency Biomarkers and the 70-Gene Ultra-High Risk Signature as Predictors of Veliparib/Carboplatin Response in the I-SPY 2 Breast Cancer Trial. NPJ Breast Cancer (2017) 3(1):1–8. doi: 10.1038/s41523-017-0025-7
122. Schneeweiss A, Möbus V, Tesch H, Hanusch C, Denkert C, Lübbe K, et al. Intense Dose-Dense Epirubicin, Paclitaxel, Cyclophosphamide Versus Weekly Paclitaxel, Liposomal Doxorubicin (Plus Carboplatin in Triple-Negative Breast Cancer) for Neoadjuvant Treatment of High-Risk Early Breast Cancer (GeparOcto—GBG 84): A Randomised Phase III Trial. Eur J Cancer (2019) 106:181–92. doi: 10.1016/j.ejca.2018.10.015
123. Prat A, Lluch A, Albanell J, Barry WT, Fan C, Chacón JI, et al. Predicting Response and Survival in Chemotherapy-Treated Triple-Negative Breast Cancer. Br J Cancer (2014) 111:1532–41. doi: 10.1038/bjc.2014.444
124. Graeser M, McCarthy A, Lord CJ, Savage K, Hills M, Salter J, et al. A Marker of Homologous Recombination Predicts Pathologic Complete Response to Neoadjuvant Chemotherapy in Primary Breast Cancer. Clin Cancer Res (2010) 16(24):6159–68. doi: 10.1158/1078-0432.CCR-10-1027
125. Peng G, Chun-Jen Lin C, Mo W, Dai H, Park Y-Y, Kim SM, et al. Genome-Wide Transcriptome Profiling of Homologous Recombination DNA Repair. Nat Commun (2014) 5(1):3361. doi: 10.1038/ncomms4361
126. Vollebergh MA, Lips EH, Nederlof PM, Wessels LF, Wesseling J, vd Vijver MJ, et al. Genomic Patterns Resembling BRCA1- and BRCA2-Mutated Breast Cancers Predict Benefit of Intensified Carboplatin-Based Chemotherapy. Breast Cancer Res (2014) 16(3):R47. doi: 10.1186/bcr3655
127. Telli ML, Jensen KC, Vinayak S, Kurian AW, Lipson JA, Flaherty PJ, et al. Phase II Study of Gemcitabine, Carboplatin, and Iniparib As Neoadjuvant Therapy for Triple-Negative and BRCA1 / 2 Mutation–Associated Breast Cancer With Assessment of a Tumour-Based Measure of Genomic Instability: PrECOG 0105. J Clin Oncol (2015) 33(17):1895–901. doi: 10.1200/JCO.2014.57.0085
128. von Minckwitz G, Timms K, Untch M, Elkin EP, Fasching PA, Schneeweiss A, et al. Prediction of Pathological Complete Response (pCR) by Homologous Recombination Deficiency (HRD) After Carboplatin-Containing Neoadjuvant Chemotherapy in Patients With TNBC: Results From GeparSixto. J Clin Oncol (2015) 33(15):1004–4. doi: 10.1200/jco.2015.33.15_suppl.1004
129. Loibl S, Weber KE, Timms KM, Elkin EP, Hahnen E, Fasching PA, et al. Survival Analysis of Carboplatin Added to an Anthracycline/Taxane-Based Neoadjuvant Chemotherapy and HRD Score as Predictor of Response—Final Results From GeparSixto. Ann Oncol (2018) 29(12):2341–7. doi: 10.1093/annonc/mdy460
130. Chopra N, Tovey H, Pearson A, Cutts R, Toms C, Proszek P, et al. Homologous recombination DNA repair deficiency and PARP inhibition activity in primary triple negative breast cancer. Nat Commun (2020) 11(1):2662. doi: 10.1038/s41467-020-16142-7
131. Staaf J, Glodzik D, Bosch A, Vallon-Christersson J, Reuterswärd C, Häkkinen J, et al. Whole-Genome Sequencing of Triple-Negative Breast Cancers in a Population-Based Clinical Study. Nat Med (2019) 25:1526–33. doi: 10.1038/s41591-019-0582-4
132. Sohn I, Jung WY, Sung CO. Somatic Hypermutation and Outcomes of Platinum Based Chemotherapy in Patients With High Grade Serous Ovarian Cancer. Gynecol Oncol (2012) 126(1):103–8. doi: 10.1016/j.ygyno.2012.03.050
133. Mei P, Freitag CE, Wei L, Zhang Y, Parwani A, Li Z. High Tumour Mutation Burden is Associated With DNA Damage Repair Gene Mutation in Breast Carcinomas. Diagn Pathol (2020) 15(1):50. doi: 10.1186/s13000-020-00971-7
134. Gatti-Mays ME, Balko JM, Gameiro SR, Bear HD, Prabhakaran S, Fukui J, et al. If We Build it They Will Come: Targeting the Immune Response to Breast Cancer. NPJ Breast Cancer (2019) 5(1):37. doi: 10.1038/s41523-019-0133-7
135. Mittendorf EA, Philips A, Meric-Bernstam F, Qiao N, Wu Y, Harrington S, et al. PD-L1 Expression in Triple-Negative Breast Cancer. Cancer Immunol Res (2014) 2(4):361–70. doi: 10.1158/2326-6066.CIR-13-0127
136. Nanda R, Liu MC, Yau C, Shatsky R, Pusztai L, Wallace A, et al. Effect of Pembrolizumab Plus Neoadjuvant Chemotherapy on Pathologic Complete Response in Women With Early-Stage Breast Cancer. JAMA Oncol (2020) 6(5):676. doi: 10.1001/jamaoncol.2019.6650
137. Schmid P, Salgado R, Park YH, Muñoz-Couselo E, Kim SB, Sohn J, et al. Pembrolizumab Plus Chemotherapy as Neoadjuvant Treatment of High-Risk, Early-Stage Triple-Negative Breast Cancer: Results From the Phase 1b Open-Label, Multicohort KEYNOTE-173 Study. Ann Oncol (2020) 31:569–81. doi: 10.1016/j.annonc.2020.01.072
138. Schmid P, Cortes J, Pusztai L, McArthur H, Kümmel S, Bergh J, et al. Pembrolizumab for Early Triple-Negative Breast Cancer. New Engl J Med (2020) 382(9):810–21. doi: 10.1056/NEJMoa1910549
139. Mittendorf EA, Zhang H, Barrios CH, Saji S, Jung KH, Hegg R, et al. Neoadjuvant Atezolizumab in Combination With Sequential Nab-Paclitaxel and Anthracycline-Based Chemotherapy Versus Placebo and Chemotherapy in Patients With Early-Stage Triple-Negative Breast Cancer (IMpassion031): A Randomised, Double-Blind, Phase 3 Trial. Lancet (2020) 396:1090–100. doi: 10.1016/S0140-6736(20)31953-X
140. Gianni L, Huang CS, Egle D, Bermejo B, Zamagni C, Thill M, et al. Pathologic Complete Response (pCR) to Neoadjuvant Treatment With or Without Atezolizumab in Triple-Negative, Early High-Risk and Locally Advanced Breast Cancer: NeoTRIP Michelangelo Randomized Study. Ann Oncol (2022) 33(5):534–43. doi: 10.1016/j.annonc.2022.02.004. S0923-7534(22)00113-2.
141. Voorwerk L, Slagter M, Horlings HM, Sikorska K, van deVijver KK, de Maaker M, et al. Immune Induction Strategies in Metastatic Triple-Negative Breast Cancer to Enhance the Sensitivity to PD-1 Blockade: The TONIC Trial. Nat Med (2019) 25:920–8. doi: 10.1038/s41591-019-0432-4
142. Loibl S, Untch M, Burchardi N, Huober J, Sinn BV, Blohmer J-U, et al. A Randomised Phase II Study Investigating Durvalumab in Addition to an Anthracycline Taxane-Based Neoadjuvant Therapy in Early Triple-Negative Breast Cancer: Clinical Results and Biomarker Analysis of GeparNuevo Study. Ann Oncol (2019) 30(8):1279–88. doi: 10.1093/annonc/mdz158
143. Loibl S, Schneeweiss A, Huober JB, Braun M, Rey J, Blohmer JU, et al. Durvalumab Improves Long-Term Outcome in TNBC: Results From the Phase II Randomised GeparNUEVO Study Investigating Neodjuvant Durvalumab in Addition to an Anthracycline/Taxane Based Neoadjuvant Chemotherapy in Early Triple-Negative Breast Cancer (TNBC). J Clin Oncol (2021) 39(15):506–6. doi: 10.1200/JCO.2021.39.15_suppl.506
144. Ademuyiwa FO, Gao F, Chen I, Northfelt DW, Wesolowski R, Arora M, et al. Abstract PD14-09: Nci 10013 - A Randomised Phase 2 Study of Neoadjuvant Carboplatin and Paclitaxel, With or Without Atezolizumab in Triple Negative Breast Cancer (TNBC). Cancer Res (2021) 81(4_Supplement). doi: 10.1158/1538-7445.SABCS20-PD14-09. Poster Spotlight Session Abstracts, PD14-09-PD14-09.
145. Yam C, Mittendorf EA, Sun R, Huo L, Damodaran S, Rauch GM, et al. Neoadjuvant Atezolizumab (Atezo) and Nab-Paclitaxel (Nab-P) in Patients (Pts) With Triple-Negative Breast Cancer (TNBC) With Suboptimal Clinical Response to Doxorubicin and Cyclophosphamide (AC). J Clin Oncol (2021) 39(15):592–2. doi: 10.1200/JCO.2021.39.15_suppl.592
146. Foldi J, Silber A, Reisenbichler E, Singh K, Fischbach N, Persico J, et al. Neoadjuvant Durvalumab Plus Weekly Nab-Paclitaxel and Dose-Dense Doxorubicin/Cyclophosphamide in Triple-Negative Breast Cancer. NPJ Breast Cancer (2021) 7(9). doi: 10.1038/s41523-021-00219-7
147. Yee D, DeMichele AM, Yau C, Isaacs C, Symmans WF, Albain KS, et al. Association of Event-Free and Distant Recurrence–Free Survival With Individual-Level Pathologic Complete Response in Neoadjuvant Treatment of Stages 2 and 3 Breast Cancer. JAMA Oncol (2020) 6(9):1355–62. doi: 10.1001/jamaoncol.2020.2535
148. Rugo HS, Loi S, Adams S, Schmid P, Schneeweiss A, Barrios CH, et al. PD-L1 Immunohistochemistry Assay Comparison in Atezolizumab Plus Nab -Paclitaxel–Treated Advanced Triple-Negative Breast Cancer. JNCI: J Natl Cancer Inst (2021) 113(12):1733–43. doi: 10.1093/jnci/djab108
149. Wei SC, Duffy CR, Allison JP. Fundamental Mechanisms of Immune Checkpoint Blockade Therapy. Cancer Discovery (2018) 8(9):1069–86. doi: 10.1158/2159-8290.CD-18-0367
150. Sha D, Jin Z, Budczies J, Kluck K, Stenzinger A, Sinicrope FA. Tumour Mutational Burden as a Predictive Biomarker in Solid Tumours. Cancer Discovery (2020) 10(12):1808–25. doi: 10.1158/2159-8290.CD-20-0522
151. Cristescu R, Mogg R, Ayers M, Albright A, Murphy E, Yearley J, et al. Pan-Tumour Genomic Biomarkers for PD-1 Checkpoint Blockade-Based Immunotherapy. Science (2018) 362(6411):eaar359. doi: 10.1126/science.aar3593
152. Yarchoan M, Albacker LA, Hopkins AC, Montesion M, Murugesan K, Vithayathil TT, et al. PD-L1 Expression and Tumour Mutational Burden are Independent Biomarkers in Most Cancers. JCI Insight (2019) 4(6):e126908. doi: 10.1172/jci.insight.126908
153. Marabelle A, Fakih M, Lopez J, Shah M, Shapira-Frommer R, Nakagawa K, et al. Association of Tumour Mutational Burden With Outcomes in Patients With Advanced Solid Tumours Treated With Pembrolizumab: Prospective Biomarker Analysis of the Multicohort, Open-Label, Phase 2 KEYNOTE-158 Study. Lancet Oncol (2020) 21(10):1353–65. doi: 10.1016/S1470-2045(20)30445-9
154. McGrail DJ, Pilié PG, Rashid NU, Voorwerk L, Slagter M, Kok M, et al. High Tumour Mutation Burden Fails to Predict Immune Checkpoint Blockade Response Across All Cancer Types. Ann Oncol (2021) 32(5):661–72. doi: 10.1016/j.annonc.2021.02.006
155. Karn T, Denkert C, Weber KE, Holtrich U, Hanusch C, Sinn BV, et al. Tumor Mutational Burden and Immune Infiltration as Independent Predictors of Response to Neoadjuvant Immune Checkpoint Inhibition in Early TNBC in GeparNuevo. Ann Oncol (2020) 31:1216–22. doi: 10.1016/j.annonc.2020.05.015
156. Moulder SL, Hess KR, Candelaria RP, Rauch GM, Santiago L, Adrada B, et al. Precision neoadjuvant therapy (P-NAT): A Planned Interim Analysis of a Randomized, TNBC Enrolling Trial to Confirm Molecular Profiling Improves Survival (ARTEMIS). J Clin Oncol (2018) 36:518–8. doi: 10.1200/JCO.2018.36.15_suppl.518
157. Criscitiello C, Bayar MA, Curigliano G, Symmans FW, Desmedt C, Bonnefoi H, et al. A Gene Signature to Predict High Tumour-Infiltrating Lymphocytes After Neoadjuvant Chemotherapy and Outcome in Patients With Triple-Negative Breast Cancer. Ann Oncol : Off J Eur Soc Med Oncol (2018) 29(1):162–9. doi: 10.1093/ANNONC/MDX691
158. Gao G, Wang Z, Qu X, Zhang Z. Prognostic Value of Tumour-Infiltrating Lymphocytes in Patients With Triple-Negative Breast Cancer: A Systematic Review and Meta-Analysis. BMC Cancer (2020) 20(1):1–15. doi: 10.1186/S12885-020-6668-Z/FIGURES/6
159. Loi S, Drubay D, Adams S, Pruneri G, Francis PA, Lacroix-Triki M, et al. Tumour-Infiltrating Lymphocytes and Prognosis: A Pooled Individual Patient Analysis of Early-Stage Triple-Negative Breast Cancers. J Clin Oncol : Off J Am Soc Clin Oncol (2019) 37(7):559–69. doi: 10.1200/JCO.18.01010
160. Callari M, Cappelletti V, D’Aiuto F, Musella V, Lembo A, Petel F, et al. Subtype-Specific Metagene-Based Prediction of Outcome After Neoadjuvant and Adjuvant Treatment in Breast Cancer. Clin Cancer Res (2016) 22(2):337–45. doi: 10.1158/1078-0432.CCR-15-0757
161. Gruosso T, Gigoux M, Manem VSK, Bertos N, Zuo D, Perlitch I, et al. Spatially Distinct Tumour Immune Microenvironments Stratify Triple-Negative Breast Cancers. J Clin Invest (2019) 129(4):1785–800. doi: 10.1172/JCI96313
162. Pérez-Pena J, Tibor Fekete J, Páez R, Baliu-Piqué M, García-Saenz JÁ, García-Barberán V, et al. A Transcriptomic Immunologic Signature Predicts Favorable Outcome in Neoadjuvant Chemotherapy Treated Triple Negative Breast Tumours. Front Immunol (2019) 10:2802/BIBTEX. doi: 10.3389/FIMMU.2019.02802/BIBTEX
163. Craven KE, Gökmen-Polar Y, Badve SS. CIBERSORT Analysis of TCGA and METABRIC Identifies Subgroups With Better Outcomes in Triple Negative Breast Cancer. Sci Rep (2021) 11:1. doi: 10.1038/s41598-021-83913-7
164. Sharma P, Barlow WE, Godwin AK, Parkes EE, Knight LA, Walker SM, et al. Validation of the DNA Damage Immune Response Signature in Patients With Triple-Negative Breast Cancer From the SWOG 9313c Trial. J Clin Oncol : Off J Am Soc Clin Oncol (2019) 37(36):3484–92. doi: 10.1200/JCO.19.00693
165. Lv Y, Lv D, Lv X, Xing P, Zhang J, Zhang Y. Immune Cell Infiltration-Based Characterization of Triple-Negative Breast Cancer Predicts Prognosis and Chemotherapy Response Markers. Front Genet (2021) 12:616469/BIBTEX. doi: 10.3389/FGENE.2021.616469/BIBTEX
166. Loibl S, Sinn B, Karn T, Untch M, Treue D, Sinn H-P, et al. Abstract PD2-07: mRNA Signatures Predict Response to Durvalumab Therapy in Triple Negative Breast Cancer (TNBC)– Results of the Translational Biomarker Programme of the Neoadjuvant Double-Blind Placebo Controlled GeparNuevo Trial. Cancer Res (2019) 79 (4_Supplement). doi: 10.1158/1538-7445.SABCS18-PD2-07. Poster Discussion Abstracts, PD2-07-PD2-07.
167. Marcus L, Lemery SJ, Keegan P, Pazdur R. FDA Approval Summary: Pembrolizumab for the Treatment of Microsatellite Instability-High Solid Tumours. Clin Cancer Res : Off J Am Assoc Cancer Res (2019) 25(13):3753–8. doi: 10.1158/1078-0432.CCR-18-4070
168. Obeid E, Ellerbrock A, Handorf E, Goldstein L, Gatalica Z, Arguello D, et al. Abstract PD6-03: Distribution of Microsatellite Instability, Tumor Mutational Load, and PD-L1 Status in Molecularly Profiled Invasive Breast Cancer. Poster Discussion Abstracts. Am Assoc Cancer Res (2018), PD6–03-PD6-03. doi: 10.1158/1538-7445.SABCS17-PD6-03
169. Marabelle A, Le DT, Ascierto PA, Di Giacomo AM, De Jesus-Acosta A, Delord J-P, et al. Efficacy of Pembrolizumab in Patients With Noncolorectal High Microsatellite Instability/Mismatch Repair–Deficient Cancer: Results From the Phase II KEYNOTE-158 Study. J Clin Oncol (2020) 38:1–10. doi: 10.1200/JCO.19.02105
170. Martín M, Chan A, Dirix L, O’Shaughnessy J, Hegg R, Manikhas A, et al. A Randomised Adaptive Phase II/III Study of Buparlisib, a Pan-Class I PI3K Inhibitor, Combined With Paclitaxel for the Treatment of HER2- Advanced Breast Cancer (BELLE-4). Ann Oncol : Off J Eur Soc Med Oncol (2017) 28(2):313–20. doi: 10.1093/ANNONC/MDW562
171. Oliveira M, Saura C, Nuciforo P, Calvo I, Andersen J, Passos-Coelho JL, et al. FAIRLANE, a Double-Blind Placebo-Controlled Randomised Phase II Trial of Neoadjuvant Ipatasertib Plus Paclitaxel for Early Triple-Negative Breast Cancer. Ann Oncol : Off J Eur Soc Med Oncol (2019) 30(8):1289–97. doi: 10.1093/ANNONC/MDZ177
172. Chien AJ, Tripathy D, Albain KS, Symmans WF, Rugo HS, Melisko ME, et al. MK-2206 and Standard Neoadjuvant Chemotherapy Improves Response in Patients With Human Epidermal Growth Factor Receptor 2-Positive and/or Hormone Receptor-Negative Breast Cancers in the I-SPY 2 Trial. J Clin Oncol : Off J Am Soc Clin Oncol (2020) 38(10):1059–69. doi: 10.1200/JCO.19.01027
173. Jovanović B, Mayer IA, Mayer EL, Abramson VG, Bardia A, Sanders ME, et al. A Randomized Phase II Neoadjuvant Study of Cisplatin, Paclitaxel With or Without Everolimus in Patients With Stage II/III Triple-Negative Breast Cancer (TNBC): Responses and Long-Term Outcome Correlated With Increased Frequency of DNA Damage Response Gene. Clin Cancer Res (2017) 23:4035–45. doi: 10.1158/1078-0432.CCR-1
174. Gonzalez-Angulo AM, Akcakanat A, Liu S, Green MC, Murray JL, Chen H, et al. Open-Label Randomised Clinical Trial of Standard Neoadjuvant Chemotherapy With Paclitaxel Followed by FEC Versus the Combination of Paclitaxel and Everolimus Followed by FEC in Women With Triple Receptor-Negative Breast Cancer†. Ann Oncol : Off J Eur Soc Med Oncol (2014) 25(6):1122–7. doi: 10.1093/ANNONC/MDU124
175. Park S, Koo J, Park HS, Kim JH, Choi SY, Lee JH, et al. Expression of Androgen Receptors in Primary Breast Cancer. Ann Oncol : Off J Eur Soc Med Oncol (2010) 21(3):488–92. doi: 10.1093/ANNONC/MDP510
176. Niemeier LA, Dabbs DJ, Beriwal S, Striebel JM, Bhargava R. Androgen Receptor in Breast Cancer: Expression in Oestrogen Receptor-Positive Tumours and in Oestrogen Receptor-Negative Tumours With Apocrine Differentiation. Modern Pathol : Off J United States Can Acad Pathol Inc (2010) 23(2):205–12. doi: 10.1038/MODPATHOL.2009.159
177. Traina TA, Miller K, Yardley DA, Eakle J, Schwartzberg LS, O’Shaughnessy J, et al. Enzalutamide for the Treatment of Androgen Receptor-Expressing Triple-Negative Breast Cancer. J Clin Oncol : Off J Am Soc Clin Oncol (2018) 36(9):884–90. doi: 10.1200/JCO.2016.71.3495
178. Lehmann BD, Abramson VG, Sanders ME, Mayer EL, Haddad TC, Nanda R, et al. TBCRC 032 IB/II Multicenter Study: Molecular Insights to AR Antagonist and PI3K Inhibitor Efficacy in Patients With AR + Metastatic Triple-Negative Breast Cancer. Clin Cancer Res : Off J Am Assoc Cancer Res (2020) 26(9):2111–23. doi: 10.1158/1078-0432.CCR-19-2170
179. Bonnefoi H, Grellety T, Tredan O, Saghatchian M, Dalenc F, Mailliez A, et al. A Phase II Trial of Abiraterone Acetate Plus Prednisone in Patients With Triple-Negative Androgen Receptor Positive Locally Advanced or Metastatic Breast Cancer (UCBG 12-1). Ann Oncol : Off J Eur Soc Med Oncol (2016) 27(5):812–8. doi: 10.1093/ANNONC/MDW067
180. Gucalp A, Tolaney S, Isakoff SJ, Ingle JN, Liu MC, Carey LA, et al. Phase II trial of bicalutamide in patients with androgen receptor-positive, oestrogen receptor-negative metastatic Breast Cancer. Clin Cancer Res : Off J Am Assoc Cancer Res (2013) 19(19):5505–12. doi: 10.1158/1078-0432.CCR-12-3327
181. Schettini F, Chic N, Brasó-Maristany F, Paré L, Pascual T, Conte B, et al. Clinical, Pathological, and PAM50 Gene Expression Features of HER2-Low Breast Cancer. NPJ Breast Cancer (2021) 7:1. doi: 10.1038/s41523-020-00208-2
182. Wulfkuhle JD, Yau C, Wolf DM, Vis DJ, Gallagher RI, Brown-Swigart L, et al. Evaluation of the HER/PI3K/AKT Family Signaling Network as a Predictive Biomarker of Pathologic Complete Response for Patients With Breast Cancer Treated With Neratinib in the I-SPY 2 TRIAL. JCO Precis Onco (2018) 2:1–20. doi: 10.1200/PO.18.00024
183. Conlon NT, Kooijman JJ, van Gerwen SJC, Mulder WR, Zaman GJR, Diala I, et al. Comparative Analysis of Drug Response and Gene Profiling of HER2-Targeted Tyrosine Kinase Inhibitors. Br J Cancer (2021) 124:7. doi: 10.1038/s41416-020-01257-x
184. Wulfkuhle JD, Yau C, Wolf DM, Gallagher RI, Deng J, Swigart LB, et al. Protein Activation Mapping and Exploratory Predictive Markers for pCR in Triple-Negative Breast Cancer Patients Treated With Neratinib in the I-SPY 2 TRIAL. J Clin Oncol (2015) 33 (15_Supplement). doi: 10.1200/JCO.2015.33.15_SUPPL.1085
185. Modi S, Park H, Murthy RK, Iwata H, Tamura K, Tsurutani J, et al. Antitumour Activity and Safety of Trastuzumab Deruxtecan in Patients With HER2-Low-Expressing Advanced Breast Cancer: Results From a Phase Ib Study. J Clin Oncol : Off J Am Soc Clin Oncol (2020) 38(17):1887–96. doi: 10.1200/JCO.19.02318
186. Banerji U, van Herpen CML, Saura C, Thistlethwaite F, Lord S, Moreno V, et al. Trastuzumab Duocarmazine in Locally Advanced and Metastatic Solid Tumours and HER2-Expressing Breast Cancer: A Phase 1 Dose-Escalation and Dose-Expansion Study. Lancet Oncol (2019) 20(8):1124–35. doi: 10.1016/S1470-2045(19)30328-6
187. Linderholm BK, Hellborg H, Johansson U, Elmberger G, Skoog L, Lehtiö J, et al. Significantly Higher Levels of Vascular Endothelial Growth Factor (VEGF) and Shorter Survival Times for Patients With Primary Operable Triple-Negative Breast Cancer. Ann Oncol : Off J Eur Soc Med Oncol (2009) 20(10):1639–46. doi: 10.1093/ANNONC/MDP062
188. Ali EM, Sheta M, El Mohsen MA. Elevated serum and tissue VEGF associated with poor outcome in breast cancer patients. Alexandria J Med (2011) 47(3):217–24. doi: 10.1016/j.ajme.2011.07.003
189. von Minckwitz G, Eidtmann H, Rezai M, Fasching PA, Tesch H, Eggemann H, et al. Neoadjuvant Chemotherapy and Bevacizumab for HER2-Negative Breast Cancer. New Engl J Med (2012) 366(4):299–309. doi: 10.1056/NEJMOA1111065/SUPPL_FILE/NEJMOA1111065_DISCLOSURES.PDF
190. Bear HD, Tang G, Rastogi P, Geyer CE, Liu Q, Robidoux A, et al. Neoadjuvant Plus Adjuvant Bevacizumab in Early Breast Cancer (NSABP B-40 [NRG Oncology]): Secondary Outcomes of a Phase 3, Randomised Controlled Trial. Lancet Oncol (2015) 16(9):1037–48. doi: 10.1016/S1470-2045(15)00041-8
191. Earl HM, Hiller L, Dunn JA, Blenkinsop C, Grybowicz L, Vallier A-L, et al. Efficacy of Neoadjuvant Bevacizumab Added to Docetaxel Followed by Fluorouracil, Epirubicin, and Cyclophosphamide, for Women With HER2-Negative Early Breast Cancer (ARTemis): An Open-Label, Randomised, Phase 3 Trial. Lancet Oncol (2015) 16(6):656–66. doi: 10.1016/S1470-2045(15)70137-3
192. Cameron D, Brown J, Dent R, Jackisch C, Mackey J, Pivot X, et al. Adjuvant Bevacizumab-Containing Therapy in Triple-Negative Breast Cancer (BEATRICE): Primary Results of a Randomised, Phase 3 Trial. Lancet Oncol (2013) 14(10):933–42. doi: 10.1016/S1470-2045(13)70335-8
193. Babina IS, Turner NC. Advances and Challenges in Targeting FGFR Signalling in Cancer. Nat Rev Cancer (2017) 17(5):318–32. doi: 10.1038/NRC.2017.8
194. Sung VYC, Knight JF, Johnson RM, Stern YE, Saleh SM, Savage P, et al. Co-dependency for MET and FGFR1 in basal triple-negative breast cancers. NPJ Breast Cancer (2021) 7:1. doi: 10.1038/s41523-021-00238-4
195. Williams CB, Soloff AC, Ethier SP, Yeh ES. Perspectives on Epidermal Growth Factor Receptor Regulation in Triple-Negative Breast Cancer: Ligand-Mediated Mechanisms of Receptor Regulation and Potential for Clinical Targeting. Adv Cancer Res (2015) 127:253–81. doi: 10.1016/bs.acr.2015.04.008
196. Lehmann BD, Bauer JA, Chen X, Sanders ME, Chakravarthy AB, Shyr Y, et al. Identification of Human Triple-Negative Breast Cancer Subtypes and Preclinical Models for Selection of Targeted Therapies. J Clin Invest (2011) 121(7):2750–67. doi: 10.1172/JCI45014
197. Teng YHF, Tan WJ, Thike AA, Cheok PY, Tse GMK, Wong NS, et al. Mutations in the Epidermal Growth Factor Receptor (EGFR) Gene in Triple Negative Breast Cancer: Possible Implications for Targeted Therapy. Breast Cancer Res : BCR (2011) 13(2):R35. doi: 10.1186/BCR2857
198. Park HS, Jang MH, Kim EJ, Kim HJ, Lee HJ, Kim YJ, et al. High EGFR Gene Copy Number Predicts Poor Outcome in Triple-Negative Breast Cancer. Modern Pathol : Off J United States Can Acad Pathol Inc (2014) 27(9):1212–22. doi: 10.1038/MODPATHOL.2013.251
199. Nabholtz JM, Chalabi N, Radosevic-Robin N, Dauplat MM, Mouret-Reynier MA, van Praagh I, et al. Multicentric Neoadjuvant Pilot Phase II Study of Cetuximab Combined With Docetaxel in Operable Triple Negative Breast Cancer. Int J Cancer (2016) 138(9):2274–80. doi: 10.1002/IJC.29952
200. Yardley DA, Ward PJ, Daniel BR, Eakle JF, Lamar RE, Lane CM, et al. Panitumumab, Gemcitabine, and Carboplatin as Treatment for Women With Metastatic Triple-Negative Breast Cancer: A Sarah Cannon Research Institute Phase II Trial. Clin Breast Cancer (2016) 16(5):349–55. doi: 10.1016/J.CLBC.2016.05.006
201. Finn RS, Press MF, Dering J, Arbushites M, Koehler M, Oliva C, et al. Oestrogen Receptor, Progesterone Receptor, Human Epidermal Growth Factor Receptor 2 (HER2), and Epidermal Growth Factor Receptor Expression and Benefit From Lapatinib in a Randomised Trial of Paclitaxel With Lapatinib or Placebo as First-Line Treatment in HER2-Negative or Unknown Metastatic Breast Cancer. J Clin Oncol : Off J Am Soc Clin Oncol (2009) 27(24):3908–15. doi: 10.1200/JCO.2008.18.1925
202. Drilon A, Laetsch TW, Kummar S, DuBois SG, Lassen UN, Demetri GD, et al. Efficacy of Larotrectinib in TRK Fusion–Positive Cancers in Adults and Children. New Engl J Med (2018) 378(8):731–9. doi: 10.1056/NEJMOA1714448/SUPPL_FILE/NEJMOA1714448_DISCLOSURES.PDF
203. Doebele RC, Drilon A, Paz-Ares L, Siena S, Shaw AT, Farago AF, et al. Entrectinib in Patients With Advanced or Metastatic NTRK Fusion-Positive Solid Tumours: Integrated Analysis of Three Phase 1–2 Trials. Lancet Oncol (2020) 21(2):271–82. doi: 10.1016/S1470-2045(19)30691-6
204. Goldenberg DM, Stein R, Sharkey RM. The Emergence of Trophoblast Cell-Surface Antigen 2 (TROP-2) as a Novel Cancer Target. Oncotarget (2018) 9(48):28989–9006. doi: 10.18632/ONCOTARGET.25615
205. Bardia A, Hurvitz SA, Tolaney SM, Loirat D, Punie K, Oliveira M, et al. Sacituzumab Govitecan in Metastatic Triple-Negative Breast Cancer. New Engl J Med (2021) 384(16):1529–41. doi: 10.1056/NEJMoa2028485
206. Harrison H, Farnie G, Howell SJ, Rock RE, Stylianou S, Brennan KR, et al. Regulation of Breast Cancer Stem Cell Activity by Signaling Through the Notch4 Receptor. Cancer Res (2010) 70(2):709–18. doi: 10.1158/0008-5472.CAN-09-1681
207. Qiu M, Peng Q, Jiang I, Carroll C, Han G, Rymer I, et al. Specific Inhibition of Notch1 Signaling Enhances the Antitumour Efficacy of Chemotherapy in Triple Negative Breast Cancer Through Reduction of Cancer Stem Cells. Cancer Lett (2013) 328(2):261–70. doi: 10.1016/J.CANLET.2012.09.023
208. Locatelli MA, Aftimos P, Claire Dees E, LoRusso PM, Pegram MD, Awada A, et al. Phase I Study of the Gamma Secretase Inhibitor PF-03084014 in Combination With Docetaxel in Patients With Advanced Triple-Negative Breast Cancer. Oncotarget (2017) 8(2):2320–8. doi: 10.18632/ONCOTARGET.13727
209. Balko JM, Cook RS, Vaught DB, Kuba MG, Miller TW, Bhola NE, et al. Profiling of Residual Breast Cancers After Neoadjuvant Chemotherapy Identifies DUSP4 Deficiency as a Mechanism of Drug Resistance. Nat Med (2012) 18(7):1052–9. doi: 10.1038/NM.2795
210. Pereira CBL, Leal MF, de Souza CRT, Montenegro RC, Rey JA, Carvalho AA, et al. Prognostic and Predictive Significance of MYC and KRAS Alterations in Breast Cancer From Women Treated With Neoadjuvant Chemotherapy. PLoS One (2013) 8(3):e60576. doi: 10.1371/JOURNAL.PONE.0060576
211. Balko JM, Schwarz LJ, Bhola NE, Kurupi R, Owens P, Miller TW, et al. Activation of MAPK Pathways Due to DUSP4 Loss Promotes Cancer Stem Cell-Like Phenotypes in Basal-Like Breast Cancer. Cancer Res (2013) 73(20):6346–58. doi: 10.1158/0008-5472.CAN-13-1385
212. Brufsky A, Kim SB, Eniu A, Mebis J, Sohn JH, Wongchenko M, et al. A Phase II Randomised Trial of Cobimetinib Plus Chemotherapy, With or Without Atezolizumab, as First-Line Treatment for Patients With Locally Advanced or Metastatic Triple-Negative Breast Cancer (COLET): Primary Analysis. Ann Oncol : Off J Eur Soc Med Oncol (2021) 32(5):652–60. doi: 10.1016/J.ANNONC.2021.01.065
213. Schmid P, Forster MD, Summers YJ, Good J, Sarker S-J, Lim L, et al. A Study of Vistusertib in Combination With Selumetinib in Patients With Advanced Cancers: TORCMEK Phase Ib Results. J Clin Oncol (2017) 35(15):2548–8. doi: 10.1200/JCO.2017.35.15_suppl.2548
214. Szekely B, Bossuyt V, Li X, Wali VB, Patwardhan GA, Frederick C, et al. Immunological Differences Between Primary and Metastatic Breast Cancer. Ann Oncol (2018) 29(11):2232–9. doi: 10.1093/annonc/mdy399
215. Berthelet J, Wimmer VC, Whitfield HJ, Serrano A, Boudier T, Mangiola S, et al. The Site of Breast Cancer Metastases Dictates Their Clonal Composition and Reversible Transcriptomic Profile. Science Advances. Sci Adv (2021) 7(28):eabf4408. doi: 10.1126/sciadv.abf4408
216. Trusolino L, Bertotti A. Compensatory Pathways in Oncogenic Kinase Signaling and Resistance to Targeted Therapies: Six Degrees of Separation: Figure 1. Cancer Discov (2012) 2(10):876–80. doi: 10.1158/2159-8290.CD-12-0400
217. Smedley D, Smith KR, Martin A, Thomas EA, McDonagh EM, Cipriani V, et al. 100,000 Genomes Pilot on Rare-Disease Diagnosis in Health Care — Preliminary Report. New Engl J Med (2021) 385(20):1868–80. doi: 10.1056/NEJMoa2035790
218. Roepman P, de Bruijn E, van Lieshout S, Schoenmaker L, Boelens MC, Dubbink HJ, et al. Clinical Validation of Whole Genome Sequencing for Cancer Diagnostics. J Mol Diagn (2021) 23(7):816–33. doi: 10.1016/j.jmoldx.2021.04.011
219. Pleasance E, Titmuss E, Williamson L, Kwan H, Culibrk L, Zhao EY, et al. Pan-Cancer Analysis of Advanced Patient Tumors Reveals Interactions Between Therapy and Genomic Landscapes. Nat Cancer (2020) 1(4):452–68. doi: 10.1038/s43018-020-0050-6
220. ICGC ARGO. Personalized Breast Cancer Programme - PBCP . Available at: https://www.icgc-argo.org/page/92/pbcp.
221. Bruna A, Rueda OM, Greenwood W, Batra AS, Callari M, Batra RN, et al. A Biobank of Breast Cancer Explants With Preserved Intra-Tumour Heterogeneity to Screen Anticancer Compounds. Cell (2016) 167(1):260–74.e22. doi: 10.1016/J.CELL.2016.08.041/ATTACHMENT/291DBAF4-326E-4D16-BED1-31FC25EDEEF1/MMC7.XLSX
Keywords: Breast cancer, precision medicine, triple negative, therapeutic, target, biomarkers, early disease
Citation: Pinilla K, Drewett LM, Lucey R and Abraham JE (2022) Precision Breast Cancer Medicine: Early Stage Triple Negative Breast Cancer—A Review of Molecular Characterisation, Therapeutic Targets and Future Trends. Front. Oncol. 12:866889. doi: 10.3389/fonc.2022.866889
Received: 31 January 2022; Accepted: 23 May 2022;
Published: 08 August 2022.
Edited by:
Angela Toss, University of Modena and Reggio Emilia, ItalyReviewed by:
Tira Tan, National Cancer Centre Singapore, SingaporeStan Lipkowitz, National Cancer Institute (NIH), United States
Copyright © 2022 Pinilla, Drewett, Lucey and Abraham. This is an open-access article distributed under the terms of the Creative Commons Attribution License (CC BY). The use, distribution or reproduction in other forums is permitted, provided the original author(s) and the copyright owner(s) are credited and that the original publication in this journal is cited, in accordance with accepted academic practice. No use, distribution or reproduction is permitted which does not comply with these terms.
*Correspondence: Jean E. Abraham, amEzNDRAbWVkc2NobC5jYW0uYWMudWs=