Corrigendum: Hydroxysafflor Yellow A Protects Against Myocardial Ischemia/Reperfusion Injury via Suppressing NLRP3 Inflammasome and Activating Autophagy
- 1Beijing Key Laboratory of Innovative Drug Discovery of Traditional Chinese Medicine (Natural Medicine) and Translational Medicine, Institute of Medicinal Plant Development, Chinese Academy of Medical Sciences & Peking Union Medical College, Beijing, China
- 2College of Pharmacy, Harbin University of Commerce, Harbin, China
- 3Pneumology Department, Xiyuan Hospital, China Academy of Chinese Medical Sciences, Beijing, China
Myocardial ischemia/reperfusion (MI/R) injury is a serious threat to human health. Hydroxysafflor yellow A (HSYA), the main water-soluble ingredient extracted from Carthami flos (Carthamus tinctorius L.), has therapeutic potential for treating MI/R injury. However, the mechanisms of HSYA−mediated protection from MI/R injury are incompletely understood. In the present study, we investigated the effects and the underlying mechanisms of HSYA during MI/R. Adult Sprague-Dawley rats were subjected to left anterior descending artery ligation for 30 min followed by 24 h of reperfusion with or without HSYA treatment. The protective effect of HSYA was detected by 2,3,5-triphenyl tetrazolium chloride (TTC) staining, hematoxylin eosin (HE) staining, and myocardial enzymes detections. Serum levels of inflammatory factors such as TNF-α, interleukin (IL)-1β, and IL-18, were detected using ELISA kits. The expression of NLRP3 and other related proteins in the myocardium was detected by western blot and immunohistochemistry. The expression of autophagy-related proteins, including Atg5, BECN1, P62, and LC3B, was detected by western blot to evaluate the effect of HSYA on autophagy. Results showed that HSYA decreased the myocardial infarct size and attenuated the cardiac dysfunction in rats after I/R. In addition, HSYA inhibited myocardial apoptosis compared with the I/R group, decreased the levels of inflammatory cytokines in rat serum, reduced NLRP3 inflammasome expression, and induced autophagy. Mechanistically, our results demonstrated that HSYA can activate AMPK to improve autophagy and inhibit NLRP3 inflammasome by inhibiting the mTOR pathway. This work provides strong data supporting for the clinical applications of HSYA in MI/R injury.
Introduction
Ischemic heart disease is the biggest threat to human health worldwide (Grant et al., 2017). Early blood flow recovery plays an important role in maintaining cardiac function and reducing myocardial cell damage in patients with myocardial ischemia. However, as blood flow is recovered, myocardial infarction caused by reperfusion injury can account for over half of the total infarct area caused by ischemia (Lesnefsky et al., 2017; Zheng et al., 2020). Therefore, the prevention and treatment of reperfusion injury is particularly critical.
The inflammatory response is one of the important pathological mechanisms of myocardial ischemia/reperfusion (MI/R) injury. Moreover, inflammatory response results in cardiomyocyte apoptosis during the acute phase. NLRP3 inflammasome has been fully characterized and plays an important role in MI/R injury. Reperfusion induces activation of NLRP3 inflammasome, and the resulting interleukin (IL)-1β, IL-18, and active caspase-1 are also involved in MI/R process (Toldo et al., 2019). Previous studies showed that inhibition or deletion of NLRP3 inflammasome could reduce myocardial infarction size and improve cardiac function in experimental animal models (Zuurbier et al., 2019). Autophagy, a lysosome-mediated pathway to degrade cell fragments and organelles, plays an important role in maintaining the balance of cell structure, metabolism, and function. Many studies have shown that autophagy of cardiomyocytes has a great influence in I/R injury and autophagy limits myocardial damage due to infarction (Bravo-San Pedro et al., 2017). Importantly, autophagy suppresses inflammasome assembly and negatively influences IL-1 and IL-18 secretion (De Biase et al., 2020). Thus, inhibiting NLRP3 inflammasome by regulating autophagy is an effective approach to alleviate MI/R injury.
Hydroxysafflor yellow A (HSYA), the main water-soluble ingredient extracted from Carthami flos (Carthamus tinctorius L.), is predominantly used in the treatment of cardiovascular diseases (Li et al., 2019; Xu et al., 2019). Previous study reported that HSYA can resist inflammation, alleviate oxidation and apoptosis, improve neurological function, alleviate infarct volume, inhibit thrombosis formation and platelet aggregation, reduce brain edema, and protect blood vessels (Han et al., 2016; Wang et al., 2016; Dong et al., 2017; Sun et al., 2018; Li et al., 2019). Recently, our laboratory found that HSYA has a protective effect on hypoxia/reoxygenation-induced cardiomyocyte damage in vitro, and the mechanism was related to NLRP3 inflammasome inhibition (Ye et al., 2020). However, whether HSYA could play a protective role in MI/R in vivo by inhibiting NLRP3 inflammasome has yet to be determined.
In the present study, we explore the cardiac protection of HSYA in an MI/R rat model in vivo and its potential mechanisms for NLRP3 inhibition. Our results provide strong data supporting for the clinical applications of HSYA in MI/R injury.
Material and Methods
Reagents
HSYA (purity > 98%) was produced by Shanghai Winherb Medical S&T Development (Shanghai, China). Diltiazem hydrochloride tablets (DTZ) ware obtained from Tianbian Pharmaceutical Co., Ltd. (Tianjin, China). Antibodies against P62 and LC3II were obtained from Sigma-Aldrich (St. Louis, MO, USA). Antibodies against NLRP3, ASC, caspase-1 were purchased from Abclonal (Wuhan, China). The primary antibodies against IL-1β, mammalian target of rapamycin (mTOR), and p-mTOR were obtained from Abcam (Cambridge, UK), and the primary antibodies against Bax, Bcl-2, and Caspase-3 were obtained from Proteintech (Wuhan, China). The primary antibodies against AMPK, p-AMPK, Atg5, and BECN1 were obtained from Cell Signaling Technology (Boston, USA).
Animals
Adult male Sprague-Dawley rats (280±20 g) were obtained from Beijing Vital River Laboratory Animal Technology Co., Ltd. (Beijing, China). All animal experiments were conducted according to the principles of the Institutional Animal Care and Use Committee (IACUC) at the Chinese Academy of Medical Sciences and Peking Union Medical College (Beijing, China) (Approval No.: SLXD-20190702003). The animals were housed under controlled temperatures and humidity on a 12 h light-dark cycle. Food and water were provided ad libitum.
Myocardial Ischemia/Reperfusion Model
Myocardial ischemia was inflicted by ligating the left anterior descending coronary artery (Xu et al., 2020). Briefly, 90 rats were anesthetized through intraperitoneal injection of pentobarbital sodium (40 mg/kg), intubated in the supine position, and monitored using electrocardiogram. After fur was removed, the thorax was opened, and the left anterior descending branch was ligated with a 6–0 suture for 30 min. The ligation was opened, and the heart was reperfused for 24 h. The sham group was subjected to the same procedures but without ligation. After the operation, the ventilator was removed when the rats were able to breathe spontaneously. They were then placed on a thermostatic blanket until they could walk, and were returned to the cage. After 24 h of reperfusion, the rats were weighed and anesthetized. Blood was obtained from the abdominal aorta, and the heart was removed and rinsed with phosphate-buffered saline (PBS). The hearts were randomly selected among the experimental group and placed in 4% paraformaldehyde for fixation, and the rest of the hearts were stored at –80°C. Whole blood obtained from the abdominal aorta was centrifuged (3,000 rpm, 15 min), and the supernatant serum was absorbed and frozen at –80°C for later use.
Drugs Administration
Ninety rats were randomly divided into six experimental groups as follows: sham group (n=15), I/R group (n=15), 4 mg/kg HSYA group (n=15), 8 mg/kg HSYA group (n=15), 16 mg/kg HSYA group (n=15), and 16 mg/kg DTZ group (n=15) as a positive control. As a calcium antagonist, DTZ is widely used to treat coronary heart disease and hypertension. Clinical studies have shown that DTZ can increase coronary blood flow in patients with myocardial ischemia and prevent arrhythmias during reperfusion (Herzog et al., 1997; Pizzetti et al., 2001). In addition, several studies have shown that DTZ can reduce oxidative stress injury, correct energy metabolism, improve endothelial function, and regulate apoptosis (Peng et al., 2014; Chen et al., 2016). Therefore, DTZ was selected as the positive control in the present study. The dosage for HSYA and DTZ was chosen based on previous pharmacodynamics studies (Mu et al., 2017; Yu L. et al., 2018; Yu et al., 2020). HSYA and DTZ were dissolved in 0.9% sodium chloride and stored at 4°C. Rats in HSYA group were administered 4, 8, and 16 mg/kg HSYA by tail intravenous injection 30 min prior to ischemia operation. The same doses of HSYA were given 30 min after the onset of reperfusion. DTZ was orally administered to the rats once a day for five consecutive days prior to ischemia operation.
Detection of Myocardium Infarct Size
The myocardial infarct size was assessed using 2,3,5-triphenyl tetrazolium chloride (TTC) (Sigma-Aldrich; Merck KgaA, Germany) as previously described (Wang R. et al., 2019). After all treatment, the heart was removed, rinsed with saline, and frozen using liquid nitrogen for 1 min. Along the heart axis, the heart was cut into six slices and incubated in 2% TTC for 15 min at 37°C in the dark, and then fixed with 4% paraformaldehyde for 24 h. The heart slices were photographed with a digital camera, and the proportion of the infarcted area was assessed from the obtained images.
Detection of Myocardial Enzymes
The levels of creatine kinase-MB (CK-MB), aspartate transaminase (AST), and lactic dehydrogenase (LDH) in the serum were detected based on the commercial kits (BioSino, Beijing, China) using a Beckman AU480 biochemical auto-analyzer (Fullerton, CA, USA).
Enzyme-Linked Immunosorbent Assay (ELISA)
The concentrations of IL-18, IL-1β, and TNF-α levels in the serum of rats were measured using commercial ELISA kits (Beijing Expandbiotech Ltd., Beijing, China) in accordance with the manufacturer's instructions.
Histopathological Examination and Immunohistochemical Analyses
The heart tissue was fixed with 4% paraformaldehyde and embedded in paraffin. Subsequently, 5 μm sections were prepared and stained with hematoxylin and eosin (H&E). Then the pathological changes of heart tissue were detected using IncuCyte™ S3 ZOOM Cell Imaging System (Essen BioScience, Ann Arbor, MI, USA).
The immunohistochemical staining of NLRP3, ASC, and caspase-1 tissue sections was carried out according to the kit instructions. Briefly, the tissue sections were dewaxed and hydrated. Their antigen was retrieved, and the endogenous peroxidase was blocked. The sections were treated with goat serum and then incubated with NLRP3, ASC, and caspase-1 antibodies overnight. The slides were stained using the DAB kit, restained with hematoxylin, and observed using Aperio S2 Leica Biosystem microscope (Leica, Wetzlar, Germany). Quantification of all data was performed with ImageJ software.
Determination of Myocardial Apoptosis
Terminal deoxynucleotidyl transferase-mediated dUTP nick end labeling (TUNEL) (Beyotime, Shanghai, China) was used to detect apoptosis in tissue sections in accordance with the reagent instructions. Briefly, the frozen sections were incubated with 1% Triton X-100 for 15 min at room temperature. The sections were incubated in proteinase K working fluid for 30 min, washed with PBS. TUNEL detection solution was added to the sample and incubated in the dark at 37° for 60 min. The sections were washed with PBS three times for 5 min each and incubated with 4′,6-diamidino-2-phenylindole (DAPI) to identify nuclei. Finally, antifading mounting medium was added to prevent fluorescence quenching. The images were acquired by Tissue Gnostics AX10 analysis system (Vienna, Austria).
Western Blot Analysis
Heart tissues were lysed in radioimmunoprecipitation assay (RIPA) buffer (Beyotime, Shanghai, China) containing protease and phosphatase inhibitors. The protein concentrations were determined using the BCA assay (CWBiotech, Beijing, China); 40 μg of total proteins were loaded per lane, separated using 10% sodium dodecyl sulfate (SDS-PAGE), and blotted onto nitrocellulose membranes. The membrane was incubated overnight with the following primary antibodies: AMPK, p-AMPK, Atg5 and BECN1 (1:1,000, Cell Signaling Technology, USA), Bcl-2, Bax, caspase-3 and β-actin (1:1,000, Proteintech, China), IL-1β, mTOR, and p-mTOR (1:1,000, Abcam, UK), NLRP3, ASC and caspase-1 (1:1,000, Abclonal, China). The membranes were washed and incubated with the corresponding secondary antibodies. Finally, the bands were visualized using an ECL Kit (CW0049, CWBIO, Beijing, China).
Statistical Analysis
Data are presented as the mean ± SEM. Statistical analyses were performed using GraphPad Prism 5.0., and one-way ANOVA followed by Tukey’s post-hoc test was used for multiple comparisons. The statistical significance was set at P < 0.05.
Results
HSYA Alleviates Myocardial Injury Caused by Myocardial Ischemia/Reperfusion in Sprague-Dawley Rats
To detect the effect of HSYA on myocardial infarction area, we performed TTC staining on cardiac tissues. As shown in Figure 1A, the infarcted areas were stained white and the uninfarcted areas were stained red. The observation showed that there was no infarction in the myocardial tissue of sham group, while significant infarction occurred in the myocardial tissue of I/R group. The areas of myocardial infarction in the HSYA groups were significantly reduced in a dose-dependent manner compared with the I/R group, and that 16 mg/kg HSYA was the most effective dose against MI/R injury, comparable to DTZ (Figures 1A, B).
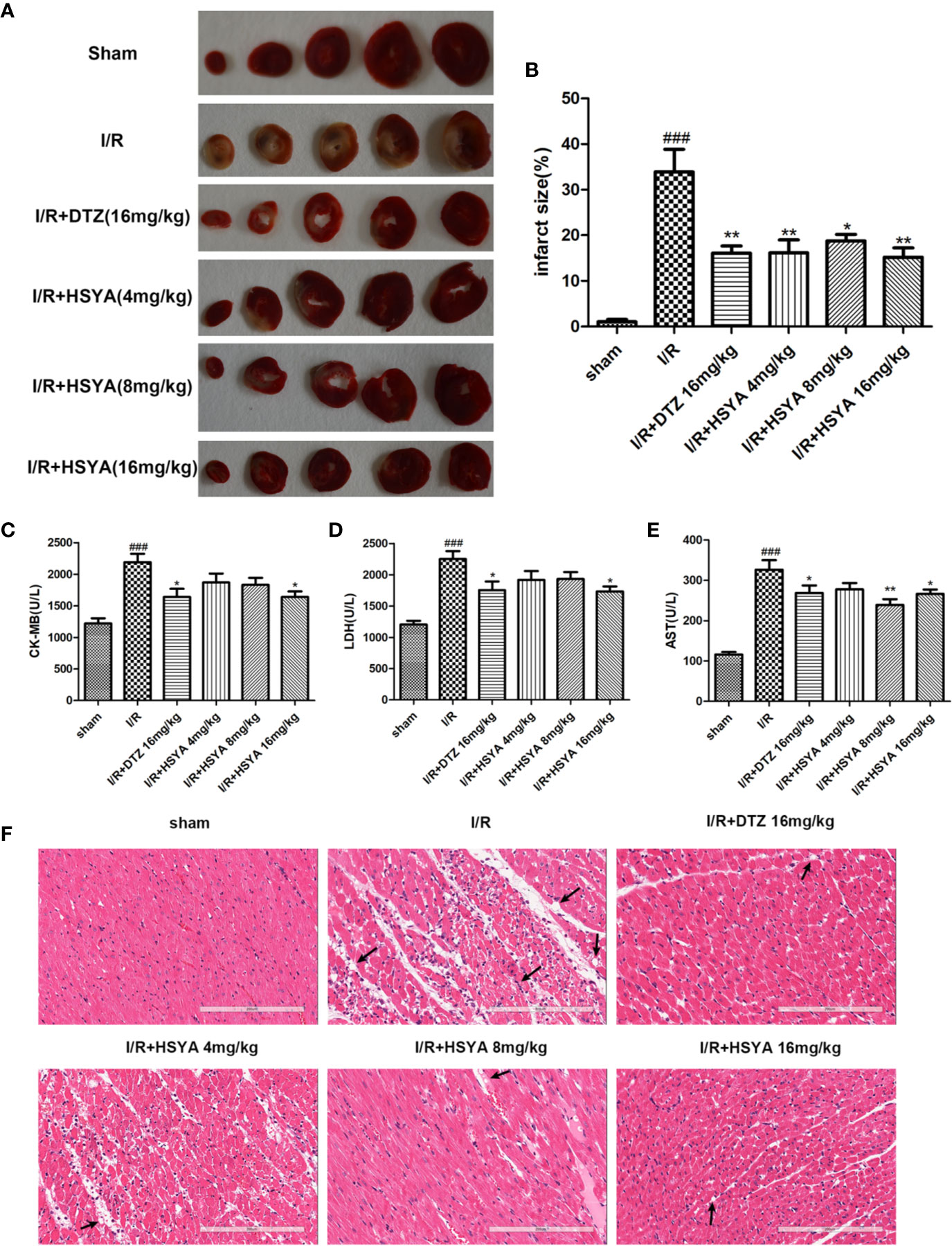
Figure 1 Hydroxysafflor yellow A (HSYA) alleviates I/R induced cardiac impairment. (A, B) The representative images and analysis results of triphenyl tetrazolium chloride (TTC) staining (n=4 per group). (C–E) Effects of HSYA on serum creatine kinase-MB (CK-MB), aspartate transaminase (AST), and lactic dehydrogenase (LDH) levels in the rats subjected to ischemia/reperfusion (I/R) injury (n=10 per group). (F) The representative images of H&E staining (Scale bar, 200 μm, n=4 per group). Data are presented as means ± SEM. ###P < 0.001 vs. the sham group, *P < 0.05, **P < 0.01 vs. the I/R group.
The elevated levels of CK-MB, LDH, and AST in the blood can reflect the degree of myocardial tissue injury after MI/R. As shown in Figures 1C–E, the serum levels of CK-MB, LDH, and AST were obviously higher in the I/R group compared with sham group, which was decreased after HSYA and DTZ treatment.
Myocardial histomorphology was assessed by HE staining, and the results were shown in Figure 1F. The sham group exhibited normal structure without lesions, edema, or neutrophils. In contrast, obvious changes, such as myocardial structure disorder, neutrophils infiltration, interstitial edema, cardiomyocyte necrosis, and nuclear dissolution, were observed in the I/R group. After treatment with HSYA (4, 8, and 16 mg/kg) or DTZ, the structural disorder of cardiac muscle fibers improved and the arrangement of cell nuclei was orderly. Moreover, the numbers of infiltrated neutrophils and necrosis cells in HSYA and DTZ-treated groups were less compared with those in the I/R group. Those indicate that HSYA reduces the morphological changes of myocardial tissue and exerts protective effects after I/R injury.
Hydroxysafflor Yellow A Alleviates Myocardial Apoptosis
Accumulating evidence indicates that activation of cardiac apoptosis plays an important role in the pathophysiology of reperfusion injury (Teringova and Tousek, 2017). Apoptosis of cardiomyocytes was detected by TUNEL immunofluorescence staining. No significant myocardial apoptosis was observed in the sham group, but apoptosis cells significantly increased in I/R group compared with that in the sham group. HSYA dose-dependently reduced the number of TUNEL-positive cells, among the doses administered, 16 mg/kg HSYA was the most effective in reducing apoptosis (Figures 2A, B). The apoptosis-related proteins including cleaved caspase-3, Bax, and Bcl-2, were measured. The results showed that HSYA could inhibit the activation of caspase-3, reduce the expression of Bax and increase the expression of Bcl-2 (Figures 2C–F). Taken together, HSYA attenuates cardiac apoptosis in the myocardium of rats after I/R injury.
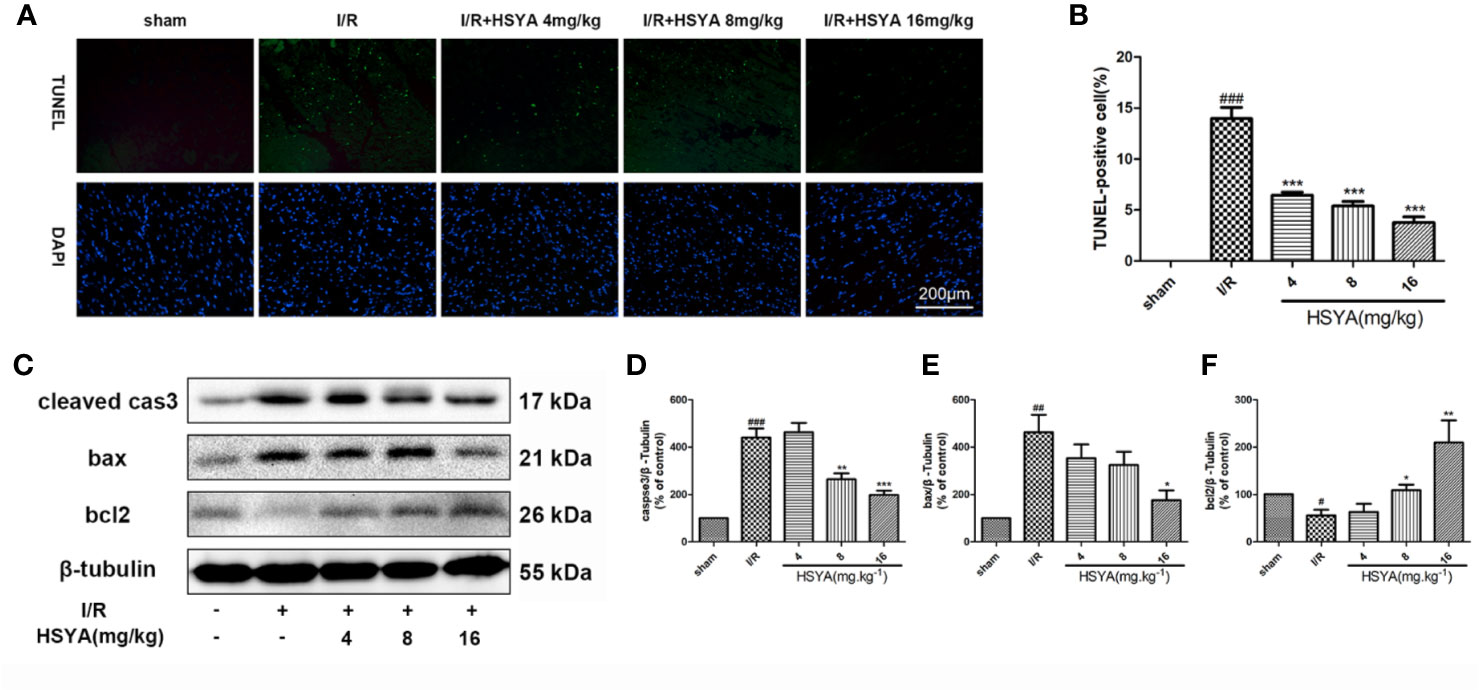
Figure 2 Hydroxysafflor yellow A (HSYA) reduces myocardial apoptosis. (A, B) Representative images and analysis results of terminal deoxynucleotidyl transferase-mediated dUTP nick end labeling (TUNEL) staining. Scale bar, 200 μm. (C–F) Representative immunoblots and quantified analysis of cleaved caspase-3, Bax, and Bcl-2 expression. Data are presented as means ± SEM (n = 3). #P < 0.05, ##P < 0.01, ###P < 0.001 vs. the sham group, *P < 0.05, **P < 0.01, ***P < 0.001 vs. the ischemia/reperfusion (I/R) group.
Hydroxysafflor Yellow A Inhibits the Secretion of Inflammatory Factors
Serum concentrations of inflammatory cytokines were determined by ELISA kits. As shown in Figures 3A–C, the serum levels of TNF-α, IL-1β, and IL-18 in the I/R group were significantly higher than those in the sham group. All HSYA groups ameliorated the excessive production of TNF-α, IL-1β, and IL-18 in serum induced by MI/R injury, indicating that HSYA reduces the inflammatory response after MI/R injury in rats.
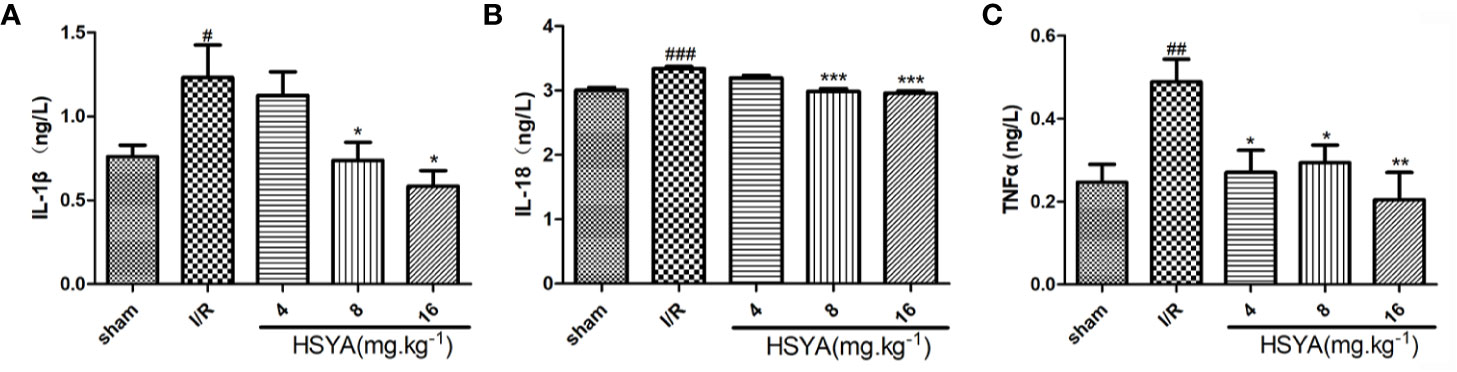
Figure 3 Hydroxysafflor yellow A (HSYA) inhibits the secretion of inflammatory factors. (A) Serum TNF-α level was analyzed by ELISA 24 h post-reperfusion (n=6 per group). (B) Serum interleukin (IL)-1β level was analyzed by ELISA 24 h post-reperfusion (n=6 per group). (C) Serum IL-18 level was analyzed by ELISA 24 h post-reperfusion (n=6 per group). Data are presented as means ± SEM. #P < 0.05, ##P < 0.01, ###P < 0.001 vs. the sham group, *P <0.05, **P < 0.01, ***P < 0.001 vs. the ischemia/reperfusion (I/R) group.
Hydroxysafflor Yellow A Inhibits the Expression of Inflammasome
More studies have shown that the inflammatory response initiated by NLRP3 inflammasomes is involved in the pathophysiological process of MI/R (Toldo et al., 2018; Toldo et al., 2019; Zahid et al., 2019). To investigate the effects of HSYA on inflammasome, we examined the expression of NLRP3, ASC, and caspase-1 by immunohistochemistry. As shown in Figures 4A–F, NLRP3, ASC, and caspase-1 levels were increased in the I/R group compared with the sham group, whereas HSYA treatment decreased their levels induced by MI/R. Consistently, the expression of NLRP3, ASC, and caspase-1 proteins was detected by Western blot. Compared with the I/R group, HSYA significantly inhibited the expression of NLRP3, activation of caspase-1, and expression of IL-1β in a dose-dependent manner (Figures 4G–J).
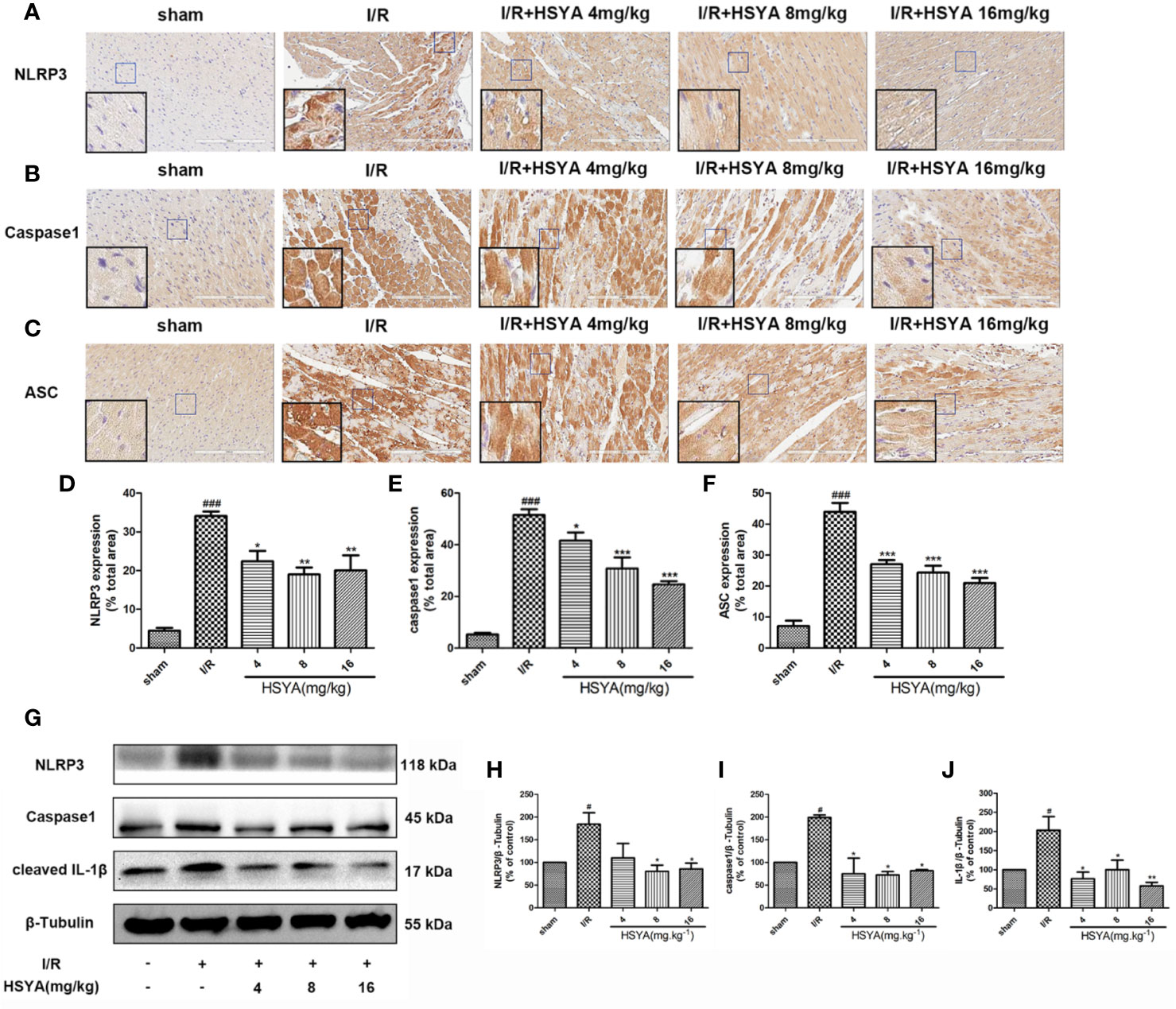
Figure 4 Hydroxysafflor yellow A (HSYA) inhibits the expression of NLRP3 inflammasome. (A–C) Immunohistochemical staining measuring NLRP3, ASC, and caspase-1 expressions in heart tissues of rats. (D–F) Quantification of NLRP3, ASC, and caspase-1 staining. (G) Western blot showing the protein expression levels of NLRP3, caspase-1, and cleaved interleukin (IL)-1β. (H–J) The ratios of NLRP3, caspase-1, and cleaved IL-1β and β-tubulin were quantified by densitometry based on immunoblot images. Data are presented as means ± SEM. #P < 0.05, ###P < 0.01 vs. the sham group, *P < 0.05, **P < 0.01, ***P < 0.001 vs. the ischemia/reperfusion (I/R) group.
Hydroxysafflor Yellow A Activates Autophagy After Myocardial Ischemia/Reperfusion in Sprague-Dawley Rats
Studies have shown that autophagy is an important process in ischemic heart disease, and autophagy flux is partially impaired and autophagosome clearance is significantly inhibited during reperfusion (Aghaei et al., 2019; Yang M. et al., 2019; Wang Y. et al., 2019). The conversion of LC3 I to LC3 II form is recognized as an indicator of autophagy activation. BECN1 is up-regulated and P62 is down-regulated in autophagy, which have important functions in autophagy regulation and are commonly used markers for autophagy detection. The expression of autophagy related proteins in the myocardial tissues was detected by western blot. As shown in Figures 5A–E, compared with the sham group, the LC3-II/LC3-I ratio and BECN1 and Atg5 expression in the I/R group significantly decreased, while the expression of P62 significantly increased in the I/R group, indicating that autophagy decreases during MI/R. HSYA reversed these changes and increased autophagy during reperfusion.
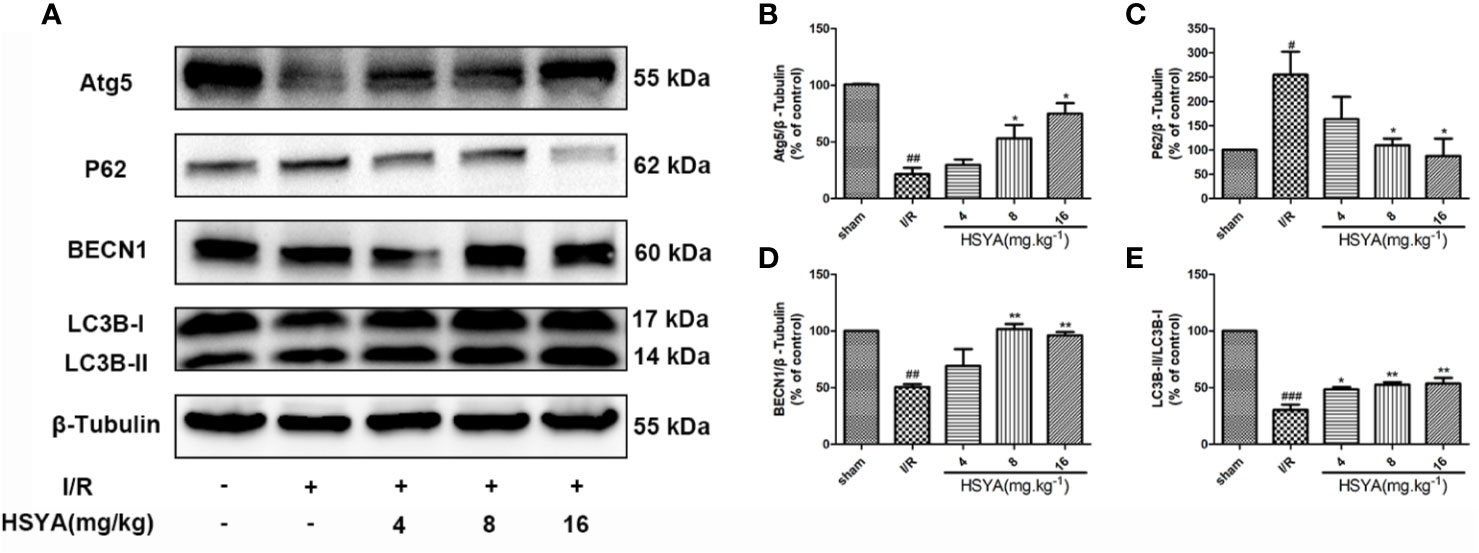
Figure 5 Hydroxysafflor yellow A (HSYA) induces autophagy in the myocardium. (A) Myocardial Atg5, P62, BECN1, and LC3B expressions were assayed by western blot analysis. (B–E) Quantification of protein expression. Data are presented as means ± SEM. #P < 0.05, ##P < 0.01, ###P < 0.001 vs. the sham group, *P < 0.05, **P < 0.01 vs. the ischemia/reperfusion (I/R) group.
Hydroxysafflor Yellow A Regulates AMPK/mTOR Signaling After Myocardial Ischemia/Reperfusion in Sprague–Dawley Rats
The AMPK/mTOR signaling is the key element for autophagy regulation and cardiac survival (Tamargo-Gómez and Mariño, 2018; Dong et al., 2019). During MI/R, the AMPK/mTOR signaling pathway modulates mitochondrial function, contractile function, autophagy, and apoptosis (Dake and Lawrence, 2015; Shi et al., 2018; Huang et al., 2018). To explore the effect of HSYA on the AMPK and mTOR proteins during MI/R in rats, we examined the levels of these proteins by Western blot. As shown in Figures 6A–C, compared with the sham group, the phosphorylation level of mTOR was significantly increased and AMPK was obviously reduced in the I/R group, whereas HSYA inhibited the phosphorylation of mTOR and increased the phosphorylation level of AMPK.
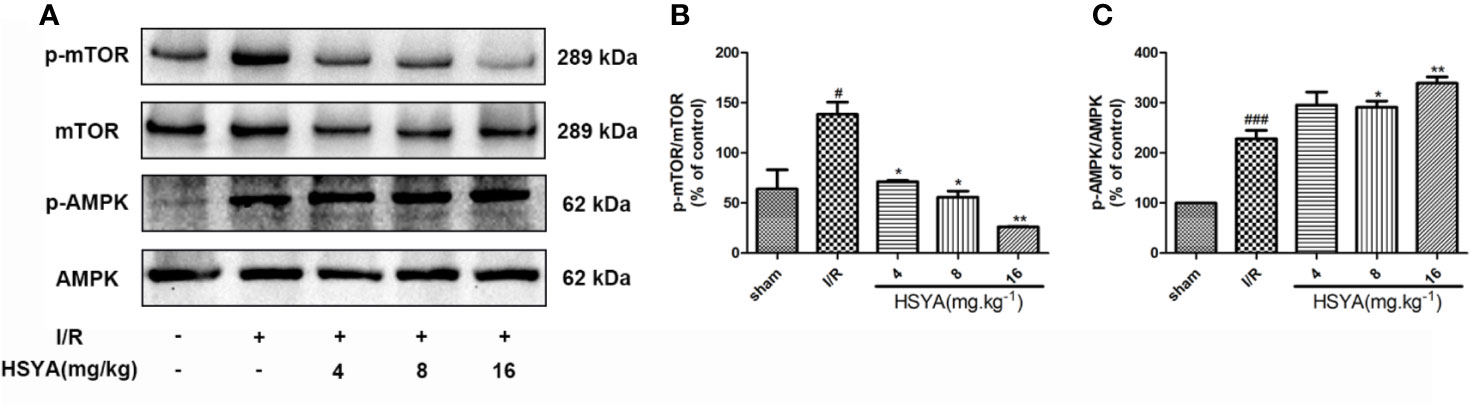
Figure 6 Hydroxysafflor yellow A (HSYA) attenuates myocardial ischemia/reperfusion injury by regulating the AMPK/mTOR pathway. (A) Protein expression levels of p-AMPK, AMPK, p-mTOR, and mTOR in heart tissues were assayed by western blot analysis. (B, C) Quantification of protein expression. Data are presented as means ± SEM. #P < 0.05, ###P < 0.001 vs. the sham group, *P < 0.05, **P < 0.01 vs. the ischemia/reperfusion (I/R) group.
Discussion
This study assesses the protective effect of HSYA on MI/R injury. Our study indicated that HSYA significantly reduces MI/R injury, inhibits myocardial inflammatory response, and reduces myocardial apoptosis. Consistent with our previous results on the cardiomyocyte injury model induced by hypoxia/reoxygenation, the myocardial protective mechanism of HSYA in the rat MI/R model may involve the promotion of myocardial autophagy by regulating AMPK/mTOR signaling pathway, inhibition of the activation of NLRP3 inflammasome, and reduction of the expression of downstream inflammatory factors to protect the ischemic myocardium.
Although early reperfusion plays an important role in improving myocardial ischemia, the recovery of blood flow could cause further damage to the ischemic tissue, namely reperfusion injury, resulting in the increase of myocardial infarction area. Many heart protection strategies have been tested on experimental animals over the past few decades, but clinical results have been disappointing. Moreover, no consensus on treatment is available (Hausenloy and Yellon, 2016). Therefore, it is necessary to further explore new therapeutic strategies to alleviate MI/R injury. HSYA has been widely used in the treatment of cerebrovascular and cardiovascular diseases. We discussed the protective effect of HSYA on MI/R injury in this study. The areas of myocardial infarction in the HSYA groups were significantly reduced in a dose-dependent manner compared with the I/R group. The elevated levels of CK-MB, LDH, and AST in the blood can reflect the degree of myocardial tissue injury after MI/R (Zhang et al., 2017). Our data showed that the serum levels of CK-MB, LDH, and AST are obviously higher in the I/R group than in the sham group, whereas HSYA inhibits these levels in a dose-dependent manner. Combined with the pathologic findings of the heart by HE staining, these results confirm the protective role of HSYA in the MI/R challenge.
Activation of myocardial apoptosis is a key feature of the pathology of MI/R injury, leading to myocardial cell loss and the subsequent inflammatory cell infiltration and inflammatory response (Xia et al., 2016; Teringova and Tousek, 2017; Toldo et al., 2018; Soares et al., 2019). It is well known that Bcl-2 and Bax are important proteins in cell apoptosis. In addition, caspase-3 is a key protein in the apoptotic pathway and plays a role when processed into cleaved caspase-3 (Zhou et al., 2019). In the present study, our results confirmed that HSYA can down-regulate the expression of pro-apoptosis proteins including Bax and cleaved caspase-3 and up-regulate the expression of anti-apoptosis protein Bcl-2 in MI/R injury. Meanwhile, TUNEL staining indicated that HSYA decreases I/R-induced apoptosis of cardiomyocytes in rats.
Inflammation is a major cause of MI/R injury (Ong et al., 2018). Recent studies have indicated that NLRP3 inflammasome plays a critical role in the development of heart inflammation (Shao et al., 2015; Liu et al., 2018). When activated, NLRP3 and ASC form inflammasome complexes that control the activation of caspase-1 (Sun et al., 2017; Zahid et al., 2019). Subsequently, NLRP3 processes the secretion of inflammatory cytokines, including IL‐1β and IL‐18 (Deretic and Levine, 2018). Therefore, inhibition of NLRP3 activation can inhibit the secretion of inflammatory factors, thereby reducing myocardial injury caused by I/R (Xu et al., 2020; Wu et al., 2020). In view of the important role of NLRP3 in MI/R injury, we examined the effect of HSYA on NLRP3 inflammasomes and the secretion of inflammatory factors. Our experimental results suggested that HSYA decreases the release of inflammatory cytokines IL‐1β and IL‐18, and effectively downregulates the expression of NLRP3, ASC, and caspase-1 in I/R-induced rats.
Autophagy regulation is believed to be related to cardiovascular disease, and plays an important role in MI/R. It has been shown that moderate autophagy upregulation can reduce the degree of myocardial ischemia injury and cardiac dysfunction (Aghaei et al., 2019). Moreover, studies have shown that autophagy is closely related to immune cellular response and inflammatory pathways (Castillo et al., 2012; Sarah et al., 2013; Yu M. et al., 2018). Increasing evidence indicates that autophagy activation inversely regulates the activation of NLRP3 inflammasome and the secretion of inflammatory cytokines (Nakahira et al., 2011; Harris et al., 2017; Liu et al., 2018). Although HSYA has been shown to inhibit NLRP3 inflammasome, it remains to be demonstrated whether autophagy is involved in HSYA heart protection in vivo. The current study’s results revealed that HSYA promoted autophagy in cardiomyocytes, showing that it up-regulated autophagy protein expression of BECN1, Atg5, and LC3II and down-regulated expression of P62.
The pathogenesis of MI/R injury is complicated and involves multiple mechanism. Apoptosis, autophagy, and inflammation interact to influence the pathological process of MI/R (Dominic et al., 2019; Dong et al., 2019). Excessive apoptosis and inflammation aggravate MI/R injury (Dong et al., 2019). In contrast, moderate autophagy protects the myocardium against I/R injury (Aghaei et al., 2019). Inhibition of autophagy has been shown to promote inflammation via inflammasome activation (Liu et al., 2018). In addition, apoptosis and autophagy have a complex interplay and interact with each other in MI/R. Some pathways such as mTOR and AMPK induce the interplay between apoptosis and autophagy (Huang et al., 2018; Dong et al., 2019). Furthermore, inflammatory response aggravates myocardial apoptosis. Studies have shown that cardiomyocyte-specific transgenic overexpression of procaspase-1 was sufficient to activate caspase-3 and exacerbate apoptosis and infarct size in response to I/R (Faisal et al., 2005). Therefore, drug targeting multiple mechanisms may be a promising therapy for MI/R. In this study, we demonstrated HSYA protected against MI/R through multiple pathways including promoting myocardial autophagy and inhibiting the inflammation and apoptosis.
To further investigate the underlying mechanisms of HSYA cardiac protection, we examined the role of HSYA in the AMPK-mTOR pathway which is an important pathway for regulating autophagy and NLRP3 inflammasome. The mTOR pathway is a well-known negative regulator of autophagic activity that has been established to regulate cell growth, proliferation, and metabolism (Galluzzi et al., 2017; Rabanal-Ruiz et al., 2017; Shi et al., 2018). Furthermore, mTOR has an accelerating effect on the metabolic inflammation response (Moon et al., 2015). Consistent with previous researchers, our data indicated that the expression of mTOR is enhanced in the MI/R rats, but downregulated by HSYA. AMPK senses cellular energy status to maintain energy homeostasis. Evidence supports the role for AMPK in autophagy induction in response to various cellular stresses (Alers et al., 2011; Kim et al., 2011; Tamargo-Gómez and Mariño, 2018; Wang Y. et al., 2019). Notably, AMPK activation attenuates NLRP3 inflammasome upregulation in some pathological processes, such as diabetes, pain, ischemic stroke, and endoplasmic reticulum stress (Yang F. et al., 2019; Qing et al., 2019). In our present study, HSYA increased the phosphorylation level of AMPK. Moreover, AMPK is known as the upstream of mTOR which play an important role in the regulation of inflammation and autophagy. However, autophagy is a complex dynamic process, and multiple signaling pathways are considered to trigger autophagy in MI/R, including Beclin-1/class III phosphatidylinositol-3 kinase (PI3K), AMPK/mTOR, and PI-3K/Akt/mTOR pathways (Wang et al., 2015; Lin et al., 2018). Whether HSYA regulates autophagy through other signaling pathways requires further experimental investigation.
In summary, our present study reveals the underlying mechanism of HSYA against MI/R injury by promoting myocardial autophagy and inhibiting the activation of NLRP3 inflammasome through regulation of the AMPK/mTOR signaling pathway.
Data Availability Statement
The raw data supporting the conclusions of this article will be made available by the authors, without undue reservation, to any qualified researcher.
Ethics Statement
The animal study was reviewed and approved by the Institutional Animal Care and Use Committee (IACUC) at the Chinese Academy of Medical Sciences and Peking Union Medical College.
Author Contributions
JY, GS, and XS conceived and designed the experiments. JY, SL, MW, WG, HL, and YQ performed the experiments. JF and QZ collected and analyzed the data. SL wrote original draft. BZ, GS, and XS revised the manuscript. BZ, GS, and XS supervised manuscripts. XS validated the manuscript, wrote review, and edited.
Funding
This work was supported by National Natural Sciences Foundation of China (No. 81673817), and the Natural Sciences Foundation of Beijing (No. 7172191).
Conflict of Interest
The authors declare that the research was conducted in the absence of any commercial or financial relationships that could be construed as a potential conflict of interest.
References
Aghaei, M., Motallebnezhad, M., Ghorghanlu, S., Jabbari, A., Enayati, A., Rajaei, M., et al. (2019). Targeting autophagy in cardiac ischemia/reperfusion injury: A novel therapeutic strategy. J. Cell. Physiol. 234, 16768–16778. doi: 10.1002/jcp.28345
Alers, S., Loffler, A. S., Wesselborg, S., Stork, B. (2011). Role of AMPK-mTOR-Ulk1/2 in the Regulation of Autophagy: Cross Talk, Shortcuts, and Feedbacks. Mol. Cell. Biol. 32, 2–11. doi: 10.1128/MCB.06159-11
Bravo-San Pedro, J. M., Kroemer, G., Galluzzi, L. (2017). Autophagy and Mitophagy in Cardiovascular Disease. Circ. Res. 120, 1812–1824. doi: 10.1161/CIRCRESAHA.117.311082
Castillo, E. F., Dekonenko, A., Arko-Mensah, J., Mandell, M. A., Dupont, N., Jiang, S., et al. (2012). Autophagy protects against active tuberculosis by suppressing bacterial burden and inflammation. Proc. Natl. Acad. Sci. 109, E3168–E3176. doi: 10.1073/pnas.1210500109
Chen, C., Chen, W., Nong, Z., Ma, Y., Qiu, S., Wu, G. (2016). Cardioprotective effects of combined therapy with hyperbaric oxygen and diltiazem pretreatment on myocardial ischemia−reperfusion injury in rats. Cell. Physiol. Biochem. 38, 2015−2029. doi: 10.1159/000445561
Dake, Q., Lawrence, H. Y. (2015). AMPK: energy sensor and survival mechanism in the ischemic heart. Trends Endocrinol. Metab. 26, 422–429. doi: 10.1016/j.tem.2015.05.010
De Biase, D., Piegari, G., Prisco, F., Cimmino, I., Pirozzi, C., Mattace Raso, G., et al. (2020). Autophagy and NLRP3 inflammasome crosstalk in neuroinflammation in aged bovine brains. J. Cell. Physiol. 235, 5394–5403. doi: 10.1002/jcp.29426
Deretic, V., Levine, B. (2018). Autophagy balances inflammation in innate immunity. Autophagy 14, 243–251. doi: 10.1080/15548627.2017.1402992
Dominic, P. D. R., Dulguun, A., Andreas, L., Liu, Q., Kitsis, R. N. (2019). Fundamental Mechanisms of Regulated Cell Death and Implications for Heart Disease. Physiol. Rev. 99, 1765–1817. doi: 10.1152/physrev.00022.2018
Dong, F., Xue, C., Wang, Y., Peng, Y., Zhang, Y., Jin, M., et al. (2017). Hydroxysafflor yellow A attenuates the expression of inflammatory cytokines in acute soft tissue injury. Sci. Rep. 7:40584. doi: 10.1038/srep40584
Dong, Y., Chen, H., Gao, J., Liu, Y., Li, J., Wang, J. (2019). Molecular machinery and interplay of apoptosis and autophagy in coronary heart disease. J. Mol. Cell Cardiol. 136, 27–41. doi: 10.1016/j.yjmcc.2019.09.001
Faisal, M. S., Harvey, S. H., Amy, O., Yiru, G., Jesus, G. V., Roy, A. L., et al. (2005). Proapoptotic Effects of Caspase-1/Interleukin-Converting Enzyme Dominate in Myocardial Ischemia. Circ. Res. 96, 1103–1109. doi: 10.1161/01.RES.0000166925.45995.ed
Galluzzi, L., Bravo-San Pedro, J. M., Levine, B., Green, D. R., Kroemer, G. (2017). Pharmacological modulation of autophagy: therapeutic potential and persisting obstacles. Nat. Rev. Drug Discovery 16, 487–511. doi: 10.1038/nrd.2017.22
Grant, W. R., Jeffrey, E. R., Christopher, P. C. (2017). Acute myocardial infarction. Lancet 389 (10065), 197–210. doi: 10.1016/S0140-6736(16)30677-8
Han, D., Wei, J., Zhang, R., Ma, W., Shen, C., Feng, Y., et al. (2016). Hydroxysafflor yellow A alleviates myocardial ischemia/reperfusion in hyperlipidemic animals through the suppression of TLR4 signaling. Sci. Rep. 6, 35319. doi: 10.1038/srep35319
Harris, J., Lang, T., Thomas, J. P. W., Sukkar, M. B., Nabar, N. R., Kehrl, J. H. (2017). Autophagy and inflammasomes. Mol. Immunol. 86, 10–15. doi: 10.1016/j.molimm.2017.02.013
Hausenloy, D. J., Yellon, D. M. (2016). Ischaemic conditioning and reperfusion injury. Nat. Rev. Cardiol. 13, 193–209. doi: 10.1038/nrcardio.2016.5
Herzog, W. R., Vogel, R. A., Schlossberg, M. L., Edenbaum, L. R., Scott, H. J., Serebruany, V. L. (1997). Short−term low dose intracoronary diltiazem administered at the onset of reperfusion reduces myocardial infarct size. Int. J. Cardiol. 59, 21−27. doi: 10.1016/s0167-5273(96)02883-5
Huang, W., Wen, J., Lin, R., Wei, P., Huang, F. (2018). Effects of mTOR/NF-κ B signaling pathway and high thoracic epidural anesthesia on myocardial ischemia-reperfusion injury via autophagy in rats. J. Cell Physiol. 233, 6669–6678. doi: 10.1038/ncb2152
Kim, J., Kundu, M. M., Viollet, B., Guan, K. (2011). AMPK and mTOR regulate autophagy through direct phosphorylation of Ulk1. Nat. Cell Biol. 13, 132–141. doi: 10.1038/ncb2152
Lesnefsky, E. J., Chen, Q., Tandler, B., Hoppel, C. L. (2017). Mitochondrial Dysfunction and Myocardial Ischemia-Reperfusion: Implications for Novel Therapies. Annu. Rev. Pharmacol. Toxicol. 57, 535–565. doi: 10.1146/annurev-pharmtox-010715-103335
Li, H., Liu, Y., Wen, M., Zhao, F., Zhao, Z., Liu, Y., et al. (2019). Hydroxysafflor yellow A (HSYA) alleviates apoptosis and autophagy of neural stem cells induced by heat stress via p38 MAPK/MK2/Hsp27-78 signaling pathway. Biomed. Pharmacother. 114, 108815. doi: 10.1016/j.biopha.2019.108815
Lin, X., Xiao, W., Xiao, L., Liu, M. (2018). Molecular mechanisms of autophagy in cardiac ischemia/reperfusion injury. Mol. Med. Rep. 18, 675–683. doi: 10.3892/mmr.2018.9028
Liu, P., Huang, G., Wei, T., Gao, J., Huang, C., Sun, M., et al. (2018). Sirtuin 3-induced macrophage autophagy in regulating NLRP3 inflammasome activation. Biochim. Biophys. Acta Mol. Basis Dis. 1864, 764–777. doi: 10.1016/j.bbadis.2017.12.027
Moon, J., Hisata, S., Park, M., DeNicola, G. M., Ryter, S. W., Nakahira, K., et al. (2015). mTORC1-Induced HK1-Dependent Glycolysis Regulates NLRP3 Inflammasome Activation. Cell. Rep. 12, 102–115. doi: 10.1016/j.celrep.2015.05.046
Mu, F., Duan, J., Bian, H., Zhai, X., Shang, P., Lin, R., et al. (2017). Metabonomic Strategy for the Evaluation of Chinese Medicine Salvia miltiorrhiza and Dalbergia odorifera Interfering with Myocardial Ischemia/Reperfusion Injury in Rats. Rejuv. Res. 20, 263–277. doi: 10.1089/rej.2016.1884
Nakahira, K., Haspel, J. A., Rathinam, V. A. K., Lee, S., Dolinay, T., Lam, H. C., et al. (2011). Autophagy proteins regulate innate immune responses by inhibiting the release of mitochondrial DNA mediated by the NALP3 inflammasome. Nat. Immunol. 12, 222–230. doi: 10.1038/ni.1980
Ong, S., Hernández-Reséndiz, S., Crespo-Avilan, G. E., Mukhametshina, R. T., Kwek, X., Cabrera-Fuentes, H. A., et al. (2018). Inflammation following acute myocardial infarction: Multiple players, dynamic roles, and novel therapeutic opportunities. Pharmacol. Therapeut. 186, 73–87. doi: 10.1016/j.pharmthera.2018.01.001
Peng, Y., Fu, X., Li, W., Geng, W., Xing, K., Ru, L., et al. (2014). Effect of intracoronary anisodamine and diltiazem administration during primary percutaneous coronary intervention in acute myocardial infarction. Coron. Artery Dis. 25, 645–652. doi: 10.1097/MCA.0000000000000167
Pizzetti, G., Mailhac, A., Volsi, L., Marco, F., Lu, C., Margonato, A., et al. (2001). Beneficial effects of diltiazem during myocardial reperfusion: A randomized trial in acute myocardial infarction. Ital. Heart. J. 2, 757−765.
Qing, L., Fu, J., Wu, P., Zhou, Z., Yu, F., Tang, J. (2019). Metformin induces the M2 macrophage polarization to accelerate the wound healing via regulating AMPK/mTOR/NLRP3 inflammasome singling pathway. Am. J. Transl. Res. 11, 655–668.
Rabanal-Ruiz, Y., Otten, E. G., Korolchuk, V. I. (2017). mTORC1 as the main gateway to autophagy. Essays. Biochem. 61, 565–584. doi: 10.1042/EBC20170027
Sarah, A. J., Kingston, H. G. M., James, H. (2013). Autophagy and inflammatory diseases. Immunol. Cell. Biol. 91, 250–258. doi: 10.1038/icb.2012.82
Shao, B., Xu, Z., Han, B., Su, D., Liu, C. (2015). NLRP3 inflammasome and its inhibitors: a review. Front. Pharmacol. 6, 262. doi: 10.3389/fphar.2015.00262
Shi, B., Ma, M., Zheng, Y., Pan, Y., Lin, X. (2018). mTOR and BECN1: Two key autophagy-related molecules and their roles in myocardial ischemia/reperfusion injury. J. Cell. Physiol. 234, 12562–12568. doi: 10.1002/jcp.28125
Soares, R. O. S., Losada, D. M., Jordani, M. C., Évora, P., Castro-e-Silva, O. (2019). Ischemia/Reperfusion Injury Revisited: An Overview of the Latest Pharmacological Strategies. Int. J. Mol. Sci. 20, 5034. doi: 10.3390/ijms20205034
Sun, Q., Fan, J., Billiar, T. R., Scott, M. J. (2017). Inflammasome and autophagy regulation - a two-way street. Mol. Med. 23, 188–195. doi: 10.2119/molmed.2017.00077
Sun, Y., Xu, D., Qin, Z., Wang, P., Hu, B., Yu, J., et al. (2018). Protective cerebrovascular effects of hydroxysafflor yellow A (HSYA) on ischemic stroke. Eur. J. Pharmacol. 818, 604–609. doi: 10.1016/j.ejphar.2017.11.033
Tamargo-Gómez, I., Mariño, G. (2018). AMPK: Regulation of Metabolic Dynamics in the Context of Autophagy. Int. J. Mol. Sci. 19, 3812. doi: 10.3390/ijms19123812
Teringova, E., Tousek, P. (2017). Apoptosis in ischemic heart disease. J. Transl. Med. 15, 87. doi: 10.1186/s12967-017-1191-y
Toldo, S., Mauro, A. G., Cutter, Z., Abbate, A. (2018). Inflammasome, pyroptosis, and cytokines in myocardial ischemia-reperfusion injury. Am. J. Physiol. Heart. Circ. Physiol. 315, H1553–H1568. doi: 10.1152/ajpheart.00158.2018
Toldo, S., Mauro, A. G., Cutter, Z., Van Tassell, B. W., Mezzaroma, E., Del Buono, M. G., et al. (2019). The NLRP3 Inflammasome Inhibitor, OLT1177 (Dapansutrile), Reduces Infarct Size and Preserves Contractile Function After Ischemia Reperfusion Injury in the Mouse. J. Cardiovasc. Pharm. 73, 215–222. doi: 10.1097/FJC.0000000000000658
Wang, L., Cheng, X., Huang, C., Huang, B., Liang, Q. (2015). Rapamycin Protects Cardiomyocytes Against anoxia/reoxygenation Injury by Inducing Autophagy Through the PI3k/Akt Pathway. J. Huazhong Univ. Sci. Technol. Med. Sci. 35, 10–15. doi: 10.1007/s11596-015-1381-x
Wang, X., Ma, Z., Fu, Z., Gao, S., Yang, L., Jin, Y., et al. (2016). Hydroxysafflor Yellow A Protects Neurons From Excitotoxic Death through Inhibition of NMDARs. ASN Neuro. 8, 166121962. doi: 10.1177/1759091416642345
Wang, R., Wang, M., Zhou, J., Ye, T., Xie, X., Ni, D., et al. (2019). Shuxuening injection protects against myocardial ischemia-reperfusion injury through reducing oxidative stress, inflammation and thrombosis. Ann. Transl. Med. 7, 562. doi: 10.21037/atm.2019.09.40
Wang, Y., Yang, Z., Zheng, G., Yu, L., Yin, Y., Mu, N., et al. (2019). Metformin promotes autophagy in ischemia/reperfusion myocardium via cytoplasmic AMPKα1 and nuclear AMPKα2 pathways. Life. Sci. 225, 64–71. doi: 10.1016/j.lfs.2019.04.002
Wu, Y., Zhang, Y., Zhang, J., Zhai, T., Hu, J., Luo, H., et al. (2020). Cathelicidin aggravates myocardial ischemia_reperfusion injury via activating TLR4 signaling and P2X7R_NLRP3 inflammasome. J. Mol. Cell. Cardiol. 139, 75–86. doi: 10.1016/j.yjmcc.2019.12.011
Xia, P., Liu, Y., Cheng, Z. (2016). Signaling Pathways in Cardiac Myocyte Apoptosis. Biomed. Res. Int. 2016, 1–22. doi: 10.1155/2016/9583268
Xu, H., Liu, T., Wang, W., Su, N., Yang, L., Yang, Z., et al. (2019). Proteomic Analysis of Hydroxysafflor Yellow A Against Cerebral Ischemia/Reperfusion Injury in Rats. Rejuv. Res. 22, 503–512. doi: 10.1089/rej.2018.2145
Xu, L. J., Chen, R. C., Ma, X. Y., Zhu, Y., Sun, G. B., Sun, X. B. (2020). Scutellarin protects against myocardial ischemia-reperfusion injury by suppressing NLRP3 inflammasome activation. Phytomedicine 68, 153169. doi: 10.1016/j.phymed.2020.153169
Yang, F., Qin, Y., Wang, Y., Meng, S., Xian, H., Che, H., et al. (2019). Metformin Inhibits the NLRP3 Inflammasome via AMPK/mTOR-dependent Effects in Diabetic Cardiomyopathy. Int. J. Biol. Sci. 15, 1010–1019. doi: 10.7150/ijbs.29680
Yang, M., Linn, B. S., Zhang, Y., Ren, J. (2019). Mitophagy and mitochondrial integrity in cardiac ischemia-reperfusion injury. BBA Mol. Basis Dis. 1865, 2293–2302. doi: 10.1016/j.bbadis.2019.05.007
Ye, J., Wang, M., Wang, R., Liu, H., Qi, Y., Fu, J., et al. (2020). Hydroxysafflor yellow A inhibits hypoxia/reoxygenation-induced cardiomyocyte injury via regulating the AMPK/NLRP3 inflammasome pathway. Int. Immunopharmacol. 82, 106316. doi: 10.1016/j.intimp.2020.106316
Yu, L., Duan, Y., Zhao, Z., He, W., Xia, M., Zhang, Q., et al. (2018). Hydroxysafflor Yellow A (HSYA) Improves Learning and Memory in Cerebral Ischemia Reperfusion-Injured Rats via Recovering Synaptic Plasticity in the Hippocampus. Front. Cell. Neurosci. 12, 371. doi: 10.3389/fncel.2018.00371
Yu, M., II, Seungmin, H., Ken, C. (2018). Autophagy and Inflammation. Annu. Rev. Immunol. 36, 73–101. doi: 10.1146/annurev-immunol-042617-053253
Yu, L., Liu, Z., He, W., Chen, H., Lai, Z., Duan, Y., et al. (2020). Hydroxysafflor Yellow A Confers Neuroprotection from Focal Cerebral Ischemia by Modulating the Crosstalk Between JAK2/STAT3 and SOCS3 Signaling Pathways. Cell Mol. Neurobiol. doi: 10.1007/s10571-020-00812-7
Zahid, A., Li, B., Kombe, A. J. K., Jin, T., Tao, J. (2019). Pharmacological Inhibitors of the NLRP3 Inflammasome. Front. Immunol. 10, 2538. doi: 10.3389/fimmu.2019.02538
Zhang, X., Du, Q., Yang, Y., Wang, J., Dou, S., Liu, C., et al. (2017). The protective effect of Luteolin on myocardial ischemia/reperfusion (I/R) injury through TLR4/NF-κB/NLRP3 inflammasome pathway. Biomed. Pharmacother. 91, 1042–1052. doi: 10.1016/j.biopha.2017.05.033
Zheng, D., Liu, Z., Zhou, Y., Hou, N., Yan, W., Qin, Y., et al. (2020). Urolithin B, a Gut Microbiota Metabolite, Protects against Myocardial Ischemia/Reperfusion Injury via p62/Keap1/Nrf2 Signaling Pathway. Pharmacol. Res. 104655. doi: 10.1016/j.phrs.2020.104655
Zhou, D., Ding, T., Ni, B., Jing, Y., Liu, S. (2019). Hydroxysafflor Yellow A mitigated myocardial ischemia/reperfusion injury by inhibiting the activation of the JAK2/STAT1 pathway. Int. J. Mol. Med. 44, 405–416. doi: 10.3892/ijmm.2019.4230
Keywords: myocardial ischemia reperfusion injury, hydroxysafflor yellow A, NLRP3, autophagy, AMPK
Citation: Ye J, Lu S, Wang M, Ge W, Liu H, Qi Y, Fu J, Zhang Q, Zhang B, Sun G and Sun X (2020) Hydroxysafflor Yellow A Protects Against Myocardial Ischemia/Reperfusion Injury via Suppressing NLRP3 Inflammasome and Activating Autophagy. Front. Pharmacol. 11:1170. doi: 10.3389/fphar.2020.01170
Received: 01 June 2020; Accepted: 17 July 2020;
Published: 30 July 2020.
Edited by:
Ismail Laher, University of British Columbia, CanadaReviewed by:
Daniela Giuliani, University of Modena and Reggio Emilia, ItalyXiang Gao, Cornell University, United States
Shunchang Li, Chengdu Sport University, China
Tapas C. Nag, All India Institute of Medical Sciences, India
Copyright © 2020 Ye, Lu, Wang, Ge, Liu, Qi, Fu, Zhang, Zhang, Sun and Sun. This is an open-access article distributed under the terms of the Creative Commons Attribution License (CC BY). The use, distribution or reproduction in other forums is permitted, provided the original author(s) and the copyright owner(s) are credited and that the original publication in this journal is cited, in accordance with accepted academic practice. No use, distribution or reproduction is permitted which does not comply with these terms.
*Correspondence: Bengang Zhang, bgzhang@implad.ac.cn; Guibo Sun, sunguibo@126.com; Xiaobo Sun, sun_xiaobo163@163.com
†These authors have contributed equally to this work