- Division of Hematology-Oncology, Department of Internal Medicine, University of Michigan, Ann Arbor, MI, United States
Peripheral T cell lymphomas (PTCL) are a heterogenous group of mature T cell lymphomas with an overall poor prognosis. Understanding the molecular heterogeneity in PTCL subtypes may lead to improved understanding of the underlying biological mechanisms driving these diseases. Mutations in the epigenetic regulator TET2 are among the most frequent mutations identified in PTCL, with the highest frequency in angioimmunoblastic T cell lymphomas and other nodal T follicular helper (TFH) lymphomas. This review dissects the role of TET2 in nodal TFH cell lymphomas with a focus on emerging biological insights into the molecular mechanism promoting lymphomagenesis and the potential for epigenetic therapies to improve clinical outcomes.
Introduction
Peripheral T cell lymphomas (PTCL) are a heterogenous group of aggressive lymphomas derived from mature T cells and account for 10-15% of all non-Hodgkin lymphomas (1). Among PTCL cases, the World Health Organization (WHO) classification has recently recognized a distinct entity termed nodal T follicular helper cell (TFH) lymphomas, which include the subtypes previously termed angioimmunoblastic T cell lymphoma (AITL), follicular T cell lymphoma and peripheral T cell lymphoma with a TFH phenotype (2). Nodal TFH lymphomas share phenotypic and gene expression similarities with normal T follicular helper (TFH) cells (3–5), a CD4+ T cell subset that promotes germinal center B cell differentiation (6). In the International Peripheral T-cell and Natural Killer/T-cell Lymphoma study, AITL (at the time the most recognized nodal TFH lymphoma) accounted for approximately 20% of PTCL cases and thus is the second most common PTCL subtype after PTCL, not otherwise specified (1). Clinically, nodal TFH lymphomas typically present at an advanced stage with lymphadenopathy, hepatosplenomegaly and constitutional symptoms, as well as various autoimmune manifestations (7–9). PTCLs, including nodal TFH lymphomas, have an overall poor prognosis with AITL patients having an expected 5-year overall survival of ~30% (1, 10). Recurrent somatic mutations in multiple epigenetic regulators, including loss-of-function mutations in TET2, inactivating mutations in DNMT3A and neomorphic mutations in IDH2, have been strongly associated with nodal TFH lymphomas (11–16). Given the poor prognosis of these lymphomas, understanding the underlying biology is critical to design therapies with improved efficacy. Given that TET2 is the most commonly mutated epigenetic regulator in these lymphomas, this review will focus on the mechanistic role of TET2 in the development and treatment of nodal TFH lymphomas.
TET2 function
TET2 is a member of the ten-eleven-translocation (TET) family of Fe2+- and alpha-ketoglutarate-dependent methylcytosine dioxygenases. These enzymes oxidize 5-methylcytosine (5mC) to 5-hydroxymethylcytosine (5hmC) and subsequent oxidized methylcytosine intermediates to ultimately generate an unmodified cytosine (17–19). DNA methylation was long thought to be a relatively stable epigenetic mark; however, the discovery of the TET family of enzymes introduced the concept of active DNA demethylation. TET2 is broadly expressed in hematopoietic cells and TET2 loss promotes hematopoietic stem cell (HSC) and myeloid cell expansion in murine models (19–22). Studies of TET2 function in murine and human hematopoietic cells reveal that TET2 deletion or loss-of-function mutations, such as those in nodal TFH lymphomas, lead to altered DNA methylation and chromatin accessibility at regulatory enhancer regions (23–25), suggesting functional epigenetic consequences.
In the study of TET2 function in T cells, deletion of TET2 in mature T cells does not result in any appreciable alteration in late T cell development or peripheral T cell activation (26). However, in antigen-specific CD4+ T cells, TET2 loss leads to an increase in TFH differentiation in a cell-intrinsic manner with hypermethylation at gene loci associated with helper T cell differentiation (27), suggesting that TET2 directly represses TFH differentiation by demethylating key regulatory loci.
TET2 and associated mutations in T cell lymphomas
Loss-of-function mutations in TET2 were first identified in myeloid malignancies (28, 29) but soon thereafter recurrent somatic mutations in TET2 were recognized in approximately 50-70% of AITL and other TFH-derived lymphomas (11–15). Subsequent gene sequencing of other T cell leukemias/lymphomas revealed TET2 mutations at much lower frequencies compared to nodal TFH lymphomas – including 17% of T-cell prolymphocytic leukemia cases (30), 14-20% of acute T-cell leukemia/lymphoma cases (31, 32) and in 4-12% cases of cutaneous T cell lymphoma and/or Sezary syndrome cases (33–35).
Furthermore, frequent mutations at isocitrate dehydrogenase 2 arginine 172 (IDH2 R172) have also been identified in AITL and other TFH-derived lymphomas (16). IDH2 is a mitochondrial enzyme that typically converts isocitrate to 2-alpha-ketoglutarate (aKG); however, the R172 mutation promotes abnormal oncometabolite production of the R-enantiomer of alpha-hydroxyglutarate (aHG) (36), which competitively inhibits aKG-dependent enzymes including the TET family (37). Thus, nodal TFH-derived lymphomas with IDH2 R172 mutations are predicted to have repressed TET activity and accordingly IDH2-mutated AITL exhibits genome-wide DNA hypermethylation compared to IDH2 wild-type AITL (38).
TET2 role in lymphomagenesis
Despite frequent TET2 mutations in a wide array of T cell lymphomas, most commonly in nodal TFH lymphomas, it was initially unclear the degree to which TET2 loss-of-function directly contributed to lymphomagenesis. TET2 deletion in murine hematopoietic stem cells (HSCs) altered early and late hematopoiesis in both myeloid and lymphoid lineages with eventual development of myeloid malignancies in the mice (14, 21, 22) but only rarely mature lymphoid malignancies (14). A murine model with a hypomorphic TET2 allele does develop TFH-like lymphomas but with a prolonged latency (39).
In several sequencing studies of nodal TFH lymphomas including AITL, multiple TET2 mutations were found in individual tumor samples implying a strong selective pressure (12, 13, 40). Additionally, in AITL cases, the majority of the cases that carried a TET2 mutation had a variant allele frequency >10% (12, 38, 40).
Since TET2 mutations were frequently found to co-occur with a glycine to valine (G17V) inactivating mutation in Rho GTPase RhoA in 50-70% of AITL cases (12, 40, 41), several groups sought to dissect the relative contribution of RhoA-G17V mutations and TET2 loss of function to T cell lymphomagenesis. Adoptive transfer of wild-type or TET2-deficient T cells retrovirally transduced to overexpress RhoA-G17V into T-cell deficient murine hosts resulted in CD4+ T cell expansion, disruption of peripheral T cell homeostasis and eventually lethal inflammation but no lymphoma was noted (42). Several other groups generated transgenic mice expressing the RhoA-G17V mutation in the setting of TET2 hematopoietic deficiency. In these various murine models, RhoA-G17V overexpression in T cells promoted TFH proliferation/expansion (43, 44) and the concomitant expression in the setting of hematopoietic TET2 deficiency led to the development of TFH lymphomas with varying penetrance (43–45). On a molecular level, RhoA-G17V and TET2 loss was found to promote mammalian target of rapamycin complex 1 (mTORC1) pathway activation (43, 44) and inactivation of forkhead box O1 (FOXO1) signaling (44), suggesting potential therapeutic targets. Together these data strongly support a role for RhoA-G17V as a driving mutation in nodal TFH lymphomas but also speak to the requirement for concomitant TET2 loss in the hematopoietic compartment to promote lymphomagenesis. Targeting of downstream pathways, such as with mTOR inhibitors, may be an attractive therapeutic target to be tested in nodal TFH lymphomas, though no trials are currently underway.
TET2 in clonal hematopoiesis and tumor microenvironment
Mutations in TET2 are among the three most frequent somatic mutations in age-related clonal hematopoiesis and are associated with an increased risk of hematologic cancers as well as all-cause mortality (46–48). The extent to which TET2 mutations in the lymphoma microenvironment and responding immune cells contributes to T cell lymphomagenesis has not been fully elucidated.
It has been posited that TET2 mutations noted in nodal TFH lymphomas largely arise in the setting of clonal hematopoiesis, which is supported by the fact that TET2 mutations in T cell lymphoma patients are frequently found to co-occur in the non-neoplastic B lymphocyte, myeloid and HSC compartments as well as the neoplastic T cells (14, 49, 50). In patients with AITL, the majority of patients had TET2 mutations identified in the neoplastic T cells as well as the myeloid compartment (51). In this case series, 4 of 22 patients with TET2 mutations and available sequencing data developed myeloid neoplasms approximately 2-4 years following their lymphoma diagnosis. The myeloid neoplasms all shared multiple TET2 mutations in the myeloid clone and AITL cells but also contained additional different mutations that were not shared. Together these data support myeloid neoplasms arising from early clonal TET2-mutated hematopoietic stem cells but with divergent evolution from the neoplastic AITL cells.
The presence of TET2-mutated immune cells in AITL patients led to the question if TET2 mutations alter tumor immunity to promote T cell lymphomagenesis. TET2 is known to have pleiotropic functions in different immune cells known to play a role in tumor immunity, including macrophages/monocytes, CD4+ helper T cells, T regulatory cells, CD8+ T cells and B cells (52). In myeloid cells, TET2 represses inflammatory gene expression (53) with increased IL-6, IL-1β and arginase 1 in TET2-deficient macrophages (54, 55). In a murine melanoma model, TET2 deletion in myeloid cells resulted in reduced tumor burden and increased tumor-infiltrating T cells suggesting that TET2 promotes a myeloid immunosuppressive program in the tumor microenvironment (56). In CD4+ T cells, TET2 inhibits cytokine production, including IFNγ, IL-17 and IL-10 (57), cytokines which can have both immunostimulatory and immunosuppressive roles. Furthermore, TET2 (in combination with either TET1 or TET3) dampens regulatory T cell immunosuppressive function (58, 59), which are a critical cellular subset known to suppress anti-tumor responses (60). In CD8+ T cells, TET2 represses memory differentiation following infection (26), though less is known about the role of TET2 deficiency in CD8+ T cell anti-tumor immunity and T cell exhaustion. Given these pleotropic roles TET2 may play in the tumor microenvironment, it is important to carefully analyze the tumor-intrinsic versus microenvironmental roles TET2 loss-of-function mutations play in promoting nodal TFH lymphomas.
A recent elegant study dissected T cell-intrinsic versus -extrinsic role of TET2 in lymphomagenesis using murine models with either hematopoietic or T cell specific loss of TET2 crossed to RhoA-G17V transgenic mice (61). TET2 deficiency in all hematopoietic cells accelerated the development of TFH lymphomas compared to either a wild-type hematopoietic compartment or TET2 deletion solely in T cells. To test which immune compartment contributed to TFH lymphomagenesis, the authors co-transplanted tumor cells with a variety of immune lineages into immunodeficient mice and monitored tumor development. Only when B cells were co-transplanted did donor-derived tumors develop suggesting that TET2 loss in B cells supported TFH lymphomagenesis. Subsequent analysis revealed clonal expansion of TET2-deficient germinal center B cells in the tumor-bearing mice, unique mutations in core histones developed in murine clonal B cells and that inhibition of CD40-CD40L interactions prolonged survival in mice. Correlative studies in human AITL samples demonstrated an expansion of germinal center B cells in involved lymph nodes and unique mutations (some also in core histone genes) in the tumor-associated B cells and plasma cells. These data strongly support a cooperating role for TET2-mutated B cells in the immune microenvironment to promote nodal TFH lymphoma development. Targeting these interactions could provide a novel therapeutic avenue in nodal TFH lymphoma patients, although it remains unclear if this mechanism occurs outside of TET2-mutated clonal hematopoiesis.
Treatment and prognosis implications
Since AITL and other nodal TFH lymphomas have an overall poor prognosis with currently available treatments (1, 10), novel therapeutic approaches are needed to improve patient outcomes. TET2 mutations have been noted to be associated with adverse clinical parameters (13, 62) but not associated with a change in overall survival (13). Given the frequency of TET2 and other epigenetic mutations (ie, DNMT3A) that occur in the majority of nodal TFH lymphomas, there is great interest in utilizing epigenetic therapies to target underlying biological mechanism in hopes to improve response rates and survival. PTCL has been shown to be uniquely responsive to one type of epigenetic therapy, specifically histone deacetylase (HDAC) inhibitors, with three HDAC inhibitors approved for systemic PTCL: romidepson, belinostat and chidamide (in China). In the phase II trial of romidepsin in relapsed/refractory PTCL, patients with relapsed/refractory AITL had an overall response rate of 33% compared to 25% of the overall cohort with two-thirds of the AITL responders achieving a complete remission (63). Similarly, in the phase II registration study of belinostat in relapsed/refractory PTCL, patients with AITL seemed to have improved response rates (45%) compared to response rate (26%) of the overall trial population (64). A more recent retrospective, multicenter study comparing HDAC inhibitor responses in TFH versus non-TFH PTCL patients found a significantly improved overall response rate in nodal TFH vs. non-TFH lymphomas (56.5% versus 29.4%) (65). Together these data support the idea that nodal TFH lymphomas may be more sensitive to epigenetic modulation than non-TFH lymphomas (summarized in Table 1), whether this sensitivity correlates with the presence of epigenetic alterations due to TET2 mutations remains unknown.
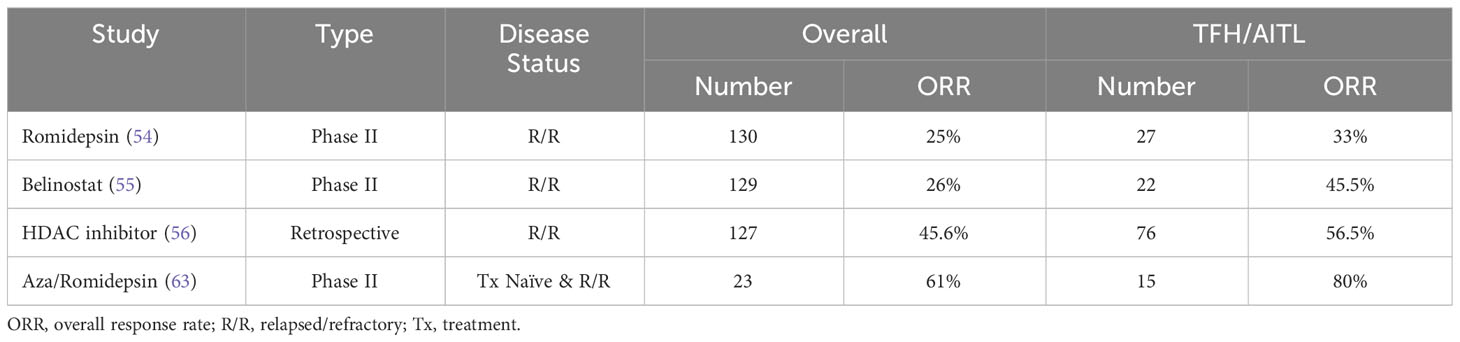
Table 1 Response rates in epigenetic therapies in relapsed/refractory nodal TFH lymphomas versus overall PTCL.
Given TET2’s function in active DNA demethylation, questions naturally arise about the role of hypomethylating agents (HMAs) in nodal TFH lymphomas. Several case reports and case series suggest some clinical efficacy of single agent HMAs (5-azacitidine or decitabine) in TET2-mutated angioimmunoblastic T cell lymphoma (66–69). Preclinical studies have suggested synergy between HMAs and HDAC inhibitors in T cell lymphomas (70, 71) providing a biologic rationale for combined epigenetic targeted therapy in PTCL patients. A multicenter phase II trial examining the combination of oral 5-azacitidine and romidepsin in treatment naïve and relapsed/refractory PTCL patients found that patients with a TFH phenotype had higher overall response rate (80%) and complete response (60%) compared to the overall response rate (25%) and complete remission rate (12.5%) among patients with other subtypes (72). In this early-phase study, there were no statistical differences in response rates between patients with wild-type or mutated TET2 but this was limited by small sample size. Together these data suggest that duel epigenetic targeting therapies may be particularly effective in nodal TFH lymphomas.
Since patients with relapsed/refractory AITL have progressively shorter remissions with each subsequent line of therapy (73), the best chance to cure patients likely lies in improving first-line therapies. Based on the emerging understanding of the underlying biology and the role of epigenetic targeted therapies in nodal TFH lymphomas, several studies have been undertaken to combine epigenetic therapy with standard front-line chemotherapy (CHOP, cyclophosphamide, doxorubicin, vincristine and prednisone). A randomized phase III trial compared to romidepsin plus CHOP to CHOP alone in treatment naïve patients with PTCL. Unfortunately, there were no differences in response rates, progression free survival or overall survival and there were more treatment-related adverse advents in the Ro-CHOP arm (74). However, in an exploratory analysis, PTCL patients with a TFH phenotype had improved progression free survival after Ro-CHOP compared CHOP suggesting that nodal TFH lymphomas may derive a unique benefit and clinical trials should be further focused on PTCL subsets. Clinical trials focused on nodal TFH PTCL populations are examining the efficacy of combining azacitidine (NCT03542266) or chidamide (NCT03853044) with frontline CHOP. Recently published results of the phase II trial of oral azacitidine plus CHOP in 20 evaluable PTCL patients demonstrated a complete response in 88.2% of PTCL-TFH patients and 2-year progression free survival of 69.2% in PTCL-TFH patients. Notably, TET2 mutations were significantly associated with complete response rates and overall survival (75). The oral azacitidine plus CHOP combination is being tested in an ongoing randomized phase II trial in previously untreated patients with CD30-negative PTCL (NCT04803201).
Conclusions
From the initial identification of TET2 mutations in AITL and other nodal TFH lymphomas just over twenty years ago, significant strides have been made to advance the understanding of TET2’s role in the pathogenesis of these lymphomas. Namely, TET2 loss of function in the lymphoma microenvironment, which arises in the setting of clonal hematopoiesis, likely play a critical role in supporting TFH transformation and AITL development. Additionally, emerging clinical evidence suggests that epigenetic targeted therapies may improve response rates and survival in patients with nodal TFH lymphomas. Using the evolving scientific knowledge about the underlying biology of these rare lymphomas, future clinical trials may need to tailor trial populations to discern true efficacy of these therapies.
Author contributions
The author reviewed the literature, performed the writing and revisions of the manuscript.
Funding
This work was supported by a Limpert Clinical Scholar Career Development award from the University of Michigan.
Conflict of interest
The authors declares that the research was conducted in the absence of any commercial or financial relationships that could be construed as a potential conflict of interest.
Publisher’s note
All claims expressed in this article are solely those of the authors and do not necessarily represent those of their affiliated organizations, or those of the publisher, the editors and the reviewers. Any product that may be evaluated in this article, or claim that may be made by its manufacturer, is not guaranteed or endorsed by the publisher.
References
1. Vose J, Armitage J, Weisenburger D, International TCLP. International peripheral T-cell and natural killer/T-cell lymphoma study: pathology findings and clinical outcomes. J Clin Oncol (2008) 26(25):4124–30. doi: 10.1200/JCO.2008.16.4558
2. Alaggio R, Amador C, Anagnostopoulos I, Attygalle AD, Araujo IBO, Berti E, et al. The 5th edition of the world health organization classification of haematolymphoid tumours: lymphoid neoplasms. Leukemia (2022) 36(7):1720–48. doi: 10.1038/s41375-022-01620-2
3. de Leval L, Rickman DS, Thielen C, Reynies A, Huang YL, Delsol G, et al. The gene expression profile of nodal peripheral T-cell lymphoma demonstrates a molecular link between angioimmunoblastic T-cell lymphoma (Aitl) and follicular helper T (Tfh) cells. Blood (2007) 109(11):4952–63. doi: 10.1182/blood-2006-10-055145
4. Grogg KL, Attygalle AD, Macon WR, Remstein ED, Kurtin PJ, Dogan A. Expression of cxcl13, a chemokine highly upregulated in germinal center T-helper cells, distinguishes angioimmunoblastic T-cell lymphoma from peripheral T-cell lymphoma, unspecified. Mod Pathol (2006) 19(8):1101–7. doi: 10.1038/modpathol.3800625
5. Dobay MP, Lemonnier F, Missiaglia E, Bastard C, Vallois D, Jais JP, et al. Integrative clinicopathological and molecular analyses of angioimmunoblastic T-cell lymphoma and other nodal lymphomas of follicular helper T-cell origin. Haematologica (2017) 102(4):e148–e51. doi: 10.3324/haematol.2016.158428
6. Crotty S. T follicular helper cell biology: A decade of discovery and diseases. Immunity (2019) 50(5):1132–48. doi: 10.1016/j.immuni.2019.04.011
7. Ma H, O'Connor OA, Marchi E. Management of angioimmunoblastic T-cell lymphoma (Aitl) and other T follicular helper cell lymphomas (Tfh ptcl). Semin Hematol (2021) 58(2):95–102. doi: 10.1053/j.seminhematol.2021.03.001
8. Federico M, Rudiger T, Bellei M, Nathwani BN, Luminari S, Coiffier B, et al. Clinicopathologic characteristics of angioimmunoblastic T-cell lymphoma: analysis of the international peripheral T-cell lymphoma project. J Clin Oncol (2013) 31(2):240–6. doi: 10.1200/JCO.2011.37.3647
9. Mourad N, Mounier N, Briere J, Raffoux E, Delmer A, Feller A, et al. Clinical, biologic, and pathologic features in 157 patients with angioimmunoblastic T-cell lymphoma treated within the groupe D'etude des lymphomes de L'adulte (Gela) trials. Blood (2008) 111(9):4463–70. doi: 10.1182/blood-2007-08-105759
10. Advani RH, Skrypets T, Civallero M, Spinner MA, Manni M, Kim WS, et al. Outcomes and prognostic factors in angioimmunoblastic T-cell lymphoma: final report from the international T-cell project. Blood (2021) 138(3):213–20. doi: 10.1182/blood.2020010387
11. Lemonnier F, Couronne L, Parrens M, Jais JP, Travert M, Lamant L, et al. Recurrent tet2 mutations in peripheral T-cell lymphomas correlate with tfh-like features and adverse clinical parameters. Blood (2012) 120(7):1466–9. doi: 10.1182/blood-2012-02-408542
12. Palomero T, Couronne L, Khiabanian H, Kim MY, Ambesi-Impiombato A, Perez-Garcia A, et al. Recurrent mutations in epigenetic regulators, rhoa and fyn kinase in peripheral T cell lymphomas. Nat Genet (2014) 46(2):166–70. doi: 10.1038/ng.2873
13. Odejide O, Weigert O, Lane AA, Toscano D, Lunning MA, Kopp N, et al. A targeted mutational landscape of angioimmunoblastic T-cell lymphoma. Blood (2014) 123(9):1293–6. doi: 10.1182/blood-2013-10-531509
14. Quivoron C, Couronne L, Della Valle V, Lopez CK, Plo I, Wagner-Ballon O, et al. Tet2 inactivation results in pleiotropic hematopoietic abnormalities in mouse and is a recurrent event during human lymphomagenesis. Cancer Cell (2011) 20(1):25–38. doi: 10.1016/j.ccr.2011.06.003
15. Couronne L, Bastard C, Bernard OA. Tet2 and dnmt3a mutations in human T-cell lymphoma. N Engl J Med (2012) 366(1):95–6. doi: 10.1056/NEJMc1111708
16. Cairns RA, Iqbal J, Lemonnier F, Kucuk C, de Leval L, Jais JP, et al. Idh2 mutations are frequent in angioimmunoblastic T-cell lymphoma. Blood (2012) 119(8):1901–3. doi: 10.1182/blood-2011-11-391748
17. Ito S, D'Alessio AC, Taranova OV, Hong K, Sowers LC, Zhang Y. Role of tet proteins in 5mc to 5hmc conversion, es-cell self-renewal and inner cell mass specification. Nature (2010) 466(7310):1129–33. doi: 10.1038/nature09303
18. Tahiliani M, Koh KP, Shen Y, Pastor WA, Bandukwala H, Brudno Y, et al. Conversion of 5-methylcytosine to 5-hydroxymethylcytosine in mammalian DNA by mll partner tet1. Science (2009) 324(5929):930–5. doi: 10.1126/science.1170116
19. Koh KP, Yabuuchi A, Rao S, Huang Y, Cunniff K, Nardone J, et al. Tet1 and tet2 regulate 5-hydroxymethylcytosine production and cell lineage specification in mouse embryonic stem cells. Cell Stem Cell (2011) 8(2):200–13. doi: 10.1016/j.stem.2011.01.008
20. Ko M, Bandukwala HS, An J, Lamperti ED, Thompson EC, Hastie R, et al. Ten-eleven-translocation 2 (Tet2) negatively regulates homeostasis and differentiation of hematopoietic stem cells in mice. Proc Natl Acad Sci U.S.A. (2011) 108(35):14566–71. doi: 10.1073/pnas.1112317108
21. Moran-Crusio K, Reavie L, Shih A, Abdel-Wahab O, Ndiaye-Lobry D, Lobry C, et al. Tet2 loss leads to increased hematopoietic stem cell self-renewal and myeloid transformation. Cancer Cell (2011) 20(1):11–24. doi: 10.1016/j.ccr.2011.06.001
22. Li Z, Cai X, Cai CL, Wang J, Zhang W, Petersen BE, et al. Deletion of tet2 in mice leads to dysregulated hematopoietic stem cells and subsequent development of myeloid Malignancies. Blood (2011) 118(17):4509–18. doi: 10.1182/blood-2010-12-325241
23. Rasmussen KD, Jia G, Johansen JV, Pedersen MT, Rapin N, Bagger FO, et al. Loss of tet2 in hematopoietic cells leads to DNA hypermethylation of active enhancers and induction of leukemogenesis. Genes Dev (2015) 29(9):910–22. doi: 10.1101/gad.260174.115
24. Izzo F, Lee SC, Poran A, Chaligne R, Gaiti F, Gross B, et al. DNA methylation disruption reshapes the hematopoietic differentiation landscape. Nat Genet (2020) 52(4):378–87. doi: 10.1038/s41588-020-0595-4
25. Tulstrup M, Soerensen M, Hansen JW, Gillberg L, Needhamsen M, Kaastrup K, et al. Tet2 mutations are associated with hypermethylation at key regulatory enhancers in normal and Malignant hematopoiesis. Nat Commun (2021) 12(1):6061. doi: 10.1038/s41467-021-26093-2
26. Carty SA, Gohil M, Banks LB, Cotton RM, Johnson ME, Stelekati E, et al. The loss of tet2 promotes cd8(+) T cell memory differentiation. J Immunol (2018) 200(1):82–91. doi: 10.4049/jimmunol.1700559
27. Baessler A, Novis CL, Shen Z, Perovanovic J, Wadsworth M, Thiede KA, et al. Tet2 coordinates with foxo1 and runx1 to balance T follicular helper cell and T helper 1 cell differentiation. Sci Adv (2022) 8(24):eabm4982. doi: 10.1126/sciadv.abm4982
28. Delhommeau F, Dupont S, Della Valle V, James C, Trannoy S, Masse A, et al. Mutation in tet2 in myeloid cancers. N Engl J Med (2009) 360(22):2289–301. doi: 10.1056/NEJMoa0810069
29. Tefferi A, Lim KH, Abdel-Wahab O, Lasho TL, Patel J, Patnaik MM, et al. Detection of mutant tet2 in myeloid Malignancies other than myeloproliferative neoplasms: cmml, mds, mds/mpn and aml. Leukemia (2009) 23(7):1343–5. doi: 10.1038/leu.2009.59
30. Lopez C, Bergmann AK, Paul U, Murga Penas EM, Nagel I, Betts MJ, et al. Genes encoding members of the jak-stat pathway or epigenetic regulators are recurrently mutated in T-cell prolymphocytic leukaemia. Br J Haematol (2016) 173(2):265–73. doi: 10.1111/bjh.13952
31. Shimoda K, Shide K, Kameda T, Hidaka T, Kubuki Y, Kamiunten A, et al. Tet2 mutation in adult T-cell leukemia/lymphoma. J Clin Exp Hematop (2015) 55(3):145–9. doi: 10.3960/jslrt.55.145
32. Marcais A, Waast L, Bruneau J, Hanssens K, Asnafi V, Gaulard P, et al. Adult T cell leukemia aggressivenness correlates with loss of both 5-hydroxymethylcytosine and tet2 expression. Oncotarget (2017) 8(32):52256–68. doi: 10.18632/oncotarget.13665
33. da Silva Almeida AC, Abate F, Khiabanian H, Martinez-Escala E, Guitart J, Tensen CP, et al. The mutational landscape of cutaneous T cell lymphoma and sezary syndrome. Nat Genet (2015) 47(12):1465–70. doi: 10.1038/ng.3442
34. Chang LW, Patrone CC, Yang W, Rabionet R, Gallardo F, Espinet B, et al. An integrated data resource for genomic analysis of cutaneous T-cell lymphoma. J Invest Dermatol (2018) 138(12):2681–3. doi: 10.1016/j.jid.2018.06.176
35. Park J, Yang J, Wenzel AT, Ramachandran A, Lee WJ, Daniels JC, et al. Genomic analysis of 220 ctcls identifies a novel recurrent gain-of-function alteration in rltpr (P.Q575e). Blood (2017) 130(12):1430–40. doi: 10.1182/blood-2017-02-768234
36. Ward PS, Patel J, Wise DR, Abdel-Wahab O, Bennett BD, Coller HA, et al. The common feature of leukemia-associated idh1 and idh2 mutations is a neomorphic enzyme activity converting alpha-ketoglutarate to 2-hydroxyglutarate. Cancer Cell (2010) 17(3):225–34. doi: 10.1016/j.ccr.2010.01.020
37. Xu W, Yang H, Liu Y, Yang Y, Wang P, Kim SH, et al. Oncometabolite 2-hydroxyglutarate is a competitive inhibitor of alpha-ketoglutarate-dependent dioxygenases. Cancer Cell (2011) 19(1):17–30. doi: 10.1016/j.ccr.2010.12.014
38. Wang C, McKeithan TW, Gong Q, Zhang W, Bouska A, Rosenwald A, et al. Idh2r172 mutations define a unique subgroup of patients with angioimmunoblastic T-cell lymphoma. Blood (2015) 126(15):1741–52. doi: 10.1182/blood-2015-05-644591
39. Muto H, Sakata-Yanagimoto M, Nagae G, Shiozawa Y, Miyake Y, Yoshida K, et al. Reduced tet2 function leads to T-cell lymphoma with follicular helper T-cell-like features in mice. Blood Cancer J (2014) 4:e264. doi: 10.1038/bcj.2014.83
40. Sakata-Yanagimoto M, Enami T, Yoshida K, Shiraishi Y, Ishii R, Miyake Y, et al. Somatic rhoa mutation in angioimmunoblastic T cell lymphoma. Nat Genet (2014) 46(2):171–5. doi: 10.1038/ng.2872
41. Yoo HY, Sung MK, Lee SH, Kim S, Lee H, Park S, et al. A recurrent inactivating mutation in rhoa gtpase in angioimmunoblastic T cell lymphoma. Nat Genet (2014) 46(4):371–5. doi: 10.1038/ng.2916
42. Zang S, Li J, Yang H, Zeng H, Han W, Zhang J, et al. Mutations in 5-methylcytosine oxidase tet2 and rhoa cooperatively disrupt T cell homeostasis. J Clin Invest (2017) 127(8):2998–3012. doi: 10.1172/JCI92026
43. Ng SY, Brown L, Stevenson K, deSouza T, Aster JC, Louissaint A Jr., et al. Rhoa G17v is sufficient to induce autoimmunity and promotes T-cell lymphomagenesis in mice. Blood (2018) 132(9):935–47. doi: 10.1182/blood-2017-11-818617
44. Cortes JR, Ambesi-Impiombato A, Couronne L, Quinn SA, Kim CS, da Silva Almeida AC, et al. Rhoa G17v induces T follicular helper cell specification and promotes lymphomagenesis. Cancer Cell (2018) 33(2):259–73 e7. doi: 10.1016/j.ccell.2018.01.001
45. Nguyen TB, Sakata-Yanagimoto M, Fujisawa M, Nuhat ST, Miyoshi H, Nannya Y, et al. Dasatinib is an effective treatment for angioimmunoblastic T-cell lymphoma. Cancer Res (2020) 80(9):1875–84. doi: 10.1158/0008-5472.CAN-19-2787
46. Jaiswal S, Fontanillas P, Flannick J, Manning A, Grauman PV, Mar BG, et al. Age-related clonal hematopoiesis associated with adverse outcomes. N Engl J Med (2014) 371(26):2488–98. doi: 10.1056/NEJMoa1408617
47. Busque L, Patel JP, Figueroa ME, Vasanthakumar A, Provost S, Hamilou Z, et al. Recurrent somatic tet2 mutations in normal elderly individuals with clonal hematopoiesis. Nat Genet (2012) 44(11):1179–81. doi: 10.1038/ng.2413
48. Genovese G, Kahler AK, Handsaker RE, Lindberg J, Rose SA, Bakhoum SF, et al. Clonal hematopoiesis and blood-cancer risk inferred from blood DNA sequence. N Engl J Med (2014) 371(26):2477–87. doi: 10.1056/NEJMoa1409405
49. Nguyen TB, Sakata-Yanagimoto M, Asabe Y, Matsubara D, Kano J, Yoshida K, et al. Identification of cell-type-specific mutations in nodal T-cell lymphomas. Blood Cancer J (2017) 7(1):e516. doi: 10.1038/bcj.2016.122
50. Schwartz FH, Cai Q, Fellmann E, Hartmann S, Mayranpaa MI, Karjalainen-Lindsberg ML, et al. Tet2 mutations in B cells of patients affected by angioimmunoblastic T-cell lymphoma. J Pathol (2017) 242(2):129–33. doi: 10.1002/path.4898
51. Lewis NE, Petrova-Drus K, Huet S, Epstein-Peterson ZD, Gao Q, Sigler AE, et al. Clonal hematopoiesis in angioimmunoblastic T-cell lymphoma with divergent evolution to myeloid neoplasms. Blood Adv (2020) 4(10):2261–71. doi: 10.1182/bloodadvances.2020001636
52. Tsiouplis NJ, Bailey DW, Chiou LF, Wissink FJ, Tsagaratou A. Tet-mediated epigenetic regulation in immune cell development and disease. Front Cell Dev Biol (2020) 8:623948. doi: 10.3389/fcell.2020.623948
53. Ko M, Huang Y, Jankowska AM, Pape UJ, Tahiliani M, Bandukwala HS, et al. Impaired hydroxylation of 5-methylcytosine in myeloid cancers with mutant tet2. Nature (2010) 468(7325):839–43. doi: 10.1038/nature09586
54. Zhang Q, Zhao K, Shen Q, Han Y, Gu Y, Li X, et al. Tet2 is required to resolve inflammation by recruiting hdac2 to specifically repress il-6. Nature (2015) 525(7569):389–93. doi: 10.1038/nature15252
55. Cull AH, Snetsinger B, Buckstein R, Wells RA, Rauh MJ. Tet2 restrains inflammatory gene expression in macrophages. Exp Hematol (2017) 55:56–70 e13. doi: 10.1016/j.exphem.2017.08.001
56. Pan W, Zhu S, Qu K, Meeth K, Cheng J, He K, et al. The DNA methylcytosine dioxygenase tet2 sustains immunosuppressive function of tumor-infiltrating myeloid cells to promote melanoma progression. Immunity (2017) 47(2):284–97 e5. doi: 10.1016/j.immuni.2017.07.020
57. Ichiyama K, Chen T, Wang X, Yan X, Kim BS, Tanaka S, et al. The methylcytosine dioxygenase tet2 promotes DNA demethylation and activation of cytokine gene expression in T cells. Immunity (2015) 42(4):613–26. doi: 10.1016/j.immuni.2015.03.005
58. Yue X, Lio CJ, Samaniego-Castruita D, Li X, Rao A. Loss of tet2 and tet3 in regulatory T cells unleashes effector function. Nat Commun (2019) 10(1):2011. doi: 10.1038/s41467-019-09541-y
59. Yang R, Qu C, Zhou Y, Konkel JE, Shi S, Liu Y, et al. Hydrogen sulfide promotes tet1- and tet2-mediated foxp3 demethylation to drive regulatory T cell differentiation and maintain immune homeostasis. Immunity (2015) 43(2):251–63. doi: 10.1016/j.immuni.2015.07.017
60. Wing JB, Tanaka A, Sakaguchi S. Human foxp3(+) regulatory T cell heterogeneity and function in autoimmunity and cancer. Immunity (2019) 50(2):302–16. doi: 10.1016/j.immuni.2019.01.020
61. Fujisawa M, Nguyen TB, Abe Y, Suehara Y, Fukumoto K, Suma S, et al. Clonal germinal center B cells function as a niche for T-cell lymphoma. Blood (2022) 140(18):1937–50. doi: 10.1182/blood.2022015451
62. Lemonnier F, Mak TW. Angioimmunoblastic T-cell lymphoma: more than a disease of T follicular helper cells. J Pathol (2017) 242(4):387–90. doi: 10.1002/path.4920
63. Pro B, Horwitz SM, Prince HM, Foss FM, Sokol L, Greenwood M, et al. Romidepsin induces durable responses in patients with relapsed or refractory angioimmunoblastic T-cell lymphoma. Hematol Oncol (2017) 35(4):914–7. doi: 10.1002/hon.2320
64. Sawas A, Ma H, Shustov A, Hsu P, Bhat G, Acosta M, et al. Characterizing the belinostat response in patients with relapsed or refractory angioimmunoblastic T-cell lymphoma. Leuk Lymphoma (2020) 61(8):2003–7. doi: 10.1080/10428194.2020.1753044
65. Ghione P, Faruque P, Mehta-Shah N, Seshan V, Ozkaya N, Bhaskar S, et al. T follicular helper phenotype predicts response to histone deacetylase inhibitors in relapsed/refractory peripheral T-cell lymphoma. Blood Adv (2020) 4(19):4640–7. doi: 10.1182/bloodadvances.2020002396
66. Cheminant M, Bruneau J, Kosmider O, Lefrere F, Delarue R, Gaulard P, et al. Efficacy of 5-azacytidine in a tet2 mutated angioimmunoblastic T cell lymphoma. Br J Haematol (2015) 168(6):913–6. doi: 10.1111/bjh.13170
67. Tobiasson M, Pandzic T, Cavelier L, Sander B, Wahlin BE. Angioimmunoblastic T-cell lymphoma and myelodysplastic syndrome with mutations in tet2, dnmt3 and cux1 - azacitidine induces only lymphoma remission. Leuk Lymphoma (2019) 60(13):3316–9. doi: 10.1080/10428194.2019.1627541
68. Saillard C, Guermouche H, Derrieux C, Bruneau J, Frenzel L, Couronne L, et al. Response to 5-azacytidine in a patient with tet2-mutated angioimmunoblastic T-cell lymphoma and chronic myelomonocytic leukaemia preceded by an ebv-positive large B-cell lymphoma. Hematol Oncol (2017) 35(4):864–8. doi: 10.1002/hon.2319
69. Yoon SE, Cho J, Kim YJ, Kim SJ, Kim WS. Real-world efficacy of 5-azacytidine as salvage chemotherapy for angioimmunoblastic T-cell lymphoma. Clin Lymphoma Myeloma Leuk (2022) 22(11):e972–e80. doi: 10.1016/j.clml.2022.07.009
70. Marchi E, Zullo KM, Amengual JE, Kalac M, Bongero D, McIntosh CM, et al. The combination of hypomethylating agents and histone deacetylase inhibitors produce marked synergy in preclinical models of T-cell lymphoma. Br J Haematol (2015) 171(2):215–26. doi: 10.1111/bjh.13566
71. Scotto L, Kinahan C, Douglass E, Deng C, Safari M, Casadei B, et al. Targeting the T-cell lymphoma epigenome induces cell death, cancer testes antigens, immune-modulatory signaling pathways. Mol Cancer Ther (2021) 20(8):1422–30. doi: 10.1158/1535-7163.MCT-20-0377
72. Falchi L, Ma H, Klein S, Lue JK, Montanari F, Marchi E, et al. Combined oral 5-azacytidine and romidepsin are highly effective in patients with ptcl: A multicenter phase 2 study. Blood (2021) 137(16):2161–70. doi: 10.1182/blood.2020009004
73. Chihara D, Fanale MA, Miranda RN, Noorani M, Westin JR, Nastoupil LJ, et al. The survival outcome of patients with relapsed/refractory peripheral T-cell lymphoma-not otherwise specified and angioimmunoblastic T-cell lymphoma. Br J Haematol (2017) 176(5):750–8. doi: 10.1111/bjh.14477
74. Bachy E, Camus V, Thieblemont C, Sibon D, Casasnovas RO, Ysebaert L, et al. Romidepsin plus chop versus chop in patients with previously untreated peripheral T-cell lymphoma: results of the ro-chop phase iii study (Conducted by lysa). J Clin Oncol (2022) 40(3):242–51. doi: 10.1200/JCO.21.01815
Keywords: TET2, angioimmunoblastic T cell lymphoma, T follicular helper cell lymphoma, epigenetic therapy, peripheral T cell lymphoma (PTCL)
Citation: Carty SA (2023) Biological insights into the role of TET2 in T cell lymphomas. Front. Oncol. 13:1199108. doi: 10.3389/fonc.2023.1199108
Received: 02 April 2023; Accepted: 18 September 2023;
Published: 29 September 2023.
Edited by:
Juliana Pereira, University of São Paulo, BrazilReviewed by:
Jean Feuillard, University of Limoges, FranceJaveed Iqbal, University of Nebraska Medical Center, United States
Copyright © 2023 Carty. This is an open-access article distributed under the terms of the Creative Commons Attribution License (CC BY). The use, distribution or reproduction in other forums is permitted, provided the original author(s) and the copyright owner(s) are credited and that the original publication in this journal is cited, in accordance with accepted academic practice. No use, distribution or reproduction is permitted which does not comply with these terms.
*Correspondence: Shannon A. Carty, c2NhcnR5QHVtaWNoLmVkdQ==