- 1Radiotherapy Unit, Veneto Institute of Oncology IOV – IRCCS, Padua, Italy
- 2Department of Radiation Oncology, Abano Terme Hospital, Padua, Italy
- 3Medical Oncology 1, Veneto Institute of Oncology IOV-IRCSS, Padua, Italy
Introduction: Radionecrosis is a consequence of SRS (stereotactic radiosurgery) for brain metastases in 34% of cases, and if symptomatic (8%–16%), it requires therapy with corticosteroids and bevacizumab and, less frequently, surgery. Oncological indications are increasing and appropriate stereotactic adapted LINACs (linear accelerators) are becoming more widely available worldwide. Efforts are being made to treat brain radionecrosis in order to relieve symptoms and spare the use of active therapies.
Case presentation: Herein, we describe a 65-year-old female patient presenting with brain radionecrosis 6 months after stereotactic radiotherapy for two brain metastatic lesions. Being symptomatic with headache and slow cognitive-motor function, the patient received corticosteroids. Because of later lung progression, the patient took cabozantinib. An impressive reduction of the two brain radionecrosis areas was seen at the brain MRI 2 months after the initiation of the angiogenic drug.
Discussion: The high incidence of radionecrosis (2/2 treated lesions) can be interpreted by the combination of SRS and previous ipilimumab that is associated with increased risk of radionecrosis. The molecular mechanisms of brain radionecrosis, and its exact duration in time, are poorly understood. We hypothesize that the antiangiogenic effect of cabozantinib may have had a strong effect in reducing brain radionecrosis areas.
Conclusion: In this clinical case, cabozantinib is associated with a fast and significant volume reduction of brain radionecrosis appearing after SRS and concomitant immunotherapy. This drug seems to show, like bevacizumab, clinical implications not only for its efficacy in systemic disease control but also in reducing brain radionecrosis. More research is needed to evaluate all molecular mechanisms of brain radionecrosis and their interaction with systemic therapies like third-generation TKIs.
Introduction
Stereotactic radiotherapy is a type of external radiation therapy that uses special equipment to position the patient and precisely give a high radiation dose to a tumor. It generally consists of less than five fractions, and it is also called hypofractionated stereotactic radiotherapy. When the radiation is delivered in one single fraction, it is called stereotactic radiosurgery (SRS).
Thanks to clinical trials and retrospective data (1, 2), stereotactic radiation therapy has almost replaced whole brain irradiation in case of multiple brain metastases. Whole brain remains a therapeutic option, eventually associated with hippocampal sparing (3), in case of multiple brain lesions (>10), uncontrolled extracranial disease, and high cumulative central volume (>15 ml) (2).
Stereotactic radiotherapy is associated with 85%–95% local control at 1 year for lesions <2 cm (4, 5) and very few adverse events (6). One of the major adverse events is brain radionecrosis.
Radionecrosis is a focal structural anomaly that forms following cranial irradiation of cerebral neoplasms, and it is observed in approximately 5%–26% of patients at 1 year and up to 25.5%–34% at 2 years of follow-up (7–11). It can be asymptomatic in approximately 14%–84% of cases and symptomatic in approximately 8.4%–16.4% of cases (12–14).
The time frame of presentation is variable and typically ranges from 3 months to 10 years post-radiotherapy, but 80% of cases occur within 3 years after the completion of radiotherapy (15).
Radionecrosis is treated with the lowest dose of corticosteroids and, if the patient becomes refractory or intolerant to corticosteroids, surgery and bevacizumab are discussed at multidisciplinary boards (16, 17).
Case presentation
A 65-year-old woman was treated with radical left nephrectomy and lymph node dissection in 2018. The pathological diagnosis was clear cell renal carcinoma, grade 2, pT3a-pN0. The full-body CT showed no metastatic lesions at baseline (cM0).
After 3 months, the full-body CT showed progression in multiple lung and abdominal lymph nodes; she therefore started first-line systemic therapy with nivolumab and ipilimumab for 3 months, followed by nivolumab as maintenance therapy. Because of the progression of lung, wedge resection was performed for two pulmonary lesions in 2020 after multidisciplinary board decision.
In October 2021, brain MRI revealed two brain metastasis, one was in the right frontal lobe (3 mm) and the other in the left occipital lobe (18 mm), both associated with extensive brain edema (Figure 1). The patient was admitted to the hospital for headache and asthenia, limiting deambulation. Stereotactic radiotherapy was performed on both lesions. The frontal lesion was treated with 20 Gy/1 fraction on PTV (planning tumor volume) and 25 Gy on GTV (gross tumor volume), with respect to V12 Gy of brain including target < 5 cm3 and V14 Gy < 5 cm3; the occipital lesion was treated with 27 Gy/3 fractions on PTV and 33 Gy on GTV, with respect to V20 Gy of brain including target < 20 cm3 and V14 Gy < 20 cm3 (18); nivolumab was reestablished soon after the tapering of corticosteroids. A 3-mm GTV–PTV margin was considered appropriate by physicians and physicists as voluminous edema and high-dose corticosteroids could dislocate the GTV, and because of setup accuracy when performing SRS with adapted LINAC. The specific prescription to GTV/PTV with respect to radiation necrosis constraints, as described, was done according to the institute’s clinical practice.
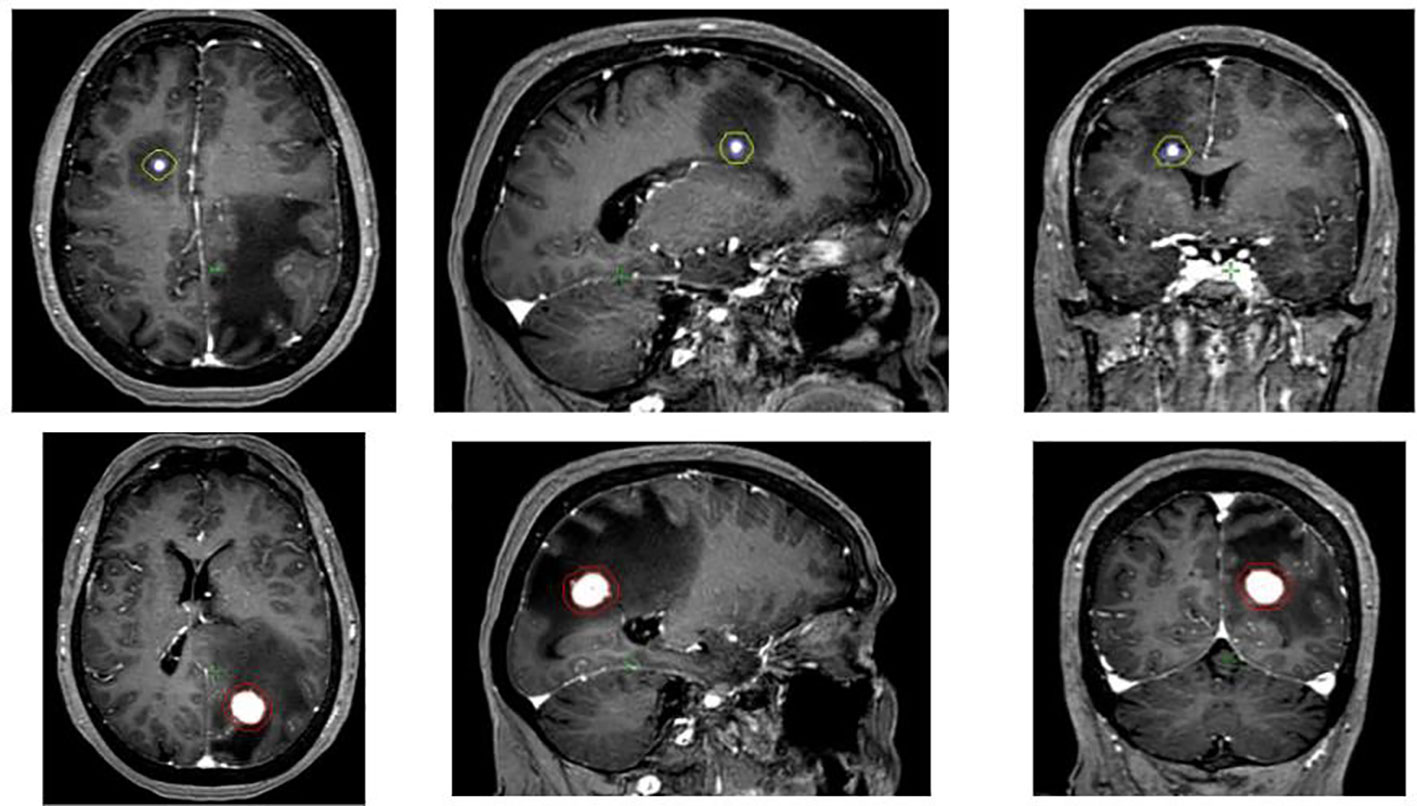
Figure 1 Brain MRI showing the two brain metastases, the right frontal (3 mm) and the left occipital one (18 mm). GTV was the T1-gadolinium enhanced lesion while PTV was defined as GTV + 3 mm.
The 1-month (November 2021) and 3-month brain MRI (January 2022) showed reduction of the occipital lesion but increased contrast enhancement area of the frontal lesion associated with edema in T2-FLAIR (Fluid Attenuated Inversion Recovery) sequence: being symptomatic with headache and cognitive-motor slowing, mannitol and high-dose corticosteroids were administered in Day Hospital.
As for extracranial single-site disease progression, stereotactic radiotherapy was delivered to a pancreatic lesion (35 Gy/5 fractions on PTV) in February 2022, in addition to nivolumab.
In April 2022, the brain MRI revealed partial response of the occipital metastasis and suspected frontal radionecrosis (mismatch T2/T1, necrotic and bubble-like lesion in T1-contrast sequence) and no new lesions.
In July 2022, the brain MRI showed increased contrast enhancement of the central lesions, both suspected for brain radionecrosis. The case was discussed several times at the neurooncological multidisciplinary team: surgery was not suggested as it was neither considered safe (deep parenchymal lesions) nor advisable considering the clinical partial benefit obtained by corticosteroids. Therefore, pathological confirmation of the diagnosis was not possible to achieve. A radiological diagnosis of brain radiation necrosis was maintained considering the radiological and clinical evolution of the lesion during the 8-month follow-up period. A neuro-radiologist was always present at multidisciplinary team meetings for a qualified review of images (DWI, T2, T1 with contrast, FLAIR, STIR, ADC, and perfusion with CBV sequences).
In September 2022, abdomen and thoracic CT scan pointed out a renal and pulmonary disease progression and the patient started second-line therapy with cabozantinib.
After only 2 months from second-line systemic therapy, the brain MRI showed excellent reduction of the two areas of brain radionecrosis with a surprising shrinkage of the associated edema (Figure 2). The patient is still alive and is being treated with cabozantinib when this article is sent to publication.
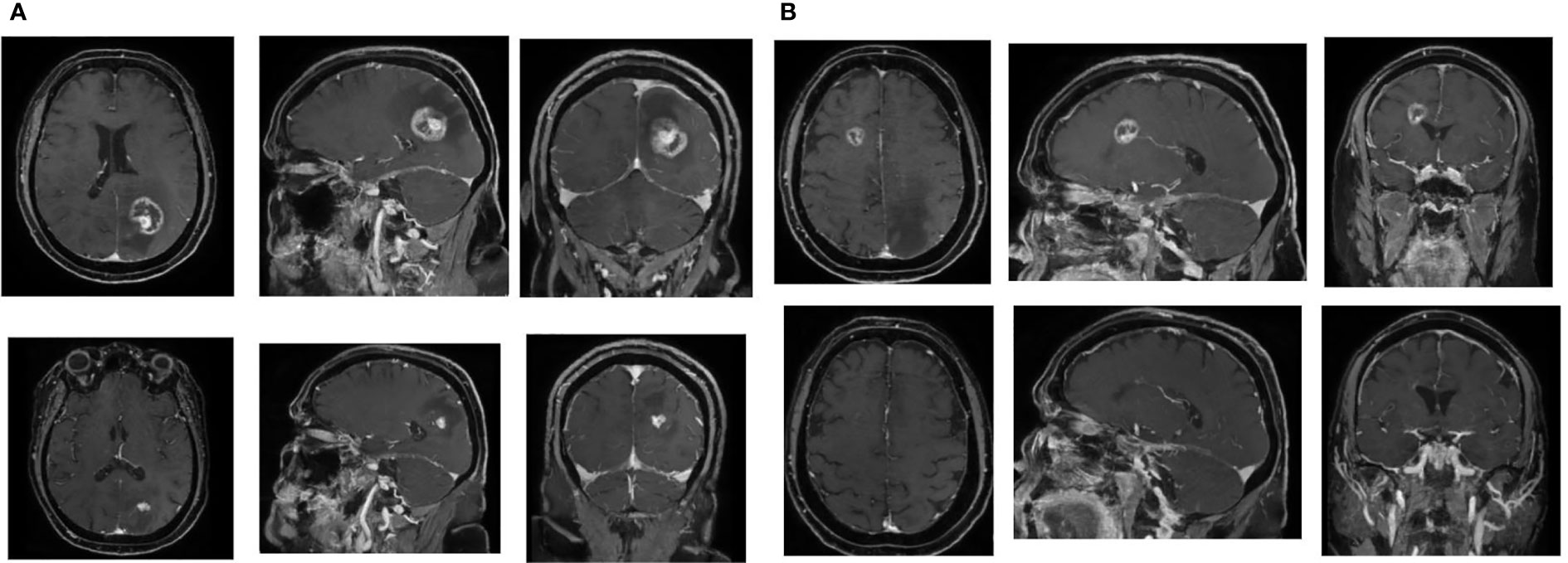
Figure 2 Impressive volume reduction of two brain radionecrosis areas. (A) Left occipital lesion. Above, MRI before cabozantinib (9 months after SRS). Below, MRI after cabozantinib (13 months after SRS). (B) Right frontal lesion: Above, MRI before cabozantinib (9 months after SRS). Below, MRI after cabozantinib (13 months after SRS).
The patient has given written consent to the publication of images and personal clinical data for this case report description.
Discussion
The incidence of brain radionecrosis after stereotactic radiotherapy for brain metastases is up to 34% (19). In our case, the incidence was higher as both treated lesions presented with signs of radionecrosis at control brain MRI. The increased incidence we observe can be interpreted by data available in the medical literature.
Firstly, we reviewed the dosimetric constraints and verified that they had been respected (18). Although a clinical trial with 49 patients has demonstrated that 1-mm margin PTV is associated with a lower risk of radionecrosis when compared to 3-mm margin PTV (20), and the same authors indicate that a 1-mm GTV–PTV margin would be ideal, but possible uncertainties such as patient setup and accuracy should be considered when choosing the margin. The reasons why we considered a 3-mm GTV–PTV margin appropriate are both clinical and dosimetric. From a clinical point of view, as shown in MRI figures, both lesions were surrounded by voluminous edema that warranted high-dose corticosteroids that could shift the target position. From a physicist point of view, radiosurgery with multi-source gamma-ray platforms requires no expansions but radiosurgery delivered by stereotactic adapted LINACs, as in our case, requires expansions to take into consideration possible uncertainties in patient setup, beam alignment, organ motion, organ deformation, and planning accuracy (fusion of images, contrast medium acquisition time, etc.). Despite the different margins recommended, there are no differences in the rate of symptomatic radionecrosis between adapted LINACs and multi-source gamma-ray platforms (21).
Secondly, we reviewed data about SRS and immunotherapy. In fact, some authors have hypothesized that concomitant immunotherapy, by promoting T-cell activation and anti-tumor response, may trigger radionecrosis (22, 23). Association between radionecrosis and previous ipilimumab has already been demonstrated (31% versus 13%) (24, 25). SRS in combination with ipilimumab was also observed to cause a temporary increase in lesion diameter to >150% (26).
Few data are available about the association between dual immunotherapy and radiation necrosis: though Johnson et al. (27) demonstrated that the dual immunotherapy (ipilimumab + nivolumab) is not associated with higher incidence of radiation necrosis, data in the literature often make no specification about radionecrosis diagnostic criteria, grading, and radiation dose prescription.
An impressive volume reduction was observed soon after the initiation of cabozantinib, an antiangiogenic drug. Antiangiogenic drugs have been used against RCC (renal cell carcinoma) for more than a decade. They are classified as first-generation TKIs (tyrosine kinase inhibitors), such as sunitinib, sorafenib, and pazopanib, and second-generation TKIs, such as axitinib and tivozanib, and, later, cabozantinib and envatinib (28).
Cabozantinib is an oral TKI targeting several receptors involved in angiogenesis pathways such as VEGFR2, c-MET, RET, c-Kit, AXL, TIE2, ROS1, TYRO3, MERTK, TRKB, and FLT3 receptors. All of these targets and pathways are implicated in cancer development and progression (29). Inhibition of the VEGFR blocks angiogenesis, cell tubule formation, cellular migration and invasion, and cell proliferation, and induces apoptosis (30). Cabozantinib is a RET inhibitor: the RET receptor is implicated in the inflammation process, with its activation resulting in an increase of cytokines in the tumor microenvironment, leading to the recruitment of suppressive immune cells and, thus, allowing tumor growth and invasion (Figure 3).
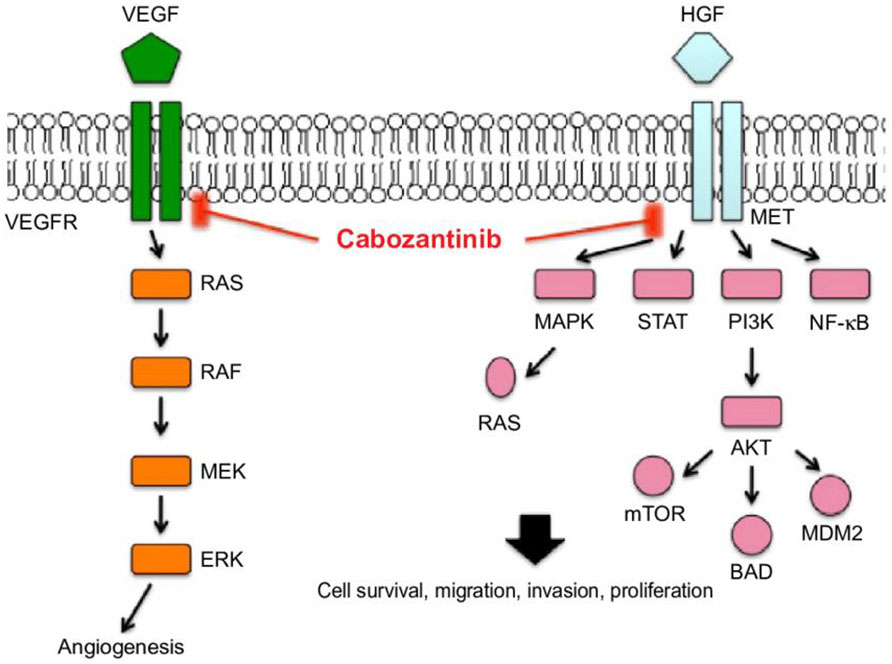
Figure 3 Molecular pathways of cabozantinib [image from Yu et al. (31)].
After first-line nivolumab–ipilimumab therapy, the median time to failure for cabozantinib is 6.90 months (32) with a median OS of 21.44 months and 37.9% of ORR (33). More data of cabozantinib as a second-line therapy will be revealed by the ongoing trial CaboPoint (34). While the association between cabozantinib and SRS has proved to be safe (35), limited data about the effect of cabozantinib started on active brain radionecrosis are available.
Currently, molecular mechanisms of radionecrosis are not fully understood. The principal hypothesis holds that stereotactic radiotherapy is responsible for direct damage to the blood vessels around the irradiated area as demonstrated in human specimens (36). This damage causes hypoxia around the irradiated area and, thus, upregulation of the hypoxia-inducible factor-1 alpha (HIF-1-alpha) in glial cells (37). HIF-1-alpha augments VEGF, which is responsible for the neo-angiogenesis and subsequent brain edema. HIF-1-alpha also augments inflammatory cytokines (IL-1, IL-6, and TNF alpha) that seem to aggravate the perilesional edema (Figure 4) (38).
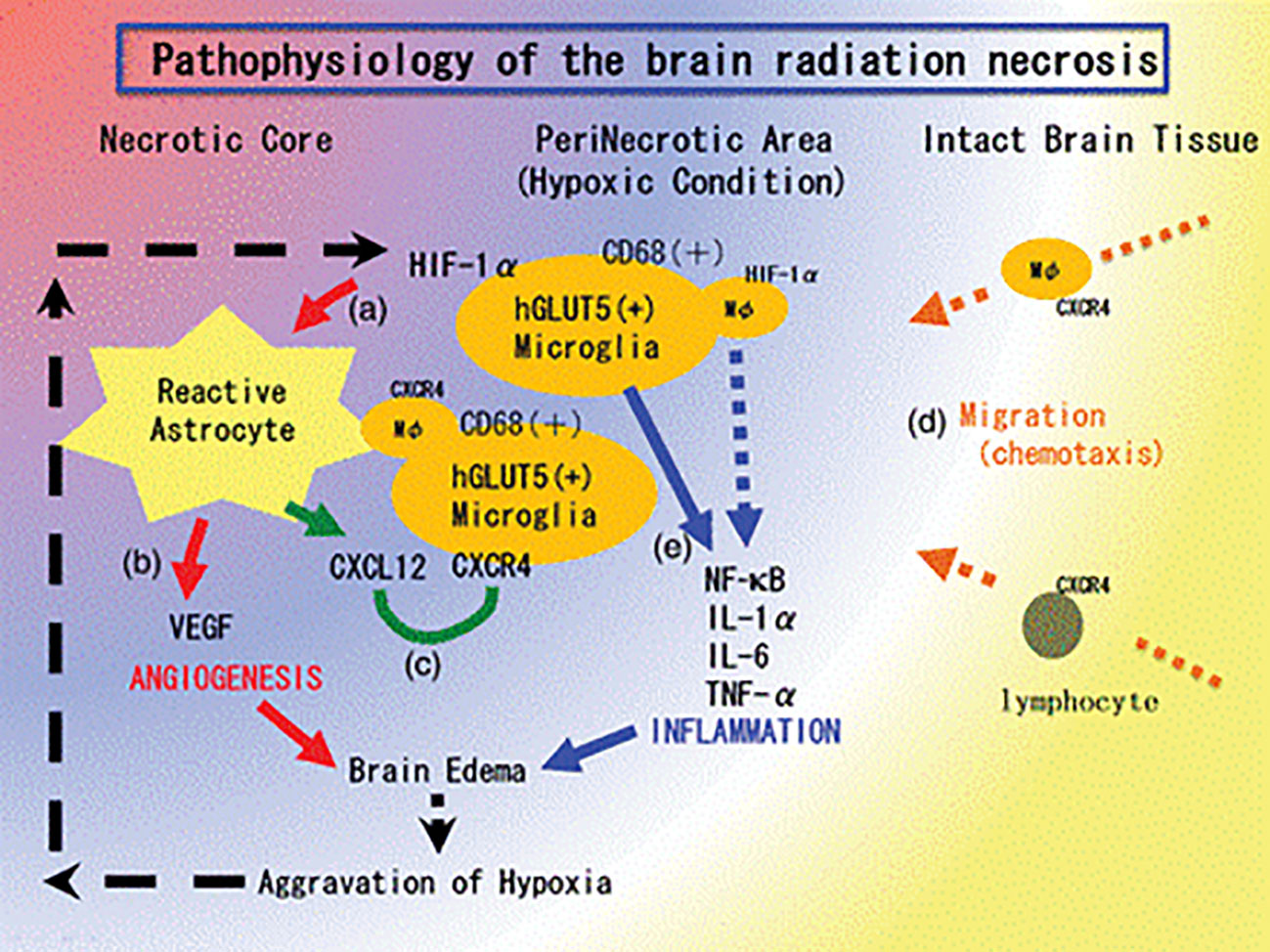
Figure 4 The pathophysiology of brain radiation necrosis from Yoritsune et al. (36).
The inhibition triggered by cabozantinib is fundamentally arresting the neoangiogenesis cascade and the fibroblast activation that is finally responsible for tissue fibrosis and brain radionecrosis. In addition, cabozantinib is a selective inhibitor of C-MET that is overexpressed in clear cell carcinoma tumors, and this aspect shows that this drug could have a direct tumoricidal effect, particularly when radionecrosis seems to be accompanied by residual disease (39). These effects are possible thanks to the drug pharmacokinetics as cabozantinib crosses the blood–brain barrier: some rodent models revealed that cabozantinib concentration in brain reaches 20% of the peak plasma level (40, 41).
This case report has some limitations. First of all, in the absence of pathological confirmation, we cannot exclude the persistence of disease in the differential diagnosis with radiation-induced inflammation. After neuro-oncological multidisciplinary discussion, surgery was neither considered safe (deep parenchymal lesions) nor advisable, considering the clinical partial benefit obtained by corticosteroids. The radiological presentation, the time duration (8 months), and the partial response after corticosteroids seem to suggest radionecrosis, although this cannot be stated with certainty.
A second limitation might be considered the 3-mm GTV–PTV expansion instead of a smaller one: voluminous edema, setup accuracy, and a stereotactic-adapted LINAC were the reasons why a 3-mm GTV–PTV margin was considered appropriate, simultaneously respecting constraints as described (brain V12 Gy and V14 Gy) (18).
Conclusion
In this clinical case, cabozantinib is associated with a fast and significant volume reduction of brain radionecrosis that appeared after radiosurgery and concomitant immunotherapy. This drug seems to show, like bevacizumab, clinical implications not only for its efficacy in systemic disease control but also in reducing brain radionecrosis. More research is needed to evaluate all molecular mechanisms of brain radionecrosis and their interaction with systemic therapies like third-generation TKIs.
Data availability statement
The raw data supporting the conclusions of this article will be made available by the authors, without undue reservation.
Ethics statement
Written informed consent was obtained from the individual(s) for the publication of any potentially identifiable images or data included in this article.
Author contributions
Authors JL, FT and FaB contributed equally. All authors contributed to the article and approved the submitted version.
Funding
This research was funded by Italian Ministry of Health Ricerca Corrente.
Conflict of interest
The authors declare that the research was conducted in the absence of any commercial or financial relationships that could be construed as a potential conflict of interest.
Publisher’s note
All claims expressed in this article are solely those of the authors and do not necessarily represent those of their affiliated organizations, or those of the publisher, the editors and the reviewers. Any product that may be evaluated in this article, or claim that may be made by its manufacturer, is not guaranteed or endorsed by the publisher.
References
1. Andrews DW, Scott CB, Sperduto PW, Flanders AE, Gaspar LE, Schell MC, et al. “Whole brain radiation therapy with or without stereotactic radiosurgery boost for patients with one to three brain metastases: Phase III results of the RTOG 9508 randomised trial,”. Lancet (2004) 363(9422):1665–72. doi: 10.1016/S0140-6736(04)16250-8
2. Yamamoto, Serizawa T, Shuto T, Akabane A, Higuchi Y, Kawagishi J, et al. “Stereotactic radiosurgery for patients with multiple brain metastases (JLGK0901): a multi-institutional prospective observational study. Lancet Oncol (2014) 15(4):387–95. doi: 10.1016/S1470-2045(14)70061-0
3. Brown PD, Gondi V, Pugh S, Tome WA, Wefel JS, Armstrong TS, et al. “Hippocampal avoidance during whole-brain radiotherapy plus memantine for patients with brain metastases: Phase III trial NRG oncology CC001,”. J Clin Oncol (2020) 38(10):1019–29. doi: 10.1200/JCO.19.02767
4. Vogelbaum MA, Angelov L, Lee S-Y, Li L, Barnett GH, Suh JH. “Local control of brain metastases by stereotactic radiosurgery in relation to dose to the tumor margin,”. J Neurosurg (2006) 104(6):907–12. doi: 10.3171/jns.2006.104.6.907
5. Leyrat B, Khalill T, Lemaire JJ, Casile M, Molnar I, Dedieu V, et al. “Local control and radionecrosis of brain metastases from non-small-cell lung cancer treated by hypofractionated stereotactic radiotherapy: Evaluation of predictive factors,”. Clin Transl Radiat Oncol (2022) 36:1–8. doi: 10.1016/j.ctro.2022.05.008
6. Patel A, Dong T, Ansari S, Cohen-Gadol A, Watson GA, de Moraes FY, et al. “Toxicity of radiosurgery for brainstem metastases,”. World Neurosurg (2018) 119:e757–64. doi: 10.1016/j.wneu.2018.07.263
7. Lee D, Riestenberg RA, Haskell-Mendoza A, Bloch O. Brain metastasis recurrence versus radiation necrosis: Evaluation and treatment. Neurosurg Clin N Am (2020) 31(4):575–87. doi: 10.1016/j.nec.2020.06.007
8. Minniti G, Clarke E, Lanzetta G, Falchett Osti M, Trasimeni G, Bozzao A, et al. “Stereotactic radiosurgery for brain metastases: Analysis of outcome and risk of brain radionecrosis,”. Radiat Oncol (2011) 6(1):48. doi: 10.1186/1748-717X-6-48
9. Kohutek ZA, Yamada Y, Chan TA, Brennan CW, Tabar V, Gutin PH, et al. “Long-term risk of radionecrosis and imaging changes after stereotactic radiosurgery for brain metastases,”. J Neurooncol (2015) 125(1):. 149–156. doi: 10.1007/s11060-015-1881-3
10. Schüttrumpf LH, Niyazi M, Nachbichler SK, Manapov F, Jansen N, Siefert A, et al. “Prognostic factors for survival and radiation necrosis after stereotactic radiosurgery alone or in combination with whole brain radiation therapy for 1–3 cerebral metastases,”. Radiat Oncol (2014) 9(1):105. doi: 10.1186/1748-717X-9-105
11. Ohtakara K, Hayashi S, Nakayama N, Ohe N, Yano H, Iwama T, et al. “Significance of target location relative to the depth from the brain surface and high-dose irradiated volume in the development of brain radionecrosis after micromultileaf collimator-based stereotactic radiosurgery for brain metastases,”. J Neurooncol (2012) 108(1):201–9. doi: 10.1007/s11060-012-0834-3
12. Daisne J-F, de Ketelaere C, Jamart J. “The individual risk of symptomatic radionecrosis after brain metastasis radiosurgery is predicted by a continuous function of the V12Gy,”. Clin Transl Radiat Oncol (2021) 27:70–4. doi: 10.1016/j.ctro.2021.01.003
13. Blonigen BJ, Steinmetz RD, Levin L, Lamba MA, Warnick RE, Breneman JC. “Irradiated volume as a predictor of brain radionecrosis after linear accelerator stereotactic radiosurgery,”. Int J Radiat OncologyBiologyPhysics (2010) 77(4):996–1001. doi: 10.1016/j.ijrobp.2009.06.006
14. Peng L, Grimm J, Gui C, Shen CJ, Redmond KJ, Sloan L, et al. “Updated risk models demonstrate low risk of symptomatic radionecrosis following stereotactic radiosurgery for brain metastases,”. Surg Neurol Int (2019) 10(1):32. doi: 10.4103/sni.sni_303_18
15. Walker AJ, Ruzevick J, Malayeri AA, Rigamonti D, Lim M, Redmond KJ, et al. “Postradiation imaging changes in the CNS: How can we differentiate between treatment effect and disease progression?,”. Future Oncol (2014) 10(7):1277–97. doi: 10.2217/fon.13.271
16. Patel U, Patel A, Cobb C, Benkers T, Vermeulen S. The management of brain necrosis as a result of SRS treatment for intra-cranial tumors. Transl Cancer Res (2014) 3(4):373–82. doi: 10.3978/j.issn.2218-676X.2014.07.05
17. Available at: https://www.aifa.gov.it/documents/20142/1288746/Allegato-1_Bevacizumab_04.01.2021.pdf.
18. Milano MT, Grimm J, Niemierko A, Soltys SG, Moiseenko V, Redmond KJ, et al. “Single- and multifraction stereotactic radiosurgery Dose/Volume tolerances of the brain,”. Int J Radiat OncologyBiologyPhysics (2021) 110(1):68–86. doi: 10.1016/j.ijrobp.2020.08.013
19. le Rhun E, Dhermain F, Vogin G, Reyns N, Metellus P. “Radionecrosis after stereotactic radiotherapy for brain metastases,”. Expert Rev Neurother (2016) 16(8):903–14. doi: 10.1080/14737175.2016.1184572
20. Kirkpatrick JP, Wang Z, Sampson JH, McSherry F, Herndon JE 2nd, Allen KJ, et al. Defining the optimal planning target volume in image-guided stereotactic radiosurgery of brain metastases: Results of a randomized trial. Int J Radiat Oncol Biol Phys (2015) 91:100–8. doi: 10.1016/j.ijrobp.2014.09.004
21. Scorsetti M, Navarria P, Cozzi L, Clerici E, Bellu L, Franceschini D, et al. Radiosurgery of limited brain metastases from primary solid tumor: Results of the randomized phase III trial (NCT02355613) comparing treatments executed with a specialized or a c-arm linac-based platform. Radiat Oncol (2023) 18:28. doi: 10.1186/s13014-023-02216-5
22. Liniker E, Menzies AM, Kong BY, Cooper A, Ramanujam S, Lo S, et al. “Activity and safety of radiotherapy with anti-PD-1 drug therapy in patients with metastatic melanoma,”. Oncoimmunology (2016) 5(9):e1214788. doi: 10.1080/2162402X.2016.1214788
23. Bernstein MB, Krishnan S, Hodge JW, Chang JY. “Immunotherapy and stereotactic ablative radiotherapy (ISABR): A curative approach?,”. Nat Rev Clin Oncol (2016) 13(8):516–24. doi: 10.1038/nrclinonc.2016.30
24. Pires da Silva I, Glitza IC, Haydu LE, Johnpulle R, Banks PD, Grass GD, et al. “Incidence, features and management of radionecrosis in melanoma patients treated with cerebral radiotherapy and anti-PD-1 antibodies.,”. Pigment Cell Melanoma Res (2019) 32(4):553–63. doi: 10.1111/pcmr.12775
25. Colaco RJ, Martin P, Kluger HM, Yu JB, Chiang VL. “Does immunotherapy increase the rate of radiation necrosis after radiosurgical treatment of brain metastases?,”. J Neurosurg (2016) 125(1):17–23. doi: 10.3171/2015.6.JNS142763
26. Kiess AP, Wolchok JD, Barker CA, Postow MA, Tabar V, Huse JT, et al. “Stereotactic radiosurgery for melanoma brain metastases in patients receiving ipilimumab: Safety profile and efficacy of combined treatment,”. Int J Radiat OncologyBiologyPhysics (2015) 92(2):368–75. doi: 10.1016/j.ijrobp.2015.01.004
27. Johnson, Postow MA, Chapman P, Wolchock JD, Brennan CW, Tabar VS, et al. Safety and clinical outcomes of ipilimumab and nivolumab plus concurrent stereotactic radiosurgery for brain metastases. Poster viewing Q&A session (2018) 102(3 supplement):E228. doi: 10.1016/j.ijrobp.2018.07.778
28. Iaxx R, Lefort F, Domblides C, Ravaud A, Bernhard J-C, Gross-Goupil M. “An evaluation of cabozantinib for the treatment of renal cell carcinoma: Focus on patient selection and perspectives,”. Ther Clin Risk Manag (2022) 18:619–32. doi: 10.2147/TCRM.S251673
29. Yakes FM, Chen J, Tan J, Yamaguchi K, Shi Y, Yu P, et al. “Cabozantinib (XL184), a novel MET and VEGFR2 inhibitor, simultaneously suppresses metastasis, angiogenesis, and tumor growth,”. Mol Cancer Ther (2011) 10(12):2298–308. doi: 10.1158/1535-7163.MCT-11-0264
30. Hicklin DJ, Ellis LM. Role of the vascular endothelial growth factor pathway in tumor growth and angiogenesis. J Clin Oncol (2005) 23(5):1011–27. doi: 10.1200/JCO.2005.06.081
31. Yu SS, Quinn DI, Dorff TB. Clinical use of cabozantinib in the treatment of advanced kidney cancer: Efficacy, safety, and patient selection. Onco Targets Ther (2016) 9:5825–37. doi: 10.2147/OTT.S97397
32. Navani V, Wells C, Boyne DJ, Cheung WY, Brenner D. “CABOSEQ: The efficacy of cabozantinib post up-front immuno-oncology combinations in patients with advanced renal cell carcinoma: Results from the international metastatic renal cell carcinoma database consortium (IMDC)”. J Clin Oncol (2022) 40(6_suppl):318–8. doi: 10.1200/JCO.2022.40.6_suppl.318
33. Procopio G, Claps M, Pircher C, Porcu L, Sepe P, Guadalupi V, et al. A multicenter phase 2 single arm study of cabozantinib in patients with advanced or unresectable renal cell carcinoma pre-treated with one immune-checkpoint inhibitor: The BREAKPOINT trial (Meet-uro trial 03). Tumori (2022) 29:3008916221138881. doi: 10.1177/03008916221138881
34. Albiges L, Schmidinger M, Taguieva-Pioger N, Perol D, Grünwald V, Guemas E. “CaboPoint: A phase II study of cabozantinib as second-line treatment in patients with metastatic renal cell carcinoma,”. Future Oncol (2022) 18(8):915–26. doi: 10.2217/fon-2021-1006
35. Peverelli G, Raimondi A, Ratta R, Verzoni E, Bregni M, Cortesi E, et al. “Cabozantinib in renal cell carcinoma with brain metastases: Safety and efficacy in a real-world population,”. Clin Genitourin Cancer (2019) 17(4):291–8. doi: 10.1016/j.clgc.2019.05.002
36. Yoritsune E, Furuse M, Kuwabara H, Miyata T, Nonoguchi N, Kawabata S, et al. “Inflammation as well as angiogenesis may participate in the pathophysiology of brain radiation necrosis,”. J Radiat Res (2014) 55(4):803–11. doi: 10.1093/jrr/rru017
37. Miyatake S-I, Nonoguchi N, Furuse M, Yoritsune E, Miyata T, Kawabata S, et al. “Pathophysiology, diagnosis, and treatment of radiation necrosis in the brain,”. Neurol Med Chir (Tokyo) (2015) 55(1):50–9. doi: 10.2176/nmc.ra.2014-0188
38. Liang Z, Brooks J, Willard M, Liang K, Yoon Y, Kang S, et al. “CXCR4/CXCL12 axis promotes VEGF-mediated tumor angiogenesis through akt signaling pathway,”. Biochem Biophys Res Commun (2007) 359(3):716–22. doi: 10.1016/j.bbrc.2007.05.182
39. Hirsch L, Martinez Chanza N, Farah S, Xie W, Flippot R, Braun DA, et al. “Clinical activity and safety of cabozantinib for brain metastases in patients with renal cell carcinoma,”. JAMA Oncol (2021) 7(12):1815–23. doi: 10.1001/jamaoncol.2021.4544
40. Zhang Y, Guessous F, Kofman A, Schiff D, Abounader R. XL-184, a MET, VEGFR-2 and RET kinase inhibitor for the treatment of thyroid cancer, glioblastoma multiforme and NSCLC. IDrugs (2010) 13(2):112–21.
Keywords: cabozantinib, radionecrosis (RN), stereotacic radiation, immunotherapy, radiosurgery
Citation: Lolli J, Tessari F, Berti F, Fusella M, Fiorentin D, Bimbatti D, Basso U and Busato F (2023) Impressive reduction of brain metastasis radionecrosis after cabozantinib therapy in metastatic renal carcinoma: A case report and review of the literature. Front. Oncol. 13:1136300. doi: 10.3389/fonc.2023.1136300
Received: 02 January 2023; Accepted: 20 February 2023;
Published: 07 March 2023.
Edited by:
Carsten Friedrich, Klinikum Oldenburg AöR, GermanyReviewed by:
Liam Welsh, Royal Marsden NHS Foundation Trust, United KingdomPaul Stephen Rava, UMass Memorial Medical Center, United States
Copyright © 2023 Lolli, Tessari, Berti, Fusella, Fiorentin, Bimbatti, Basso and Busato. This is an open-access article distributed under the terms of the Creative Commons Attribution License (CC BY). The use, distribution or reproduction in other forums is permitted, provided the original author(s) and the copyright owner(s) are credited and that the original publication in this journal is cited, in accordance with accepted academic practice. No use, distribution or reproduction is permitted which does not comply with these terms.
*Correspondence: Fabio Busato, ZmFiaW8xOTg4YnVzYXRvQGdtYWlsLmNvbQ==
†These authors have contributed equally to this work