- 1Department of Thyroid and Breast Surgery, The Fourth People’s Hospital of Jinan, Jinan, China
- 2Department of Breast Surgery, The Second Hospital of Jilin University, Changchun, China
- 3Department of Thyroid and Breast Surgery, Central Hospital Affiliated to Shandong First Medical University, Jinan, China
Breast cancer stands as the most prevalent malignancy globally. Despite the array of treatment options, its mortality rate continues to rise annually. Thus, reevaluation of factors directly linked to breast cancer emergence is imperative, alongside the development of more effective preventive measures. Estrogen levels, profoundly tied to reproduction, play a pivotal role in breast cancer development. Speculation centers on the potential of breastfeeding to mitigate cancer risk in women. However, the precise mechanism remains elusive. Breastfeeding is a modifiable reproductive factor extensively studied. Studies highlight a direct connection between lack of breastfeeding and breast cancer emergence, potentially linked to DNA methyltransferase expression alteration, aberrant methylation levels, pregnancy-associated plasma protein-A, cellular microenvironment, and oncogenes. This study reviews recent mechanisms underlying breastfeeding’s role in reducing breast cancer incidence.
1 Background
Statistics from GLOBLCAN 2020 underscore breast cancer’s ascension as the world’s most prevalent cancer type, surpassing lung cancer (1). Developed regions like Western and Northern Europe exhibit nearly 88% higher breast cancer incidence rates than underdeveloped counterparts (East and Central Africa). This global shift necessitates consideration of known risk factors against the backdrop of cancer incidence rate alterations. Notably, age emerges as a primary risk factor, with older females witnessing the highest age-specific incidence rates. Average youthfulness in underdeveloped nations is shaped by aging populations and a ten-year drop in life expectancy. Economic growth in these areas corresponds with elevated life expectancies, indicating imminent cancer incidence rate increases (1, 2). Reproductive and non-reproductive factors, both influenced by economic development, comprise vital risk factors. Reduced menarche age, delayed menopause age, fewer children, and decreased breastfeeding escalate breast cancer risk (3). Improved human development often accelerates menarche onset by enhancing nutritional status, a determinant of menarche initiation age (4). Non-reproductive risk factors include obesity, with breast cancer risk doubling in overweight postmenopausal women. It’s projected that increased alcohol consumption will contribute to about 4% of diagnosed cancer cases in 2020 (5). While potential genetic or hereditary causes like BRCA1 or BRCA2 mutations account for 5-10% of breast cancers, eight out of nine cases lack affected female reproductive systems (6). Moreover, variations in breastfeeding prevalence might underlie observed differences, along with differences in the healthcare systems levels (7). While West African countries report 70% exclusive breastfeeding rates for five consecutive months, European countries average 30% (8). Despite this, even in the US, access to pumping facilities remains limited, constraining breastfeeding duration. Breastfeeding moderately affects breast cancer occurrence; Almasi-Hashiani et al. determined that 27.3% of breast cancer patients developed cancer due to inadequate breastfeeding (9). Notably, US black women exhibit lower breastfeeding rates and nearly double the triple-negative breast cancer (an aggressive subtype) incidence rates compared to white women (10). A Malaysian case-control study involving 7,663 women found that ever-breastfeeding and longer breastfeeding durations correlated with reduced breast cancer risk (11). The study further suggested that breastfeeding for under three months raised breast cancer risk, while exceeding 12 months lowered risk (12, 13). This raises speculation regarding breastfeeding’s potential to mitigate cancer risk via (1) breast cell differentiation, modifying them for post-production milk production, thereby reducing breast tissue vulnerability to carcinogenesis (estrogen), and (2) impeding ovulation by diminishing estrogen’s mitosis-promoting effect (14). Carcinogen secretion in breast milk and breast tissue shedding contribute to damaged DNA cell elimination, curtailing mutation responsiveness (15). However, the precise mechanism remains uncertain. Understanding how breastfeeding mechanisms impact breast cancer can identify pharmacologic preventive measures for non-breastfeeding women, curbing breast cancer prevalence and mortality. Additionally, these findings might inform novel treatment approaches. Therefore, this review consolidates recent discoveries concerning pertinent mechanisms and processes.
2 Breastfeeding and DNA methylation
DNA methylation, an epigenetic modification prevalent in mammals, is catalyzed by DNA methyltransferase enzymes, including DNMT1, DNMT3a, and DNMT3b (16). This alteration affects DNA’s transcriptional participation, leading to gene silencing and diminished transcriptional capacity. Elevated DNA methyltransferase content was observed in breast cancer patients (17).
Forkhead box protein A1 (Fox A1), a transcription factor, fosters luminal progenitor cell differentiation into mature luminal cells during breast development while repressing the basal phenotype (18). Highly methylated in BRCA-1related breast cancers (19), Fox A1’s methylation possibly stems from BRCA-1 gene control over Fox A1 expression via methyltransferase inhibition (20). Conversely, BRCA-1 gene mutation or silencing abolishes this inhibitory effect, enabling Fox A1 hypermethylation, fostering breast cancer (21).
Contrastingly, parous women with breastfeeding history exhibit lower Fox A1 methylation, while non-breastfeeding induces Fox A1 hypermethylation akin to BRCA-1 mutation effects (21, 22). Two protective breastfeeding mechanisms include pregnancy and exosomes. Pregnancy triggers mammary epithelial cell DNA methylation for subsequent lactation readiness (23). Prolactin, during lactation, stimulates mammary epithelial cell milk protein and lipid synthesis, demethylating breastfeeding-related genes (24). Breast milk’s exosomes, small vesicles rich in various molecules, including proteins, lipids, and microRNAs, exert significant physiological and pathological influence (25). In breast tissue, exosomes govern lactation and mammary gland involution (26). Breast milk’s exosomes contain abundant mir-29s and mir-148a, downregulating methyltransferases DNMT3/b and DNMT1 (27, 28). In vitro studies confirm epithelial cell exosome uptake and maintenance of functionality (29). Breastfeeding’s absence potentially reduces mammary epithelial cell exosome exposure, increasing DNA methyltransferase expression and aberrant methylation (Figure 1).
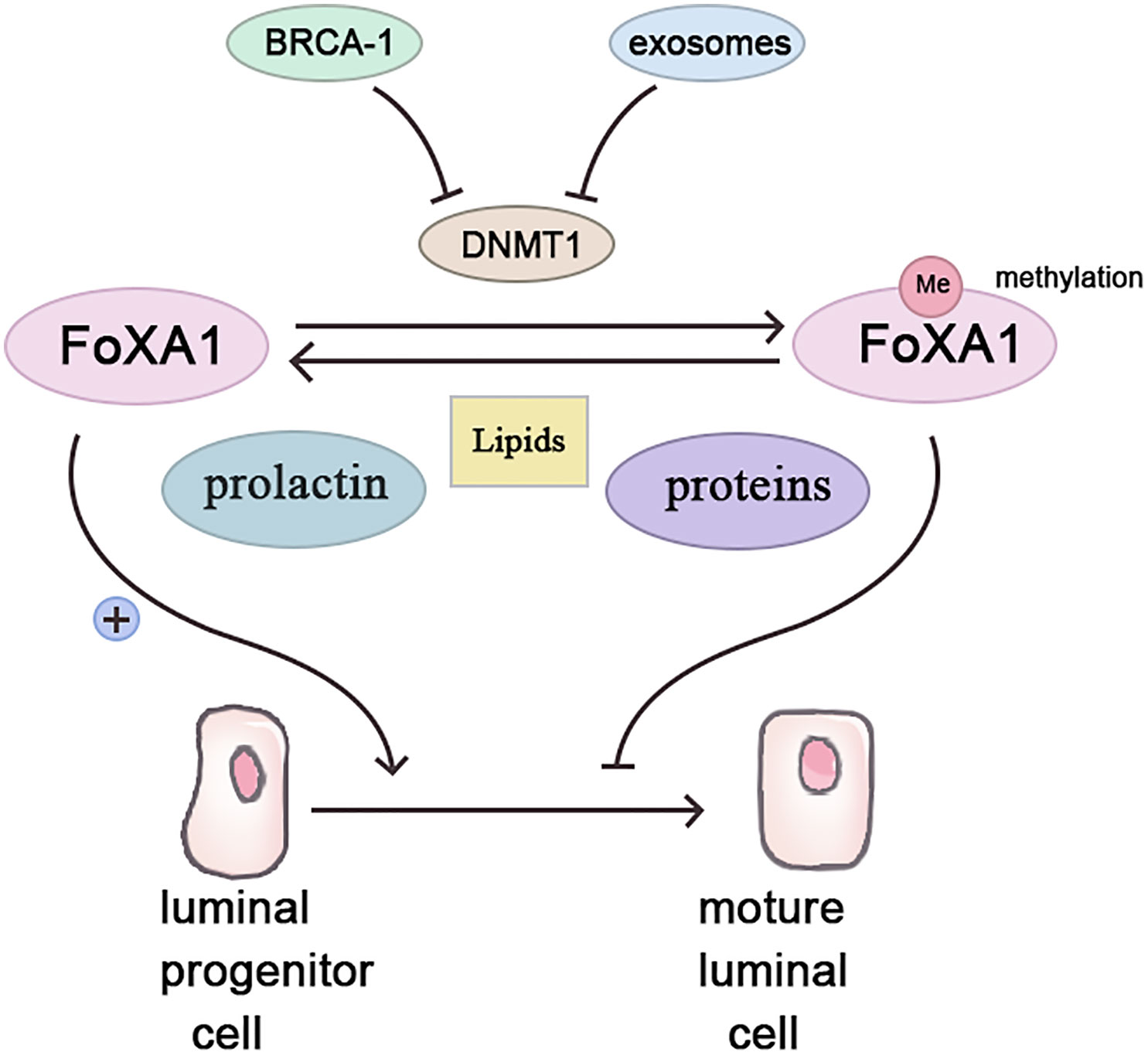
Figure 1 Breastfeeding inhibits the occurrence of breast cancer by affecting the methylation of FoxA1.
3 Breastfeeding and pregnancy-associated plasma protein-A
Pregnancy-associated plasma protein-A (PAPP-A), a metzincin (30)metalloproteinase, is overexpressed in most breast cancer patients (31). Insulin-like growth factor 1 (IGF-1) safeguards mammary epithelium against apoptosis (32). Insulin-like growth factor binding protein-5 (IGFBP-5), a vital mammary gland involution regulator, impedes IGF receptor activation by sequestering IGF-1 (33). Conversely, PAPP-A hampers IGFBP-5 via hydrolysis, delaying breast involution and heightening pregnancy-associated breast cancer (PABC) risk (34). Collagen deposition elevates PAPP-A, enhancing IGFBP-5 cleavage, reinforcing IGF signaling, intensifying collagen deposition, thereby augmenting PABC risk (30, 34).
Moreover, PAPP-A activates collagen receptor DDR2, promoting tumor metastasis via ERK-Snail axis activation. Lack of breastfeeding triggers elevated collagen deposition during breast degeneration, amplifying PAPP-A activity (31, 35). Prolonged breastfeeding duration directly correlates with decreased PAPP-A activity during lactation (35). Glycoproteins Stanniocalcin-1 (STC1) and Stanniocalcin-2 (STC-2) inhibit PAPP-A, preventing PABC development; they are present in breast milk (35). STC, abundant during late pregnancy and lactation, diminishes post-lactation (36). Prolonged breastfeeding heightens STC1 and STC2 levels, inactivating PAPP-A, averting IGFBP-5 cleavage, allowing normal involution. Post-lactation, STC1 and STC2 levels decline, but PAPP-A activity doesn’t recover (35). In vitro studies confirm STC2’s PAPP-proteolytic A suppression through covalent bonding, curtailing PAPP-A-mediated IGF signaling (37). Conversely, STC1, lacking essential cysteine residue, binds PAPP-A with high affinity, sans covalent bonding (38). Absence of breastfeeding may hinder STC1 and STC2 from halting PAPP-A, fostering excess PAPP-A, IGFBP-5 cleavage, IGF signaling feedback, fostering PABC (Figure 2) (35).
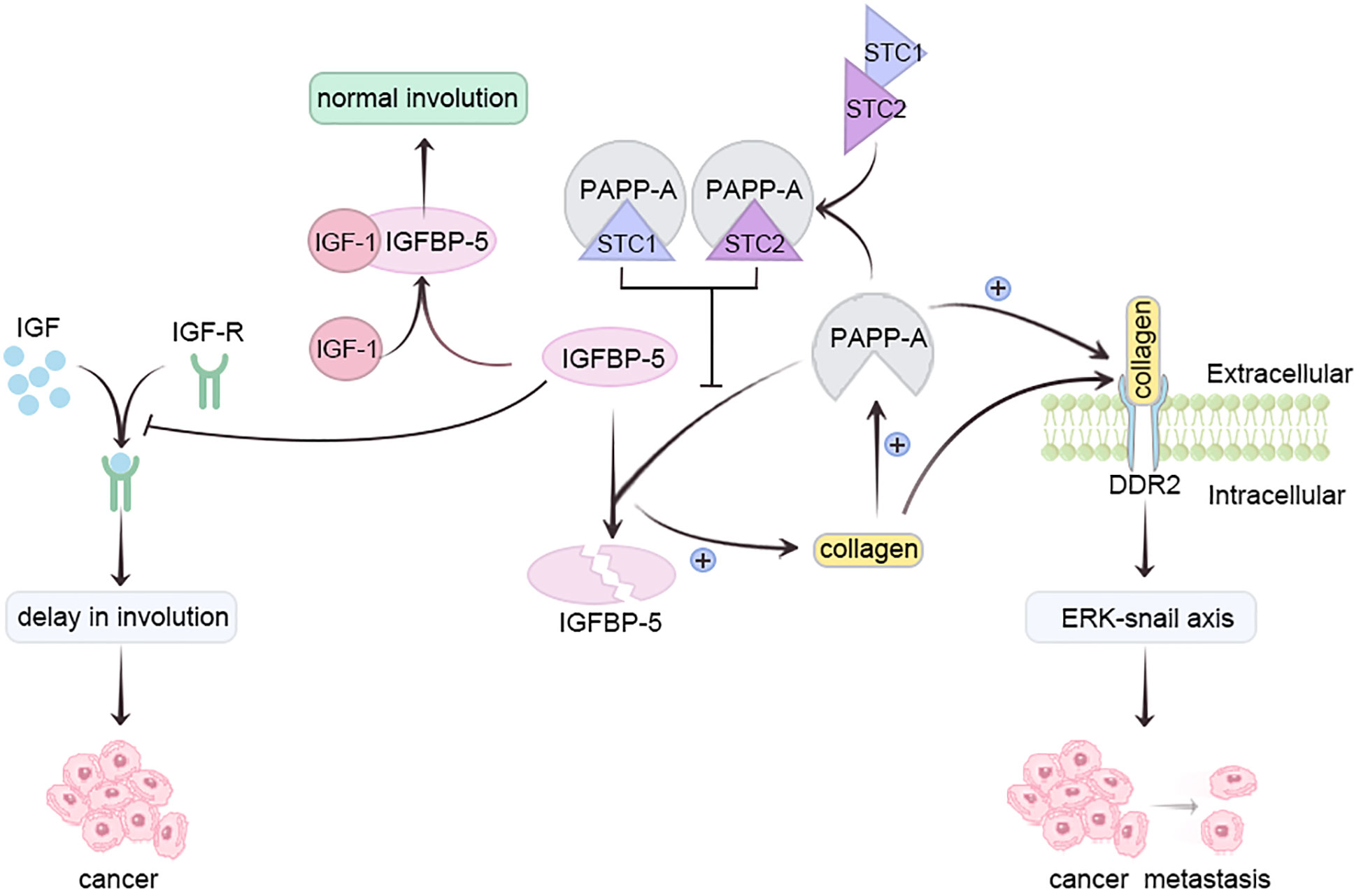
Figure 2 Prolonging breastfeeding duration can reduce the activity of PAPP-A through STC and inhibit the occurrence of breast cancer.
4 Breastfeeding and the cellular microenvironment
Cellular surroundings profoundly influence growth and development. Breast milk markedly curbs cancer cells in the microenvironment. Throughout breastfeeding, calcium ion-rich breast milk inhibits cell apoptosis and necrosis (39, 40). Frequent exposure to elevated calcium ions can disrupt intercellular connections (41). Additionally, breast milk’s Secretory IgA (SIgA) and Lactalbumin Alpha (LALBA) compound suppress breast cancer cell development and induce apoptosis (42).
5 Breastfeeding and oncogenes
Oncogene BRCA1-IRIS, tied to breast cancer, arises from differential BRCA1 locus utilization. IRIS mRNA and protein exhibit significant upregulation in breast tumors (43), and normal breast tissue (44). IRIS overexpression spurs mammary progenitor cell growth, survival during gestational preparation for lactation (45). However, IRIS overexpression also leads to normal mammary epithelial cell differentiation into Triple Negative Breast Cancer (TNBC)-like cells (46). Beyond 12 months of breastfeeding, signaling pathways like VD/VDR/STAT3 elevate, diminishing IRIS expression, fostering mammary epithelial terminal differentiation (45). These cells also serve as tumor-specific peptide-presenting cells, cleared by immune cells upon breastfeeding cessation and involution. Inadequate breastfeeding might result in numerous IRIS-overexpressing progenitor cells during involution (47). Elshamy et al.’s “oncogene elimination hypothesis” elucidates this process (48). Known oncogenes possess normal functions at normal expression levels, sometimes overexpressed at developmental stages for specific roles (49). Breast cells’ initial gestational stage expression rise, sustaining survival, proliferation readiness for subsequent lactation. These cells must exit at breast degeneration’s onset, given inflammatory environment during degeneration. Differentiated cells might die, while progenitor cells thrive, turning more invasive (50). Prolonged breastfeeding induces terminal differentiation, clearing terminally-differentiated cells after involution onset (51). Inadequate breastfeeding might result in immune escape of IRIS-overexpressing progenitor cells during involution, fostering breast cancer development (48).
6 Breastfeeding and involution
Breast tissues undergoing involution after breastfeeding differ from those experiencing immediate involution after pregnancy without breastfeeding (52). During mammary gland involution, a few mammary epithelial cells revert to their pre-pregnancy state, while most undergo programmed death (53). However, the remodeling process varies based on breastfeeding duration. A prospective cohort study with mice revealed that the absence of breastfeeding leads to abrupt breast tissue remodeling, escalating inflammatory marker levels, and collagen deposition. In vivo mouse studies demonstrated that this sudden breast tissue remodeling results in substantial ductal hyperplasia, squamous metaplasia, and sustained elevation of luminal progenitor cells. Though unverified in human studies, these changes enhance cancer development potential (54). Following breastfeeding, breast tissues undergo involution, accompanied by a genetic signature expressing genes linked to apoptotic pathways like p53, c-myc, and BCL-xl. This expression enables efficient carcinogen metabolism and DNA damage repair (10). Inadequate breastfeeding sustains a terminal bud structure in breast tissue after involution, harboring numerous epithelial cells vulnerable to carcinogen stimulation, thereby facilitating breast cancer cell transformation (55).
7 Conclusion
Breastfeeding constitutes a positive, health-promoting behavior, with breastfeeding duration reducing breast cancer risk. Building on multiple breastfeeding-breast cancer associations, we comprehensively outlined mechanisms (Figure 3) through which breastfeeding averts breast cancer development. Numerous questions remain regarding breastfeeding’s role in reducing breast cancer risk. For instance, how much breastfeeding is required to mitigate risk? Is three months sufficient? Is the first or last pregnancy more pivotal, or is complete breastfeeding month duration key to risk reduction? Addressing these queries mandates extensive epidemiological studies providing detailed reproductive and breastfeeding histories, coupled with laboratory research illuminating these variables’ impact on breast cell populations. Future population-based inquiries must consider potential confounding effects of menarche age on breastfeeding associations, alongside possible interactions with other lifestyle factors such as oral contraceptive use, alcohol consumption, and body mass index. Enhanced understanding of breastfeeding’s impact on breast cancer mechanisms might uncover preventive pharmaceutical options for women unable or unwilling to breastfeed, curtailing cancer prevalence and its mortality, thereby setting our research’s future trajectory.
Author contributions
YC: Conceptualization, Data curation, Resources, Writing – original draft. PJ: Data curation, Resources, Writing – review & editing. YG: Writing – review & editing.
Funding
The authors declare that no financial support was received for the research, authorship, and/or publication of this article.
Conflict of interest
The authors declare that the research was conducted in the absence of any commercial or financial relationships that could be construed as a potential conflict of interest.
Publisher’s note
All claims expressed in this article are solely those of the authors and do not necessarily represent those of their affiliated organizations, or those of the publisher, the editors and the reviewers. Any product that may be evaluated in this article, or claim that may be made by its manufacturer, is not guaranteed or endorsed by the publisher.
Abbreviations
Fox A1, Forkhead box protein A1; PAPP-A, Pregnancy-associated plasma protein-A; IGF-1, Insulin-like growth factor 1; IGFBP-5, Insulin-like growth factor binding protein-5; IGF, Insulin-like growth factor; PABC, pregnancy-associated breast cancer; STC1, Stanniocalcin-1; STC2, Stanniocalcin-2; STC, Stanniocalcin; SIgA, Secretory IgA; LALBA, Lactalbumin Alpha; TNBC, Triple Negative Breast Cancer.
References
1. Sung H, Ferlay J, Siegel RL, Laversanne M, Soerjomataram I, Jemal A, et al. Global cancer statistics 2020: globocan estimates of incidence and mortality worldwide for 36 cancers in 185 countries. CA Cancer J Clin (2021) 71(3):209–49. doi: 10.3322/caac.21660
2. Heer E, Harper A, Escandor N, Sung H, McCormack V, Fidler-Benaoudia MM. Global burden and trends in premenopausal and postmenopausal breast cancer: A population-based study. Lancet Glob Health (2020) 8(8):e1027–e37. doi: 10.1016/s2214-109x(20)30215-1
3. Collaborative Group on Hormonal Factors in Breast Cancer. Breast cancer and breastfeeding: collaborative reanalysis of individual data from 47 epidemiological studies in 30 countries, including 50302 women with breast cancer and 96973 women without the disease. Lancet (2002) 360(9328):187–95. doi: 10.1016/s0140-6736(02)09454-0
4. Key T, Appleby P, Barnes I, Reeves G. Endogenous sex hormones and breast cancer in postmenopausal women: reanalysis of nine prospective studies. J Natl Cancer Inst (2002) 94(8):606–16. doi: 10.1093/jnci/94.8.606
5. Rumgay H, Shield K, Charvat H, Ferrari P, Sornpaisarn B, Obot I, et al. Global burden of cancer in 2020 attributable to alcohol consumption: A population-based study. Lancet Oncol (2021) 22(8):1071–80. doi: 10.1016/s1470-2045(21)00279-5
6. Collaborative Group on Hormonal Factors in Breast Cancer. Familial breast cancer: collaborative reanalysis of individual data from 52 epidemiological studies including 58,209 women with breast cancer and 101,986 women without the disease. Lancet (2001) 358(9291):1389–99. doi: 10.1016/s0140-6736(01)06524-2
7. Denny L, de Sanjose S, Mutebi M, Anderson BO, Kim J, Jeronimo J, et al. Interventions to close the divide for women with breast and cervical cancer between low-income and middle-income countries and high-income countries. Lancet (2017) 389(10071):861–70. doi: 10.1016/s0140-6736(16)31795-0
8. Victora CG, Bahl R, Barros AJ, França GV, Horton S, Krasevec J, et al. Breastfeeding in the 21st century: epidemiology, mechanisms, and lifelong effect. Lancet (2016) 387(10017):475–90. doi: 10.1016/s0140-6736(15)01024-7
9. Almasi-Hashiani A, Nedjat S, Ghiasvand R, Safiri S, Nazemipour M, Mansournia N, et al. The causal effect and impact of reproductive factors on breast cancer using super learner and targeted maximum likelihood estimation: A case-control study in Fars province, Iran. BMC Public Health (2021) 21(1):1219. doi: 10.1186/s12889-021-11307-5
10. Anstey EH, Shoemaker ML, Barrera CM, O’Neil ME, Verma AB, Holman DM. Breastfeeding and breast cancer risk reduction: implications for black mothers. Am J Prev Med (2017) 53(3s1):S40–s6. doi: 10.1016/j.amepre.2017.04.024
11. Tan MM, Ho WK, Yoon SY, Mariapun S, Hasan SN, Lee DS, et al. A case-control study of breast cancer risk factors in 7,663 women in Malaysia. PloS One (2018) 13(9):e0203469. doi: 10.1371/journal.pone.0203469
12. Dydjow-Bendek DA, Zagożdżon P. Early alcohol use initiation, obesity, not breastfeeding, and residence in a rural area as risk factors for breast cancer: A case-control study. Cancers (Basel) (2021) 13(16):3925. doi: 10.3390/cancers13163925
13. Mejri N, El Benna H, Rachdi H, Labidi S, Benna M, Daoud N, et al. Reproductive risk factors of inflammatory breast cancer according to luminal, her2-overexpressing, and triple-negative subtypes: A case comparison study. Oncol Res Treat (2020) 43(5):204–10. doi: 10.1159/000506691
14. Beaber EF, Holt VL, Malone KE, Porter PL, Daling JR, Li CI. Reproductive factors, age at maximum height, and risk of three histologic types of breast cancer. Cancer Epidemiol Biomarkers Prev (2008) 17(12):3427–34. doi: 10.1158/1055-9965.Epi-08-0641
15. Xing P, Li J, Jin F. A case-control study of reproductive factors associated with subtypes of breast cancer in Northeast China. Med Oncol (2010) 27(3):926–31. doi: 10.1007/s12032-009-9308-7
16. Sharma S, Kelly TK, Jones PA. Epigenetics in cancer. Carcinogenesis (2010) 31(1):27–36. doi: 10.1093/carcin/bgp220
17. Hazra A, Bose P, Sunita P, Pattanayak SP. Molecular epigenetic dynamics in breast carcinogenesis. Arch Pharm Res (2021) 44(8):741–63. doi: 10.1007/s12272-021-01348-0
18. Arruabarrena-Aristorena A, Maag JLV, Kittane S, Cai Y, Karthaus WR, Ladewig E, et al. Foxa1 mutations reveal distinct chromatin profiles and influence therapeutic response in breast cancer. Cancer Cell (2020) 38(4):534–50.e9. doi: 10.1016/j.ccell.2020.08.003
19. Cheng TD, Yao S, Omilian AR, Khoury T, Buas MF, Payne-Ondracek R, et al. Foxa1 protein expression in er(+) and er(-) breast cancer in relation to parity and breastfeeding in black and white women. Cancer Epidemiol Biomarkers Prev (2020) 29(2):379–85. doi: 10.1158/1055-9965.Epi-19-0787
20. Wang C, Qu L, Li S, Yin F, Ji L, Peng W, et al. Discovery of first-in-class dual parp and ezh2 inhibitors for triple-negative breast cancer with wild-type brca. J Med Chem (2021) 64(17):12630–50. doi: 10.1021/acs.jmedchem.1c00567
21. Sribenja S, Maguire O, Attwood K, Buas MF, Palmer JR, Ambrosone CB, et al. Deletion of foxa1 in the mouse mammary gland results in abnormal accumulation of luminal progenitor cells: A link between reproductive factors and er-/tnbc breast cancer? Am J Cancer Res (2021) 11(6):3263–70.
22. Ambrosone CB, Higgins MJ. Relationships between breast feeding and breast cancer subtypes: lessons learned from studies in humans and in mice. Cancer Res (2020) 80(22):4871–7. doi: 10.1158/0008-5472.Can-20-0077
23. Melnik BC, Schmitz G. Milk’s role as an epigenetic regulator in health and disease. Diseases (2017) 5(1):12. doi: 10.3390/diseases5010012
24. Chen Z, Luo J, Zhang C, Ma Y, Sun S, Zhang T, et al. Mechanism of prolactin inhibition of mir-135b via methylation in goat mammary epithelial cells. J Cell Physiol (2018) 233(1):651–62. doi: 10.1002/jcp.25925
25. Tkach M, Théry C. Communication by extracellular vesicles: where we are and where we need to go. Cell (2016) 164(6):1226–32. doi: 10.1016/j.cell.2016.01.043
26. Hendrix A, Hume AN. Exosome signaling in mammary gland development and cancer. Int J Dev Biol (2011) 55(7-9):879–87. doi: 10.1387/ijdb.113391ah
27. Bian Y, Lei Y, Wang C, Wang J, Wang L, Liu L, et al. Epigenetic regulation of mir-29s affects the lactation activity of dairy cow mammary epithelial cells. J Cell Physiol (2015) 230(9):2152–63. doi: 10.1002/jcp.24944
28. Braconi C, Huang N, Patel T. Microrna-dependent regulation of DNA methyltransferase-1 and tumor suppressor gene expression by interleukin-6 in human Malignant cholangiocytes. Hepatology (2010) 51(3):881–90. doi: 10.1002/hep.23381
29. Qin W, Tsukasaki Y, Dasgupta S, Mukhopadhyay N, Ikebe M, Sauter ER. Exosomes in human breast milk promote emt. Clin Cancer Res (2016) 22(17):4517–24. doi: 10.1158/1078-0432.Ccr-16-0135
30. Bale LK, Schafer MJ, Atkinson EJ, Le Brasseur NK, Haak AJ, Oxvig C, et al. Pregnancy-associated plasma protein-a (Papp-a) is a key component of an interactive cellular mechanism promoting pulmonary fibrosis. J Cell Physiol (2022) 237(4):2220–9. doi: 10.1002/jcp.30687
31. Borges VF, Lyons TR, Germain D, Schedin P. Postpartum involution and cancer: an opportunity for targeted breast cancer prevention and treatments? Cancer Res (2020) 80(9):1790–8. doi: 10.1158/0008-5472.Can-19-3448
32. Cao H, Huang G, Fu H, Zhang N. 14-3-3β Is essential for milk composition stimulated by leu/igf-1 via igf1r signaling pathway in bmecs. In Vitro Cell Dev Biol Anim (2022) 58(5):384–95. doi: 10.1007/s11626-022-00682-x
33. Jenkins EC, Brown SO, Germain D. The multi-faced role of papp-a in post-partum breast cancer: igf-signaling is only the beginning. J Mammary Gland Biol Neoplasia (2020) 25(3):181–9. doi: 10.1007/s10911-020-09456-1
34. Zhang J, Zhang Y, Li L, Nian Y, Chen Y, Shen R, et al. Pregnancy-associated plasma protein-a (Pappa) promotes breast cancer progression. Bioengineered (2022) 13(1):291–307. doi: 10.1080/21655979.2021.2000724
35. Takabatake Y, Oxvig C, Nagi C, Adelson K, Jaffer S, Schmidt H, et al. Lactation opposes pappalysin-1-driven pregnancy-associated breast cancer. EMBO Mol Med (2016) 8(4):388–406. doi: 10.15252/emmm.201606273
36. Bishop A, Cartwright JE, Whitley GS. Stanniocalcin-1 in the female reproductive system and pregnancy. Hum Reprod Update (2021) 27(6):1098–114. doi: 10.1093/humupd/dmab028
37. Jepsen MR, Kløverpris S, Mikkelsen JH, Pedersen JH, Füchtbauer EM, Laursen LS, et al. Stanniocalcin-2 inhibits mamMalian growth by proteolytic inhibition of the insulin-like growth factor axis. J Biol Chem (2015) 290(6):3430–9. doi: 10.1074/jbc.M114.611665
38. Kløverpris S, Mikkelsen JH, Pedersen JH, Jepsen MR, Laursen LS, Petersen SV, et al. Stanniocalcin-1 potently inhibits the proteolytic activity of the metalloproteinase pregnancy-associated plasma protein-A. J Biol Chem (2015) 290(36):21915–24. doi: 10.1074/jbc.M115.650143
39. Karbasi S, Bahrami A, Asadi Z, Shahbeiki F, Naseri M, Zarban A, et al. The association of maternal dietary quality and the antioxidant-proxidant balance of human milk. Int Breastfeed J (2022) 17(1):56. doi: 10.1186/s13006-022-00498-1
40. Xie Y, Chen D, Jiang K, Song L, Qian N, Du Y, et al. Hair shaft miniaturization causes stem cell depletion through mechanosensory signals mediated by a piezo1-calcium-tnf-α Axis. Cell Stem Cell (2022) 29(1):70–85.e6. doi: 10.1016/j.stem.2021.09.009
41. Bayram R, Yavuz MZ, Benek BS, Aydoğar Bozkurt A, Ucbek A, Özünal ZG, et al. Effect of breast milk calcium and fluidity on breast cancer cells: an in vitro cell culture study. Breastfeed Med (2016) 11:474–8. doi: 10.1089/bfm.2016.0048
42. Honorio-França AC, Nunes GT, Fagundes DL, de Marchi PG, Fernandes RT, França JL, et al. Intracellular calcium is a target of modulation of apoptosis in mcf-7 cells in the presence of iga adsorbed to polyethylene glycol. Onco Targets Ther (2016) 9:617–26. doi: 10.2147/ott.S99839
43. Sami E, Paul BT, Koziol JA, ElShamy WM. The immunosuppressive microenvironment in brca1-iris-overexpressing tnbc tumors is induced by bidirectional interaction with tumor-associated macrophages. Cancer Res (2020) 80(5):1102–17. doi: 10.1158/0008-5472.Can-19-2374
44. Blanchard Z, Paul BT, Craft B, ElShamy WM. Brca1-iris inactivation overcomes paclitaxel resistance in triple negative breast cancers. Breast Cancer Res (2015) 17(1):5. doi: 10.1186/s13058-014-0512-9
45. Castillo P, Aisagbonhi O, Saenz CC, ElShamy WM. Novel insights linking brca1-iris role in mammary gland development to formation of aggressive pabcs: the case for longer breastfeeding. Am J Cancer Res (2022) 12(1):396–426.
46. Ryan D, Paul BT, Koziol J, ElShamy WM. The pro- and anti-tumor roles of mesenchymal stem cells toward brca1-iris-overexpressing tnbc cells. Breast Cancer Res (2019) 21(1):53. doi: 10.1186/s13058-019-1131-2
47. Petrou P, Giannopoulou K, Papakonstantinou E, Karamaounas A, Kandarakis S, Georgalas I. Episodes of monocular blurring of vision during breastfeeding. Am J Ophthalmol Case Rep (2020) 20:100976. doi: 10.1016/j.ajoc.2020.100976
48. ElShamy WM. The protective effect of longer duration of breastfeeding against pregnancy-associated triple negative breast cancer. Oncotarget (2016) 7(33):53941–50. doi: 10.18632/oncotarget.9690
49. Panciera T, Citron A, Di Biagio D, Battilana G, Gandin A, Giulitti S, et al. Reprogramming normal cells into tumour precursors requires ecm stiffness and oncogene-mediated changes of cell mechanical properties. Nat Mater (2020) 19(7):797–806. doi: 10.1038/s41563-020-0615-x
50. Bhurosy T, Niu Z, Heckman CJ. Breastfeeding is possible: A systematic review on the feasibility and challenges of breastfeeding among breast cancer survivors of reproductive age. Ann Surg Oncol (2021) 28(7):3723–35. doi: 10.1245/s10434-020-09094-1
51. Paris I, Di Giorgio D, Carbognin L, Corrado G, Garganese G, Franceschini G, et al. Pregnancy-associated breast cancer: A multidisciplinary approach. Clin Breast Cancer (2021) 21(1):e120–e7. doi: 10.1016/j.clbc.2020.07.007
52. Cabrera L, Trapero I. Evaluation of the effectiveness of breastfeeding as a factor in the prevention of breast cancer. Endocr Metab Immune Disord Drug Targets (2022) 22(1):15–25. doi: 10.2174/1871530321666210427083707
53. Watson CJ. Post-lactational mammary gland regression: molecular basis and implications for breast cancer. Expert Rev Mol Med (2006) 8(32):1–15. doi: 10.1017/s1462399406000196
54. Basree MM, Shinde N, Koivisto C, Cuitino M, Kladney R, Zhang J, et al. Abrupt involution induces inflammation, estrogenic signaling, and hyperplasia linking lack of breastfeeding with increased risk of breast cancer. Breast Cancer Res (2019) 21(1):80. doi: 10.1186/s13058-019-1163-7
Keywords: breastfeeding, breast cancer, mechanism, methylation, breast involution
Citation: Chen Y, Jiang P and Geng Y (2023) The role of breastfeeding in breast cancer prevention: a literature review. Front. Oncol. 13:1257804. doi: 10.3389/fonc.2023.1257804
Received: 13 July 2023; Accepted: 23 August 2023;
Published: 07 September 2023.
Edited by:
Nicola Fusco, University of Milan, ItalyReviewed by:
Victoria Seewaldt, City of Hope, United StatesKonstantinos Venetis, University of Milan, Italy
Copyright © 2023 Chen, Jiang and Geng. This is an open-access article distributed under the terms of the Creative Commons Attribution License (CC BY). The use, distribution or reproduction in other forums is permitted, provided the original author(s) and the copyright owner(s) are credited and that the original publication in this journal is cited, in accordance with accepted academic practice. No use, distribution or reproduction is permitted which does not comply with these terms.
*Correspondence: Yongqin Geng, Z2VuZ3lxMTlAbWFpbHMuamx1LmVkdS5jbg==