- Department of Nephrology, the First Affiliated Hospital of Nanjing Medical University, Nanjing Medical University, Nanjing, China
Chronic kidney disease (CKD) is a common progressive disease that is typically characterized by the permanent loss of nephrons and an eventual decline in glomerular filtration rate. CKD increases mortality and has a significant impact on the quality of life and the economy, which is becoming a major public health issue worldwide. Since current conventional-medicine treatment options for CKD are not satisfactory, many patients seek complementary and alternative medicine treatments including Traditional Chinese Medicine. Herbal medicine is often used to relieve symptoms of renal diseases in the clinic. The kidney is abundant in the number of mitochondria, which provide enough energy for renal function and metabolism. In recent years, a vital role for mitochondrial dysfunction has been suggested in CKD. Mitochondria have become a new target for the treatment of diseases. A growing number of studies have demonstrated herbal medicine could restore mitochondrial function and alleviate renal injury both in vivo and in vitro. In this review, we sum up the therapeutic effect of herbal medicine in CKD via targeting mitochondrial function. This implies future strategies in preventing CKD.
Introduction
Chronic kidney disease (CKD) is a major public health issue worldwide, which is related to severe complications, poor quality of life and increased financial burden (Bello et al., 2017). CKD is defined as a gradual decrease in renal function and eventually resulting in end-stage renal disease (ESRD). The main aims of treatment are to slow the progression of the decline in renal function and limit extrarenal complications (Nasrallah et al., 2014). However, the current therapeutic approaches for CKD have not achieved the desired effects in the clinic (Leporini et al., 2016).
The search for complementary and alternative medicine treatments for CKD is thus arousing more and more attention. In the clinic, many patients seek Traditional Chinese Medicine for alternative therapy. Traditional Chinese Medicine plays an important role in the Chinese healthcare system that has been developed over the centuries to prevent and treat diseases. It includes mind-body practices and herbal medicines (Spatz et al., 2018). Herbal medicine is abundant in various chemical components, such as active substances with therapeutic value and nutritional active ingredients, which determines the characteristics of its multi-target. Studies showed that herbal medicine could relieve symptoms, decrease proteinuria, and slow the reduction of renal function (Zhong et al., 2015).
Mitochondria provide energy for the kidney to clear waste in the blood and regulate water and electrolyte balance (Sun et al., 2019). Mitochondrial dysfunction is closely related to the development and progression of CKD. The maintenance of mitochondrial function relies on the balance of mitochondrial turnover and mitochondrial dynamics (Aparicio-Trejo et al., 2018). Mitochondrial turnover includes mitochondrial biogenesis, which augments mitochondrial mass and ATP production, and mitophagy that removes damaged mitochondria (Pedraza-Chaverri et al., 2016). Mitochondria are in a dynamic equilibrium of fission and fusion to maintain mitochondrial homeostasis.
Although a vast amount of evidence showed herbal medicines could alleviate renal injury by regulating mitochondrial function, a more detailed understanding of the role of mitochondrial homeostasis in herbal medicines is still limited. This review pays attention to the therapeutical effect of herbal medicines in CKD by targeting mitochondrial function, especially the application prospect of herbal medicines (Table 1 and Figure 1).
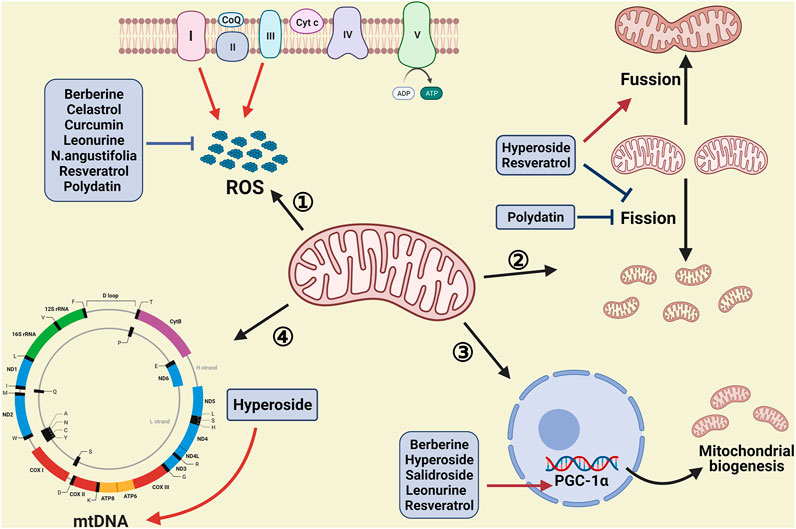
FIGURE 1. Therapeutic interventions of herbal medicine by targeting mitochondrial homeostasis. Many procedures are involved in mitochondrial homeostasis, such as the production of ROS, mitochondrial dynamics and mitochondrial biogenesis. Herbal medicine plays a protective role in CKD via decreasing the augmentation of ROS, inhibiting mitochondrial fission, promoting mitochondrial fusion, as well as increasing mitochondrial biogenesis and the copies of mtDNA (Created with BioRender.com).
Herbal Medicines in CKD via Targeting Mitochondrial Biogenesis
Mitochondrial biogenesis is a complicated process that new mitochondria are generated from preexisting mitochondria to increase mitochondrial mass. Mitochondrial biogenesis mainly includes four parts: the inner and outer mitochondrial membranes synthesis, synthesis of the proteins encoded by mitochondria, synthesis and imported mitochondrial proteins encoded by nuclear, as well as replication of mitochondrial DNA (mtDNA) (Li et al., 2017; Fontecha-Barriuso et al., 2020). Peroxisome proliferator-activated receptor γ coactivator-1α (PGC-1α) is the key regulator of mitochondrial biogenesis which can not only improve the expression of nuclear respiratory factor 1 and 2 (NRF1 and NRF2) but also enhance the interaction with them. The activation of NRF1 and NRF2 stimulates mtDNA replication and transcription via mitochondrial transcription factor A (Tfam). Also, the activation of NRF1 and NRF2 increases the transcription of subunits of mitochondrial electron transport chain encoded by nuclear (Uittenbogaard and Chiaramello, 2014; Li et al., 2017). Berberine, a naturally occurring isoquinoline alkaloid, is present in Coptidis rhizoma and Cortex Phellodendri (Zhang et al., 2016). Qin et al. reported that PGC-1α was significantly decreased in db/db mice and palmitic acid-induced podocytes. Berberine increased the expression of PGC-1α and improved mitochondrial function via the AMPK/PGC-1α pathway both in vivo and in vitro (Qin et al., 2020). Hyperoside, also named quercetin 3-o- β -D-galactopyranoside, is a flavonol glycoside extracted from Rhododendron ponticum L that is widely used in Traditional Chinese Medcine (Li et al., 2013; Wang et al., 2018). Chen et al. proposed hyperoside improved the loss of PGC-1α and mitochondrial dysfunction caused by adriamycin both in vivo and in vitro (Chen et al., 2017). Leonurine (C14H21N3O5), a natural alkaloid, is derived from Herba Leonuri (Hu et al., 2019; Zhang et al., 2019). Leonurine could ameliorate the expression of PGC-1α in adriamycin-treated podocytes (Liu et al., 2018). Resveratrol is a polyphenolic phytoalexin belonging to the stilbenes derivative which is abundant in berries, grapes, nuts (Asgary et al., 2019). Kim et al. observed that resveratrol increased the protein level of PGC-1α and Nrf2 and ameliorated mitochondrial dysfunction in aging-related renal injury (Kim et al., 2018). Salidroside is a bioactive compound isolated from Traditional Chinese Medicine Rhodiola rosea (Zhao et al., 2019). Xue et al. proposed salidroside attenuated the damage of renal structure and function in STZ-induced diabetic mice. Furthermore, the decrease of PGC-1α was reversed in salidroside-treated mice (Xue et al., 2019).
Herbal Medicines in CKD via Targeting Mitochondrial Dynamics
Mitochondria are continuously changing their morphology by constant fission and fusion to adapt to environmental imperatives and cellular energetic needs (Rovira-Llopis et al., 2017). The disruption between the balance of fission and fusion leads to mitochondrial dysfunction and eventually evokes the development of mitochondria-related diseases. Mitochondrial dynamics depend on several dynamin-related guanosine triphosphatases (GTPases). Mitochondrial fission, split one mitochondrion into two daughter mitochondria, is mainly regulated by Dynamin-related protein 1 (Drp1) in the cytosol and its receptors on the outer membrane, including fission protein 1 (Fis1), mitochondrial fission factor (MFF) and mitochondrial dynamics proteins of 49 kDa and 51 kDa (MiD49 and MiD51) (Benigni et al., 2016). Mitochondrial fusion, combine two mitochondria into one mitochondrion, is a two-step process resulting from its double membranes. Mitofusin1 and 2 (Mfn1/2) contribute to outer-membrane fusion, while Optic Atrophy 1 (OPA1) mediates the fusion of the inner membrane (Mishra and Chan, 2016). Chen et al. showed adriamycin triggered mitochondrial fission and reduced the expression of Mfn1, which were reversed after treating with hyperoside (Chen et al., 2017). Polydatin is a monocrystalline and polyphenolic drug extracted from the traditional herb Polygonum cuspidatum (Gao et al., 2015). Zheng et al. pointed that polydatin ameliorated renal function in KKAy mice and reduced apoptosis in KKAy mice and high glucose-exposed podocytes. In addition, they found polydatin decreased the elevation of p-Drp1 and fragmented mitochondria caused by high glucose. Furthermore, they demonstrated the upregulation of Drp1 by transfection with Drp1-GFP lentivirus enhanced apoptosis and fragmented mitochondria while polydatin blocked the increase of apoptosis (Zheng et al., 2017). Hui et al. showed the expressions of Mfn2 and OPA1 were declined but the level of Drp1 was increased in 5/6 nephrectomized rats model and TGF-β1-treated mesangial cells. Resveratrol treatment reversed these changes and restored mitochondrial function both in vivo and in vitro (Hui et al., 2017).
Herbal Medicines in CKD via Targeting Mitochondrial ROS
Reactive oxygen species (ROS), byproducts of cellular aerobic metabolism, play a significant part in cellular signaling processes. ROS consists of superoxide anion (O2•−), hydroxyl radicals (OH•), and hydrogen peroxide (H2O2) (Schieber and Chandel, 2014). As the main production of endogenous ROS, mitochondria consumed 1–2% of oxygen to produce ROS in the process of mitochondrial respiration (Pinegin et al., 2018). It has been reported that complex I and complex III of the mitochondrial electron transport chain are the major sites of the production of ROS in mitochondria (Mazat et al., 2020). ROS produced by complex I (NADH: ubiquinone oxidoreductase) is mainly in the mitochondrial matrix, while ROS created by complex III (ubiquinol: cytochrome oxidoreductase) is both in the matrix and intermembrane space. Mitochondrial matrix dehydrogenases contribute a lot to the generation of ROS as well (Pinegin et al., 2018; Stefanatos and Sanz, 2018; Bouchez and Devin, 2019). Scavenging of ROS contributes to the homeostasis of ROS in mitochondria. The O2•− produced in the mitochondrial matrix is dismutated to H2O2 by superoxide dismutase protein 2 (SOD2). The O2•− generated in intermembrane space is converted to H2O2 by SOD1 (Diebold and Chandel, 2016). H2O2 is degraded to H2O by glutathione peroxidases (GPX) or peroxiredoxins (PRX). The excessive production of mitochondrial ROS or the impaired antioxidant defense leads to mitochondrial dysfunction and renal injury (Angelova and Abramov, 2018). Zhang et al. discovered, in high glucose treated cultured tubular epithelial cell line and human kidney proximal tubular cell line, berberine mitigated ROS production and elevated the level of GSH and SOD by PI3K/Akt pathway (Zhang et al., 2016). In palmitic acid-induced podocytes, berberine attenuated the increased generation of ROS reflected by MitoSOX Red (Qin et al., 2020). As a plant-derived pentacyclic lupane-type triterpenoid, betulinic acid can be extracted from white birch trees (Phillips et al., 2018; Ríos and Máñez, 2018). Xie et al. proposed betulinic acid raised the expression of SOD through AMPK/NF-κB/Nrf2 pathway in streptozotocin-induced diabetic rats (Xie et al., 2017). Celastrol, a naturally occurring pentacyclic triterpene, is extracted from Tripterygium wilfordii (Fang et al., 2019). Zhan et al. found treatment with celastrol decreased the production of ROS in high glucose-treated podocytes (Zhan et al., 2018). Curcumin, a natural polyphenol, is an active component of turmeric that has been used as a spice and traditional medicine for centuries in India and China (Bahrami et al., 2019). Sun et al. discovered the level of ROS was escalated in a time-dependent way in high glucose-induced podocytes. In contrast, the activity of SOD was gradually reduced in podocytes exposed to high glucose in a time-dependent manner. Curcumin inhibited ROS and improved the activity of SOD in diabetic nephropathy both in vivo and in vitro (Sun et al., 2016). Liu et al. found leonurine alleviated the augmentation of ROS and increased the level of SOD2 in adriamycin-induced podocyte injury (Liu et al., 2018). Nepeta angustifolia C. Y. Wu, a traditional Tibetan medicine, consists of pentacyclic triterpenes and bioactive flavonoids which are reported to have anti-inflammatory and antioxidant effects (Huang et al., 2019). In the kidney of diabetic rats, the expressions of SOD and GSH were remarkably augmented after exposure to Nepeta angustifolia C. Y. Wu. In addition, Nepeta angustifolia C. Y. Wu attenuated ROS production in H2O2-induced rat glomerular mesangial cells. Compared with the group treated with H2O2, Nepeta angustifolia C. Y. Wu also improved the expression of SOD in rat glomerular mesangial cells (Huang et al., 2020). Polydatin, a resveratrol glycoside, ameliorated ROS levels in high glucose-treated primary podocytes and glomerular mesangial cells. Additionally, Gong et al. pointed out that polydatin increased the activity of SOD in the kidney and serum of diabetic mice (Gong et al., 2017; Zheng et al., 2017). Hui et al. observed resveratrol reversed the enhancement of ROS in 5/6 nephrectomized rats and TGF-β1-treated mouse mesangial cells (Hui et al., 2017). Furthermore, resveratrol improved SOD1 and SOD2 in aged mice and H2O2-treated human kidney proximal tubular cells by activating Nrf2 and SIRT1 pathways (Kim et al., 2018).
Herbal Medicines in CKD via Targeting Mitochondrial DNA
Mitochondrial DNA (mtDNA), consisting of 16,569 base pairs in humans, is a circular double-stranded genome (El-Hattab et al., 2017). mtDNA carries 37 genes containing 13 polypeptides, 22 tRNAs and 2 rRNAs (Srirattana and St. John, 2019). The proteins encoded by 13 polypeptides are major parts of mitochondrial oxidative phosphorylation including 7 subunits of Complex I, 1 of Complex III, 3 of Complex IV, and 2 of Complex V (Roubicek and Souza-Pinto, 2017). Apart from them, other proteins mitochondria needed are encoded by nuclear, synthesized in the cytoplasm, and eventually transported into mitochondria (Szczepanowska and Trifunovic, 2015). The mtDNA is coated with proteins and organized in a complex named mitochondrial nucleoid. Mitochondrial nucleoids are located on the matrix side of the mitochondrial inner membrane and well-distributed in the mitochondria for cellular metabolic needs (Almannai et al., 2018). The copy number of mtDNA is the indicator of mitochondrial function (Tin et al., 2016). In adriamycin (Chen et al., 2017) mice, the level of urinary albumin (Chen et al., 2017) was lifted while mtDNA was significantly reduced. Compared with the adriamycin group, the group exposed to hyperoside exhibited lower urinary albumin and increased mtDNA. In addition, co-incubated with hyperoside augmented the copies of mtDNA in adriamycin-induced human podocytes (Chen et al., 2017).
There are some limitations in this review that merit attention. First, herbal medicine usually has multiple targets. Further studies are needed to confirm whether mitochondria are the specific target. Second, very little is known about how herbal medicine enters the mitochondria and regulates mitochondrial homeostasis. Third, most of the Traditional Chinese Medicine we mentioned above showed protective effects in animal and cellular experiments, it remains unclear if they could be applied to clinical trials.
Conclusion
Recently, more and more attention has been paid to the role of mitochondria in the occurrence and development of chronic kidney disease. A growing number of studies have proved mitochondria gradually became the promising target for the therapy of CKD. Traditional Chinese Medicine targeting mitochondria provides new ideas for the clinical treatment of CKD.
Author Contributions
QL drafted the review. CX and YY revised it. All authors have read the final paper and approved the submission.
Funding
This work was supported by grants from the National Natural Science Foundation of China (No. 81670628, 81870469, 81300573), the Natural Science Foundation of Jiangsu Province (No. BK20131030), the “333 Project” of Jiangsu Province, the Six Talent Peaks Project in Jiangsu Province (WSN-010), the China Scholarship Council (CSC, File No. 201608320124), Chinese Society of Nephrology (17010060675), the Clinic Research Center of Jiangsu Province (No. BL2014080) and the Priority Academic Program Development (PAPD) of Jiangsu Higher Education Institution.
Conflict of Interest
The authors declare that the research was conducted in the absence of any commercial or financial relationships that could be construed as a potential conflict of interest.
References
Almannai, M., El-Hattab, A. W., and Scaglia, F. (2018). Mitochondrial DNA Replication: Clinical Syndromes. Essays Biochem. 62 (3), 297–308. doi:10.1042/ebc20170101
Angelova, P. R., and Abramov, A. Y. (2018). Role of Mitochondrial ROS in the Brain: from Physiology to Neurodegeneration. FEBS Lett. 592 (5), 692–702. doi:10.1002/1873-3468.12964
Aparicio-Trejo, O. E., Tapia, E., Sánchez-Lozada, L. G., and Pedraza-Chaverri, J. (2018). Mitochondrial Bioenergetics, Redox State, Dynamics and Turnover Alterations in Renal Mass Reduction Models of Chronic Kidney Diseases and Their Possible Implications in the Progression of This Illness. Pharmacol. Res. 135, 1–11. doi:10.1016/j.phrs.2018.07.015
Asgary, S., Karimi, R., Momtaz, S., Naseri, R., and Farzaei, M. H. (2019). Effect of Resveratrol on Metabolic Syndrome Components: A Systematic Review and Meta-Analysis. Rev. Endocr. Metab. Disord. 20 (2), 173–186. doi:10.1007/s11154-019-09494-z
Bahrami, A., Majeed, M., and Sahebkar, A. (2019). Curcumin: a Potent Agent to Reverse Epithelial-To-Mesenchymal Transition. Cell Oncol. 42 (4), 405–421. doi:10.1007/s13402-019-00442-2
Bello, A. K., Levin, A., Tonelli, M., Okpechi, I. G., Feehally, J., Harris, D., et al. (2017). Assessment of Global Kidney Health Care Status. JAMA 317 (18), 1864–81. doi:10.1001/jama.2017.4046
Benigni, A., Perico, L., and Macconi, D. (2016). Mitochondrial Dynamics Is Linked to Longevity and Protects from End-Organ Injury: The Emerging Role of Sirtuin 3. Antioxid. Redox Signaling 25 (4), 185–199. doi:10.1089/ars.2016.6682
Bouchez, C., and Devin, A. (2019). Mitochondrial Biogenesis and Mitochondrial Reactive Oxygen Species (ROS): A Complex Relationship Regulated by the cAMP/PKA Signaling Pathway. Cells 8 (4), 287. doi:10.3390/cells8040287
Chen, Z., An, X., Liu, X., Qi, J., Ding, D., Zhao, M., et al. (2017). Hyperoside Alleviates Adriamycin-Induced Podocyte Injury via Inhibiting Mitochondrial Fission. Oncotarget 8 (51), 88792–88803. doi:10.18632/oncotarget.21287
Diebold, L., and Chandel, N. S. (2016). Mitochondrial ROS Regulation of Proliferating Cells. Free Radic. Biol. Med. 100, 86–93. doi:10.1016/j.freeradbiomed.2016.04.198
El-Hattab, A. W., Craigen, W. J., and Scaglia, F. (2017). Mitochondrial DNA Maintenance Defects. Biochim. Biophys. Acta (Bba) - Mol. Basis Dis. 1863 (6), 1539–1555. doi:10.1016/j.bbadis.2017.02.017
Fang, P., He, B., Yu, M., Shi, M., Zhu, Y., Zhang, Z., et al. (2019). Treatment with Celastrol Protects against Obesity through Suppression of Galanin-Induced Fat Intake and Activation of PGC-1α/GLUT4 Axis-Mediated Glucose Consumption. Biochim. Biophys. Acta (Bba) - Mol. Basis Dis. 1865 (6), 1341–1350. doi:10.1016/j.bbadis.2019.02.002
Fontecha-Barriuso, M., Martin-Sanchez, D., Martinez-Moreno, J., Monsalve, M., Ramos, A., Sanchez-Niño, M., et al. (2020). The Role of PGC-1α and Mitochondrial Biogenesis in Kidney Diseases. Biomolecules 10 (2), 347. doi:10.3390/biom10020347
Gao, Y., Zeng, Z., Li, T., Xu, S., Wang, X., Chen, Z., et al. (2015). Polydatin Inhibits Mitochondrial Dysfunction in the Renal Tubular Epithelial Cells of a Rat Model of Sepsis-Induced Acute Kidney Injury. Anesth. Analgesia 121(5):1251–1260. doi:10.1213/ane.0000000000000977
Gong, W., Li, J., Chen, Z., Huang, J., Chen, Q., Cai, W., et al. (2017). Polydatin Promotes Nrf2-ARE Anti-oxidative Pathway through Activating CKIP-1 to Resist HG-Induced Up-Regulation of FN and ICAM-1 in GMCs and Diabetic Mice Kidneys. Free Radic. Biol. Med. 106:393–405. doi:10.1016/j.freeradbiomed.2017.03.003
Hu, Z.-C., Gong, L.-F., Li, X.-B., Fu, X., Xuan, J.-W., Feng, Z.-H., et al. (2019). Inhibition of PI3K/Akt/NF-Κb Signaling with Leonurine for Ameliorating the Progression of Osteoarthritis: In vitro and In Vivo Studies. J. Cell. Physiol. 234 (5), 6940–6950. doi:10.1002/jcp.27437
Hui, Y., Lu, M., Han, Y., Zhou, H., Liu, W., Li, L., et al. (2017). Resveratrol Improves Mitochondrial Function in the Remnant Kidney from 5/6 Nephrectomized Rats. Acta Histochem. 119 (4), 392–399. doi:10.1016/j.acthis.2017.04.002
Huang, S., Liu, Z., Liu, H., Lee, D., Wang, J., Yuan, R., et al. (2019). Nepeta Angustifolia Attenuates Responses to Vascular Inflammation in High Glucose-Induced Human Umbilical Vein Endothelial Cells through Heme Oxygenase-1 Induction. J. Ethnopharmacology 231, 187–196. doi:10.1016/j.jep.2018.11.015
Huang, S., Tan, M., Guo, F., Dong, L., Liu, Z., Yuan, R., et al. (2020). Nepeta Angustifolia C. Y. Wu Improves Renal Injury in HFD/STZ-induced Diabetic Nephropathy and Inhibits Oxidative Stress-Induced Apoptosis of Mesangial Cells. J. Ethnopharmacology 255, 112771. doi:10.1016/j.jep.2020.112771
Kim, E. N., Lim, J. H., Kim, M. Y., Ban, T. H., Jang, I.-A., Yoon, H. E., et al. (2018). Resveratrol, an Nrf2 Activator, Ameliorates Aging-Related Progressive Renal Injury. Aging 10(1):83–99. doi:10.18632/aging.101361
Leporini, C., Pisano, A., Russo, E., DArrigo, G., de Sarro, G., Coppolino, G., et al. (2016). Effect of Pentoxifylline on Renal Outcomes in Chronic Kidney Disease Patients: A Systematic Review and Meta-Analysis. Pharmacol. Res. 107, 315–332. doi:10.1016/j.phrs.2016.03.001
Li, P. A., Hou, X., and Hao, S. (2017). Mitochondrial Biogenesis in Neurodegeneration. J. Neuro Res. 95 (10), 2025–2029. doi:10.1002/jnr.24042
Li, Z.-l., Hu, J., Li, Y.-l., Xue, F., Zhang, L., Xie, J.-q., et al. (2013). The Effect of Hyperoside on the Functional Recovery of the Ischemic/reperfused Isolated Rat Heart: Potential Involvement of the Extracellular Signal-Regulated Kinase 1/2 Signaling Pathway. Free Radic. Biol. Med. 57, 132–140. doi:10.1016/j.freeradbiomed.2012.12.023
Liu, X., Cao, W., Qi, J., Li, Q., Zhao, M., Chen, Z., et al. (2018). Leonurine Ameliorates Adriamycin-Induced Podocyte Injury via Suppression of Oxidative Stress. Free Radic. Res. 52 (9), 952–960. doi:10.1080/10715762.2018.1500021
Mazat, J.-P., Devin, A., and Ransac, S. (2020). Modelling Mitochondrial ROS Production by the Respiratory Chain. Cell. Mol. Life Sci. 77 (3), 455–465. doi:10.1007/s00018-019-03381-1
Mishra, P., and Chan, D. C. (2016). Metabolic Regulation of Mitochondrial Dynamics. J. Cel. Biol. 212 (4), 379–387. doi:10.1083/jcb.201511036
Nasrallah, R., Hassouneh, R., and Hébert, R. L. (2014). Chronic Kidney Disease: Targeting Prostaglandin E2 Receptors. Am. J. Physiology-Renal Physiol. 307 (3), F243–F250. doi:10.1152/ajprenal.00224.2014
Ni, Z., Tao, L., Xiaohui, X., Zelin, Z., Jiangang, L., Zhao, S., et al. (2017). Polydatin Impairs Mitochondria Fitness and Ameliorates Podocyte Injury by Suppressing Drp1 Expression. J. Cel. Physiol. 232(10):2776–2787. doi:10.1002/jcp.25943
Pedraza-Chaverri, J., Sánchez-Lozada, L. G., Osorio-Alonso, H., Tapia, E., and Scholze, A. (2016). New Pathogenic Concepts and Therapeutic Approaches to Oxidative Stress in Chronic Kidney Disease. Oxidative Med. Cell. longevity 2016, 1–21. doi:10.1155/2016/6043601
Phillips, J., Phillips, I., Enya, B., Zhao, H., and Nitta, T. (2018). Effect of Betulinic Acid and its Ionic Derivatives on M-MuLV Replication. Biochem. Biophysical Res. Commun. 500 (2), 365–369. doi:10.1016/j.bbrc.2018.04.080
Pinegin, B., Vorobjeva, N., Pashenkov, M., and Chernyak, B. (2018). The Role of Mitochondrial ROS in Antibacterial Immunity. J. Cell. Physiol. 233 (5), 3745–3754. doi:10.1002/jcp.26117
Qin, X., Jiang, M., Zhao, Y., Gong, J., Su, H., Yuan, F., et al. (2020). Berberine Protects against Diabetic Kidney Disease via Promoting PGC‐1α‐regulated Mitochondrial Energy Homeostasis. Br. J. Pharmacol., 177, 3646–3661. doi:10.1111/bph.14935
Ríos, J., and Máñez, S. (2018). New Pharmacological Opportunities for Betulinic Acid. Planta Med. 84 (1), 8–19. doi:10.1055/s-0043-123472
Roubicek, D. A., and Souza-Pinto, N. C. d. (2017). Mitochondria and Mitochondrial DNA as Relevant Targets for Environmental Contaminants. Toxicology 391, 100–108. doi:10.1016/j.tox.2017.06.012
Rovira-Llopis, S., Bañuls, C., Diaz-Morales, N., Hernandez-Mijares, A., Rocha, M., and Victor, V. M. (2017). Mitochondrial Dynamics in Type 2 Diabetes: Pathophysiological Implications. Redox Biol. 11, 637–645. doi:10.1016/j.redox.2017.01.013
Schieber, M., and Chandel, N. S. (2014). ROS Function in Redox Signaling and Oxidative Stress. Curr. Biol. 24 (10), R453–R462. doi:10.1016/j.cub.2014.03.034
Spatz, E. S., Wang, Y., Beckman, A. L., Wu, X., Lu, Y., Du, X., et al. (2018). Traditional Chinese Medicine for Acute Myocardial Infarction in Western Medicine Hospitals in China. Circ. Cardiovasc. Qual. Outcomes 11 (3), e004190. doi:10.1161/circoutcomes.117.004190
Srirattana, K., and St. John, J. C. (2019). Transmission of Dysfunctional Mitochondrial DNA and its Implications for Mammalian Reproduction. Adv. Anat. Embryol. Cell. Biol. 231, 75–103. doi:10.1007/102_2018_3
Stefanatos, R., and Sanz, A. (2018). The Role of Mitochondrial ROS in the Aging Brain. FEBS Lett. 592 (5), 743–758. doi:10.1002/1873-3468.12902
Sun, J., Zhang, J., Tian, J., Virzì, G. M., Digvijay, K., Cueto, L., et al. (2019). Mitochondria in Sepsis-Induced AKI. Jasn 30 (7), 1151–1161. doi:10.1681/asn.2018111126
Sun, L.-n., Liu, X.-c., Chen, X.-j., Guan, G.-j., and Liu, G. (2016). Curcumin Attenuates High Glucose-Induced Podocyte Apoptosis by Regulating Functional Connections between Caveolin-1 Phosphorylation and ROS. Acta Pharmacol. Sin 37 (5), 645–655. doi:10.1038/aps.2015.159
Szczepanowska, K., and Trifunovic, A. (2015). Different Faces of Mitochondrial DNA Mutators. Biochim. Biophys. Acta (Bba) - Bioenerg. 1847 (11), 1362–1372. doi:10.1016/j.bbabio.2015.05.016
Tin, A., Grams, M. E., Ashar, F. N., Lane, J. A., Rosenberg, A. Z., Grove, M. L., et al. (2016). Association between Mitochondrial DNA Copy Number in Peripheral Blood and Incident CKD in the Atherosclerosis Risk in Communities Study. Jasn 27 (8), 2467–2473. doi:10.1681/asn.2015060661
Uittenbogaard, M., and Chiaramello, A. (2014). Mitochondrial Biogenesis: a Therapeutic Target for Neurodevelopmental Disorders and Neurodegenerative Diseases. Cpd 20 (35), 5574–5593. doi:10.2174/1381612820666140305224906
Wang, X., Liu, Y., Xiao, L., Li, L., Zhao, X., Yang, L., et al. (2018). Hyperoside Protects Against Pressure Overload-Induced Cardiac Remodelling via the AKT Signaling Pathway. Cell Physiol. Biochem 51 (2), 827–841. doi:10.1159/000495368
Xie, R., Zhang, H., Wang, X.-Z., Yang, X.-Z., Wu, S.-N., Wang, H.-G., et al. (2017). The Protective Effect of Betulinic Acid (BA) Diabetic Nephropathy on Streptozotocin (STZ)-induced Diabetic Rats. Food Funct. 8 (1), 299–306. doi:10.1039/c6fo01601d
Xue, H., Li, P., Luo, Y., Wu, C., Liu, Y., Qin, X., et al. (2019). Salidroside Stimulates the Sirt1/PGC-1α axis and Ameliorates Diabetic Nephropathy in Mice. Phytomedicine 54, 240–247. doi:10.1016/j.phymed.2018.10.031
Zhan, X., Yan, C., Chen, Y., Wei, X., Xiao, J., Deng, L., et al. (2018). Celastrol Antagonizes High Glucose-Evoked Podocyte Injury, Inflammation and Insulin Resistance by Restoring the HO-1-Mediated Autophagy Pathway. Mol. Immunol. 104, 61–68. doi:10.1016/j.molimm.2018.10.021
Zhang, Q.-Y., Wang, Z.-J., Miao, L., Wang, Y., Chang, L.-L., Guo, W., et al. (2019). Neuroprotective Effect of SCM-198 through Stabilizing Endothelial Cell Function. Oxidative Med. Cell Longevity 2019, 1–13. doi:10.1155/2019/7850154
Zhang, X., Liang, D., Lian, X., Jiang, Y., He, H., Liang, W., et al. (2016). Berberine Activates Nrf2 Nuclear Translocation and Inhibits Apoptosis Induced by High Glucose in Renal Tubular Epithelial Cells through a Phosphatidylinositol 3-kinase/Akt-dependent Mechanism. Apoptosis 21 (6), 721–736. doi:10.1007/s10495-016-1234-5
Zhao, D., Sun, X., Lv, S., Sun, M., Guo, H., Zhai, Y., et al. (2019). Salidroside Attenuates Oxidized Lowdensity Lipoproteininduced Endothelial Cell Injury via Promotion of the AMPK/SIRT1 Pathway. Int. J. Mol. Med. 43 (6), 2279–2290. doi:10.3892/ijmm.2019.4153
Zheng, N., Liang, T., Xu, X., Zhao, Z., Liu, J., Song, Z., et al. (2017). Polydatin Impairs Mitochondria Fitness and Ameliorates Podocyte Injury by Suppressing Drp1 Expression. J. Cel. Physiol. 232 (10), 2776–2787. doi:10.1002/jcp.25943
Keywords: chronic kidney disease, herbal medicine, mitochondria, ROS, mitochondrial dysfunction
Citation: Li Q, Xing C and Yuan Y (2021) Mitochondrial Targeting of Herbal Medicine in Chronic Kidney Disease. Front. Pharmacol. 12:632388. doi: 10.3389/fphar.2021.632388
Received: 23 November 2020; Accepted: 20 April 2021;
Published: 26 May 2021.
Edited by:
Ying-Yong Zhao, Northwest University, ChinaReviewed by:
Go Kanzaki, Jikei University School of Medicine, JapanWendy Hoy, The University of Queensland, Australia
Copyright © 2021 Li, Xing and Yuan. This is an open-access article distributed under the terms of the Creative Commons Attribution License (CC BY). The use, distribution or reproduction in other forums is permitted, provided the original author(s) and the copyright owner(s) are credited and that the original publication in this journal is cited, in accordance with accepted academic practice. No use, distribution or reproduction is permitted which does not comply with these terms.
*Correspondence: Changying Xing, Y3l4aW5nNjJAMTI2LmNvbQ==; Yanggang Yuan, eWd5dWFuQG5qbXUuZWR1LmNu
†These authors have contributed equally to this work and share senior authorship