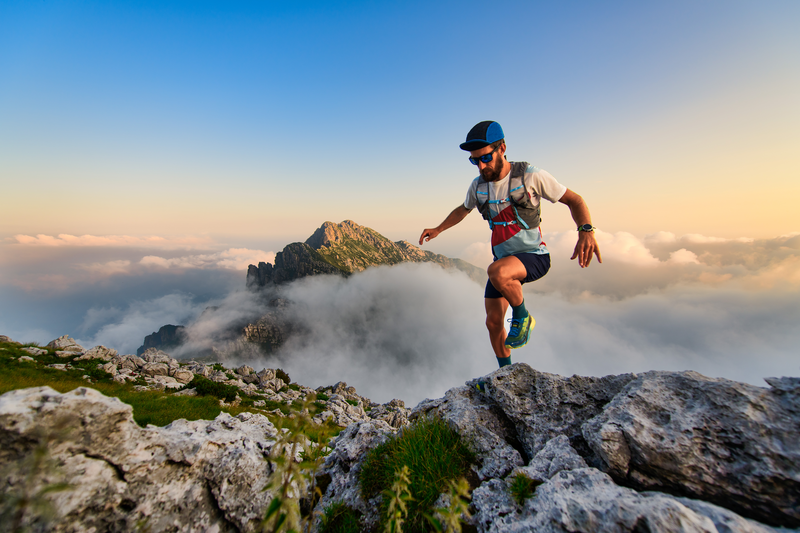
95% of researchers rate our articles as excellent or good
Learn more about the work of our research integrity team to safeguard the quality of each article we publish.
Find out more
REVIEW article
Front. Pharmacol. , 01 September 2021
Sec. Inflammation Pharmacology
Volume 12 - 2021 | https://doi.org/10.3389/fphar.2021.728458
This article is part of the Research Topic New Therapeutic Approaches Against Inflammation and Immune Regulation in Metabolic Related Diseases View all 19 articles
Periodontitis is one of the most prevalent oral inflammatory diseases leading to teeth loss and oral health problems in adults. Periodontitis mainly affects periodontal tissue by affecting the host immune system and bone homeostasis. Moreover, periodontitis is associated with various systemic diseases. Diabetes is a metabolic disease with systemic effects. Both periodontitis and diabetes are common inflammatory diseases, and comorbidity of two diseases is linked to exacerbation of the pathophysiology of both diseases. Since bacterial dysbiosis is mainly responsible for periodontitis, antibiotics are widely used drugs to treat periodontitis in clinics. However, the outcomes of antibiotic treatments in periodontitis are not satisfactory. Therefore, the application of anti-inflammatory drugs in combination with antibiotics could be a treatment option for periodontitis-diabetes comorbidity. Anti-diabetic drugs usually have anti-inflammatory properties and have shown beneficial effects on periodontitis. Sulfonylureas, insulin secretagogues, are the earliest and most widely used oral hypoglycemic drugs used for type-2 diabetes. Studies have found that sulfonylurea drugs can play a certain role in the mitigation of periodontitis and inflammation. This article reviews the effects of sulfonylurea drugs on the mitigation of periodontitis-diabetes comorbidity-related inflammation, bone loss, and vascular growth as well as the involved molecular mechanisms. We discuss the possibility of a new application of sulfonylureas (old drug) to treat periodontitis-diabetes comorbidity.
Periodontitis refers to the chronic inflammation of tooth-supporting tissues. The loss of periodontal attachment, surrounding bone, and teeth are clinical features of periodontitis (Gasner and Schure, 2020). According to the fourth national oral health epidemiological survey, almost 90% of Chinese adults suffered from periodontal disease of various severities and >30% of them had severe periodontitis (Jiao et al., 2021). Periodontitis has a high incidence rate and the prevalence of severe periodontitis in the world has reached approximately 10% (Frencken et al., 2017). In 2019, there were 1.1 billion (95% uncertainty interval: 0.8–1.4 billion) prevalent cases of severe periodontitis globally. From 1990 to 2019, the age-standardized prevalence rate of severe periodontitis increased by 8.44% (6.62–10.59%) worldwide (Chen et al., 2021). Shreds of evidence indicate that periodontitis is associated with many systemic diseases, including cardiovascular disease, type 2 diabetes mellitus (T2DM), rheumatoid arthritis, inflammatory bowel disease (IBD), Alzheimer’s disease, nonalcoholic fatty liver disease, and certain cancers (Hajishengallis and Chavakis, 2021). Periodontitis leads to a 19% increase in the risk of cardiovascular disease (Nazir, 2017). Oral inflammatory diseases aggravate chronic inflammatory lung diseases such as asthma and chronic obstructive pulmonary disease (COPD) (Coffee and Sockrider, 2019). There are many common risk factors between periodontitis and osteoporosis, and the two influence each other (Wang and McCauley, 2016). Periodontitis is also associated with maternal infections, premature birth, low birth weight, and pre-eclampsia (Nazir, 2017). Therefore, periodontitis is not only an oral health problem but has a direct systemic connection and aggravates systemic inflammatory diseases. Due to the unique comorbidities and exacerbation relation between periodontitis and various systemic inflammatory diseases, periodontitis has become a huge public health burden.
Plaque microorganisms play an indispensable role in the pathogenesis of periodontitis, but their existence alone does not mean that periodontitis will inevitably occur. The combined action of some risk factors eventually leads to the occurrence of periodontitis (Darveau, 2010; Hajishengallis, 2014). In periodontitis, oral microbiome dysbiosis triggers host response inducing inflammatory mediators in oral tissues. This effect causes the periodontitis and oral tissue degeneration. Oral bacteria and inflammatory mediators enter the circulation exerting systemic effects. Moreover, oral microbiome can directly enter to oropharyngeal and orogastrointestinal route that further causes systemic effect as indicated in Figure 1. Over the past 30 years, a large number of research results have proved the host’s defense response, or the host’s susceptibility to periodontal disease as a necessary condition to develop disease (Darveau, 2010). The multi-microbial synergy and biological disorders of susceptible hosts are the initiation events of periodontitis. The host’s defense barrier and defense cells have anti-inflammatory or pro-inflammatory. As the host’s first line of defense against pathogenic microorganisms, neutrophils not only engulf bacteria through their Fc receptor-binding antibodies but also release collagenase, which has a destructive effect on tissues (Hajishengallis, 2020). Porphyromonas gingivalis, the main pathogen of periodontitis, polarizes macrophages to the M1 phenotype and their penetration into the gum tissue also leads to alveolar bone resorption (Zhu et al., 2019). Compared with healthy tissues, the proportion of monocytes observed in periodontitis tissue is significantly higher, and these monocytes overexpress human leukocyte antigen-DR (HLA-DR) and programmed cell death 1 ligand-1 (PDL-1) molecules, indicating that monocytes are in an inflammatory state during periodontitis. The ratio of M1/M2 macrophages is also significantly higher at the site of periodontal infection, indicating an imbalance between inflammation and repair mechanisms (Almubarak et al., 2020). Actinobacillus actinomycetemcomitans, a Gram-negative anaerobic microorganism that causes periodontitis in humans, activates CD4+ cells in periodontitis tissues and cause local alveolar bone destruction (Teng et al., 2000). Immune cells release a large number of cytokines, such as interleukin-1β (IL-1β), IL-6, IL-8, tumor necrosis factor-α (TNF-α), prostaglandin-E2 (PGE2), matrix metalloproteinases (MMPs), interferon-γ (IFN-γ), and receptor activator of nuclear factor-κB ligand (RANKL) in response to bacterial invasion. These cytokines respective effects causing tissue damage to the periodontal region (Ramadan et al., 2020). Under physiological conditions, bone remodeling is the result of coordination between bone formation and resorption. The balance between osteoblast-mediated bone formation and osteoclast-mediated bone resorption ensures bone homeostasis. In an inflammatory state, excessive activation of the immune system and increased pro-inflammatory factors promote RANKL-mediated osteoclast formation, leading to bone resorption (Jung et al., 2014; Renn et al., 2018). At the same time, the host’s susceptibility factors, including genetic factors, endocrine, immune function, psychological regulation, and certain diseases such as diabetes, are important in the development and aggravation of periodontitis (Badran et al., 2015). Both diabetes and periodontitis are systemic inflammatory diseases that accelerate the development and progression of each other. Comorbidity of the two diseases is associated with poor health and quality of life.
Diabetes is a chronic metabolic disease. In the past few decades, the prevalence of diabetes has increased significantly in the world. More than 422 million adults worldwide had diabetes in 2014 (Collaboration, 2016). Diabetes is categorized as type 1 diabetes mellitus (T1DM), T2DM, and pregnancy diabetes. T1DM is an autoimmune disease characterized by the destruction of pancreatic β-cells. T2DM is characterized by insulin resistance and impaired insulin secretion. The ability of T2DM patients to produce insulin in the body is not completely lost, and this type of diabetes treated with certain oral drugs that stimulate insulin secretion in the body. Gestational diabetes refers to a situation where women without a history of diabetes have elevated blood sugar during pregnancy. In this case, the baby has a high risk of developing diabetes in adulthood (Lovic et al., 2020). The global burden of diabetes is increasing with the increasing incidence rate of the disease.
The prevalence of diabetes-affected people worldwide is expected to be 642 million by 2040 (Ogurtsova et al., 2017). Periodontitis is closely related to the progression of diabetes. There was sufficient evidence in the mid-1990s that regards periodontitis as the sixth complication of diabetes (Loe, 1993). New consensus indicates periodontal disease and diabetes as comorbidity (American Diabetes, 2018). Periodontitis is closely related to the aggravation of diabetes and T2DM is regarded as the risk factor of periodontitis (Chávarry et al., 2009). Similarly, the severity of periodontitis affects the blood sugar control of diabetic patients and exacerbates diabetes-related complications (Lalla and Papapanou, 2011). Multiple meta-analysis studies have shown that periodontal treatment alone can have a beneficial effect on glycemic control (Simpson et al., 2010; Teeuw et al., 2010; Sgolastra et al., 2013). On the other hand, anti-diabetic drugs not only play an indirect role in treating inflammation due to diabetes control but also have a direct anti-inflammatory effect (Kothari et al., 2016). The anti-inflammatory properties of anti-diabetic drugs have the potential to ameliorate periodontitis. At the same time, prospective studies had shown that patients with moderate or severe periodontitis have a significantly increased risk of diabetes in the future (Morita et al., 2012). Therefore, the exploration of a drug that can treat periodontitis-diabetes comorbidity could be of clinical significance.
As aforementioned, the clinical observation that periodontitis and diabetes can interact with each other is well researched. The mechanism of the association between these two diseases is still under study, but there are some accepted theories. It is generally believed that diabetes affects periodontitis in four ways. Diabetes causes a hyperinflammatory response to infection, oxidative stress, bone metabolism disorders, and increased expression of advanced glycation end products (AGEs) and their receptors (RAGE) (Lalla and Papapanou, 2011). Hyperglycemia predisposes to exaggerated inflammatory response, primes leukocytes for marginalization and superoxide production, as well as increases the free radical load in the gingival microvasculature (Gyurko et al., 2006). Cytokine dysregulation also represents the mechanism by which bacteria may induce destructive inflammatory responses in diabetic individuals (Naguib et al., 2004). Hyperglycemia can modulate the ratio of RANKL to osteoprotegerin (OPG) in periodontal tissue (Wu et al., 2014). Increased the ratio of RANKL/OPG and the secretion of AGEs, reactive oxygen species (ROS), TNF-α, IL-1β, and IL-6 in diabetes enhance periodontal ligament (PDL) and osteoblast apoptosis, induces osteoclastogenesis, and reduces bone formation (Pacios et al., 2013; Wu et al., 2015). AGEs increase IL-6 and intercellular adhesion molecule-1 (ICAM-1) expression via the RAGE, MAPK, and nuclear factor-κB (NF-κB) pathways in human gingival fibroblasts and exacerbate the progression of the pathogenesis of periodontal diseases (Nonaka et al., 2018). The AGE-RAGE interaction induces the production of ROS, the production of inflammatory cytokines such as TNF-α, and the activation of NF-κB (Brownlee, 2001). AGE-RAGE also serves as a promoting factor to regulate osteoclast formation (Ding et al., 2006).
Oxidative stress plays a critical role in diabetes complications. Inflammation induced by increased intracellular ROS also contributes to diabetes complications (Pitocco et al., 2010). The upregulation of ROS play important role in the establishment and progression of periodontitis through the development of oxidative stress (Sczepanik et al., 2020). The increase in superoxide production activates the increase in AGE formation and RAGE expression, as well as the protein kinase C (PKC) and hexosamine pathways. Through these pathways, increased intracellular ROS cause defective angiogenesis in response to ischemia activates many proinflammatory pathways (Giacco and Brownlee, 2010). Regarding the effect of periodontitis on diabetes, it is generally believed that periodontitis induced periodontal microbiota dysbiosis. The disease concomitantly impacts the regional and systemic immune response impairing glucose metabolism (Su et al., 2013; Ilievski et al., 2015; Huang et al., 2016; Blasco-Baque et al., 2017). Inflammation markers such as c-reactive protein (CRP) and IL-6 are involved in insulin resistance, which positively correlates with the progress of diabetes (Pradhan et al., 2001). NAcht Leucine-rich repeat protein 3 (NLRP3), a pathogen recognition molecule, activates caspase-1. Activated caspase-1 cleaves and promotes the maturation of IL-1β that induces pancreatic inflammation, β-cell death, T2DM progression, and insulin-dependent diabetes development (Zhou et al., 2010). NLRP3 inflammasome-mediated effector adipose tissue T cells activation also leads to a decrease in insulin sensitivity. These cells regulate insulin resistance by releasing IFN-γ (Vandanmagsar et al., 2011). Pieces of literature had indicated that periodontitis causes an increase in systemic oxidative stress which could influence diabetes (Allen et al., 2011; Bastos et al., 2012). In addition, the microbiota plays a critical role in the connection between diabetes and periodontitis. T2DM patients are more susceptible to subgingival microbiome dysbiosis, potentially due to impaired host metabolic and immune regulation (Shi et al., 2020). Hypoglycemic therapy reconstructs the salivary microbiota of diabetes patients (Sun et al., 2020). Periodontitis affects diabetes through oral and gut microbiota dysbiosis. Gut microbial shifting driven by periodontitis contributes to systemic inflammation and metabolic dysfunction during the progression of T2DM (Li et al., 2020). Effective periodontal therapy reduced insulin resistance and improved periodontal health status and insulin sensitivity in patients with T2DM and chronic periodontitis (Mammen et al., 2017). The anti-diabetic drugs not only play an indirect role in treating inflammation due to diabetes control but also have a direct anti-inflammatory effect (Kothari et al., 2016). Figure 2 illustrates the mechanisms of two-way relationship of periodontitis and diabetes that intensify the severity of both diseases. Antibacterial drugs are often used as adjuvant treatments for patients with diabetes and periodontitis. A prospective clinical study showed the adjunct of doxycycline (Doxy) to conventional periodontal therapy provides an additional benefit in reducing the glycemic level and improves periodontal health (Das et al., 2019). Photodynamic therapy + Doxy possesses the best efficacy in lowering HbA1c% of non-smoking chronic periodontitis patients with diabetes (Cao et al., 2019). The adjunctive use of systemic metronidazole and amoxicillin improves the microbiological and clinical outcomes of scaling and root planing in subjects with chronic periodontitis and T2DM (Tamashiro et al., 2016). Adjunctive omega-3 polyunsaturated fatty acids (ω-3 PUFA) and low-dose aspirin (ASA) after periodontal debridement provides clinical and immunological benefits to periodontitis patients with T2DM (Castro Dos Santos et al., 2020). Metformin, a traditional hypoglycemic drug, has been found to have anti-inflammatory effects. Metformin reduces the activity of NLRP3 inflammasomes by inhibiting the expression of Nek7, and significantly reduces experimental diabetic periodontitis in vivo and in vitro (Zhou et al., 2019). Hypoglycemic therapy has the potential to reconstruct the salivary microbiota and improve the localized conditions of diabetes patients with periodontitis (Sun et al., 2020). There are also some uncommon drugs used to treat periodontitis-diabetes comorbidities. A double-blind clinical trial study showed that melatonin supplementation in adjunct with non-surgical periodontal therapy improves inflammatory and periodontal status in T2DM with chronic periodontitis (Bazyar et al., 2019). Locally delivered atorvastatin was found to be effective in the treatment of intrabony defects in chronic periodontitis patients with T2DM (Kumari et al., 2016). Propolis can also be used as an adjuvant drug for scaling and root planning that significantly reduces the levels of fasting plasma glucose, and serum N-(carboxymethyl) lysine, and improves the periodontal treatment effect in patients with T2DM and chronic periodontitis (El-Sharkawy et al., 2016). The above clinical studies have not clarified whether the treatment effects are through unilateral benefit to diabetes or periodontitis that indirectly affects the other side. However, it is predictable that the drugs that mitigate the inflammation or reconstruct the microbiome could be beneficial to treat periodontitis-diabetes comorbidity.
FIGURE 2. The mechanisms of the two-way relationship of periodontitis and diabetes. AGE/RAGE: advanced glycation end product/receptor of advanced glycation end products.
Sulfonylurea is currently one of the most common diabetes drugs. French scientist Marcel J. Janbon discovered the hypoglycemic effect of sulfa drugs in 1842. Since then, sulfonylurea has been extensively researched and developed to treat diabetes (Loubatieres-Mariani, 2007). Sulfonylurea drugs have been developed into more than ten kinds of oral hypoglycemic drugs, which are usually divided into two generations. Various sulfonylureas are derived by changing the nitrogen of the urea group and the para substituent of the benzene ring structure (Figure 3). In the first generation of sulfonylurea drugs chlorpropamide, tolbutamide, tolazamide, acetohexamide, and carbutamide the urea substituents are smaller and more polar, thus making the aryl-sulfonylurea more soluble in water. In the second generation of sulfonylurea drugs glyburide, glipizide, Gliclazide, glisoxepide, and glimepiride the substituents are large with nonpolar lipophilic groups that are easier to penetrate cell membranes and are therefore more effective (Doyle and Egan, 2003). The pancreatic β-cell membrane contains sulfonylurea receptors coupled with adenosine triphosphate (ATP)-sensitive potassium current IK(ATP) as well as voltage-dependent calcium channels. After binding with receptors sulfonylurea drugs block IK(ATP) and prevent potassium outflow, resulting in depolarization of cell membranes, enhancing voltage-dependent calcium channel opening, and extracellular calcium influx. The increased intracellular free calcium concentration triggers exocytosis and insulin release (Ashcroft and Rorsman, 2012; Lv et al., 2020). Therefore, sulfonylurea drugs only exert an effect on patients with T2DM who still have functional pancreatic β-cells in the body.
Periodontitis-related systemic diseases are likely induced by three ways: hematogenous dissemination of periodontal bacteria, transfer of inflammatory mediators from periodontal tissue to blood, and oropharyngeal or oral-intestinal transfer of periodontal bacteria (Figure 1) (Teles and Wang, 2011; Mammen et al., 2020). Structural equation modeling was used to evaluate periodontal treatment effects on oral and systemic inflammation, and researchers found that diabetes and periodontitis together appear to increase systemic inflammation, with evidence of inflammation reduction following periodontal treatment (Preshaw et al., 2020). Diabetes increases inflammation in the periodontium, which causes changes in bacterial composition. Diabetes results in increased inflammation reflected by an increase in leukocytes and increased cytokine expression as well as a change in bacterial composition that enhances the overall pathogenicity of the microbiota (Graves et al., 2020).
Hematopoietic stem cells and progenitor cells sense systemic infections or inflammation in the bone marrow through inflammatory cytokine receptors, thereby differentiate into infection-resistant cells such as neutrophils and monocytes (Teitelbaum and Ross, 2003). This process relies on IL-6 serum levels, which increase during Porphyromonas gingivalis (Zhao et al., 2020). Monocytic lineage is the precursor of osteoclasts and differentiates into osteoclasts by further stimulation of macrophage colony-stimulating factor (M-CSF) and RANKL (Teitelbaum and Ross, 2003). Diabetes increases the levels of glucose, AGEs, and ROS in periodontal tissues leading to increased inflammation. Intensified inflammation further impacts the oral microbiota dysbiosis and stimulates periodontal ligament fibroblasts, osteoblasts, and osteocytes to produce pro-osteoclastogenic factors. The inflammation also affects these cells to reduce bone formation, resulting in greater net bone loss (Figure 4) (Graves et al., 2020).
FIGURE 4. Periodontitis-diabetes comorbidity induces host response triggering the release of inflammatory mediators form variety of cells including immune cells. Elevated levels of inflammatory mediators cause both local and systemic effects. Inflammatory mediator-induced osteoclastogenesis and compromised osteoblast function are the main causes of excessive alveolar bone loss in periodontitis-diabetes comorbidity. AGEs: advanced glycation end products; ROS: reactive oxygen species; OB: osteoblasts, OCP: osteoclast precursors; OC: osteoclasts; DC: dendritic cells; Mac: Macrophages.
Vascular inflammation is attributed to periodontitis-induced activation and adhesion of circulating monocytes to aortic endothelial cells via NF-κB/p65 nuclear translocation and upregulation of VCAM1 (Miyajima et al., 2014). Local treatment of periodontitis attenuates vascular inflammation and atherosclerosis in experimental models of periodontitis (Hasturk et al., 2015). Porphyromonas gingivalis is the most abundant bacterial species detected in non-diseased vascular tissue of patients with atherosclerosis (Mougeot et al., 2017). Gingipains are important for the virulence of Porphyromonas gingivalis in extra-oral sites. Porphyromonas gingivalis induces edema by increasing vascular permeability, which is attributed to gingipain-dependent degradation of platelet endothelial cell adhesion molecule (PECAM1) and vascular endothelial cadherin disrupting the endothelial barrier (Farrugia et al., 2021). Periodontitis-associated insulin resistance and hyperglycemia also induce the formation of AGEs, which is involved in several metabolic disorders, including vascular inflammation (Chavakis et al., 2003), and atherosclerosis (Ruiz et al., 2020) in experimental periodontitis in diabetic mice (Figure 5) (Lalla et al., 2000).
FIGURE 5. The mechanisms involved in periodontitis-diabetes comorbidity-mediated vascular inflammation and atherosclerosis. Pg: Porphyromonas gingivalis; EC: endothelial cells; RAGE: receptor of advanced glycation end products.
Sulfonylureas have shown the potential to alleviate inflammation. Insulin stimulation leads to the activation of different pathways involved in metabolic regulation, including the phosphatidylinositol-3-kinase (PI3K) cascade (Kumar and Dey, 2002). Compared to the healthy gingival tissue, PI3K expression is increased in periodontal tissues affected by periodontitis (Ozcan et al., 2017) (Sarkar et al., 2012). Gliclazide reduces oxidative stress, blocks PI3K signal transmission, and reduces matrix metalloproteinase 2 (MMP-2), cathepsin K, and RANKL levels in the rat periodontitis model. Gliclazide treatment alleviates periodontitis and alveolar bone resorption (Araújo et al., 2019).
MMPs, as a class of zinc-dependent endopeptidases, are produced in large quantities when host reactions occur in a variety of pathological conditions. MMPs degrade extracellular matrix and basement membrane destroying periodontal tissue (Sorsa et al., 2006). The researchers had reported the elevated levels of MMP in the serum of patients with inflammatory periodontal tissue and gingival crevicular fluid of periodontitis patients (Silosi et al., 2015). Glyburide has been reported to reduce plasma MMP-9 levels (Sheth et al., 2016). Reducing the elevated MMP levels could mitigate the periodontitis-induced oral tissue destruction. However, the ability of various sulfonylureas to reduce the MMPs level during periodontitis still needs to be investigated.
Macrophages and monocytes are key regulators of the inflammatory process. During inflammation, IL-1β is mainly secreted by mononuclear cells and macrophages (Koh and DiPietro, 2011). Cryopyrin/NALP3/NLRP3 is an essential component of inflammasomes triggered by microbial ligands, danger-associated molecular patterns (DAMPs), and crystals. Glyburide is the first identified compound to prevent cryopyrin activation and microbial ligand-, DAMPs-, and crystal-induced IL-1β secretion (Lamkanfi et al., 2009; Masters et al., 2010). Nine different sulfonylureas have been reported to inhibit the activation of NLRP3 in mouse bone marrow-derived macrophages in a dose-dependent manner. Nano molar concentration of MCC950, glyburide, sulofenur, glimepiride, gliquidone, or glisoxepide inhibits NLRP3 activation and stimulates insulin secretion in mouse pancreatic cells, indicating the dual effects (Hill et al., 2017). Chronic inflammation often causes delayed wound healing in diabetes. Mitigation of inflammation is part of the treatment of diabetes-related complications. Glyburide promotes the inflammatory regulator-140 receptor-interacting protein ubiquitin degradation by activating Ca2+/calmodulin dependent protein kinase II (CamKII) that leads to anti-inflammatory M2 macrophage polarization (Lin et al., 2018). Glyburide and glimepiride have been shown to inhibit the release of IL-1β in macrophage-like THP-1 cells by targeting the activity of NLRP3 inflammasomes. Similarly, the rat model of periodontitis has shown the inhibitory effect of glyburide on inflammatory cell infiltration and alveolar bone osteoclast formation during the development of periodontitis (Kawahara et al., 2020). Glyburide inhibits NLRP3 formation, and induces the expression of pro-healing growth factors insulin-like growth factor-1 (IGF-1), TGF-β and IL-10, thereby promotes wound healing in diabetic mice (Mirza et al., 2014).
CRP is an acute-phase reactive protein and a non-specific marker of inflammation. Cross-sectional studies have shown that periodontitis and poor oral hygiene are associated with elevated serum CRP levels (Torrungruang et al., 2019). Glyburide reduces CRP level during treating diabetes (Putz et al., 2004). Clinical studies have shown that gliclazide reduces soluble ICAM-1 (sICAM-10, serum soluble E-selectin (sE-selectin), and hsCRP in diabetic patients (Räkel et al., 2007). Moreover, gliclazide blocks insulin-mediated neutrophil migration through endothelial cells and the expression of PECAM-1 by inhibiting the activation of MAPK (Okouchi et al., 2004).
There is a clear connection between diabetes and inflammation, and sulfonylurea drugs have obvious positive effects on inflammation mitigation. The literature had reported the various mechanisms of action of different sulfonylurea drugs during inflammation mitigation (Table 1).
Periodontitis eventually destroys periodontal tissues and alveolar bone leading to tooth looseness and even loss. The main reason for alveolar bone loss in periodontitis is the imbalance of bone metabolism, mainly due to the increased osteoclasts’ number and activity (Straka et al., 2015). Activation of nuclear factor-kappa B receptor activator (RANK) signaling in osteoclasts precursors during bacterial infection is a key driver of enhanced osteoclast number and activity in periodontitis. Bacterial components induce the production of proinflammatory cytokines TNF-α and IL-1β, which enhance the activation of RANK by RANKL and down-regulating OPG signaling (Darveau, 2010).
In the rat periodontitis model, oral administration of glyburide significantly inhibits the infiltration of inflammatory cells and osteoclasts in the periodontal tissue (Kawahara et al., 2020). MCC950, a diarylsulfonylurea compound, which is the most specific inhibitor of NLRP3 inflammasome (Laliberte et al., 2003), inhibits the classical and non-classical pathways of NLRP3 inflammasome activation (Coll et al., 2015). The administration of MCC950 prevents alveolar bone loss in aging mice, but not in young mice. In vitro studies have shown that MCC950 treatment directly inhibits osteoclast differentiation (Zang et al., 2020). These effects are achieved through the inhibition of NLRP3 inflammasome and IL-1β expression by sulfonylurea drugs. Table 2 summarizes the effect of sulfonylureas on bone metabolism.
Sulfonylurea drugs stimulate pancreatic β-cells to increase insulin secretion. Insulin is a bone anabolic factor that acts through insulin receptor substrate (IRS) signaling and glucose uptake. In vivo studies suggest that the absence of IRS gene decreases bone mass, reduces osteoblast/osteoclast function, and impairs bone turnover (Ogata et al., 2000). IRS-2 signal maintains the tendency of bone formation to exceed bone resorption, and IRS-1 signal maintains bone renewal. The integration of these two signals leads to strong bone anabolic effects of insulin and IGF-1 (Akune et al., 2002). The reduction of bone mass in most diabetic patients is related to the function of β-cells. Moreover, in vitro studies have shown that insulin regulates bone metabolism by stimulating osteoblast proliferation and inhibiting osteoclast activity (Kalaitzoglou et al., 2019). Therefore, the sulfonylureas that can stimulate insulin secretion may also play a role in making bone metabolism tend to the direction of bone formation.
Many studies have proven that bone metabolism and energy metabolism are mutually regulated by closely related mechanisms. OPG induces islet β-cell replication by regulating CREB and GSK3 pathways (Kondegowda et al., 2015). Under the same pathological conditions, hyperglycemia has various adverse effects on glucose metabolism. Diabetes affects bone through glucose metabolism disorders, destruction of bone microvascular function, and muscle endocrine function, which is also a unique bone feature in diabetes (Lecka-Czernik, 2017). Hyperglycemia interferes with the proliferation and mineralization of osteoblasts. Studies have shown that hyperglycemia inhibits the PI3K/Akt/eNOS pathway in rat osteoblasts, thereby interfering with the osteoblast transcription factors such as RUNX2, which have an important effect on osteoblast differentiation. Glimepiride stimulates the proliferation and differentiation of rat osteoblasts through the PI3K/Akt/eNOS signaling pathway (Ma et al., 2014). Glimepiride reverses the inhibitory effect of high glucose on the PI3K/Akt/eNOS pathway, enhances osteoblast proliferation, expression of RUNX2, osteocalcin, and alkaline phosphatase mRNA, and protects rat mandibular osteoblast-apoptosis from high concentrations of glucose (Ma et al., 2011).
Studies have shown that glimepiride alleviates the skeletal damage caused by estrogen deficiency in ovariectomized rats and promotes bone formation (Fronczek-Sokol and Pytlik, 2014). There is also a gender-wise difference in the effect of glyburide on bone metabolism. The results of clinical sampling and the diabetes endpoint progression test showed that after glyburide treatment, bone resorption marker type I collagen c-terminal peptide (CTX) is significantly reduced in women but not in men. Glyburide alters the bone formation markers: type 1 procollagen amino-terminal peptide (P1NP), and bone alkaline phosphatase (bsALP). The effect of glyburide had been reported as changes of +0.2% P1NP in males and −11.6% bsALP in females (Zinman et al., 2010).
There is still considerable disagreement about the association of sulfonylurea drugs with fracture risk. Some studies have shown that compared with thiazolidinediones, sulfonylurea-treated patients have a lower fracture risk (Vestergaard et al., 2005; Dormuth et al., 2009; Solomon et al., 2009), and the same results have been obtained in cross-sectional surveys in postmenopausal populations (Kanazawa et al., 2010). However, some studies have found that there is no significant correlation between sulfonylurea treatment and fracture risk (Melton et al., 2008; Colhoun et al., 2012). These controversial results may be due to differences in study design, patient age/sex, drug concentration, and treatment duration. Multicenter clinical studies using the same protocol are needed to confirm the inconsistent outcome of these studies.
Angiogenesis is an important process that occurs under physiological and pathological conditions. Inflammatory diseases are one of the angiogenesis-dependent diseases. Inflammation-induced angiogenesis affects many diseases, and periodontitis is one of them (Carmeliet, 2003). The formation of blood vessels is strictly regulated and this process involves the synergistic effect between various cells, cytokines, growth factors, and extracellular matrix components (Andreuzzi et al., 2017). Among them, vascular endothelial growth factor (VEGF) is the essential factor for vascular endothelium, which is also an important factor in the pathogenesis of invasive periodontitis and chronic periodontitis (Unlü et al., 2003). Gingival tissue, gingival crevicular fluid, and serum show a high expression trend of VEGF in periodontitis (Noguchi et al., 2011; Pradeep et al., 2011). VEGF also interacts with factors that regulate bone homeostasis and bone development, affecting bone metabolism (Peng et al., 2002). Under high glucose conditions, VEGF inhibits insulin secretion of pancreatic β-cells, sulfonylurea receptor expression, and inwardly rectifying potassium channel gene 6.2 (Kir6.2) expression (An et al., 2017). Gliclazide reduces protein kinase C (PKC) translocation, extracellular signal-regulated protein kinase 1/2, and NF-κB induced by AGEs in the bovine retinal endothelial cell (BREC), inhibiting BREC proliferation and VEGF expression (Mamputu and Renier, 2004).
H2S and C-type Natriuretic Peptide (CNP) also act as vasoactive agents and induce angiogenic responses. H2S enhances EC migration through a KATPchannel/p38/hsp27 pathway. Blockade of KATP channels in ECs by glibenclamide blocked H2S-induced EC migration (Papapetropoulos et al., 2009). Similarly, CNP mediates angiogenesis via KATP activation. Subsequent research pointed out that ATP-sensitive potassium channel activation induces angiogenesis effects in vitro and in vivo (Khambata et al., 2011). KATP activation and the expression of the Kir6.1 KATP subunit may underpin angiogenesis to a variety of vasoactive stimuli, including H2S, VEGF, and CNP (Umaru et al., 2015). Sulfonylurea drugs may have inhibitory effects on angiogenesis via targeting Kir6.1 KATP subunit.
The effects of sulfonylurea drugs on angiogenesis have been proven by multiple animal experiments. Table 2 summarizes the effect of sulfonylureas on vascular functions. Male Wistar rats treated with low-dose glyburide simulate stroke after middle cerebral artery occlusion treatment. Histological evaluation showed that glyburide treatment enhances angiogenesis in the hippocampus (Ortega et al., 2013). Under the stimulation of angiogenesis, MMP-9 degrades multimerin 2 (MMRN2). The decreased MMRN2 acts as a negative feedback regulator to promote the expression of VEGFA and angiogenesis (Andreuzzi et al., 2017). In periodontitis, MMP-9 is up-regulated in serum and gingival crevicular fluid (Yang et al., 2019). Intravenous glyburide shows a decrease in plasma MMP-9 during a clinical study on the safety and efficacy of glyburide in the treatment of brain swelling after cerebral infarction (Sheth et al., 2016). These reports suggest that glyburide may have an inhibitory effect on angiogenesis by reducing the MMP-9 and modulating MMRN2/VEGFA pathway.
Anti-diabetes drugs also have a direct effect on tumor growth. The second-generation sulfonylurea glipizide has obvious vascular inhibitory effects and inhibits tumor-induced angiogenesis by up-regulating natriuretic peptide that inhibits tumor growth and metastasis (Qi et al., 2014; Qi et al., 2016). The results of the studies on the effects of sulfonylurea drugs on angiogenesis are not consistent. Some studies have shown that glyburide inhibits vascular function. However, the anabolic effect of sulfonylurea drugs on vascular function cannot be denied (Matsuzawa et al., 2015). Different sulfonylurea drugs have different effects on vascular function. The different study models and drugs used might be responsible for the contradictory results.
Gliclazide attenuates oxidative stress induced by high glucose in human umbilical vein endothelial cells and protect vascular endothelial cells from apoptosis (Corgnali et al., 2008). Gliclazide also reduces the adhesion of monocytes and endothelial cells induced by oxidized low-density lipoprotein (oxLDL) and AGEs in vitro. This effect reduces the ability of monocytes adhesion in the blood vessel walls and the continuous inflammatory process that occurs in atherosclerotic plaques (Renier et al., 2003). Similar events might occur during periodontitis. However, future studies are needed to support this hypothesis.
Glimepiride induces the production of nitric oxide (NO) in human coronary artery endothelial cells by activating PI3K/Akt, and more NO release will play a role in vasodilation (Ueba et al., 2005). Gliclazide reduces oxidative stress in patients with T2DM by improving plasma antioxidant status, and this effect is also related to NO-mediated vasodilation enhancement (Fava et al., 2002). Sulfonylurea receptors are also present in vascular smooth muscle cells (VSMC), and KATP channels play an important role in the proliferation and migration of VSMC induced by glyburide and glimepiride. In contrast, gliclazide has an inhibitory effect on VSMC, which makes gliclazide a potential therapeutic to prevent vascular obstructive diseases in T2DM (Zhang et al., 2019).
In summary, sulfonylurea drugs have multiple therapeutic potential other than the traditional treatment of diabetes. The occurrence and development of periodontal disease are closely related to inflammatory factors, bone metabolism balance, and neovascularization. Affected by systemic metabolic changes, periodontitis combined with diabetes aggravates the pathology of both diseases. Sulfonylurea drugs alleviate periodontitis possibly via direct mitigation of inflammation or indirectly via anti-diabetic effect (Figure 6). The above-mentioned studies indicated that sulfonylurea drugs are likely to have a therapeutic effect by directly acting on periodontitis suggesting its possible application to treat periodontitis alone. Moreover, sulfonylureas can be used as a “one stone two birds” to treat periodontitis-diabetes comorbidity. However, more in vitro and in vivo studies are needed to test the efficacy of sulfonylureas on periodontitis or periodontitis-diabetes comorbidity and to unravel the underlying mechanisms of action.
FIGURE 6. Periodontitis, diabetes, and periodontitis-diabetes comorbidity affects various local and systemic functions, and sulfonylureas had shown potential to alleviate those effects. SUs: sulfonylureas; ECs: endothelial cells.
JP and MM: study design and manuscript final editing; LY and QG: literature survey and manuscript writing; ZY, LjW, and LpW: Figure preparation and manuscript editing.
This study was supported by the project of Guangzhou Science and Technology Bureau (202002030301), Department of Education of Guangdong Province (2018KTSCX186), and highlevel university construction funding of Guangzhou Medical University (02-412-B205002-1003018, 02-410-B205001293, B185006003014, B195002003017, and 02-412-B205002-1003017). The funding bodies played no role in the design of the study and collection, analysis, and interpretation of data, and in writing the manuscript.
The authors declare that the research was conducted in the absence of any commercial or financial relationships that could be construed as a potential conflict of interest.
All claims expressed in this article are solely those of the authors and do not necessarily represent those of their affiliated organizations, or those of the publisher, the editors and the reviewers. Any product that may be evaluated in this article, or claim that may be made by its manufacturer, is not guaranteed or endorsed by the publisher.
PI3K, phosphatidylinositol-4,5-bisphosphate3-kinase; MMP, matrix metalloproteinase; PECAM-1, platelet endothelial cell adhesion molecule-1; MAPK, mitogen-activated protein (MAP) kinase; sICAM-1, soluble intercellular adhesion molecule-1; hsCRP, high-sensitivity C-reactive protein; SUR1-TRPM, sulfonylurea receptor 1-transient receptor potential melastatin 4; NLRP3, NLR family pyrin domain containing 3; THP-1, human monocytic leukemia cell line; BMDM, bone marrow-derived macrophages; Ca2+/CamKII, Ca2+/calcium calmodulin-dependent kinase II; IGF-1, insulin-like growth factor 1; TGF-β, transforming growth factor-β; IL-10, interleukin-10; HCAECs, human coronary artery endothelial cells; HASMCs, human aortic smooth muscle cells; VSMC, vascular smooth muscle cell; VEGF, vascular endothelial growth factor; MCAO, middle cerebral artery occlusion; KATP, ATP sensitive potassium (K) channel; oxLDL, oxidized low-density lipoprotein; AGE, advanced glycation end-products; PKC, protein kinase C; MAPK, mitogen-activated protein kinase; NF-κB, nuclear factor kappa B; HUVECs, human umbilical vein endothelial cells; ECs, endothelial cells
Akune, T., Ogata, N., Hoshi, K., Kubota, N., Terauchi, Y., Tobe, K., et al. (2002). Insulin Receptor Substrate-2 Maintains Predominance of Anabolic Function over Catabolic Function of Osteoblasts. J. Cel Biol 159, 147–156. doi:10.1083/jcb.200204046
Allen, E. M., Matthews, J. B., O' Halloran, D. J., Griffiths, H. R., and Chapple, I. L. (2011). Oxidative and Inflammatory Status in Type 2 Diabetes Patients with Periodontitis. J. Clin. Periodontol. 38, 894–901. doi:10.1111/j.1600-051X.2011.01764.x
Almubarak, A., Tanagala, K. K. K., Papapanou, P. N., Lalla, E., and Momen-Heravi, F. (2020). Disruption of Monocyte and Macrophage Homeostasis in Periodontitis. Front. Immunol. 11, 330. doi:10.3389/fimmu.2020.00330
American Diabetes, A. (2018). 3. Comprehensive Medical Evaluation and Assessment of Comorbidities: Standards of Medical Care in Diabetes-2018. Diabetes Care 41, S28–S37. doi:10.2337/dc18-S003
An, S. Y., Lee, Y. J., Neupane, S., Jun, J. H., Kim, J. Y., Lee, Y., et al. (2017). Effects of Vascular Formation during Alveolar Bone Process Morphogenesis in Mice. Histochem. Cel Biol 148, 435–443. doi:10.1007/s00418-017-1584-2
Andreuzzi, E., Colladel, R., Pellicani, R., Tarticchio, G., Cannizzaro, R., Spessotto, P., et al. (2017). The Angiostatic Molecule Multimerin 2 Is Processed by MMP-9 to Allow Sprouting Angiogenesis. Matrix Biol. 64, 40–53. doi:10.1016/j.matbio.2017.04.002
Araújo, A. A., Morais, H. B., Medeiros, C. A. C. X., Brito, G. A. C., Guedes, P. M. M., Hiyari, S., et al. (2019). Gliclazide Reduced Oxidative Stress, Inflammation, and Bone Loss in an Experimental Periodontal Disease Model. J. Appl. Oral Sci. 27, e20180211. doi:10.1590/1678-7757-2018-0211
Ashcroft, F. M., and Rorsman, P. (2012). Diabetes Mellitus and the β Cell: the Last Ten Years. Cell 148, 1160–1171. doi:10.1016/j.cell.2012.02.010
Badran, Z., Struillou, X., Verner, C., Clee, T., Rakic, M., Martinez, M. C., et al. (2015). Periodontitis as a Risk Factor for Systemic Disease: Are Microparticles the Missing Link? Med. Hypotheses 84, 555–556. doi:10.1016/j.mehy.2015.02.013
Bastos, A. S., Graves, D. T., Loureiro, A. P., Rossa Júnior, C., Abdalla, D. S., Faulin, T. E. S., et al. (2012). Lipid Peroxidation Is Associated with the Severity of Periodontal Disease and Local Inflammatory Markers in Patients with Type 2 Diabetes. J. Clin. Endocrinol. Metab. 97, E1353–E1362. doi:10.1210/jc.2011-3397
Bazyar, H., Gholinezhad, H., Moradi, L., Salehi, P., Abadi, F., Ravanbakhsh, M., et al. (2019). The Effects of Melatonin Supplementation in Adjunct with Non-surgical Periodontal Therapy on Periodontal Status, Serum Melatonin and Inflammatory Markers in Type 2 Diabetes Mellitus Patients with Chronic Periodontitis: a Double-Blind, Placebo-Controlled Trial. Inflammopharmacology 27, 67–76. doi:10.1007/s10787-018-0539-0
Blasco-Baque, V., Garidou, L., Pomié, C., Escoula, Q., Loubieres, P., Le Gall-David, S., et al. (2017). Periodontitis Induced by Porphyromonas Gingivalis Drives Periodontal Microbiota Dysbiosis and Insulin Resistance via an Impaired Adaptive Immune Response. Gut 66, 872–885. doi:10.1136/gutjnl-2015-309897
Brownlee, M. (2001). Biochemistry and Molecular Cell Biology of Diabetic Complications. Nature 414, 813–820. doi:10.1038/414813a
Cao, R., Li, Q., Wu, Q., Yao, M., Chen, Y., and Zhou, H. (2019). Effect of Non-surgical Periodontal Therapy on Glycemic Control of Type 2 Diabetes Mellitus: a Systematic Review and Bayesian Network Meta-Analysis. BMC Oral Health 19, 176. doi:10.1186/s12903-019-0829-y
Carmeliet, P. (2003). Angiogenesis in Health and Disease. Nat. Med. 9, 653–660. doi:10.1038/nm0603-653
Castro Dos Santos, N. C., Andere, N. M. R. B., Araujo, C. F., De Marco, A. C., Kantarci, A., Van Dyke, T. E., et al. (2020). Omega-3 PUFA and Aspirin as Adjuncts to Periodontal Debridement in Patients with Periodontitis and Type 2 Diabetes Mellitus: Randomized Clinical Trial. J. Periodontol. 91, 1318–1327. doi:10.1002/JPER.19-0613
Chávarry, N. G., Vettore, M. V., Sansone, C., and Sheiham, A. (2009). The Relationship between Diabetes Mellitus and Destructive Periodontal Disease: a Meta-Analysis. Oral Health Prev. Dent 7, 107–127. doi:10.3290/j.ohpd.a15518
Chavakis, T., Bierhaus, A., Al-Fakhri, N., Schneider, D., Witte, S., Linn, T., et al. (2003). The Pattern Recognition Receptor (RAGE) Is a Counterreceptor for Leukocyte Integrins: a Novel Pathway for Inflammatory Cell Recruitment. J. Exp. Med. 198, 1507–1515. doi:10.1084/jem.20030800
Chen, M. X., Zhong, Y. J., Dong, Q. Q., Wong, H. M., and Wen, Y. F. (2021). Global, Regional, and National burden of Severe Periodontitis, 1990-2019: An Analysis of the Global Burden of Disease Study 2019. J. Clin. Periodontol. 48,1165–1188. doi:10.1111/jcpe.13506
Coffee, L., and Sockrider, M. (2019). Dental Health and Lung Disease. Am. J. Respir. Crit. Care Med. 199, P9–P10. doi:10.1164/rccm.1995P9
Colhoun, H. M., Livingstone, S. J., Looker, H. C., Morris, A. D., Wild, S. H., Lindsay, R. S., et al. (2012). Hospitalised Hip Fracture Risk with Rosiglitazone and Pioglitazone Use Compared with Other Glucose-Lowering Drugs. Diabetologia 55, 2929–2937. doi:10.1007/s00125-012-2668-0
Coll, R. C., Robertson, A. A., Chae, J. J., Higgins, S. C., Muñoz-Planillo, R., Inserra, M. C., et al. (2015). A Small-Molecule Inhibitor of the NLRP3 Inflammasome for the Treatment of Inflammatory Diseases. Nat. Med. 21, 248–255. doi:10.1038/nm.3806
Collaboration, N. C. D. R. F. (2016). Worldwide Trends in Diabetes since 1980: a Pooled Analysis of 751 Population-Based Studies with 4.4 Million Participants. Lancet 387, 1513–1530. doi:10.1016/S0140-6736(16)00618-8
Corgnali, M., Piconi, L., Ihnat, M., and Ceriello, A. (2008). Evaluation of Gliclazide Ability to Attenuate the Hyperglycaemic 'memory' Induced by High Glucose in Isolated Human Endothelial Cells. Diabetes Metab. Res. Rev. 24, 301–309. doi:10.1002/dmrr.804
Darveau, R. P. (2010). Periodontitis: a Polymicrobial Disruption of Host Homeostasis. Nat. Rev. Microbiol. 8, 481–490. doi:10.1038/nrmicro2337
Das, A. C., Das, S. J., Panda, S., Sharma, D., Taschieri, S., and Fabbro, M. D. (2019). Adjunctive Effect of Doxycycline with Conventional Periodontal Therapy on Glycemic Level for Chronic Periodontitis with Type 2 Diabetes Mellitus Subjects. J. Contemp. Dent Pract. 20, 1417–1423. doi:10.5005/jp-journals-10024-2722
Ding, K. H., Wang, Z. Z., Hamrick, M. W., Deng, Z. B., Zhou, L., Kang, B., et al. (2006). Disordered Osteoclast Formation in RAGE-Deficient Mouse Establishes an Essential Role for RAGE in Diabetes Related Bone Loss. Biochem. Biophys. Res. Commun. 340, 1091–1097. doi:10.1016/j.bbrc.2005.12.107
Dormuth, C. R., Carney, G., Carleton, B., Bassett, K., and Wright, J. M. (2009). Thiazolidinediones and Fractures in Men and Women. Arch. Intern. Med. 169, 1395–1402. doi:10.1001/archinternmed.2009.214
Doyle, M. E., and Egan, J. M. (2003). Pharmacological Agents that Directly Modulate Insulin Secretion. Pharmacol. Rev. 55, 105–131. doi:10.1124/pr.55.1.7
El-Sharkawy, H. M., Anees, M. M., and Van Dyke, T. E. (2016). Propolis Improves Periodontal Status and Glycemic Control in Patients with Type 2 Diabetes Mellitus and Chronic Periodontitis: A Randomized Clinical Trial. J. Periodontol. 87, 1418–1426. doi:10.1902/jop.2016.150694
Farrugia, C., Stafford, G. P., Potempa, J., Wilkinson, R. N., Chen, Y., Murdoch, C., et al. (2021). Mechanisms of Vascular Damage by Systemic Dissemination of the Oral Pathogen Porphyromonas Gingivalis. FEBS J. 288, 1479–1495. doi:10.1111/febs.15486
Fava, D., Cassone-Faldetta, M., Laurenti, O., De Luca, O., Ghiselli, A., and De Mattia, G. (2002). Gliclazide Improves Anti-oxidant Status and Nitric Oxide-Mediated Vasodilation in Type 2 Diabetes. Diabet Med. 19, 752–757. doi:10.1046/j.1464-5491.2002.00762.x
Frencken, J. E., Sharma, P., Stenhouse, L., Green, D., Laverty, D., and Dietrich, T. (2017). Global Epidemiology of Dental Caries and Severe Periodontitis - a Comprehensive Review. J. Clin. Periodontol. 44 Suppl 18 (Suppl. 18), S94–S105. doi:10.1111/jcpe.12677
Fronczek-Sokół, J., and Pytlik, M. (2014). Effect of Glimepiride on the Skeletal System of Ovariectomized and Non-ovariectomized Rats. Pharmacol. Rep. 66, 412–417. doi:10.1016/j.pharep.2013.12.013
Gasner, N. S., and Schure, R. S. (2020). “Periodontal Disease,” in StatPearls. (Treasure Island (FL). StatPearls Publishing LLC. Copyright © 2020.
Giacco, F., and Brownlee, M. (2010). Oxidative Stress and Diabetic Complications. Circ. Res. 107, 1058–1070. doi:10.1161/CIRCRESAHA.110.223545
Graves, D. T., Ding, Z., and Yang, Y. (2020). The Impact of Diabetes on Periodontal Diseases. Periodontol. 2000 82, 214–224. doi:10.1111/prd.12318
Gyurko, R., Siqueira, C. C., Caldon, N., Gao, L., Kantarci, A., and Van Dyke, T. E. (2006). Chronic Hyperglycemia Predisposes to Exaggerated Inflammatory Response and Leukocyte Dysfunction in Akita Mice. J. Immunol. 177, 7250–7256. doi:10.4049/jimmunol.177.10.7250
Hajishengallis, G., and Chavakis, T. (2021). Local and Systemic Mechanisms Linking Periodontal Disease and Inflammatory Comorbidities. Nat. Rev. Immunol. 21, 426–440. doi:10.1038/s41577-020-00488-6
Hajishengallis, G. (2014). Immunomicrobial Pathogenesis of Periodontitis: Keystones, Pathobionts, and Host Response. Trends Immunol. 35, 3–11. doi:10.1016/j.it.2013.09.001
Hajishengallis, G. (2020). New Developments in Neutrophil Biology and Periodontitis. Periodontol. 2000 82, 78–92. doi:10.1111/prd.12313
Hasturk, H., Abdallah, R., Kantarci, A., Nguyen, D., Giordano, N., Hamilton, J., et al. (2015). Resolvin E1 (RvE1) Attenuates Atherosclerotic Plaque Formation in Diet and Inflammation-Induced Atherogenesis. Arterioscler Thromb. Vasc. Biol. 35, 1123–1133. doi:10.1161/ATVBAHA.115.305324
Hill, J. R., Coll, R. C., Sue, N., Reid, J. C., Dou, J., Holley, C. L., et al. (2017). Sulfonylureas as Concomitant Insulin Secretagogues and NLRP3 Inflammasome Inhibitors. ChemMedChem 12, 1449–1457. doi:10.1002/cmdc.201700270
Huang, Y., Zeng, J., Chen, G., Xie, X., Guo, W., and Tian, W. (2016). Periodontitis Contributes to Adipose Tissue Inflammation through the NF-B, JNK and ERK Pathways to Promote Insulin Resistance In a rat Model. Microbes Infect. 18, 804–812. doi:10.1016/j.micinf.2016.08.002
Ilievski, V., Cho, Y., Katwala, P., Rodriguez, H., Tulowiecka, M., Kurian, D., et al. (2015). TLR4 Expression by Liver Resident Cells Mediates the Development of Glucose Intolerance and Insulin Resistance in Experimental Periodontitis. PLoS One 10, e0136502. doi:10.1371/journal.pone.0136502
Jiao, J., Jing, W., Si, Y., Feng, X., Tai, B., Hu, D., et al. (2021). The Prevalence and Severity of Periodontal Disease in Mainland China: Data from the Fourth National Oral Health Survey (2015-2016). J. Clin. Periodontol. 48, 168–179. doi:10.1111/jcpe.13396
Jung, S. M., Kim, K. W., Yang, C. W., Park, S. H., and Ju, J. H. (2014). Cytokine-mediated Bone Destruction in Rheumatoid Arthritis. J. Immunol. Res. 2014, 263625. doi:10.1155/2014/263625
Kalaitzoglou, E., Fowlkes, J. L., Popescu, I., and Thrailkill, K. M. (2019). Diabetes Pharmacotherapy and Effects on the Musculoskeletal System. Diabetes Metab. Res. Rev. 35, e3100. doi:10.1002/dmrr.3100
Kanazawa, I., Yamaguchi, T., Yamamoto, M., and Sugimoto, T. (2010). Relationship between Treatments with Insulin and Oral Hypoglycemic Agents versus the Presence of Vertebral Fractures in Type 2 Diabetes Mellitus. J. Bone Miner Metab. 28, 554–560. doi:10.1007/s00774-010-0160-9
Kawahara, Y., Kaneko, T., Yoshinaga, Y., Arita, Y., Nakamura, K., Koga, C., et al. (2020). Effects of Sulfonylureas on Periodontopathic Bacteria-Induced Inflammation. J. Dent Res. 99, 830–838. doi:10.1177/0022034520913250
Khambata, R. S., Panayiotou, C. M., and Hobbs, A. J. (2011). Natriuretic Peptide Receptor-3 Underpins the Disparate Regulation of Endothelial and Vascular Smooth Muscle Cell Proliferation by C-type Natriuretic Peptide. Br. J. Pharmacol. 164, 584–597. doi:10.1111/j.1476-5381.2011.01400.x
Koh, T. J., and Dipietro, L. A. (2011). Inflammation and Wound Healing: the Role of the Macrophage. Expert Rev. Mol. Med. 13, e23. doi:10.1017/S1462399411001943
Kondegowda, N. G., Fenutria, R., Pollack, I. R., Orthofer, M., Garcia-Ocaña, A., Penninger, J. M., et al. (2015). Osteoprotegerin and Denosumab Stimulate Human Beta Cell Proliferation through Inhibition of the Receptor Activator of NF-Κb Ligand Pathway. Cell Metab 22, 77–85. doi:10.1016/j.cmet.2015.05.021
Kothari, V., Galdo, J. A., and Mathews, S. T. (2016). Hypoglycemic Agents and Potential Anti-inflammatory Activity. J. Inflamm. Res. 9, 27–38. doi:10.2147/JIR.S86917
Kumar, N., and Dey, C. S. (2002). Gliclazide Increases Insulin Receptor Tyrosine Phosphorylation but Not P38 Phosphorylation in Insulin-Resistant Skeletal Muscle Cells. J. Exp. Biol. 205, 3739–3746. doi:10.1242/jeb.205.23.3739
Kumari, M., Martande, S. S., Pradeep, A. R., and Naik, S. B. (2016). Efficacy of Subgingivally Delivered 1.2% Atorvastatin in the Treatment of Chronic Periodontitis in Patients with Type 2 Diabetes Mellitus: A Randomized Controlled Clinical Trial. J. Periodontol. 87, 1278–1285. doi:10.1902/jop.2016.130227
Laliberte, R. E., Perregaux, D. G., Hoth, L. R., Rosner, P. J., Jordan, C. K., Peese, K. M., et al. (2003). Glutathione S-Transferase omega 1-1 Is a Target of Cytokine Release Inhibitory Drugs and May Be Responsible for Their Effect on Interleukin-1beta Posttranslational Processing. J. Biol. Chem. 278, 16567–16578. doi:10.1074/jbc.M211596200
Lalla, E., and Papapanou, P. N. (2011). Diabetes Mellitus and Periodontitis: a Tale of Two Common Interrelated Diseases. Nat. Rev. Endocrinol. 7, 738–748. doi:10.1038/nrendo.2011.106
Lalla, E., Lamster, I. B., Feit, M., Huang, L., Spessot, A., Qu, W., et al. (2000). Blockade of RAGE Suppresses Periodontitis-Associated Bone Loss in Diabetic Mice. J. Clin. Invest. 105, 1117–1124. doi:10.1172/JCI8942
Lamkanfi, M., Mueller, J. L., Vitari, A. C., Misaghi, S., Fedorova, A., Deshayes, K., et al. (2009). Glyburide Inhibits the Cryopyrin/Nalp3 Inflammasome. J. Cel Biol 187, 61–70. doi:10.1083/jcb.200903124
Lecka-Czernik, B. (2017). Diabetes, Bone and Glucose-Lowering Agents: Basic Biology. Diabetologia 60, 1163–1169. doi:10.1007/s00125-017-4269-4
Li, J., Lu, H., Wu, H., Huang, S., Chen, L., Gui, Q., et al. (2020). Periodontitis in Elderly Patients with Type 2 Diabetes Mellitus: Impact on Gut Microbiota and Systemic Inflammation. Aging (Albany NY) 12, 25956–25980. doi:10.18632/aging.202174
Lin, Y. W., Liu, P. S., Pook, K. A., and Wei, L. N. (2018). Glyburide and Retinoic Acid Synergize to Promote Wound Healing by Anti-inflammation and RIP140 Degradation. Sci. Rep. 8, 834. doi:10.1038/s41598-017-18785-x
Löe, H. (1993). Periodontal Disease. The Sixth Complication of Diabetes Mellitus. Diabetes Care 16, 329–334. doi:10.2337/diacare.16.1.329
Loubatières-Mariani, M.-M. (2007). La découverte des sulfamides hypoglycémiants. J. Soc. Biol. 201, 121–125. doi:10.1051/jbio:2007014
Lovic, D., Piperidou, A., Zografou, I., Grassos, H., Pittaras, A., and Manolis, A. (2020). The Growing Epidemic of Diabetes Mellitus. Curr. Vasc. Pharmacol. 18, 104–109. doi:10.2174/1570161117666190405165911
Lv, W., Wang, X., Xu, Q., and Lu, W. (2020). Mechanisms and Characteristics of Sulfonylureas and Glinides. Curr. Top. Med. Chem. 20, 37–56. doi:10.2174/1568026620666191224141617
Ma, P., Xiong, W., Liu, H., Ma, J., Gu, B., and Wu, X. (2011). Extrapancreatic Roles of Glimepiride on Osteoblasts from Rat Manibular Bone In Vitro: Regulation of Cytodifferentiation through PI3-kinases/Akt Signalling Pathway. Arch. Oral Biol. 56, 307–316. doi:10.1016/j.archoralbio.2010.10.009
Ma, P., Gu, B., Xiong, W., Tan, B., Geng, W., Li, J., et al. (2014). Glimepiride Promotes Osteogenic Differentiation in Rat Osteoblasts via the PI3K/Akt/eNOS Pathway in a High Glucose Microenvironment. PLoS One 9, e112243. doi:10.1371/journal.pone.0112243
Mammen, J., Vadakkekuttical, R. J., George, J. M., Kaziyarakath, J. A., and Radhakrishnan, C. (2017). Effect of Non-surgical Periodontal Therapy on Insulin Resistance in Patients with Type II Diabetes Mellitus and Chronic Periodontitis, as Assessed by C-Peptide and the Homeostasis Assessment Index. J. Investig. Clin. Dent. 8. e12221. doi:10.1111/jicd.12221
Mammen, M. J., Scannapieco, F. A., and Sethi, S. (2020). Oral-lung Microbiome Interactions in Lung Diseases. Periodontol. 2000 83, 234–241. doi:10.1111/prd.12301
Mamputu, J. C., and Renier, G. (2004). Signalling Pathways Involved in Retinal Endothelial Cell Proliferation Induced by Advanced Glycation End Products: Inhibitory Effect of Gliclazide. Diabetes Obes. Metab. 6, 95–103. doi:10.1111/j.1462-8902.2004.00320.x
Masters, S. L., Dunne, A., Subramanian, S. L., Hull, R. L., Tannahill, G. M., Sharp, F. A., et al. (2010). Activation of the NLRP3 Inflammasome by Islet Amyloid Polypeptide Provides a Mechanism for Enhanced IL-1β in Type 2 Diabetes. Nat. Immunol. 11, 897–904. doi:10.1038/ni.1935
Matsuzawa, Y., Guddeti, R. R., Kwon, T. G., Lerman, L. O., and Lerman, A. (2015). Treating Coronary Disease and the Impact of Endothelial Dysfunction. Prog. Cardiovasc. Dis. 57, 431–442. doi:10.1016/j.pcad.2014.10.004
Melton, L. J., Leibson, C. L., Achenbach, S. J., Therneau, T. M., and Khosla, S. (2008). Fracture Risk in Type 2 Diabetes: Update of a Population-Based Study. J. Bone Miner Res. 23, 1334–1342. doi:10.1359/jbmr.080323
Mirza, R. E., Fang, M. M., Weinheimer-Haus, E. M., Ennis, W. J., and Koh, T. J. (2014). Sustained Inflammasome Activity in Macrophages Impairs Wound Healing in Type 2 Diabetic Humans and Mice. Diabetes 63, 1103–1114. doi:10.2337/db13-0927
Miyajima, S., Naruse, K., Kobayashi, Y., Nakamura, N., Nishikawa, T., Adachi, K., et al. (2014). Periodontitis-activated Monocytes/macrophages Cause Aortic Inflammation. Sci. Rep. 4, 5171. doi:10.1038/srep05171
Morita, I., Inagaki, K., Nakamura, F., Noguchi, T., Matsubara, T., Yoshii, S., et al. (2012). Relationship between Periodontal Status and Levels of Glycated Hemoglobin. J. Dent Res. 91, 161–166. doi:10.1177/0022034511431583
Mougeot, J. C., Stevens, C. B., Paster, B. J., Brennan, M. T., Lockhart, P. B., and Mougeot, F. K. (2017). Porphyromonas Gingivalis Is the Most Abundant Species Detected in Coronary and Femoral Arteries. J. Oral Microbiol. 9, 1281562. doi:10.1080/20002297.2017.1281562
Naguib, G., Al-Mashat, H., Desta, T., and Graves, D. T. (2004). Diabetes Prolongs the Inflammatory Response to a Bacterial Stimulus through Cytokine Dysregulation. J. Invest. Dermatol. 123, 87–92. doi:10.1111/j.0022-202X.2004.22711.x
Nazir, M. A. (2017). Prevalence of Periodontal Disease, its Association with Systemic Diseases and Prevention. Int. J. Health Sci. (Qassim) 11, 72–80.
Noguchi, K., Miwa, Y., Sunohara, M., and Sato, I. (2011). Analysis of Vascular Distribution and Growth Factors in Human Gingival Tissue Associated with Periodontal Probing Depth. Okajimas Folia Anat. Jpn. 88, 75–83. doi:10.2535/ofaj.88.75
Nonaka, K., Kajiura, Y., Bando, M., Sakamoto, E., Inagaki, Y., Lew, J. H., et al. (2018). Advanced Glycation End-Products Increase IL-6 and ICAM-1 Expression via RAGE, MAPK and NF-Κb Pathways in Human Gingival Fibroblasts. J. Periodontal Res. 53, 334–344. doi:10.1111/jre.12518
Ogata, N., Chikazu, D., Kubota, N., Terauchi, Y., Tobe, K., Azuma, Y., et al. (2000). Insulin Receptor Substrate-1 in Osteoblast Is Indispensable for Maintaining Bone Turnover. J. Clin. Invest. 105, 935–943. doi:10.1172/JCI9017
Ogurtsova, K., Da Rocha Fernandes, J. D., Huang, Y., Linnenkamp, U., Guariguata, L., Cho, N. H., et al. (2017). IDF Diabetes Atlas: Global Estimates for the Prevalence of Diabetes for 2015 and 2040. Diabetes Res. Clin. Pract. 128, 40–50. doi:10.1016/j.diabres.2017.03.024
Okouchi, M., Okayama, N., Omi, H., Imaeda, K., Fukutomi, T., Nakamura, A., et al. (2004). The Antidiabetic Agent, Gliclazide, Reduces High Insulin-Enhanced Neutrophil-Transendothelial Migration through Direct Effects on the Endothelium. Diabetes Metab. Res. Rev. 20, 232–238. doi:10.1002/dmrr.444
Ortega, F. J., Jolkkonen, J., Mahy, N., and Rodríguez, M. J. (2013). Glibenclamide Enhances Neurogenesis and Improves Long-Term Functional Recovery after Transient Focal Cerebral Ischemia. J. Cereb. Blood Flow Metab. 33, 356–364. doi:10.1038/jcbfm.2012.166
Özcan, E., Saygun, N. I., Ilıkçı, R., Karslıoğlu, Y., Muşabak, U., and Yeşillik, S. (2017). Increased Visfatin Expression Is Associated with Nuclear Factor-Kappa B and Phosphatidylinositol 3-kinase in Periodontal Inflammation. Clin. Oral Investig. 21, 1113–1121. doi:10.1007/s00784-016-1871-7
Pacios, S., Andriankaja, O., Kang, J., Alnammary, M., Bae, J., De Brito Bezerra, B., et al. (2013). Bacterial Infection Increases Periodontal Bone Loss in Diabetic Rats through Enhanced Apoptosis. Am. J. Pathol. 183, 1928–1935. doi:10.1016/j.ajpath.2013.08.017
Papapetropoulos, A., Pyriochou, A., Altaany, Z., Yang, G., Marazioti, A., Zhou, Z., et al. (2009). Hydrogen Sulfide Is an Endogenous Stimulator of Angiogenesis. Proc. Natl. Acad. Sci. U S A. 106, 21972–21977. doi:10.1073/pnas.0908047106
Peng, H., Wright, V., Usas, A., Gearhart, B., Shen, H. C., Cummins, J., et al. (2002). Synergistic Enhancement of Bone Formation and Healing by Stem Cell-Expressed VEGF and Bone Morphogenetic Protein-4. J. Clin. Invest. 110, 751–759. doi:10.1172/JCI15153
Pitocco, D., Zaccardi, F., Di Stasio, E., Romitelli, F., Santini, S. A., Zuppi, C., et al. (2010). Oxidative Stress, Nitric Oxide, and Diabetes. Rev. Diabet Stud. 7, 15–25. doi:10.1900/RDS.2010.7.15
Pradeep, A. R., Prapulla, D. V., Sharma, A., and Sujatha, P. B. (2011). Gingival Crevicular Fluid and Serum Vascular Endothelial Growth Factor: Their Relationship in Periodontal Health, Disease and after Treatment. Cytokine 54, 200–204. doi:10.1016/j.cyto.2011.02.010
Pradhan, A. D., Manson, J. E., Rifai, N., Buring, J. E., and Ridker, P. M. (2001). C-reactive Protein, Interleukin 6, and Risk of Developing Type 2 Diabetes Mellitus. Jama 286, 327–334. doi:10.1001/jama.286.3.327
Preshaw, P. M., Taylor, J. J., Jaedicke, K. M., De Jager, M., Bikker, J. W., Selten, W., et al. (2020). Treatment of Periodontitis Reduces Systemic Inflammation in Type 2 Diabetes. J. Clin. Periodontol. 47, 737–746. doi:10.1111/jcpe.13274
Putz, D. M., Goldner, W. S., Bar, R. S., Haynes, W. G., and Sivitz, W. I. (2004). Adiponectin and C-Reactive Protein in Obesity, Type 2 Diabetes, and Monodrug Therapy. Metabolism 53, 1454–1461. doi:10.1016/j.metabol.2004.06.013
Qi, C., Zhou, Q., Li, B., Yang, Y., Cao, L., Ye, Y., et al. (2014). Glipizide, an Antidiabetic Drug, Suppresses Tumor Growth and Metastasis by Inhibiting Angiogenesis. Oncotarget 5, 9966–9979. doi:10.18632/oncotarget.2483
Qi, C., Bin Li, L., Yang, Y., Yang, Y., Li, J., Zhou, Q., et al. (2016). Glipizide Suppresses Prostate Cancer Progression in the TRAMP Model by Inhibiting Angiogenesis. Sci. Rep. 6, 27819. doi:10.1038/srep27819
Räkel, A., Renier, G., Roussin, A., Buithieu, J., Mamputu, J. C., and Serri, O. (2007). Beneficial Effects of Gliclazide Modified Release Compared with Glibenclamide on Endothelial Activation and Low-Grade Inflammation in Patients with Type 2 Diabetes. Diabetes Obes. Metab. 9, 127–129. doi:10.1111/j.1463-1326.2006.00571.x
Ramadan, D. E., Hariyani, N., Indrawati, R., Ridwan, R. D., and Diyatri, I. (2020). Cytokines and Chemokines in Periodontitis. Eur. J. Dent 14, 483–495. doi:10.1055/s-0040-1712718
Renier, G., Mamputu, J. C., and Serri, O. (2003). Benefits of Gliclazide in the Atherosclerotic Process: Decrease in Monocyte Adhesion to Endothelial Cells. Metabolism 52, 13–18. doi:10.1016/s0026-0495(03)00212-9
Renn, T. Y., Huang, Y. K., Feng, S. W., Wang, H. W., Lee, W. F., Lin, C. T., et al. (2018). Prophylactic Supplement with Melatonin Successfully Suppresses the Pathogenesis of Periodontitis through Normalizing RANKL/OPG Ratio and Depressing the TLR4/MyD88 Signaling Pathway. J. Pineal Res. 64, e12464. doi:10.1111/jpi.12464
Ruiz, H. H., Ramasamy, R., and Schmidt, A. M. (2020). Advanced Glycation End Products: Building on the Concept of the "Common Soil" in Metabolic Disease. Endocrinology 161, bqz006. doi:10.1210/endocr/bqz006
Sarkar, A., Hellberg, L., Bhattacharyya, A., Behnen, M., Wang, K., Lord, J. M., et al. (2012). Infection with Anaplasma Phagocytophilum Activates the Phosphatidylinositol 3-Kinase/Akt and NF-Κb Survival Pathways in Neutrophil Granulocytes. Infect. Immun. 80, 1615–1623. doi:10.1128/IAI.05219-11
Sczepanik, F. S. C., Grossi, M. L., Casati, M., Goldberg, M., Glogauer, M., Fine, N., et al. (2020). Periodontitis Is an Inflammatory Disease of Oxidative Stress: We Should Treat it that Way. Periodontol. 2000 84, 45–68. doi:10.1111/prd.12342
Sgolastra, F., Severino, M., Pietropaoli, D., Gatto, R., and Monaco, A. (2013). Effectiveness of Periodontal Treatment to Improve Metabolic Control in Patients with Chronic Periodontitis and Type 2 Diabetes: a Meta-Analysis of Randomized Clinical Trials. J. Periodontol. 84, 958–973. doi:10.1902/jop.2012.120377
Sheth, K. N., Elm, J. J., Molyneaux, B. J., Hinson, H., Beslow, L. A., Sze, G. K., et al. (2016). Safety and Efficacy of Intravenous Glyburide on Brain Swelling after Large Hemispheric Infarction (GAMES-RP): a Randomised, Double-Blind, Placebo-Controlled Phase 2 Trial. Lancet Neurol. 15, 1160–1169. doi:10.1016/S1474-4422(16)30196-X
Shi, B., Lux, R., Klokkevold, P., Chang, M., Barnard, E., Haake, S., et al. (2020). The Subgingival Microbiome Associated with Periodontitis in Type 2 Diabetes Mellitus. ISME J. 14, 519–530. doi:10.1038/s41396-019-0544-3
Silosi, I., Cojocaru, M., Foia, L., Boldeanu, M. V., Petrescu, F., Surlin, P., et al. (2015). Significance of Circulating and Crevicular Matrix Metalloproteinase-9 in Rheumatoid Arthritis-Chronic Periodontitis Association. J. Immunol. Res. 2015, 218060. doi:10.1155/2015/218060
Simpson, T. C., Needleman, I., Wild, S. H., Moles, D. R., and Mills, E. J. (2010). Treatment of Periodontal Disease for Glycaemic Control in People with Diabetes. Cochrane Database Syst Rev. 5, Cd004714. doi:10.1002/14651858.cd004714.pub2
Solomon, D. H., Cadarette, S. M., Choudhry, N. K., Canning, C., Levin, R., and Stürmer, T. (2009). A Cohort Study of Thiazolidinediones and Fractures in Older Adults with Diabetes. J. Clin. Endocrinol. Metab. 94, 2792–2798. doi:10.1210/jc.2008-2157
Sorsa, T., Tjäderhane, L., Konttinen, Y. T., Lauhio, A., Salo, T., Lee, H. M., et al. (2006). Matrix Metalloproteinases: Contribution to Pathogenesis, Diagnosis and Treatment of Periodontal Inflammation. Ann. Med. 38, 306–321. doi:10.1080/07853890600800103
Straka, M., Straka-Trapezanlidis, M., Deglovic, J., and Varga, I. (2015). Periodontitis and Osteoporosis. Neuro Endocrinol. Lett. 36, 401–406.
Su, Y., Wang, D., Xuan, D., Ni, J., Luo, S., Xie, B., et al. (2013). Periodontitis as a Novel Contributor of Adipose Tissue Inflammation Promotes Insulin Resistance in a Rat Model. J. Periodontol. 84, 1617–1626. doi:10.1902/jop.2013.120442
Sun, X., Li, M., Xia, L., Fang, Z., Yu, S., Gao, J., et al. (2020). Alteration of Salivary Microbiome in Periodontitis with or without Type-2 Diabetes Mellitus and Metformin Treatment. Sci. Rep. 10, 15363. doi:10.1038/s41598-020-72035-1
Tamashiro, N. S., Duarte, P. M., Miranda, T. S., Maciel, S. S., Figueiredo, L. C., Faveri, M., et al. (2016). Amoxicillin Plus Metronidazole Therapy for Patients with Periodontitis and Type 2 Diabetes: A 2-year Randomized Controlled Trial. J. Dent Res. 95, 829–836. doi:10.1177/0022034516639274
Teeuw, W. J., Gerdes, V. E., and Loos, B. G. (2010). Effect of Periodontal Treatment on Glycemic Control of Diabetic Patients: a Systematic Review and Meta-Analysis. Diabetes Care 33, 421–427. doi:10.2337/dc09-1378
Teitelbaum, S. L., and Ross, F. P. (2003). Genetic Regulation of Osteoclast Development and Function. Nat. Rev. Genet. 4, 638–649. doi:10.1038/nrg1122
Teles, R., and Wang, C. Y. (2011). Mechanisms Involved in the Association between Periodontal Diseases and Cardiovascular Disease. Oral Dis. 17, 450–461. doi:10.1111/j.1601-0825.2010.01784.x
Teng, Y. T., Nguyen, H., Gao, X., Kong, Y. Y., Gorczynski, R. M., Singh, B., et al. (2000). Functional Human T-Cell Immunity and Osteoprotegerin Ligand Control Alveolar Bone Destruction in Periodontal Infection. J. Clin. Invest. 106, R59–R67. doi:10.1172/jci10763
Torrungruang, K., Katudat, D., Mahanonda, R., Sritara, P., and Udomsak, A. (2019). Periodontitis Is Associated with Elevated Serum Levels of Cardiac Biomarkers-Soluble ST2 and C-Reactive Protein. J. Clin. Periodontol. 46, 809–818. doi:10.1111/jcpe.13149
Ueba, H., Kuroki, M., Hashimoto, S., Umemoto, T., Yasu, T., Ishikawa, S. E., et al. (2005). Glimepiride Induces Nitric Oxide Production in Human Coronary Artery Endothelial Cells via a PI3-Kinase-Akt Dependent Pathway. Atherosclerosis 183, 35–39. doi:10.1016/j.atherosclerosis.2005.01.055
Umaru, B., Pyriochou, A., Kotsikoris, V., Papapetropoulos, A., and Topouzis, S. (2015). ATP-sensitive Potassium Channel Activation Induces Angiogenesis In Vitro and In Vivo. J. Pharmacol. Exp. Ther. 354, 79–87. doi:10.1124/jpet.114.222000
Unlü, F., Güneri, P. G., Hekimgil, M., Yeşilbek, B., and Boyacioğlu, H. (2003). Expression of Vascular Endothelial Growth Factor in Human Periodontal Tissues: Comparison of Healthy and Diabetic Patients. J. Periodontol. 74, 181–187. doi:10.1902/jop.2003.74.2.181
Vandanmagsar, B., Youm, Y. H., Ravussin, A., Galgani, J. E., Stadler, K., Mynatt, R. L., et al. (2011). The NLRP3 Inflammasome Instigates Obesity-Induced Inflammation and Insulin Resistance. Nat. Med. 17, 179–188. doi:10.1038/nm.2279
Vestergaard, P., Rejnmark, L., and Mosekilde, L. (2005). Relative Fracture Risk in Patients with Diabetes Mellitus, and the Impact of Insulin and Oral Antidiabetic Medication on Relative Fracture Risk. Diabetologia 48, 1292–1299. doi:10.1007/s00125-005-1786-3
Wang, C. J., and Mccauley, L. K. (2016). Osteoporosis and Periodontitis. Curr. Osteoporos. Rep. 14, 284–291. doi:10.1007/s11914-016-0330-3
Wu, Y., Liu, F., Zhang, X., and Shu, L. (2014). Insulin Modulates Cytokines Expression in Human Periodontal Ligament Cells. Arch. Oral Biol. 59, 1301–1306. doi:10.1016/j.archoralbio.2014.07.002
Wu, Y. Y., Xiao, E., and Graves, D. T. (2015). Diabetes Mellitus Related Bone Metabolism and Periodontal Disease. Int. J. Oral Sci. 7, 63–72. doi:10.1038/ijos.2015.2
Yang, S., Gu, B., Zhao, L., Shi, Q., Xu, J., and Wen, N. (2019). Meta-analysis of the Association between Serum and Gingival Crevicular Fluid Matrix Metalloproteinase-9 and Periodontitis. J. Am. Dent Assoc. 150, 34–41. doi:10.1016/j.adaj.2018.08.025
Zang, Y., Song, J. H., Oh, S. H., Kim, J. W., Lee, M. N., Piao, X., et al. (2020). Targeting NLRP3 Inflammasome Reduces Age-Related Experimental Alveolar Bone Loss. J. Dent Res. 99, 1287–1295. doi:10.1177/0022034520933533
Zhang, R., Zou, Z., Zhou, X., Shen, X., Fan, Z., Xie, T., et al. (2019). Comparative Effects of Three Sulfonylureas (Glibenclamide, Glimepiride, and Gliclazide) on Proliferation and Migration of Vascular Smooth Muscle Cells. Cell Physiol Biochem 52, 16–26. doi:10.33594/000000002
Zhao, Y., Li, Z., Su, L., Ballesteros-Tato, A., Katz, J., Michalek, S. M., et al. (2020). Frontline Science: Characterization and Regulation of Osteoclast Precursors Following Chronic Porphyromonas Gingivalis Infection. J. Leukoc. Biol. 108, 1037–1050. doi:10.1002/JLB.1HI0620-230R
Zhou, R., Tardivel, A., Thorens, B., Choi, I., and Tschopp, J. (2010). Thioredoxin-interacting Protein Links Oxidative Stress to Inflammasome Activation. Nat. Immunol. 11, 136–140. doi:10.1038/ni.1831
Zhou, X., Zhang, P., Wang, Q., Ji, N., Xia, S., Ding, Y., et al. (2019). Metformin Ameliorates Experimental Diabetic Periodontitis Independently of Mammalian Target of Rapamycin (mTOR) Inhibition by Reducing NIMA-Related Kinase 7 (Nek7) Expression. J. Periodontol. 90, 1032–1042. doi:10.1002/JPER.18-0528
Zhu, L. F., Li, L., Wang, X. Q., Pan, L., Mei, Y. M., Fu, Y. W., et al. (2019). M1 Macrophages Regulate TLR4/AP1 via Paracrine to Promote Alveolar Bone Destruction in Periodontitis. Oral Dis. 25, 1972–1982. doi:10.1111/odi.13167
Keywords: periodontitis-diabetes comorbidity, sulfonylureas, inflammation, bone metabolism, angiogenesis
Citation: Yang L, Ge Q, Ye Z, Wang L, Wang L, Mashrah MA and Pathak JL (2021) Sulfonylureas for Treatment of Periodontitis-Diabetes Comorbidity-Related Complications: Killing Two Birds With One Stone. Front. Pharmacol. 12:728458. doi: 10.3389/fphar.2021.728458
Received: 21 June 2021; Accepted: 19 August 2021;
Published: 01 September 2021.
Edited by:
Liang Weng, Central South University, ChinaCopyright © 2021 Yang, Ge, Ye, Wang, Wang, Mashrah and Pathak. This is an open-access article distributed under the terms of the Creative Commons Attribution License (CC BY). The use, distribution or reproduction in other forums is permitted, provided the original author(s) and the copyright owner(s) are credited and that the original publication in this journal is cited, in accordance with accepted academic practice. No use, distribution or reproduction is permitted which does not comply with these terms.
*Correspondence: Mubarak Ahmed Mashrah, mubarak198226@gmail.com; Janak L. Pathak, j.pathak@gzhmu.edu.cn
†These authors have contributed equally to this work and share first authorship
Disclaimer: All claims expressed in this article are solely those of the authors and do not necessarily represent those of their affiliated organizations, or those of the publisher, the editors and the reviewers. Any product that may be evaluated in this article or claim that may be made by its manufacturer is not guaranteed or endorsed by the publisher.
Research integrity at Frontiers
Learn more about the work of our research integrity team to safeguard the quality of each article we publish.