- 1Department of Radiation Oncology, Samsung Medical Center, Sungkyunkwan University School of Medicine, Seoul, Republic of Korea
- 2Division of Cancer Epidemiology and Genetics, National Cancer Institute, National Institutes of Health, Rockville, MD, United States
Background: We estimated the dose of circulating blood cells (CBCs) in patients with locally advanced non-small cell lung cancer for predicting severe radiation-induced lymphopenia (SRIL) and compared pencil-beam scanning proton therapy (PBSPT) and intensity-modulated (photon) radiotherapy (IMRT).
Materials and methods: After reviewing 325 patients who received definitive chemoradiotherapy with PBSPT (n = 37) or IMRT (n = 164). SRIL was diagnosed when two or more events of an absolute lymphocyte count < 200 µL occurred during the treatment course. Dose information for the heart and lungs was utilized for the time-dependent computational dose calculation of CBCs.
Results: The dose distribution of CBCs was significantly lesser in the PBSPT group than that in the IMRT group. Overall, 75 (37.3%) patients experienced SRIL during the treatment course; 72 and 3 patients were treated with IMRT and PBSPT, respectively. SRIL was associated with poor progression-free and overall survival outcomes. Upon incorporating the dose information of CBCs for predicting SRIL, CBC D90% > 2.6 GyE was associated with the development of SRIL with the baseline lymphocyte count and target volume. Furthermore, PBSPT significantly reduced the dose of CBC D90% (odds ratio = 0.11; p = 0.004) compared with IMRT.
Conclusion: The results of this study demonstrate the significance of the dose distribution of CBCs in predicting SRIL. Furthermore, reducing the dose of CBCs after PBSPT minimized the risk of SRIL. Lymphocyte-sparing radiotherapy in PBSPT could improve outcomes, particularly in the setting of maintenance immunotherapy.
1 Introduction
Given the physical characteristics of proton and photon (X-ray) beam therapies, proton beam radiation therapy (RT) has intrigued physicians by improving treatment outcomes in patients with non-small cell lung cancer (NSCLC) (1). However, a randomized controlled trial comparing intensity-modulated (photon) RT (IMRT) and proton beam therapy failed to demonstrate clinical benefit in terms of oncologic outcomes and normal tissue toxicities (2). Furthermore, previous retrospective studies showed a trend but no significant benefit in preventing radiation pneumonitis following proton beam therapy (3–5).
Severe radiation-induced lymphopenia (SRIL), which is significant depletion of lymphocytes due to radiation exposure, has been investigated with the emerging interest in immune responses against tumors (6–8). Its clinical significance has been evaluated in various solid tumors (6, 9–12). In this context, we previously showed that pencil-beam scanning proton therapy (PBSPT), an advanced beam delivery technique in proton beam therapy, decreased the occurrence of SRIL (12). Although the etiology of SRIL is multifaceted, the consensus is lacking for dose constraints, which could lead to SRIL because of the lack of tools to compute the dose to circulating lymphocytes.
We previously developed a time-dependent computational framework called the hematological dose (HEDOS), which estimates the dose to circulating blood cells (CBCs) based on a whole-body blood flow simulation and is used to construct the dose–volume histogram of blood cells (bDVH) (13). It has been applied to selective cases. Xing et al. demonstrated the impact of external beam delivery techniques, including IMRT, volumetric-modulated arc therapy, passive proton beam, and PBSPT for the liver treatment plan without clinical data (14). Qian et al. applied HEDOS to correlate the dose to CBCs for patients with metastatic NSCLC, melanoma, or renal cell carcinoma who received immunotherapy and underwent palliative RT (15).
The present study aimed to investigate the clinical effect of the dose information of CBCs on the occurrence of SRIL in patients with NSCLC undergoing concurrent chemoradiotherapy (CCRT) and further determine the association between the occurrence of SRIL and the treatment modality (IMRT or PBPST).
2 Materials and methods
2.1 Patient population
Upon approval from the institutional review board (approval no.: 2020-01-034), we retrospectively reviewed the data obtained from 325 patients treated with CCRT between November 2016 and December 2019. A total of 124 patients were excluded from the analysis for the following reasons (Supplementary Figure 1): lacking information for the dose distribution to the healthy organs (n = 40), missing follow-up data (n = 26), incomplete CCRT course (n = 20), provision of induction chemotherapy before CCRT (n = 18), use of the hybrid IMRT/PBSPT technique (n = 15), and missing weekly blood test reports (n = 5). Finally, the data obtained from 201 patients were analyzed (164 in the IMRT group and 37 in the PBSPT group). The requirement to obtain informed consent was waived because of the retrospective nature of this study.
2.2 Treatment
The detailed institutional policies of planning IMRT and PBSPT have been previously described (3). Briefly, based on four-dimensional computed tomography in ten breathing phases, the planning target volume (PTV) was delineated with a 5-mm margin from the clinical target/gross tumor volume. A total dose of 66 GyE in 30 fractions was prescribed in both IMRT and PBSPT groups. Supplementary Table 1 summarizes the planning criteria for the organs at risk. In the IMRT group, the volumetric-modulated arc therapy was the most frequently used (n = 100, 61.0%), followed by the step-and-shoot method with the 6-MV photon coplanar beam (n = 64, 39.0%). In the PBSPT group, single-field optimization was adopted in 22 (59.5%) patients. The two-field plan was used in 23 (62.2%) patients, and the pencil beam algorithm was applied to all patients. Pinnacle (version 9.2, Royal Phillips Electronics, Miami, FL, USA) and RayStation (RaySearch Laboratories, Stockholm, Sweden) were used for planning IMRT and PBSPT, respectively.
Regarding chemotherapy, 189 (94.0%) patients received six cycles of paclitaxel/cisplatin; six patients received paclitaxel/carboplatin; four patients received gemcitabine/cisplatin; and two patients received combined etoposide and cisplatin. Subsequently, 19 (9.5%) patients received maintenance therapy with durvalumab.
2.3 SRIL
Following weekly peripheral blood count assessments during CCRT, lymphopenia was graded based on the Common Terminology Criteria for Adverse Events version 5.00. Based on the absolute lymphocyte count (ALC), SRIL was diagnosed when two or more events of ALC < 200/µL (grade 4) occurred during the CCRT course.
2.4 bDVH
We used the pre-generated spatiotemporal blood distribution based on International Commission on Radiological Protection 89th publication that has been described before (13). Dose data of the heart and lungs from each patient’s treatment plan were utilized for HEDOS calculations. The beam-on-time of IMRT and PBPST were both assumed to be 60 s/beam, and the detailed time structure of beam delivery was not considered. We used the dose after all fractions for the analysis. Also, we used 5.3 L for total CBC volume for all patients to compute dose distribution to CBC and thus the volume of one CBC was 0.053 mL (16).
2.5 Statistical analysis
Overall survival (OS) rates were calculated from the first date of CCRT to the date of death or last follow-up. Progression-free survival (PFS) rates were calculated from the first date of CCRT to the date of progression, death, or the last follow-up. Baseline characteristics were evaluated using the chi-squared test or Fisher’s exact and Mann–Whitney U tests to assess categorical and continuous variables, respectively. The Kaplan–Meier method and log-rank test were used for OS and PFS. A Cox regression model was used for the multivariable analysis of factors affecting OS and PFS that had a p-value < 0.05 in the univariable analysis. The logistic regression analysis was performed to evaluate the predictive factors of SRIL. The factors were selected in stepwise regression after ten-fold cross-validation and included in the multivariate analysis of SRIL. To identify the optimal cutoffs for dosimetric parameters of CBCs, maximally selected rank statistics were performed. A null multivariate model was built based on patient and tumor characteristics. The Akaike information criterion (AIC) was used to compare multivariate models to select the most discriminative dosimetric predictor of SRIL. Statistical significance was set at a two-tailed p-value < 0.05. All statistical analyses were performed using SPSS version 25.0 (IBM Corp., Armonk, NY, USA) and R version 4.0.2 (R Foundation for Statistical Computing, Vienna, Austria).
3 Results
3.1 Patient population
Overall, compared with the patients in the PBSPT group, patients in the IMRT group were younger, more frequently diagnosed with adenocarcinoma, and had a more advanced nodal disease (Supplementary Table 2). Although the target volume or prescription dose did not differ between the two groups (PBSPT and IMRT groups), the dose parameters for V5GyE, V10GyE, and V20GyE of the lungs, mean lung dose, V30GyE of the heart, and mean heart dose were significantly lower in the PBPST group than those in the IMRT group (Table 1). Moreover, bDVH was significantly lower in the PBPST group than that in the IMRT group (Table 1). Figure 1 shows bDVHs for the entire population stratified by RT modality.
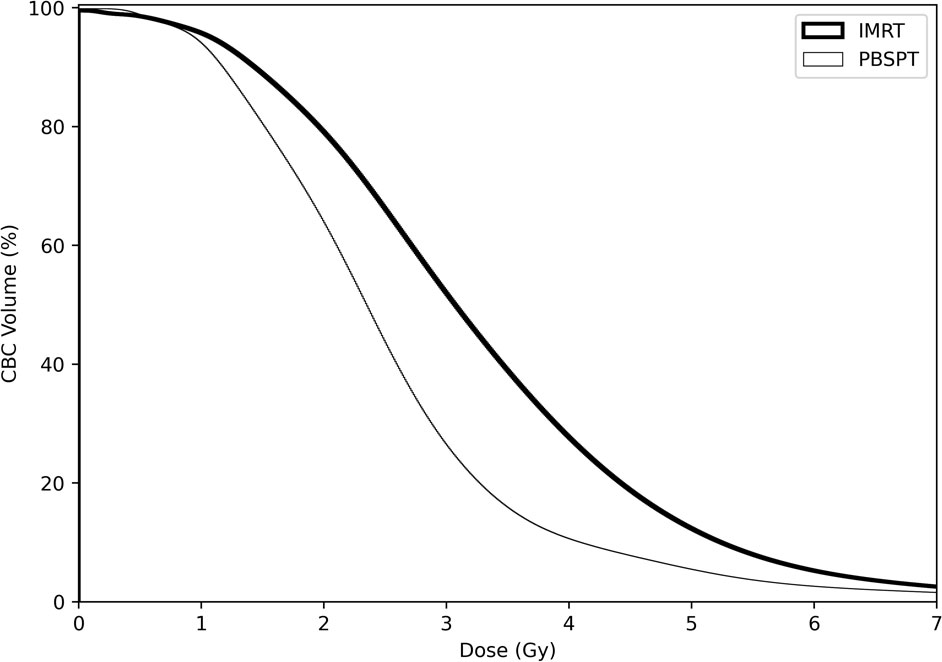
Figure 1 Dose-volume parameters of circulating blood cells in both treatment modalities for all patients.
3.2 SRIL
ALC in the entire cohort decreased gradually during the CCRT course and recovered afterward (Supplementary Figure 2). From week 4 to the last week of the CCRT course, ALCs were significantly lower in the IMRT group than in the PBSPT group (Supplementary Figure 2, Supplementary Table 3). Among 107 (53.2%) patients who developed grade 4 lymphopenia during the CCRT course, 75 (37.3% of entire patients) experienced SRIL, including three (8.1% of PBPST group) in the PBPST group and 72 (43.9% of IMRT group) in the IMRT group (p < 0.001). In addition, none of the patient or tumor characteristics, except for baseline ALC values, differed between the two groups. Patients with SRIL showed significantly lower baseline ALC than those without SRIL (median, 2,010/µL vs. 2,140/µL, p = 0.029, Table 2). Regarding dose-volume parameters, patients with SRIL had a larger target volume and showed a higher dose distribution to the lung and heart than those without SRIL (all p < 0.001, Table 2). Moreover, bDVH for patients with SRIL also differed from that for patients without SRIL (Figure 2).
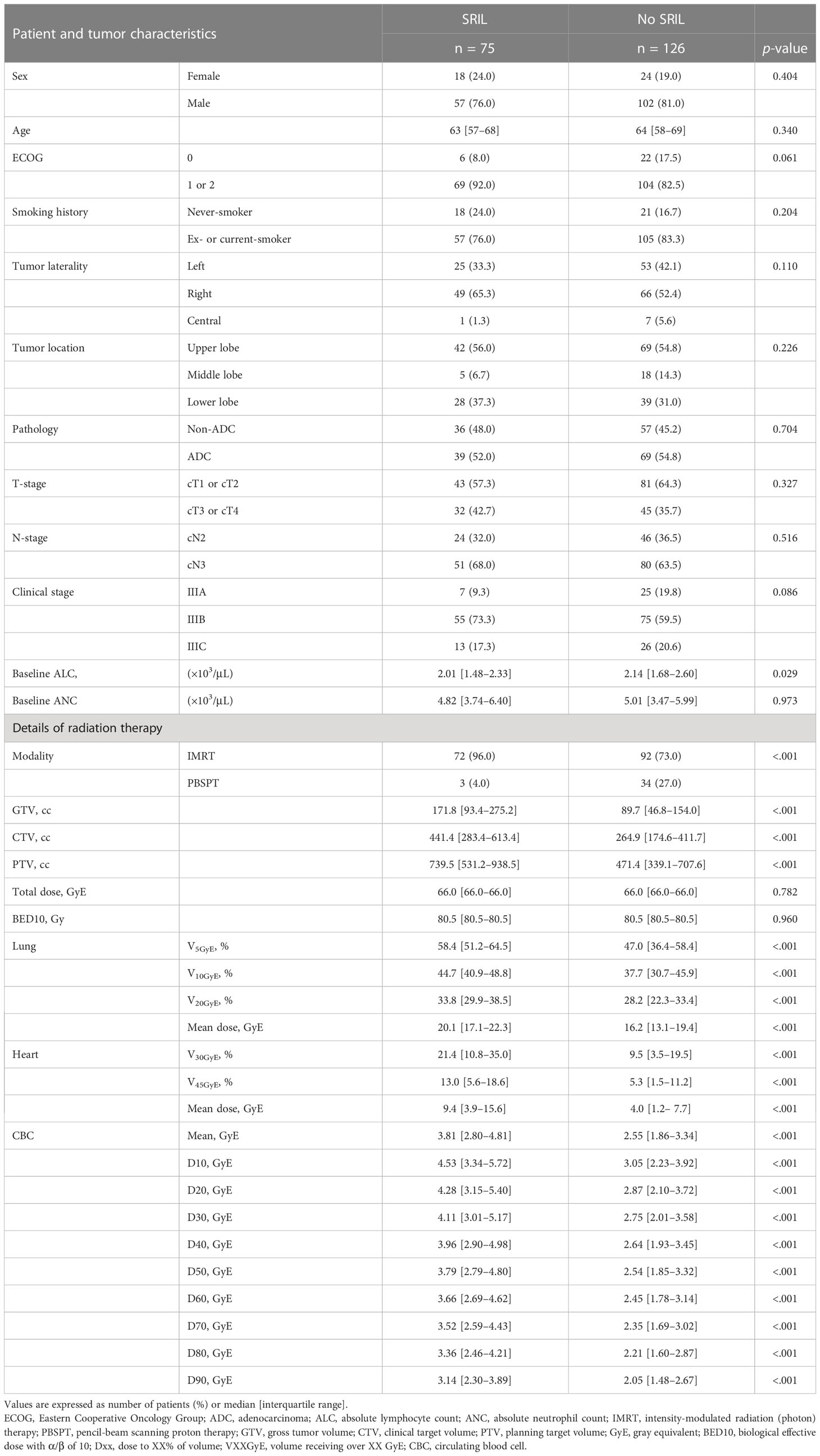
Table 2 Patient, tumor, and treatment characteristics stratified by severe radiation-induced lymphopenia. .
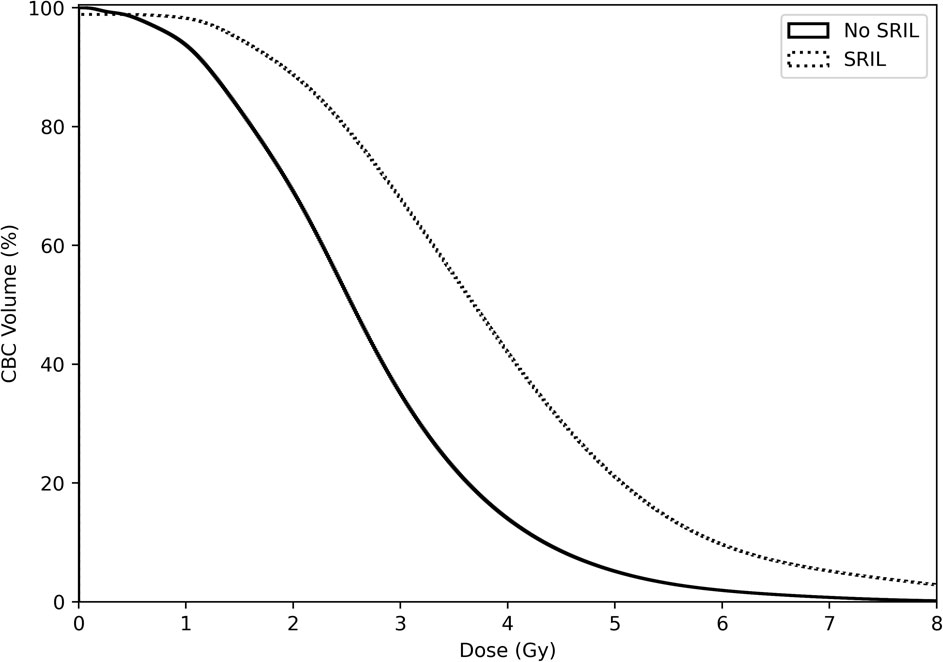
Figure 2 Dose-volume parameters for circulating blood cells stratified by severe radiation-induced lymphopenia.
3.3 Prognostic value of SRIL
With a median follow-up of 39.8 (IQR [interquartile range], 21.0–49.4) months, the 3-year OS and PFS rates were 62.4% and 26.2% for the entire cohort, respectively. Patients with SRIL showed poorer OS and PFS outcomes than those without SRIL (3-year OS, 48.3% vs. 70.9%, p < 0.001; 3-year PFS, 10.5% vs. 36.1%, p < 0.001, Figures 3A, B). The multivariable analysis revealed that SRIL remained a significantly unfavorable factor for both OS and PFS (Table 3).
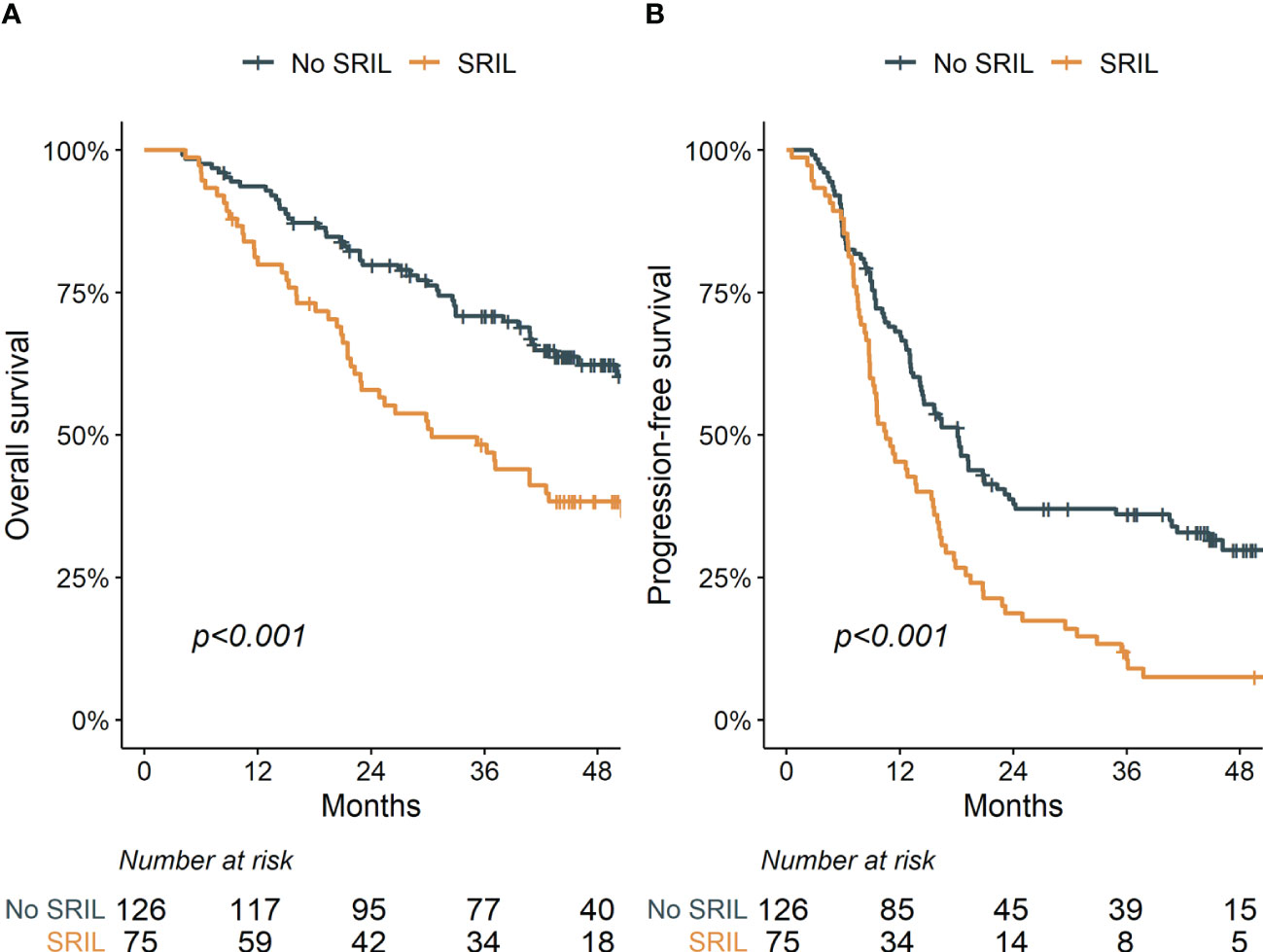
Figure 3 Overall (A) and progression-free (B) survival stratified by severe radiation-induced lymphopenia.
3.4 Factors predicting SRIL
First, we performed a multivariate analysis to predict the development of SRIL based on clinical and treatment factors other than dose information of CBCs. Both baseline ALC (odds ratio [OR] = 0.60, 95% confidence interval [CI]: 0.37–0.94, p = 0.026) and PTV (OR = 1.02, 95% CI: 1.01–1.03, p = 0.001) were related to an increased risk of SRIL (Table 4, Supplementary Table 4). AIC in the null model, including baseline ALC and PTV, was 245.52 (Supplementary Table 5). Subsequent analysis showed that bDVH was related to an increased risk of SRIL in the univariate analysis (Supplementary Table 4). In the stepwise regression model, CBC of D90% as the continuous variable (OR = 3.25, 95% CI: 1.98–5.67, p < 0.001) was the only significant factor among bDVH along with baseline ALC (OR = 0.46, 95% CI: 0.27–0.76, p = 0.004, Table 4).
Finally, we separately generated models with each significant dosimetric variable of CBCs together with the null model. After comparing AIC, the model including CBC D90% > 2.6 GyE was associated with the lowest AIC at 218.46 (Supplementary Table 5) and remained significant in the multivariate analysis (OR = 6.38, 95% CI: 3.19–13.26, p < 0.001), along with baseline ALC and PTV (all p < 0.05, Table 4).
A dose-volume relationship between CBC D90% and the probability of SRIL was observed (Figure 4). In addition, the number of grade 4 lymphopenia events and CBC D90% were positively correlated (Supplementary Figure 3). When analyzing factors affecting D90% > 2.6 GyE, PBSPT significantly satisfied the dose criteria for CBC D90% of 2.6 GyE (OR = 0.11, 95% CI: 0.03–0.31, p = 0.004, Table 5). Furthermore, CBC D90% > 2.6 GyE was associated with poorer OS and PFS outcomes after excluding SRIL from the multivariable analysis (Supplementary Table 6).
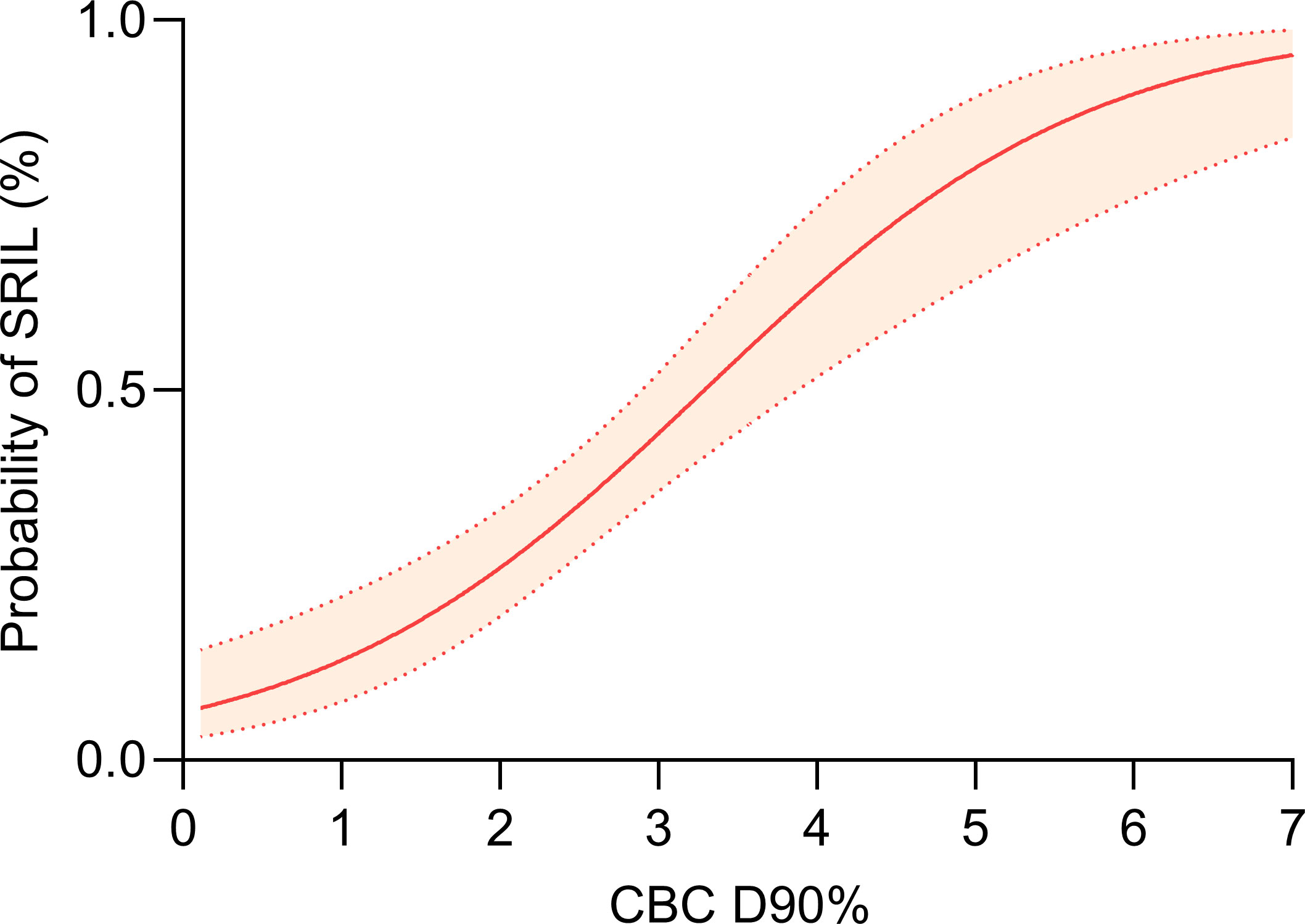
Figure 4 Dose-response relationship between dose to 90% of circulating blood cells and severe radiation-induced lymphopenia.
4 Discussion
In this study, we investigated the prognostic value of SRIL and the correlation of bDVH with the development of SRIL in patients with locally advanced NSCLC treated with CCRT. SRIL was associated with poorer OS and PFS outcomes compared with the control. Moreover, at CBC D90% > 2.6 GyE, the risk of SRIL significantly increased together with baseline ALC and PTV. Moreover, PBPST was a significant contributor to minimizing CBC D90%. In addition, CBC D90% had a prognostic value for OS and PFS outcomes. To the best of our knowledge, this was the first study to discover the clinical impact of CBCs in patients with NSCLC and the potential benefit of PBSPT in minimizing radiation exposure to CBCs compared with IMRT (photon).
The negative impact of treatment-related lymphopenia on treatment outcomes in NSCLC has been widely investigated in recent studies (12, 17–23). Upadhyay et al. systematically reviewed 14 studies involving patients with lung cancer and reported that severe lymphopenia increased the risk of death with a pooled hazard ratio of 1.59 (p < 0.001) and the risk of death/progression with a pooled hazard ratio of 2.1 (p < 0.001) (17). Considering the radiosensitivity of lymphocytes, thoracic RT, which inevitably irradiates highly vascularized lymphocyte-rich organs, such as the lungs and heart, is a major contributor to lymphopenia or SRIL (24). We had previously reported the prognostic value of SRIL in patients with NSCLC (12). Although we could not perform subgroup analyses for patients treated with immunotherapy owing to the small sample size, the clinical significance of SRIL in the context of immunotherapy for NSCLC was highlighted (18, 19, 21–23). Jing et al. discovered that SRIL (defined as ALC < 230/µL at the end of CCRT) disrupted survival benefits of maintenance therapy with durvalumab following CCRT (21). In addition to the development of SRIL, Cho et al. reported that recovery from SRIL at 3 months after CCRT was significantly related to PFS and OS outcomes in patients treated with maintenance immunotherapy (18). The dismal effect of SRIL might stem from the reduced systemic anti-tumor immune response by lymphocytes and depletion of tumor-infiltrating lymphocyte (7, 25). The depletion of CD4+ T cells from SRIL, which control cell-mediated immunity against tumors and exert anti-tumor effects on CD8+ T cells, could influence the prognosis (26).
In view of decreasing the incidence of SRIL, several studies suggested various dose-volume criteria for predicting SRIL (12, 17, 20, 23, 27–29). In addition to the baseline ALC and target volume, most studies revealed that the dose to the lung or heart was predictive of SRIL (17). In this context, we had previously reported that lung V5Gy (OR = 1.07) and baseline ALC (OR = 0.73) were independent predictive factors of SRIL in 223 patients with NSCLC treated with CCRT (12). However, a dose–volume correlation in the lung or heart only indirectly provides the potential impact of RT on SRIL. Joseph et al. reported a negative correlation between the integral body dose and post-RT ALC in patients with lung cancer (29). Furthermore, neither dose to the lungs nor heart was significantly related to post-RT ALC. In addition, several reports highlighted that the effective dose to circulating immune cells (EDIC), which incorporates the mean lung dose, mean heart dose, and integral dose, was related to SRIL in lung, breast, and esophageal cancers (29–31). However, the EDIC equation was formulated based on limited patient data, i.e., patients receiving IMRT with over 25 fractions, and built for thoracic radiation fields only. The EDIC equation is yet to be validated with proton patient data. We chose HEDOS over EDIC in this study because using HEDOS, we can calculate the dose to CBCs utilizing the organ DVH from any treatment modality or treatment site. With a patient-specific bDVH from HEDOS results, the various DVH metrics were tested to find the most significant dosimetric factors for SRIL that could be used to guide the treatment plan in a way to reduce the SRIL risk. The last feature we employed in HEDOS was the dose rates from beam-on-times during beam delivery. Therefore, dose criteria for CBCs could be considered when planning for patients scheduled to receive immunotherapy.
Proton beam therapy is advantageous over photon RT in reducing the low-dose radiation to out-of-field. Based on the aforementioned evidence of the dose-response relationship between the healthy organs and SRIL, proton beam therapy is considered to be a potential therapeutic tool for lymphocyte-sparing RT. PBPST alleviates the risk of SRIL by reducing the lung V5Gy compared with IMRT in patients with NSCLC (12). The clinical significance of proton beam therapy in reducing SRIL has also been explored in primary brain tumors and esophageal cancer (32–34). However, to the best of our knowledge, this is the first analysis determining the clinical significance of PBSPT when incorporated with the dose calculation of CBCs. PBPST could positively affect the dose distribution to CBCs compared with IMRT. Furthermore, FLASH RT with an ultra-high dose-rate could enhance the immune-related tumor response by minimizing radiation to CBCs and subsequently reducing SRIL.
This study has several limitations. First, this study was associated with inherent limitations owing to its retrospective design. Second, the limited number of patients in the PBSPT group might have led to an overestimation of the effect of PBSPT. However, the target volume or baseline ALC, which could affect the SRIL development, did not differ significantly. Other limitations related to HEDOS calculations are using a uniform blood path distribution for all patients, implying that we used same the blood flow rate and blood volume for all patients, as these values were unavailable and impossible to measure in this retrospective study. We did not consider the realistic time structure of the beam delivery of IMRT or PBSPT. As these beams consist of many beamlets with a high dose to a small irradiation area, the realistic beam may produce a few CBCs irradiated with very high dose rates, thereby changing the bDVH shapes. The impact of the realistic time structure on the dose to CBCs should be further investigated. Further analyses are required to develop a model for predicting healthy tissue complication probability for SRIL based on CBCs.
The occurrence of SRIL was associated with an increased risk of tumor progression and death in patients with NSCLC treated with CCRT. We proposed and clinically validated the significance of bDVH in predicting SRIL. CBC D90% > 2.6 GyE significantly increased the risk of SRIL, and PBPST could decrease CBC D90% compared to IMRT. This analysis should be further validated through randomized controlled trials comparing PBPST and IMRT, particularly providing evidence of lymphocyte-sparing from the sparing dose to CBCs in the setting of maintenance immunotherapy.
Data availability statement
The raw data supporting the conclusions of this article will be made available by the authors, without undue reservation.
Ethics statement
The studies involving human participants were reviewed and approved by Samsung medical center. Written informed consent for participation was not required for this study in accordance with the national legislation and the institutional requirements.
Author contributions
Conception, design, data collection, interpretation, and drafting of the manuscript were performed by NK, JS, and SA. Statistical analysis and editing of the manuscript were performed by NK and JS. All authors contributed to the article and approved the submitted version.
Funding
This work was supported by the National Research Foundation of Korea (NRF) grant funded by the Korean government (MSIT) (NRF-2021R1F1A1063383).
Conflict of interest
The authors declare that the research was conducted in the absence of any commercial or financial relationships that could be construed as a potential conflict of interest.
Publisher’s note
All claims expressed in this article are solely those of the authors and do not necessarily represent those of their affiliated organizations, or those of the publisher, the editors and the reviewers. Any product that may be evaluated in this article, or claim that may be made by its manufacturer, is not guaranteed or endorsed by the publisher.
Supplementary material
The Supplementary Material for this article can be found online at: https://www.frontiersin.org/articles/10.3389/fonc.2023.1119173/full#supplementary-material
References
1. Han Y. Current status of proton therapy techniques for lung cancer. Radiat Oncol J (2019) 37(4):232–48. doi: 10.3857/roj.2019.00633
2. Liao Z, Lee JJ, Komaki R, Gomez DR, O'Reilly MS, Fossella FV, et al. Bayesian Adaptive randomization trial of passive scattering proton therapy and intensity-modulated photon radiotherapy for locally advanced non-Small-Cell lung cancer. J Clin Oncol (2018) 36(18):1813–22. doi: 10.1200/jco.2017.74.0720
3. Kim N, Noh JM, Lee W, Park B, Pyo H. Clinical outcomes of pencil beam scanning proton therapy in locally advanced non-small cell lung cancer: Propensity score analysis. Cancers (Basel) (2021) 13(14):3497. doi: 10.3390/cancers13143497
4. Zou Z, Bowen SR, Thomas HMT, Sasidharan BK, Rengan R, Zeng J. Scanning beam proton therapy versus photon IMRT for stage III lung cancer: Comparison of dosimetry, toxicity, and outcomes. Adv Radiat Oncol (2020) 5(3):434–43. doi: 10.1016/j.adro.2020.03.001
5. Bae BK, Yang K, Noh JM, Pyo H, Ahn YC. Clinical outcomes following proton and photon stereotactic body radiation therapy for early-stage lung cancer. Cancers (Basel) (2022) 14(17):4152. doi: 10.3390/cancers14174152
6. Grossman SA, Ellsworth S, Campian J, Wild AT, Herman JM, Laheru D, et al. Survival in patients with severe lymphopenia following treatment with radiation and chemotherapy for newly diagnosed solid tumors. J Natl Compr Canc Netw (2015) 13(10):1225–31. doi: 10.6004/jnccn.2015.0151
7. Formenti SC, Demaria S. Combining radiotherapy and cancer immunotherapy: a paradigm shift. J Natl Cancer Inst (2013) 105(4):256–65. doi: 10.1093/jnci/djs629
8. Hiam-Galvez KJ, Allen BM, Spitzer MH. Systemic immunity in cancer. Nat Rev Cancer (2021) 21(6):345–59. doi: 10.1038/s41568-021-00347-z
9. Grossman SA, Ye X, Lesser G, Sloan A, Carraway H, Desideri S, et al. Immunosuppression in patients with high-grade gliomas treated with radiation and temozolomide. Clin Cancer Res (2011) 17(16):5473–80. doi: 10.1158/1078-0432.Ccr-11-0774
10. Byun HK, Kim N, Yoon HI, Kang SG, Kim SH, Cho J, et al. Clinical predictors of radiation-induced lymphopenia in patients receiving chemoradiation for glioblastoma: Clinical usefulness of intensity-modulated radiotherapy in the immuno-oncology era. Radiat Oncol (2019) 14(1):51. doi: 10.1186/s13014-019-1256-6
11. Byun HK, Kim N, Park S, Seong J. Acute severe lymphopenia by radiotherapy is associated with reduced overall survival in hepatocellular carcinoma. Strahlenther Onkol (2019) 195(11):1007–17. doi: 10.1007/s00066-019-01462-5
12. Kim N, Myoung Noh J, Lee W, Park B, Park H, Young Park J, et al. Proton beam therapy reduces the risk of severe radiation-induced lymphopenia during chemoradiotherapy for locally advanced non-small cell lung cancer: A comparative analysis of proton versus photon therapy. Radiother Oncol (2021) 156:166–73. doi: 10.1016/j.radonc.2020.12.019
13. Shin J, Xing S, McCullum L, Hammi A, Pursley J, Correa CA, et al. HEDOS-a computational tool to assess radiation dose to circulating blood cells during external beam radiotherapy based on whole-body blood flow simulations. Phys Med Biol (2021) 66(16). doi: 10.1088/1361-6560/ac16ea
14. Xing S, Shin J, Pursley J, Correa-Alfonso CM, Depauw N, Domal S, et al. A dynamic blood flow model to compute absorbed dose to circulating blood and lymphocytes in liver external beam radiotherapy. Phys Med Biol (2022) 67(4). doi: 10.1088/1361-6560/ac4da4
15. Qian JM, Akama-Garren E, Shin J, Gunasti L, Bang A, Pike LRG, et al. Dosimetric modeling of lymphopenia in patients with metastatic cancer receiving palliative radiation and PD-1 immune checkpoint inhibitors. Adv Radiat Oncol (2022) 7(2):100880. doi: 10.1016/j.adro.2021.100880
16. Basic anatomical and physiological data for use in radiological protection: Reference values. a report of age- and gender-related differences in the anatomical and physiological characteristics of reference individuals. ICRP publication 89. Ann ICRP (2002) 32(3-4):5–265.
17. Upadhyay R, Venkatesulu BP, Giridhar P, Kim BK, Sharma A, Elghazawy H, et al. Risk and impact of radiation related lymphopenia in lung cancer: A systematic review and meta-analysis. Radiother Oncol (2021) 157:225–33. doi: 10.1016/j.radonc.2021.01.034
18. Cho Y, Kim Y, Chamseddine I, Lee WH, Kim HR, Lee IJ, et al. Lymphocyte dynamics during and after chemo-radiation correlate to dose and outcome in stage III NSCLC patients undergoing maintenance immunotherapy. Radiother Oncol (2022) 168:1–7. doi: 10.1016/j.radonc.2022.01.007
19. Cho Y, Park S, Byun HK, Lee CG, Cho J, Hong MH, et al. Impact of treatment-related lymphopenia on immunotherapy for advanced non-small cell lung cancer. Int J Radiat Oncol Biol Phys (2019) 105(5):1065–73. doi: 10.1016/j.ijrobp.2019.08.047
20. Abravan A, Faivre-Finn C, Kennedy J, McWilliam A, Van Herk M. Radiotherapy-related lymphopenia affects overall survival in patients with lung cancer. J Thorac Oncol (2020) 15(10):1624–35. doi: 10.1016/j.jtho.2020.06.008
21. Jing W, Xu T, Wu L, Lopez PB, Grassberger C, Ellsworth SG, et al. Severe radiation-induced lymphopenia attenuates the benefit of durvalumab after concurrent chemoradiotherapy for NSCLC. JTO Clin Res Rep (2022) 3(9):100391. doi: 10.1016/j.jtocrr.2022.100391
22. Friedes C, Chakrabarti T, Olson S, Prichett L, Brahmer JR, Forde PM, et al. Association of severe lymphopenia and disease progression in unresectable locally advanced non-small cell lung cancer treated with definitive chemoradiation and immunotherapy. Lung Cancer (2021) 154:36–43. doi: 10.1016/j.lungcan.2021.01.022
23. Chen D, Patel RR, Verma V, Ramapriyan R, Barsoumian HB, Cortez MA, et al. Interaction between lymphopenia, radiotherapy technique, dosimetry, and survival outcomes in lung cancer patients receiving combined immunotherapy and radiotherapy. Radiother Oncol (2020) 150:114–20. doi: 10.1016/j.radonc.2020.05.051
24. Golden EB, Apetoh L. Radiotherapy and immunogenic cell death. Semin Radiat Oncol (2015) 25(1):11–7. doi: 10.1016/j.semradonc.2014.07.005
25. Bremnes RM, Busund LT, Kilvær TL, Andersen S, Richardsen E, Paulsen EE, et al. The role of tumor-infiltrating lymphocytes in development, progression, and prognosis of non-small cell lung cancer. J Thorac Oncol (2016) 11(6):789–800. doi: 10.1016/j.jtho.2016.01.015
26. Eberst G, Vernerey D, Laheurte C, Meurisse A, Kaulek V, Cuche L, et al. Prognostic value of CD4+ T lymphopenia in non-small cell lung cancer. BMC Cancer (2022) 22(1):529. doi: 10.1186/s12885-022-09628-8
27. Tang C, Liao Z, Gomez D, Levy L, Zhuang Y, Gebremichael RA, et al. Lymphopenia association with gross tumor volume and lung V5 and its effects on non-small cell lung cancer patient outcomes. Int J Radiat Oncol Biol Phys (2014) 89(5):1084–91. doi: 10.1016/j.ijrobp.2014.04.025
28. Xie X, Lin SH, Welsh JW, Wei X, Jin H, Mohan R, et al. Radiation-induced lymphopenia during chemoradiation therapy for non-small cell lung cancer is linked with age, lung V5, and XRCC1 rs25487 genotypes in lymphocytes. Radiother Oncol (2021) 154:187–93. doi: 10.1016/j.radonc.2020.09.002
29. Joseph N, McWilliam A, Kennedy J, Haslett K, Mahil J, Gavarraju A, et al. Post-treatment lymphocytopaenia, integral body dose and overall survival in lung cancer patients treated with radical radiotherapy. Radiother Oncol (2019) 135:115–9. doi: 10.1016/j.radonc.2019.03.008
30. Chen F, Jin JY, Hui TSK, Jing H, Zhang H, Nong Y, et al. Radiation induced lymphopenia is associated with the effective dose to the circulating immune cells in breast cancer. Front Oncol (2022) 12:768956. doi: 10.3389/fonc.2022.768956
31. Xu C, Jin JY, Zhang M, Liu A, Wang J, Mohan R, et al. The impact of the effective dose to immune cells on lymphopenia and survival of esophageal cancer after chemoradiotherapy. Radiother Oncol (2020) 146:180–6. doi: 10.1016/j.radonc.2020.02.015
32. Mohan R, Liu AY, Brown PD, Mahajan A, Dinh J, Chung C, et al. Proton therapy reduces the likelihood of high-grade radiation-induced lymphopenia in glioblastoma patients: Phase II randomized study of protons vs photons. Neuro Oncol (2021) 23(2):284–94. doi: 10.1093/neuonc/noaa182
33. Ebrahimi S, Lim G, Liu A, Lin SH, Ellsworth SG, Grassberger C, et al. Radiation-induced lymphopenia risks of photon versus proton therapy for esophageal cancer patients. Int J Part Ther (2021) 8(2):17–27. doi: 10.14338/ijpt-20-00086
Keywords: proton beam therapy, lung cancer, lymphopenia, radiation therapy, blood
Citation: Kim N, Shin J, Ahn SH, Pyo H, Noh JM, Yang K, Lee W and Park B (2023) Reduced radiation exposure to circulating blood cells in proton therapy compared with X-ray therapy in locally advanced lung cancer: Computational simulation based on circulating blood cells. Front. Oncol. 13:1119173. doi: 10.3389/fonc.2023.1119173
Received: 10 January 2023; Accepted: 14 February 2023;
Published: 27 February 2023.
Edited by:
Kevin Ni, St George Hospital Cancer Care Centre, AustraliaReviewed by:
Antonino Romeo, Scientific Institute of Romagna for the Study and Treatment of Tumors (IRCCS), ItalyAtsuto Katano, The University of Tokyo Hospital, Japan
Copyright © 2023 Kim, Shin, Ahn, Pyo, Noh, Yang, Lee and Park. This is an open-access article distributed under the terms of the Creative Commons Attribution License (CC BY). The use, distribution or reproduction in other forums is permitted, provided the original author(s) and the copyright owner(s) are credited and that the original publication in this journal is cited, in accordance with accepted academic practice. No use, distribution or reproduction is permitted which does not comply with these terms.
*Correspondence: Nalee Kim, cm9kci5uYWxlZWtpbUBnbWFpbC5jb20=
†These authors have contributed equally to this work