- 1Department of Anatomy, Faculty of Medicine, Bioscience and Nursing, MAHSA University, Jenjarom, Malaysia
- 2Department of Pharmacology, Faculty of Medicine, University of Malaya, Kuala Lumpur, Malaysia
- 3Centre for Craniofacial Diagnostics and Biosciences, Faculty of Dentistry, Universiti Kebangsaan Malaysia, Kuala Lumpur, Malaysia
- 4Department of Human Anatomy, Faculty of Medicine and Health Sciences, Universiti Putra Malaysia, Serdang, Malaysia
- 5Department of Basic Sciences and Oral Biology, Faculty of Dentistry, Universiti Sains Islam Malaysia, Kuala Lumpur, Malaysia
- 6Centre for Natural Product research and Drug Discovery (CENAR), Wellness Research Cluster, University of Malaya, Kuala Lumpur, Malaysia
Cardiovascular diseases (CVDs) such as angina, hypertension, myocardial ischemia, and heart failure are the leading causes of morbidity and mortality worldwide. One of the major transcription factors widely associated with CVDs is nuclear factor-kappa B (NFκB). NFκB activation initiates the canonical and non-conical pathways that promotes activation of transcription factors leading to inflammation, such as leukocyte adhesion molecules, cytokines, and chemokines. Flavonoids are bioactive polyphenolic compounds found abundantly in various fruits, vegetables, beverages (tea, coffee), nuts, and cereal products with cardiovascular protective properties. Flavonoids can be classified into six subgroups based on their chemical structures: flavanones, flavones, flavonols, flavan-3-ols, isoflavones, and anthocyanidins. As NFκB inhibitors, these flavonoids may modulate the expression of pro-inflammatory genes leading to the attenuation of the inflammatory responses underlying various cardiovascular pathology. This review presents an update on the anti-inflammatory actions of flavonoids via inhibition of NFκB mechanism supporting the therapeutic potential of these natural compounds in various CVDs.
Introduction
Cardiovascular diseases (CVDs) represent the major burden of mortality and morbidity in the developed countries (Benjamin et al., 2017). The most common pathogeneses of CVDs are inflammatory processes (Ruparelia et al., 2017). Various transcription factors are related to inflammatory responses in CVDs such as T-bet (Haybar et al., 2019), signal transducer and activator of transcription 3 (STAT3) (Kurdi et al., 2018), interferon regulatory factors (IRFs), activator protein 1 (AP-1) (Smale and Natoli, 2014), and transcription factor Bcl11b (Daher et al., 2019). However, the key player in the regulation of inflammation is the transcription factor nuclear factor kappa B (NFκB) (Van Der Heiden et al., 2010). The inhibition of NFκB pathway has been demonstrated to show beneficial effect in various CVDs including hypertension (Koeners et al., 2016), myocardial infraction (Zhao et al., 2017), and arteriosclerosis (Wang et al., 2016). These findings support that targeted inhibition of NFκB appears to be a promising strategy in reducing cardiovascular complications.
Flavonoids are plant polyphenolic compound derivatives from natural origin found in fruits, grains, vegetables, roots, bark, flowers, stems, tea, and wine (Zeinali et al., 2017). Non-plant natural products such as mushrooms and honey, plant extracts, plant juices, plant powders, and essential oils have shown to possess anti-inflammatory activities and many of these plant natural products have polyphenols as their major compound (Khalil and Sulaiman, 2010; Azab et al., 2016). However, the protective effects of flavonoid in CVDs via inhibition of NFκB are yet to be reviewed. Therefore, in this mini-review, we focused on the anti-inflammatory actions of flavonoids via inhibition of NFκB mechanism in CVDs.
Flavonoids and Its Subclass
Flavonoids are categorized into six subclasses depending on its chemical structures: flavones, flavonols, flavanones, isoflavones, flavan-3-ols, and anthocyanidins (Panche et al., 2016).
Flavones are found abundant in flowers, fruits, and leaves such as red peppers, celery, parsley, chamomile, mint, and ginkgo biloba (Manach et al., 2004). The most studied flavones are luteolin, apigenin, and tangeritin (Manach et al., 2004).
Flavonols such as kaempferol, myricetin, quercetin, rutin, fisetin, silymarin, and isorhamnetin are ubiquitous in foods such as saffron, onions, kale, lettuce, tomatoes, apples, grapes, berries, red wine, and tea (Pollastri and Tattini, 2011).
Flavanones widely present in all citrus fruits, which gives the bitter taste of the juice and its peel. Oranges, lemons, and grapes are rich sources of flavanones and major compounds are hesperitin, naringenin, and eriodictyol (Barreca et al., 2017).
Isoflavones are unique in that they resemble estrogen in structure and, therefore, are classified as phytoestrogens. There are found abundantly in soy products such as tofu, roasted soy nuts, and miso (Marzocchella et al., 2011).
Flavan-3-ols, also called as dihydroflavonols, include catechin, epicatechin, gallocatechin, epigallocatechin, epicatechingallate, epigallocatechingallate, and procyanidin (Alkhalidy et al., 2018). The most commonly associated food with the flavan-3-ol compounds is black and green tea and fruits such as bananas, apples, blueberries, peaches, and pears (Osakabe, 2013).
Anthocyanins are rich in outer cell layers of fruits such as merlot grapes, raspberries, cranberries, red grapes, strawberries, blueberries, bilberries, and blackberries. The most commonly studied anthocyanins are cyanidin, delphinidin, malvidin, pelargonidin, and peonidin (Khoo et al., 2017).
Nfκb Induced Inflammation and CVDs
There are a few cellular redox pathways involved in the development of the chronic inflammatory CVD, which includes NFκB. NFκB is a transcription factor that activates inhibitor of kappa B (IκB) kinase in the cytosol upon being stimulated by inflammatory stimuli (Brasier, 2010). Subsequent signaling pathways via canonical or non-canonical lead to migration of NFκB toward the nucleus and hence initiates the targeting gene such as pro-inflammatory cells, monocytes, macrophages, and T and B cells (Figure 1).
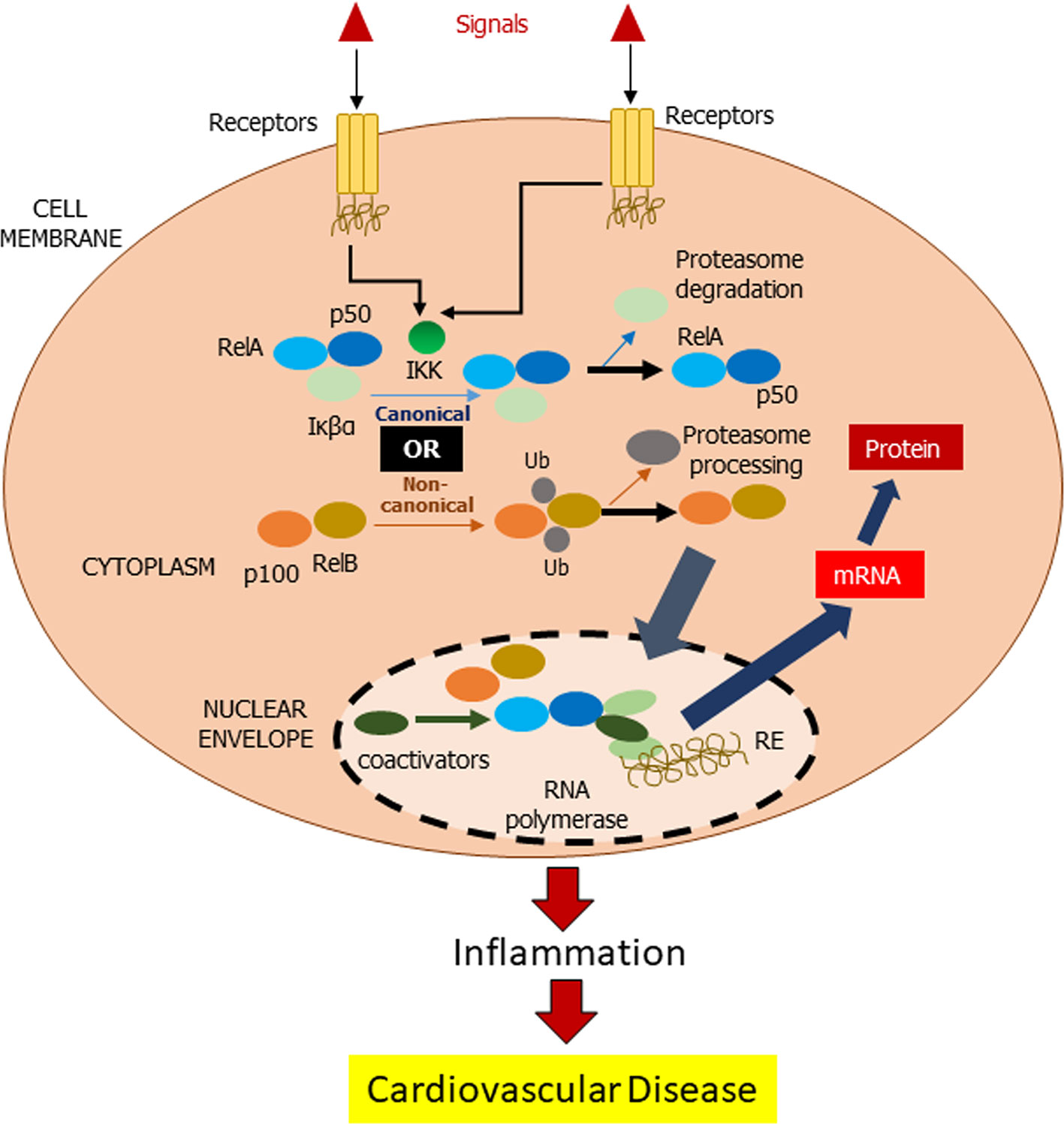
Figure 1 Mechanism of NFκB action. In inactivated state, NF-κβ, which consists of Rel and p50 proteins, is located in the cytosol complexed with the inhibitory protein Iκβα. IκB kinase (IKK) is activated by extracellular signals via membrane receptors. Subsequently, IKK phosphorylates the Iκβα protein resulting in ubiquitination of Iκβα and eventually by the proteasome for Iκβα degradation (canonical pathway). In non-canonical pathway, RelB favors the activation of NF-κβ via RelB. Activated NF-κβ is further translocated into the nucleus for DNA bindings, called response elements (RE). The DNA/NF-κβ complex attracts coactivators and RNA polymerase, which transcribe the DNA into mRNA resulting in a cell transformation.
The canonical NFκB pathway responds rapidly to stimuli and activates NFκB, which increases pro-inflammatory cytokines such as interleukin (IL)-1β, IL-6, and tumor necrosis factor alpha (TNF-α), which results in cell apoptosis. TNF-α receptor signaling plays an important role in the canonical pathway of NFκB in cell death via Jun N-terminal kinases (JNK), p38, and caspase 8 cascades (Ghosh et al., 2009). Furthermore, NFκB also activates angiotensin II, endothelin-1, and phenylephrine as hypertrophic agonist via IκB degradation and p65 nuclear translocation.
A central signaling component of the non-canonical NFκB pathway is NFκB-inducing kinase, which induces p100 phosphorylation through kinase IKKα in a slow manner (Sun, 2017). Ligands of a subset of tumor necrosis factor receptor (TNFR) superfamily members are typical inducers of the non-canonical NFκB pathway (Shih et al., 2011).
Anti-Inflammatory Effects of Flavonoids in CVDs via Modulation of NFκB Signaling
Quercetin
Quercetin or 3, 3′, 4′, 5, 7-pentahydroxyflvanone that falls into the category of flavonol is widely found in plants such as Ginkgo biloba, Hypericum perforatum, and Sambucus canadensis as well as vegetables such as apples, berries, grapes, onions, shallots, and tomatoes (Li et al., 2016).
In a clinical study involving patients with chronic systemic inflammation (CSI) in stable coronary artery disease (CAD), quercetin showed anti-inflammatory effects with reduction in indicators of CSI (Chekalina et al., 2018). Quercetin decreased IL-1β and TNF-α levels in blood serum, in addition to decreasing the transcriptional activity of NFкB in blood mononuclear cells (Chekalina et al., 2018). In leptin-induced inflammation model using human umbilical vein endothelial cells (HUVECs), quercetin significantly suppressed the upregulation of Ob-Ra (leptin receptor) expression, ERK1/2 phosphorylation, NFкB, and TNF-α (Indra et al., 2013). Furthermore, in a neonatal rat cardiac fibroblast, quercetin inhibited TNF-α, IL-1β, and IL-6 secretion by inhibiting the activation of NFкB and Akt induced by lipopolysaccharide (LPS) (Tang et al., 2014).
Luteolin
Luteolin or 3’, 4’, 5, 7-tetrahydroxyflavone is one of the most prevalent flavones widely found in fruits and vegetables such as carrots, cabbages, parsley, broccoli, celery, and apple skins (Weng and Yen, 2012).
In an in-vivo sodium fluoride-induced hypertensive model, administration of luteolin increased nitric oxide (NO) bioavailability, reversed prolongation of QT and QTc intervals, and reduced the expressions of kidney injury marker 1 (Kim-1), NFκB, and cardiac troponin I (CTnI), which eventually normalized the blood pressure (Oyagbemi et al., 2018a). Previous study in neonatal rat cardiac myocytes exposed to LPS showed luteolin reduced the TNF-α levels in the medium, downregulated the TNF-α mRNA in myocytes, inhibited degradation of IκB-β and nuclear translocation of NFκB, as well as reduced NFκB DNA binding, proposing the therapeutic potential of luteolin the management of inflammation-related myocardial diseases (Lv et al., 2011).
Fisetin
Fisetin or 3, 3c,4c,7-tetrahydroxyflavone is a bioactive molecule found in fruits such as strawberry, apple, persimmon, and grape and vegetables such as onion and cucumber (Arai et al., 2000).
Garg et al. (2019) reported the protective effect of fisetin against isoproterol-induced myocardial injury by suppressing myocardial injury markers, creatine kinase-muscle/brain (CK-MB), lactate dehydrogenase (LDH), and inflammatory markers (TNF-α and IL-6) in the blood serum as well as normalization of histological and ultrastructure of the heart. In addition, fisetin regulated the balance between pro- or anti-oxidants and pro- or anti-apoptotics proteins in the myocardial tissue (Garg et al., 2019). These protective effects of fisetin are attributed to the downregulation of receptor for advanced glycation end products (RAGE) and NFκB (Garg et al., 2019).
Fisetin attenuated the development of diabetic cardiomyopathy by attenuating the expression of myocardial NFκB and the pro-inflammatory cytokines IL-1β, IL-6, and TNF-α in the heart of diabetic rats. These result in reduction of cardiac function markers such as CK-MB, LDH, and cTn as well as normalization heart morphology (Althunibat et al., 2019).
Apigenin
Apigenin, a flavone, is found widely available in fruits and vegetables, such as grapefruits, oranges, celeries, and onions (Ren et al., 2018).
In LPS-treated macrophages, apigenin has been shown to reduce toll-like receptor 4 (TLR-4), MyD88, and p-IκB-α expression levels via nuclear NFκB p65 signaling pathway (Ren et al., 2018). Similarly, in LPS-challenged apoE-/- mice, treatment with apigenin increased expression of ATP binding cassette A1 (ABCA1), which alleviated extra lipid accumulation, reduced miR-33, TLR-4, and NFκB p65 levels, lessened the macrophages and smooth muscle cell contents in the atherosclerotic region, and improved plasma lipid profile (Ren et al., 2018). These results suggested that apigenin attenuates atherogenesis by inhibition of nuclear NFκB p65 that up-regulates ABCA1-mediated cholesterol efflux (Ren et al., 2018).
Apigenin was also shown to improve cardiac dysfunction and fibrosis in diabetic cardiomyopathy. Apigenin blunted the activity of NFκB and downregulated the activity of caspase3 accompanying with decreasing oxidative stress marker, glutathione peroxidase (GSH-Px), malondialdehyde (MAD), and superoxide dismutase (SOD) (Huangjun et al., 2016).
Isoliquiritigenin
Isoliquiritigenin is extracted from root of licorice and has been used traditionally for the treatment of inflammatory or pulmonary diseases (Peng et al., 2015). In HUVECS exposed to TNF-α, isoliquiritigenin blocked the involvement of NFкB at the transcriptional levels, and thus attenuated the downstream expression of VCAM-1, E-selectin, THP-1 monocyte adhesion, IкB-α, and PECAM-1, suggesting the protective effects of isoliquiritigenin through NFкB-dependent mechanisms (Kwon et al., 2007). In angiotensin II induced hypertension model, isoliquiritigenin attenuated inflammation cytokines including IL-1β and TNF-α, excessive deposition of extracellular matrix, and oxidative stress-induced apoptosis via nuclear factor E2-related factor 2 (Nrf2) and NFκB pathways (Xiong et al., 2018).
Rutin
Rutin is a flavonol that presents in buckwheat and citrus fruits. In a sodium fluoride-induced hypertensive rats, administration of rutin reduced blood pressure elevation by enhancing NO bioavailability via down-regulation of NFκB expression and up-regulation of Nrf2 (Oyagbemi et al., 2018b).
In carfilzomib-induced cardiotoxicity in rat, rutin protected against myocardial hypertrophy by upregulating IκB-α and downregulating NFκB expression, resulting in attenuation of β-myosin heavy chain, reduction in B-type natriuretic peptide mRNA expressions, and normalization of cardiac muscle fiber morphology (Imam et al., 2017).
In addition, rutin increased activities of Nrf, decreased activation of NFκB in human embryonic kidney reporter cell line, and preserved relaxation of fetal placental arteries derived from human chorionic plate (Sthijns et al., 2017).
In high mobility group box 1 (HMGBI)-induced inflammatory response in HUVECs, rutin attenuated NFκB and ERK1/2, which, in turn, reduced IL-6 and TNF-α levels (Yoo et al., 2014). Up-regulation of VCAM-1, intercellular adhesion molecule-1 (ICAM-1), and E-selectin induced by HMGB1 were similarly inhibited by rutin, suggesting that the protective effect of rutin on vascular inflammation is by inhibiting the HMGB1 and NFκB pathways.
In LPS-induced inflammation in HUVECs, rutin reversed barrier disruption, expression of cell adhesion molecules, and adhesion and migration of monocytes in endothelial cells. The barrier protective effects of rutin were linked to a down-regulation of TNF-α, deactivation of NFκB, and reduced phosphorylation of IκB-α (Lee et al., 2012).
Chrysin
Chrysin (5,7-dihydroxyflavone) is a flavone, which is found in the blue passion flower, honey, and propolis (Mantawy et al., 2017). Chrysin prevented doxorubicin (DOX)-induced cardiomyopathy including disturbance of cardiac conduction, increased serum cardiac markers and histopathological alteration in heart of rat via downregulation of NFκB, mitogen-activated protein kinase (MAPK), suppression of AKT pathway and its upstream activator, vascular endothelial growth factor (VEGF) (Mantawy et al., 2017).
In a rat model of monocrotaline-induced pulmonary arterial hypertension (PAH), chrysin reduced right ventricular systolic pressure and mean pulmonary artery pressure. In addition to suppression of right ventricular remodeling, chrysin abolished increased expression of collagen I, collagen III, and NFκB (Li et al., 2015).
In isoprenaline-induced myocardial injury in rats, chrysin relieved hemodynamic and ventricular dysfunction as well as reduced ultrastructural myocardial damage via inhibition of NFκB, IκKβ expression, and TNF-α level as well as increased peroxisome proliferator-activated receptor-gamma (PPAR-γ) expression (Rani et al., 2016).
In a rat model of myocardial infarction, fibrosis in the interstitial and perivascular regions and expression of collagen was reduced following chrysin treatment (Yang et al., 2018). This effect is associated with increased PPAR-γ expression and decreased NFκB expression via inhibition of IκKβ phosphorylation, leading to reduction of matrix metalloproteinase-2 (MMP-2), MMP-9 levels, and suppression of activator protein 1 (AP-1) level.
Genistein
Genistein under the subgroup of an isoflavone [4′,5,7-trihydroxyisoflavone,5,7-dihydroxy-3-(4-hydroxyphenyl)-4-H-1-benzopyran-4-one] is primarily found in soy-based foods, legumes, and red clover. In homocysteine-induced endothelial cell inflammatory injury, genistein prevented endothelial damage via blockade of activation of NFκB, expression of inflammatory cytokine and adhesion molecule, IL-6, and ICAM-1 (Han et al., 2015).
Xu et al. (2019) explored the effect of genistein on angiotensin II-induced vascular smooth muscle cell inflammation. Angiotensin II-induced expression of NFκB, C-reactive protein (CRP), MMP-9, phosphorylation of ERK1/2 and p-38, which lead to atherosclerotic inflammatory damage, were reversed following genistein treatment. Furthermore, genistein enhanced expression of PPAR-γ, suggesting cardiovascular protective effect by the isoflavone is through regulation of p38/ERK1/2-PPARγ-NFκB signaling pathway (Xu et al., 2019).
Silymarin
Silymarin is a flavonolignan extracted from the milk thistle. Silymarin augmented relaxation of pulmonary arteries isolated from a lung ischemia-reperfusion (I/R) injury model (Jin et al., 2016). Vascular protective effect of silymarin is due to inhibition of NFκB, thus suppressing the serum concentration of inflammatory cytokines and reducing protein expression of hypoxia inducible factor-1α (HIF-1α) and iNOS.
Silibinin, a major active constituent of silymarin, was able to reduce the abnormal size of cardiac myocytes and prevent hypertrophy by alleviating the production of epidermal growth factor receptor (EGFR) (Ai et al., 2010). Silibilin exerted its anti-inflammatory effect by suppressing the activation of NFκB stimulated by angiotensin II in cardiac myocytes or in the aortic banding male mice. Furthermore, silibilin interfered with the phosphorylation and degradation of IκB-α and activation of IκKβ in vivo.
Kaempferol
Kaempferol (3,4′,5,7-tetrahydroxyflavone) is a flavonol that is present widely in fruits, vegetables, and herbs, including grapes, tomatoes, and tea. In cardiac fibroblasts stimulated with LPS, kaempferol decreased release of pro-inflammatory cytokines by inhibiting AKT phosphorylation and NFκB activation (Tang et al., 2015). In isoprenaline-induced cardiac damage, kaempferol improved the hemodynamic and left ventricular functions in male rats, which abated the increased serum concentration of CK-MB and LDH, preserved the morphology of myocardium, and reduced the levels of pro-inflammatory cytokines (Suchal et al., 2016a). Similarly, kaempferol prevented cardiac damage by inhibiting the protein expression of NFκB, p38, and JNK (Suchal et al., 2016b) suggesting that cardioprotective and anti-inflammatory action of kaempferol was associated with NFκB signaling pathway.
Table 1 summarizes the effects and mechanisms of action of flavonoids in CVD.
Conclusion
The actions of flavonoids in mitigating inflammation by modulation of NFкB offer potential agents for the treatment of CVDs. However, several of these actions reported in vitro may yet to be fully recognized due to their low bioavailabilities following oral administration (Hollman and Katan, 1998; Thilakarathna and Rupasinghe, 2013). Flavonoids have shown promising results in reducing atherosclerosis in several animal experimental models; however, conflicting results were reported in human clinical trials (Arts and Hollman, 2005; Zordoky et al., 2015). The low bioavailability and clinical efficacy of flavonoids are attributed to their poor absorption, metabolism by the metabolizing enzymes in the intestine and liver, and structural modifications by the colonic bacteria remain as the major problems. Continuous investigation is required to enhance the bioavailability and efficacy of the flavonoids to tap the full potential of these natural agents.
Author Contributions
All authors contributed to the writing. KC, DM, and MM conceived, designed, and revised the manuscript.
Funding
This study was funded by Government Agency grant GA001-2017, MAHSA University and USIM research project code: PPPI/FPG/0118/051000/15618 Universiti Kebangsaan Malaysia and Universiti Putra Malaysia. The funding agencies played no role in the design of the study and collection, analysis, and interpretation of data and in writing the manuscript, which are fully the responsibilities of the authors.
Conflict of Interest
The authors declare that the research was conducted in the absence of any commercial or financial relationships that could be construed as a potential conflict of interest.
The reviewer ZJ declared a shared affiliation, with no collaboration, with one of the authors, XFL, to the handling editor at time of review
References
Ai, W., Zhang, Y., Tang, Q. Z., Yan, L., Bian, Z. Y., Liu, C., et al. (2010). Silibinin attenuates cardiac hypertrophy and fibrosis through blocking EGFR-dependent signaling. J. Cell. Biochem. 110, 1111–1122. doi: 10.1002/jcb.22623
Alkhalidy, H., Wang, Y., Liu, D. (2018). Dietary flavonoids in the prevention of t2d: an overview. Nutrients 10, 1–33. doi: 10.3390/nu10040438
Althunibat, O. Y., Al Hroob, A. M., Abukhalil, M. H., Germoush, M. O., Bin-Jumah, M., Mahmoud, A. M. (2019). Fisetin ameliorates oxidative stress, inflammation and apoptosis in diabetic cardiomyopathy. Life Sci. 221, 83–92. doi: 10.1016/j.lfs.2019.02.017
Arai, Y., Watanabe, S., Kimira, M., Shimoi, K., Mochizuki, R., Kinae, N. (2000). Dietary intakes of flavonols, flavones and isoflavones by Japanese women and the inverse correlation between quercetin intake and plasma LDL cholesterol concentration. J. Nutr. 130, 2243–2250. doi: 10.1093/jn/130.9.2243
Arikawa, M., Kakinuma, Y., Noguchi, T., Todaka, H., Sato, T. (2016). Donepezil, an acetylcholinesterase inhibitor, attenuates LPS-induced inflammatory response in murine macrophage cell line RAW 264.7 through inhibition of nuclear factor kappa B translocation. Eur. J. Pharmacol. 789, 17–26. doi: 10.1016/j.ejphar.2016.06.053
Arts, I. C., Hollman, P. C. (2005). Polyphenols and disease risk in epidemiologic studies. Am. J. Clin. Nutr. 81(1 Suppl), 317s–325s. doi: 10.1093/ajcn/81.1.317S
Azab, A., Nassar, A., Azab, A. N. (2016). Anti-inflammatory activity of natural products. Molecules 21, 1–19. doi: 10.3390/molecules21101321
Barreca, D., Gattuso, G., Bellocco, E., Calderaro, A., Trombetta, D., Smeriglio, A., et al. (2017). Flavanones: Citrus phytochemical with health-promoting properties. Biofactors 43, 495–506. doi: 10.1002/biof.1363
Benjamin, E. J., Blaha, M. J., Chiuve, S. E., Cushman, M., Das, S. R., Deo, R., et al. (2017). Heart disease and stroke statistics-2017 update: a report from the american heart association. Circulation 135, e146–e603. doi: 10.1161/CIR.0000000000000485
Brasier, A. R. (2010). The nuclear factor-κB-interleukin-6 signalling pathway mediating vascular inflammation. Cardiovasc. Res. 86, 211–218. doi: 10.1093/cvr/cvq076
Chekalina, N., Burmak, Y., Petrov, Y., Borisova, Z., Manusha, Y., Kazakov, Y., et al. (2018). Quercetin reduces the transcriptional activity of NF-kB in stable coronary artery disease. Indian Heart J. 70, 593–597. doi: 10.1016/j.ihj.2018.04.006
Da Silva Franco, N., Lubaczeuski, C., Guizoni, D. M., Victorio, J. A., Santos-Silva, J. C., Brum, P. C., et al. (2017). Propranolol treatment lowers blood pressure, reduces vascular inflammatory markers and improves endothelial function in obese mice. Pharmacol. Res. 122, 35–45. doi: 10.1016/j.phrs.2017.05.018
Daher, M. T., Mougenot, N., Rohr, O., Bausero, P., Liu, P., Li, Z., et al. (2019). Role of the transcription factor Bcl11b in cardiac homeostasis and remodeling. Arch. Cardiovasc. Dis. Suppl. 11, 231. doi: 10.1016/j.acvdsp.2019.02.109
Di Raimondo, D., Tuttolomondo, A., Butta, C., Miceli, S., Licata, G., Pinto, A. (2012). Effects of ACE-inhibitors and angiotensin receptor blockers on inflammation. Curr. Pharm. Des. 18, 4385–4413. doi: 10.2174/138161212802481282
Garg, S., Malhotra, R. K., Khan, S. I., Sarkar, S., Susrutha, P. N., Singh, V., et al. (2019). Fisetin attenuates isoproterenol-induced cardiac ischemic injury in vivo by suppressing RAGE/NF-kappaB mediated oxidative stress, apoptosis and inflammation. Phytomedicine 56, 147–155. doi: 10.1016/j.phymed.2018.09.187
Ghosh, J., Das, J., Manna, P., Sil, P. C. (2009). Taurine prevents arsenic-induced cardiac oxidative stress and apoptotic damage: Role of NF-κB, p38 and JNK MAPK pathway. Toxicol. Appl. Pharmacol. 240, 73–87. doi: 10.1016/j.taap.2009.07.008
Han, S., Wu, H., Li, W., Gao, P. (2015). Protective effects of genistein in homocysteine-induced endothelial cell inflammatory injury. Mol. Cell. Biochem. 403, 43–49. doi: 10.1007/s11010-015-2335-0
Haybar, H., Rezaeeyan, H., Shahjahani, M., Shirzad, R., Saki, N. (2019). T-bet transcription factor in cardiovascular disease: attenuation or inflammation factor? J. Cell Physiol. 234, 7915–7922. doi: 10.1002/jcp.27935
Hollman, P. C., Katan, M. B. (1998). Bioavailability and health effects of dietary flavonols in man. Arch. Toxicol. Suppl. 20, 237–248.
Huangjun, L., Zheng, Y., Wei, D., Qizhu, T. (2016). GW27-e0367 Apigenin attenuates the cardiac remodeling in experimental diabetic cardiomyopathy. J. Am. Coll. Cardiol. 68, 1–10. doi: 10.1016/j.jacc.2016.07.054
Imam, F., Al-Harbi, N. O., Al-Harbia, M. M., Korashy, H. M., Ansari, M. A., Sayed-Ahmed, M. M., et al. (2017). Rutin attenuates carfilzomib-induced cardiotoxicity through inhibition of NF-κB, hypertrophic gene expression and oxidative stress. Cardiovasc. Toxicol. 17, 58–66. doi: 10.1007/s12012-015-9356-5
Indra, M. R., Karyono, S., Ratnawati, R., Malik, S. G. (2013). Quercetin suppresses inflammation by reducing ERK1/2 phosphorylation and NF kappa B activation in Leptin-induced Human Umbilical Vein Endothelial Cells (HUVECs). BMC Res. Notes 6, 275–275. doi: 10.1186/1756-0500-6-275
Jin, Y., Zhao, X., Zhang, H., Li, Q., Lu, G., Zhao, X. (2016). Modulatory effect of silymarin on pulmonary vascular dysfunction through HIF-1α-iNOS following rat lung ischemia-reperfusion injury. Exp. Therapeutic Med. 12, 1135–1140. doi: 10.3892/etm.2016.3370
Khalil, M. I., Sulaiman, S. A. (2010). The potential role of honey and its polyphenols in preventing heart diseases: a review. Afr. J. Tradition. Complementary Alternative Med.: AJTCAM 7, 315–321. doi: 10.4314/ajtcam.v7i4.56693
Khoo, H. E., Azlan, A., Tang, S. T., Lim, S. M. (2017). Anthocyanidins and anthocyanins: colored pigments as food, pharmaceutical ingredients, and the potential health benefits. Food Nutr. Res. 61, 1361779–1361779. doi: 10.1080/16546628.2017.1361779
Koeners, M. P., Wesseling, S., Sanchez, M., Braam, B., Joles, J. A. (2016). Perinatal Inhibition of NF-KappaB has long-term antihypertensive and renoprotective effects in fawn-hooded hypertensive rats. Am. J. Hypertens. 29, 123–131. doi: 10.1093/ajh/hpv065
Kurdi, M., Zgheib, C., Booz, G. W. (2018). Recent developments on the crosstalk between STAT3 and inflammation in heart function and disease. Front. Immunol. 9, 1–10. doi: 10.3389/fimmu.2018.03029
Kwon, H. M., Choi, Y. J., Choi, J. S., Kang, S. W., Bae, J. Y., Kang, I. J., et al. (2007). Blockade of cytokine-induced endothelial cell adhesion molecule expression by licorice isoliquiritigenin through NF-kappaB signal disruption. Exp. Biol. Med. (Maywood) 232, 235–245. doi: 10.3181/00379727-207-2320235
Lee, W., Ku, S.-K., Bae, J.-S. (2012). Barrier protective effects of rutin in LPS-induced inflammation in vitro and in vivo. Food Chem. Toxicol. 50, 3048–3055. doi: 10.1016/j.fct.2012.06.013
Li, X., Guo, B., Shen, Y., Yang, J. (2015). Effect of chrysin on expression of NOX4 and NF-κB in right ventricle of monocrotaline-induced pulmonary arterial hypertension of rats. Yao Xue Xue Bao= Acta Pharma. Sin. 50, 1128–1134. doi: 10.1016/j.jash.2017.03.008
Li, Y., Yao, J., Han, C., Yang, J., Chaudhry, M. T., Wang, S., et al. (2016). Quercetin, Inflammation and Immunity. Nutrients 8, 167–167. doi: 10.3390/nu8030167
Lin, X., Wu, M., Liu, B., Wang, J., Guan, G., Ma, A., et al. (2015). Candesartan ameliorates acute myocardial infarction in rats through inducible nitric oxide synthase, nuclear factorkappaB, monocyte chemoattractant protein1, activator protein1 and restoration of heat shock protein 72. Mol. Med. Rep. 12, 8193–8200. doi: 10.3892/mmr.2015.4432
Lv, L., Lv, L., Zhang, Y., Kong, Q. (2011). Luteolin prevents LPS-induced TNF-alpha expression in cardiac myocytes through inhibiting NF-kappaB signaling pathway. Inflammation 34, 620–629. doi: 10.1007/s10753-010-9271-7
Manach, C., Scalbert, A., Morand, C., Remesy, C., Jimenez, L. (2004). Polyphenols: food sources and bioavailability. Am. J. Clin. Nutr. 79, 727–747. doi: 10.1093/ajcn/79.5.727
Mantawy, E. M., Esmat, A., El-Bakly, W. M., Eldin, R.a.S., El-Demerdash, E. (2017). Mechanistic clues to the protective effect of chrysin against doxorubicin-induced cardiomyopathy: plausible roles of p53, MAPK and AKT pathways. Sci. Rep. 7, 4795. doi: 10.1038/s41598-017-05005-9
Marzocchella, L., Fantini, M., Benvenuto, M., Masuelli, L., Tresoldi, I., Modesti, A., et al. (2011). Dietary flavonoids: molecular mechanisms of action as anti- inflammatory agents. Recent Pat. Inflamm. Allergy Drug Discov. 5, 200–220. doi: 10.2174/187221311797264937
Osakabe, N. (2013). Flavan 3-ols improve metabolic syndrome risk factors: evidence and mechanisms. J. Clin. Biochem. Nutr. 52, 186–192. doi: 10.3164/jcbn.12-130
Oyagbemi, A. A., Omobowale, T. O., Ola-Davies, O. E., Asenuga, E. R., Ajibade, T. O., Adejumobi, O. A., et al. (2018a). Luteolin-mediated Kim-1/NF-kB/Nrf2 signaling pathways protects sodium fluoride-induced hypertension and cardiovascular complications. BioFactors 44, 518–531. doi: 10.1002/biof.1449
Oyagbemi, A. A., Omobowale, T. O., Ola-Davies, O. E., Asenuga, E. R., Ajibade, T. O., Adejumobi, O. A., et al. (2018b). Ameliorative effect of Rutin on sodium fluoride-induced hypertension through modulation of Kim-1/NF-κB/Nrf2 signaling pathway in rats. Environ. Toxicol. 33, 1284–1297. doi: 10.1002/tox.22636
Panche, A. N., Diwan, A. D., Chandra, S. R. (2016). Flavonoids: an overview. J. Nutr. Sci. 5, e47–e47. doi: 10.1017/jns.2016.41
Peng, F., Du, Q., Peng, C., Wang, N., Tang, H., Xie, X., et al. (2015). A review: the pharmacology of isoliquiritigenin. Phytother. Res. 29, 969–977. doi: 10.1002/ptr.5348
Pollastri, S., Tattini, M. (2011). Flavonols: old compounds for old roles. Ann. Bot. 108, 1225–1233. doi: 10.1093/aob/mcr234
Quintana-Villamandos, B., Goukassian, D. A., Sasi, S. P., Delgado-Baeza, E. (2018). Short-term treatment with esmolol reverses left ventricular hypertrophy in adult spontaneously hypertensive rats via inhibition of Akt/NF-κB and NFATc4. BioMed. Res. Int. 2018, 9. doi: 10.1155/2018/2691014
Rani, N., Bharti, S., Bhatia, J., Nag, T., Ray, R., Arya, D. S. (2016). Chrysin, a PPAR-γ agonist improves myocardial injury in diabetic rats through inhibiting AGE-RAGE mediated oxidative stress and inflammation. Chemico-Biol. Interact. 250, 59–67. doi: 10.1016/j.cbi.2016.03.015
Ren, K., Jiang, T., Zhou, H. F., Liang, Y., Zhao, G. J. (2018). Apigenin retards atherogenesis by promoting abca1-mediated cholesterol efflux and suppressing inflammation. Cell Physiol. Biochem. 47, 2170–2184. doi: 10.1159/000491528
Ruparelia, N., Chai, J. T., Fisher, E. A., Choudhury, R. P. (2017). Inflammatory processes in cardiovascular disease: a route to targeted therapies. Nat. Rev. Cardiol. 14, 133–144. doi: 10.1038/nrcardio.2016.185
Shih, V. F.-S., Tsui, R., Caldwell, A., Hoffmann, A. (2011). A single NFκB system for both canonical and non-canonical signaling. Cell Res. 21, 86–102. doi: 10.1038/cr.2010.161
Smale, S. T., Natoli, G. (2014). Transcriptional control of inflammatory responses. Cold Spring Harb. Perspect. Biol. 6, a016261. doi: 10.1101/cshperspect.a016261
Sthijns, M. M., Schiffers, P. M., Janssen, G. M., Lemmens, K. J., Ides, B., Vangrieken, P., et al. (2017). Rutin protects against H2O2-triggered impaired relaxation of placental arterioles and induces Nrf2-mediated adaptation in human umbilical vein endothelial cells exposed to oxidative stress. Biochim. Biophys. Acta (BBA)-General Subjects 1861, 1177–1189. doi: 10.1016/j.bbagen.2017.03.004
Suchal, K., Malik, S., Gamad, N., Malhotra, R. K., Goyal, S. N., Bhatia, J., et al. (2016a). Kampeferol protects against oxidative stress and apoptotic damage in experimental model of isoproterenol-induced cardiac toxicity in rats. Phytomedicine 23, 1401–1408. doi: 10.1016/j.phymed.2016.07.015
Suchal, K., Malik, S., Gamad, N., Malhotra, R. K., Goyal, S. N., Chaudhary, U., et al. (2016b). Kaempferol attenuates myocardial ischemic injury via inhibition of MAPK signaling pathway in experimental model of myocardial ischemia-reperfusion injury. Oxidative Med. Cell. Longevity 2016, 1–1. doi: 10.1155/2016/7580731
Sun, S.-C. (2017). The non-canonical NF-κB pathway in immunity and inflammation. Nat. reviews. Immunol. 17, 545–558. doi: 10.1038/nri.2017.52
Tang, X.-l., Liu, J.-x., Dong, W., Li, P., Li, L., Hou, J.-c., et al. (2015). Protective effect of kaempferol on LPS plus ATP-induced inflammatory response in cardiac fibroblasts. Inflammation 38 (1), 94–101. doi: 10.1007/s10753-014-0011-2
Tang, X.-L., Liu, J.-X., Dong, W., Li, P., Li, L., Zheng, Y.-Q., et al. (2014). Intervention effect of quercetin on inflammatory secretion of cardiac fibroblasts.
Thilakarathna, S. H., Rupasinghe, H. P. V. (2013). Flavonoid bioavailability and attempts for bioavailability enhancement. Nutrients 5 (9), 3367–3387. doi: 10.3390/nu5093367
Van Der Heiden, K., Cuhlmann, S., Luong Le, A., Zakkar, M., Evans, P. C. (2010). Role of nuclear factor kappaB in cardiovascular health and disease. Clin. Sci. (Lond) 118, 593–605. doi: 10.1042/CS20090557
Wang, X., Chen, Q., Pu, H., Wei, Q., Duan, M., Zhang, C., et al. (2016). Adiponectin improves NF-kappaB-mediated inflammation and abates atherosclerosis progression in apolipoprotein E-deficient mice. Lipids Health Dis. 15, 33. doi: 10.1186/s12944-016-0202-y
Weng, C. J., Yen, G. C. (2012). Flavonoids, a ubiquitous dietary phenolic subclass, exert extensive in vitro anti-invasive and in vivo anti-metastatic activities. Cancer Metastasis Rev. 31, 323–351. doi: 10.1007/s10555-012-9347-y
Xiong, D., Hu, W., Ye, S.-T., Tan, Y.-S. (2018). Isoliquiritigenin alleviated the Ang II-induced hypertensive renal injury through suppressing inflammation cytokines and oxidative stress-induced apoptosis via Nrf2 and NF-κB pathways. Biochem. Biophysical Res. Commun. 506, 161–168. doi: 10.1016/j.bbrc.2018.09.013
Xu, L., Liu, J.-T., Li, K., Wang, S.-Y., Xu, S. (2019). Genistein inhibits Ang II-induced CRP and MMP-9 generations via the ER-p38/ERK1/2-PPARγ-NF-κB signaling pathway in rat vascular smooth muscle cells. Life Sci. 216, 140–146. doi: 10.1016/j.lfs.2018.11.036
Yang, M., Xiong, J., Zou, Q., Wang, D.-D., Huang, C.-X. (2018). Chrysin attenuates interstitial fibrosis and improves cardiac function in a rat model of acute myocardial infarction. J. Mol. histology 49, 555–565. doi: 10.1007/s10735-018-9793-0
Yoo, H., Ku, S.-K., Baek, Y.-D., Bae, J.-S. (2014). Anti-inflammatory effects of rutin on HMGB1-induced inflammatory responses in vitro and in vivo. Inflammation Res. 63, 197–206. doi: 10.1007/s00011-013-0689-x
Zeinali, M., Rezaee, S. A., Hosseinzadeh, H. (2017). An overview on immunoregulatory and anti-inflammatory properties of chrysin and flavonoids substances. Biomed Pharmacother 92, 998–1009. doi: 10.1016/j.biopha.2017.06.003
Zhao, H., Shen, R., Dong, X., Shen, Y. (2017). Murine double minute-2 inhibition attenuates cardiac dysfunction and fibrosis by modulating nf-kappab pathway after experimental myocardial infarction. Inflammation 40, 232–239. doi: 10.1007/s10753-016-0473-5
Keywords: flavonoids, nuclear factor-kappa B signalling, anti-inflammatory, cardiovascular diseases, natural compounds
Citation: Choy KW, Murugan D, Leong X-F, Abas R, Alias A and Mustafa MR (2019) Flavonoids as Natural Anti-Inflammatory Agents Targeting Nuclear Factor-Kappa B (NFκB) Signaling in Cardiovascular Diseases: A Mini Review. Front. Pharmacol. 10:1295. doi: 10.3389/fphar.2019.01295
Received: 16 August 2019; Accepted: 10 October 2019;
Published: 31 October 2019.
Edited by:
Cheng Xue Helena Qin, Baker Heart and Diabetes Institute, AustraliaReviewed by:
Agnieszka Barbara Najda, University of Life Sciences of Lublin, PolandZakiah Jubri, National University of Malaysia, Malaysia
Copyright © 2019 Choy, Murugan, Leong, Abas, Alias and Mustafa. This is an open-access article distributed under the terms of the Creative Commons Attribution License (CC BY). The use, distribution or reproduction in other forums is permitted, provided the original author(s) and the copyright owner(s) are credited and that the original publication in this journal is cited, in accordance with accepted academic practice. No use, distribution or reproduction is permitted which does not comply with these terms.
*Correspondence: Ker Woon Choy, Y2VsaW5lX2tlcndvb25AeWFob28uY29t; Dharmani Murugan, ZGhhcm1hbmk3OUB1bS5lZHUubXk=; Mohd Rais Mustafa, cmFpc0B1bS5lZHUubXk=