- Department of Biochemistry and Molecular Biology and Physiology, Institute of Biology and Molecular Genetics (IBGM), Faculty of Medicine, University of Valladolid – CSIC, Valladolid, Spain
The sarco-endoplasmic reticulum Ca2+-ATPase (SERCA) refills the endoplasmic reticulum (ER) with Ca2+ up to the millimolar range and is therefore the main controller of the ER [Ca2+] level ([Ca2+]ER), which has a key role in the modulation of cytosolic Ca2+ signaling and ER-mitochondria Ca2+ transfer. Given that both cytosolic and mitochondrial Ca2+ dynamics strongly interplay with energy metabolism and nutrient-sensitive pathways, both of them involved in the aging process, we have studied the effect of SERCA inhibitors on lifespan in C. elegans. We have used thapsigargin and 2,5-Di-tert-butylhydroquinone (2,5-BHQ) as SERCA inhibitors, and the inactive analog 2,6-Di-tert-butylhydroquinone (2,6-BHQ) as a control for 2,5-BHQ. Every drug was administered to the worms either directly in the agar or via an inclusion compound with γ-cyclodextrin. The results show that 2,6-BHQ produced a small but significant increase in survival, perhaps because of its antioxidant properties. However, 2,5-BHQ produced in all the conditions a much higher increase in lifespan, and the potent and specific SERCA inhibitor thapsigargin also extended the lifespan. The effects of 2,5-BHQ and thapsigargin had a bell-shaped concentration dependence, with a maximum effect at a certain dose and smaller or even toxic effects at higher concentrations. Our data show therefore that submaximal inhibition of SERCA pumps has a pro-longevity effect, suggesting that Ca2+ signaling plays an important role in the aging process and that it could be a promising novel target pathway to act on aging.
Introduction
The molecular mechanisms responsible for aging are not yet fully understood. In recent years, evidence has accumulated suggesting a key role in aging for the so-called nutrient-sensitive signaling pathways: the insulin/IGF-1 pathway, the AMP kinase pathway (AMPK), the mTOR kinase pathway, and the sirtuin pathway (López-Otín et al., 2016; Riera et al., 2016). These pathways keep complex interactions among them at several levels, and one of them is Ca2+ signaling, a key messenger in cellular activation.
Both the mTOR and AMPK cascades are related to Ca2+ signaling, but the specific mechanisms are not known in detail (Decuypere et al., 2013). First, AMPK can be phosphorylated and activated by Ca2+-calmodulin protein kinase β (CaMKKβ or CaMKK2). In turn, AMPK inhibits mTORC1, so that this pathway provides an indirect link between the Ca2+ signal and mTOR. According to this mechanism, cytosolic [Ca2+] ([Ca2+]c) increase would inhibit mTOR, thus activating processes such as autophagy. In fact, there is a large body of evidence showing that [Ca2+]c signals from different sources can trigger autophagy by this “canonical” CaMKKβ-AMPK-mTORC1 pathway (Høyer-Hansen et al., 2007; Cárdenas and Foskett, 2012; Bootman et al., 2018).
On the other hand, there is also evidence that Ca2+ could have the contrary effect, that is, a direct activation of mTORC1. In this alternative pathway, [Ca2+]c signals would have an inhibitory effect on autophagy. For example, addition of amino acids to nutrient-deficient cells activates mTORC1, leading to suppression of autophagy (Carroll et al., 2015). It has been reported that this effect can be mediated by an amino acid-induced increase in [Ca2+]c that activates mTORC1 through binding of the Ca2+-calmodulin complex to a kinase (PI3K class III) necessary for the activation of mTOR (Gulati et al., 2008). Similarly, it has been reported that physical activity generates muscle hypertrophy through activation of Ca2+ entry into the cytosol, which would induce a direct activation of mTOR, responsible for initiating the hypertrophy process (Ito et al., 2013). In turn, it has also been described that mTOR activates the store-operated Ca2+-entry pathway (SOCE), through an increase in the expression of the STIM1/Orai1 proteins responsible for this pathway (Ogawa et al., 2012).
One of the key points in Ca2+ signaling linked to the activity of mTOR and AMPK is the inositol trisphosphate receptor (IP3R), which releases Ca2+ from the endoplasmic/sarcoplasmic reticulum (ER/SR) and is able to transfer Ca2+ from the ER to the mitochondria through close contacts that connect both organelles. These contacts, called Mitochondrial Associated ER Membranes (MAMs), contain both IP3R and mitochondrial Ca2+ uniporters (MCU), the main channel responsible for mitochondrial Ca2+ uptake. In addition, it has been reported that mTORC2 is also an important component of MAMs, able to activate AKT kinase, which may then phosphorylate and inhibit IP3R (Betz et al., 2013). Moreover, mTOR is also able to directly phosphorylate and activate IP3R (MacMillan et al., 2005; Frégeau et al., 2011; Régimbald-Dumas et al., 2011).
In turn, IP3R activity can also modulate the nutrient sensitive pathways. When ER Ca2+-release mediated by IP3R is reduced, both Ca2+-entry and ATP synthesis in mitochondria decrease. The resulting energy depletion activates AMPK, which then activates autophagy. The activity of the IP3R and the Ca2+-transfer between ER and mitochondria may therefore control AMPK and autophagy, and the final outcome may depend on the [Ca2+] transients induced in both cytosol and mitochondria after IP3R activation. ER-Ca2+ transfer to mitochondria fuels ATP production and decreases AMPK activity and autophagy. ER-Ca2+ release to the cytosol may activate AMPK and autophagy via CAMKKβ or alternatively activate directly mTOR and suppress autophagy (Cárdenas and Foskett, 2012; Parys et al., 2012; Bootman et al., 2018). Finally, resveratrol treatment decreases ER calcium storage and store-operated Ca2+-entry, inducing ER stress, activating AMPK and inhibiting the mTOR pathway (Selvaraj et al., 2016).
In conclusion, there is little doubt that [Ca2+]c dynamics modulates mTOR, AMPK and autophagy in a number of ways, although a complete understanding of the mechanisms involved requires much further work. We believe therefore that Ca2+ signaling is a route that is worth exploring for the modulation of nutrient-sensitive pathways and aging. In particular, the level of [Ca2+] in the ER ([Ca2+]ER) appears to be closely related to the activity of the nutrient sensitive pathways. In this work we have studied the effect of the inhibition of the sarco-endoplasmic reticulum Ca2+ ATPase (SERCA) on survival in C. elegans. The rationale of this treatment is that submaximal SERCA inhibition should decrease [Ca2+]ER, leading to a reduced Ca2+ release from the ER and a smaller Ca2+ transfer from ER to mitochondria. This effect should in theory activate AMPK, inhibit mTOR and have a pro-longevity effect. Our data show in fact that SERCA inhibitors induced a significant increase in C. elegans lifespan, suggesting that Ca2+ homeostasis plays an important role in the mechanisms responsible for aging and survival.
Materials and Methods
[Ca2+]ER Measurements With Aequorin in HeLa Cells
HeLa cells expressing double-mutated aequorin targeted to the ER (de la Fuente et al., 2013) were reconstituted with coelenterazine i, placed in the perfusion chamber of a purpose-built luminometer and perfused with external medium prior to the stimuli.
C. elegans Strains and Maintenance
Strains used were: AQ2038, integrated strain expressing cytosolic cameleon 2.1. (YC2.1) on pharynx due to the promoter sequence of the myo-2 gene (pmyo-2::YC2.1) (Alvarez-Illera et al., 2016), kindly provided by Drs. Robyn Branicky and W. Schafer, MRC Laboratory of Molecular Biology, Cambridge, United Kingdom. Its lifespan was not significantly different from that of the N2 strain (data not shown), and it is used here as a control strain to study the effects of the SERCA inhibitors. The mutant eat-2 strain (ad1113) was obtained from the Caenorhabditis Genetics Center. Worms were maintained and handled as previously described (Stiernagle, 2006). Hardened agar was seeded with Escherichia coli (OP50) and all the strains were maintained at 20°C.
Preparation of the γ-Cyclodextrin Inclusion Compounds
The γ-cyclodextrin inclusion compounds were prepared as described before (Kashima et al., 2012). Briefly, a 230 mg/ml water solution of γ-cyclodextrin was mixed 10:1 with a 50 mM DMSO solution of the corresponding compound, stirred in shaker at 1200 rpm during 20 h and centrifuged at 12,500 rpm for 10 min. The supernatant was carefully discarded and the resulting inclusion compound was dried in the hood and dissolved in M9 buffer. The inclusion compounds containing either 2,5-BHQ, 2,6-BHQ or thapsigargin were added directly to the plates in the amounts indicated before transferring the worms for the lifespan assay. In the case of 2,5-BHQ, in some assays it was dissolved directly in the Nematode Growth Medium (NGM) agar by strong stirring at the concentrations indicated. In the case of thapsigargin, in some assays it was added directly to the plate. 10 μl of either 10 or 50 μM thapsigargin were added on top of the 10 ml OP50-seeded NGM agar to obtain final concentrations (assuming homogeneous distribution) of 10 and 50 nM, respectively.
C. elegans Lifespan Assay
Eggs were obtained as described previously (Stiernagle, 2006) and transferred to E. coli (OP50) seeded NGM plates, either control plates or plates prepared in the presence of the required drug. For each assay, around 100 synchronized young adults (day 1) were transferred to E. coli (OP50) seeded NGM plates (35 mm plates, 10 worms/plate) containing 15 μM Fluorodeoxyuridine (FUdR) to avoid progeny. Control and drug-containing assays were always carried out in parallel. Plates were scored for dead worms every day. Worms that did not respond to touch with a platinum wire were considered dead. Age refers to days following adulthood. Plates with fungal contamination during the first 10 days of the assay were excluded from the study. Missing worms, individuals with extruded gonad or desiccated by crawling in the edge of the plate were censored, as well as plates with fungal contamination after the first 10 days. Control and drug-containing plates were kept close together in a temperature-controlled incubator set at 20°C. Statistics was made with the SPSS software using the Kaplan-Meier estimator and the log-rank routine for significance.
Materials
2,5-BHQ and 2,6-BHQ were form Sigma-Aldrich, Madrid, Spain. Thapsigargin was from Abcam, Madrid, Spain. γ-cyclodextrin was from PanReac, Barcelona, Spain. FuDR was from Alfa Aesar, Karlsruhe, Germany. Other reagents were from Sigma, Madrid, Spain or Merck, Darmstadt, Germany.
Results
The SERCA Inhibitor 2,5-BHQ Extends C. elegans Lifespan More Than the Inactive Analog 2,6-BHQ
In mammalians there are three SERCA isoforms, encoded by the ATP2A 1-3 genes, and further isoform diversity is generated by alternative processing of the primary gene transcripts at a conserved 3prime-terminal site (Lytton et al., 1992; Vandecaetsbeek et al., 2011). In the nematode Caenorhabditis elegans, there is only one SERCA homolog, encoded by the sca-1 gene (Cho et al., 2000; Zwaal et al., 2001), which has about 70% amino acid identity and 80% similarity to the three human SERCA proteins (Cho et al., 2000). The sca-1 transcript is also alternatively spliced in a way similar to that of mammalian SERCA2 gene, generating two protein variants which show differential functional characteristics and expression patterns (Cho et al., 2000). As all the mammalian SERCA isoforms, the C. elegans protein is also inhibited by thapsigargin, a very potent and specific irreversible inhibitor of SERCA pumps (Zwaal et al., 2001). In addition, all the known SERCA pumps are also reversibly inhibited by the small compound 2,5-di-tert-butylhydroquinone (2,5-BHQ). We have therefore used here both 2,5-BHQ and thapsigargin to study their effect on worm lifespan.
As a control for the activity of 2,5-BHQ, we have used 2,6-BHQ, a compound which is inactive on the SERCA (see Figure 1I), but should have very similar side effects, such as, for example, antioxidant properties. Figure 1I shows the effects of either 2,5-BHQ or 2,6-BHQ on the [Ca2+]ER measured in HeLa cells expressing ER-targeted aequorin. Addition of 2,5-BHQ rapidly induced a decrease in [Ca2+]ER due to the inhibition of the SERCA pump. In contrast, addition of 2,6-BHQ hardly produced any effect.
Because of the poor water solubility of 2,5-BHQ, we decided to use inclusion compounds for drug administration to the worms. 2,5-BHQ was enclosed in a chemical cover of γ-cyclodextrin, forming an inclusion compound (Kashima et al., 2012), which was then added to the plates together with the E. coli OP50. Young adults (day 1) were then transferred to these plates to start the lifespan assay. In this way, worms ingest the inclusion compound by the pharynx together with the food, and the active 2,5-BHQ is then released directly into the digestive tube. Table 1 shows the results of a series of lifespan assays performed with three concentrations of either 2,5-BHQ or 2,6-BHQ. Figure 1 shows representative lifespan assays performed in each of the conditions (2,5-BHQ, panels A–C; 2,6-BHQ, panels D–F). Although 2,6-BHQ produced small increases in survival, the pro-survival effect was much larger in the case of 2,5-BHQ, and its effect was concentration-dependent. The effect was maximum when 50 μg of the inclusion compound was added to the plate, and for this concentration all the lifespan assays were highly significant and produced increases in survival between 8.2 and 21.3% (mean 13.9 ± 2.4%). The effects on lifespan were smaller at concentrations of 2,5-BHQ below and above 50 μg. In contrast, for 2,6-BHQ, all the concentrations used produced smaller and similar increases in survival, and the effect showed a much higher variability.
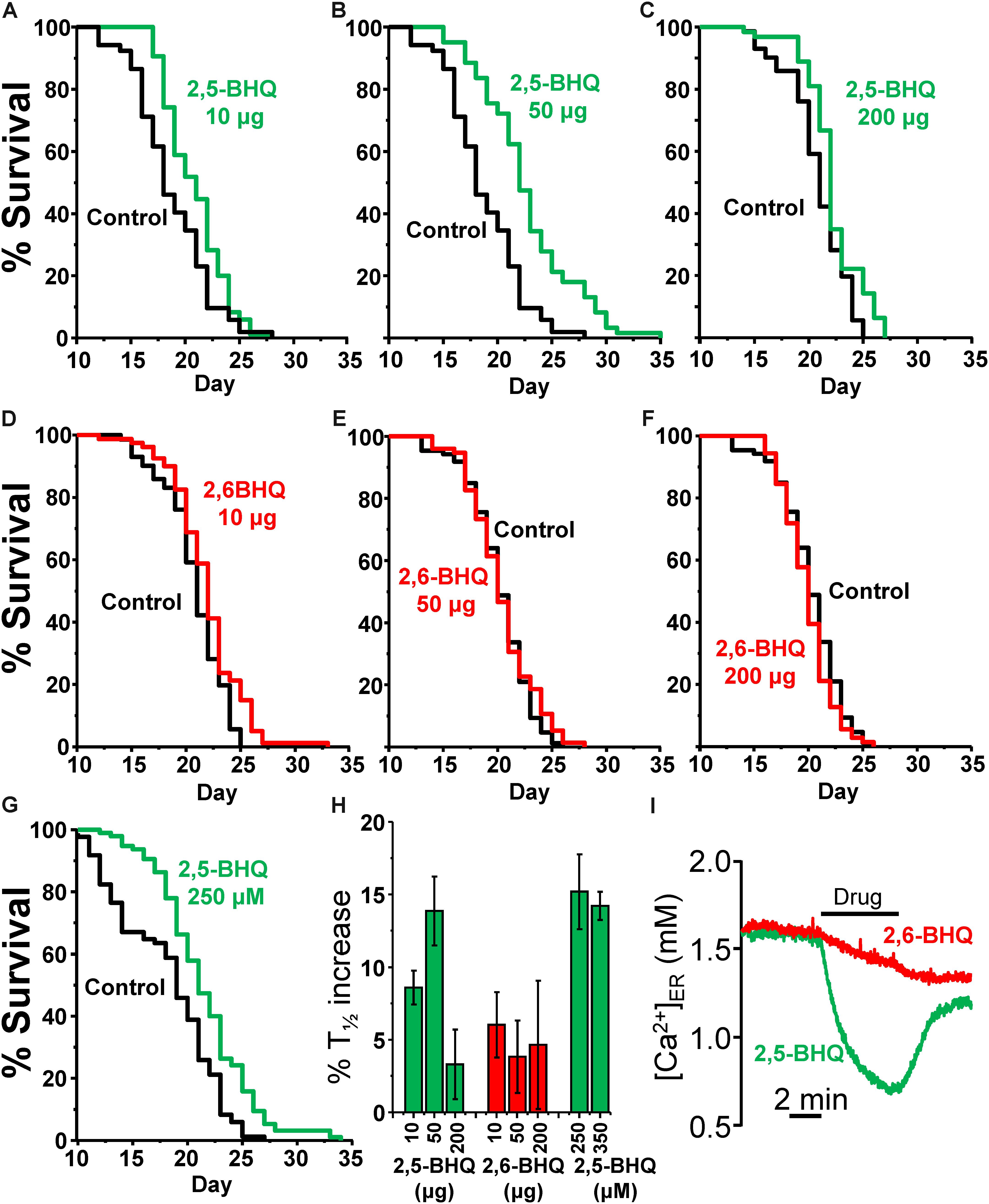
FIGURE 1. Effects of 2,5-BHQ and 2,6-BHQ on survival in C. elegans. Panels A–F show representative survival plots corresponding to parallel lifespan assays performed using γ-cyclodextrin-inclusion compounds in the following conditions: Control/2,5-BHQ (A:10 μg; B:50 μg; C:200 μg) and Control/2,6-BHQ (D:10 μg; E:50 μg; F:200 μg), respectively. (G) shows a representative survival plot from a Control/2,5-BHQ lifespan assay in which 250 μM 2,5-BHQ was dissolved in the NGM agar. The assays shown correspond to those marked in bold in Table 1. (H) shows the mean increase in survival obtained in a series of lifespan assays of each kind as those shown in the figure (more details of each assay in Table 1). (I) shows the effect of 10 μM of either 2,5-BHQ or 2,6-BHQ on the [Ca2+]ER level in HeLa cells. This experiment is representative of 6-7 similar ones.
In spite of the low water solubility of BHQ, we have also tested the effect of two concentrations of 2,5-BHQ directly dissolved in the NGM agar after strong stirring, 250 and 350 μM. Both produced also an increase in the C. elegans lifespan of similar magnitude as that induced by 50 μg of the compound (Table 1 and Figure 1G) showing that the effect is not dependent on the method of administration of the drug. Panel H shows a comparison of the mean increase in survival obtained in every condition.
The Specific SERCA Inhibitor Thapsigargin Extends C. elegans Lifespan at Low Concentrations
We have then studied the effect of thapsigargin, a very potent and specific irreversible inhibitor of SERCA pumps, on the lifespan of the worms. For drug administration, we have used two different mechanisms, either inclusion compounds or direct addition to the plates. In the first method, the inclusion compound with thapsigargin was prepared and used as mentioned above. In the second method, as previously described (Zwaal et al., 2001), the drug was added directly to the plates seeded with E. coli (OP50), and young adults (day 1) were then transferred to these plates to start the lifespan assay.
The same effects were obtained with both drug administration methods. Table 2 shows the results of a series of lifespan assays performed with three concentrations of γ-cyclodextrin enclosed thapsigargin (0.1, 1, and 10 μg) and two concentrations of thapsigargin added directly to the plates (10 and 50 nM). Representative lifespan assays performed in each of the conditions are shown in Figures 2A–E, together with the mean increase in survival obtained from the different assays made at each thapsigargin concentration (Figure 2F). Thapsigargin produced also an increase in survival which was dependent on the concentration. The effect was maximum at 1 μg of the inclusion compound (mean increase 8.0 ± 0.3%), and was smaller at concentrations below and above that. Also, when the compound was added directly to the plates, the increase in lifespan was obtained at 10 nM (mean increase 9.6 ± 2.1%), but disappeared at 50 nM.
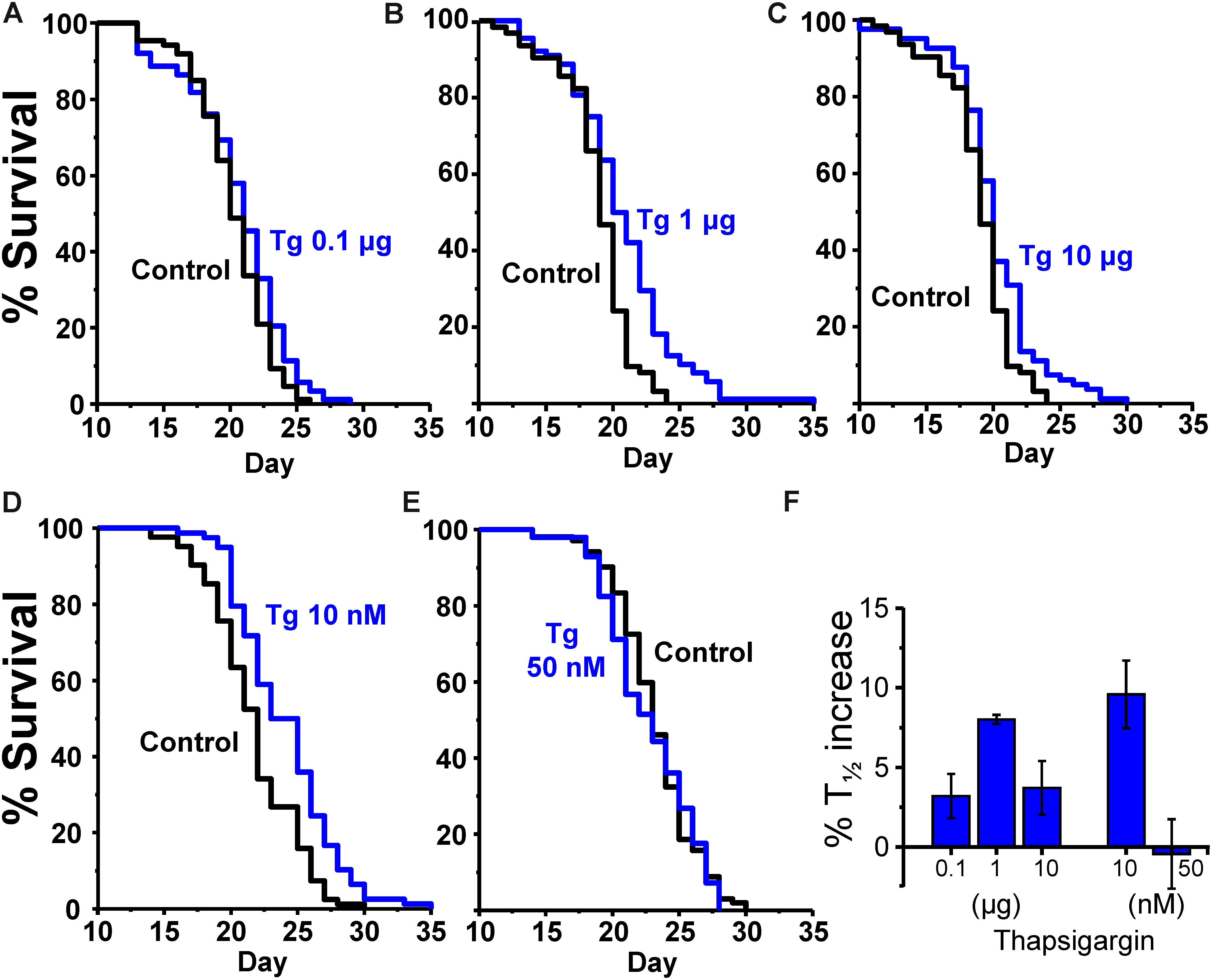
FIGURE 2. Effects of thapsigargin on survival in C. elegans. (A–C) show representative survival plots corresponding to parallel lifespan assays performed using γ-cyclodextrin-inclusion compounds in the following conditions: Control/thapsigargin (A:0.1 μg; B: 1μg; C:10 μg). (D,E) Show representative survival plots from Control/thapsigargin lifespan assays in which thapsigargin was directly added onto the OP50-seeded NGM agar (final concentration 10 or 50 nM). The assays correspond to those marked in bold in Table 2. (F) Shows the mean increase in survival obtained in a series of lifespan assays of each kind as those shown in the figure (more details of each assay in Table 2).
SERCA Inhibitors Extend the Lifespan in Eat-2 Mutants
To exclude that the mechanism of the increased lifespan in the presence of the SERCA inhibitors could be related to caloric restriction, we have studied the effects of the SERCA inhibitors on the survival of eat-2 mutants. These mutants have a reduced rate of pharynx pumping and an increased lifespan, which has been shown to be due to caloric restriction (Lakowski and Hekimi, 1998). Table 3 shows the results of a series of lifespan assays performed with several concentrations of 2,5-BHQ, 2,6-BHQ and thapsigargin in eat-2 (ad1113) mutants. Representative plots of assays made at each of the conditions studied are shown in Figure 3. The results show that SERCA inhibitors increased also the lifespan of eat-2 (ad1113) worms with a similar magnitude to that found in wild-type worms. Therefore, this suggests that caloric restriction is not involved in the mechanism of the lifespan increase induced by these compounds.
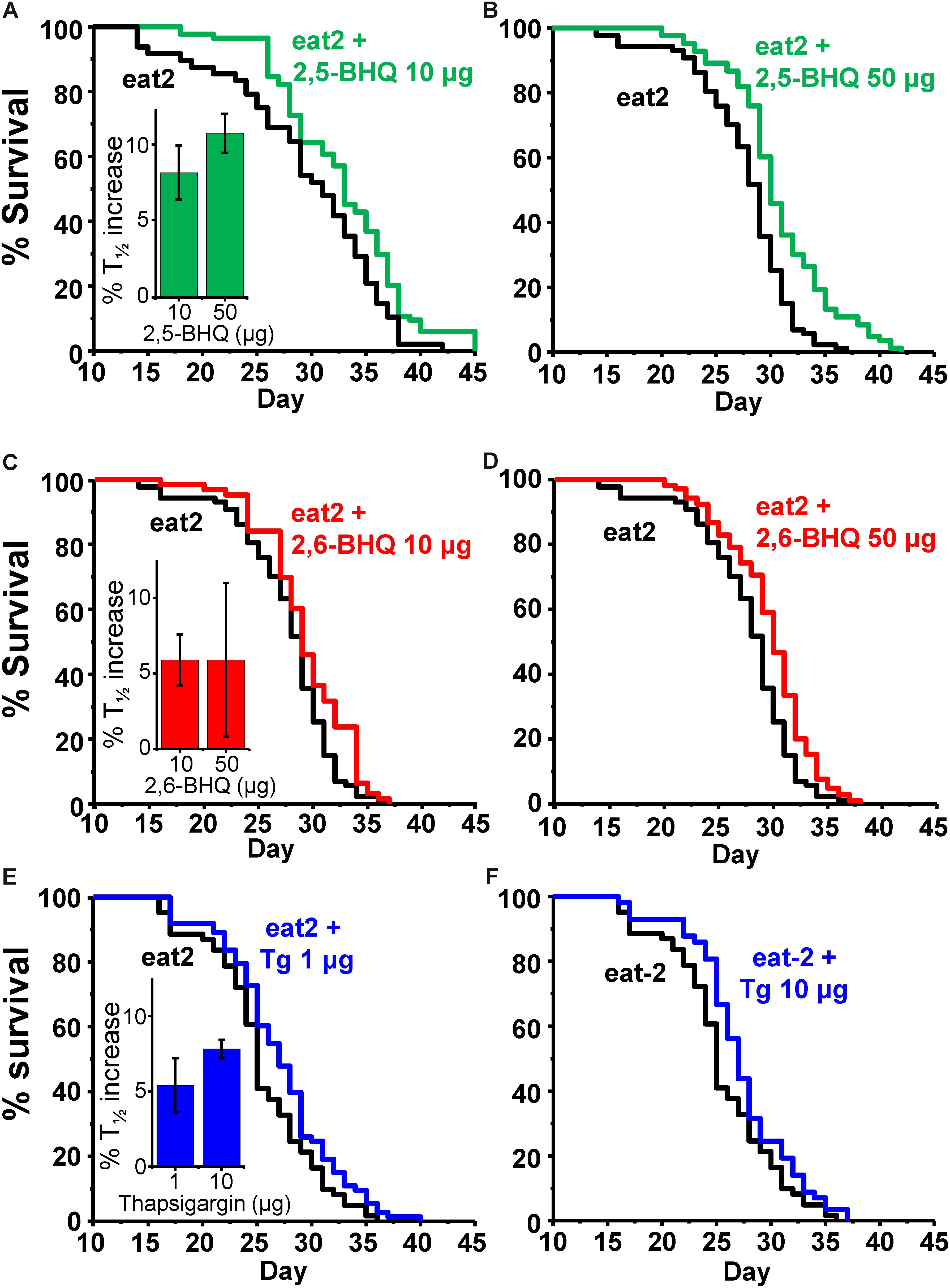
FIGURE 3. Effect of SERCA inhibitors on survival in eat-2 C. elegans mutants. The panels show representative survival plots corresponding to parallel lifespan assays performed using γ-cyclodextrin-inclusion compounds in the following conditions: eat-2/ eat-2 + 2,5-BHQ (A:10 μg; B:50 μg), eat-2/ eat-2 + 2,6-BHQ (C:10 μg; D:50 μg) and eat-2/ eat-2 + thapsigargin (E:1 μg, F:10 μg). The assays correspond to those marked in bold in Table 3. The inserts show the mean increase in survival obtained in a series of lifespan assays of each kind as those shown in the figure (more details of each assay in Table 3).
Discussion
Intracellular Ca2+ dynamics is a key controller of cellular metabolism. The rate of mitochondrial ATP synthesis depends on the [Ca2+] inside mitochondria ([Ca2+]M), which stimulates several key dehydrogenases responsible for NADH production (McCormack et al., 1990). As a consequence of this, an increase in [Ca2+]M immediately leads to an increase in ATP production (Robb-Gaspers et al., 1998; Jouaville et al., 1999). The increase in [Ca2+]M is generally a consequence of the stimulation of Ca2+-release from the ER that occurs during cell activation. This mechanism constitutes a basic homeostatic response that associates cell activation to energy production, in order to cover the energy requirements of the response to the stimulus.
On the other hand, the increase in the level of [Ca2+]c is directly responsible for the activation of many cellular responses that consume energy, such as muscle contraction, secretion, proliferation, hypertrophy and many metabolic reactions (Berridge et al., 2000). In addition, many Ca2+ signals adopt prolonged oscillatory patterns that require a continuous activity of the Ca2+ pumps to restore the Ca2+ gradients after each oscillation. These oscillatory Ca2+ patterns consume large amounts of energy only at the level of the Ca2+ pumps, both in the plasma membrane and in the ER. It has been shown, for example, that ATP consumption by SERCA pumps accounts for 40–50% of the resting metabolic consumption in mouse skeletal muscle (Smith et al., 2013).
The concepts of energy balance and nutrient-sensitive pathways are considered nowadays key determinants of lifespan (López-Otín et al., 2016; Riera et al., 2016). As Ca2+ signaling is tightly related to both of them, we decided to study the effects of Ca2+ modulators on survival. On the first place, we have investigated the effect of submaximal inhibition of the SERCA pumps, the Ca2+ pumps responsible for Ca2+-storage in the ER. The level of [Ca2+]ER is key to determine the amount of Ca2+ released during cell stimulation, not only because the rate of Ca2+ release depends on the Ca2+-gradient between the ER and the cytosol, but mainly because [Ca2+]ER is a very important activator of both IP3R and ryanodine receptors (Yamasaki-Mann et al., 2010; Zhang et al., 2015). As a consequence, partial Ca2+ depletion of the ER should reduce both [Ca2+]c signaling and ER-mitochondria Ca2+ transfer during cell stimulation.
To test this hypothesis, we have used two inhibitors of SERCA pumps, 2,5-BHQ and thapsigargin. Thapsigargin is the most potent and specific SERCA inhibitor available. It binds SERCA with a Kd in the sub-nM range (Thastrup et al., 1990) and stabilizes the Ca2+-free conformation, a property which has been useful to obtain SERCA crystals for diffraction studies (Toyoshima et al., 2000; Inesi et al., 2005, 2006). Thapsigargin has been reported to act also on different targets, particularly on plasma membrane Ca2+ channels (Rossier et al., 1993; Nelson et al., 1994; Shmigol et al., 1995). However, this off-target effect required always much higher concentrations, in the micromolar range. It is therefore considered highly specific for SERCA inhibition at low concentrations, in the nM range.
Regarding 2,5-BHQ, it has been known to be a SERCA inhibitor for more than 30 years (Moore et al., 1987). It binds SERCA close to the thapsigargin-binding site, although not in the same place, and stabilizes also the Ca2+-free conformation (Inesi et al., 2005). The Kd for SERCA inhibition by 2,5-BHQ is higher, around 1–2 μM (Moore et al., 1987; Llopis et al., 1991), and this compound has been shown to inhibit also some additional targets, including L-type Ca2+ channels (Nelson et al., 1994; Scamps et al., 2000; Miller et al., 2015) other types of Ca2+ channels (Scamps et al., 2000) or the plasma membrane Ca2+ ATPase (Luo et al., 2000). Most of these off-target effects of 2,5-BHQ required also higher concentrations of the drug (IC50 ≥ 30 μM), but could be significant at the concentrations usually applied to obtain maximum SERCA inhibition (∼10 μM). In addition, 2,5-BHQ has also been shown to generate superoxide anion (Tsukii et al., 1996) and inhibition of L-type Ca2+ channels by this compound (with IC50 = 66 μM) has been attributed to this effect (Fusi et al., 2001). On the other hand, this compound belongs to a family of phenolic antioxidants, some of which are used in food preservation. To account for these alternative effects of 2,5-BHQ, we have used the analog 2,6-BHQ, which is inactive on the SERCA pump but should have similar chemical properties, particularly on oxidative processes. Regarding the 2,5-BHQ concentration in our experiments, it is difficult to know the exact concentration inside the worms, as it depends on the lipophilicity and bioavailability of the drug. Usually, the drug concentrations in the NGM agar required to produce effects in the worms are 10–100 fold higher than those necessary in cell cultures. Assuming that we are producing submaximal inhibition of SERCA, worm concentrations should be close to the IC50, around 1–2μM.
Our data show in fact that submaximal inhibition of SERCA pumps with 2,5-BHQ or thapsigargin produced a significant increase in the lifespan in C. elegans worms. The effect on survival of both inhibitors was concentration-dependent, reached a maximum at a particular concentration, and decayed below and above it. Our data show that, although 2,6-BHQ produced a small increase in survival, the lifespan extension induced by 2,5-BHQ was 3-fold larger, suggesting that SERCA inhibition is clearly involved in the effect. The effect of 2,6-BHQ could be attributed to its antioxidant properties, although antioxidants may have variable effects of C. elegans lifespan (Desjardins et al., 2017). Regarding thapsigargin, it is a much more potent and specific SERCA inhibitor, so that much lower concentrations need to be used to obtain the effects and fewer side-effects are expected to occur. It is also an irreversible inhibitor of SERCA, and it has been shown before that inhibits the SERCA pump in C. elegans and that concentrations in the plate above ∼200 nM are deleterious for worm fertility and growth (Zwaal et al., 2001). This is consistent with the fact that knockout of the SERCA homolog in C. elegans, sca-1, results in embryonic and larval lethality (Cho et al., 2000; Zwaal et al., 2001). In our hands, an increase in the lifespan was obtained with a much lower concentration, just 10 nM, and the effect disappeared when the concentration was increased to 50 nM. At this concentration, the effects of thapsigargin should be highly specific on SERCA.
The molecular mechanisms linking SERCA inhibition and lifespan extension still await clarification. We have seen that the SERCA pump inhibitors increased also with a similar magnitude the lifespan of eat-2 (ad1113) mutant worms, a model for caloric restriction because of the reduced rate of pharynx pumping (Lakowski and Hekimi, 1998). This suggests that the increase in lifespan induced by the compounds is not due to caloric restriction, but rather to the effect of SERCA inhibition on Ca2+ signaling. The SERCA pump plays a key role in shaping the intracellular [Ca2+] transients. Its activity determines the level of filling with Ca2+ of the ER/SR, which is one of the factors conditioning the rate of Ca2+ release through either IP3R or ryanodine receptors. In addition, ER/SR Ca2+ reuptake after stimulation also depends on SERCA activity. Our hypothesis is that a decrease in SERCA activity would reduce [Ca2+]ER, with the subsequent decrease in Ca2+ release, Ca2+ transfer from ER to mitochondria and metabolic activity. It is remarkable that SERCA is one of the proteins that undergo a largest decrease in concentration during aging in C. elegans. In fact, sca-1 decreases in abundance nearly 10-fold from day 4 to day 10 of worm life (Copes et al., 2015). This phenomenon is not specific of C. elegans, because a marked decrease in SERCA2a mRNA and activity during aging has also been reported in mammals (Feridooni et al., 2015). This decrease could be interpreted in several ways, but perhaps could reflect the fact that excess Ca2+ signaling is negative for survival during aging. Young individuals have a higher SERCA content, and this may be optimal for rapid response to external stimuli. Older individuals have instead a much smaller SERCA content, and this may dampen Ca2+ signaling and favor survival. Submaximal SERCA inhibition may extend lifespan in this way. Further work will be necessary to clarify the molecular mechanism involved in the effect of SERCA inhibitors. Measuring the changes in [Ca2+]ER along the life of the worm, although challenging from a experimental point of view, would be a exciting approach. In addition, the decrease in [Ca2+]ER should produce changes in the cytosolic Ca2+ dynamics, which could be detected in live treated or untreated worms. Using other mutants with alterations in nutrient-sensitive pathways or with ER stress may also provide clues on the mechanism of the pro-survival effect of SERCA inhibitors.
In summary, we have shown here that partial inhibition of SERCA pumps increases the lifespan in C. elegans. This indicates that Ca2+ signaling plays a role in the aging process and that reduction in [Ca2+]ER and ER-Ca2+ release has a pro-longevity effect. Our data also suggest that Ca2+ signaling could be a promising novel target pathway to act on aging.
Data Availability Statement
The raw data supporting the conclusions of this manuscript will be made available by the authors, without undue reservation, to any qualified researcher.
Author Contributions
MM and JA designed the project. PG-C performed most of the lifespan experiments. JA-d-V and PA-I joined in performing some of them. JA wrote the manuscript and RF and MM helped in discussing and editing the manuscript. All authors read and approved the final manuscript.
Funding
This work was supported by a grant from the Spanish Ministerio de Economía y Competitividad [BFU2014-55731-R], project co-financed by the European Union through the European Regional Development Fund. Some C. elegans strains were provided by the Caenorhabditis Genetics Center (CGC), which is funded by NIH Office of Research Infrastructure Programs (P40 OD010440). JA-d-V has a fellowship from Junta de Castilla y León (JCyL), co-financed by the Fondo Social Europeo (FSE). PG-C has a FPI fellowship from Ministerio de Economía y Competitividad.
Conflict of Interest Statement
The authors declare that the research was conducted in the absence of any commercial or financial relationships that could be construed as a potential conflict of interest.
Acknowledgments
We thank Drs. Robyn Branicky and W. Schafer for the pharynx YC2.1 cameleon expressing AQ2038 line.
References
Alvarez-Illera, P., Sanchez-Blanco, A., Lopez-Burillo, S., Fonteriz, R. I., Alvarez, J., and Montero, M. (2016). Long-term monitoring of Ca2+ dynamics in C. elegans pharynx: an in vivo energy balance sensor. Oncotarget 7, 67732–67747. doi: 10.18632/oncotarget.12177
Berridge, M. J., Lipp, P., and Bootman, M. D. (2000). The versatility and universality of calcium signalling. Nat. Rev. Mol. Cell Biol. 1, 11–21. doi: 10.1038/35036035
Betz, C., Stracka, D., Prescianotto-Baschong, C., Frieden, M., Demaurex, N., and Hall, M. N. (2013). Feature article: mTOR complex 2-Akt signaling at mitochondria-associated endoplasmic reticulum membranes (MAM) regulates mitochondrial physiology. Proc. Natl. Acad. Sci. U.S.A. 110, 12526–12534. doi: 10.1073/pnas.1302455110
Bootman, M. D., Chehab, T., Bultynck, G., Parys, J. B., and Rietdorf, K. (2018). The regulation of autophagy by calcium signals: Do we have a consensus? Cell Calcium 70, 32–46. doi: 10.1016/j.ceca.2017.08.005
Cárdenas, C., and Foskett, J. K. (2012). Mitochondrial Ca2+ signals in autophagy. Cell Calcium 52, 44–51. doi: 10.1016/j.ceca.2012.03.001
Carroll, B., Korolchuk, V. I., and Sarkar, S. (2015). Amino acids and autophagy: cross-talk and co-operation to control cellular homeostasis. Amino Acids 47, 2065–2088. doi: 10.1007/s00726-014-1775-2
Cho, J. H., Bandyopadhyay, J., Lee, J., Park, C. S., and Ahnn, J. (2000). Two isoforms of sarco/endoplasmic reticulum calcium ATPase (SERCA) are essential in Caenorhabditis elegans. Gene 261, 211–219. doi: 10.1016/S0378-1119(00)00536-9
Copes, N., Edwards, C., Chaput, D., Saifee, M., Barjuca, I., Nelson, D., et al. (2015). Metabolome and proteome changes with aging in Caenorhabditis elegans. Exp. Gerontol. 72, 67–84. doi: 10.1016/j.exger.2015.09.013
de la Fuente, S., Fonteriz, R. I., Montero, M., and Alvarez, J. (2013). Ca2+ homeostasis in the endoplasmic reticulum measured with a new low-Ca2+-affinity targeted aequorin. Cell Calcium 54, 37–45. doi: 10.1016/j.ceca.2013.04.001
Decuypere, J.-P., Paudel, R. C., Parys, J., and Bultynck, G. (2013). Intracellular Ca2+ signaling: a novel player in the canonical mTOR-controlled autophagy pathway. Commun. Integr. Biol. 6:e25429. doi: 10.4161/cib.25429
Desjardins, D., Cacho-Valadez, B., Liu, J. L., Wang, Y., Yee, C., Bernard, K., et al. (2017). Antioxidants reveal an inverted U-shaped dose-response relationship between reactive oxygen species levels and the rate of aging in Caenorhabditis elegans. Aging Cell 16, 104–112. doi: 10.1111/acel.12528
Feridooni, H. A., Dibb, K. M., and Howlett, S. E. (2015). How cardiomyocyte excitation, calcium release and contraction become altered with age. J. Mol. Cell. Cardiol. 83, 62–72. doi: 10.1016/j.yjmcc.2014.12.004
Frégeau, M.-O., Régimbald-Dumas, Y., and Guillemette, G. (2011). Positive regulation of inositol 1,4,5-trisphosphate-induced Ca2+ release by mammalian target of rapamycin (mTOR) in RINm5F cells. J. Cell. Biochem. 112, 723–733. doi: 10.1002/jcb.23006
Fusi, F., Saponara, S., Gagov, H., and Sgaragli, G. (2001). 2,5-Di-t-butyl-1,4-benzohydroquinone (BHQ) inhibits vascular L-type Ca2+ channel via superoxide anion generation. Br. J. Pharmacol. 133, 988–996. doi: 10.1038/sj.bjp.0704183
Gulati, P., Gaspers, L. D., Dann, S. G., Joaquin, M., Nobukuni, T., Natt, F., et al. (2008). Amino acids activate mTOR complex 1 via Ca2+/CaM signaling to hVps34. Cell Metab. 7, 456–465. doi: 10.1016/j.cmet.2008.03.002
Høyer-Hansen, M., Bastholm, L., Szyniarowski, P., Campanella, M., Szabadkai, G., Farkas, T., et al. (2007). Control of macroautophagy by calcium, calmodulin-dependent kinase kinase-β, and Bcl-2. Mol. Cell 25, 193–205. doi: 10.1016/j.molcel.2006.12.009
Inesi, G., Hua, S., Xu, C., Ma, H., Seth, M., Prasad, A. M., et al. (2005). Studies of Ca2+ ATPase (SERCA) Inhibition. J. Bioenerg. Biomembr. 37, 365–368. doi: 10.1007/s10863-005-9472-1
Inesi, G., Lewis, D., Ma, H., and Prasad, A. (2006). Concerted conformational effects of Ca2+ and ATP are required for activation of sequential reactions in the Ca2+ ATPase (SERCA) catalytic cycle. Biochemistry 45, 13769–13778. doi: 10.1021/bi061255d
Ito, N., Ruegg, U. T., Kudo, A., Miyagoe-Suzuki, Y., and Takeda, S. (2013). Activation of calcium signaling through Trpv1 by nNOS and peroxynitrite as a key trigger of skeletal muscle hypertrophy. Nat. Med. 19, 101–106. doi: 10.1038/nm.3019
Jouaville, L. S., Pinton, P., Bastianutto, C., Rutter, G. A., and Rizzuto, R. (1999). Regulation of mitochondrial ATP synthesis by calcium: evidence for a long-term metabolic priming. Proc. Natl. Acad. Sci. U.S.A. 96, 13807–13812. doi: 10.1073/pnas.96.24.13807
Kashima, N., Fujikura, Y., Komura, T., Fujiwara, S., Sakamoto, M., Terao, K., et al. (2012). Development of a method for oral administration of hydrophobic substances to Caenorhabditis elegans: pro-longevity effects of oral supplementation with lipid-soluble antioxidants. Biogerontology 13, 337–344. doi: 10.1007/s10522-012-9378-3
Lakowski, B., and Hekimi, S. (1998). The genetics of caloric restriction in Caenorhabditis elegans. Proc. Natl. Acad. Sci. U.S.A. 95, 13091–13096. doi: 10.1073/pnas.95.22.13091
Llopis, J., Chow, S. B., Kass, G. E., Gahm, A., and Orrenius, S. (1991). Comparison between the effects of the microsomal Ca2+-translocase inhibitors thapsigargin and 2,5-di-(t-butyl)-1,4-benzohydroquinone on cellular calcium fluxes. Biochem. J. 277(Pt 2), 553–556. doi: 10.1042/bj2770553
López-Otín, C., Galluzzi, L., Freije, J. M. P., Madeo, F., and Kroemer, G. (2016). Metabolic control of longevity. Cell 166, 802–821. doi: 10.1016/j.cell.2016.07.031
Luo, D., Nakazawa, M., Yoshida, Y., Cai, J., and Imai, S. (2000). Effects of three different Ca2+pump ATPase inhibitors on evoked contractions in rabbit aorta and activities of Ca2+pump ATPases in porcine aorta. Gen. Pharmacol. Vasc. Syst. 34, 211–220. doi: 10.1016/S0306-3623(00)00064-1
Lytton, J., Westlin, M., Burk, S. E., Shull, G. E., and MacLennan, D. H. (1992). Functional comparisons between isoforms of the sarcoplasmic or endoplasmic reticulum family of calcium pumps. J. Biol. Chem. 267, 14483–14489.
MacMillan, D., Currie, S., Bradley, K. N., Muir, T. C., and McCarron, J. G. (2005). In smooth muscle, FK506-binding protein modulates IP3 receptor-evoked Ca2+ release by mTOR and calcineurin. J. Cell Sci. 118, 5443–5451. doi: 10.1242/jcs.02657
McCormack, J. G., Halestrap, A. P., and Denton, R. M. (1990). Role of calcium ions in regulation of mammalian intramitochondrial metabolism. Physiol. Rev. 70, 391–425. doi: 10.1152/physrev.1990.70.2.391
Miller, L., Greensmith, D. J., Sankaranarayanan, R., Neill, S. C. O., and Eisner, D. A. (2015). Cell Calcium intracellular Ca2+ handling in rat ventricular myocytes. Cell Calcium 58, 208–214. doi: 10.1016/j.ceca.2015.05.002
Moore, G. A., McConkey, D. J., Kass, G. E. N., O’Brien, P. J., and Orrenius, S. (1987). 2,5-Di(tert-butyl)-1,4-benzohydroquinone - a novel inhibitor of liver microsomal Ca2+ sequestration. FEBS Lett. 224, 331–336. doi: 10.1016/0014-5793(87)80479-9
Nelson, E. J., Li, C. C., Bangalore, R., Benson, T., Kass, R. S., and Hinkle, P. M. (1994). Inhibition of L-type calcium-channel activity by thapsigargin and 2,5-t-butylhydroquinone, but not by cyclopiazonic acid. Biochem. J. 302(Pt 1), 147–154. doi: 10.1042/bj3020147
Ogawa, A., Firth, A. L., Smith, K. A., Maliakal, M. V., and Yuan, J. X.-J. (2012). PDGF enhances store-operated Ca2+ entry by upregulating STIM1/Orai1 via activation of Akt/mTOR in human pulmonary arterial smooth muscle cells. Am. J. Physiol. Cell Physiol. 302, C405–C411. doi: 10.1152/ajpcell.00337.2011
Parys, J. B., Decuypere, J.-P., and Bultynck, G. (2012). Role of the inositol 1,4,5-trisphosphate receptor/Ca2+-release channel in autophagy. Cell Commun. Signal. 10:17. doi: 10.1186/1478-811X-10-17
Régimbald-Dumas, Y., Frégeau, M.-O., and Guillemette, G. (2011). Mammalian target of rapamycin (mTOR) phosphorylates inositol 1,4,5-trisphosphate receptor type 2 and increases its Ca2+ release activity. Cell. Signal. 23, 71–79. doi: 10.1016/j.cellsig.2010.08.005
Riera, C. E., Merkwirth, C., De Magalhaes Filho, C. D., and Dillin, A. (2016). Signaling networks determining life span. Annu. Rev. Biochem. 85, 35–64. doi: 10.1146/annurev-biochem-060815-014451
Robb-Gaspers, L. D., Burnett, P., Rutter, G. A., Denton, R. M., Rizzuto, R., and Thomas, A. P. (1998). Integrating cytosolic calcium signals into mitochondrial metabolic responses. EMBO J. 17, 4987–5000. doi: 10.1093/emboj/17.17.4987
Rossier, M. F., Python, C. P., Burnay, M. M., Schlegel, W., Vallotton, M. B., and Capponi, A. M. (1993). Thapsigargin inhibits voltage-activated calcium channels in adrenal glomerulosa cells. Biochem. J. 296(Pt 2), 309–312. doi: 10.1042/bj2960309
Scamps, F., Vigues, S., Restituito, S., Campo, B., Roig, A., Charnet, P., et al. (2000). Sarco-endoplasmic ATPase blocker 2,5-Di(tert-butyl)-1,4-benzohydroquinone inhibits N-, P-, and Q- but not T-, L-, or R-type calcium currents in central and peripheral neurons. Mol. Pharmacol. 58, 18–26. doi: 10.1124/mol.58.1.18
Selvaraj, S., Sun, Y., Sukumaran, P., and Singh, B. B. (2016). Resveratrol activates autophagic cell death in prostate cancer cells via downregulation of STIM1 and the mTOR pathway. Mol. Carcinog. 55, 818–831. doi: 10.1002/mc.22324
Shmigol, A., Kostyuk, P., and Verkhratsky, A. (1995). Dual action of thapsigargin on calcium mobilization in sensory neurons: inhibition of Ca2+ uptake by caffeine-sensitive pools and blockade of plasmalemmal Ca2+ channels. Neuroscience 65, 1109–1118. doi: 10.1016/0306-4522(94)00553-H
Smith, I. C., Bombardier, E., Vigna, C., and Tupling, A. R. (2013). ATP consumption by sarcoplasmic reticulum Ca2+ pumps accounts for 40-50% of resting metabolic rate in mouse fast and slow twitch skeletal muscle. PLoS One 8:e68924. doi: 10.1371/journal.pone.0068924
Stiernagle, T. (2006). “Maintenance of C. elegans,” in WormBook, ed. The C. elegans Research Community (Minneapolis, MN: WormBook), 1–11. doi: 10.1895/wormbook.1.101.1
Thastrup, O., Cullen, P. J., Drobak, B. K., Hanley, M. R., and Dawson, A. P. (1990). Thapsigargin, a tumor promoter, discharges intracellular Ca2+ stores by specific inhibition of the endoplasmic reticulum Ca2+-ATPase. Proc. Natl. Acad. Sci. U.S.A. 87, 2466–2470. doi: 10.1073/pnas.87.7.2466
Toyoshima, C., Nakasako, M., Nomura, H., and Ogawa, H. (2000). Crystal structure of the calcium pump of sarcoplasmic reticulum at 2.6 A resolution. Nature 405, 647–655. doi: 10.1038/35015017
Tsukii, K., Nakahata, N., Watanabe, K., Tsurufuji, S., and Ohizumi, Y. (1996). Comparison of tBuBHQ with chemotactic peptide and phorbol ester in O-2 production in HL-60 cells. Eur. J. Pharmacol. 298, 87–95. doi: 10.1016/0014-2999(95)00787-3
Vandecaetsbeek, I., Vangheluwe, P., Raeymaekers, L., Wuytack, F., and Vanoevelen, J. (2011). The Ca2+ pumps of the endoplasmic reticulum and golgi apparatus. Cold Spring Harb. Perspect. Biol. 3:a004184. doi: 10.1101/cshperspect.a004184
Yamasaki-Mann, M., Demuro, A., and Parker, I. (2010). Modulation of endoplasmic reticulum Ca2+ store filling by cyclic ADP-ribose promotes inositol trisphosphate (IP3)-evoked Ca2+ signals. J. Biol. Chem. 285, 25053–25061. doi: 10.1074/jbc.M109.095257
Zhang, J. Z., Waddell, H. M., and Jones, P. P. (2015). Regulation of RYR2 by sarcoplasmic reticulum Ca2+. Clin. Exp. Pharmacol. Physiol. 42, 720–726. doi: 10.1111/1440-1681.12364
Keywords: C. elegans, lifespan, Ca2+ signaling, SERCA, thapsigargin, aging, calcium, endoplasmic reticulum
Citation: García-Casas P, Arias-del -Val J, Alvarez -Illera P, Fonteriz RI, Montero M and Alvarez J (2018) Inhibition of Sarco-Endoplasmic Reticulum Ca2+ ATPase Extends the Lifespan in C. elegans Worms. Front. Pharmacol. 9:669. doi: 10.3389/fphar.2018.00669
Received: 15 March 2018; Accepted: 04 June 2018;
Published: 25 June 2018.
Edited by:
Paul Roos Ernsberger, Case Western Reserve University, United StatesReviewed by:
InKyeom Kim, Kyungpook National University, South KoreaHong Zhan, University of Wisconsin-Madison, United States
Sudheer Kumar Ravuri, Steadman Philippon Research Institute, United States
Copyright © 2018 García-Casas, Arias-del-Val, Alvarez-Illera, Fonteriz, Montero and Alvarez. This is an open-access article distributed under the terms of the Creative Commons Attribution License (CC BY). The use, distribution or reproduction in other forums is permitted, provided the original author(s) and the copyright owner are credited and that the original publication in this journal is cited, in accordance with accepted academic practice. No use, distribution or reproduction is permitted which does not comply with these terms.
*Correspondence: Javier Alvarez, amFsdmFyZXpAaWJnbS51dmEuZXM=