- 1Naturalis Biodiversity Center, Endless Forms Group, Leiden, Netherlands
- 2Center for Plant Conservation, Bogor Botanic Garden, Indonesian Institute of Sciences (LIPI), Bogor, Indonesia
- 3Science and Technology Faculty, University of Applied Sciences Leiden, Leiden, Netherlands
- 4Lankester Botanical Garden, University of Costa Rica, Cartago, Costa Rica
- 5Institute of Environmental Sciences, Leiden University, Leiden, Netherlands
- 6Department of Econometrics and Operations Research, Vrije Universiteit Amsterdam, Amsterdam, Netherlands
- 7Hortus botanicus, Leiden University, Leiden, Netherlands
- 8Institute of Biology Leiden, Leiden University, Leiden, Netherlands
- 9Ecology, Evolution and Biodiversity Conservation, KU Leuven, Heverlee, Belgium
- 10Institute of Water and Wetland Research, Radboud University, Nijmegen, Netherlands
Necklace orchids (Coelogyninae, Epidendroideae) have been used in traditional medicine practices for centuries. Previous studies on a subset of unrelated orchid species utilized in these traditional practices revealed they possessed antimicrobial, anti-inflammatory, and anti-oxidant activity, providing experimental proof for their medicinal properties. To date however none of these species have been investigated ethno-botanically in a phylogenetic context. This study carried out comparative bioprospecting for a group of wild orchids using EBDCS (the Economic Botany Data Collection Standards) organ targeted and biological response methods. The traditional medicinal use of necklace orchids was recorded from books and journals published between 1984 and 2016. Two orchids, Coelogyne cristata and Coelogyne fimbriata, were selected, cultivated both indoors and outdoors, and the antimicrobial properties on extracts from their leaves and pseudobulbs tested against a selection of human pathogens. A molecular phylogeny of Coelogyninae based on nuclear ribosomal ITS and plastid matK DNA sequences obtained from 148 species was reconstructed with Maximum Likelihood (ML) using RAxML, Maximum Parsimony (MP) using PAUP and Bayesian Inference using MrBayes. Bioprospecting comparison of EBDCS and biological response was carried out using customized R scripts. Ethanolic extracts obtained from leaves of C. fimbriata inhibited growth of Bacillus cereus, Staphylococcus aureus, and Yersinia enterocolitica, confirming the antimicrobial properties of these extracts. Leaf extracts were found to have slightly stronger antimicrobial properties for plants cultivated outdoors than indoors. These differences were not found to be statistically significant though. Three hot nodes with high potency for antimicrobial activities were detected with the EBDCS organ targeted classification method, and eight hot nodes were detected with the biological response classification method. The biological response classification method is thus a more effective tool in finding hot nodes amongst clades of species with high medicinal potential.
Introduction
For millennia, products of nature have been an important source of traditional medicine (Cragg and Newman, 2013). Even today, between 70 and 95% of the world population in developing countries continues to use traditional medicines (Robinson and Zhang, 2011). Plant-based antibiotics form the basis of these traditional medicinal systems (Newman et al., 2000). There is an increasing interest in the study of these plant-based antibiotics as a source of novel antibiotics that human pathogens may not have developed resistance against, and because of the increasing extinction rate of wild plant species (Savoia, 2012; Cragg and Newman, 2013; Ernst et al., 2016).
To discover potential new plant species with antimicrobial properties, a time-efficient and systematic approach is needed. Bioprospecting is an approach combining phylogeny with ethnobotanical knowledge to identify potential sources of bioactive compounds. The underlying assumption is that phylogenies can predict the traditional medicinal use of natural products in a bioprospecting approach (Saslis-Lagoudakis et al., 2012; Leonti et al., 2013; Ernst et al., 2016). The hypothesis is that closely related species share similar biochemical pathways and that the same bioactive compounds are present in all descendants of a single ancestor rather than in species scattered over unrelated clades. This method has been used in different plant species (Douwes et al., 2008; Zhu et al., 2011; Saslis-Lagoudakis et al., 2012; Siqueira et al., 2012; Leonti et al., 2013) and animal groups (Smith and Wheeler, 2006). For bioprospecting, two different methods are mainly used. The first method is the Economic Botany Data Collection Standard (EBDCS) classification method. The EBDCS provides a system where cultural plant uses are described using standardized descriptors and terms, and attached to taxonomic data sets. This classification is based on the treatment of symptoms, i.e. a medicine against stomach pain (Cook, 1995). The other method is a classification based on the biological response, such as a medicine with antimicrobial effects (Ernst et al., 2016).
Pathogens cause an array of diseases in humans, and their identification is important in administering the correct treatment. (Washington, 1996). It is expected that bioprospecting based on biological responses will produce different results from the organ targeted EBDCS method, as biological responses are focused on a classification based on a single effect in the entire human body rather than a single organ (Ernst et al., 2016). A growing number of studies report on the bioprospecting of medicinal plants, including orchids (Beena, 2011; Purkayastha, 2016). We have not yet come across any study carried out on a group of wild orchids from a phylogenetic perspective.
The orchid family is historically well-known for its medicinal properties (Lawler, 1984; Singh and Singh, 2012). Medicinal orchids contain phytochemicals such as alkaloids, bibenzyl derivatives, flavonoids, phenanthrenes and terpenoids, which are present in leaves, roots, pseudobulbs (modified stem parts for water and nutrient storage), and flowers (Gutiérrez, 2010; Hsiao et al., 2011; Pant, 2014). Necklace orchids (Coelogyninae, Epidendroideae) comprise over 680 species, that are distributed throughout Southeast Asia (Pridgeon et al., 2005). Bletilla, Coelogyne, Dendrochilum, Otochilus, Pholidota, Pleione, and Thunia are examples of necklace orchid genera with documented medicinal properties (Singh and Duggal, 2009; Subedi et al., 2011; Pant and Raskoti, 2013; Teoh, 2016) (see Figure 1).
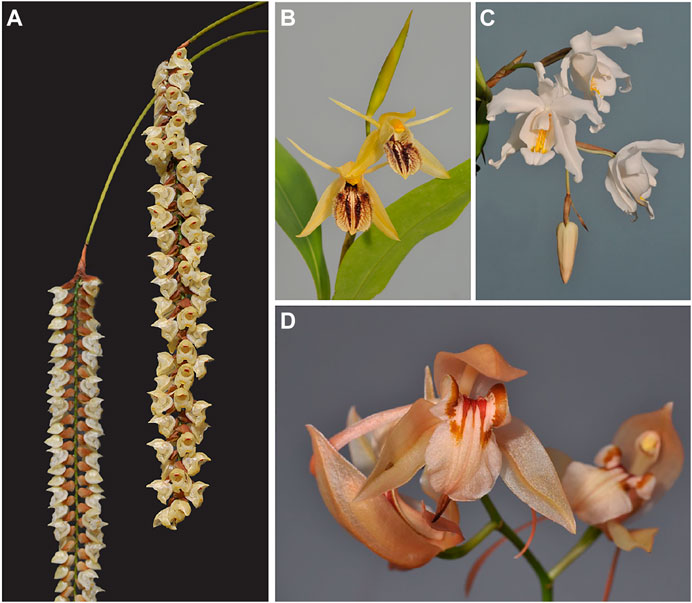
FIGURE 1. Examples of medicinally used necklace orchids investigated in this study. (A)Pholidota imbricata.(B)Coelogyne fimbriata. (C)Coelogyne cristata. (D)Coelogyne fuscescens. The Pholidota species depicted under A can only be cultivated in a humid greenhouse. The Coelogyne species depicted under B-D can be cultivated outdoors during the summer and early fall in temperate regions to stimulate the production of secondary compounds. Photographs by Rogier van Vugt.
In this study, we 1) compiled traditional medicinal uses of necklace orchids from the literature, 2) carried out bio-assays on six human pathogens with ethanol and hexane extracts of leaves and pseudobulbs from C. cristata and C. fimbriata plants grown both inside a glasshouse and outside to experimentally validate whether traditional growth methods impacted the orchid medicinal properties, and 3) investigated whether an organ-targeted EBDCS or biological response-based classification was most informative for predicting the biological activity of related species.
Materials and Methods
Medicinal Uses of Necklace Orchids Recorded in the Literature
Information on the medicinal use of different species of necklace orchids was compiled from scientific journals and books through September 2019 (Table 1). We included all data from publications that stated the local names, latin names and the traditional uses for the orchid species. We excluded the publications where only the local name and/or genus were given. All records were compiled into a list and coded according to the Economic Botany Data Collection Standard (EBDCS) as recommended by the Biodiversity Information Standards of the Taxonomic Databases Working Group (TDWG) (Cook, 1995). The medicinal properties of the orchid species were categorized into EBDCS level 2 characters and into biological response characters. We used the antimicrobial response character as defined in MedlinePlus with three different states: no response, possible response or unknown response. This definition assumes that a plant species should be categorized as no response when it is applied for anything other than antimicrobial treatments, such as bone fracture treatments. A possible response was scored when the disease dictionary of MedlinePlus dictated this. Reducing fever was for instance scored as a possible antimicrobial effect since fever is a biological response to infection. Finally, an ‘unknown response’ was given if no records of plant use were available. Medicinal properties of all Glomera species were categorized as unknown, as to the best of our knowledge no ethnobotanical information for this genus has been published.
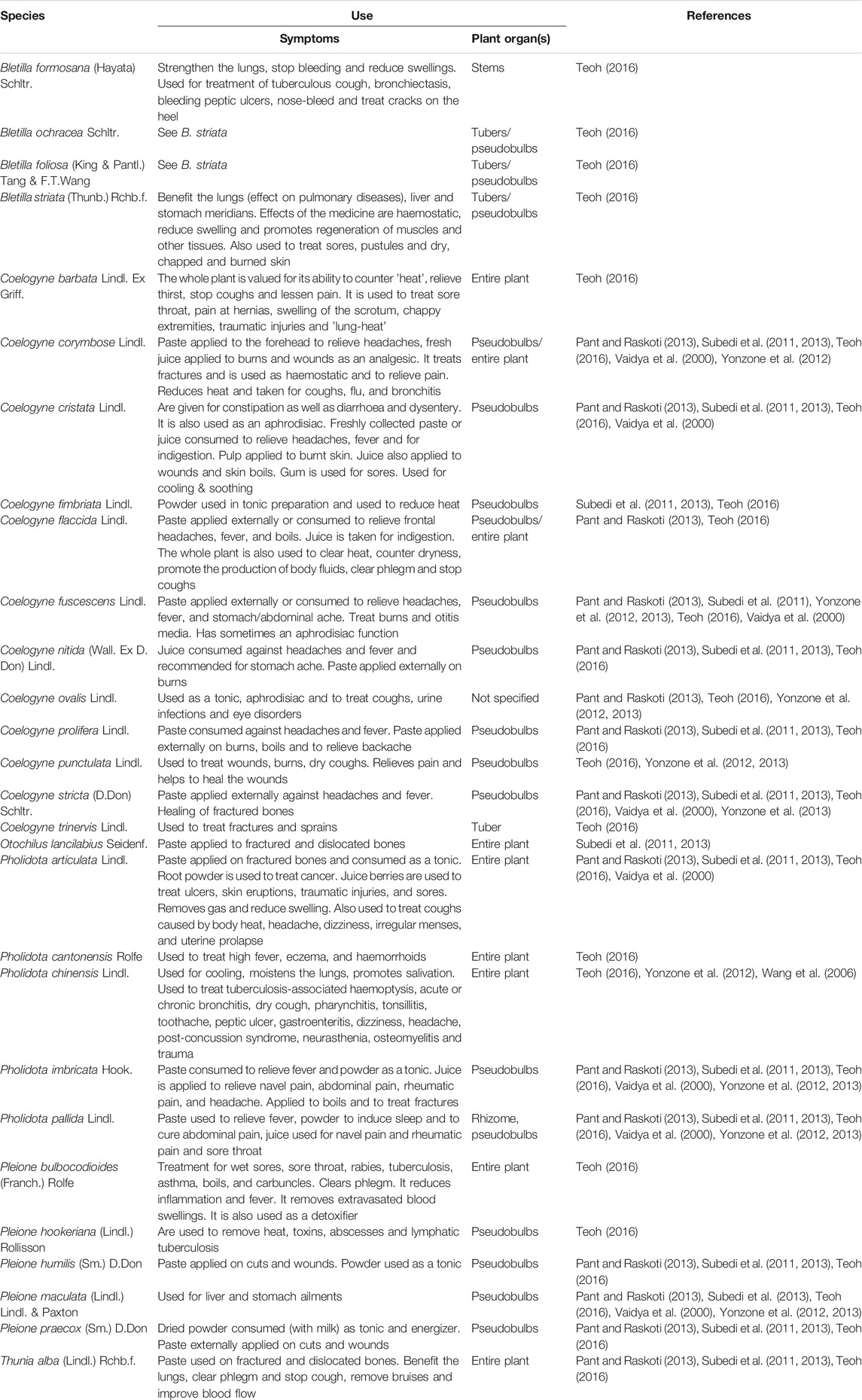
TABLE 1. Information on traditional medicinal use of necklace orchids (Coelogyninae) compiled from the literature.
Antimicrobial Activity
Plant Material
Fresh pseudobulbs and leaves of mature sterile plants of C. cristata and C. fimbriata (3–5 different individuals per species) grown in greenhouses were obtained from Orchideeën Wubben (Hollandsche Rading, Netherlands) and Claessen Orchideeën (Nederweert-Eind, Netherlands). The same species were subsequently grown outside where they were exposed to UV light and herbivorous snails and insects during a period of 5 months in the Hortus botanicus (Leiden, Netherlands). A second batch of fresh pseudobulbs and leaves was then harvested from these species. All leaves and pseudobulbs were sterilized and freeze-dried in a VirTis Benchtop Pro Freeze Dryer at −104°C until they reached a constant weight. The dried pseudobulbs and leaves were ground into a fine powder and about 1 g of the powder was extracted with 70% ethanol and 100% hexane in a vacuum speed extractor E-916 (Buchi, Switzerland) (40°C, 100 bar). The extracts were stored in a freezer (−20°C) before further use.
Bacterial Strains
The antimicrobial properties of extracts from pseudobulbs and leaves of C. cristata and C. fimbriata were evaluated with five bacterial strains that are common causes of human gastrointestinal tract infections and are resistant against a range of synthetic antibiotics (Mutsaers et al., 2001). Two strains of Gram-positive Staphylococcus aureus (ATCC 12600) and Bacillus cereus (ATCC 14579) and three strains of Gram-negative Escherichia coli (ATCC 10798), Klebsiella pneumoniae (ATCC BAA-3079), and Yersinia enterocolitica (ATCC 9610) bacteria were used for the experiments. The bacterial strains were provided by the University of Applied Sciences Leiden, Netherlands. All strains were cultured on Columbia Agar with 5% Sheep Blood (COL-S (BDTM)) overnight at aerobic conditions at 37°C (except for Y. enterocolitica, which was typically cultured at 30°C), followed by storage at 4°C for up to 1 week.
Antimicrobial Activity of Plants Extracts
A disk diffusion method was used to evaluate the antimicrobial activity for each plant extract. Each bacterial strain was streaked onto a plate, grown overnight, and used to inoculate Mueller-Hinton cation-adjusted agar broth 2 (Sigma-Aldrich). The cultures were incubated overnight under aerobic conditions at 37°C (except for Y. enterocolitica, which was grown at 30°C) on a rotary shaker (180 rpm) until a McFarland Standard of 0.5 (107 CFU/ml) was reached. The cultures were subsequently used to make a confluent growth on COL-S agar plates. Sterile filter paper disks with (10 mm diameter, Sigma-Aldrich) were loaded with the different plant extracts with a total content of 55 mg/ml. The disks were then evaporated by air at room temperature inside a laminar air flow hood for 20 min before they were placed onto the top of the inoculated plates. Sterile filter paper disks loaded with 7.5 µg of Levofloxacin (Sigma-Aldrich) were used as positive control, and sterile paper disks loaded with 5% DMSO (Sigma-Aldrich) were used as the negative control. All the samples were then incubated at 37°C (except for Y. enterocolitica at 30°C) for 24 h. All tests were performed in triplicate and the zones of inhibition were measured with an automatic Vernier caliper. The scale of the inhibitory effect was scored as follows: high (diameter zone ≥17 mm), intermediate (14 ≤ diameter zone <16 mm), low (diameter zone ≤13 mm) (CLSI, 2011).
Phylogenetic Reconstructions
Plant Sampling and DNA Extraction
Previously generated DNA sequences for necklace orchids (Gravendeel et al., 2001; Subedi et al., 2011; Sulistyo et al., 2015; Pedersen et al., 2020) were downloaded from NCBI GenBank (see Supplementary Table S2 for more details). In addition, new DNA sequences were generated from 77 specimens of the necklace orchid genus Glomera. From these, 14 specimens were collected in the field in Seram, Papua and Papua New Guinea (Indonesia). The first author also identified living orchid material from the Bogor Botanical Garden with identification keys and taxonomic descriptions from Schuiteman and de Vogel (2001), Wati et al. (2018) and the website of de Vogel et al. (2019). Additionally, a total of 42 specimens from the living orchid collection of the Hortus botanicus Leiden, Netherlands were analysed. Lastly, 21 dried herbarium specimens from the Herbarium Bogoriense, Indonesia and the herbarium of Naturalis Biodiversity Center, Leiden, Netherlands, were analysed (see Supplementary Table S2 for more details). Total genomic DNA was extracted from 50 mg of leaf tissue from herbarium or silica-gel dried material using the 2x CTAB (Cetyltrimethylammonium bromide) method of Doyle and Doyle (1987), or with the Qiagen DNeasy Plant mini kit (Qiagen) following the manufacturer’s protocol.
Amplification and Sanger Sequencing
The nuclear ribosomal ITS-5.8S-ITS2 (nrITS) region of silica-gel dried leaf material was amplified using primers 17SE (5′-ACGAATTCATGGTCCGGTGAAGTGTTC-3′) and 26SE (5′-TAGAATTCCCCGGTTCGCTCGCCGTTAC-3′) as described by Sulistyo et al. (2015). Subsequently, a M13 universal sequencing primer was added to the 5′ end of the forward (ACGAATTCATGGTCCGGTGAAGTGTTC) and reverse (TAGAATTCCCCGGTTCGCTCGCCGTTAC) primers to improve Sanger sequencing efficiency. Each PCR reaction was 25 µL and included the template DNA, CoralLoad PCR buffer (Qiagen), dNTPs, Taq DNA Polymerase (Qiagen), and both primers. All PCR reactions were done on a C1000 Touch Thermal Cycler (Bio-Rad) instrument. The thermal cycling protocol began with a 5 min initial denaturation at 96°C, followed by 35 amplification cycles, each with 30 s denaturation at 96°C, 30 s annealing at 50°C, and 1 min extension at 72°C, followed by a final 7 min final extension at 72 °C.
The nrITS region of herbarium preserved leaf material was amplified using primer p3 (5′-GACTCYCGGCAATGGATATCTCG-3′) and p4 (5′-CCGCTTATTGATATGCTTAAACTCRGC-3′) as described by Cheng et al. (2016) and primer efgF1 (5′-CGAGTCTTTGAACGCAAGTTGCG-3′) and efgR1 (5′-GGCCAACGAGACGATAACCC-3′) that were newly designed. Each PCR reaction consisted of 25 μL, containing the template DNA, 5x Phire PCR buffer (ThermoScientific), BSA, dNTPs, Phire Hot Start II DNA Polymerase (ThermoScientific), and both primers. The thermal cycling protocol began with a 1 min initial denaturation at 98°C, followed by 40 amplification cycles, each with 10 s denaturation at 98°C, 10 s annealing at 50°C, and 20 s extension at 72°C, followed by a 1 min final extension at 72 °C.
The matK region of silica dried silica-gel dried leaf material was amplified using two primer sets: 731F (5′-TCTGGAGTCTTTCTTGAGCGA-3′) and 2R (5′-AACTAGTCGGAGTAG-3′), and 19F (5′-CGTTCTGACCATATTGCACTATG-3′) and 881R (5′-TMTTCATCAGAATAAGAGT-3′) as described by Sulistyo et al. (2015). The PCR reaction setup was the same as for nrITS with fresh plant material, but with additional BSA. The thermal cycling protocol began with a 5 min initial denaturation at 94°C, followed by 35 amplification cycles, each with a 1 min denaturation at 94°C, 30 s annealing at 50°C, and 1 min extension at 72°C, followed by a 7 min final extension at 72°C.
Sanger sequencing of the amplification products were performed at Baseclear (http://www.baseclear.com/), using an ABI-3730XL DNA Sequencer (Applied Biosystems). All sequences were deposited in NCBI GenBank. Accession numbers of all sequences can be found in Supplementary Table S2.
Sequence Editing and Phylogenetic Analysis
Sanger sequences were assembled and edited in Geneious® R8 (Biomatters Ltd., Auckland, New Zealand) (Kearse et al., 2012). The ends of all data sets were trimmed to avoid character misinterpretation. Ambiguous bases were replaced with “N” in the data matrix. DNA sequences were aligned using the MAFFT platform (Multiple Alignment Fast Fourier Transform) (Katoh and Standley, 2013) as implemented in Geneious® R8 with subsequent manual adjustment. Missing data were replaced with “?”.
A phylogenetic analysis was carried out using Bayesian Inference (BI) with Arundina graminifolia as an outgroup based on earlier studies (Gravendeel et al., 2001; Pedersen et al., 2020) that showed this genus to be most closely related to the necklace orchids. The chosen nucleotide substitution model GTR+G was calculated using the Akaike Information Criterion (AIC) in jModelTest2 v.2.1.6 (Darriba et al., 2015). The analyses were run in the CIPRES Science Gateway v.3.1. (Miller et al., 2010). We performed Bayesian interference analyses with Mr. Bayes v.3.2.6 on XSEDE (Huelsenbeck et al., 2004) with the following parameters for the alignment dataset: number of runs (nruns = 2), number of chains to run (nchains = 4), number of generations (ngen = 5 × 107), temperature parameter (temp = 2) and sampling frequency of 2000 yielding 25,000 trees per run. The log files from MrBayes were inspected in Tracer v.1.6 to check for convergence of independent runs (i.e. with estimated sample size (ESS) > 200). Maximum Likelihood analyses were performed with RAxML-HPC2 on XSEDE (8.2.10) (Stamakis et al., 2008) choosing the GTRGAMMA model for bootstrapping and 1,000 bootstrap iterations. Parsimony analyses were performed with PAUPRat: Parsimony ratchet searches using PAUP* (Nixon, 1999; Sikes and Lewis, 2001; Swofford, 2002) with 1,000 ratchet repetitions, seed value = 0, 20% percent of characters to perturb (pct = 20), original weights 1 for all characters (wtmode = uniform) and a tree bisection-reconnection branch swapping algorithm (swap = TBR). The 50% majority rule consensus for MP was obtained with PAUP v4.0a152. and inspected in FigTree v.1.3.1. The statistical support of the clades was evaluated with the values of posterior probability (PP) for BI reconstruction, bootstrap for ML (MLB) and parsimony bootstrap for MP (MPB). The support values (PP) were added to the branches on the Bayesian 50% majority-rule consensus tree with additional support values shown for ML and MP when the same topology was retrieved.
Bioprospecting Analysis
A randomly selected subset of 1.000 trees within the 95% highest posterior density (HPD) interval was used for further analyses using the packages caper, ape, plyr, and (Paradis et al., 2004; Kembel et al., 2010; Wickham, 2011; Orme et al., 2013) scripts in the R programming language (R Core Team, 2018) under RStudio (Gandrud, 2015). The R bioprospecting script of Ernst et al. (2016) was used to assess evolutionary patterns of medicinal properties of the necklace orchids analysed. The strength of the phylogenetic signal of the EBDCS category and the antimicrobial biological response category were investigated using D statistics (Fritz and Purvis, 2010), that was calculated with the phylo. d function implemented in the R package caper (Orme et al., 2013). A boxplot of the D values for each category of the two classification methods investigated was made using ggplot. If 95% of the 1.000 trees had a median value of D > 1, the medicinal properties were considered as randomly distributed; for D < 1, the phylogenetic signal was considered as strong (Ernst et al., 2016). D > 0 indicates that the medicinal properties of the orchids possess a significantly different distribution from the standard Brownian model, implying that they are clustered within the phylogeny. D < 0 indicates that the categories are extremely clustered. The prevalence of each category was measured by Ntotal species included in the category/Ntotal number of species. For a prevalence <0.020 the category was considered as too biased, and omitted from further analyses.
We also tested the phylogenetic diversity (PD) of the EBDCS category and the antimicrobial biological response category with the function pd in the R package picante v.1.6-2 (Kembel et al., 2010). The percentage of the possible response category of the antimicrobial biological response was compared with the Infections/Infestations category of the EBDCS classification method. A higher PD percentage means that species in this category are more scattered throughout the phylogeny. As a consequence, more potential species with medicinal properties are present because the PD-values are based on the total branch length spanned by the species (Ernst et al., 2016).
A consensus BI tree with 10% burnin was used to visualize the distribution of the two categories over the necklace orchid species investigated. Using the nodesigl command in R with the system PHYLOCOM v4.2 (Webb et al., 2008), so-called ‘hot nodes’ were calculated to visualize potential medicinal species.
Results
Medicinal Uses of Necklace Orchids Recorded in the Literature
For 28 necklace orchid species, traditional medicinal uses were compiled to determine 19 organ-targeted categories and a single biological response (i.e., antimicrobial) category with three different character states (see Tables 1, 2, and 3 for an overview of all data obtained from the literature). The prevalence of the categories Mental Disorders, Nervous System Disorders and Sensory System Disorders in the EBDCS classification method showed the lowest value of 0.006 because only one species was used in these categories.
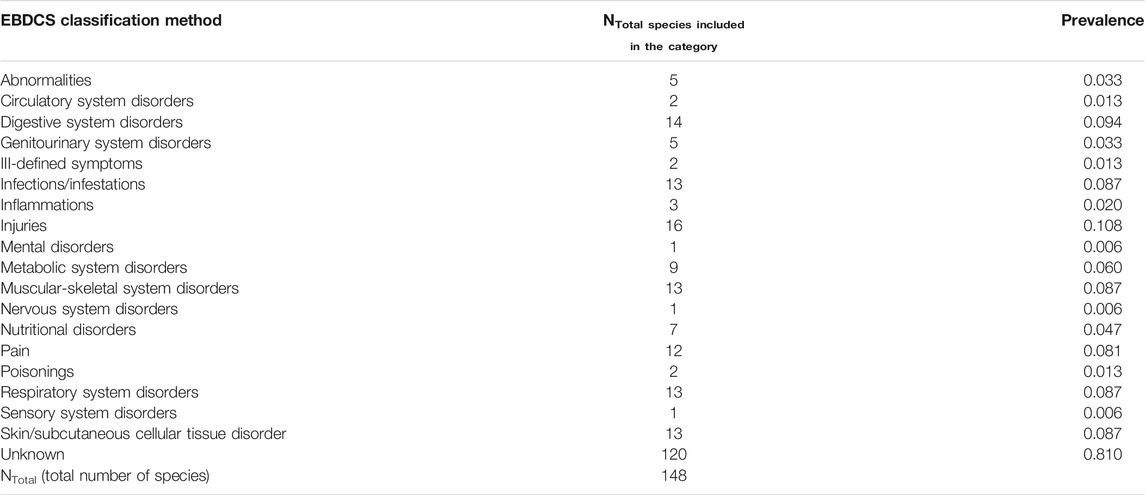
TABLE 2. Prevalence of various categories of medicinal use of necklace orchids for the organ targeted EBDCS classification method.

TABLE 3. Prevalence of various categories of medicinal use of necklace orchids for the antimicrobial response classification method.
Bioassays
None of the 100% hexane leaf and pseudobulb extracts and 70% of the ethanol pseudobulb extracts showed any antimicrobial effect in the bio-assays conducted. On the contrary, the 70% ethanol leaf extracts inhibited the growth of several of the human pathogens investigated (Table 4). Extracts obtained from freshly harvested leaves of C. cristata and C. fimbriata were found to inhibit growth of Y. enterocolitica, B. cereus and S. aureus and confirmed the traditional medicine uses recorded in the literature (Pyakurel and Gurung, 2008; Subedi, 2002; Subedi et al., 2013).
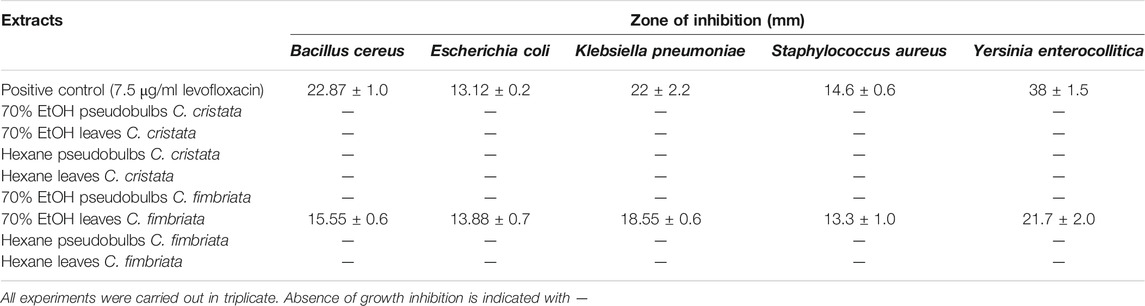
TABLE 4. Antimicrobial activity of extracts of Coelogyne cristata and C. fimbriata as recorded in the bioassays carried out in this study of five plants per species grown in greenhouses.
The highest effect was recorded for the 70% EtOH leaf extracts of C. fimbriata against Y. enterocollitica (19.6 ± 4.2 mm). Intermediate effects were recorded for leaf extracts of the same Coelogyne species against B. cereus (14.3 ± 1.4 mm) and S. aureus (13.6 ± 1.2 mm). Leaf extracts were found to have slightly stronger (but not significant) antimicrobial properties for plants cultivated outdoors than indoors (Table 5).

TABLE 5. Antimicrobial activity of extracts of Coelogyne cristata and C. fimbriata as recorded in the bioassays carried out in this study of five plants per species grown outside for 5 months in the Hortus botanicus Leiden, Netherlands .
Bioprospecting of Necklace Orchids
The majority consensus reconstructed BI tree, which is based on combined nrITS and plastid matK sequences for 148 species of necklace orchid species, is depicted in Figure 2. The consensus tree of ML and MP shows relatively high support for (>70%) and was congruent with the topology of the majority consensus BI tree. The Infections/Infestation category of the organ targeted EBDCS (Figure 2A) classification method and the biological (i.e. antimicrobial response) method (Figure 2B) were plotted on the BI tree.
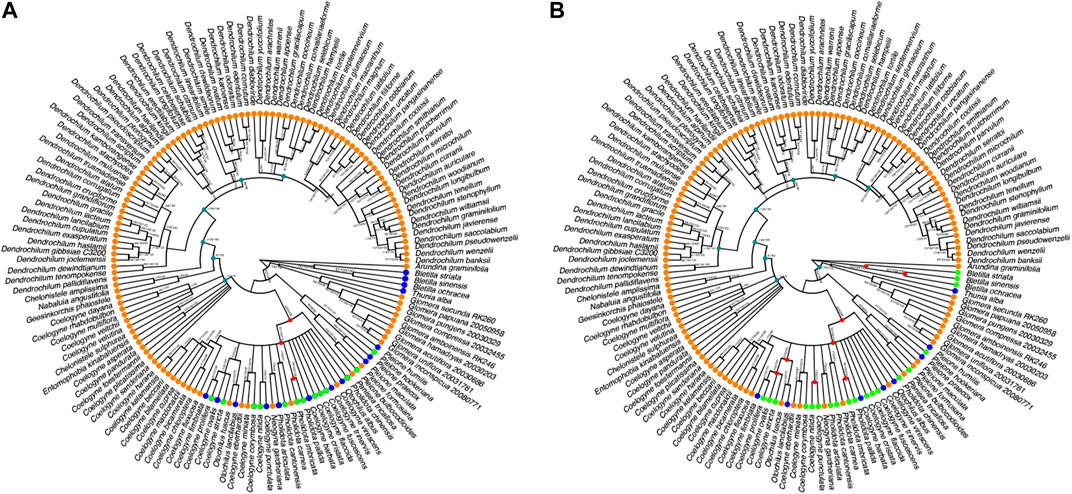
FIGURE 2. Majority consensus Bayesian Inference tree reconstructed on combined nrITS and plastid matK sequences of species of necklace orchids (Coelogyninae). (A) Plotting of the Infections/Infestation category of the organ targeted EBDCS classification method on this BI tree. (B) Plotting of the Antimicrobial biological response classification method. Explanation of color codes: species with no antimicrobial use (blue), species with unknown antimicrobial use (orange), species with possible antimicrobial use (light green), ancestral hot nodes of clades with high potency of species with antimicrobial properties (red). Hot nodes were identified with the nodesigl command in the PHYLOCOM package. Plotted branch values for Maximum Likelihood Bootstrap Support (BS), Maximum Parsimony BS, and Posterior Probability are given for each well-supported clade.
The boxplots of the D-statistics for the organ-based EBDCS classification method and the antimicrobial biological response classification method are shown in Figures 3 and 4. For the EBDCS classification method, 7 of the 19 categories showed a D > 1, indicating that a minority of these categories were randomly distributed. A total of 12 of the 19 categories were (extremely) clustered. For the antimicrobial response method, all the categories were found to be (extremely) clustered.
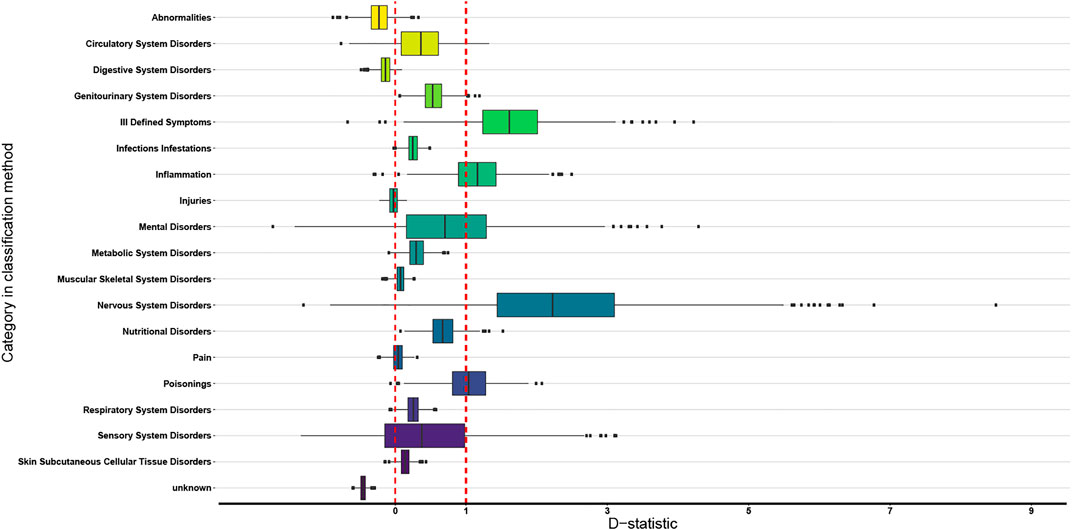
FIGURE 3. Boxplot of the 19 categories of the organ targeted EBDCS classification method (indicated with different colors) over which the data on medicinally used necklace orchid species that were analysed phylogenetically can be divided. The red lines indicate the D reference values 0 (on the left) and 1 (on the right). The box boundaries indicate the first and third quartile (Q1 and Q3), the line indicates the median, and the whiskers extend to either the extreme values or 1.5 times the interquartile range (Q3–Q1).
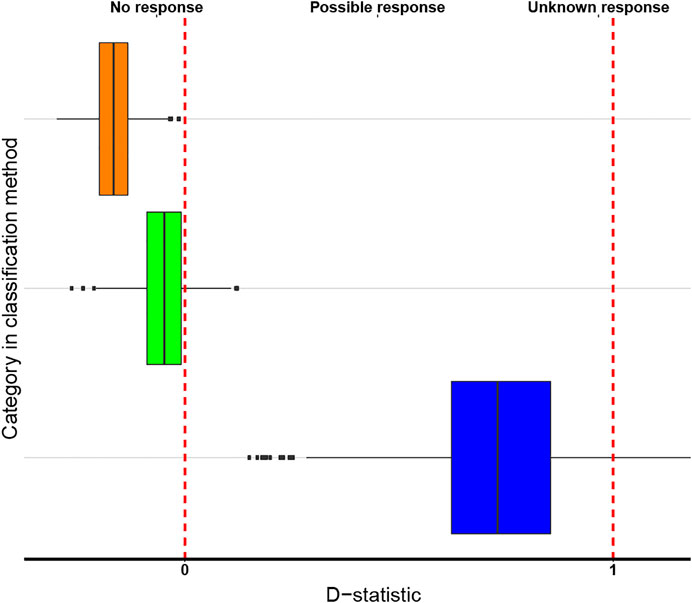
FIGURE 4. Boxplot of the Antimicrobial biological response classification method of the three characters states over which the data on medicinally used necklace orchid species, that were analysed phylogenetically, could be divided. Color codes: no response (orange), possible response (green), unknown response (blue). The red lines indicate the D reference values 0 (on the left) and 1 (on the right). The box boundaries indicate the first and third quartile (Q1 and Q3), the line indicates the median, and the whiskers extend to either the extreme values or 1.5 times the interquartile range (Q3–Q1).
The median of the phylogenetic diversity (PD) was calculated to compare the phylogenetic distribution of medicinal species from the Unknown, Possible and No Antimicrobial Response categories with the 19 categories of the organ based EBDSC classification method. In Figure 5, these medians are depicted. The Possible Antimicrobial Response category of the biological classification method had a median of 18.83%, whereas the Infections/Infestations category of the organ based EBDSC classification method had a median of 13.32%.
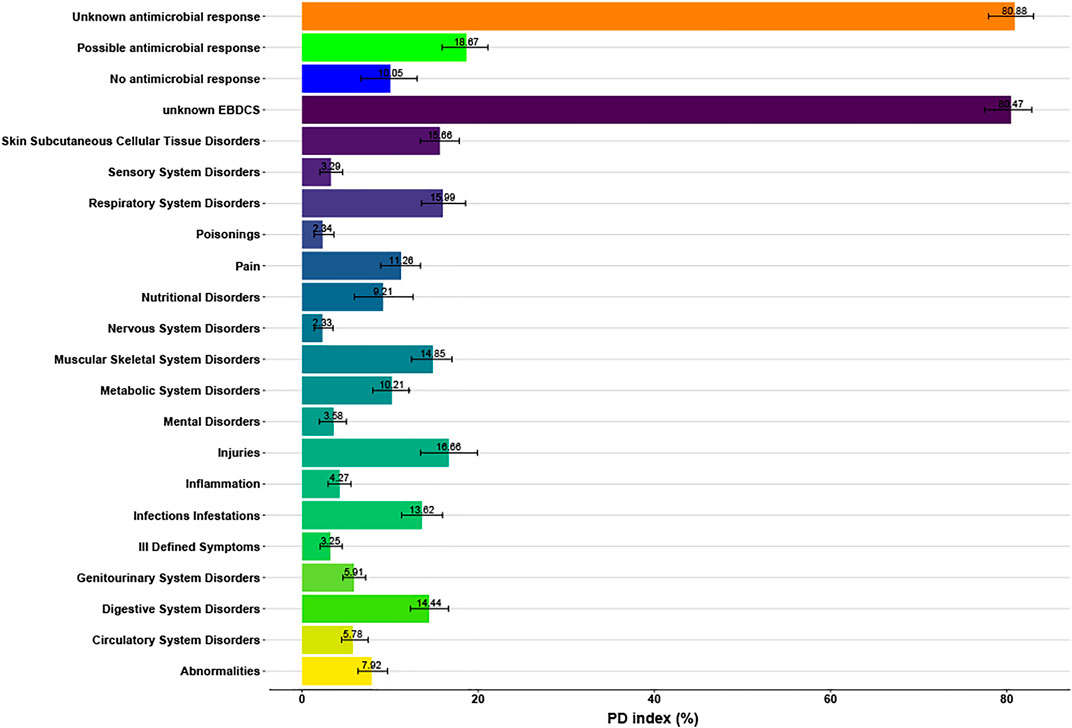
FIGURE 5. Median and standard errors of the Phylogenetic Diversity (PD) indices (in %) of the biological (i.e. antimicrobial) response and organ targeted EBDCS classification methods (indicated with different colors).
To narrow down potential new species with antimicrobial activities, the Possible Antimicrobial Response state of the biological response classification method was compared with the Infections/Infestations category of the EBDCS classification method using the PHYLOCOM platform. Figure 2 depicts the recovered hot nodes. Figure 2A shows the three hot nodes detected for the category within the EBDCS classification method with high potency for antimicrobial activities. Figure 2B shows the eight hot nodes detected for the biological response classification method.
Discussion
When compiling data on medicinal use of necklace orchids from the scientific literature, we noted that information for specific species was not always provided. This was for instance the case for the genus Dendrochilum. We thus urge ethnobotanists to make vouchers so that more detailed information for a particular genus can be obtained to link medicinal uses to the species level. Detailed information on plant organs used for medicinal purposes was not always provided either. We therefore recommend ethnobotanists to ask more detailed questions about specific organs used when interviewing traditional plant healers working with orchids. When information about plant organs was mentioned, this was sometimes contradictory among different publications. Our bio-assays showed that antimicrobial effects for extracts of leaves from C. fimbriata were much higher than those for pseudobulbs, which is not fully in agreement with previous publications, where it was found that pseudobulbs were the main source of secondary metabolites (Tóth et al., 2018). Our results are however supported by the publication of Buyun et al. (2016), who found that leaf extracts from Coelogyne ovalis produced larger inhibition zones than pseudobulbs extracts. The ethanol extract of leaves for Bulbophyllum neilgherrense also showed the same result (Priya and Krishnaveni, 2005). A possible explanation might be that the metabolites present in the pseudobulbs are more diluted as the relative amount of water is usually higher in these organs than in the leaves.
The exact method used to obtain plant extracts was also not always provided. Extracts dissolved in 70% ethanol had a higher antimicrobial effect in our bio-assays than extracts dissolved in hexane. This difference might be explained by the fact that hexane is a non-polar solvent that mostly extracts large fatty acid methyl esters with hydrocarbons and terpenes, whereas known antimicrobial substances isolated from necklace orchids are mostly phenanthrenes (Majumder et al., 2001; Kovács et al., 2008; Yang et al., 2012; Pant, 2014; Qian et al., 2015), which dissolve more readily in polar solvents such as ethanol.
Whether medicinal orchids were collected in the wild or from gardens or greenhouses was not mentioned in any of the publications that we screened. Our bio-assays show that antimicrobial effects of extracts of plants grown outside were higher (but not significantly so) than those of plants cultivated in greenhouses. A possible explanation for the difference in antimicrobial activity may be that plants naturally produce secondary metabolites that have a role in the defence against abiotic and biotic stresses (Dangle and Jones, 2001; Kim et al., 2009; Ramakrishna and Ravishankar, 2011). Recent studies by Isah (2019) also show that both stress and defense responses are involved in secondary metabolite production in plants. The insignificant differences found in our experiments when comparing indoor vs. outdoor cultivation methods might be a result of a too short exposure to UV light and herbivory, resulting in a too low level of secondary metabolites to create a significant difference between the cultivation methods. Plants grown in temperature controlled sterile greenhouses are generally exposed to less abiotic (UV light) and biotic (herbivores) stress and might therefore produce fewer secondary metabolites. Li et al. (1996) for instance reported that a longer exposure period to direct sunlight promoted higher ginsenoside production in American ginseng plants. Nevertheless, our results show that while exposure to UV light and herbivores may increase the antimicrobial activity of leaf extracts for necklace orchids, plants grown indoors also possess antimicrobial activity. This result contradicts the common folk belief that medicinal orchids can only be harvested from the wild to maintain their potency. We therefore encourage cultivation of medicinal necklace orchids in order to prevent overexploitation and extinction of rare species in the wild.
The 70% ethanol leaf extracts of C. fimbriata showed in vitro antimicrobial activity against S. aureus, B. cereus and Y. enterocolitica, all known to cause gastrointestinal tract infections in humans. Activity was observed against both Gram-positive and Gram-negative bacteria, which indicates a broad spectrum of antimicrobial effects of leaf extracts of necklace orchids. The extracts were not able to inhibit growth of E. coli and K. pneumoniae. This can be explained by the fact that Gram-negative bacteria generally develop more resistance against synthetic antibiotics as compared with Gram-positive bacteria because they can more efficiently regulate genes involved in antibiotic drug resistance (Peleg and Hooper, 2010).
In contrast with the organ targeted EBDCS classification method, all the categories from the biological response (i.e. antimicrobial) classification method were found to be (highly) clustered. The biological response classification method can therefore be considered as more informative for bioprospecting. The biological response classification method also had a more scattered distribution of medicinal species on the phylogeny than the EBDCS classification method, covering a wider group of potential medicinal necklace orchid species by retrieving eight hot nodes as compared with the organ targeted EBDCS classification method, that only found three hot nodes. One of the eight hot nodes detected by the biological response classification method, but not by the organ targeted EBDCS classification method, encompasses species of the necklace orchid genus Bletilla. Yang et al. (2012) successfully isolated bletilin A, bletilin B and other phenanthrenes from Bletilla ochracea tuber extracts that showed antibacterial activities against S. aureus, S. epidermis and B. subtilis. The fibrous roots and tubers from Bletilla striata contain biphenanthrenes and stilbenoids, which possess antibacterial activity (Kovács et al., 2008; Qian et al., 2015), Additionally, dihydrophenanthrenes, phenanthrene, flavonoids, bibenzyl and phenolic compounds were isolated from entire plants of B. formosana by Lin et al. (2005). These research findings support the results of our bioprospecting analyses and show that the biological response classification method is more effective in uncovering potential clades with high medicinal potential as compared with the EBDCS classification method.
Ethno-directed approaches to identifying plants traditionally used to treat specific diseases received significantly more attention over the past decade as this method shows a relatively high success rate compared to random plant screening programmes (Douwes et al., 2008; Siqueira et al., 2012). Plotting ethno-pharmacological data on a phylogenetic tree can be used as a time-efficient approach to discover potential new plant species with medicinal properties (Ernst et al., 2015), especially when a plant group is as large and diverse as the orchid family. We could only analyse 10% of all necklace orchid species for their medicinal properties. The reason for this was that while for some species with recorded medicinal use no DNA sequences were available, other species with known DNA sequences had not yet been investigated for their medicinal uses. We encourage more work into the ethnobotany and pharmacology of necklace orchids to increase species sampling. Especially species of the genera Bletilla, Coelogyne sect. Bicellae, sect. Brachypterae, sect. Coelogyne, sect. Elatae, sect. Flaccidae, sect. Fuscescentes, sect. Hologyne, sect. Lawrenceana, sect. Lentiginosae, sect. Longifoliae, sect. Ocellatae, sect. Proliferae, sect. Ptychogyne, sect. Speciosae, Neogyna, Otochilus and Pholidota sect. Articulatae, sect. Chinenses, sect. Crinonia, sect. Pholidota and sect. Repentes seem very promising for further research as these were identified to belong to hot node clades with high potency of antimicrobial activity.
Conclusion
We successfully applied bioprospecting to discover new necklace orchid species with antimicrobial activity. The traditional antimicrobial use of necklace orchids could be confirmed with bio-assays for leaf extracts prepared with 70% ethanol. Additionally, outdoor cultivation may result in increased antimicrobial activity, though this needs to be further explored. The biological response classification method was more effective in uncovering hot nodes leading to clades of species of necklace orchids with high antimicrobial potential as compared to the EBDCS classification method.
Data Availability Statement
The datasets presented in this study can be found in online repositories. The names of the repository/repositories and accession number(s) can be found in the article/Supplementary Material.
Author Contributions
RKW and EFG performed the experiments and analyzed the data. DB assisted with R analysis, RH helped with statistics, RV helped with orchid cultivation. RKW wrote the article with assistance from BG and EFS. All authors have read and approved the manuscript.
Funding
LPDP funded the PhD project of RKW (0044737/SC/D/2/lpdp2014) and Naturalis Biodiversity Center funded the open access publication fees. This research was partially supported by a machine allocation on the Kabré supercomputer at the Costa Rica National High Technology Center (CNCA/CeNAT).
Conflict of Interest
The authors declare that the research was conducted in the absence of any commercial or financial relationships that could be construed as a potential conflict of interest.
Supplementary Material
The Supplementary Material for this article can be found online at: https://doi.org/10.6084/m9.figshare.13071893.v2.
Acknowledgments
The authors would like to thank Lina Juswara, Aninda Wibowo, Bima Atmadja, Wenni Setyo Lestari and Nicolien Sol for granting permission to extract DNA from specimens from the Herbarium Bogoriense (BO), Bali Botanic Gardens and the herbarium of Naturalis Biodiversity Center (L). Lengguru Scientific Exploration of Papuan Karst, Koos Wubben and Rik Claessen are thanked for providing fresh orchid material. Henrie Korthout from Fytagoras helped us with finding the most effective extraction protocol for the bioassays. Rieneke Boeije and Kiki Fontein helped with optimising protocols for the bioassays. We also thank Mark Arentshorst from the Institute of Biology of Leiden University for granting us access to lab facilities certified for carrying out bioassays and Jolanda van Schie of the University of Applied Sciences Leiden for providing us with bacterial stocks. Marcella Orwick Rydmark is thanked for helping with language checking and Ivo Horn and Hugo de Boer for valuable suggestions for this manuscript. Last but not least we thank the Indonesia Endowment Fund for Education (LPDP) of the Ministry of Finance of Indonesia for funding the PhD project of RW.
References
Beena, C. (2011). Bioprospecting on the endangered medicinal plant Nervilia aragoana gaud. J. Progress. Agric. 2, 75–78. doi:10.7324/JABB.2019.70215
Buyun, L., Tkachenko, H., Kovalska, L., and Osadowski, Z. (2016). “Preliminary screening of Coelogyne ovalis Lindl. (Orchidaceae) for antimicrobial activity against Staphylococcus aureus”, in Agrobiodiversity for improving Nutrition, health and life quality 2017, 43–49. doi:10.15414/agrobiodiversity.2017.2585-8246
Cheng, T., Xu, C., Lei, L., Li, C., Zhang, Y., and Zhou, S. (2016). Barcoding the kingdom Plantae: New PCR primers for ITS regions of plants with improved universality and specificity. Mol. Ecol. Resour. 16, 138–149. doi:10.1111/1755-0998.12438
Cook, F. E. M. (1995). Economic Botany data collection standard. Editors J. M. Lock, and H. D. Prendergast (Kew: the Royal Botanic Gardens). doi:10.1111/j.1756-1051.1997.tb00317.x
CLSI, (2011). Performance Standards for Antimicrobial Susceptibility Testing; Twenty-First Informational Supplement. Wayne, PA:Clinical and Laboratory Standards Institute.
Cragg, G. M., and Newman, D. J. (2013). Natural products: a continuing source of novel drug leads, Biochim. Biophys. Acta 1830, 3670–3695. doi:10.1016/j.bbagen.2013.02.008
Dangle, J. L., and Jones, J. D. (2001). Plant pathogens and integrated defence responses to infection. Nature 411, 826–833. doi:10.1038/35081161
Darriba, D., Taboada, G. L., Doallo, R., and Posada, D. (2015). jModelTest 2: more models, new heuristics and high-performance computing Europe PMC Funders Group. Nat. Methods 9, 772. doi:10.1038/nmeth.2109
de Vogel, E. F., Vermeulen, J. J., and Schuiteman, A. (2019). Orchids of New Guinea http://www.orchidsnewguinea.com/. Accessed in 2019.
Douwes, E., Crouch, N. R., Edwards, T. J., and Mulholland, D. A. (2008). Regression analyses of southern African ethnomedicinal plants: informing the targeted selection of bioprospecting and pharmacological screening subjects. J. Ethnopharmacol. 119, 356–364. doi:10.1016/j.jep.2008.07.040
Doyle, J. J., and Doyle, J. L. (1987). A rapid DNA isolation procedure for small quantities of fresh leaf tissue. Phytochem. Bull. 19, 11–15.
Ernst, M., Grace, O. M., Saslis-Lagoudakis, C. H., Nilsson, N., Simonsen, H. T., and Rønsted, N. (2015). Global medicinal uses of Euphorbia L. (Euphorbiaceae). J. Ethnopharmacol. 176, 90–101. doi:10.1016/j.jep.2015.10.025
Ernst, M., Saslis-Lagoudakis, C., Grace, O. M., Nilsson, N., Simonsen, H. T., Horn, J. W., et al. (2016). Evolutionary prediction of medicinal properties in the genus Euphorbia L. Sci. Rep. 6, 30531. doi:10.1038/srep30531
Fritz, S. A., and Purvis, A. (2010). Selectivity in mammalian extinction risk and threat types: a new measure of phylogenetic signal strength in binary traits. Conserv. Biol. 24, 1042–1051. doi:10.1111/j.1523-1739.2010.01455.x
Gandrud, C. (2015). Reproducible research with R and RStudio. 2nd ed. Boca Raton, Florida: CRC Press. doi:10.1201/9781315382548
Gravendeel, B., Chase, M. W., De Vogel, E. F., Roos, M. C., Mes, T. H., and Bachmann, K. (2001). Molecular phylogeny of Coelogyne (Epidendroideae; Orchidaceae) based on plastid RFLPS, matK, and nuclear ribosomal ITS sequences: evidence for polyphyly. Am. J. Bot. 88, 1915–1927. doi:10.2307/3558367
Gutiérrez, R. M. P. (2010). Orchids: a review of uses in traditional medicine, its phytochemistry and pharmacology. J. Med. Plants Res. 4, 592–638. doi:10.5897/JMPR10.012
Hsiao, Y. Y., Pan, Z. J., Hsu, C. C., Yang, Y. P., Hsu, Y. C., Chuang, Y. C., et al. (2011). Research on orchid biology and biotechnology. Plant Cell Physiol. 52, 1467–1486. doi:10.1093/pcp/pcr100
Huelsenbeck, J. P., Larget, B., and Alfaro, M. E. (2004). Bayesian phylogenetic model selection using reversible jump Markov chain Monte Carlo. Mol. Biol. Evol. 21, 1123–1133. doi:10.1093/molbev/msh123
Isah, T. (2019). Stress and defense responses in plant secondary metabolites production. Biol. Res. 52, 39. doi:10.1186/s40659-019-0246-3
Katoh, K., and Standley, D. M. (2013). MAFFT multiple sequence alignment software version 7: improvements in performance and usability. Mol. Biol. Evol. 30, 772–780. doi:10.1093/molbev/mst010
Kearse, M., Moir, R., Wilson, A., Stones-Havas, S., Cheung, M., Sturrock, S., et al. (2012). Geneious Basic: an integrated and extendable desktop software platform for the organization and analysis of sequence data. Bioinformatics 28, 1647–1649. doi:10.1093/bioinformatics/bts199
Kembel, S. W., Cowan, P. D., Helmus, M. R., Cornwell, W. K., Morlon, H., Ackerly, D. D., et al. (2010). Picante: R tools for integrating phylogenies and ecology. Bioinformatics 26, 1463–1464. doi:10.1093/bioinformatics/btq166
Kim, J. Y., Park, S. C., Hwang, I., Cheong, H., Nah, J. W., Hahm, K. S., et al. (2009). Protease inhibitors from plants with antimicrobial activity. Int. J. Mol. Sci. 10, 2860–2872. doi:10.3390/ijms10062860
Kovács, A., Vasas, A., and Hohmann, J. (2008). Natural phenanthrenes and their biological activity. Phytochemistry 69, 1084–1110. doi:10.1016/j.phytochem.2007.12.005
Lawler, L. J. (1984). “Ethnobotany of the Orchidaceae”, in Orchid Biology: reviews and perspectives. Editor J. Arditti (New York: Cornell University Pressm), Vol. III, 27–149.
Leonti, M., Cabras, S., Castellanos, M., Challenger, A., Gertsch, J., and Casu, L. (2013). Bioprospecting: evolutionary implications from a post-olmec pharmacopoeia and the relevance of widespread taxa. J. Ethnopharmacol. 147, 92–107. doi:10.1016/j.jep.2013.02.012
Li, T. S. C., Mazza, G., Cottrell, A. C., and Gao, L. (1996). Ginsenosides in roots and leaves of American Ginseng. J. Agric. Food Chem. 44, 717–720.
Lin, Y. L., Chen, W. P., and Macabalang, A. D. (2005). Dihydrophenanthrenes from Bletilla formosana. Chem. Pharm. Bull. 53, 1111–1113. doi:10.1248/cpb.53.1111
Majumder, P. L., Sen, S., and Majumder, S. (2001). Phenanthrene derivatives from the orchid Coelogyne cristata. Phytochemistry 58, 581–586. doi:10.1016/s0031-9422(01)00287-4
Miller, M. A., Wayne, P., and Schwartz, T. (2010). “Creating the CIPRES Science Gateway for Inference of Large Phylogenetic Trees.” 2010 Gateway Computing Environments Workshop (GCE), (New Orleans, LA: GCE), 1–8.
Mutsaers, J. A. E. M., Doornbos, L., and Knecht, N. M. (2001). Bacteriologie voor laboratorium en kliniek 2. 4th ed. Utrecht: Syntax Media.
Newman, D. J., Cragg, G. M., and Snader, K. M. (2000). The influence of natural products upon drug discovery. Nat. Prod. Rep. 17, 215–234. doi:10.1039/a902202c
Nixon, K. C. (1999). The parsimony ratchet, a new method for rapid parsimony analysis. Cladistics 1, 407–414.
Orme, D., Freckleton, R., Thomas, G., Thomas, P., Fritz, S., Isaac, N., et al. (2013). CAPER: Comparative analyses of Phylogenetics and evolution in R. Methods Ecol. Evol. 3, 145–151. 10.1098/rsos.181182
Pant, B. (2014). Medicinal orchids and their uses: tissue culture a potential alternative for conservation. Afr. J. Plant Sci. 7, 448–467. doi:10.5897/ajps2013.1031
Pant, B., and Raskoti, B. B. (2013). Medicinal orchid of Nepal. Kathmandu: Himalayan Map House Pvt. Ltd.
Paradis, E., Claude, J., and Strimmer, K. (2004). APE: analyses of phylogenetics and evolution in R language. Bioinformatics 20, 289–290. doi:10.1093/bioinformatics/btg412
Pedersen, H., Petersen, G., Gravendeel, B., Barkman, T. J., de Boer, H., Sulistyo, B. P., et al. (2020). Phylogenetics of Dendrochilum (Orchidaceae): evidence of pronounced morphological homoplasy and predominantly centric endemism. Taxon 68, 1173–1188. doi:10.1002/tax.12184
Peleg, A. Y., and Hooper, D. C. (2010). Hospital-acquired infections due to gram-Negative Bacteria. N. Engl. J. Med. 362, 1804–1813. doi:10.1056/NEJMra0904124
Pridgeon, A. M., Cribb, P. J., Chase, M. W., and Rasmussen, F. N. (2005). Genera Orchidacearum, Epidendroideae (Part 1), Vol. 4. Oxford, USA: Oxford University Press.
Priya, K., and Krishnaveni, C. (2005). Antibacterial effect of bulbophyllum neilgherrense wt. (Orchidaceae). An in vitro study. Ancient Sci. Life 25, 50–52. doi:10.1007/S00044012-0228-2
Pyakurel, D., and Gurung, K. (2008). Enumeration of orchids and estimation of current stock of traded orchids in Rolpa district : Final report. Rolpa, Nepal: District forest office Rolpa.
Purkayastha, J. (2016). Bioprospecting of Indigenous Bioresources of North-East India. Singapore, Singapore: Springer. doi:10.1007/978-981-10-0620-3
Qian, C. D., Jiang, F. S., Yu, H. S., Shen, Y., Fu, Y. H., Cheng, D. Q., et al. (2015). Antibacterial biphenanthrenes from the fibrous roots of Bletilla striata. J. Nat. Prod. 78, 939–943. doi:10.1021/np501012n
Ramakrishna, A., and Ravishankar, G. A. (2011). Influence of abiotic stress signals on secondary metabolites in plants. Plant Signal. Behav. 6, 1720–1731. doi:10.4161/psb.6.11.17613
Robinson, M. M., and Zhang, X. (2011). Traditional medicines: global situation, issues and Challenges. Geneva, Switzerland: WHO.
Saslis-Lagoudakis, C. H., Savolainen, V., Williamson, E. M., Forest, F., Wagstaff, S. J., Baral, S. R., et al. (2012). Phylogenies reveal predictive power of traditional medicine in bioprospecting. Proc. Natl. Acad. Sci. USA 109, 15835–15840. doi:10.1073/pnas.1202242109
Savoia, D. (2012). Plant-derived antimicrobial compounds: alternatives to antibiotics. Future Microbiol. 7, 979–990. doi:10.2217/fmb.12.68
Schuiteman, A., and de Vogel, E. F. (2001). Orchids of New Guinea, Vol. I. Illustrated Checklist and Genera CD-ROM. 90-75000-20-0.
Sikes, D. S., and Lewis, P. O. (2001). Beta software, version 1.PAUPRat:PAUP* implementation of the parsimony ratchet.
Singh, S., and Singh, A. K. (2012). Medicinal properties and uses of orchids: a concise review. Elixir Appl. Bot. 52, 11627–11634.
Siqueira, C. F., Cabral, D. L., Peixoto Sobrinho, T. J., De Amorim, E. L., De Melo, J. G., Araújo, T. A., et al. (2012). Levels of tannins and flavonoids in medicinal plants: evaluating bioprospecting strategies. Evid. Based Complem. Alternat. Med. 2012, 434782. doi:10.1155/2012/434782
Smith, W. L., and Wheeler, W. C. (2006). Venom evolution widespread in fishes: a phylogenetic road map for the bioprospecting of piscine venoms. J. Hered. 97, 206–217. doi:10.1093/jhered/esj034
Stamakis, A., Hoover, P., and Rougemontm, J. (2008). A rapid bootstrap algorithm for the RAxML web servers. Syst. Biol. 57, 758–771. doi:10.1080/10635150802429642
Subedi, A. (2002). Orchids around Pokhara valley of Nepal. Local Initiatives for Biodiversity, Pokhara, Nepal: Research and Development.
Subedi, A., Chaudhary, R. P., van Achterberg, C., Heijerman, T., Lens, F., van Dooren, T. J., et al. (2011). Pollination and protection against herbivory of Nepalese Coelogyninae (Orchidaceae). Am. J. Bot. 98, 1095–1103. doi:10.3732/ajb.1000306
Subedi, A., Kunwar, B., Choi, Y., Dai, Y., van Andel, T., Chaudhary, R. P., et al. (2013). Collection and trade of wild-harvested orchids in Nepal. J. Ethnobiol. Ethnomed. 9, 64–10. doi:10.1186/1746-4269-9-64
Sulistyo, B. P., Boos, R., Cootes, J. E., and Gravendeel, B. (2015). Dendrochilum hampelii (Coelogyninae, Epidendroideae, Orchidaceae) traded as ‘Big Pink' is a new species, not a hybrid: evidence from nrITS, matK and ycf1 sequence data. PhytoKeys 56, 83–97. doi:10.3897/phytokeys.56.5432
Swofford, D. L. (2002). PAUP. Phylogenetic analysis using Parsimony (and other methods), Version 4.0 Beta 10. Sunderland: Sinauer Associates.
Tóth, B., Hohmann, J., and Vasas, A. (2018). Phenanthrenes: a Promising Group of Plant secondary metabolites. J. Nat. Prod. 81, 661–678. doi:10.1021/acs.jnatprod.7b00619
Vaidya, B., Shrestha, M., and Joshee, N. (2000). “Report on Nepalese orchid species with medicinal Properties,” in Proceeding of Nepal-Japan Joint Symposium-2000. Editors T. Watanabe, A. Tokano, M. Bista, and H. Saiju (Japan Society for Conservation and Development of Himalaya Medicinal Resources), 146–152.
Wang, J., Matsuzaki, K., and Kitanaka, S. (2006). Stilbene derivatives from Pholidota chinensis and their anti-inflammatory activity. Chem. Pharm. Bull. 54, 1216–1218. doi:10.1248/cpb.54.1216
Washington, J. A. (1996). Principles of Diagnosis. Editor S. Baron Galveston (Texas: University of Texas Medical Branch).
Wati, R. K., van Vugt, R. R., and Gravendeel, B. (2018). A Linnaeus NG interactive key to the species of Glomera (Orchidaceae, Coelogyninae) from Southeast Asia. PhytoKeys 110, 9–22. doi:10.3897/phytokeys.110.28435
Webb, C. O., Ackerly, D. D., and Kembel, S. W. (2008). Phylocom: software for the analysis of phylogenetic community structure and trait evolution. Bioinformatics 24, 2098–2100. doi:10.1093/bioinformatics/btn358
Wickham, H. (2011). The split-apply-combine strategy for data analysis. J. Stat. Softw. 40, 1–29. doi:10.18637/jss.v040.i01
Yang, X., Tang, C., Zhao, P., Shu, G., and Mei, Z. (2012). Antimicrobial constituents from the tubers of bletilla ochracea. Planta Med. 78, 606–610. doi:10.1055/s-0031-1298264
Yonzone, R., Kamran, A., and Bhujel, R. B. (2012). “Orchids in ethnobotany”, in International seminar on “Multidisciplinary approaches in angiospenn systematics, 661–669.
Yonzone, R., Lama, D., Bhujel, R. B., and Rai, S. (2013). Present availability status, diversity resources and distribution of medicinal orchid species in Darjeeling Himalaya of West Bengal, India. Int. J. Pharm. Pharmaceut. Sci. 1, 14–35.
Keywords: bio-assays, bioprospecting, Coelogyninae, herbal medicine, horticulture, hot nodes
Citation: Wati RK, de Graaf EF, Bogarín D, Heijungs R, van Vugt R, Smets EF and Gravendeel B (2021) Antimicrobial Activity of Necklace Orchids is Phylogenetically Clustered and can be Predicted With a Biological Response Method. Front. Pharmacol. 11:586345. doi: 10.3389/fphar.2020.586345
Received: 23 July 2020; Accepted: 24 November 2020;
Published: 12 March 2021.
Edited by:
Prof Pulok Kumar Mukherjee, Institute of Bio-Resources and Sustainable Development (IBSD), IndiaReviewed by:
Guo-Qiang Zhang, Orchid Conservation and Research Center, ChinaChristian Agyare, Kwame Nkrumah University of Science and Technology, Ghana
Copyright © 2021 Wati, de Graaf, Bogarín, Heijungs, van Vugt, Smets and Gravendeel. This is an open-access article distributed under the terms of the Creative Commons Attribution License (CC BY). The use, distribution or reproduction in other forums is permitted, provided the original author(s) and the copyright owner(s) are credited and that the original publication in this journal is cited, in accordance with accepted academic practice. No use, distribution or reproduction is permitted which does not comply with these terms.
*Correspondence: Richa Kusuma Wati, cmljaGEua3VzdW1hd2F0aUBuYXR1cmFsaXMubmw=;, cmljaDAwMkBsaXBpLmdvLmlk