- 1Department of Biomedical Sciences, City University of Hong Kong, Kowloon, Hong Kong
- 2Vitargent (International) Biotechnology Limited, Sha Tin, Hong Kong
- 3Department of Infectious Diseases and Public Health, City University of Hong Kong, Kowloon, Hong Kong
- 4Department of Physics, City University of Hong Kong, Kowloon, Hong Kong
Cancer stem cells are involved in cancer establishment, progression, and resistance to current treatments. We demonstrated the in vitro and in vivo anti-breast cancer effect of bakuchiol in a previous study. However, the ability of bakuchiol to target breast cancer stem cells (BCSCs) and inhibit breast cancer metastasis remains unknown. In the current study, we used the cell surface markers CD44 and CD24 to distinguish BCSCs from MCF-7 cells. Bakuchiol inhibited mammosphere formation and aldehyde dehydrogenase activity in BCSCs. Moreover, bakuchiol induced apoptosis and suppressed the mitochondrial membrane potential of BCSCs. Bakuchiol upregulated the expression levels of pro-apoptotic genes, BNIP3 and DAPK2. Bakuchiol induced oxidative stress and altered lipogenesis in BCSCs. In zebrafish xenografts, bakuchiol inhibited breast cancer cell metastasis in vivo. In addition, bakuchiol altered the expression levels of metastasis-related genes through upregulating CK18 and downregulating Notch3, FASN, TGFBR1, and ACVR1B. Our study provides evidence for the anti-breast cancer potential of bakuchiol.
Introduction
Breast cancer is the second leading cause of cancer death among the women in the United States. Both metastases found at diagnosis (de novo) and those occurring later (recurrence) are considered the most severe forms of breast cancer (Mariotto et al., 2017). The 5-year survival rate of stage 4 metastatic breast cancer is 26%, which is much lower than the survival rates of the early stage breast cancers1. Tumors contain heterogeneous cell populations, in which a small subpopulation of cells, known as cancer stem cells, is responsible for tumor initiation, maintenance, metastasis, and recurrence (Velasco-Velázquez et al., 2011; Shiozawa et al., 2013; Peitzsch et al., 2017). Tumorigenesis can be induced in immunodeficient mice through the injection of as few as 200 breast cancer stem cells (BCSCs), which are identified on the basis of the expression of the cell surface makers, such as CD44+/CD24-/low (Al-Hajj et al., 2003). Traditional cancer therapies are effective at debulking certain tumors but often fail to produce long-term clinical remissions given their inability to eradicate the BCSCs population. Thus, therapies that target BCSCs may improve the recurrent and metastatic rates.
Phytoestrogens are plant-derived substances that are structurally similar to the mammalian steroid hormone E2. Although the anti-cancer effects of phytoestrogens have been widely investigated (reviewed by Patisaul and Jefferson, 2010; Bilal et al., 2014), few studies have focused on the effects of phytoestrogens on BCSCs. Soy isoflavone genistein and blueberry polyphenolic acids repress mammosphere formation (Montales et al., 2012, 2013). Resveratrol inhibits BCSCs through the suppression of the Wnt/β-catenin and lipogenesis signaling pathway (Pandey et al., 2011; Fu et al., 2014). In vitro, in vivo, and clinical studies on the effects of phytoestrogen on metastasis have mainly focused on genistein, daidzein, and resveratrol. The anti-metastatic effects of these compounds have been reported in several tumor models, such as prostate cancer, breast cancer, melanoma, liver cancer, and colorectal cancer (Menon et al., 1998; Vantyghem et al., 2005; Gu et al., 2009; Sheth et al., 2012; Pavese et al., 2014; Ji et al., 2015).
Bakuchiol is a meroterpene found in the traditional Chinese herbal medicine Fructus Psoraleae, the dried ripe fruit of Psoralea corylifolia L. (Fabaceae). Bakuchiol possesses estrogenic, anti-microbial, anti-inflammatory, anti-oxidative, anti-osteoporosis, anti-depression, or anti-stress, and anti-cancer activities (reviewed by Li et al., 2016). In a previous study, we demonstrated the in vitro and in vivo inhibitory effect of bakuchiol on breast cancer (Li et al., 2016). However, the effects of bakuchiol on the growth of BCSCs and breast cancer metastasis have yet to be reported. We designed the current study according to the doses used in our previous in vitro and in vivo experiments (Li et al., 2016). The elucidation of pathways that how bakuchiol regulates BCSCs may lead to the identification of potential therapeutic targets. Prospective results will provide important evidence for the potential of bakuchiol as an anti-breast cancer agent.
Materials and Methods
Cell Culture
MCF-7 (ATCC: HTB-22) were purchased from American Type Culture Collection (ATCC, Manassas, VA, United States) and routinely maintained in Dulbecco’s modified Eagle’s medium (DMEM) supplemented with 10% fetal bovine serum (FBS), 0.37% Na2CO3, 50 units/mL penicillin, and 50 μg/mL streptomycin. The cells were incubated at 37°C in a humidified atmosphere with 5% CO2.
Effects of Bakuchiol on Mammosphere Formation in MCF-7 Cells
Complete MammoCult medium was freshly prepared with 45 mL MammoCult basal medium (Stemcell Technologies, Canada), 5 mL growth supplement (Stemcell Technologies, Canada), 200 μL heparin adjusted to a final concentration of 4 μg/mL (Stemcell Technologies, Canada), 250 μL hydrocortisone adjusted to a final concentration of 0.48 μg/mL (Sigma, St. Louis, MO, United States), and 500 μL 100× penicillin and streptomycin (Invitrogen, Carlsbad, CA, United States). Complete MammoCult medium was filter-sterilized with a 0.22-μm syringe filter.
MCF-7 cells were collected and seeded in ultra-low attachment six-well plates (BD Biosciences, San Jose, CA, United States) with complete MammoCult medium at a density of 20,000 cells/well. Bakuchiol {4-[(1E,3S)-3-ethenyl-3,7-dimethylocta-1,6-dienyl] phenol} purity 98% by HPLC was obtained from Enzo (Farmingdale, NY, United States). Cells were exposed to ethanol (vehicle control, 0.1% v/v, same amount in all the treatment conditions) or treated with 4 or 7 μg/mL bakuchiol, then allowed to grow for a week to form mammospheres (p1). Mammospheres from each group were collected and trypsinized separately. Cells from different groups were seeded and treated with ethanol or bakuchiol again for a week to form mammospheres (p2). Cell images were taken under an inverted microscope (model DMI3000 B, Leica Microsystems, Germany).
Cell Sorting for the Identification of CD44+/CD24-/low Populations from MCF-7 Cells
Cells were collected and washed with staining buffer (PBS containing 1% BSA). Then, 1 × 106 cells were resuspended in 100 μL staining medium with 20 μL fluorochrome-conjugated antibodies for 30 min on ice. Cells were washed with staining buffer and filtered using a strainer cap tube (BD Bioscience, San Jose, CA, United States) to avoid cell clumps.
Cell phenotypes were determined by flow cytometry using a FACS Aria III cell-sorting flow cytometer (BD Biosciences, San Jose, CA, United States) with FACS Diva 6.1 software (BD Biosciences, San Jose, CA, United States). Several dot-plots of different parameters were created on a global worksheet. Then, gates were drawn on corresponding populations of breast cancer cells to identify the subpopulation of interest, including SSC-A versus FSC-A for live cells, SSC-A versus PE-A for CD24+ cells, SSC-A versus FITC-A for CD44+ cells, and PE-A versus FITC-A for CD44+/CD24-/low cells. The CD44+/CD24-/low cells were BCSCs.
Effect of Bakuchiol on Mammosphere Formation in BCSCs
A total of 1 × 106 CD44+/CD24-/low cells were seeded in a low-attachment, 60 mm plate in complete MammoCult medium with ethanol (vehicle control) or different bakuchiol concentrations. Cells were allowed to grow for 7 days to form mammospheres. Mammospheres were imaged at 8–10 random fields on days 4 and 7 with a phase contrast microscope.
Determination of ALDH Activity
CD44+/CD24-/low cells in low-attachment plates were exposed to ethanol (vehicle control) or different bakuchiol concentrations for 4 days. Aldehyde dehydrogenase (ALDH) activity was determined by using ALDEFLUOR Kit (Stemcell Technologies, Canada) according to manufacturer’s instructions. Briefly speaking, cells were incubated in ALDEFLUOR assay buffer containing ALDH substrate. In each treatment condition a sample of cells was stained under identical conditions with diethylaminobenzaldehyde (DEAB), a specific ALDH inhibitor, as negative control. Following incubation, the cells were collected, resuspended in ALDEFLUOR assay buffer, and analyzed with flow cytometry (Becton Dickinson, Mountain View, CA, United States).
Annexin-V/PI Staining for Apoptosis Analysis
CD44+/CD24-/low cells in low-attachment plates were exposed to ethanol (vehicle control) or different bakuchiol concentrations for 4 days. Annexin-V/propidium iodide (PI) (Promega, Fitchburg, WI, United States) staining was conducted as described by the manufacturer.
Mitochondrial Membrane Potential Analysis
CD44+/CD24-/low cells in low-attachment plates were exposed to ethanol (vehicle control) or different bakuchiol concentrations for 4 days. JC-1 staining (Invitrogen, Carlsbad, CA, United States) was conducted in accordance with the manufacturer’s instructions.
ROS Production Analysis
The production of reactive oxygen species (ROS) was determined by using a ROS/superoxide detection kit (Enzo, Farmingdale, NY, United States). CD44+/CD24-/low cells were exposed to ethanol (vehicle control) or different bakuchiol concentrations for 4 days. In accordance with the manufacturer’s instructions, staining buffer with oxidative stress detection reagent (green fluorescence) and superoxide detection reagent (orange fluorescence) were added to washed, trypsinized, and resuspended cells. The stained cells were analyzed by flow cytometry (Becton Dickinson, Mountain View, CA, United States). The ROS inhibitor (N-acetyl-L-cysteine) was used as negative control.
Lipid Content Determination
Intracellular lipid content was quantified using AdipoRed Assay Reagent (Lonza, Walkersville, MD, United States). AdipoRed is a solution of the hydrophilic stain Nile Red, which can stain intracellular lipid droplets. CD44+/CD24-/low cells were treated with ethanol (vehicle control) or different bakuchiol concentrations for 4 days. Cells were collected, washed, and stained with AdipoRed in accordance with the manufacturer’s recommendations. After 10 min of staining, fluorescence was measured using a SpectraMax Microplate Reader (Molecular Devices, Hong Kong) under excitation at 485 nm and emission at 572 nm.
Semi-quantitative Real-Time PCR
After CD44+/CD24-/low cells were exposed to ethanol (vehicle control) or different bakuchiol concentrations for 4 days, RNA extraction, genomic DNA decontamination, and semi-quantitative real-time PCR were performed as described in our previous study (Li et al., 2016). Primers are listed in Table 1.
Zebrafish Xenograft Establishment and Fish Exposure
Wild-type AB zebrafish embryos at 48 hpf were dechorionated prior to cell injection. Approximately 500 CM-DiI (Invitrogen, Carlsbad, CA, United States)-labeled cells were injected into embryonic yolk sacs. Then, the embryos were exposed to ethanol (solvent control) or different bakuchiol concentrations. The exposed embryos were maintained at 28°C for 1 h and then placed in an incubator at 34°C for 7 days. The incubation medium was replaced every 2 days. All procedures with fish were conducted following the License to Conduct Experiments approved by the Government of the Hong Kong SAR Department of Health [Refs. (15–10) in DH/HA&P/8/2/5 Pt.3].
Lightsheet Microscope Imaging
To determine the percentage of metastasis (number of embryos with tail or head metastasis/number of total embryos), xenotransplanted embryos were examined using a lightsheet microscope (Lightsheet Z.1, ZEISS, Germany) equipped with 5× water lens. Images were taken using Z-stack functions. The results were projected with the maximum projection by using lightsheet software (Zen, ZEISS, Germany).
Statistical Analysis
Statistical analyses were conducted using SigmaPlot 10.0 and SPSS 13.0. Experiments were conducted in triplicate and data were presented as mean values ± standard deviation. Data were analyzed with Student’s t-test and one-way ANOVA. A p-value < 0.05 was considered statistically significant.
Results
Bakuchiol Decreased Mammosphere Formation in MCF-7 Cells
MCF-7 cells were seeded in ultra-low attachment plates and treated with ethanol (vehicle control) or with 4 or 7 μg/mL bakuchiol for a week to form mammospheres. Mammosphere formation occurred in the control and 4 μg/mL bakuchiol treatment groups (Figure 1A) but not in the 7 μg/mL bakuchiol treatment group (data not shown). Mammospheres in control and treatment groups exhibited significant morphological differences: mammospheres in the treatment groups were smaller, looser, and less spherical than those in the control group (Figure 1A). The percentages of mammosphere formation (number of mammospheres/number of seeded cells) were downregulated by bakuchiol in both p1 and p2 passages (Figure 1B).
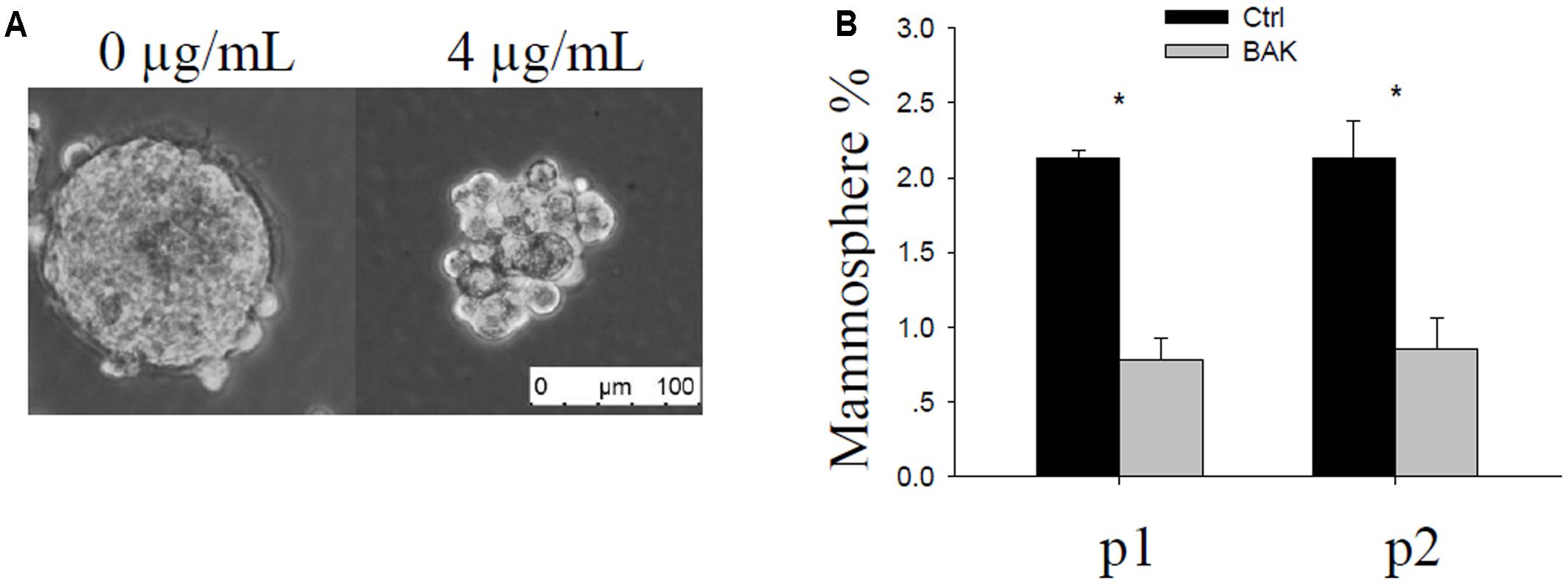
FIGURE 1. Bakuchiol inhibited mammosphere formation in MCF-7 cells. (A) Representative morphology of mammospheres formed in the control and bakuchiol treatment groups. The white bar represents a length of 100 μm. (B) Comparison of percentage of mammospheres in the total number of seeded cells. Each condition was performed in triplicate (∗p < 0.05, t-test). Ctrl, control; BAK, bakuchiol.
Bakuchiol Inhibited Mammosphere Formation in CD44+/CD24-/low BCSCs
The subpopulation of CD44+/CD24-/low cells accounted for less than 1% of the total cells (Figure 2A). We used this subpopulation of cells for the following mammosphere formation assay. Cells were imaged on days 1, 4, and 7 to monitor mammosphere growth after CD44+/CD24-/low cells were treated with different bakuchiol concentrations. The majority of cells were separated on day 1 (Figure 2B). On days 4 and 7, control group mammospheres were compact and spherical, and treatment group mammospheres became less compact, fewer in number, and smaller as bakuchiol concentration increased (Figure 2B). When cells were exposed to 7 μg/mL bakuchiol, mammospheres appeared only on day 4 but not on day 7 (Figure 2B).
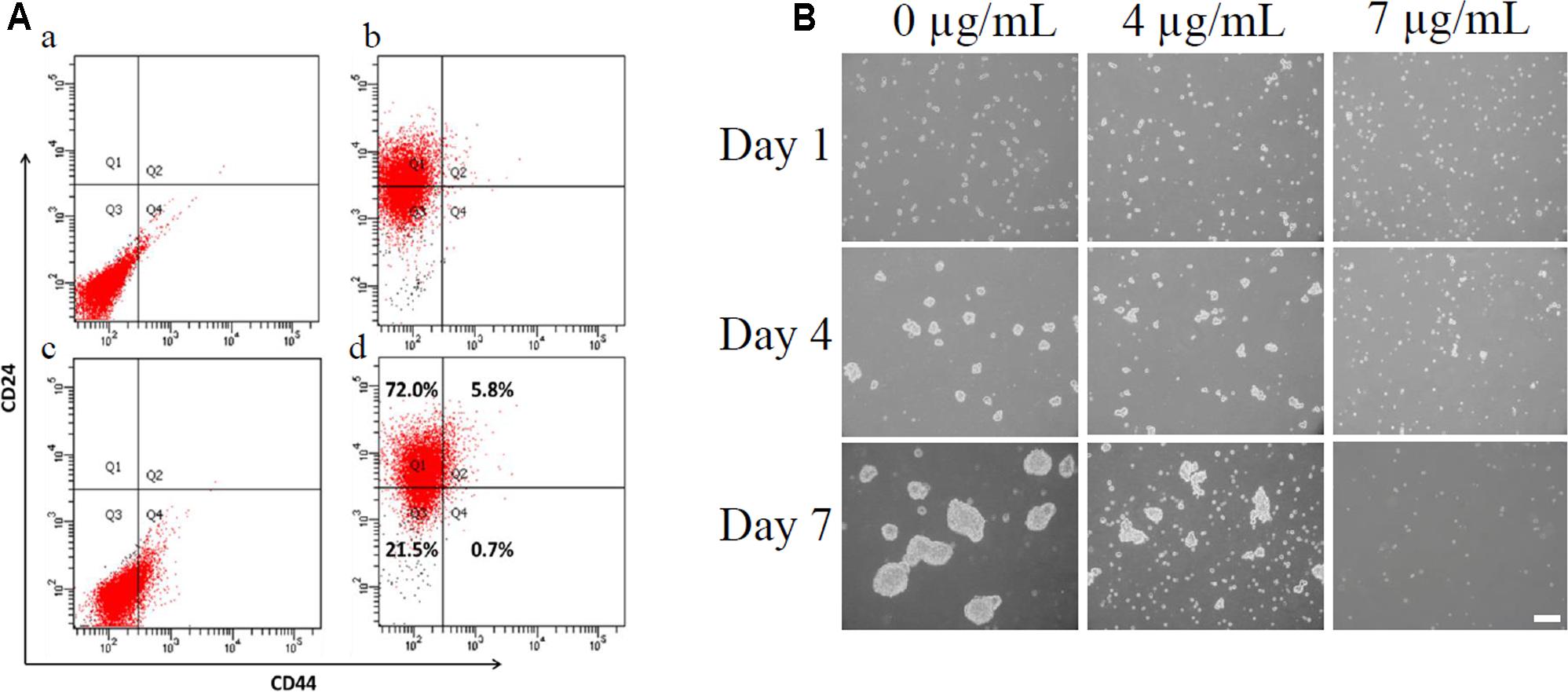
FIGURE 2. Bakuchiol inhibited mammosphere formation in CD44+/CD24-/low BCSCs. (A) Analysis of percentage of CD44+/CD24-/low population in MCF-7 cells. (a) Cells stained with PE-IgG2a and FITC-IgG2b as control. (b) Cells stained with PE-CD24. (c) Cells stained with FITC-CD44. (d) Cells double-stained with PE-CD24 and FITC-CD44. CD44+/CD24-/low population was sorted out using a cell-sorting flow cytometer. (B) Pictures of mammospheres in CD44+/CD24-/low MCF-7 cells in the control and bakuchiol treatment groups on days 1, 4, and 7. The white bar represents a length of 100 μm. The experiments were carried out in triplicates.
Bakuchiol Reduced ALDH Activity in CD44+/CD24-/low BCSCs
The background ALDH activity varied in different treatments. Thus, we investigated the background ALDH activity of each treatment condition by using DEAB as manufacturer suggested. After 4 days of treatment, bakuchiol significantly reduced the ALDH activity in a dose-dependent manner (Figure 3).
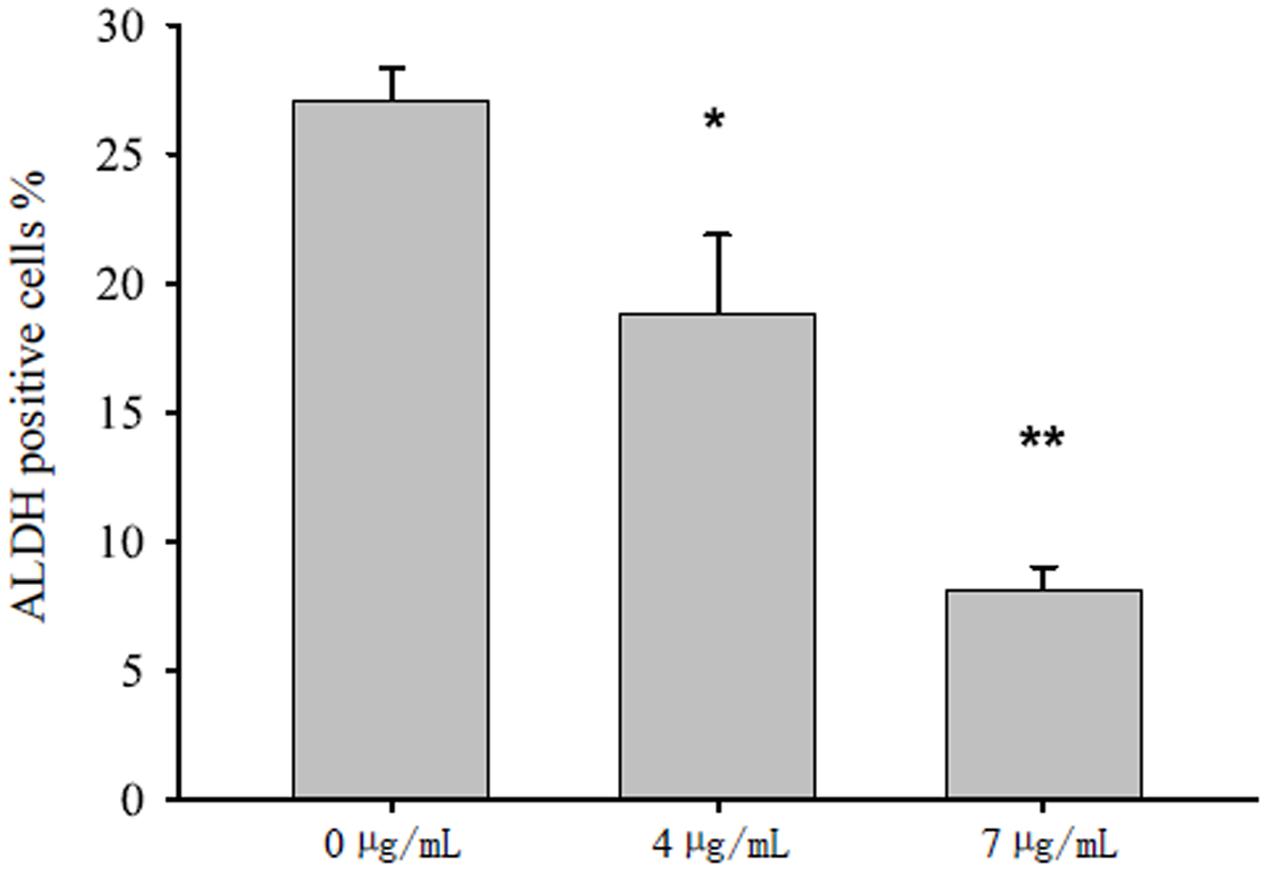
FIGURE 3. Bakuchiol inhibited ALDH activity in CD44+/CD24-/low BCSCs. Cells were exposed to ethanol (vehicle control) or were treated with 4 or 7 μg/mL bakuchiol for 4 days. ALDH activity was detected with a ALDEFLUOR Kit. Each condition was performed in triplicate. Data were presented as mean ± SD (∗∗∗p < 0.001, ∗∗p < 0.01, ∗p < 0.05, one-way ANOVA).
Bakuchiol Induced Apoptosis in CD44+/CD24-/low BCSCs
We exposed CD44+/CD24-/low cells to ethanol (vehicle control) or to 4 or 7 μg/mL bakuchiol for 4 days. We then analyzed cell apoptosis using Annexin V/PI staining and mitochondrial membrane potential using JC-1 staining. As bakuchiol concentration increased, the percentage of early apoptotic cells increased (Figure 4A) and mitochondrial membrane potential decreased (Figure 4B) in CD44+/CD24-/low cells. The mRNA levels of pro-apoptotic genes, BNIP3 and DAPK2 were significantly upregulated in cells after bakuchiol treatment (Figure 4C).
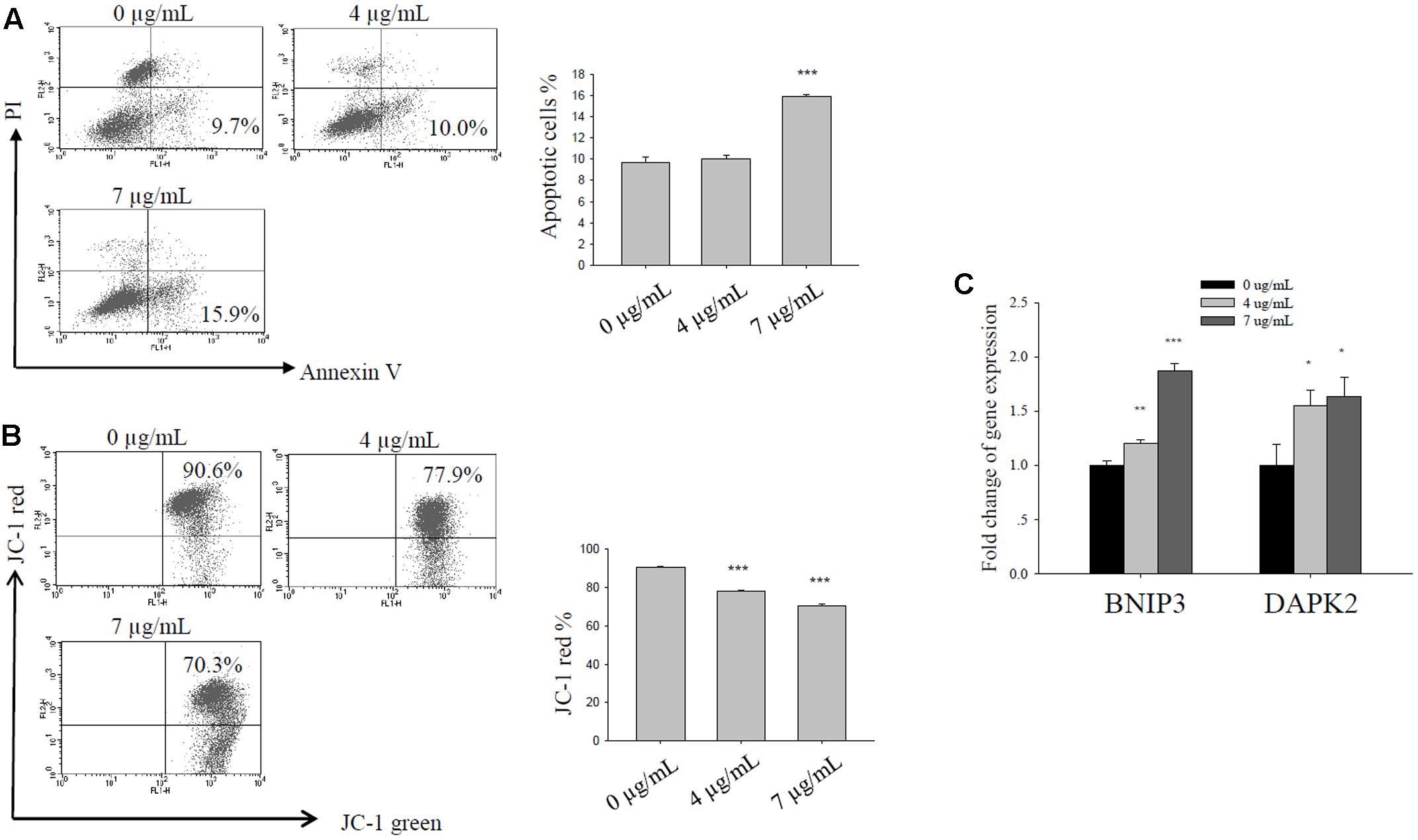
FIGURE 4. Bakuchiol induced apoptosis in CD44+/CD24-/low BCSCs. Cells were exposed to ethanol (vehicle control), 4, or 7 μg/mL bakuchiol for 4 days. (A) Analysis of bakuchiol-induced early apoptosis in CD44+/CD24-/low cells with Annexin V/PI staining. (B) JC-1 staining for the analysis of mitochondrial membrane potential in CD44+/CD24-/low cells. (C) mRNA expression levels of BNIP3 and DAPK2 in CD44+/CD24-/low cells. GAPDH was used as an internal reference. The experiments were carried out in triplicates (∗p < 0.05, ∗∗p < 0.01, ∗∗∗p < 0.001, one-way ANOVA).
Bakuchiol Induced Oxidative Stress in CD44+/CD24-/low BCSCs
After 4 days of bakuchiol treatment, staining with ROS detection dye revealed that bakuchiol induced total ROS production (Figure 5A) and superoxide production (Figure 5B). Although total ROS production and superoxide production were upregulated in both treatment groups, total ROS production and superoxide production in the 7 μg/mL treatment group were lower than that in 4 μg/mL group (Figures 5A,B).
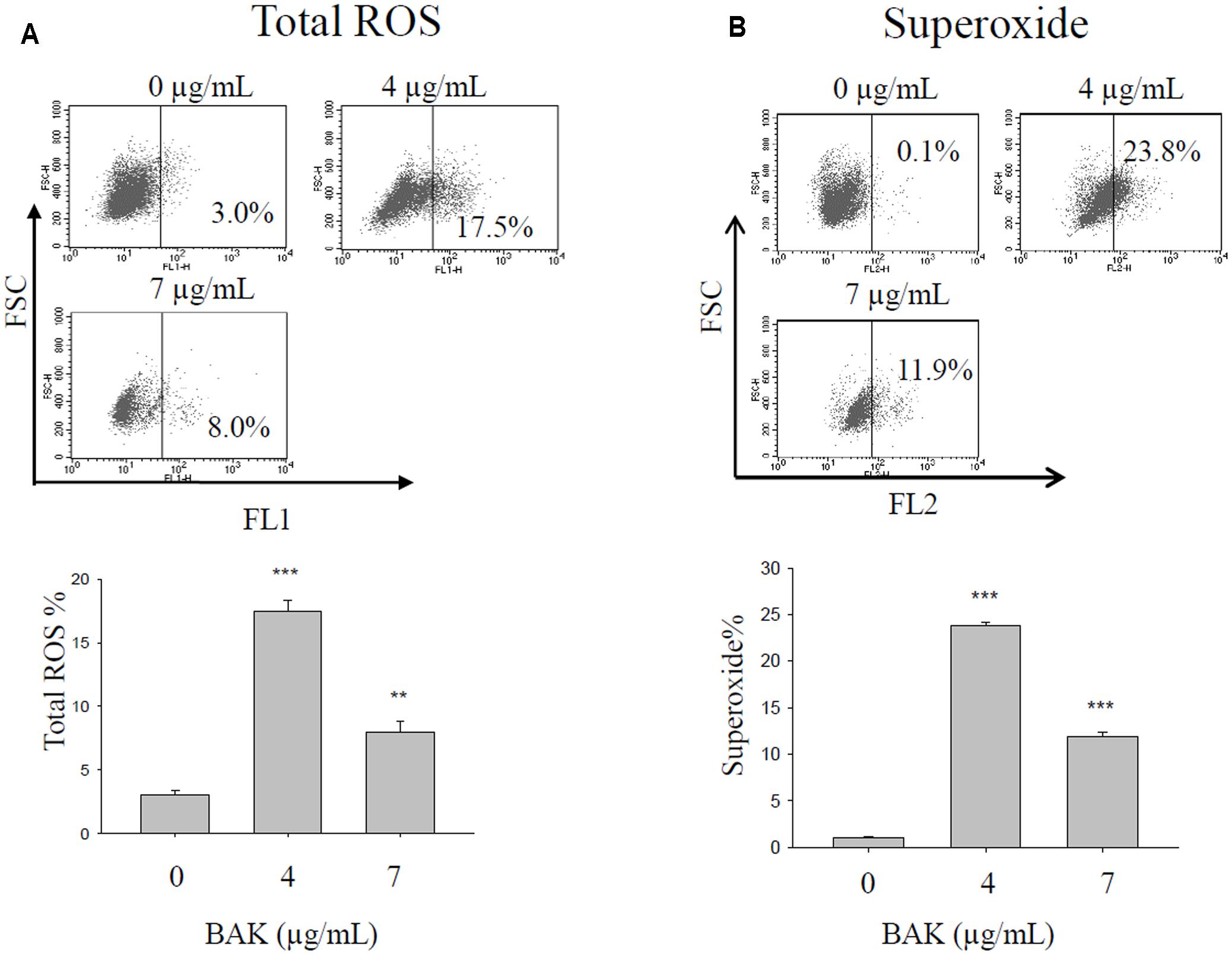
FIGURE 5. Bakuchiol treatment changed ROS production in CD44+/CD24-/low BCSCs. Cells were exposed to ethanol (vehicle control) or were treated with 4 or 7 μg/mL bakuchiol for 4 days. Total ROS (A) and superoxide (B) production was determined with a ROS/Superoxide detection kit. Each condition was performed in triplicate. Data were presented as mean ± SD (∗∗∗p < 0.001, ∗∗p < 0.01, ∗p < 0.05, one-way ANOVA). BAK, bakuchiol.
Bakuchiol Affected the Lipogenesis of CD44+/CD24-/low BCSCs
We analyzed lipid content and FASN mRNA expression levels in CD44+/CD24-/low cells that were exposed to different bakuchiol concentrations for 4 days. AdipoRed staining revealed that lipid content increased in cells that were exposed to 4 μg/mL bakuchiol but decreased in cells that were exposed to 7 μg/mL bakuchiol (Figure 6A). Lipid contents in the treatment and control groups were not significantly different, whereas those in the two treatment groups were significantly different (Figure 6A). FASN mRNA level was elevated in the 4 μg/mL bakuchiol treatment group and downregulated in the 7 μg/mL bakuchiol treatment group (Figure 6B). The trend of upregulation at 4 μg/mL and downregulation at 7 μg/mL is consistent with that for lipid content.
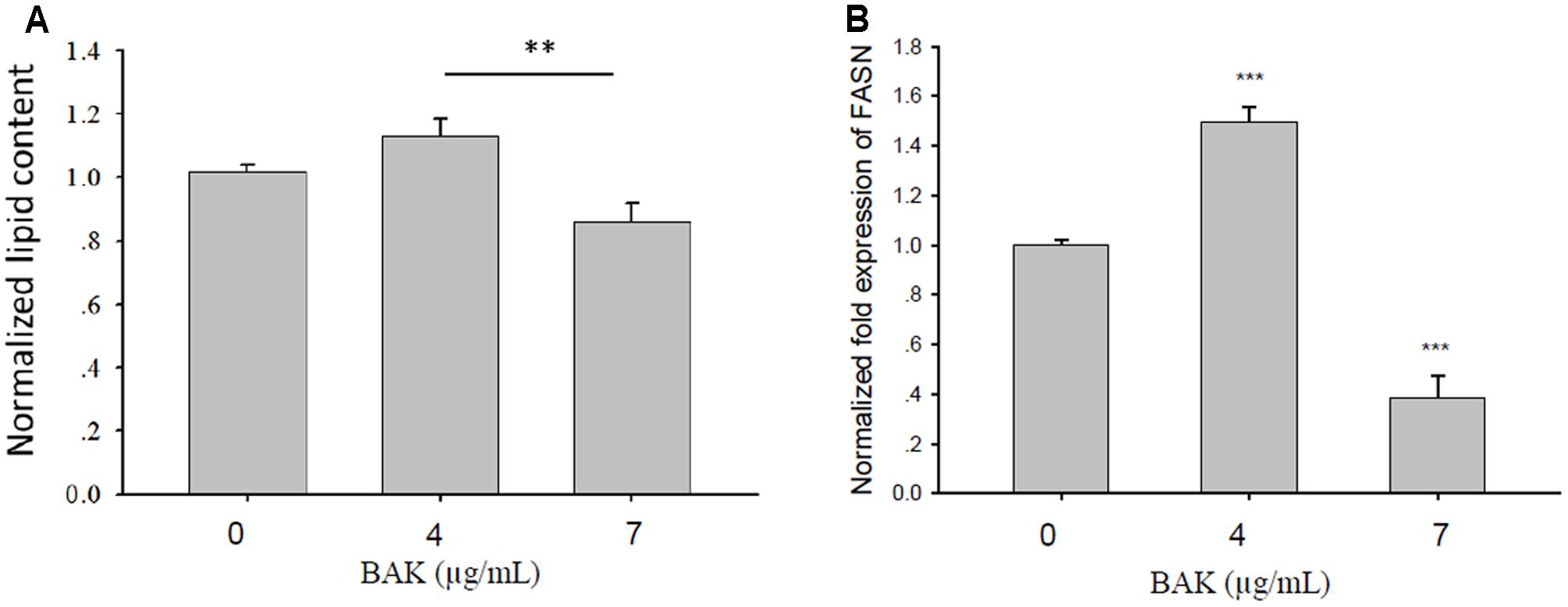
FIGURE 6. Bakuchiol affected lipogenesis of CD44+/CD24-/low BCSCs. Cells were exposed to ethanol (vehicle control) or treated with 4 or 7 μg/mL bakuchiol for 4 days. (A) Intracellular lipid content was quantified using AdipoRed Assay Reagent. Relative fold changes of lipid content were normalized to the level of control (arbitrarily set to 1). Data were presented as mean ± SD. (B) mRNA expression levels of FASN in CD44+/CD24-/low cells. GAPDH was used as an internal reference. The experiments were carried out in triplicates (∗∗∗p < 0.001, ∗∗p < 0.01, ∗p < 0.05, one-way ANOVA). BAK, bakuchiol.
Bakuchiol Inhibited MCF-7 Cell Metastasis in Zebrafish Embryos
Cell metastasis was observed on day 7 after cell injection in 48-hpf zebrafish embryos that were exposed to 0, 0.5, and 1 μg/mL bakuchiol. The representative images of embryos with and without cell metastasis are shown in Figure 7A. The percentages of embryos without metastasis increased in a dose-dependent manner, and the numbers of fluorescent particles decreased in a dose-dependent manner (Figure 7B). Mortality in the control and treatment groups was not significantly different, as is consistent with our previous result (Li et al., 2016).
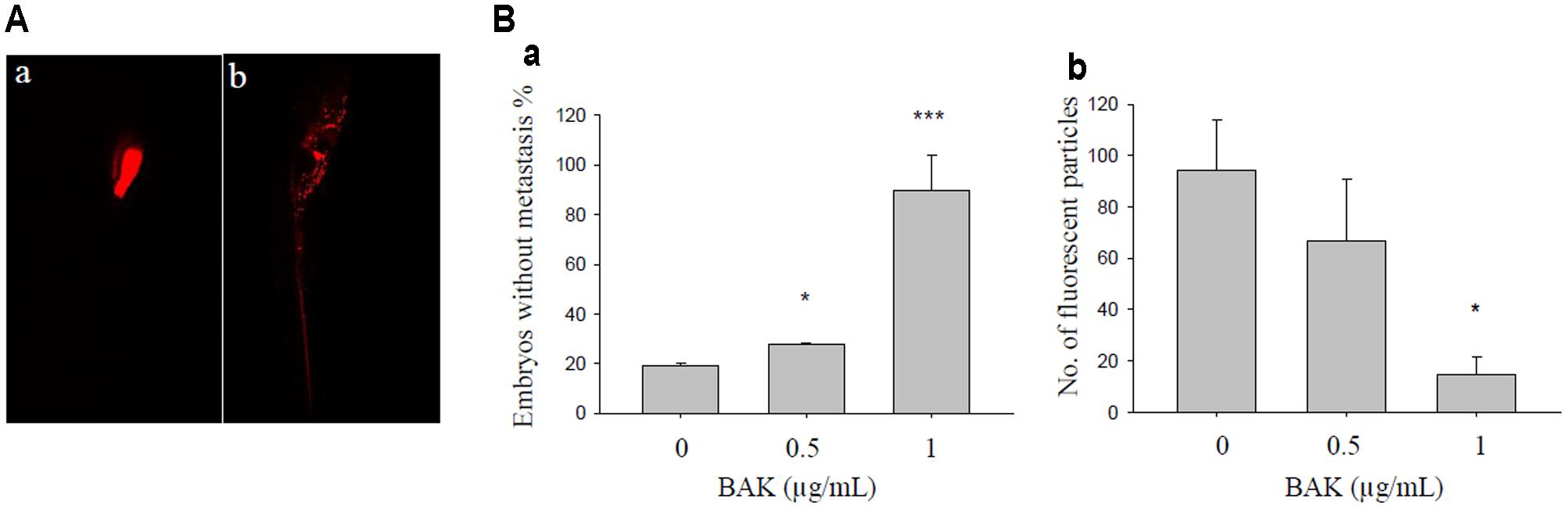
FIGURE 7. Bakuchiol inhibited in vivo cell metastasis in zebrafish. (A) Representative images of zebrafish embryo (a) without and (b) with cell metastasis. (B) Approximately 500 unsorted MCF-7 cells were injected into the yolk sacs of 48 hpf zebrafish embryos. Then, 40 embryos were treated with ethanol (solvent control) or with 0.5 or 1 μg/mL of bakuchiol for 7 days. Each condition was performed in triplicate. Statistical analysis of (a) the percentages of embryos without cell metastasis in control and treatment groups, and of (b) the numbers of fluorescent particles in control and treatment groups (∗∗∗p < 0.001, ∗∗p < 0.01, ∗p < 0.05, one-way ANOVA). BAK, bakuchiol.
Bakuchiol Altered Metastasis-Related Gene Expression in CD44+/CD24-/low BCSCs
Considering that bakuchiol inhibits cell metastasis in zebrafish embryos, we investigated how bakuchiol affected the expression levels of metastasis-related genes in CD44+/CD24-/low cells. Treatment with different concentrations of bakuchiol upregulated CK18 and downregulated Notch3 in a dose-dependent manner (Figure 8A). Genes in the transforming growth factor-β (TGF-β) signaling pathway, such as TGFBR1 and ACVR1B, decreased upon exposure to bakuchiol. When cells were co-treated with the TGF-β signaling pathway inhibitor SB-431542, the expression levels of TGFBR1 and ACVR1B further decreased (Figure 8B). FASN expression exhibited a similar trend (Figure 8B).
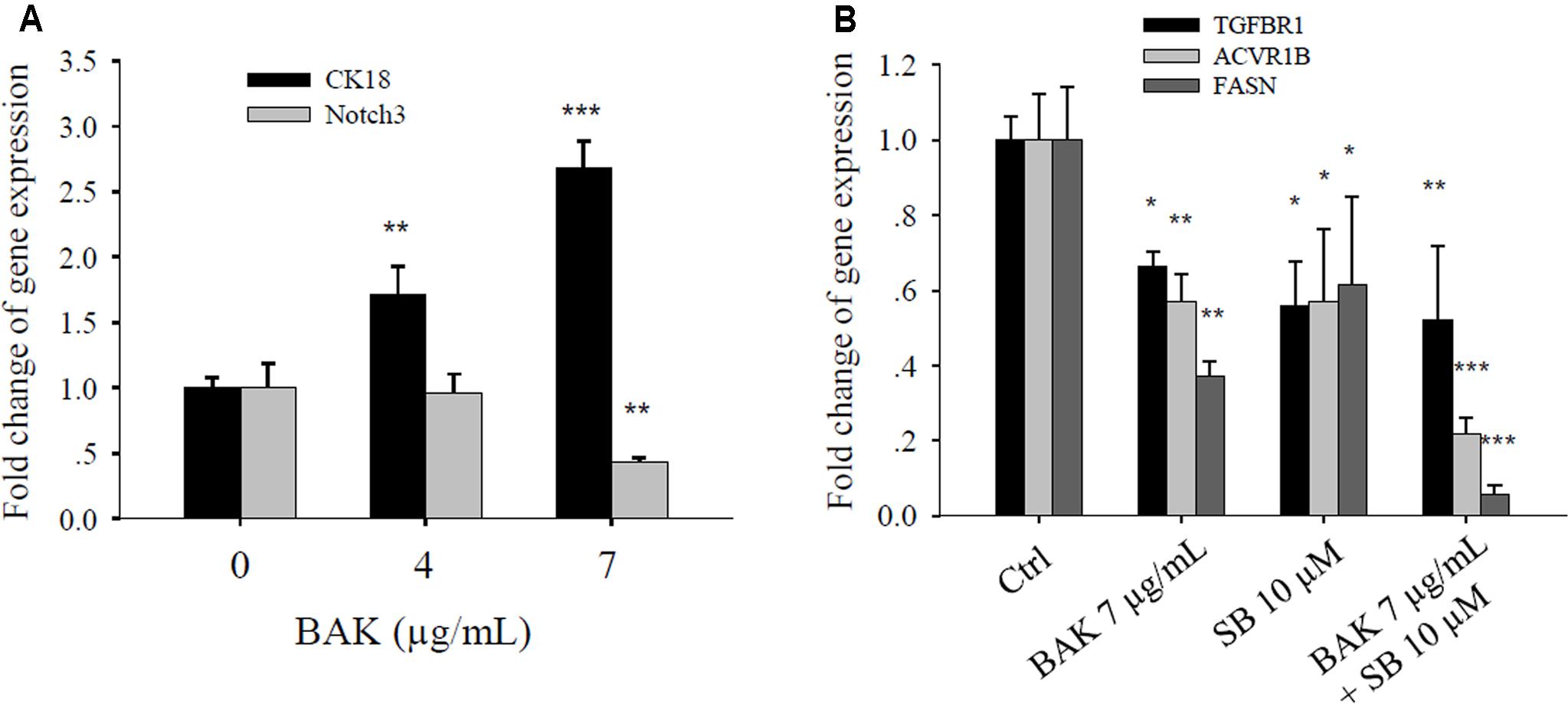
FIGURE 8. Bakuchiol affected the metastasis-related gene expression in CD44+/CD24-/low BCSCs. (A) Cells were exposed to ethanol (vehicle control) or were treated with 4 or 7 μg/mL bakuchiol for 4 days. The mRNA expression levels of CK18 and Notch3 were quantified by real-time PCR. (B) Cells were treated with ethanol (Ctrl) or with 7 μg/mL bakuchiol (BAK) or/and 10 μM SB-431542 (SB) for 4 days. The mRNA expression levels of TGFBR1, ACVR1B, and FASN were quantified by real-time PCR. GAPDH was used as an internal reference. The experiments were carried out in triplicates (∗p < 0.05, ∗∗p < 0.01, ∗∗∗p < 0.001, one-way ANOVA). BAK, bakuchiol.
Discussion
In our previous study, we found that high-dose bakuchiol inhibited MCF-7 cells in a dose-dependent manner; and we investigated the mechanisms of bakuchiol-induced apoptosis and cell cycle arrest with the doses of 4 and 7 μg/mL in that study (Li et al., 2016). We adopted the same doses in current study to investigate if bakuchiol can target BCSCs. Mammosphere formation has been developed as an in vitro assay to confirm the self-renewal potential of BCSCs. The results of the present study revealed that bakuchiol inhibits self-renew of BCSCs. The ALDHs are a group of NAD(P)+-dependent enzymes that are involved in oxidizing aldehydes. The expression of ALDH is enriched in cancer stem cells (Ginestier et al., 2007; Kakarala and Wicha, 2007). We showed that bakuchiol inhibited the ALDH activity in BCSCs, which further proved its anti-BCSCs effect. Moreover, bakuchiol induces the apoptosis of BCSCs, and inhibits the metastasis of breast cancer cells in zebrafish embryos. Thus, we examined the possible signaling pathways, including ROS production, lipogenic, pro-apoptotic, Notch, cytoskeletal, and TGF-β signaling pathways, which underlie these effects.
Reactive oxygen species can be categorized as free oxygen radicals (such as superoxide and nitric oxide) and non-radical ROS (such as hydrogen peroxide, singlet oxygen, and ozone/trioxygen) (Liou and Storz, 2010). With threshold limits, ROS contribute to cancer cell homeostasis and growth. However, aberrant ROS that exceeds the threshold can elicit cancer cell apoptosis through both extrinsic (receptor-mediated) and intrinsic (mitochondrial-mediated) apoptotic pathways (Ryter et al., 2007; Liou and Storz, 2010). BNIP3 is a pro-apoptotic BH3-only protein, functioning as a mitochondrial stress sensor. Activation of BNIP3 results in loss of mitochondrial membrane potential, generation of ROS, and induction of apoptosis (Chinnadurai et al., 2008). However, the role of ROS in cancer metastasis remains controversial. Piskounova et al. (2015) reported that ROS limit distant metastasis and that only cells with increased antioxidant capacity can metastasize. Meanwhile, several other studies have reported contrasting results, as reviewed by Peiris-Pagès et al. (2015). In the present study, bakuchiol induced total ROS and superoxide production in CD44+/CD24-/low BCSCs, implicating the potential of bakuchiol as a ROS-inducing agent for chemotherapy.
FASN is a key lipogenic enzyme that catalyzes the terminal steps in the biogenesis of fatty acids. FASN is lowly expressed or undetectable in most tissues but is overexpressed in a wide range of epithelial tumors, including breast cancer, because rapidly growing cancer cells require lipids for membrane synthesis and energy supply (Kuhajda, 2000). The inhibition of FASN can induce apoptosis in breast cancer cells through upregulating pro-apoptotic genes BNIP3, DAPK2, and TRAIL (Bandyopadhyay et al., 2006). Several phytoestrogens, such as resveratrol and genistein, suppress BCSCs by inhibiting FASN and mammary lipogenesis (Pandey et al., 2011; Montales et al., 2013). The FASN inhibitor attenuates the metastasis of different tumors, such as melanomas, breast cancer, oral cancer, and colorectal cancer (Seguin et al., 2012; Zaytseva et al., 2012, 2015; Agostini et al., 2014; Singh et al., 2015). Pharmacological limitations and side effects of current FASN inhibitors prevent their development as systemic drugs (Flavin et al., 2010). However, natural product-derived FASN inhibitors, such as bakuchiol, may provide new therapeutic moieties for breast cancer patient care. In the current study, treatment with 7 μg/mL bakuchiol decreased lipid content and repressed FASN expression in CD44+/CD24-/low BCSCs, suggesting that bakuchiol may induce apoptosis in BCSCs and inhibit in vivo metastasis by inhibiting lipogenesis. However, treatment with 4 μg/mL bakuchiol increased lipid content and FASN expression, and induced a high level of oxidative stress. In our previous study (Li et al., 2016), we found that bakuchiol exerted a biphasic effect on the growth of MCF-7 cells – stimulating cellular proliferation at low concentrations because of the ER agonist effect, and inhibiting cell proliferation at high concentrations because of the ER antagonist effect. The concentration of 4 μg/mL is around the biphasic border, which may explain the differences in oxidative stress and lipogenesis between 4 and 7 μg/mL such as a narrow window. Thus, further clinical experiments should be carefully designed.
Transforming growth factor-β induces the expression of cell surface markers associated with cancer stem cells (Mani et al., 2008). By regulating EMT-related and extravasation-related genes, TGF-β induces tumor invasion and metastasis in numerous cancer models (reviewed by Sheen et al., 2013; Cantelli et al., 2017). Cytokeratins are the major component of the epithelial cytoskeleton, which provide mechanical stability to tissues. CK18 is downregulated in metastatic breast cancer (Hedenfalk et al., 2001; Zajchowski et al., 2001; Woelfle et al., 2004). The EMT–FASN positive loop may contribute to the metastatic potential of breast and lung cancers (Hung et al., 2011; Li et al., 2014; Yang et al., 2016). In the current study, we showed that bakuchiol inhibited the mRNA expression of TGF-β receptors (TGFBR1 and ACVR1B), CK18, and FASN. The co-treatment of bakuchiol with the TGF-β receptor inhibitor SB-431542 further suppressed the expression levels of TGFBR1, ACVR1B, and FASN, thus implicating that TGF-β may be involved in the bakuchiol-induced inhibition of metastasis.
Notch signaling promotes the self-renewal capacity of mammospheres. Dontu et al. (2004) have reported a 10-fold increase in secondary mammosphere formation upon the addition of a Notch-activating delta-Serrate-Lag2 peptide. Notch inhibition decreases ductal carcinoma in situ-derived mammosphere production (Farnie and Clarke, 2007). Notch 3 is involved in mammosphere formation (Sansone et al., 2007). Moreover, positive feedback between Notch 3 and TGF-β contributes to the metastasis of breast and lung cancers (Zhang et al., 2010; Liu et al., 2014). In the present study, we found that bakuchiol repressed Notch 3 expression, possibly exerting an inhibitory effect on the self-renewal capacity of BCSCs and metastasis of breast cancer cells.
Zebrafish xenograft has increasingly become a desirable tool for cancer metastasis study (Tat et al., 2013; Teng et al., 2013; Heilmann et al., 2015; Brown et al., 2017). In current study, we found that bakuchiol inhibited breast cancer cell metastasis in zebrafish embryos. Although we have investigated several pathways that may be related to the anti-metastasis effect with in vitro experiments, including FASN, TGF-β, and Notch. The doses we used for in vivo metastasis experiment were lower than that for in vitro experiments. According to our previous study (Li et al., 2016) and current study, low-dose bakuchiol induced in vitro MCF-7 cell growth, but also inhibited in vivo cell growth and cell metastasis. Thus, the mechanisms described at higher doses may not be utilized to explain the in vivo finding. To further investigate if these signaling pathways are involved in the in vivo anti-metastasis effects, cells with overexpression of FASN, TGFBR1, ACVR1B, and Notch3 should be injected to see if the bakuchiol-induced anti-metastasis effects can be rescued.
Conclusion
Our results showed that bakuchiol targets BCSCs and inhibits breast cancer cell metastasis in zebrafish embryos. These findings suggest the potential of bakuchiol in breast cancer treatment. However, further experiments should be carried out to overcome the limitations of current study.
Author Contributions
LL was involved in the project design, carried out most of the experiments, and drafted the manuscript. CL helped with the zebrafish xenograft establishment and imaging. XC participated in fish maintenance and exposure. SX and SH helped with the ALDH activity experiment. SC contributed substantially to the experimental design, manuscript preparation, and submission. All authors read and approved the final manuscript.
Funding
The work was supported by Strategic Research Grant from City University of Hong Kong, Hong Kong, China (Grant No. 7004806).
Conflict of Interest Statement
SC served on the Scientific Advisory Board of the Company Vitargent Biotechnology Limited. XC was employed by the company Vitargent Biotechnology Limited.
The other authors declare that the research was conducted in the absence of any commercial or financial relationships that could be construed as a potential conflict of interest.
Acknowledgments
The authors heartedly thank Dr. Cornelia Man and Dr. Lai Sheung Lee (PolyU, Hong Kong) for providing the breast cancer stem cells.
Footnotes
References
Agostini, M., Almeida, L. Y., Bastos, D. C., Ortega, R. M., Moreira, F. S., Seguin, F., et al. (2014). The fatty acid synthase inhibitor orlistat reduces the growth and metastasis of orthotopic tongue oral squamous cell carcinomas. Mol. Cancer Ther. 13, 585–595. doi: 10.1158/1535-7163.MCT-12-1136
Al-Hajj, M., Wicha, M. S., Benito-Hernandez, A., Morrison, S. J., and Clarke, M. F. (2003). Prospective identification of tumorigenic breast cancer cells. Proc. Natl. Acad. Sci. U.S.A. 100, 3983–3988. doi: 10.1073/pnas.0530291100
Bandyopadhyay, S., Zhan, R., Wang, Y., Pai, S. K., Hirota, S., Hosobe, S., et al. (2006). Mechanism of apoptosis induced by the inhibition of fatty acid synthase in breast cancer cells. Cancer Res. 66, 5934–5940. doi: 10.1158/0008-5472.CAN-05-3197
Bilal, I., Chowdhury, A., Davidson, J., and Whitehead, S. (2014). Phytoestrogens and prevention of breast cancer: the contentious debate. World J. Clin. Oncol. 5, 705–712. doi: 10.5306/wjco.v5.i4.705
Brown, H. K., Schiavone, K., Tazzyman, S., Heymann, D., and Chico, T. J. (2017). Zebrafish xenograft models of cancer and metastasis for drug discovery. Expert Opin. Drug Discov. 12, 379–389. doi: 10.1080/17460441.2017.1297416
Cantelli, G., Crosas-Molist, E., Georgouli, M., and Sanz-Moreno, V. (2017). TGFB-induced transcription in cancer. Semin. Cancer Biol. 42, 60–69. doi: 10.1016/j.semcancer.2016.08.009
Chinnadurai, G., Vijayalingam, S., and Gibson, S. B. (2008). BNIP3 subfamily BH3-only proteins: mitochondrial stress sensors in normal and pathological functions. Oncogene 27(Suppl. 1), S114–S127. doi: 10.1038/onc.2009.49
Dontu, G., Jackson, K. W., McNicholas, E., Kawamura, M. J., Abdallah, W. M., and Wicha, M. S. (2004). Role of Notch signaling in cell-fate determination of human mammary stem/progenitor cells. Breast Cancer Res. 6, R605–R615. doi: 10.1186/bcr920
Farnie, G., and Clarke, R. B. (2007). Mammary stem cells and breast cancer–role of Notch signalling. Stem Cell Rev. 3, 169–175. doi: 10.1007/s12015-007-0023-5
Flavin, R., Peluso, S., Nguyen, P. L., and Loda, M. (2010). Fatty acid synthase as a potential therapeutic target in cancer. Future Oncol. 6, 551–562. doi: 10.2217/fon.10.11
Fu, Y., Chang, H., Peng, X., Bai, Q., Yi, L., Zhou, Y., et al. (2014). Resveratrol inhibits breast cancer stem-like cells and induces autophagy via suppressing Wnt/β-catenin signaling pathway. PLOS ONE 9:e102535. doi: 10.1371/journal.pone.0102535
Ginestier, C., Hur, M. H., Charafe-Jauffret, E., Monville, F., Dutcher, J., Brown, M., et al. (2007). ALDH1 is a marker of normal and malignant human mammary stem cells and a predictor of poor clinical outcome. Cell Stem Cell 1, 555–567. doi: 10.1016/j.stem.2007.08.014
Gu, Y., Zhu, C. F., Dai, Y. L., Zhong, Q., and Sun, B. (2009). Inhibitory effects of genistein on metastasis of human hepatocellular carcinoma. World J. Gastroenterol. 15, 4952–4957. doi: 10.3748/wjg.15.4952
Hedenfalk, I., Duggan, D., Chen, Y., Radmacher, M., Bittner, M., Simon, R., et al. (2001). Gene-expression profiles in hereditary breast cancer. N. Engl. J. Med. 344, 539–548. doi: 10.1056/NEJM200102223440801
Heilmann, S., Ratnakumar, K., Langdon, E., Kansler, E., Kim, I., Campbell, N. R., et al. (2015). A quantitative system for studying metastasis using transparent zebrafish. Cancer Res. 75, 4272–4282. doi: 10.1158/0008-5472.CAN-14-3319
Hung, C. M., Kuo, D. H., Chou, C. H., Su, Y. C., Ho, C. T., and Way, T. D. (2011). Osthole suppresses hepatocyte growth factor (HGF)-induced epithelial-mesenchymal transition via repression of the c-Met/Akt/mTOR pathway in human breast cancer cells. J. Agric. Food Chem. 59, 9683–9690. doi: 10.1021/jf2021489
Ji, Q., Liu, X., Han, Z., Zhou, L., Sui, H., Yan, L., et al. (2015). Resveratrol suppresses epithelial-to-mesenchymal transition in colorectal cancer through TGF-β1/Smads signaling pathway mediated Snail/E-cadherin expression. BMC Cancer 15:97. doi: 10.1186/s12885-015-1119-y
Kakarala, M., and Wicha, M. S. (2007). Cancer stem cells: implications for cancer treatment and prevention. Cancer J. 13, 271–275. doi: 10.1097/PPO.0b013e318156da4e
Kuhajda, F. P. (2000). Fatty-acid synthase and human cancer: new perspectives on its role in tumor biology. Nutrition 16, 202–208. doi: 10.1016/S0899-9007(99)00266-X
Li, J., Dong, L., Wei, D., Wang, X., Zhang, S., and Li, H. (2014). Fatty acid synthase mediates the epithelial-mesenchymal transition of breast cancer cells. Int. J. Biol. Sci. 10, 171–180. doi: 10.7150/ijbs.7357
Li, L., Chen, X., Liu, C. C., Lee, L. S., Man, C., and Cheng, S. H. (2016). Phytoestrogen bakuchiol exhibits in vitro and in vivo anti-breast cancer effects by inducing S phase arrest and apoptosis. Front. Pharmacol. 7:128. doi: 10.3389/fphar.2016.00128
Liou, G. Y., and Storz, P. (2010). Reactive oxygen species in cancer. Free Radic. Res. 44, 479–496. doi: 10.3109/10715761003667554
Liu, L., Chen, X., Wang, Y., Qu, Z., Lu, Q., Zhao, J., et al. (2014). Notch3 is important for TGF-β-induced epithelial-mesenchymal transition in non-small cell lung cancer bone metastasis by regulating ZEB-1. Cancer Gene Ther. 21, 364–372. doi: 10.1038/cgt.2014.39
Mani, S. A., Guo, W., Liao, M. J., Eaton, E. N., Ayyanan, A., Zhou, A. Y., et al. (2008). The epithelial-mesenchymal transition generates cells with properties of stem cells. Cell 133, 704–715. doi: 10.1016/j.cell.2008.03.027
Mariotto, A. B., Etzioni, R., Hurlbert, M., Penberthy, L., and Mayer, M. (2017). Estimation of the number of women living with metastatic breast cancer in the United States. Cancer Epidemiol. Biomarkers Prev. 26, 809–815. doi: 10.1158/1055-9965.EPI-16-0889
Menon, L. G., Kuttan, R., Nair, M. G., Chang, Y. C., and Kuttan, G. (1998). Effect of isoflavones genistein and daidzein in the inhibition of lung metastasis in mice induced by B16F-10 melanoma cells. Nutr. Cancer 30, 74–77. doi: 10.1080/01635589809514644
Montales, M. T., Rahal, O. M., Kang, J., Rogers, T. J., Prior, R. L., Wu, X., et al. (2012). Repression of mammosphere formation of human breast cancer cells by soy isoflavone genistein and blueberry polyphenolic acids suggests diet-mediated targeting of cancer stem-like/progenitor cells. Carcinogenesis 33, 652–660. doi: 10.1093/carcin/bgr317
Montales, M. T., Rahal, O. M., Nakatani, H., Matsuda, T., and Simmen, R. C. (2013). Repression of mammary adipogenesis by genistein limits mammosphere formation of human MCF-7 cells. J. Endocrinol. 218, 135–149. doi: 10.1530/JOE-12-0520
Pandey, A. K., Verma, G., Vig, S., Srivastava, S., Srivastava, A. K., and Datta, M. (2011). miR-29a levels are elevated in the db/db mice liver and its overexpression leads to attenuation of insulin action on PEPCK gene expression in HepG2 cells. Mol. Cell Endocrinol. 332, 125–133. doi: 10.1016/j.mce.2010.10.004
Patisaul, H. B., and Jefferson, W. (2010). The pros and cons of phytoestrogens. Front. Neuroendocrinol. 31, 400–419. doi: 10.1016/j.yfrne.2010.03.003
Pavese, J. M., Krishna, S. N., and Bergan, R. C. (2014). Genistein inhibits human prostate cancer cell detachment, invasion, and metastasis. Am. J. Clin. Nutr. 100(Suppl. 1), 431S–436S. doi: 10.3945/ajcn.113.071290
Peiris-Pagès, M., Martinez-Outschoorn, U. E., Sotgia, F., and Lisanti, M. P. (2015). Metastasis and oxidative stress: are antioxidants a metabolic driver of progression? Cell Metab. 22, 956–958. doi: 10.1016/j.cmet.2015.11.008
Peitzsch, C., Tyutyunnykova, A., Pantel, K., and Dubrovska, A. (2017). Cancer stem cells: the root of tumor recurrence and metastases. Semin. Cancer Biol. 44, 10–24. doi: 10.1016/j.semcancer.2017.02.011
Piskounova, E., Agathocleous, M., Murphy, M. M., Hu, Z., Huddlestun, S. E., Zhao, Z., et al. (2015). Oxidative stress inhibits distant metastasis by human melanoma cells. Nature 527, 186–191. doi: 10.1038/nature15726
Ryter, S. W., Kim, H. P., Hoetzel, A., Park, J. W., Nakahira, K., Wang, X., et al. (2007). Mechanisms of cell death in oxidative stress. Antioxid. Redox Signal. 9, 49–89. doi: 10.1089/ars.2007.9.49
Sansone, P., Storci, G., Giovannini, C., Pandolfi, S., Pianetti, S., Taffurelli, M., et al. (2007). p66Shc/Notch-3 interplay controls self-renewal and hypoxia survival in human stem/progenitor cells of the mammary gland expanded in vitro as mammospheres. Stem Cells 25, 807–815. doi: 10.1634/stemcells.2006-0442
Seguin, F., Carvalho, M. A., Bastos, D. C., Agostini, M., Zecchin, K. G., Alvarez-Flores, M. P., et al. (2012). The fatty acid synthase inhibitor orlistat reduces experimental metastases and angiogenesis in B16-F10 melanomas. Br. J. Cancer 107, 977–987. doi: 10.1038/bjc.2012.355
Sheen, Y. Y., Kim, M. J., Park, S. A., Park, S. Y., and Nam, J. S. (2013). Targeting the transforming growth factor-β signaling in cancer therapy. Biomol. Ther. 21, 323–331. doi: 10.4062/biomolther.2013.072
Sheth, S., Jajoo, S., Kaur, T., Mukherjea, D., Sheehan, K., Rybak, L. P., et al. (2012). Resveratrol reduces prostate cancer growth and metastasis by inhibiting the Akt/MicroRNA-21 pathway. PLOS ONE 7:e51655. doi: 10.1371/journal.pone.0051655
Shiozawa, Y., Nie, B., Pienta, K. J., Morgan, T. M., and Taichman, R. S. (2013). Cancer stem cells and their role in metastasis. Pharmacol. Ther. 138, 285–293. doi: 10.1016/j.pharmthera.2013.01.014
Singh, R., Yadav, V., Kumar, S., and Saini, N. (2015). MicroRNA-195 inhibits proliferation, invasion and metastasis in breast cancer cells by targeting FASN, HMGCR, ACACA and CYP27B1. Sci. Rep. 5:17454. doi: 10.1038/srep17454
Tat, J., Liu, M., and Wen, X. Y. (2013). Zebrafish cancer and metastasis models for in vivo drug discovery. Drug Discov. Today Technol. 10, e83–e89. doi: 10.1016/j.ddtec.2012.04.006
Teng, Y., Xie, X., Walker, S., White, D. T., Mumm, J. S., and Cowell, J. K. (2013). Evaluating human cancer cell metastasis in zebrafish. BMC Cancer 13:453. doi: 10.1186/1471-2407-13-453
Vantyghem, S. A., Wilson, S. M., Postenka, C. O., Al-Katib, W., Tuck, A. B., and Chambers, A. F. (2005). Dietary genistein reduces metastasis in a postsurgical orthotopic breast cancer model. Cancer Res. 65, 3396–3403. doi: 10.1158/0008-5472.CAN-04-4109
Velasco-Velázquez, M. A., Popov, V. M., Lisanti, M. P., and Pestell, R. G. (2011). The role of breast cancer stem cells in metastasis and therapeutic implications. Am. J. Pathol. 179, 2–11. doi: 10.1016/j.ajpath.2011.03.005
Woelfle, U., Sauter, G., Santjer, S., Brakenhoff, R., and Pantel, K. (2004). Down-regulated expression of cytokeratin 18 promotes progression of human breast cancer. Clin. Cancer Res. 10, 2670–2674. doi: 10.1158/1078-0432.CCR-03-0114
Yang, L., Zhang, F., Wang, X., Tsai, Y., Chuang, K. H., Keng, P. C., et al. (2016). A FASN-TGF-β1-FASN regulatory loop contributes to high EMT/metastatic potential of cisplatin-resistant non-small cell lung cancer. Oncotarget 7, 55543–55554. doi: 10.18632/oncotarget.10837
Zajchowski, D. A., Bartholdi, M. F., Gong, Y., Webster, L., Liu, H. L., Munishkin, A., et al. (2001). Identification of gene expression profiles that predict the aggressive behavior of breast cancer cells. Cancer Res. 61, 5168–5178.
Zaytseva, Y. Y., Harris, J. W., Mitov, M. I., Kim, J. T., Butterfield, D. A., Lee, E. Y., et al. (2015). Increased expression of fatty acid synthase provides a survival advantage to colorectal cancer cells via upregulation of cellular respiration. Oncotarget 6, 18891–18904. doi: 10.18632/oncotarget.3783
Zaytseva, Y. Y., Rychahou, P. G., Gulhati, P., Elliott, V. A., Mustain, W. C., O’Connor, K., et al. (2012). Inhibition of fatty acid synthase attenuates CD44-associated signaling and reduces metastasis in colorectal cancer. Cancer Res. 72, 1504–1517. doi: 10.1158/0008-5472.CAN-11-4057
Keywords: bakuchiol, breast cancer stem cells, zebrafish xenografts, apoptosis, metastasis
Citation: Li L, Liu CC, Chen X, Xu S, Hernandez Cortes-Manno S and Cheng SH (2017) Mechanistic Study of Bakuchiol-Induced Anti-breast Cancer Stem Cell and in Vivo Anti-metastasis Effects. Front. Pharmacol. 8:746. doi: 10.3389/fphar.2017.00746
Received: 18 July 2017; Accepted: 03 October 2017;
Published: 18 October 2017.
Edited by:
Salvatore Salomone, Università degli Studi di Catania, ItalyReviewed by:
Claudio Sorio, University of Verona, ItalyAnkita Thakkar, Burke Medical Research Institute, United States
Copyright © 2017 Li, Liu, Chen, Xu, Hernandez Cortes-Manno and Cheng. This is an open-access article distributed under the terms of the Creative Commons Attribution License (CC BY). The use, distribution or reproduction in other forums is permitted, provided the original author(s) or licensor are credited and that the original publication in this journal is cited, in accordance with accepted academic practice. No use, distribution or reproduction is permitted which does not comply with these terms.
*Correspondence: Shuk H. Cheng, YmhjaGVuZ0BjaXR5dS5lZHUuaGs=