- 1Jiangsu Province Key Laboratory of Anesthesiology, Xuzhou Medical University, Xuzhou, China
- 2Emergency Department, The Affiliated Hospital of Xuzhou Medical University, Xuzhou, China
- 3Department of Psychology, Florida Atlantic University, Boca Raton, FL, United States
- 4College of Basic Medical Sciences, Anhui Medical University, Hefei, China
Visceral hypersensitivity is a highly complex and subjective phenomenon associated with multiple levels of the nervous system and a wide range of neurotransmission. The dorsal horn (DH) in spinal cord relays the peripheral sensory information into the brain. Small conductance Ca2+-activated K+ (SK) channels regulate neuronal excitability and firing by allowing K+ to efflux in response to increase in the intracellular Ca2+ level. In this study, we examined the influence of SK2 channels in the spinal DH on the pathogenesis of visceral hypersensitivity induced by colorectal distension (CRD) in rats. Electrophysiological results showed that rats with visceral hypersensitivity presented a decrease in the SK channel-mediated afterhyperpolarization current (IAHP), and an increase in neuronal firing rates and c-Fos positive staining in the spinal DH. Western blot data revealed a decrease in the SK2 channel protein in the membrane fraction. Moreover, intrathecal administration of the SK2 channel activator 1-EBIO or CyPPA alleviated visceral hypersensitivity, reversed the decrease in IAHP and the increase in neuronal firing rates in spinal DH in rats that experienced CRD. 1-EBIO or CyPPA effect could be prevented by SK2 channel blocker apamin. CRD induced an increase in c-Fos protein expression in the spinal DH, which was prevented by 1-EBIO. Together, these data suggest that visceral hypersensitivity and pain is associated with a decrease in the number and function of membrane SK2 channels in the spinal DH. Pharmacological manipulation of SK2 channels may open a new avenue for the treatment of visceral hypersensitivity and pain.
Highlights:
- Neonatal colorectal distension induced visceral hypersensitivity in rats.
- Visceral hypersensitivity rats presented a decrease in afterhyperpolarization current (IAHP) and membrane SK2 channel protein in the spinal dorsal horn.
- Visceral hypersensitivity rats presented an increase in neuronal firing rate in the spinal dorsal horn.
- Intrathecal administration of SK2 channel activator 1-EBIO or CyPPA prevented visceral hypersensitivity and decrease in IAHP.
Introduction
Visceral hypersensitivity is a hallmark of irritable bowel syndrome and other gastrointestinal disorders that cause pain. The mechanism of visceral hypersensitivity and pain is still poorly understood due to the diverse nature of visceral hypersensitivity compounded by multiple factors such as sexual dimorphism, psychological and physical stresses, genetic and epigenetic traits, and the nature of predisposed disease (Chen et al., 2015; Elsenbruch and Enck, 2016). We recently demonstrated that early life stresses, for example, neonatal colorectal distension (CRD), maternal separation, could induce visceral hypersensitivity in rats (Wang et al., 2016; Zhang et al., 2016a,b). Visceral information is collected by thoracolumbar and sacral spinal visceral afferents, and coded by viscerosomatic neurons in spinal cord, thalamus, limbic system, and cortex (Larauche et al., 2012; Moloney et al., 2015). The dorsal horn (DH) in spinal cord is a major component of the nociceptive system, and the alteration in spinal neuronal activity may modulate visceral hypersensitivity and pain (Kawamata and Omote, 1996; Wang et al., 2014; Fu et al., 2016; Medrano et al., 2016).
Peripheral visceral afferents project into the spinal lamina I, II (outer layer), V, and X before reaching the intermediolateral nucleus where the preganglionic neurons are localized (Sugiura et al., 1989). Visceral hypersensitivity can be induced by the sensitization of primary sensory neurons innervating the visceral organs, hyperexcitability of spinal ascending neurons receiving visceral afferents, and/or dysregulation of descending pathways that modulate spinal nociceptive transmission (Sengupta, 2009). Previous studies revealed that nociceptive neurons in the spinal DH presented higher excitability and exaggerated sensory reflex to the visceral stimuli in subjects with visceral hypersensitivity (Lu et al., 2007; Hipolito et al., 2015; Wu et al., 2016). Neuronal excitability is modulated by membrane ion channels, for example, small conductance Ca2+-activated K+ (SK) channels (Adelman et al., 2012).
The SK channels (SK1, SK2, and SK3 channels) distribute extensively in the brain (Kohler et al., 1996; Deignan et al., 2012), spinal dorsal root ganglia (DRG) and DH neurons (Mongan et al., 2005). SK2 channels were localized almost exclusively in the superficial laminae of the spinal DH, a region in which many sensory afferents terminate (Sailer et al., 2004; Mongan et al., 2005). SK2 channels regulate neuronal excitability and firing by allowing K+ to efflux in response to increases in the intracellular Ca2+ level (Adelman et al., 2012). SK channels in the spinal cord are involved in nociception. For example, 1-EBIO, a SK channel opener, inhibits neuronal activity in the dorsal root (Bahia et al., 2005). The SK2/SK3 channel blocker UCL 1,848 increases DH neuronal responses to naturally evoked nociceptive stimuli, and intraplantar injection of the selective SK channel blocker, apamin, induces mechanical allodynia and heat hyperalgesia in naive rats (Pagadala et al., 2013). SK channels in the brain modulate pathologic pain (Thompson et al., 2015), however, the role of spinal SK2 channels on the etiology of visceral hypersensitivity and pain remains elusive. In this study, we adopted CRD to develop visceral hypersensitivity and examined the mechanism of SK2 channels on visceral hypersensitivity and pain by examining SK2 channel-mediated current change and SK2 channel protein expression. Our data reveal that visceral hypersensitivity is associated with a decrease in SK2 channel activity and protein expression in spinal DH neurons.
Materials and Methods
Animals
Sprague-Dawley (SD) rats (220–250 g) were purchased from the Experimental Animal Center of Xuzhou Medical University (Xuzhou, China). One male rat and two female rats were mated to produce offspring. After separation on postnatal day 25, male juvenile rats were grouped into 4 per cage. All rats were housed in a colony with a standard 12–12 h light–dark cycle (lights on at 07:00 AM), constant temperature and humidity (22°C and 50%) with food and water ad libitum. Young rats were subsequently assigned to different groups when their body weight reached 200–250 g. All procedures were conducted in accordance with the NIH Guide for the Care and Use of Laboratory Animals (2011) and approved by the Institutional Animal Care and Use Committee at Xuzhou Medical University.
Reagents
Rabbit anti-SK2/KCa2.2 (APC-028) polyclonal antibody was purchased from Alomone labs (Jerusalem, Israel). Rabbit anti-c-Fos mAb (CST-2250s), rabbit NeuN (D4G4O) XP mAb (#24307), and mouse glial fibrillary acidic protein (GFAP) mAb (#3670) were purchased from Cell Signaling Technology (Boston, MA, United States). Mouse anti-β-actin mAb (sc-47778) was purchased from Santa Cruz Biotechnology (Santa Cruz, CA, United States). Goat anti-Iba-1 (ab5076) polyclonal antibody was purchased from Abcam (Cambridge, United Kingdom). Mouse anti-GAPDH (AC001) mAb was purchased from ABclonal Biotechnology (Woburn, MA, United States). Rabbit anti-Tubulin β (AP0064) was purchased from Bioworld Technology (Minneapolis, MN, United States). Alexa Fluor 488 donkey anti-rabbit IgG (H + L) antibody and Alexa Fluor 594 donkey anti-mouse IgG (H + L) were purchased from Life Technologies (Carlsbad, CA, United States). Alkaline phosphatase goat anti-rabbit IgG (ZB-2308), alkaline phosphatase horse anti-mouse IgG (ZB-2310), HRP-labeled goat Anti-Rabbit IgG (H + L) (A0208), HRP-labeled goat anti-mouse IgG (H + L) (A0216), BCA protein assay kit (P0012), sodium dodecyl sulfate (SDS)-polyacrylamide gel electrophoresis (PAGE) sample loading buffer (P0015), BCIP/NBT alkaline phosphatase color development kit (C3206) and BeyoECL Moon kit (P0018FFT) were purchased from Beyotime Institute of Biotechnology (Shanghai, China). Syn-PERTM Synaptic Protein Extraction Reagent (#87793) was purchased from Thermo Fisher Scientific (Waltham, MA, United States).
Development and Assessment of Visceral Hypersensitivity
The paradigm of development and assessment of visceral hypersensitivity has been well-established (Zhang et al., 2016a). In brief, rats received CRD on postnatal days 8, 10, and 12 by inserting an angioplasty balloon (length, 20.0 mm; diameter, 3.0 mm) into the upper rectum and descending colon (Figure 1A). The balloon was distended with 0.3 mL water at a pressure of 60 mmHg for 1 min before deflation and withdrawal. The distention was repeated twice with at least 30 min interval. The sham group rats were not given water inflation to distension balloon. Adult CRD was given to rats at 60 mmHg distention for 60 s 10 times with a 15 s interval. Visceral hypersensitivity was determined by visceral pain threshold in response to adult CRD and external oblique muscle discharge as described previously (Zhang et al., 2016a).
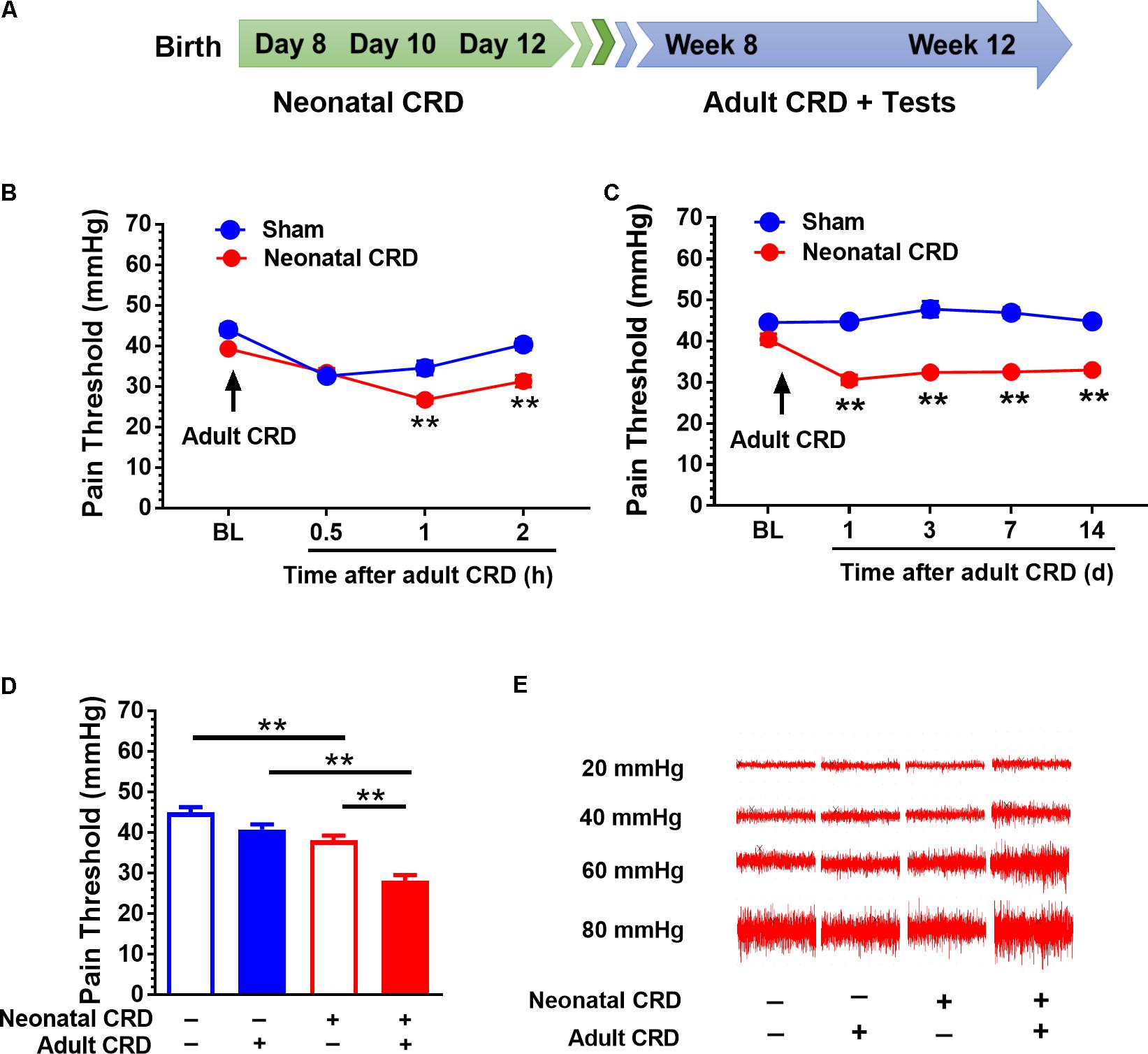
FIGURE 1. Adult CRD precipitates the visceral hypersensitivity in rats that experienced neonatal CRD. (A) Timeline for CRD and behavioral test in rats. Neonatal CRD were conducted on postnatal days 8, 10, and 12. Adult CRD and behavioral test occurred between weeks 8 and 12. (B) The pain thresholds of rats that experienced neonatal CRD decreased significantly at 1 and 2 h after re-exposed to adult CRD compared with that in sham group (p < 0.01, n = 6 per group). (C) The pain thresholds of rats that experienced neonatal CRD decreased consistently after re-exposed to adult CRD and lasted for 14 days compared with that in sham group (p < 0.01, n = 6 per group). (D) Neonatal CRD rats presented a significant decrease in pain threshold compared with naïve rats or rats that experienced either neonatal or adult CRD (p < 0.01; n = 8 per group). (E) Representative EMG recordings at 20, 40, 60, and 80 mmHg CRD in rats at different condition. Data are expressed as mean ± SEM. ∗∗p < 0.01.
Assessment of Pain Threshold
Rats were placed in transparent plastic boxes (20 cm × 10 cm × 18 cm) on an elevated plexiglas platform and allowed to habituate to the recording environments for 15–30 min. Rubber balloon in fixed size were inserted into the upper rectum and descending colon before applying different expansion pressures to observe the response of abdominal wall. The pain threshold was defined by the stimulus intensity that evoked a visible contraction of abdominal wall. CRD was applied starting at 10 mmHg. For accuracy, each distension was repeated for three times to calculate an average.
Intrathecal Catheter Implantation
Intrathecal catheter implantation was similar to that described by Yaksh and Rudy (1976) with some modifications. In brief, rats were anesthetized with 2% pentobarbital sodium (40 mg/kg, i.p.). A 2–3 cm longitudinal incision was made through the skin and muscle on the back of rats to fully expose the L4–5 vertebrae. PE-10 catheter (OD 0.28 mm, OD 0.61 mm) containing 0.9% sterile saline was inserted between L4 and L5. Sterile saline (10 μL) were injected into subarachnoid gap slowly without resistance and cerebrospinal fluid was out from the crevasse, which showed that the catheter was unobstructed. The catheter was secured to the surrounding tissue and the outside end of the catheter was sealed. The surgery wounds were dressed with antibiotic powder and rats were allowed to recover for 5 days. 1-EBIO (300 μg), CyPPA (140 ng), and apamin (5 ng) drugs were given intrathecally in a volume of 10 μL using a microsyringe infusion pump (KDS Scientific, Boston, MA, United States) loaded with a 10 μL Hamilton microsyringe.
Electrophysiological Recording
Transverse sections of the lower lumbar and upper sacral segment (L4–S4) were taken at 250–300 μm in ice-cold slicing solution (mM): 80 NaCl, 3.5 KCl, 4.5 MgSO4, 0.5 CaCl2, 1.25 NaH2PO4, 90 sucrose, 25 NaHCO3, and 10 glucose. Artificial cerebral spinal fluid (ACSF) (mM) consisted of 126 NaCl, 2.5 KCl, 1.2 NaH2PO4, 1.2 MgSO4, 26 NaHCO3, 10 glucose, and 2.4 CaCl2. All solutions were saturated with 95% O2 and 5% CO2. The slices were incubated in cutting solution at 32°C for 15 min, then transferred to the ACSF at room temperature for at least 1 h prior to a submersion recording chamber. Whole cell patch-clamp recordings were conducted in DH laminae I–II neurons with a glass pipette filled with an internal solution (mM): 10 phosphocreatine-Tris, 10 HEPES, 10 EGTA, 2 ATP-Mg, 0.5 GTP-Na, 115 K gluconate, 20 KCl, 1.5 MgCl2; pH was adjusted to 7.2 with KOH (285 mOsm). The resistance was 4–8 MΩ. Whole-cell recoding: cells were held in the current-clamp mode at −60 mV and action potential firings in response to the injection of depolarizing current pulses were recorded with a patch-clamp amplifier (MultiClamp 700A, Axon Instruments, Union City, CA, United States). To measure SK currents, DH neurons were held in voltage-clamp at a holding potential of −60 mV and 100 ms depolarizing pulse to 60 mV, which was used to evoke an outward current. Cell-attached recordings were performed in voltage clamp with the current held around 0 pA. Data acquisition and analysis were performed using Clampex and Clampfit 10 (Axon Instruments, San Jose, CA, United States).
Immunofluorescent Staining
Rats were deeply anesthetized and transcardially perfused with 0.9% saline (100 mL/100 g), followed by 4% polyformaldehyde in phosphate buffer (100 mL/100 g). Spinal cord was harvested and fixed again in 4% polyformaldehyde overnight and equilibrated in 30% sucrose solution before being sliced at 30 μm thickness with a cryostat (Leica CM1800; Heidelberg, Germany). Selected slices were washed with PBS three times for 10 min and blocked with 10% donkey serum at room temperature for 2 h before being incubated with anti-c-Fos antibody (1:6,000), anti-NeuN antibody (1:1,000), anti-GFAP antibody (1:1,000), anti-Iba1 (1:1,000) and anti-SK2 (1:150) at 4°C for 24 h, then was incubated with Alexa Fluor 488 (1:200) or Alexa Fluor 594 (1:200) at room temperature for 2 h. DH regions were visualized with a confocal laser microscope (FV1000, Olympus, Tokyo, Japan).
Western Blot Analysis
Rats were sacrificed approximately 2 h after adult CRD. The lower lumbar and upper sacral segment (L4–S4) was extracted and lysed with Syn-PERTM Synaptic Protein Extraction Reagent (1 mL/100 mg) containing PMSF. Cell lysis was centrifuged at 8.000 rpm for 10 min at 4°C. The supernatant (whole cell lysis) was collected and further centrifuged at 15,000 rpm for 20 min at 4°C. The supernatant (cytoplasmic fraction) were collected. RIPA lysis buffer (500 μL/200 mg) was added to the pellet (membrane fraction). The sample protein concentration was determined with BCA. Equal amounts of protein were separated by SDS-PAGE gels and transferred to the PVDF membrane. After blockade with 5% non-fat milk for 2 h at room temperature, the PVDF membrane was incubated with anti-SK2 (1:200) anti-GAPDH (1:1,000) or anti-Tubulin β (1:3,000) primary antibody at 4°C overnight. After TBST washing, the PVDF membrane was incubated with AP-conjugated secondary antibody (1:1,000) or HRP-conjugated secondary antibody (1:1,000) for 2 h at room temperature. Protein bands were illuminated using the BCIP/NBT alkaline phosphatase color development kit or BeyoECL Moon kit and captured by using Image ProPlus image analysis system (Media Cybernetics, Inc., Rockville, MD, United States).
Statistical Analysis
Data are expressed as mean ± SEM. One-way analysis of variance (ANOVA) and repeated measures ANOVA were used. If significance was found, post hoc Bonferroni or S–N–K multiple comparisons were used. Independent samples Student’s t-test was also used. All statistical tests were conducted using the SPSS 19.0 (IBM, Armonk, NY, United States) software package. p < 0.05 was considered statistically significant.
Results
Adult CRD Precipitates Visceral Hypersensitivity in Rats That Experienced Neonatal CRD
Rats underwent neonatal CRD on postnatal days 8, 10, and 12, and adult CRD in week 8 to 12 (Figure 1A). Pain threshold was recorded 0.5, 1, and 2 h after adult CRD. Neonatal CRD altered the pain threshold (F(1,10) = 12.39). Rats that experienced neonatal CRD presented lower pain threshold compared to sham rats at 1 h (t(10) = 4, p < 0.01) and 2 h (t(10) = 4.56, p < 0.01) after adult CRD (Figure 1B). Pain threshold on days 1, 3, 7, and 14 after adult CRD revealed a significant change (F(1,10) = 236.19, p < 0.01). Rats that experienced neonatal CRD presented lower pain threshold compared to sham rats on day 1 (t(10) = 10.72, p < 0.01), day 3 (t(10) = 7.6, p < 0.01), day 7 (t(10) = 10.29, p < 0.01), and day 14 (t(10) = 12.46, p < 0.01) after adult CRD (Figure 1C). Rats that experienced both neonatal and adult CRD presented a significant decrease in pain threshold compared with naïve rats or rats that experienced either neonatal or adult CRD (F(3,28) = 30.87, p < 0.01; Figure 1D). Figure 1E showed the respective EMG recording to various distension pressure in rats that experienced neonatal and/or adult CRD.
SK2 Channel Protein in Membrane Fraction and IAHP Are Reduced in Rats That Experienced Neonatal CRD
Western blot data showed that the spinal SK2 channel protein in membrane fraction was decreased in rats that experienced neonatal with or without adult CRD compared with that in rats only received adult CRD (F(3,8) = 13.67, p < 0.01; Figure 2A). There was no difference in total spinal SK2 protein in rats that experienced either neonatal or adult CRD (F(2,18) = 1.18, p = 0.33; Figure 2B).
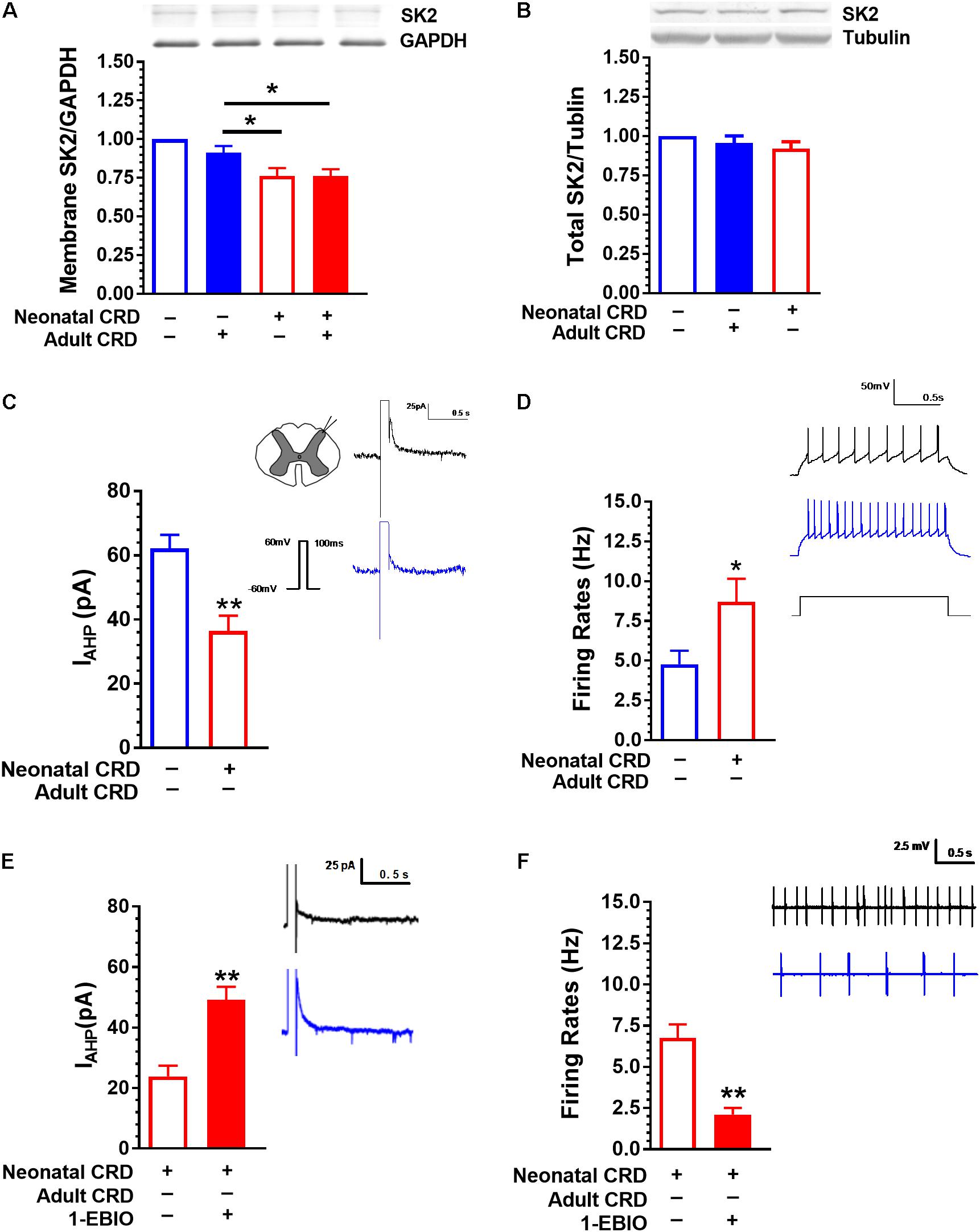
FIGURE 2. SK2 Channel protein in membrane fraction and IAHP are reduced in rats that experienced neonatal CRD. (A) Spinal SK2 channel in membrane fraction presented a significant decrease in rats that experienced neonatal and adult CRD compared with that in rats received adult CRD alone (p < 0.05, n = 3 per group). (B) There was no difference in total spinal SK2 channel protein in rats that experienced neonatal or adult CRD as compared to sham rats (p > 0.05, n = 7 per group). (C) The average peak amplitude of IAHP in neonatal CRD rats significantly reduced compared with that in naïve rats (p < 0.01, n = 16–21 neurons, six rats per group). (D) The spontaneous neuronal firing rate in spinal DH increased significantly in neonatal CRD rats compared with that in naïve rats (p < 0.05, n = 9–10 neurons, five rats per group). (E) 1-EBIO can increase the amplitude of IAHP in neonatal CRD rats (p < 0.01, n = 10 neurons per group). (F) 1-EBIO can decrease the neuronal firing rate in the spinal DH in rats that experienced neonatal CRD (p < 0.01, n = 10 neurons per group). Data are expressed as mean ± SEM. ∗p < 0.05, ∗∗p < 0.01.
Rats that experienced neonatal CRD presented low IAHP compared to rats without neonatal CRD. The average peak amplitude of IAHP was 62.2 ± 19 pA and 36.43 ± 18.9 pA in naïve and neonatal CRD rats, respectively (t(35) = 4.09, p < 0.01; Figure 2C). Consistently, rats that experienced neonatal CRD presented an increase in spontaneous neuronal firing rates compared to the naïve rats (4.77 ± 0.85 vs. 8.70 ± 1.45; t(17) = 2.27, p = 0.04; Figure 2D). The changes could be reversed by SK channel activator 1-EBIO (100 μM). Incubation of the spinal slice of rats that experienced neonatal CRD. 1-EBIO elevated IAHP (23.90 ± 3.51 vs. 49.11 ± 4.37; t(18) = −4.53, p < 0.01; Figure 2E) and decreased the neuron firing rate (6.76 ± 0.82 vs. 2.104 ± 0.40; t(18) = 5.11, p < 0.01; Figure 2F).
SK Channel Activator 1-EBIO Prevents the Decrease in Pain Threshold and Increase in c-Fos Protein Expression in Rats That Experienced Neonatal and Adult CRD
Rats received intrathecal administration of 1-EBIO (300 μg/10 μL) 20 min before adult CRD, and behavioral tests were conducted 2 h after adult CRD. 1-EBIO prevented the decrease in pain threshold in rats that experienced neonatal and adult CRD (p < 0.01; Figure 3A). The increase in pain threshold induced by 1-EBIO could be further blocked by SK2 channel blocker apamin (5 ng/10 μL) (p < 0.01; Figure 3B). Figure 3D shows the c-Fos immunostaining in the spinal DH under different conditions (Figure 3D). Quantitative results showed 1-EBIO could prevent neonatal and adult CRD induced increase in c-Fos overexpression (p < 0.01; Figure 3C).
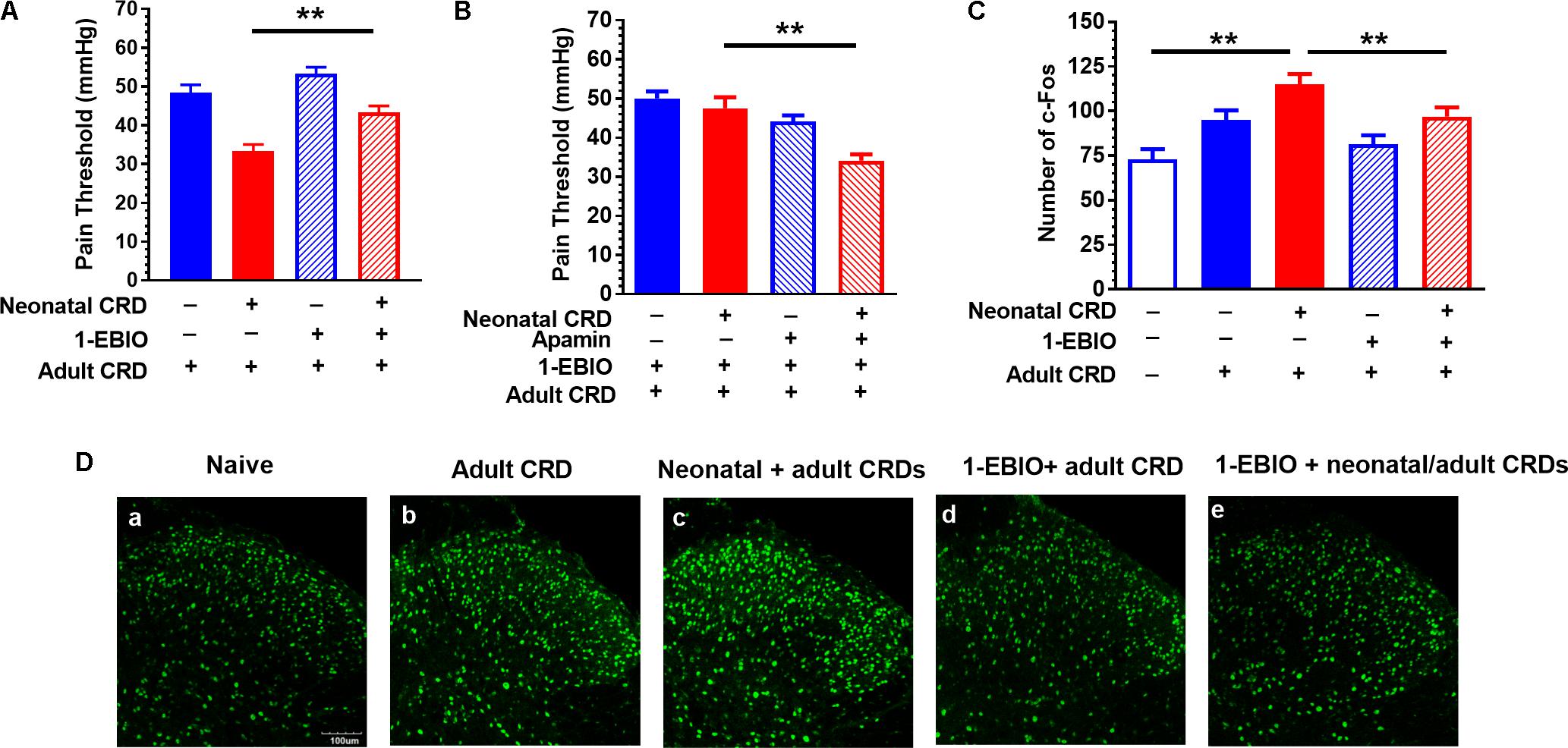
FIGURE 3. SK channel activator 1-EBIO prevents the decrease in pain threshold and increase in c-Fos protein expression in rats that experienced neonatal and adult CRD. (A) 1-EBIO prevented the decrease in pain threshold in rats that experienced neonatal and adult CRD (p < 0.01, n = 6 per group). (B) Apamin blocked the effect of 1-EBIO (n = 6). (C) 1-EBIO can prevent neonatal and adult CRD induced c-Fos overexpression (p < 0.01, n = 6 per group). (D) c-Fos immunostaining. Scale bar = 100 μm. Data are expressed as mean ± SEM. ∗∗p < 0.01.
SK2 Channel Activator CyPPA and Blocker Apamin Affect IAHP and Pain Threshold
Similar to 1-EBIO, CyPPA (20 μM) increased IAHP (32.01 ± 3.42 vs. 49.311 ± 4.63; t(18) = −3.01, p < 0.01; Figure 4A) and suppressed neuronal firing rate (5.49 ± 0.51 vs. 2.09 ± 0.20; t(26) = 6.19, p < 0.01; Figure 4B). Intrathecal CyPPA (140 ng/10 μL) prevented CRD induced decrease in pain threshold, which could be blocked by SK2 channel blocker apamin (5 ng/10 μL) (p < 0.05; Figure 4C). Apamin (100 nM) decreased IAHP current compared with that in control group (40.00 ± 7.54 vs. 7.28 ± 2.15; t(10) = 4.18, p < 0.01; Figure 4D). Figure 4E showed the SK2 channel protein in membrane fraction under different conditions. Intrathecal administration of apamin facilitated the decrease in pain threshold caused by CRD (p < 0.05; Figure 4F).
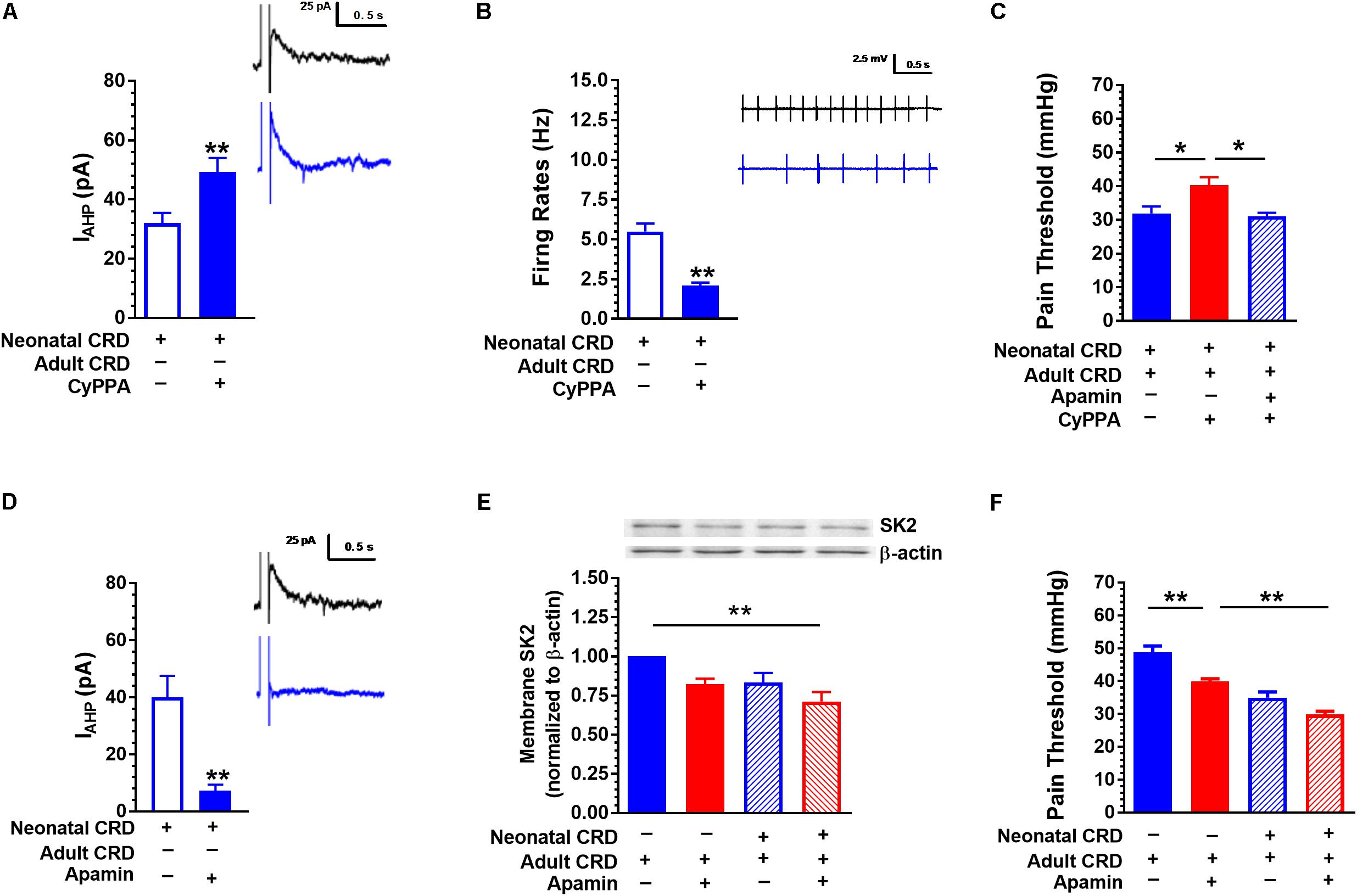
FIGURE 4. SK2 channel activator CyPPA and blocker apamin affect IAHP and pain threshold. (A) CyPPA increased the amplitude of IAHP in rats that experienced neonatal CRD (p < 0.01, n = 10 neurons per group). (B) CyPPA decreased the neuronal firing rate in the spinal DH in rats that experienced neonatal CRD (p < 0.01, n = 14 neurons per group). (C) CyPPA prevented the decrease in pain threshold in rats that experienced neonatal and adult CRD, which could be prevented by apamin (p < 0.01, n = 5 per group). (D) Apamin decreased the amplitude of IAHP in rats that experienced neonatal CRD (p < 0.01, n = 6 neurons per group). (E) Apamin induced a decrease in membrane SK2 channel protein level (p < 0.01, n = 6 neurons per group). (F) Apamin promoted a decrease in pain threshold in rats that experienced neonatal or adult CRD (p < 0.01, n = 5 per group). Data are expressed as mean ± SEM. ∗p < 0.05; ∗∗p < 0.01.
Discussion
In this study, we explored the role of spinal SK2 channels in visceral hypersensitivity in a rat model developed by CRD. Rats with visceral hypersensitivity presented a significant decrease in membrane SK2 channel protein and IAHP, and an increase in neuronal firing rate in the spinal DH. SK2 channel activators 1-EBIO and CyPPA could reverse the decrease in IAHP and neuronal firing rate, and prevent the visceral hypersensitivity in CRD rats. SK2 channel blocker apamin reduced IAHP and pain threshold in CRD rats. Our results for the first time reported the involvement of spinal SK2 channels in the visceral hypersensitivity, and pharmacological manipulation of SK2 channels may open a new avenue for the treatment of chronic visceral hypersensitivity and pain.
In line with previous reports (Zhang et al., 2016a), we validated that neonatal CRD can induce visceral hypersensitivity and facilitate pain precipitation. Early life is a critical period for the nervous system development. The adverse events in early life can disrupt neuronal development, leading to various endocrine, metabolic, autoimmune, and psychiatric disorders (Amath et al., 2012), as well as increase the vulnerability to stressors (Luthar and Zigler, 1991; Gunnar and Quevedo, 2008). We previously reported that microglial activation in the hippocampus and paraventricular nucleus participates in the etiology of visceral hypersensitivity to neonatal CRD (Zhang et al., 2016a). The spinal DH converges peripheral sensory information and relays to the brain. Abnormalities in the structure and function of DH are observed in neuropathic pain, cancer pain, and other pain disorders (Kawamata and Omote, 1996; Wang et al., 2014; Fu et al., 2016; Medrano et al., 2016). Nociceptive neurons in the spinal DH presented higher excitability and exaggerated sensory reflex to the visceral stimuli in subjects with visceral hypersensitivity (Lu et al., 2007; Hipolito et al., 2015; Wu et al., 2016). Exploration of the molecular mechanism in the spinal DH responsible for visceral hypersensitivity is critical for translational research and drug development.
Our data revealed that membrane SK2 channel protein in spinal DH was decreased in rats that experienced CRD. Consistently, the SK2 channel-mediated IAHP was decreased. SK2 channels regulate synaptic transmission, neuronal excitability and firing by allowing K+ to efflux in response to increase in the intracellular Ca2+ level. The decrease in membrane SK2 channel will facilitate the intrinsic neuronal excitability and synaptic transmission, which may induce visceral hypersensitivity and pain. Moreover, SK2 channel activators 1-EBIO and CyPPA were found to prevent the precipitation of visceral hypersensitivity and pain, which further supports the hypothesis that SK2 channels participate in the pathogenesis of visceral hypersensitivity and pain. c-Fos is a proto-oncogene that is expressed within some neurons following depolarization. c-Fos expression might be used as a marker for neuronal activity throughout the neuraxis following peripheral stimulation. We found an increase in c-Fos positive staining in the spinal DH, which was in line with the increase in spinal neuronal firing in rats that experienced visceral hypersensitivity. 1-EBIO prevented the increase in c-Fos protein expression in spinal DH in rats that experienced CRD further support that activation of SK2 channels presents an inhibitory effect.
The mechanism underlying the decrease in membrane SK2 channel protein remains unknown. Activation of cyclic AMP-dependent protein kinase (PKA) with forskolin causes a dramatic decrease in surface localization of the SK2 channel subunit expressed in COS7 cells due to direct phosphorylation of the SK2 channel intracellular domain (Ren et al., 2006; Abiraman et al., 2016). The internalization of synaptic SK channels by PKA enhances excitatory synaptic transmission and plasticity in the Amygdala (Faber et al., 2008). Blocking PKA or PKA target domain in SK2 channels blocks the internalization of SK2 channels after long-term potentiation (LTP) induction at Schaffer collateral-CA1 synapses (Lin et al., 2008). The cAMP-PKA pathway augments presynaptic neurotransmitter synthesis and vesicular transport, maintains DRG neuronal hyperexcitability by phosphorylating key transcription factors and synaptic vesicle proteins (King et al., 2005; Tumati et al., 2011; Zhu et al., 2014; Shao et al., 2016). These findings suggest that CRD causes the internalization of SK2 channels by increasing cAMP-PKA activity in spinal DH.
SK channels are specifically involved in the medium afterhyperpolarization potential (mAHP) following single or multiple action potentials in neurons, affecting the intrinsic excitability of neurons, synaptic transmission and pain sensation (Bahia et al., 2005; Pedarzani et al., 2005; Lu et al., 2009). In an animal model of nociception, the AHP is down-regulated in DRG cells and reticulospinal neurons after nerve injury (McClellan et al., 2008). In this study, we found that SK2 channel protein in membrane fraction was decreased, which may result in the reduction in the amplitude of IAHP contributing to an increase in neuronal excitability, e.g., increase in neuronal firing rate.
Besides SK2 channels, SK3 channel may also participate the development of visceral pain. Similar to SK2 channels, high level of SK3 channel positive staining are expressed in the DH of spinal cord. Particularly, dense fiber staining was observed in the laminae I and II (Sailer et al., 2004; Hipólito et al., 2015). Our data confirm this finding (Supplementary Figure 2). Moreover, neonatal and/or adult CRD did not alter the SK3 channel protein expression in the spinal cord (Supplementary Figure 3). Considering the limitation of SK3 channel selective activator/blocker, the role of SK3 channel in the etiology of visceral pain need further exploration.
Neuroinflammation participates in the development of visceral hypersensitivity and pain. We previously reported microglial activation and increase in proinflammatory cytokines facilitate the development of visceral hypersensitivity in rats that experienced CRD (Zhang et al., 2016a). KCNN1-3 genes are expressed in microglia (Schlichter et al., 2010). SK channels regulates microglial function and neuroninflammation (Armstrong et al., 2005; Schlichter et al., 2010; Dolga and Culmsee, 2012). For example, the SK3 channel mRNA is expressed in microglia in the rat striatum (Schlichter et al., 2010), substantia nigra pars compacta (Armstrong et al., 2005) of adult rat and mouse brains. SK3 channel blockade decreased the activity of p38 mitogen-activated protein kinase (MAPK) in microglia cells and attenuated tyrosine-nitrated proteins in the neurons exposed to activated microglia (Schlichter et al., 2010). We found that SK2 channels are co-labeled with GAFP and Iba-1 at the layer I and II of the spinal DH, supporting the involvement of SK2 channels in the modulation of neuroinflammation (Supplementary Figure 1).
In this study, we explored the participation of SK2 channels in spinal DH on visceral hypersensitivity in rats. Our data reveals that rats with visceral hypersensitivity present a decrease in the number and function of membrane SK2 channels in the spinal DH. Pharmacological activation of SK2 channels can prevent the precipitation of visceral hypersensitivity, and blockade of SK2 channel can facilitate the visceral hypersensitivity. Pharmacological manipulation of SK2 channels may open a new avenue for the treatment of visceral hypersensitivity and pain.
Author Contributions
Y-MZ, GZ, and RS conceived and planned the experiments. J-SZ, YS, LD, S-TH, and RH carried out the experiments. All authors contributed to data analysis and manuscript preparation.
Funding
This project was supported by grants from the National Natural Science Foundation of China (Grant Nos. 81771203, 81772065, and 81471161) and the Natural Science Foundation of Jiangsu Province (Grant No. BK20161171).
Conflict of Interest Statement
The authors declare that the research was conducted in the absence of any commercial or financial relationships that could be construed as a potential conflict of interest.
Supplementary Material
The Supplementary Material for this article can be found online at: https://www.frontiersin.org/articles/10.3389/fphar.2018.00840/full#supplementary-material
References
Abiraman, K., Sah, M., Walikonis, R. S., Lykotrafitis, G., and Tzingounis, A. V. (2016). Tonic PKA activity regulates SK channel nanoclustering and somatodendritic distribution. J. Mol. Biol. 428, 2521–2537. doi: 10.1016/j.jmb.2016.04.014
Adelman, J. P., Maylie, J., and Sah, P. (2012). Small-conductance Ca2+-activated K+ channels: form and function. Annu. Rev. Physiol. 74, 245–269. doi: 10.1146/annurev-physiol-020911-153336
Amath, A., Foster, J. A., and Sidor, M. M. (2012). Developmental alterations in CNS stress-related gene expression following postnatal immune activation. Neuroscience 220, 90–99. doi: 10.1016/j.neuroscience.2012.06.037
Armstrong, W. E., Rubrum, A., Teruyama, R., Bond, C. T., and Adelman, J. P. (2005). Immunocytochemical localization of small-conductance, calcium-dependent potassium channels in astrocytes of the rat supraoptic nucleus. J. Comp. Neurol. 491, 175–185. doi: 10.1002/cne.20679
Bahia, P. K., Suzuki, R., Benton, D. C., Jowett, A. J., Chen, M. X., Trezise, D. J., et al. (2005). A functional role for small-conductance calcium-activated potassium channels in sensory pathways including nociceptive processes. J. Neurosci. 25, 3489–3498. doi: 10.1523/JNEUROSCI.0597-05.2005
Chen, Z. Y., Zhang, X. W., Yu, L., Hua, R., Zhao, X. P., Qin, X., et al. (2015). Spinal toll-like receptor 4-mediated signalling pathway contributes to visceral hypersensitivity induced by neonatal colonic irritation in rats. Eur. J. Pain 19, 176–186. doi: 10.1002/ejp.534
Deignan, J., Lujan, R., Bond, C., Riegel, A., Watanabe, M., Williams, J. T., et al. (2012). SK2 and SK3 expression differentially affect firing frequency and precision in dopamine neurons. Neuroscience 217, 67–76. doi: 10.1016/j.neuroscience.2012.04.053
Dolga, A. M., and Culmsee, C. (2012). Protective roles for potassium SK/KCa2 channels in microglia and neurons. Front. Pharmacol. 3:196. doi: 10.3389/fphar.2012.00196
Elsenbruch, S., and Enck, P. (2016). [Psychobiological mechanisms in the pathophysiology of chronic visceral pain]. Schmerz 30, 407–411. doi: 10.1007/s00482-016-0130-9
Faber, E. S., Delaney, A. J., Power, J. M., Sedlak, P. L., Crane, J. W., and Sah, P. (2008). Modulation of SK channel trafficking by beta adrenoceptors enhances excitatory synaptic transmission and plasticity in the amygdala. J. Neurosci. 28, 10803–10813. doi: 10.1523/JNEUROSCI.1796-08.2008
Fu, Q., Shi, D., Zhou, Y., Zheng, H., Xiang, H., Tian, X., et al. (2016). MHC-I promotes apoptosis of GABAergic interneurons in the spinal dorsal horn and contributes to cancer induced bone pain. Exp. Neurol. 286, 12–20. doi: 10.1016/j.expneurol.2016.09.002
Gunnar, M. R., and Quevedo, K. M. (2008). Early care experiences and HPA axis regulation in children: a mechanism for later trauma vulnerability. Prog. Brain Res. 167, 137–149. doi: 10.1016/S0079-6123(07)67010-1
Hipolito, L., Fakira, A. K., Cabanero, D., Blandon, R., Carlton, S. M., Moron, J. A., et al. (2015). In vivo activation of the SK channel in the spinal cord reduces the NMDA receptor antagonist dose needed to produce antinociception in an inflammatory pain model. Pain 156, 849–858. doi: 10.1097/j.pain.0000000000000124
Hipólito, L., Fakira, A. K., Cabañero, D., Blandón, R., Carlton, S. M., Morón, J. A., et al. (2015). In vivo activation of the SK channel in the spinal cord reduces the NMDA receptor antagonist dose needed to produce antinociception in an inflammatory pain model. Pain 156, 849–858. doi: 10.1097/j.pain.0000000000000124
Kawamata, M., and Omote, K. (1996). Involvement of increased excitatory amino acids and intracellular Ca2+ concentration in the spinal dorsal horn in an animal model of neuropathic pain. Pain 68, 85–96. doi: 10.1016/S0304-3959(96)03222-8
King, T., Ossipov, M. H., Vanderah, T. W., Porreca, F., and Lai, J. (2005). Is paradoxical pain induced by sustained opioid exposure an underlying mechanism of opioid antinociceptive tolerance? Neurosignals 14, 194–205.
Kohler, M., Hirschberg, B., Bond, C. T., Kinzie, J. M., Marrion, N. V., Maylie, J., et al. (1996). Small-conductance, calcium-activated potassium channels from mammalian brain. Science 273, 1709–1714. doi: 10.1126/science.273.5282.1709
Larauche, M., Mulak, A., and Tache, Y. (2012). Stress and visceral pain: from animal models to clinical therapies. Exp. Neurol. 233, 49–67. doi: 10.1016/j.expneurol.2011.04.020
Lin, M. T., Lujan, R., Watanabe, M., Adelman, J. P., and Maylie, J. (2008). SK2 channel plasticity contributes to LTP at Schaffer collateral-CA1 synapses. Nat. Neurosci. 11, 170–177. doi: 10.1038/nn2041
Lu, C. L., Hsieh, J. C., Tsaur, M. L., Huang, Y. H., Wang, P. S., Wu, L. L., et al. (2007). Estrogen rapidly modulates mustard oil-induced visceral hypersensitivity in conscious female rats: a role of CREB phosphorylation in spinal dorsal horn neurons. Am. J. Physiol. Gastrointest. Liver Physiol. 292, G438–G446. doi: 10.1152/ajpgi.00210.2006
Lu, L., Timofeyeva, V., Li, N., Rafizadeha, S., Singapuria, A., Harrisa, T. R., et al. (2009). α-Actinin2 cytoskeletal protein is required for the functional membrane localization of a Ca2+-activated K+ channel. Proc. Natl. Acad. Sci. U.S.A. 106, 18402–18407. doi: 10.1073/pnas.0908207106
Luthar, S. S., and Zigler, E. (1991). Vulnerability and competence: a review of research on resilience in childhood. Am. J. Orthopsychiatry 61, 6–22. doi: 10.1037/h0079218
McClellan, A. D., Kovalenko, M. O., Benes, J. A., and Schulz, D. J. (2008). Spinal cord injury induces changes in electrophysiological properties and ion channel expression of reticulospinal neurons in larval lamprey. J. Neurosci. 28, 650–659. doi: 10.1523/JNEUROSCI.3840-07.2008
Medrano, M. C., Dhanasobhon, D., Yalcin, I., Schlichter, R., and Cordero-Erausquin, M. (2016). Loss of inhibitory tone on spinal cord dorsal horn spontaneously and nonspontaneously active neurons in a mouse model of neuropathic pain. Pain 157, 1432–1442. doi: 10.1097/j.pain.0000000000000538
Moloney, R. D., O’Mahony, S. M., Dinan, T. G., and Cryan, J. F. (2015). Stress-induced visceral pain: toward animal models of irritable-bowel syndrome and associated comorbidities. Front. Psychiatry 6:15. doi: 10.3389/fpsyt.2015.00015
Mongan, L. C., Hill, M. J., Chen, M. X., Tate, S. N., Collins, S. D., Buckby, L., et al. (2005). The distribution of small and intermediate conductance calcium-activated potassium channels in the rat sensory nervous system. Neuroscience 131, 161–175. doi: 10.1016/j.neuroscience.2004.09.062
Pagadala, P., Park, C. K., Bang, S., Xu, Z. Z., Xie, R. G., Liu, T., et al. (2013). Loss of NR1 subunit of NMDARs in primary sensory neurons leads to hyperexcitability and pain hypersensitivity: involvement of Ca(2+)-activated small conductance potassium channels. J. Neurosci. 33, 13425–13430. doi: 10.1523/JNEUROSCI.0454-13.2013
Pedarzani, P., McCutcheon, J. E., Rogge, G., Jensen, B. S., Christophersen, P., Hougaard, C., et al. (2005). Specific enhancement of SK channel activity selectively potentiates the afterhyperpolarizing current I(AHP) and modulates the firing properties of hippocampal pyramidal neurons. J. Biol. Chem. 280, 41404–41411. doi: 10.1074/jbc.M509610200
Ren, Y., Barnwell, L. F., Alexander, J. C., Lubin, F. D., Adelman, J. P., Pfaffinger, P. J., et al. (2006). Regulation of surface localization of the small conductance Ca2+-activated potassium channel. J. Biol. Chem. 281, 11769–11779. doi: 10.1074/jbc.M513125200
Sailer, C. A., Kaufmann, W. A., Marksteiner, J., and Knaus, H. G. (2004). Comparative immunohistochemical distribution of three small-conductance Ca2+-activated potassium channel subunits. Mol. Cell. Neurosci. 26, 458–469. doi: 10.1016/j.mcn.2004.03.002
Schlichter, L. C., Kaushal, V., Moxon-Emre, I., Sivagnanam, V., and Vincent, C. (2010). The Ca2+ activated SK3 channel is expressed in microglia in the rat striatum and contributes to microglia-mediated neurotoxicity in vitro. J. Neuroinflammation 7:4. doi: 10.1186/1742-2094-7-4
Sengupta, J. N. (2009). Visceral pain: the neurophysiological mechanism. Handb. Exp. Pharmacol. 194, 31–74. doi: 10.1007/978-3-540-79090-7_2
Shao, X. M., Sun, J., Jiang, Y. L., Liu, B. Y., Shen, Z., Fang, F., et al. (2016). Inhibition of the cAMP/PKA/CREB Pathway contributes to the analgesic effects of electroacupuncture in the anterior cingulate cortex in a rat pain memory model. Neural Plast. 2016:5320641. doi: 10.1155/2016/5320641
Sugiura, Y., Terui, N., and Hosoya, Y. (1989). Difference in distribution of central terminals between visceral and somatic unmyelinated (C) primary afferent fibers. J. Neurophysiol. 62, 834–840. doi: 10.1152/jn.1989.62.4.834
Thompson, J. M., Ji, G., and Neugebauer, V. (2015). Small-conductance calcium-activated potassium (SK) channels in the amygdala mediate pain-inhibiting effects of clinically available riluzole in a rat model of arthritis pain. Mol. Pain 11:51. doi: 10.1186/s12990-015-0055-9
Tumati, S., Roeske, W. R., Largent-Milnes, T. M., Vanderah, T. W., and Varga, E. V. (2011). Intrathecal PKA-selective siRNA treatment blocks sustained morphine-mediated pain sensitization and antinociceptive tolerance in rats. J. Neurosci. Methods 199, 62–68. doi: 10.1016/j.jneumeth.2011.04.036
Wang, H., Tao, X., Huang, S. T., Wu, L., Tang, H. L., Song, Y., et al. (2016). Chronic stress is associated with pain precipitation and elevation in DeltaFosb expression. Front. Pharmacol. 7:138. doi: 10.3389/fphar.2016.00138
Wang, X. T., Lian, X., Xu, Y. M., Suo, Z. W., Yang, X., and Hu, X. D. (2014). Alpha(2) noradrenergic receptor suppressed CaMKII signaling in spinal dorsal horn of mice with inflammatory pain. Eur. J. Pharmacol. 724, 16–23. doi: 10.1016/j.ejphar.2013.12.026
Wu, B., Xu, C., and Huang, H. H. (2016). [Expression profiles of brain-derived neurotrophic factor in the spinal dorsal horn of young rats with visceral hypersensitivity]. Zhongguo Dang Dai Er Ke Za Zhi 18, 277–281.
Yaksh, T. L., and Rudy, T. A. (1976). Chronic catheterization of the spinal subarachnoid space. Physiol. Behav. 17, 1031–1036. doi: 10.1016/0031-9384(76)90029-9
Zhang, G., Yu, L., Chen, Z. Y., Zhu, J. S., Hua, R., Qin, X., et al. (2016a). Activation of corticotropin-releasing factor neurons and microglia in paraventricular nucleus precipitates visceral hypersensitivity induced by colorectal distension in rats. Brain Behav. Immun. 55, 93–104. doi: 10.1016/j.bbi.2015.12.022
Zhang, G., Zhao, B. X., Hua, R., Kang, J., Shao, B. M., Carbonaro, T. M., et al. (2016b). Hippocampal microglial activation and glucocorticoid receptor down-regulation precipitate visceral hypersensitivity induced by colorectal distension in rats. Neuropharmacology 102, 295–303. doi: 10.1016/j.neuropharm.2015.11.028
Keywords: small-conductance Ca2+-activated K+ channel 2, visceral hypersensitivity, colorectal distension, spinal dorsal horn, rats
Citation: Song Y, Zhu J-S, Hua R, Du L, Huang S-T, Stackman RW Jr., Zhang G and Zhang Y-M (2018) Small-Conductance Ca2+-Activated K+ Channel 2 in the Dorsal Horn of Spinal Cord Participates in Visceral Hypersensitivity in Rats. Front. Pharmacol. 9:840. doi: 10.3389/fphar.2018.00840
Received: 11 April 2018; Accepted: 11 July 2018;
Published: 03 August 2018.
Edited by:
Domenico Tricarico, Università degli Studi di Bari Aldo Moro, ItalyReviewed by:
Hai Minh Nguyen, University of California, Davis, United StatesLishuang Cao, Vertex Pharmaceuticals, United Kingdom
Copyright © 2018 Song, Zhu, Hua, Du, Huang, Stackman, Zhang and Zhang. This is an open-access article distributed under the terms of the Creative Commons Attribution License (CC BY). The use, distribution or reproduction in other forums is permitted, provided the original author(s) and the copyright owner(s) are credited and that the original publication in this journal is cited, in accordance with accepted academic practice. No use, distribution or reproduction is permitted which does not comply with these terms.
*Correspondence: Gongliang Zhang, Z29uZ2xpYW5nemhhbmdAaG90bWFpbC5jb20=; Yong-Mei Zhang, emhhbmd5bTcwMEAxNjMuY29t
†These authors have contributed equally to this work.