- 1Grupo de investigación en biología del cáncer, Instituto Nacional de Cancerología, Bogotá, Colombia
- 2Doctorado en Ciencias Biomédicas, Universidad del Valle, Cali, Colombia
- 3Facultad de Ciencias de la Salud, Universidad Simón Bolívar, Barranquilla, Colombia
- 4Grupo de apoyo y seguimiento para la investigación, Instituto Nacional de Cancerología, Bogotá, Colombia
Triple-negative breast cancer (TNBC) occurs more frequently in young (<50 years) non-Hispanic black and Hispanic/Latina women. It is considered the most aggressive subtype of breast cancer, although, recently, immune infiltrate has been associated with long-term survival, lower risk of death and recurrence, and response to neoadjuvant chemotherapy. The aim of this review was to evaluate the clinical impact of the immune infiltrate in TNBC by discussing whether its prognostic value varies across different populations. A comprehensive systematic search in databases such as PubMed and Web of Science was conducted to include papers focused on tumor-infiltrating lymphocytes (TILs) in TNBC in different population groups and that were published before January 2021. TNBC patients with higher levels of TILs had longer overall survival and disease-free survival times compared with TNBC patients with low TIL levels. Similar results were observed for CD4+, CD8+ TIL populations. On the other hand, patients with high TIL levels showed a higher rate of pathological complete response regardless of the population group (Asian, European, and American). These results altogether suggest that TIL subpopulations might have a prognostic role in TNBC, but the underlying mechanism needs to be elucidated. Although the prognosis value of TILs was not found different between the population groups analyzed in the revised literature, further studies including underrepresented populations with different genetic ancestries are still necessary to conclude in this regard.
Introduction
Breast cancer (BC) is a heterogeneous disease in its phenotypic and genomic features (1). Four intrinsic subtypes, luminal A, luminal B, HER2-enriched, and triple negative, have been reported, each one characterized by differences in the transcriptional profile and clinical behavior (2–4). The prevalence of these subtypes is variable between population groups (5, 6). Several studies have agreed that the triple-negative subtype is more prevalent in NHB and in H/L compared with non-Hispanic white (NHW) women (7–10).
Triple-negative breast cancer (TNBC) is characterized by the lack of expression of estrogen receptor (ER), progesterone receptor (PR), and human epidermal growth factor receptor 2 (HER2). It constitutes 10–20% of all breast cancers and occurs more frequently in young women (<50 years) (11, 12). It is the most aggressive subtype of BC considering that it presents with a larger tumor size and a higher histological grade at the time of diagnosis and has a high expression of cell proliferation genes, which correlated with their clinical characteristics and poor prognosis (13).
TNBC has been described as a transcriptionally heterogeneous subtype (14–16). Lehmann et al. (14) identified 6 subtypes through gene expression analysis: basal-like 1 (BL1) characterized by a high expression of genes involved in cell cycle and cellular division, basal-like 2 (BL2) that expresses genes that enrich the signaling by growth factors such as MET and EGFR and expresses myoepithelial markers, immunomodulator (IM) subtype that expresses genes involved in the signaling of immune cells and cytokine-mediated translation pathways, and the mesenchymal (M) and mesenchymal stem-like (MSL) subtypes which display similarities in terms of the high expression of genes involved in cell motility, epithelial–mesenchymal transition pathways, and growth factors (such as, NOTCH, PDGFR, FGFR, and TGFbeta dysregulation). However, the MSL subtype differs from the M subtype as it presents a lower expression of cell proliferation genes. Finally, the luminal androgen receptor (LAR) subtype presents a high expression of genes that participate mainly in hormonally regulated pathways, for example, by the androgen receptor (AR) (14, 17–19).
An important characteristic of TNBC is that it is the most immunogenic BC subtype. Its immune infiltrate has been associated with both the control of tumor cells and with the processes of tumor growth and metastasis (20–22). It has been likewise associated with the effectiveness of neoadjuvant and adjuvant therapy, thus correlating with the clinical outcome of the disease (23).
The variability in the immune infiltrate and its clinical impact in TNBC has been studied mainly in NHW women, but it is unknown how it may vary according to the population group. The aim of this review was to systematize those studies that have evaluated the clinical impact of the immune infiltrate in TNBC, discussing whether there are differences in its prognostic value based on the population groups.
Tumor microenvironment and immune infiltrate In breast cancer
The neoplastic progression of BC at the cellular level depends on the interaction of the tumor microenvironment (TME) and the adjacent immune system, which can act to promote or suppress the tumor growth and invasion (24, 25).
TME is composed of tumor cells and different stromal cells, such as fibroblasts, mesenchymal cells, immune cells, and adipocytes. These stromal cells secrete growth factors, cytokines, chemokines, and exosomes, molecules that maintain a constant interaction among cells within the TME (26, 27). Tumor cells are the only ones that have mutations within the TME and can promote epigenetic modifications on non-tumor cells. These modifications facilitate tumoral invasion, survival, and growth in an autocrine and paracrine way (25) (Figure 1).
Composition of tumor-infiltrating lymphocytes in TNBC
The antitumor immune response in the TME is mainly driven by tumor-infiltrating lymphocytes (TILS) which, according to their location in the TME, are divided into stromal (sTILs) and intratumoral (iTILs). Most of the lymphocytes are sTILs, which infiltrate the tissue adjacent to the tumor and are considered the real tumor-infiltrating cells; on the other hand, iTILs are in direct contact with the tumor, actively infiltrating it into nests (28). It is noteworthy that different subtypes of TILs may have inhibitory or stimulatory effects on tumor progression (29)—for instance, CD8+ T cells show the highest antitumor activity that is mediated by interferon-gamma (IFN-γ), perforin, and granzyme B secretion (30). In BC, a high number of CD8+ T cells has been associated with a better prognosis and response to neoadjuvant treatment (31). On the other hand, T helper cells CD4+ have the function of enhancing the adaptive immune response by increasing the infiltration and the effector functions of CD8+ T cells and other immune cells (32). Regulatory T cells (Treg), a subpopulation of CD4+ T cells, are positive for FOXP3 and CD25 markers and participate in immune escape by suppressing the antitumor activity of CD8+ T cells (33). The presence of Treg cells within the TME is commonly associated with a poor prognosis in cancer (34). However, recent studies have demonstrated the opposite in TNBC, where the presence of Tregs in the TME was associated with longer overall survival (OS) and disease-free survival (DFS) (35, 36).
B cells can produce specific antibodies for antigens present in tumor cells; however, it has not yet been demonstrated if these cells have the same degree of clinical significance as T cells (37). The presence of B cells in the tumor stroma has been correlated with longer DFS and metastasis-free survival (MFS) in TNBC patients (38).
The role of both functionally distinct macrophage subpopulations M1 and M2 has been reported. M1 macrophages exhibit antitumoral activity by activating natural killer (NK) cells and Th1 cells (IFN- γ, IL-2, and TNF-alpha producers), which contributes to the activation of CD8+ T cells (39). In contrast, M2 macrophages or tumor-associated macrophages (TAMs) favor tumor growth and progression by facilitating tumor invasion and angiogenesis, thus being associated with a poor prognosis in patients with TNBC (40, 41).
Myeloid-derived suppressor cells (MDSCs) are a heterogeneous group of cells with immunosuppressive activity, composed mainly of granulocytes and monocytes. The MDSCs have been associated with tumoral progression through the production of immunosuppressive and pro-angiogenic cytokines that inhibit the immune response of antitumor T cells (42, 43). It should be noted that the role of MDSCs specifically in TNBC patients remains relatively unexplored (44, 45).
NK cells recognize and delete tumor cells lacking MHC-1 expression on their cell surface, whose expression is necessary for the activation of CD8 + T cells (46). Recent studies have shown that NK cells are associated with a better prognosis in the early stages of TNBC (47). More studies are needed.
TILS as prognostic and predictive biomarker in triple-negative breast cancer
In the last few years, the predictive and prognostic role of TILs in TNBC have been studied. The relations between the composition of TILs subpopulations, clinico-pathological characteristics, and the survival of patients have likewise been explored (Table 1) (29, 62).
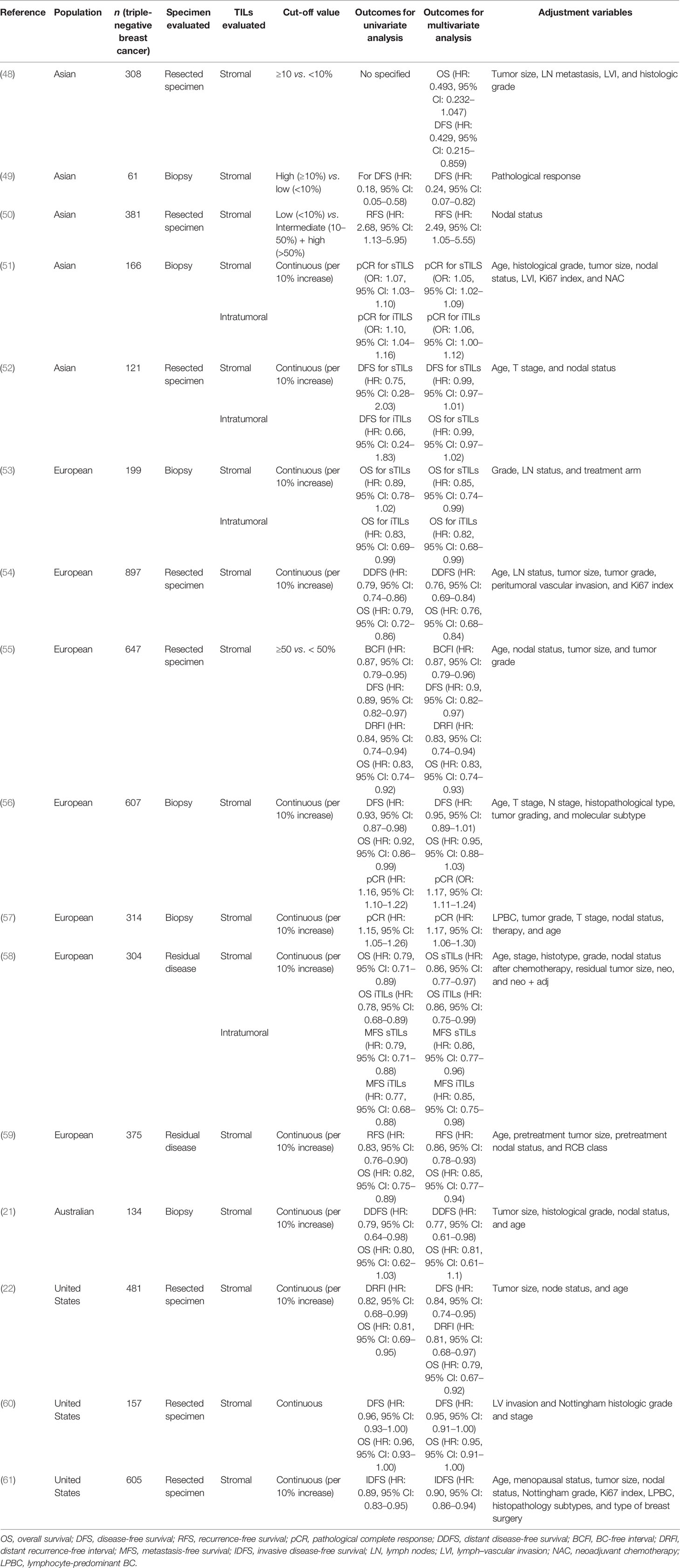
Table 1 Outcomes from studies that analyzed tumor-infiltrating lymphocytes (TILs) according to the region of origin.
Studies carried out in Asian populations mostly showed that TILs, when evaluated in resected specimens, have a positive impact on the prognosis of TNBC (48) (50). Some studies have 95% CI with OS (HR: 0.493, 95% CI: 0.232–1.047, p = 0.066) when patients with high TILs (≥10%) vs. low TILs (<10%) were compared (48). Hida et al. (50) reported a poorer prognosis in TNBC patients with low TIL levels (<10%) compared with intermediate/high-TIL groups (>50%) (HR: 2.68, 95% CI: 1.13–5.95). This association remained significant in the multivariate model (HR: 2.49, 95% CI: 1.05–5.55). Moreover, TILs analyzed at the biopsy, before neoadjuvant chemotherapy, were found to be associated with pCR rate (p = 0.024). Despite previous results, opposite results have also been reported where TILs did not correlate with survival outcomes (52).
When TILs have been evaluated in biopsies, a lower likelihood of recurrence has been observed in patients with a high TIL infiltration (≥10%) compared with those with a low TIL infiltration (<10%) in univariate (HR: 0.18, 95% CI: 0.05–0.58) and multivariate analyses (HR: 0.24, 95% CI: 0.07–0.82). In addition, patients with higher TIL infiltration presented with higher pCR rates (p = 0.013) when compared with patients with low TIL infiltration (49) Similarly, Ruan et al. (51) reported a significant association between the percentage of TILs and pCR in a model adjusted for age, lymph–vascular invasion, and Ki67, both for iTILs (OR: 1.06, 95% CI: 1.00–1.12, p = 0.04, per 10% increase) and for sTILs (OR: 1.05, 95% CI: 1.02–1.09, p = 0.006, per 10% increase). When the optimal thresholds for TILs were analyzed, the results suggested that 20% is a better cutoff to determine high or low sTILs infiltration since it seems to be a better predictor of pCR (OR 2.85, 95% CI: 1.38–5.90, p = 0.005).
The differences in the prognosis impact of TILs between studies might be related to the clinical stage of the patients included. Presumably, there are lower amounts of tumor antigens among patients at earlier stages (31, 52), which could lead to misinterpretations regarding the relationship of TILs and clinico-pathological variables and outcomes of interest, as few studies have assessed the prognosis impact of TILs in early-stage TNBC patients.
Studies in a European population show similar findings to those in the Asian population. A study in France that evaluated TILs in the primary tumor reported a 15% reduction in the risk of death for every 10% of increase in sTIL levels (HR: 0.85, 95% CI: 0.74–0.99) and 18% reduction in the risk of death for every 10% of increase in iTILs (HR: 0.82, 95% CI: 0.68–0.99) in the multivariate analysis adjusted for the grade of lymph nodes (LN) (53).
In Italy, two studies were carried out in a larger number of TNBC patients and analyzed TILs in the resected specimen (54, 55). The first study included 897 women and reported TILs as an independent prognostic factor for a longer distant disease-free survival (HR: 0.76, 95% CI: 0.69–0.84, for every 10% increase in TILs) and longer OS (HR: 0.76, 95% CI: 0.68–0.84, for every 10% increase in TILs) in a model adjusted for age at diagnosis, lymph node stage, peritumoral vascular invasion, tumor size and grade, and Ki67 (54). The second study that evaluated sTILs in the resected specimen and dichotomized patients in having TILs ≥50% vs. patients with TILs <50% likewise found a 13% risk reduction in BC-free interval (HR: 0.87, 95% CI: 0.79–0.96, p = 0.006), 10% risk reduction for DFS (HR: 0.9, 95% CI: 0.82–0.97, p = 0.01), 17% for distance recurrence-free interval (HR: 0.83; 95% CI 0.74–0.94, p = 0.004) in a model adjusted for age, nodal status, tumor size, and tumor grade (55). A study carried out in France and Italy reported that the high presence of TILs in the residual disease after neoadjuvant treatment had a positive impact on MFS (sTIL: HR = 0.86, 95% CI: 0.77–0.96, p = 0.01; iTILs: HR: 0.85, 95% CI: 0.75–0.98, p = 0.02, per 10% increase in TILs) and longer OS (sTIL: HR: 0.86, 95% CI: 0.77–0.97, p = 0.01; iTILs: HR: 0.86, 95% CI: 0.75–0.99, p = 0.03, per 10% increase in TILs). The 5-year OS rate was 91% (95% CI, 68 to 97%) for patients with higher TILs in residual disease compared with 55% (95% CI, 48 to 61%) for patients with low TIL levels (58). Similarly, Luen et al. (59) found that a higher percentage of TILs in residual disease was associated with a longer recurrence-free survival (RFS) (HR: 0.86, 95% CI: 0.78–0.93, per 10% increase in TILs) and a longer OS (HR: 0.85, 95% CI: 0.77–0.94, per 10% increase in TILs).
Denkert et al. (56) also reported in a model adjusted for clinical parameters that patients with high TIL levels in the biopsy have longer DFS (HR: 0.93, 95% CI: 0.87–0.98, p = 0.011) and longer OS (HR: 0.92, 95% CI: 0.86–0.99, p = 0.032). However, when pCR was included in the multivariate analysis for both outcomes, the TILs were no longer significantly associated (HR: 0.95, 95% CI: 0.89–1.01, p = 0.11 for DFS, HR: 0.95, 95% CI: 0.88–1.03, p = 0.24 for OS). They also analyzed if TILs are predictors for pCR in TNBC and found a positive association for sTILs (OR: 1.17, 95% CI: 1.11–1.24, per 10% increase in sTILs). Similar results were reported by the same authors in a different study (57). A different effect of TILs according to chemotherapy regimen has been observed. TILs conferred the greatest survival benefit in patients treated with cyclophosphamide, methotrexate, and 5-fluorouracil + cyclophosphamide doxorubicin regimen (HR: 0.60, 95% CI: 0, 48 to 0.76) (54). More studies are needed to explore differences in the prognosis value of TILs according to the chemotherapy regimen.
The relationship between higher TIL levels and higher pCR rates could be explained by the degree of antitumor immune response by TILs against cancer cells that act synergistically with the natural-immunity-restoring antitumor response (20, 22). In addition, it has been demonstrated that chemotherapy treatment can promote the antitumor immune response due to the production of danger signals—danger-associated molecular patterns—during cell death. The expression of calreticulin (CALR) and release box 1 of the high mobility group (HMGB1) also boosts this antitumor immune response (63). All these could be together related to the presence of TILs in residual disease (58), and thus a good prognosis was reported for TILs in residual disease (64).
In the Australian population, an analysis that included early-stage TNBC patients showed that for every 10% increase in the presence of TILs in the primary tissue, there was a 13% decrease in the risk of distant relapse (HR: 0.77, 95% CI: 0.61 –0.98, p = 0.02) in a model adjusted for clinico-pathological characteristics. No statistically significant differences were observed for OS (21).
In the United States, Adams and colleagues (22) reported that for every 10% increase in sTILs evaluated in surgical specimens, there was a 16% reduction in the risk of recurrence (HR: 0.84, 95% CI: 0.74–0.95, p = 0.005) and a 21% reduction in the risk of death (HR: 0.79, 95% CI: 0.67–0.92). In the same direction, Krishnamurti and colleagues (60) showed that higher peripheral TILs were associated with a better survival (HR: 0.95, 95% CI: 0.91–1.00, p = 0.0354) and less chance of recurrence (HR: 0.95, 95% CI: 0.91–1.00, p = 0.0314). Leon-Ferre et al. (61) reported a similar association between sTILs and invasive disease-free survival in patients with TNBC diagnosed at early stages (HR: 0.90, 95% CI: 0.86–0.94, per 10% increment in TILs).
The case-only study that includes 86 Peruvian women with TNBC observed a statistically significant association between TIL density and a higher tumor grade (p = 0.006), but no significant association was found regarding the relationship between sTILs and survival (65). More studies are needed in the Latino population.
The subpopulation of TILS and its prognostic value
Due to the relevance of TILs in TNBC, in recent years, an attempt has been made to elucidate the role of the different TIL subpopulations, in particular, the most recurrent ones such as CD8, CD4, and FOXP3 (Table 2).
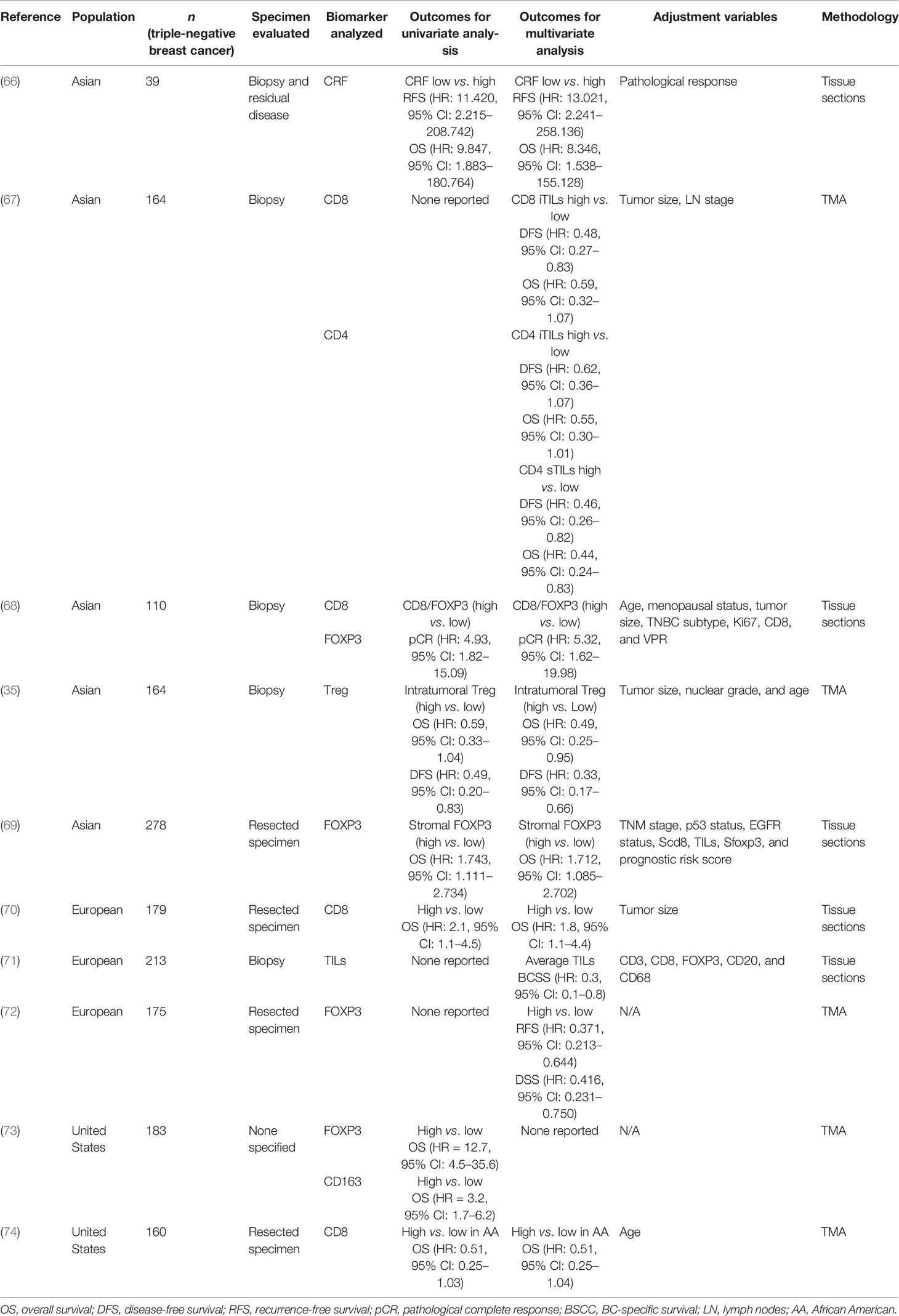
Table 2 Outcomes from studies that analyzed tumor-infiltrating lymphocytes (TIL) subpopulations according to the region of origin.
A study conducted in the Asian population in which the number of TILs CD8+ and TILs FOXP3+ was analyzed in biopsy and residual tissue reported that a high rate of change in the CD8+/FOXP3+ ratio was an independent prognostic factor for recurrence and survival (66). In a different study, high levels of iTILs CD8+ were associated with DFS (HR: 0.48, 95% CI: 0.27–0.83) but not with OS (HR: 0.59, 95% CI: 0.32–1.07). On the other hand, patients with higher levels of sTILs CD4+ presented longer DFS (HR: 0.46, 95% CI: 0.26–0.82) and OS (HR: 0.44, 95% CI: 0.24–0,83) (67). Regarding clinico-pathological variables, a correlation between the immune infiltrate and age at diagnosis has also been reported. The highest rates of the CD8+/FOXP3+ ratio were observed more frequently in women diagnosed at an early age (p = 0.003), specifically when they are still in a premenopausal state (p = 0.002) (68). Moreover, a high CD8+/FOXP3+ ratio was found as a strong predictor of pCR (OR: 5.32, 95% CI: 1.62 to 19.98) (68).
Studies in less common subpopulations, such as B-cell (CD20+) and Tregs (FOXP3+/CD3+), have found them positively associated to better outcomes. A Kaplan–Meier analysis showed that patients with higher intratumoral Treg presented longer DFS (p = 0.001). A multivariate analysis confirmed this association (HR: 0.33, 95% CI: 0.165 to 0.659). High intratumoral Treg infiltration was also found to be associated with OS (HR: 0.49, 95% CI: 0.25–0.95). Additionally, patients with higher CD20+ B-cell infiltration in both the intratumoral (DFS: p = 0.015; OS: p = 0.020) and stromal (DFS: p = 0.012; OS: p = 0.031) compartments presented better clinical outcomes (35). Tian and colleagues (69), in a Chinese study, categorized patients according to the DFS times and reported that patients in the DFS ≥5 years group had higher NK cell stromal infiltration (p < 0.001) and low stromal TAM infiltration (p = 0.004). Stromal FOXP3+ TILs were found as an independent prognostic factor for OS (sTILs FOXP3+ low/high HR: 1.712, 95% CI: 1.085–2.702) (69).
Regarding the studies in a European population, it was observed that patients with low TIL CD8+ infiltration were associated with a higher risk of death from BC (HR: 2.2, 95% CI: 1.0–3.8) (70). On the contrary, Althobiti and colleagues (71) only found TILs as an independent predictor of good prognosis in a model that included various immune cells, such as CD3, CD8, FOXP3, CD20, and CD68. West and colleagues (72) reported that a high infiltration of TILs FOXP3+ was strongly associated with better outcomes (RFS: HR = 0.371, 95% CI: 0.213–0.644; p = 0.0004) and disease-specific survival (HR = 0.416, 95%: CI 0.231–0.750; p = 0.0036). In contrast, a study from the United States reported that a high expression of FOXP3 and CD163 was associated to a worse OS (HR = 12.7, 95% CI: 4.5–35.6 and HR = 3.2, 95% CI: 1.7–6.2, respectively) (73).
Few studies have analyzed the differences in the tumor microenvironment between European American (EA) women and African American (AA) women, and the results have been contradictory. Preliminary data from Wright and colleagues (75) found higher levels of TILs in early-stage (I–II) tumors from AA patients compared with EA (p = 0.019), but this difference was not observed for late-stage (III–IV) tumors. TILs also correlated negatively with AR expression and positively with PD-L1 expression. The analysis of CD8+ T cell infiltration in AA and EA women revealed that AAs with high CD8 infiltration have a trend towards better survival compared with AA with low CD8 infiltration (HR: 0.51, 95% CI: 0.25–1.04) (74). On the other hand, a study that analyzed The Cancer Genome Atlas database and compared the immune gene expression between AA and EA women did not find large-scale immunogenic differences (76).
TILs have a useful prognostic role in TNBC based on TIL populations. Nevertheless, the immune infiltrate phenotype and its prognostic value require better understanding. Thus, it is necessary to include other immune cell populations in future studies. The association reported between the high Treg FOXP3 infiltrate and better DFS and OS in TNBC is interesting considering that Treg has been associated with a poor prognosis as it can suppress antigen-presenting cells and other immune cells, events that are regulated through the secretion of inhibitory cytokines, granzyme B, and perforin (77). On the contrary, the favorable prognosis may be explained by the positive correlation between FOXP3 infiltration and TILs CD8+ infiltration (68). There is a need to clarify the prognostic role of Treg FOXP3+ in TNBC tumors.
Expression of membrane markers in the immune infiltrate
In addition to the different immune cell’s populations mentioned before, there are other biomarkers of special interest, such as the expression of PD-L1. Studies in different populations have consistently showed a correlation between a high expression of PD-L1 in tumor cells and higher levels of sTILs (78–80).
Regarding the impact of PD-L1 in a patient’s prognosis, controversial results have been published. A study from Japan found PD-L1 positive/TILs low expression as an independent negative prognostic factor for RFS (HR = 4.7, 95% CI: 1.6–12.7) and OS (HR = 8.4, 95% CI: 2.3–30.3) (79). AiErken and colleagues (80) conducted a study that included Chinese patients diagnosed with TNBC and reported a positive PD-L1 expression as an independent prognosis factor for OS (HR: 0.302, 95% CI: 0.127–0.721) and DFS (HR: 0.451, 95% CI: 0.211–0.963). A study from the United States reported that elevated levels of PD-L1 were associated with decreased OS compared with a low expression (HR: 10.4, 95% CI: 3.6–29.6) (73). On the contrary, Li and colleagues found that any stromal PD-L1 expression was associated with better DFS but not OS (81).
The association between the expression of PD-L1 and a high percentage of TILs could be explained by activated T cells, which produce IFNγ (82). It has been proposed that IFNγ induce PD-L1 expression as an immune evasion mechanism by the tumor (83). Additionally, the relationship of high TIL levels and PD-L1 expression could also explain the association between PD-L1 expression and DFS and OS in Asian populations (83) and the pCR rates in European populations (80).
Cerbelli et al. (78) analyzed 54 TNBC biopsies taken from different institutions in Rome, Italy, and found a statistically significant association between PD-L1 expression in ≥25% of neoplastic cells and pCR (OR: 1.13, 95% CI: 1.01–1.27). Additionally, it was observed that 100% of the patients who achieved a pCR presented jointly a higher percentage of TILs and PD-L1 expression in ≥25% of tumor cells (p = 0.011). These results suggest that PD-L1 expression could be a marker of response to neoadjuvant chemotherapy in patients with TNBC. However, to reach this conclusion, more and larger studies that focus on the expression of PD-L1 in TNBC patients treated or not with neoadjuvant chemotherapy are needed—for instance, PD-L1 is described to be more commonly expressed in primary tumors than metastatic tumors (p = 0.002) (84), although some controversial results have also been published (85).
TIM3 is an immune checkpoint molecule that is expressed on CD4+ helper 1 (Th1) cells, CD8+ T cells, dendritic cells, and other subpopulations of lymphocytes, macrophages, and monocytes (86). The high expression of PD-1 and PD-L1 was each associated with a high expression of TIM-3 (p = 0.0001 and p = 0.0019, respectively). Patients with a higher TIM-3 expression presented better DFS (HR: 0.1072, 95% CI: 0.0319–0.3603) and longer OS (HR: 0.1129, 95% CI: 0.0323–0.3948) (86).
Interestingly, a German study analyzed the expression levels of 12 immune genes that included T cells, B cells, cytokines, and immune checkpoints markers (CXCL9, CCL5, CD8A, CD80, CXCL13, IGKC, CD21, IDO1, PD-1, PD -L1, CTLA4, and FOXP3). Based on their gene expression, they categorized the patients in three immune groups: low expression (A), intermediate expression (B), and high expression (C). They observed differences in the pCR rates among the three groups: 24% for A, 37.4% for B, and 50.4% for C (p <0.001). All 12 immune genes at the mRNA level were significantly linked to pCR; the best predictors were PD-L1 (OR: 1.44, 95% CI: 1.18 to 1.77, per ΔCt) and CD80 (OR: 1.74, 95% CI: 1.28 to 2.38, per ΔCt) (57).
Conclusions
Although it is not doubted that TILs play an essential role in tumor development, the methods used across studies to measure the infiltrate are heterogeneous (87)—for example, it has been recommended to consider as high an infiltration value >50% (88) or a cutoff point >60% (89) or even to consider three cutoff points (<10%, between 10 and 50%, and >50%) (90). Moreover, studies differ in their sample sizes and inclusion criteria. Some studies evaluate TILs in biopsies and others in the resected specimens of patients that received neoadjuvant chemotherapy or not. Other studies included only early-stage patients. Therefore, all these circumstances make it difficult to provide an assertive comparison between studies to conclude on the role of TILs in carcinogenesis.
The classification of triple-negative breast cancer by immunohistochemical techniques could also be a source of heterogeneity. As mentioned above, some studies included biopsies and others resected specimens. The heterogeneity in the expression of immunohistochemistry markers such as ER, PR, and HER2, when evaluated in core needle biopsies or in a resected specimen, could lead to the misclassification of breast cancer into intrinsic subtypes (91–93). We cannot rule out that there may be misclassified cases among studies and that this may explain, in part, why some studies did not find statistically significant differences in some of the outcomes evaluated. It is also important to consider if TILs were evaluated in resected specimens from patients who previously received neoadjuvant chemotherapy since it is well known that chemotherapy can modify the panorama of the immune infiltrate, and this could impact the results of TIL characterization (94–96).
Germline BRCA1/2 mutations range between 9 and 21% in unselected TNBC patients (97, 98). The presence of mutations in repair genes could lead to a greater formation of neoantigens, which would translate into an increase in immune infiltrate in these cases (99–102). For this reason, it is important to analyze the results of the studies considering the germinal component to avoid bias in the results.
In any case, the results presented below on the prognostic and predictive value of TILs in different populations such as Asian, European, Australian, and American present similar risk directions highlighting that TILs might be an independent prognostic factor for recurrence and survival and an independent predictor factor for pCR regardless on the origin of the patients.
Author Contributions
Writing—review of the draft: CH-C, MR, and HG-T. Conception and study design: SS-G, CH-C, and MS-S. Manuscript preparation: CH-C, MR, HG-T, and SS-G. Writing—reviewing and editing: SS-G and MS-S. All authors contributed to the article and approved the submitted version.
Funding
This work was supported by Minciencias (Contrato 838-2018 to SS-G) and the Colombian National Cancer Institute (C-19010300431 to SS-G).
Conflict of Interest
The authors declare that the research was conducted in the absence of any commercial or financial relationships that could be construed as a potential conflict of interest.
Publisher’s Note
All claims expressed in this article are solely those of the authors and do not necessarily represent those of their affiliated organizations, or those of the publisher, the editors and the reviewers. Any product that may be evaluated in this article, or claim that may be made by its manufacturer, is not guaranteed or endorsed by the publisher.
References
1. Turashvili G, Brogi E. Tumor Heterogeneity in Breast Cancer. Front Med (2017) 4:227. doi: 10.3389/fmed.2017.00227
2. Rakha EA, Green AR. Molecular Classification of Breast Cancer: What the Pathologist Needs to Know. Pathology (2017) 49(2):111–119. doi: 10.1016/j.pathol.2016.10.012
3. Sachs N, de Ligt J, Kopper O, Gogola E, Bounova G, Weeber F, et al. A Living Biobank of Breast Cancer Organoids Captures Disease Heterogeneity. Cell (2018) 172(1-2):373–386.e10. doi: 10.1016/j.cell.2017.11.010
4. Barnard ME, Boeke CE, Tamimi RM. Established Breast Cancer Risk Factors and Risk of Intrinsic Tumor Subtypes. Biochim Biophys Acta - Rev Cancer (2015) 1856(1):73–85. doi: 10.1016/j.bbcan.2015.06.002
5. DeSantis CE, Ma J, Gaudet MM, Newman LA, Miller KD, Goding Sauer A, et al. Breast Cancer Statistics, 2019. CA: A Cancer J Clin (2019) 69(6):438–451. doi: 10.3322/caac.21583
6. Siegel RL, Miller KD, Jemal A. Cancer Statistics, 2019. CA: A Cancer J Clin (2019) 69(1):7–34. doi: 10.3322/caac.21551
7. Yip CH, Evans DG, Agarwal G, Buccimazza I, Kwong A, Morant R, et al. Global Disparities in Breast Cancer Genetics Testing, Counselling and Management. World J Surg (2019) 43(5):1264–1270. doi: 10.1007/s00268-018-04897-6
8. Parise CA, Caggiano V. The Influence of Socioeconomic Status on Racial/Ethnic Disparities Among the ER/PR/HER2 Breast Cancer Subtypes. J Cancer Epidemiol (2015) 2015:813456. doi: 10.1155/2015/813456
9. Carvalho FM, Bacchi LM, Pincerato KM, Van de Rijn M, Bacchi CE. Geographic Differences in the Distribution of Molecular Subtypes of Breast Cancer in Brazil. BMC Women’s Health (2014) 14:102. doi: 10.1186/1472-6874-14-102
10. Jiagge E, Jibril AS, Chitale D, Bensenhaver JM, Awuah B, Hoenerhoff M, et al. Comparative Analysis of Breast Cancer Phenotypes in African American, White American, and West Versus East African Patients: Correlation Between African Ancestry and Triple-Negative Breast Cancer. Ann Surg Oncol (2016) 23(12):3843–3849. doi: 10.1245/s10434-016-5420-z
11. Telli ML. Triple-Negative Breast Cancer. In: Badve S., Gökmen-Polar Y (eds) Molecular Pathology of Breast Cancer (2016), Springer, Cham 71–80. doi: 10.1007/978-3-319-41761-5_6
12. Foulkes WD, Smith IE, Reis-Filho JS. Triple-Negative Breast Cancer. N Engl J Med (2010) 363:1938–48. doi: 10.1056/NEJMra1001389
13. López-Ozuna VM, Hachim IY, Hachim MY, Lebrun JJ, Ali S. Prolactin Modulates TNBC Aggressive Phenotype Limiting Tumorigenesis. Endocr Relat Cancer (2019) 26(3):321–337. doi: 10.1530/ERC-18-0523
14. Lehmann BD, Bauer JA, Chen X, Sanders ME, Chakravarthy AB, Shyr Y, et al. Identification of Human Triple-Negative Breast Cancer Subtypes and Preclinical Models for Selection of Targeted Therapies. J Clin Invest (2011) 121(7):2750–67. doi: 10.1172/JCI45014
15. Lehmann BD, Jovanović B, Chen X, Estrada MV, Johnson KN, Shyr Y, et al. Refinement of Triple-Negative Breast Cancer Molecular Subtypes: Implications for Neoadjuvant Chemotherapy Selection. PLoS One (2016) 11:e0157368. doi: 10.1371/journal.pone.0157368
16. Burstein MD, Tsimelzon A, Poage GM, Covington KR, Contreras A, Fuqua SAW, et al. Comprehensive Genomic Analysis Identifies Novel Subtypes and Targets of Triple-Negative Breast Cancer Analysis and Interpretation of Data: HHS Public Access. Clin Cancer Res (2015) 21:1688–98. doi: 10.1158/1078-0432.CCR-14-0432
17. Santonja A, Sánchez-Muñoz A, Lluch A, Chica-Parrado MR, Albanell J, Chacón JI, et al. Triple Negative Breast Cancer Subtypes and Pathologic Complete Response Rate to Neoadjuvant Chemotherapy. Oncotarget (2018) 9(41):26406–26416. doi: 10.18632/oncotarget.25413
18. Gong Y, Ji P, Yang YS, Xie S, Yu TJ, Xiao Y, et al. Metabolic-Pathway-Based Subtyping of Triple-Negative Breast Cancer Reveals Potential Therapeutic Targets. Cell Metab (2021) 33:51–64.e9. doi: 10.1016/J.CMET.2020.10.012
19. Angajala A, Mothershed E, Davis MB, Tripathi S, He Q, Bedi D, et al. Quadruple Negative Breast Cancers (QNBC) Demonstrate Subtype Consistency Among Primary and Recurrent or Metastatic Breast Cancer. Transl Oncol (2019) 12:493–501. doi: 10.1016/j.tranon.2018.11.008
20. Loi S. Tumor-Infiltrating Lymphocytes, Breast Cancer Subtypes and Therapeutic Efficacy. OncoImmunology (2013) 2:e24720. doi: 10.4161/onci.24720
21. Loi S, Michiels S, Salgado R, Sirtaine N, Jose V, Fumagalli D, et al. Tumor Infiltrating Lymphocytes are Prognostic in Triple Negative Breast Cancer and Predictive for Trastuzumab Benefit in Early Breast Cancer: Results From the FinHER Trial. Ann Oncol (2014) 25:1544–50. doi: 10.1093/annonc/mdu112
22. Adams S, Gray RJ, Demaria S, Goldstein L, Perez EA, Shulman LN, et al. Prognostic Value of Tumor-Infiltrating Lymphocytes in Triple-Negative Breast Cancers From Two Phase III Randomized Adjuvant Breast Cancer Trials: ECOG 2197 and ECOG 1199. J Clin Oncol (2014) 32:2959–66. doi: 10.1200/JCO.2013.55.0491
23. Pruneri G, Vingiani A, Denkert C. Tumor Infiltrating Lymphocytes in Early Breast Cancer. Breast (2018) 37:207–214. doi: 10.1016/j.breast.2017.03.010
24. Chen DS, Mellman I. Elements of Cancer Immunity and the Cancer-Immune Set Point. Nature (2017) 541(7637):321–330. doi: 10.1038/nature21349
25. Mao Y, Keller ET, Garfield DH, Shen K, Wang J. Stromal Cells in Tumor Microenvironment and Breast Cancer. Cancer Metastasis Rev (2013) 32(1-2):303–15. doi: 10.1007/s10555-012-9415-3
26. Bahrami A, Hassanian SM, Khazaei M, Hasanzadeh M, Shahidsales S, Maftouh M, et al. The Therapeutic Potential of Targeting Tumor Microenvironment in Breast Cancer: Rational Strategies and Recent Progress. J Cell Biochem (2018) 119(1):111–122. doi: 10.1002/jcb.26183
27. Xie HY, Shao ZM, Li DQ. Tumor Microenvironment: Driving Forces and Potential Therapeutic Targets for Breast Cancer Metastasis. Chin J Cancer (2017) 36(1):36. doi: 10.1186/s40880-017-0202-y
28. Salgado R, Denkert C, Demaria S, Sirtaine N, Klauschen F, Pruneri G, et al. The Evaluation of Tumor-Infiltrating Lymphocytes (TILS) in Breast Cancer: Recommendations by an International TILS Working Group 2014. Ann Oncol (2015) 26(2):259–71. doi: 10.1093/annonc/mdu450
29. Ibrahim EM, Al-Foheidi ME, Al-Mansour MM, Kazkaz GA. The Prognostic Value of Tumor-Infiltrating Lymphocytes in Triple-Negative Breast Cancer: A Meta-Analysis. Breast Cancer Res Treat (2014) 148(3):467–76. doi: 10.1007/s10549-014-3185-2
30. Prizment AE, Vierkant RA, Smyrk TC, Tillmans LS, Nelson HH, Lynch CF, et al. Cytotoxic T Cells and Granzyme B Associated With Improved Colorectal Cancer Survival in a Prospective Cohort of Older Women. Cancer Epidemiol Biomarkers Prev (2017) 26(4):622–631. doi: 10.1158/1055-9965.EPI-16-0641
31. Savas P, Salgado R, Denkert C, Sotiriou C, Darcy PK, Smyth MJ, et al. Clinical Relevance of Host Immunity in Breast Cancer: From TILs to the Clinic. Nat Rev Clin Oncol (2016) 13(4):228–41. doi: 10.1038/nrclinonc.2015.215
32. Zander R, Schauder D, Xin G, Nguyen C, Wu X, Zajac A, et al. CD4+ T Cell Help Is Required for the Formation of a Cytolytic CD8+ T Cell Subset That Protects Against Chronic Infection and Cancer. Immunity (2019) 51(6):1028–1042.e4. doi: 10.1016/j.immuni.2019.10.009
33. Ma C, Zhang Q, Ye J, Wang F, Zhang Y, Wevers E, et al. Tumor-Infiltrating γδ T Lymphocytes Predict Clinical Outcome in Human Breast Cancer. J Immunol (2012) 189(10):5029–36. doi: 10.4049/jimmunol.1201892
34. Joshi NS, Akama-Garren EH, Lu Y, Lee DY, Chang GP, Li A, et al. Regulatory T Cells in Tumor-Associated Tertiary Lymphoid Structures Suppress Anti-Tumor T Cell Responses. Immunity (2015) 43(3):579–90. doi: 10.1016/j.immuni.2015.08.006
35. Yeong J, Thike AA, Lim JCT, Lee B, Li H, Wong SC, et al. Higher Densities of Foxp3+ Regulatory T Cells are Associated With Better Prognosis in Triple-Negative Breast Cancer. Breast Cancer Res Treat (2017) 163:21–35. doi: 10.1007/s10549-017-4161-4
36. Lee S, Cho EY, Park YH, Ahn JS, Im YH. Prognostic Impact of FOXP3 Expression in Triple-Negative Breast Cancer. Acta Oncol (2013) 52(1):73–81. doi: 10.3109/0284186X.2012.731520
37. Mahmoud SMA, Lee AHS, Paish EC, MacMillan RD, Ellis IO, Green AR. The Prognostic Significance of B Lymphocytes in Invasive Carcinoma of the Breast. Breast Cancer Res Treat (2012) 132(2):545–53. doi: 10.1007/s10549-011-1620-1
38. Iglesia MD, Vincent BG, Parker JS, Hoadley KA, Carey LA, Perou CM, et al. Prognostic B-Cell Signatures Using mRNA-Seq in Patients With Subtype-Specific Breast and Ovarian Cancer. Clin Cancer Res (2014) 20 (14):3818–3829. doi: 10.1158/1078-0432.CCR-13-3368
39. Yang M, Ma B, Shao H, Clark AM, Wells A. Macrophage Phenotypic Subtypes Diametrically Regulate Epithelial-Mesenchymal Plasticity in Breast Cancer Cells. BMC Cancer (2016) 16:419. doi: 10.1186/s12885-016-2411-1
40. Zhang W-J, Wang X-H, Gao S-T, Cheng C, Xu X-Y, Sun Q, et al. Tumor-Associated Macrophages Correlate With Phenomenon of Epithelial-Mesenchymal Transition and Contribute to Poor Prognosis in Triple-Negative Breast Cancer Patients. J Surg Res (2018) 222:93–101. doi: 10.1016/j.jss.2017.09.035
41. Zhao X, Qu J, Sun Y, Wang J, Liu X, Wang F, et al. Prognostic Significance of Tumor-Associated Macrophages in Breast Cancer: A Meta-Analysis of the Literature. Oncotarget (2017) 8(18):30576–30586. doi: 10.18632/oncotarget.15736
42. Bergenfelz C, Roxå A, Mehmeti M, Leandersson K, Larsson AM. Clinical Relevance of Systemic Monocytic-MDSCs in Patients With Metastatic Breast Cancer. Cancer Immunol Immunother (2020) 69(3):435–448. doi: 10.1007/s00262-019-02472-z
43. Bergenfelz C, Larsson AM, Von Stedingk K, Gruvberger-Saal S, Aaltonen K, Jansson S, et al. Systemic Monocytic-MDSCs are Generated From Monocytes and Correlate With Disease Progression in Breast Cancer Patients. PLoS One (2015) 10(5):e0127028. doi: 10.1371/journal.pone.0127028
44. Gabrilovich DI. Myeloid-Derived Suppressor Cells. Cancer Immunol Res (2017) 5:3–8. doi: 10.1158/2326-6066.CIR-16-0297
45. Dysthe M, Parihar R. Myeloid-Derived Suppressor Cells in the Tumor Microenvironment. Adv Exp Med Biol (2020) 1224:117–40. doi: 10.1007/978-3-030-35723-8_8
46. Mamessier E, Bertucci F, Sabatier R, Birnbaum D, Olive D. “Stealth” Tumors: Breast Cancer Cells Shun NK-Cells Anti-Tumor Immunity. OncoImmunology (2012) 1(3):366–368. doi: 10.4161/onci.18528
47. Stovgaard ES, Nielsen D, Hogdall E, Balslev E. Triple Negative Breast Cancer–Prognostic Role of Immune-Related Factors: A Systematic Review. Acta Oncol (2018) 57(1):74–82. doi: 10.1080/0284186X.2017.1400180
48. Jang N, Kwon HJ, Park MH, Kang SH, Bae YK. Prognostic Value of Tumor-Infiltrating Lymphocyte Density Assessed Using a Standardized Method Based on Molecular Subtypes and Adjuvant Chemotherapy in Invasive Breast Cancer. Ann Surg Oncol (2018) 25:937–46. doi: 10.1245/s10434-017-6332-2
49. Asano Y, Kashiwagi S, Goto W, Takada K, Takahashi K, Hatano T, et al. Prediction of Treatment Response to Neoadjuvant Chemotherapy in Breast Cancer by Subtype Using Tumor-Infiltrating Lymphocytes. Anticancer Res (2018) 38:2311–21. doi: 10.21873/anticanres.12476
50. Hida AI, Sagara Y, Yotsumoto D, Kanemitsu S, Kawano J, Baba S, et al. Prognostic and Predictive Impacts of Tumor-Infiltrating Lymphocytes Differ Between Triple-Negative and HER2-Positive Breast Cancers Treated With Standard Systemic Therapies. Breast Cancer Res Treat (2016) 158:1–9. doi: 10.1007/s10549-016-3848-2
51. Ruan M, Tian T, Rao J, Xu X, Yu B, Yang W, et al. Predictive Value of Tumor-Infiltrating Lymphocytes to Pathological Complete Response in Neoadjuvant Treated Triple-Negative Breast Cancers. Diagn Pathol (2018) 13(1):66. doi: 10.1186/s13000-018-0743-7
52. Park HS, Heo I, Kim JY, Kim S, Nam S, Park S, et al. No Effect of Tumor-Infiltrating Lymphocytes (TILs) on Prognosis in Patients With Early Triple-Negative Breast Cancer: Validation of Recommendations by the International TILs Working Group 2014. J Surg Oncol (2016) 114:17–21. doi: 10.1002/jso.24275
53. Dieci MV, Mathieu MC, Guarneri V, Conte P, Delaloge S, Andre F, et al. Prognostic and Predictive Value of Tumor-Infiltrating Lymphocytes in Two Phase III Randomized Adjuvant Breast Cancer Trials. Ann Oncol (2015) 26:1698–704. doi: 10.1093/annonc/mdv239
54. Pruneri G, Vingiani A, Bagnardi V, Rotmensz N, de Rose A, Palazzo A, et al. Clinical Validity of Tumor-Infiltrating Lymphocytes Analysis in Patients With Triple-Negative Breast Cancer. Ann Oncol (2016) 27:249–56. doi: 10.1093/annonc/mdv571
55. Pruneri G, Gray KP, Vingiani A, Viale G, Curigliano G, Criscitiello C, et al. Tumor-Infiltrating Lymphocytes (TILs) are a Powerful Prognostic Marker in Patients With Triple-Negative Breast Cancer Enrolled in the IBCSG Phase III Randomized Clinical Trial 22-00. Breast Cancer Res Treat (2016) 158:323–31. doi: 10.1007/s10549-016-3863-3
56. Denkert C, von Minckwitz G, Darb-Esfahani S, Lederer B, Heppner BI, Weber KE, et al. Tumour-Infiltrating Lymphocytes and Prognosis in Different Subtypes of Breast Cancer: A Pooled Analysis of 3771 Patients Treated With Neoadjuvant Therapy. Lancet Oncol (2018) 19:40–50. doi: 10.1016/S1470-2045(17)30904-X
57. Denkert C, Von Minckwitz G, Brase JC, Sinn BV, Gade S, Kronenwett R, et al. Tumor-Infiltrating Lymphocytes and Response to Neoadjuvant Chemotherapy With or Without Carboplatin in Human Epidermal Growth Factor Receptor 2-Positive and Triple-Negative Primary Breast Cancers. J Clin Oncol (2015) 33:983–91. doi: 10.1200/JCO.2014.58.1967
58. Dieci MV, Criscitiello C, Goubar A, Viale G, Conte P, Guarneri V, et al. Prognostic Value of Tumor-Infiltrating Lymphocytes on Residual Disease After Primary Chemotherapy for Triple-Negative Breast Cancer: A Retrospective Multicenter Study. Ann Oncol (2014) 25:611–8. doi: 10.1093/annonc/mdt556
59. Luen SJ, Salgado R, Dieci MV, Vingiani A, Curigliano G, Gould RE, et al. Prognostic Implications of Residual Disease Tumor-Infiltrating Lymphocytes and Residual Cancer Burden in Triple-Negative Breast Cancer Patients After Neoadjuvant Chemotherapy. Ann Oncol (2019) 30:236–42. doi: 10.1093/annonc/mdy547
60. Krishnamurti U, Wetherilt CS, Yang J, Peng L, Li X. Tumor-Infiltrating Lymphocytes are Significantly Associated With Better Overall Survival and Disease-Free Survival in Triple-Negative But Not Estrogen Receptor–Positive Breast Cancers. Hum Pathol (2017) 64:7–12. doi: 10.1016/j.humpath.2017.01.004
61. Leon-Ferre RA, Polley MY, Liu H, Gilbert JA, Cafourek V, Hillman DW, et al. Impact of Histopathology, Tumor-Infiltrating Lymphocytes, and Adjuvant Chemotherapy on Prognosis of Triple-Negative Breast Cancer. Breast Cancer Res Treat (2018) 167:89–99. doi: 10.1007/s10549-017-4499-7
62. Castaneda CA, Mittendorf E, Casavilca S, Wu Y, Castillo M, Arboleda P, et al. Tumor Infiltrating Lymphocytes in Triple Negative Breast Cancer Receiving Neoadjuvant Chemotherapy. World J Clin Oncol (2016) 7(5):387–394. doi: 10.5306/wjco.v7.i5.387
63. Galluzzi L, Buqué A, Kepp O, Zitvogel L, Kroemer G. Immunogenic Cell Death in Cancer and Infectious Disease. Nat Rev Immunol 2016 17:2 (2016) 17:97–111. doi: 10.1038/nri.2016.107
64. Ladoire S, Mignot G, Dabakuyo S, Arnould L, Apetoh L, Rébé C, et al. In Situ Immune Response After Neoadjuvant Chemotherapy for Breast Cancer Predicts Survival. J Pathol (2011) 224:389–400. doi: 10.1002/PATH.2866
65. Galvez M, Castaneda CA, Sanchez J, Castillo M, Rebaza LP, Calderon G, et al. Clinicopathological Predictors of Long-Term Benefit in Breast Cancer Treated With Neoadjuvant Chemotherapy. World J Clin Oncol (2018) 9(2):33–41. doi: 10.5306/wjco.v9.i2.33
66. Goto W, Kashiwagi S, Asano Y, Takada K, Takahashi K, Hatano T, et al. Predictive Value of Improvement in the Immune Tumour Microenvironment in Patients With Breast Cancer Treated With Neoadjuvant Chemotherapy. ESMO Open (2018) 3(6):e000305. doi: 10.1136/esmoopen-2017-000305
67. Matsumoto H, Thike AA, Li H, Yeong J, Koo SL, Dent RA, et al. Increased CD4 and CD8-Positive T Cell Infiltrate Signifies Good Prognosis in a Subset of Triple-Negative Breast Cancer. Breast Cancer Res Treat (2016) 156:237–47. doi: 10.1007/s10549-016-3743-x
68. Miyashita M, Sasano H, Tamaki K, Chan M, Hirakawa H, Suzuki A, et al. Tumor-Infiltrating CD8+ and FOXP3+ Lymphocytes in Triple-Negative Breast Cancer: Its Correlation With Pathological Complete Response to Neoadjuvant Chemotherapy. Breast Cancer Res Treat (2014) 148:525–34. doi: 10.1007/s10549-014-3197-y
69. Tian W, Wang L, Yuan L, Duan W, Zhao W, Wang S, et al. A Prognostic Risk Model for Patients With Triple Negative Breast Cancer Based on Stromal Natural Killer Cells, Tumor-Associated Macrophages and Growth-Arrest Specific Protein 6. Cancer Sci (2016) 107:882–9. doi: 10.1111/cas.12964
70. Vihervuori H, Autere TA, Kurki S, Kallio L, Lintunen MM, Talvinen K, et al. Tumor-Infiltrating Lymphocytes and CD8+ T Cells Predict Survival of Triple-Negative Breast Cancer. J Cancer Res Clin Oncol (2019) 145(12):3105–3114. doi: 10.1007/s00432-019-03036-5
71. Althobiti M, Aleskandarany MA, Joseph C, Toss M, Mongan N, Diez-Rodriguez M, et al. Heterogeneity of Tumour-Infiltrating Lymphocytes in Breast Cancer and its Prognostic Significance. Histopathology (2018) 73:887–96. doi: 10.1111/his.13695
72. West N, Kost S, Martin S, Milne K, Deleeuw R, Nelson B. Tumour-Infiltrating FOXP3(+) Lymphocytes are Associated With Cytotoxic Immune Responses and Good Clinical Outcome in Oestrogen Receptor-Negative Breast Cancer. Br J Cancer (2013) 108:155–62. doi: 10.1038/bjc.2012.524
73. Adams TA, Vail PJ, Ruiz A, Mollaee M, McCue PA, Knudsen ES, et al. Composite Analysis of Immunological and Metabolic Markers Defines Novel Subtypes of Triple Negative Breast Cancer. Modern Pathol (2017) 31:288–98. doi: 10.1038/modpathol.2017.126
74. Abdou Y, Attwood K, Cheng TYD, Yao S, Bandera EV, Zirpoli GR, et al. Racial Differences in CD8+ T Cell Infiltration in Breast Tumors From Black and White Women. Breast Cancer Research : BCR (2020) 22(1):62. doi: 10.1186/S13058-020-01297-4
75. Wright N, Lee C, Guanhao W, Krishnamurti U, Li X, Rida PCG, et al. Abstract PR06: Differences in Tumor-Infiltrating Lymphocytes Between Racially Distinct Triple-Negative Breast Tumors. Cancer Epidemiol Prev Biomarkers (2018) 27(7 Suppl). doi: 10.1158/1538-7755.DISP17-PR06
76. O’Meara T, Safonov A, Casadevall D, Qing T, Silber A, Killelea B, et al. Immune Microenvironment of Triple-Negative Breast Cancer in African-American and Caucasian Women. Breast Cancer Res Treat (2019) 175(1):247–259. doi: 10.1007/s10549-019-05156-5
77. Takeuchi Y, Nishikawa H. Roles of Regulatory T Cells in Cancer Immunity. Int Immunol (2016) 28:401–9. doi: 10.1093/INTIMM/DXW025
78. Cerbelli B, Pernazza A, Botticelli A, Fortunato L, Monti M, Sciattella P, et al. PD-L1 Expression in TNBC: A Predictive Biomarker of Response to Neoadjuvant Chemotherapy? BioMed Res Int (2017) 2017:1750925. doi: 10.1155/2017/1750925
79. Mori H, Kubo M, Yamaguchi R, Nishimura R, Osako T, Arima N, et al. The Combination of PD-L1 Expression and Decreased Tumorinfiltrating Lymphocytes is Associated With a Poor Prognosis in Triple-Negative Breast Cancer. Oncotarget (2017) 8(9):15584–15592. doi: 10.18632/oncotarget.14698
80. AiErken NJ, Shi HJ, Zhou Y, Shao N, Zhang J, Shi Y, et al. High PD-L1 Expression is Closely Associated With Tumor-Infiltrating Lymphocytes and Leads to Good Clinical Outcomes in Chinese Triple Negative Breast Cancer Patients. Int J Biol Sci (2017) 13:1172–9. doi: 10.7150/ijbs.20868
81. Li X, Wetherilt CS, Krishnamurti U, Yang J, Ma Y, Styblo TM, et al. Stromal PD-L1 Expression is Associated With Better Disease-Free Survival in Triple-Negative Breast Cancer. Am J Clin Pathol (2016) 146(4):496–502. doi: 10.1093/ajcp/aqw134
82. Street D, Kaufmann AM, Vaughan A, Fisher SG, Hunter M, Schreckenberger C, et al. Interferon-Gamma Enhances Susceptibility of Cervical Cancer Cells to Lysis by Tumor-Specific Cytotoxic T Cells. Gynecol Oncol (1997) 65:265–72. doi: 10.1006/gyno.1997.4667
83. Mandai M, Hamanishi J, Abiko K, Matsumura N, Baba T, Konishi I. Dual Faces of Ifnγ in Cancer Progression: A Role of PD-L1 Induction in the Determination of Pro- and Antitumor Immunity. Clin Cancer Res (2016) 22:2329–34. doi: 10.1158/1078-0432.CCR-16-0224
84. Hoda RS, Brogi E, dos Anjos CH, Grabenstetter A, Ventura K, Patil S, et al. Clinical and Pathologic Features Associated With PD-L1 (SP142) Expression in Stromal Tumor-Infiltrating Immune Cells of Triple-Negative Breast Carcinoma. Modern Pathol (2020) 33:2221–32. doi: 10.1038/s41379-020-0606-0
85. Boman C, Zerdes I, Mårtensson K, Bergh J, Foukakis T, Valachis A, et al. Discordance of PD-L1 Status Between Primary and Metastatic Breast Cancer: A Systematic Review and Meta-Analysis. Cancer Treat Rev (2021) 99:102257. doi: 10.1016/j.ctrv.2021.102257
86. Byun K, Hwang HJ, Park KJ, Kim MC, Cho SH, Ju MH, et al. T-Cell Immunoglobulin Mucin 3 Expression on Tumor Infiltrating Lymphocytes as a Positive Prognosticator in Triple-Negative Breast Cancer. J Breast Cancer (2018) 21(4):406–414. doi: 10.4048/jbc.2018.21.e61
87. Herrero-Vicent C, Guerrero A, Gavilá J, Gozalbo F, Hernández A, Sandiego S, et al. Predictive and Prognostic Impact of Tumour-Infiltrating Lymphocytes in Triple-Negative Breast Cancer Treated With Neoadjuvant Chemotherapy. Ecancermedicalscience (2017) 11:759. doi: 10.3332/ECANCER.2017.759
88. Loi S, Sirtaine N, Piette F, Salgado R, Viale G, Van Eenoo F, et al. Prognostic and Predictive Value of Tumor-Infiltrating Lymphocytes in a Phase III Randomized Adjuvant Breast Cancer Trial in Node-Positive Breast Cancer Comparing the Addition of Docetaxel to Doxorubicin With Doxorubicin-Based Chemotherapy: BIG 02-98. J Clin Oncol (2013) 31(7):860–7. doi: 10.1200/JCO.2011.41.0902
89. Denkert C, Loibl S, Noske A, Roller M, Müller BM, Komor M, et al. Tumor-Associated Lymphocytes as an Independent Predictor of Response to Neoadjuvant Chemotherapy in Breast Cancer. J Clin Oncol (2010) 28:105–13. doi: 10.1200/JCO.2009.23.7370
90. Ono M, Tsuda H, Shimizu C, Yamamoto S, Shibata T, Yamamoto H, et al. Tumor-Infiltrating Lymphocytes are Correlated With Response to Neoadjuvant Chemotherapy in Triple-Negative Breast Cancer. Breast Cancer Res Treat (2012) 132:793–805. doi: 10.1007/S10549-011-1554-7
91. Rey-Vargas L, Mejía-Henao JC, Sanabria-Salas MC, Serrano-Gomez SJ. Effect of Neoadjuvant Therapy on Breast Cancer Biomarker Profile. BMC Cancer (2020) 20:675. doi: 10.1186/s12885-020-07179-4
92. Parinyanitikul N, Lei X, Chavez-MacGregor M, Liu S, Mittendorf EA, Litton JK, et al. Receptor Status Change From Primary to Residual Breast Cancer After Neoadjuvant Chemotherapy and Analysis of Survival Outcomes. Clin Breast Cancer (2015) 15:153–60. doi: 10.1016/j.clbc.2014.09.006
93. Gahlaut R, Bennett A, Fatayer H, Dall BJ, Sharma N, Velikova G, et al. Effect of Neoadjuvant Chemotherapy on Breast Cancer Phenotype, ER/PR and HER2 Expression – Implications for the Practising Oncologist. Eur J Cancer (2016) 60:40–8. doi: 10.1016/j.ejca.2016.03.006
94. Hamy A-S, Bonsang-Kitzis H, de Croze D, Laas E, Darrigues L, Topciu L, et al. Interaction Between Molecular Subtypes and Stromal Immune Infiltration Before and After Treatment in Breast Cancer Patients Treated With Neoadjuvant Chemotherapy. Clin Cancer Res (2019) 25:6731–41. doi: 10.1158/1078-0432.CCR-18-3017
95. Pelekanou V, Barlow WE, Nahleh ZA, Wasserman B, Lo Y-C, von Wahlde M-K, et al. Tumor-Infiltrating Lymphocytes and PD-L1 Expression in Pre- and Posttreatment Breast Cancers in the SWOG S0800 Phase II Neoadjuvant Chemotherapy Trial. Mol Cancer Ther (2018) 17:1324–31. doi: 10.1158/1535-7163.MCT-17-1005
96. Urueña C, Lasso P, Bernal-Estevez D, Rubio D, Salazar AJ, Olaya M, et al. The Breast Cancer Immune Microenvironment is Modified by Neoadjuvant Chemotherapy. Sci Rep (2022) 12:7981. doi: 10.1038/s41598-022-12108-5
97. Gonzalez-Angulo AM, Timms KM, Liu S, Chen H, Litton JK, Potter J, et al. Incidence and Outcome of BRCA Mutations in Unselected Patients With Triple Receptor-Negative Breast Cancer. Clin Cancer Res (2011) 17:1082–9. doi: 10.1158/1078-0432.CCR-10-2560
98. Mansouri M, Derkaoui T, Bakkach J, Loudiyi A, Ghailani Nourouti N, Barakat A, et al. Screening of BRCA1 and BRCA2 Germline Mutations in Unselected Triple-Negative Breast Cancer Patients: A Series From North of Morocco. Precis Med Sci (2020) 9:43–8. doi: 10.1002/prm2.12009
99. de Boo L, Cimino-Mathews A, Lubeck Y, Daletzakis A, Opdam M, Sanders J, et al. Tumour-Infiltrating Lymphocytes (TILs) and BRCA-Like Status in Stage III Breast Cancer Patients Randomised to Adjuvant Intensified Platinum-Based Chemotherapy Versus Conventional Chemotherapy. Eur J Cancer (2020) 127:240–50. doi: 10.1016/j.ejca.2019.12.003
100. Liu Z, Li M, Jiang Z, Wang X. A Comprehensive Immunologic Portrait of Triple-Negative Breast Cancer. Trans Oncol (2018) 11:311–29. doi: 10.1016/j.tranon.2018.01.011
101. Jiang T, Shi W, Wali VB, Pongor LS, Li C, Lau R, et al. Predictors of Chemosensitivity in Triple Negative Breast Cancer: An Integrated Genomic Analysis. PLoS Med (2016) 13:e1002193. doi: 10.1371/journal.pmed.1002193
Keywords: triple-negative breast cancer, tumor-infiltrating lymphocytes, prognosis, predictive, population groups
Citation: Huertas-Caro CA, Ramirez MA, Gonzalez-Torres HJ, Sanabria-Salas MC and Serrano-Gómez SJ (2022) Immune Lymphocyte Infiltrate and its Prognostic Value in Triple-Negative Breast Cancer. Front. Oncol. 12:910976. doi: 10.3389/fonc.2022.910976
Received: 01 April 2022; Accepted: 20 June 2022;
Published: 18 July 2022.
Edited by:
Tomás Pascual Martínez, SOLTI breast cancer research group, SpainReviewed by:
Yong-Qu Zhang, Xiamen University, ChinaAnthony Magliocco, Protean BioDiagnostics Inc., United States
Copyright © 2022 Huertas-Caro, Ramirez, Gonzalez-Torres, Sanabria-Salas and Serrano-Gómez. This is an open-access article distributed under the terms of the Creative Commons Attribution License (CC BY). The use, distribution or reproduction in other forums is permitted, provided the original author(s) and the copyright owner(s) are credited and that the original publication in this journal is cited, in accordance with accepted academic practice. No use, distribution or reproduction is permitted which does not comply with these terms.
*Correspondence: Silvia J. Serrano-Gómez, c3NlcnJhbm9AY2FuY2VyLmdvdi5jbw==