- 1National of Metabolism Management Center (MMC), Tianjin Medical University Affiliated Tianjin Fourth Center Hospital, Nankai University Affiliated Hospital, Tianjin Fourth Center Hospital, Tianjin, China
- 2Department of Endocrinology and Metabolism, Tianjin Medical University General Hospital, Tianjin, China
- 3Department of Psychiatric-Neuroimaging-Genetics Laboratory (PNGC_Lab), Tianjin Mental Health Center, Tianjin Medical University, Tianjin, China
- 4Department of Psychiatry, Wenzhou Seventh Peoples Hospital, Wenzhou, China
- 5Department of Pharmacy, The First Hospital of Hebei Medical University, Shijiazhuang, China
- 6Big Data Analysis Center of Health Management Institute, The Second Medical Center and National Clinical Research Center for Geriatric Diseases, Chinese PLA General Hospital, Beijing, China
Background: Cardio-renal profiles are available from cardiovascular outcome trials of glucagon-like peptide-1 receptor agonists (GLP-1 RAs).
Methods: A comprehensive systematic review of Embase, Medline, Web of Knowledge, and CENTRAL databases was conducted. Randomized controlled cardiovascular outcome trials of type 2 diabetes mellitus (T2DM) patients administered GLP-1 RAs were included. The following primary outcomes were examined: cardiovascular death, major adverse cardiovascular events (MACE), myocardial infarction, stroke, mortality, heart failure, hypoglycemia, pancreatitis, and thyroid carcinoma. Secondary outcomes included: composite kidney outcome, worsening kidney function, macroalbuminuria, and retinopathy.
Results: Seven trials involving 56,004 patients and eight interventions were identified. Albiglutide was associated with fewer MACE and myocardial infarction events compared with lixisenatide. Lixisenatide was related to a greater number of stroke events and cardiovascular deaths compared to once-weekly semaglutide and oral semaglutide, respectively. Improved mortality was associated with oral semaglutide compared with once-weekly semaglutide, albiglutide, dulaglutide, exenatide, or lixisenatide. Risks of heart failure, thyroid carcinoma, and pancreatitis were similar among all the treatments. Weighting of the nine primary outcomes identified oral semaglutide as first among the eight treatments examined. Among three of the secondary outcomes, once-weekly semaglutide ranked first. Better composite kidney outcome was observed with once-weekly semaglutide than with dulaglutide or exenatide; once-weekly semaglutide improved macroalbuminuria compared with exenatide or lixisenatide; and albiglutide, exenatide, and placebo was associated with fewer cases of retinopathy compared with once-weekly semaglutide. Meanwhile, kidney function was less likely to worsen with dulaglutide than with lixisenatide or placebo.
Conclusion: Semaglutide should be considered when GLP-1 RAs are indicated for T2DM patients.
Introduction
Type 2 diabetes (T2DM) is a prevalent illness that causes major cardiovascular, renal, and neurologic complications (Chatterjee et al., 2017). Use of traditional antidiabetic drugs to control blood glucose has reduced the numbers of micro-vascular events in T2DM patients, yet has not been able to significantly reduce the risk of macro-vascular events in all patients (Group UPDSU, 1998; Action to Control Cardiovascular Risk in Diabetes Study Group et al., 2008; Holman et al., 2008; Control Group et al., 2009; Gerstein et al., 2014; Hayward et al., 2015; Wong et al., 2016). Furthermore, concerns about myocardial infarction and greater risk of heart failure that are associated with effective hypoglycemic agents, such as pioglitazone and rosiglitazone, have led to greater awareness of potentially harmful cardiovascular effects (Home et al., 2007; Lago et al., 2007; Nissen and Wolski 2007). Therefore, in 2008, the U.S. Food and Drug Administration (FDA) started providing regulatory guidance for industry mandated cardiovascular outcome trials (CVOTs) to evaluate novel antidiabetic agents and cardiovascular safety (Wilcox et al., 2020). The FDA has also provided evidence that hemoglobin A1c (HbA1C) reduction does not significantly reduce cardiovascular morbidity or mortality (Wilcox et al., 2020).
Over the past 10 years, the number of CVOTs for new glucose-lowering medications which target numerous novel pathways has been steadily increasing. Early CVOTs included patients with pre-existing cardiovascular diseases, while later CVOTs have included populations at high risk of cardiovascular disease (Del Olmo-Garcia and Merino-Torres, 2018; Acharya and Deedwania 2019). Initially, these CVOTs were designed to evaluate the safety of glucose-lowering medications. However, trials of sodium-glucose co-transporter 2 inhibitors (SGLT2i) and glucagon-like peptide-1 receptor agonists (GLP-1 RAs) have demonstrated cardiovascular benefits in secondary prevention of major adverse cardiovascular events (MACE), including cardiovascular death, heart failure, stroke, and myocardial infarction (Vijan 2019). Therefore, the American Diabetes Association (ADA) and the European Association for the Study of Diabetes (EASD), while previously employing a purely glucocentric approach for T2DM, have recently adopted a more holistic strategy with use of agents that demonstrate cardiorenal superiority (Davies et al., 2018; Cosentino et al., 2020). This recommendation reflects the wealth of clinical trial evidence regarding the health benefits associated with GLP-1RAs and SGLT2i.
GLP-1 is predominantly produced in enteroendocrine cells and it coordinates energy homeostasis and glucose metabolism via regulation of food intake, islet hormone secretion, and gastrointestinal motility. Consequently, GLP-1 RAs have been developed for the treatment of T2DM and obesity (Drucker 2016). In general, CVOTs of GLP-1 RAs have included randomized patients with T2DM in active therapy or placebo groups. These studies have investigated whether specific GLP-1 RAs improve cardiovascular and renal outcomes. To date, two studies have tried to synthesize these complex data. In one meta-analysis study, GLP-1 RAs were shown to provide beneficial effects on mortality, kidney, and cardiovascular outcomes in patients with T2DM (Kristensen et al., 2019). In a second network meta-analysis, mortality and cardiovascular safety were indirectly compared among various GLP-1 RAs in T2DM patients (Alfayez et al., 2020). However, to date, there remains no clear evidence to indicate which GLP-1 RA is optimal in terms of cardio-renal and other key outcomes. Therefore, the objective of the present study was to conduct a network meta-analysis with a systematic review of all COVTs of GLP-1 RAs in order to: (1) compare the primary outcomes reported, including cardiovascular outcomes, hypoglycemia, pancreatitis, thyroid medullary, and papillary carcinoma, (2) compare the secondary outcomes reported in a subset of the CVOTs, including composite kidney outcome, worsening kidney function, macroalbuminuria, retinopathy, and (3) rank the integrative effect of GFP-1 RAs on both primary and secondary outcomes in T2DM patients.
Methods
Systematic Literature Search
According to the PRISMA Extension Statement for Reporting of Systematic Reviews Incorporating Network Meta-analyses of Health Care Interventions: Checklist and Explanations (Hutton et al., 2015), a network meta-analysis was conducted. The study protocol was prespecified and registered on International Platform of Registered Systematic Review and Meta-analysis (INPLASY) (registration number, INPLASY202080122). A systematic review was conducted to identify published CVOTs for GLP-1 RAs in the following databases from the inception of these databases up to August 1, 2020: Embase, Medline (via PubMed), Web of Knowledge, and Cochrane Central Register of Controlled Trials (CENTRAL). The following keywords and MeSH terms were used: glucagon like peptide-1, GLP-1 receptor agonist, lixisenatide, liraglutide, semaglutide, exenatide, albiglutide, dulaglutide, myocardial infarction, heart failure, death, mortality, stroke, and angina. References cited in identified articles and those in relevant meta-analyses and systematic reviews were examined to identify articles not identified in the computerized database search. There were no limitations on language or publication year for study selection.
Study Selection
Randomized controlled trials which examined cardiovascular safety as a primary outcome for both injectable and oral GLP-1 RAs (at least two GLP-1 RAs or a GLP-1 RA and a placebo) administered to patients with T2DM were selected for the network meta-analysis conducted in this study (Supplementary Table S1). Conflicts regarding study inclusion were resolved by consensus.
Data Extraction
Two authors independently extracted data from the selected published studies. Study design, characteristics of participants, treatments, follow-up, primary and secondary outcomes, cardiovascular disease, systolic blood pressure, history of heart failure, estimated glomerular filtration rate (eGFR), and use of other drugs were documented. Any disagreements regarding data extraction were resolved with a third investigator.
Primary and Secondary Outcomes
The primary outcomes of interest included: non-fatal or fatal myocardial infarction, non-fatal or fatal stroke, MACE, cardiovascular death, all-cause mortality, hospital admission for heart failure, severe hypoglycemia, pancreatitis, and thyroid carcinoma. Secondary outcomes of interest included: composite kidney outcomes, worsening kidney function, macroalbuminuria, and retinopathy. Both primary and secondary outcomes are defined in Supplementary Table S2.
Risk of Bias Assessment
For individual studies of randomized trials, the Cochrane risk of bias tool was applied by two independent reviewers (Higgins et al., 2011). Studies were classified as having low, high, or unclear risk of bias depending on allocation concealment, sequence generation adequacy, blinding of personnel and participants, as well as blinding of outcome assessment, selective reporting, and method of addressing incomplete data. Graphic representations of potential bias both across and within studies were generated with RevMan V.5.1. To resolve disagreements, discussion, then consultation of a third arbitrator, was employed, respectively.
Statistical Analysis
To compare different GLP-1 RAs, a frequentist network meta-analysis was conducted by using the network command in STATA (White, 2009, 2011; Higgins et al., 2012; White et al., 2012). Evaluation of safety between at least two GLP-1 RAs, or between a GLP-1 RA and placebo, is reported with odds ratio (OR) values and corresponding 95% confidence intervals (CIs). It was assumed that a common heterogeneity parameter exists across all of the loops in the network. Therefore, inconsistency checks could be performed within a closed loop in the network (Higgins et al., 2003; Veroniki et al., 2013). A simple transformation of mean rank generated a surface under the cumulative ranking curve (SUCRA). As a result, a hierarchy of the treatments could be obtained and both the variance and location of all the relative treatment effects could be accounted for (Salanti et al., 2011). SUCRA values could vary from 100 to 0, representing the best to worst interventions, respectively. STATA version 14.0 software (Stata Corporation, College Station, TX, United States) was used to perform all of the statistical analyses described.
Results
Characteristics of the Studies Examined
A total of 1,245 studies were retrieved from databases and relevant articles, of which seven met the inclusion criteria for analysis (Figure 1). These seven studies included a total of 56,004 T2DM patients who received the following treatments: lixisenatide (ELIXA) (Pfeffer et al., 2015; Muskiet et al., 2018), liraglutide (LEADER) (Mann et al., 2017), once-weekly semaglutide (SUSTAIN-6) (Marso et al., 2016), albiglutide (Harmony Outcomes) (Hernandez et al., 2018), exenatide (EXSCEL) (Holman et al., 2017), oral semaglutide (PIONEER 6) (Husain et al., 2019), and dulaglutide (REWIND) (Gerstein et al., 2019a,b). Lixisenatide and exenatide are short-acting exendin-4 compounds, while semaglutide, albiglutide, liraglutide, and dulaglutide are long-acting human GLP-1 compounds. All seven studies, published between 2015 and 2019, had sample sizes which ranged from 3,183 to 14,752 participants (Table 1).
ELIXA (Pfeffer et al., 2015) included T2DM patients who recently experienced acute coronary syndrome, while the other six studies included T2DM patients with at least one co-existing cardiovascular condition or cardiovascular risk factor. The latter studies were conducted in the United Kingdom, Germany, United States, and Canada. The mean age of the participants ranged from 59.9 to 66.2 years, the proportion of male patients ranged from 53.7 to 69.0%, body mass index values ranged from 30.1 to 32.8, the mean duration of T2DM ranged from 9.2 to 11.9 years, and the median HbA1c value ranged from 7.7 to 8.7. The median duration of follow-up ranged from 1.3 years (PIONEER 6) to 5.4 years (REWIND). The proportion of patients with a history of heart failure or established cardiovascular disease ranged from 9% (REWIND) to 24% (SUSTAIN-6), or 31% (REWIND) to 100% (ELIXA), respectively. The REWIND, REWIND, ELIXA, and HARMONY trials reported the lowest use of statins (66.1%), insulin (24.0%), biguanides (66%), and sulfonylurea (29%) at baseline, respectively. Use of thiazolidinedione was similar across all seven trials, ranging from 2 to 6%. Values for eGFR ranged from 74 to 80 ml/min per m2 (Table 1). All seven studies were characterized as high quality with a lower risk of bias according to the Cochrane risk-of-bias tool (Figure 2).
Network Meta-Analyses of Primary Outcomes
All seven trials reported all-cause mortality, MACE, hospital admittance for heart failure, severe hypoglycemia, pancreatitis, and thyroid carcinoma. Placebo was associated with a greater number of MACE compared with albiglutide (OR, 1.29; 95% CI: 1.11, 1.50), dulaglutide (OR, 1.13; 95% CI: 1.01, 1.28), liraglutide (OR, 1.16; 95% CI: 1.04, 1.31), and once-weekly semaglutide (OR, 1.39; 95% CI: 1.07, 1.79). Meanwhile, albiglutide was superior to lixisenatide (OR, 0.76; 95% CI: 0.61, 0.94) (Table 2).
Fewer non-fatal and fatal myocardial infarction events were associated with albiglutide compared with lixisenatide (OR, 0.72; 95% CI: 0.55, 0.94), exenatide (OR, 0.76; 95% CI: 0.60, 0.96), and placebo (placebo vs. albiglutide: OR, 1.34; 95% CI: 1.10, 1.64), respectively (Table 2). Conversely, placebo was associated with a greater number of fatal or non-fatal stroke events compared with dulaglutide (OR, 1.31; 95% CI: 1.061, 1.62) and oral semaglutide (OR, 1.65; 95% CI: 1.01, 2.67). In addition, lixisenatide was associated with a greater number of fatal or non-fatal stroke events compared with semaglutide (OR, 1.84; 95% CI: 1.01, 3.35) (Table 2). A greater number of cardiovascular deaths was associated with placebo compared with liraglutide (OR, 1.29; 95% CI: 1.07, 1.54) and oral semaglutide (OR, 2.02; 95% CI: 1.08, 3.77). Moreover, lixisenatide was associated with a greater number of cardiovascular deaths compared with oral semaglutide (OR, 1.99; 95% CI: 1.03, 3.87) (Table 2).
Placebo was associated with higher all-cause mortality compared with exenatide (OR, 1.16; 95% CI: 1.02, 1.31), liraglutide (OR, 1.19; 95% CI: 1.03, 1.37), and oral semaglutide (OR, 1.98; 95% CI: 1.19, 2.29). Conversely, oral semaglutide was associated with lower all-cause mortality compared with once-weekly semaglutide (OR, 0.49; 95% CI: 0.26, 0.91), albiglutide (OR, 0.53; 95% CI: 0.31, 0.51), dulaglutide (OR, 0.56; 95% CI: 0.33, 0.95), exenatide (OR, 0.58; 95% CI: 0.35, 0.98), and lixisenatide (OR, 0.54; 95% CI: 0.31, 0.92) (Table 2).
Albiglutide was significantly less likely to induce hypoglycemia than exenatide (OR, 0.49; 95% CI: 0.31, 0.80), oral semaglutide (OR, 0.31; 95% CI: 0.14, 0.71), once-weekly semaglutide (OR, 0.52; 95% CI: 0.33, 0.84), or placebo (placebo vs. albiglutide: OR, 1.78; 95% CI: 1.15, 2.77), respectively. Meanwhile, liraglutide was significantly less likely to induce hypoglycemia than oral semaglutide (OR, 0.41; 95% CI: 0.20, 0.86), once-weekly semaglutide (OR, 0.69; 95% CI: 0.51, 0.93), or placebo (placebo vs. liraglutide: OR, 1.35; 95% CI: 1.06, 1.73), respectively. Lixisenatide was significantly less likely to induce hypoglycemia than oral semaglutide (OR, 0.33; 95% CI: 0.13, 0.84), while exenatide was more likely to induce hypoglycemia than liraglutide (OR, 1.54; 95% CI: 1.13, 2.09) (Table 2). In contrast, none of these treatments were significantly better than another in terms of hospital admission for heart failure, thyroid carcinoma, or pancreatitis (Table 2).
When the eight interventions were ranked according to SUCRA, albiglutide (80.6%), albiglutide (88.2%), once-weekly semaglutide (74.1%), oral semaglutide (82.8%), oral semaglutide (84.5%), albiglutide (74.2%), albiglutide (90.5%), semaglutide (65.2%), and oral semaglutide (73.4%) had the highest possibilities of being ranked first in terms of MACE, myocardial infarction, stroke, cardiovascular death, all-cause mortality, hospital admission for heart failure, severe hypoglycemia, thyroid carcinoma, and pancreatitis, respectively (Table 3). Ranking all eight interventions according to their overall probability of being ranked first after equal weighting of the nine primary outcomes identified the top four ranked interventions as: oral semaglutide (72.4%), albiglutide (61.5%), once-weekly semaglutide (60.7%), and liraglutide (56.1%), respectively (Figure 3).
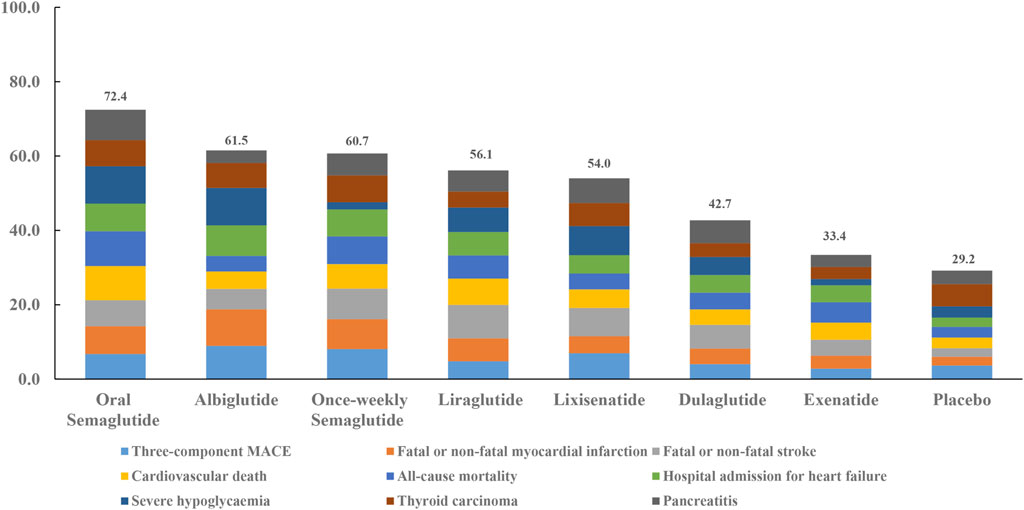
FIGURE 3. Ranking of treatments according to nine primary outcomes. Cumulative percentages after normalization (0–100%) are shown in the key. Each intervention was scored with points up to a maximum of 11.1 for each primary outcome (overall maximum score, 100) with data from rankograms and SUCRAs.
Network Meta-Analyses of Secondary Outcomes
Five trials (ELIXA, EXSCEL, LEADER, REWIND, SUSTAIN-6) were included in a network meta-analysis of composite kidney outcome, worsening kidney function, and macroalbuminuria. Placebo was associated with worse composite kidney outcome compared with dulaglutide (OR, 1.18; 95% CI: 1.06, 1.30), liraglutide (OR, 1.28; 95% CI: 1.08, 1.51), and once-weekly semaglutide (OR, 1.65; 95% CI: 1.19, 2.28). In addition, dulaglutide (OR, 1.40; 95% CI: 1.00, 1.97) and exenatide (OR, 1.47; 95% CI: 1.03, 2.09) were associated with worse kidney outcome compared to once-weekly semaglutide (Table 4). Dulaglutide was also associated with fewer cases of worsening kidney function compared with lixisenatide (OR, 0.60; 95% CI: 0.36, 0.98) and placebo (OR, 0.70; 95% CI: 0.58, 0.86) (Table 4). Meanwhile, placebo was associated with a greater number of macroalbuminuria events compared with dulaglutide (OR, 1.31; 95% CI: 1.15, 1.49), liraglutide (OR, 1.35; 95%CI 1.10, 1.66), and once-weekly semaglutide (OR, 1.88; 95% CI: 1.30, 2.74). Exenatide (OR, 1.54; 95% CI: 1.00, 2.38) and lixisenatide (OR, 1.57; 95% CI: 1.02, 2.41) were associated with a greater number of macroalbuminuria events compared with once-weekly semaglutide (Table 4).
Except for placebo, once-weekly semaglutide, dulaglutide, and once-weekly semaglutide had the highest probabilities of being ranked first in terms of composite kidney outcome (76.6%), worsening of kidney function (95.9%), and macroalbuminuria (77.2%), respectively (Table 5). All seven interventions were ranked by their overall probability to be ranked first after equal weighting composite kidney outcome, worsening kidney function, and macroalbuminuria. The top four ranked interventions were once-weekly semaglutide (73.8%), placebo (67.2%), dulaglutide (67.1%), and liraglutide (45.9%), respectively (Figure 4).
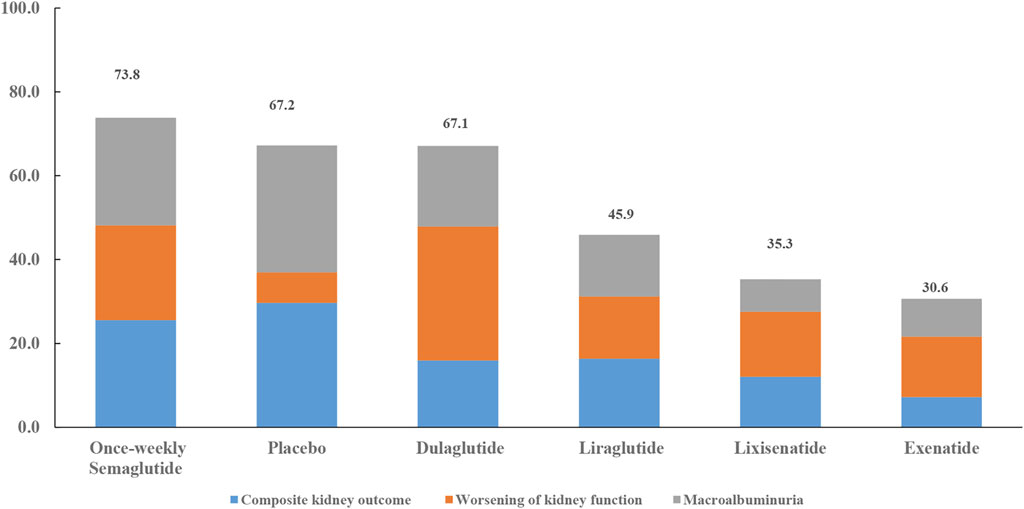
FIGURE 4. Ranking of treatments according to composite kidney outcome, worsening kidney function, and macroalbuminuria. Cumulative percentages after normalization (0–100%) are shown in the key. Each intervention was scored with points up to a maximum of 33.3 for each primary outcome (overall maximum score, 100) with data from rankograms and SUCRAs.
There were six trials (EXSCEL, HARMONY, LEADER, PIONEER-6, REWIND, SUSTAIN-6) which were included in a network meta-analysis of retinopathy. Albiglutide (OR, 0.50; 95% CI: 0.29, 0.87), exenatide (OR, 0.52; 95% CI: 0.31, 0.85), and placebo (OR, 0.57; 95% CI: 0.36, 0.91) was associated with fewer retinopathy events compared with once-weekly semaglutide (Table 4). Among the seven interventions, albiglutide (85.8%) had the highest probability of being ranked first (Table 5).
Discussion
The goal of the present study was to perform a network meta-analysis which would provide unified hierarchies of evidence for all of the RCTs of GLP-1 RAs published to date. In addition to the beneficial cardiovascular effect observed among diabetic patients in our network meta-analysis, clinical data also support a protective effect of incretin therapies on cardiovascular outcomes in diabetic patients with either ST elevation myocardial infarction with culprit obstructive lesion and multivessel non-obstructive coronary stenosis (Marfella et al., 2018), or non-ST-elevation myocardial infarction with non-obstructive coronary artery stenosis (Marfella et al., 2017). Moreover, GLP-1 RA therapy plus standard hypoglycemic drugs, compared to standard hypoglycemic drugs alone, for the treatment of diabetic patients that are undergoing cardiac resynchronization therapy with a defibrillator (CRT-d) for a failing heart has lead to significant improvements in left ventricular ejection fraction, a reduction in New York Heart Association class, arrhythmic burden, and hospitalization for heart failure worsening, and a 3.7-fold higher rate of CRTd responders (Sardu et al., 2018).
In the present study, mitigation of albuminuria and reduced deterioration of kidney function were observed. For example, short-term liraglutide treatment decreased proximal tubular sodium reabsorption and angiotensin II concentration, thereby contributing to renal protection (Skov et al., 2016). In another study, it was demonstrated that GLP-1 can act as a physiologically natriuretic factor in the proximal tubule by modulating the activity of sodium-hydrogen exchanger isoform-3 (NHE3). As a result, GLP-1 contributes to a reduction of albuminuria through amelioration of tubule-glomerular feedback (Farah et al., 2016). Another study confirmed that administration of a GLP-1 RA increases urinary pH and urinary sodium excretion, probably due to GLP-1 RA-induced pressure natriuresis or inhibition of NHE3 in the proximal tubule (Tonneijck et al., 2016).
Regarding the beneficial effect of GLP-1 RA on risk of hypoglycemia, this effect was found to vary between studies. GLP-1 is an incretin hormone that is expressed in the gut. This hormone suppresses secretion of glucagon and stimulates production of insulin to reduce food intake and appetite and inhibit gastric emptying (Holst, 2007). However, exendin-4-based, short-acting GLP-1 RAs primarily inhibit gastric emptying to lower postprandial blood glucose levels. Meanwhile, long-acting compounds based on human GLP-1 have exhibited a stronger effect on fasting glucose levels via glucagonostatic and insulinotropic mechanisms (Meier, 2012). None of the GLP-1 RAs examined performed significantly better than the others in relation to thyroid carcinoma.
According to the overall ranking of the GLP-1 RAs examined after considering nine primary outcomes, we observed that human GLP-1 analogs achieve more pronounced effects than exendin-based GLP-1 RAs, possibly due to structural differences in the GLP-1 RA groups. While exenatide and lixisenatide are remarkably similar structurally to albiglutide, dulaglutide, exenatide, and liraglutide, there are small differences in the molecular structures of these compounds which can potentially lead to critical differences. Thus, our findings may reflect functional differences between the GLP-1 RAs or features of the trials conducted.
It should be considered that the ranking probabilities identified from this network meta-analysis are based on indirect comparisons and involve several potential limitations. For example, aggregate trial-level data were used to pool the overall estimate instead of patient-level data. Secondly, the precise exclusion and inclusion criteria of the included trials, as well as the definitions of outcomes, varied slightly. Thirdly, variations in treatment duration could have had a significant impact on overall outcomes. The median follow-up time for the COVTs compared ranged from 1.3 to 5.4 years. Fourth, the number of comparisons and the sample size for each comparison varied significantly. Consequently, a subset of the studies may have had a greater significant impact on overall effect size than anticipated, while another subset of the studies may have had less of an impact on effect size than expected. Fifth, although the proportion of patients receiving SGLT2 inhibitors and DPP-4 inhibitors was quite low, the initial usage and introduction of these treatments to patients who were receiving GLP1 therapy during the COVT trials may have affected the clinical outcomes and results of our network meta-analysis. Sixth, it should be recognized that all of the statements made which compare the merits of one GLP-1 RA with another include potential uncertainties and biases which derive from the cohorts and treatment doses studied. Finally, our analysis was limited by the small number of CVOTs conducted for GLP-1 RAs. However, despite these considerations, the suggested hierarchies of GLP-1 RAs which were elucidated in this network meta-analysis may help clinicians individualize treatment plans for T2DM patients, while also improving clinical practice guidelines.
Conclusion
Cardio-renal benefits of GLP-1 RAs vary in patients with T2DM. Therefore, selection of GLP-1 RAs for treatment of T2DM should be individualized according to the safety profiles of the agonists considered.
Data Availability Statement
The original contributions presented in the study are included in the article/Supplementary Material, further inquiries can be directed to the corresponding authors.
Author Contributions
CZ designed this study, analyzed the data, and drafted this manuscript. CL, CZ, XG, HS, TF, HT, LD, and ML selected the studies, extracted data, and analyzed data. All of the authors approved the final manuscript.
Funding
This work was supported by grants from the National Natural Science Foundation of China (81871052 to CZ and 81830025 and 81620108004 to ML), Key Projects of the Natural Science Foundation of Tianjin, China (17JCZDJC35700 to CZ), a project of talent award (30000 yuan to CZ), Tianjin Health Bureau Foundation (2014KR02 to CZ), National Key R&D Program of China (2019YFA0802502 to ML), and Tianjin Municipal Science and Technology Commission (19JCQNJC11800 to LD).
Conflict of Interest
The authors declare that the research was conducted in the absence of any commercial or financial relationships that could be construed as a potential conflict of interest.
Publisher’s Note
All claims expressed in this article are solely those of the authors and do not necessarily represent those of their affiliated organizations, or those of the publisher, the editors and the reviewers. Any product that may be evaluated in this article, or claim that may be made by its manufacturer, is not guaranteed or endorsed by the publisher.
Supplementary Material
The Supplementary Material for this article can be found online at: https://www.frontiersin.org/articles/10.3389/fphar.2021.759262/full#supplementary-material
References
Acharya, T., and Deedwania, P. (2019). Cardiovascular Outcome Trials of the Newer Anti-diabetic Medications. Prog. Cardiovasc. Dis. 62 (4), 342–348. doi:10.1016/j.pcad.2019.08.003
Alfayez, O. M., Almohammed, O. A., Alkhezi, O. S., Almutairi, A. R., and Al Yami, M. S. (2020). Indirect Comparison of Glucagon like Peptide-1 Receptor Agonists Regarding Cardiovascular Safety and Mortality in Patients with Type 2 Diabetes Mellitus: Network Meta-Analysis. Cardiovasc. Diabetol. 19 (1), 96. doi:10.1186/s12933-020-01070-z
Chatterjee, S., Khunti, K., and Davies, M. J. (2017). Type 2 Diabetes. Lancet 389 (10085), 2239–2251. doi:10.1016/S0140-6736(17)30058-2
Cosentino, F., Grant, P. J., Aboyans, V., Bailey, C. J., Ceriello, A., Delgado, V., et al. ESC Scientific Document Group (2020). ESC Guidelines on Diabetes, Pre-diabetes, and Cardiovascular Diseases Developed in Collaboration with the EASD. Eur. Heart J. 41 (2), 255–323. doi:10.1093/eurheartj/ehz486
Davies, M. J., D'Alessio, D. A., Fradkin, J., Kernan, W. N., Mathieu, C., Mingrone, G., et al. (2018). Management of Hyperglycemia in Type 2 Diabetes, 2018. A Consensus Report by the American Diabetes Association (ADA) and the European Association for the Study of Diabetes (EASD). Diabetes Care 41 (12), 2669–2701. doi:10.2337/dci18-0033
Del Olmo-Garcia, M. I., and Merino-Torres, J. F. (2018). GLP-1 Receptor Agonists and Cardiovascular Disease in Patients with Type 2 Diabetes. J. Diabetes Res. 2018, 4020492. doi:10.1155/2018/4020492
Drucker, D. J. (2016). The Cardiovascular Biology of Glucagon-like Peptide-1. Cell Metab. 24 (1), 15–30. doi:10.1016/j.cmet.2016.06.009
Farah, L. X., Valentini, V., Pessoa, T. D., Malnic, G., McDonough, A. A., and Girardi, A. C. (2016). The Physiological Role of Glucagon-like Peptide-1 in the Regulation of Renal Function. Am. J. Physiol. Ren. Physiol. 310 (2), F123–F127. doi:10.1152/ajprenal.00394.2015
Gerstein, H. C., Colhoun, H. M., Dagenais, G. R., Diaz, R., Lakshmanan, M., Pais, P., et al. (2019a). Dulaglutide and Renal Outcomes in Type 2 Diabetes: an Exploratory Analysis of the REWIND Randomised, Placebo-Controlled Trial. Lancet 394 (10193), 131–138. doi:10.1016/S0140-6736(19)31150-X
Gerstein, H. C., Colhoun, H. M., Dagenais, G. R., Diaz, R., Lakshmanan, M., Pais, P., et al. (2019b). Dulaglutide and Cardiovascular Outcomes in Type 2 Diabetes (REWIND): a Double-Blind, Randomised Placebo-Controlled Trial. Lancet 394 (10193), 121–130. doi:10.1016/S0140-6736(19)31149-3
Gerstein, H. C., Miller, M. E., Ismail-Beigi, F., Largay, J., McDonald, C., Lochnan, H. A., et al. ACCORD Study Group (2014). Effects of Intensive Glycaemic Control on Ischaemic Heart Disease: Analysis of Data from the Randomised, Controlled ACCORD Trial. Lancet 384 (9958), 1936–1941. doi:10.1016/S0140-6736(14)60611-5
Action to Control Cardiovascular Risk in Diabetes Study Group Gerstein, H. C., Miller, M. E., Byington, R. P., Goff, D. C., Bigger, J. T., Buse, J. B., et al. (2008). Effects of Intensive Glucose Lowering in Type 2 Diabetes. N. Engl. J. Med. 358 (24), 2545–2559. doi:10.1056/NEJMoa0802743
Group UPDSU (1998). Intensive Blood-Glucose Control with Sulphonylureas or Insulin Compared with Conventional Treatment and Risk of Complications in Patients with Type 2 Diabetes (UKPDS 33). UK Prospective Diabetes Study (UKPDS) Group. Lancet 352 (9131), 837–853.
Hayward, R. A., Reaven, P. D., Wiitala, W. L., Bahn, G. D., Reda, D. J., Ge, L., et al. (2015). Follow-up of Glycemic Control and Cardiovascular Outcomes in Type 2 Diabetes. N. Engl. J. Med. 372 (23), 2197–2206. doi:10.1056/NEJMoa1414266
Hernandez, A. F., Green, J. B., Janmohamed, S., D'Agostino, R. B., Sr, , Granger, C. B., et al. (2018). Albiglutide and Cardiovascular Outcomes in Patients with Type 2 Diabetes and Cardiovascular Disease (Harmony Outcomes): a Double-Blind, Randomised Placebo-Controlled Trial. Lancet (London, England) 392 (10157), 1519–1529. doi:10.1016/S0140-6736(18)32261-X
Higgins, J. P., Jackson, D., Barrett, J. K., Lu, G., Ades, A. E., and White, I. R. (2012). Consistency and Inconsistency in Network Meta-Analysis: Concepts and Models for Multi-Arm Studies. Res. Synth. Methods 3 (2), 98–110. doi:10.1002/jrsm.1044
Higgins, J. P., Thompson, S. G., Deeks, J. J., and Altman, D. G. (2003). Measuring Inconsistency in Meta-Analyses. BMJ 327 (7414), 557–560. doi:10.1136/bmj.327.7414.557
Higgins, J. P. T., Altman, D. G., Gotzsche, P. C., Juni, P., Moher, D., Oxman, A. D., et al. (2011). Cochrane Bias Methods Group, & Cochrane Statistical Methods GroupThe Cochrane Collaboration's Tool for Assessing Risk of Bias in Randomised Trials. Bmj 343, d5928. doi:10.1136/bmj.d5928
Holman, R. R., Bethel, M. A., Mentz, R. J., Thompson, V. P., Lokhnygina, Y., Buse, J. B., et al. EXSCEL Study Group (2017). Effects of Once-Weekly Exenatide on Cardiovascular Outcomes in Type 2 Diabetes. N. Engl. J. Med. 377 (13), 1228–1239. doi:10.1056/NEJMoa1612917
Holman, R. R., Paul, S. K., Bethel, M. A., Matthews, D. R., and Neil, H. A. (2008). 10-year Follow-Up of Intensive Glucose Control in Type 2 Diabetes. N. Engl. J. Med. 359 (15), 1577–1589. doi:10.1056/NEJMoa0806470
Holst, J. J. (2007). The Physiology of Glucagon-like Peptide 1. Physiol. Rev. 87 (4), 1409–1439. doi:10.1152/physrev.00034.2006
Home, P. D., Pocock, S. J., Beck-Nielsen, H., Gomis, R., Hanefeld, M., Jones, N. P., et al. RECORD Study Group (2007). Rosiglitazone Evaluated for Cardiovascular Outcomes-Aan Interim Analysis. N. Engl. J. Med. 357 (1), 28–38. doi:10.1056/NEJMoa073394
Husain, M., Birkenfeld, A. L., Donsmark, M., Dungan, K., Eliaschewitz, F. G., Franco, D. R., et al. PIONEER 6 Investigators (2019). Oral Semaglutide and Cardiovascular Outcomes in Patients with Type 2 Diabetes. N. Engl. J. Med. 381 (9), 841–851. doi:10.1056/NEJMoa1901118
Hutton, B., Salanti, G., Caldwell, D. M., Chaimani, A., Schmid, C. H., Cameron, C., et al. (2015). The PRISMA Extension Statement for Reporting of Systematic Reviews Incorporating Network Meta-Analyses of Health Care Interventions: Checklist and Explanations. Ann. Intern. Med. 162 (11), 777–784. doi:10.7326/M14-2385
Kristensen, S. L., Rørth, R., Jhund, P. S., Docherty, K. F., Sattar, N., Preiss, D., et al. (2019). Cardiovascular, Mortality, and Kidney Outcomes with GLP-1 Receptor Agonists in Patients with Type 2 Diabetes: a Systematic Review and Meta-Analysis of Cardiovascular Outcome Trials. Lancet Diabetes Endocrinol. 7 (10), 776–785. doi:10.1016/S2213-8587(19)30249-9
Lago, R. M., Singh, P. P., and Nesto, R. W. (2007). Congestive Heart Failure and Cardiovascular Death in Patients with Prediabetes and Type 2 Diabetes Given Thiazolidinediones: a Meta-Analysis of Randomised Clinical Trials. Lancet 370 (9593), 1129–1136. doi:10.1016/S0140-6736(07)61514-1
Mann, J. F. E., Ørsted, D. D., Brown-Frandsen, K., Marso, S. P., Poulter, N. R., Rasmussen, S., et al. LEADER Steering Committee and Investigators (2017). Liraglutide and Renal Outcomes in Type 2 Diabetes. N. Engl. J. Med. 377 (9), 839–848. doi:10.1056/NEJMoa1616011
Marfella, R., Sardu, C., Balestrieri, M. L., Siniscalchi, M., Minicucci, F., Signoriello, G., et al. (2018). Effects of Incretin Treatment on Cardiovascular Outcomes in Diabetic STEMI-Patients with Culprit Obstructive and Multivessel Non Obstructive-Coronary-Stenosis. Diabetol. Metab. Syndr. 10, 1. doi:10.1186/s13098-017-0304-3
Marfella, R., Sardu, C., Calabrò, P., Siniscalchi, M., Minicucci, F., Signoriello, G., et al. (2017). Non-ST-elevation Myocardial Infarction Outcomes in Patients with Type 2 Diabetes with Non-obstructive Coronary Artery Stenosis: Effects of Incretin Treatment. Diabetes Obes. Metab. 20 (3), 723–729. doi:10.1111/dom.13122
Marso, S. P., Bain, S. C., Consoli, A., Eliaschewitz, F. G., Jódar, E., Leiter, L. A., et al. SUSTAIN-6 Investigators (2016). Semaglutide and Cardiovascular Outcomes in Patients with Type 2 Diabetes. N. Engl. J. Med. 375 (19), 1834–1844. doi:10.1056/NEJMoa1607141
Meier, J. J. (2012). GLP-1 Receptor Agonists for Individualized Treatment of Type 2 Diabetes Mellitus. Nat. Rev. Endocrinol. 8 (12), 728–742. doi:10.1038/nrendo.2012.140
Muskiet, M. H. A., Tonneijck, L., Huang, Y., Liu, M., Saremi, A., Heerspink, H. J. L., et al. (2018). Lixisenatide and Renal Outcomes in Patients with Type 2 Diabetes and Acute Coronary Syndrome: an Exploratory Analysis of the ELIXA Randomised, Placebo-Controlled Trial. Lancet Diabetes Endocrinol. 6 (11), 859–869. doi:10.1016/S2213-8587(18)30268-7
Nissen, S. E., and Wolski, K. (2007). Effect of Rosiglitazone on the Risk of Myocardial Infarction and Death from Cardiovascular Causes. N. Engl. J. Med. 356 (24), 2457–2471. doi:10.1056/NEJMoa072761
Pfeffer, M. A., Claggett, B., Diaz, R., Dickstein, K., Gerstein, H. C., Køber, L. V., et al. ELIXA Investigators (2015). Lixisenatide in Patients with Type 2 Diabetes and Acute Coronary Syndrome. N. Engl. J. Med. 373 (23), 2247–2257. doi:10.1056/NEJMoa1509225
Salanti, G., Ades, A. E., and Ioannidis, J. P. (2011). Graphical Methods and Numerical Summaries for Presenting Results from Multiple-Treatment Meta-Analysis: an Overview and Tutorial. J. Clin. Epidemiol. 64 (2), 163–171. doi:10.1016/j.jclinepi.2010.03.016
Sardu, C., Paolisso, P., Sacra, C., Santamaria, M., de Lucia, C., Ruocco, A., et al. (2018). Cardiac Resynchronization Therapy with a Defibrillator (CRTd) in Failing Heart Patients with Type 2 Diabetes Mellitus and Treated by Glucagon-like Peptide 1 Receptor Agonists (GLP-1 RA) Therapy vs. Conventional Hypoglycemic Drugs: Arrhythmic burden, Hospitalizations for Heart Failure, and CRTd Responders Rate. Cardiovasc. Diabetol. 17 (1), 137. doi:10.1186/s12933-018-0778-9
Skov, J., Pedersen, M., Holst, J. J., Madsen, B., Goetze, J. P., Rittig, S., et al. (2016). Short-term Effects of Liraglutide on Kidney Function and Vasoactive Hormones in Type 2 Diabetes: a Randomized Clinical Trial. Diabetes Obes. Metab. 18 (6), 581–589. doi:10.1111/dom.12651
Tonneijck, L., Smits, M. M., Muskiet, M. H. A., Hoekstra, T., Kramer, M. H. H., Danser, A. H. J., et al. (2016). Acute Renal Effects of the GLP-1 Receptor Agonist Exenatide in Overweight Type 2 Diabetes Patients: a Randomised, Double-Blind, Placebo-Controlled Trial. Diabetologia 59 (7), 1412–1421. doi:10.1007/s00125-016-3938-z
Control Group Turnbull, F. M., Turnbull, F. M., Abraira, C., Anderson, R. J., Byington, R. P., Chalmers, J. P., et al. (2009). Intensive Glucose Control and Macrovascular Outcomes in Type 2 Diabetes. Diabetologia 52 (11), 2288–2298. doi:10.1007/s00125-009-1470-0
Veroniki, A. A., Vasiliadis, H. S., Higgins, J. P., and Salanti, G. (2013). Evaluation of Inconsistency in Networks of Interventions. Int. J. Epidemiol. 42 (1), 332–345. doi:10.1093/ije/dys222
Vijan, S. (2019). Type 2 Diabetes. Ann. Intern. Med. 171 (9), ITC65–ITC80. doi:10.7326/AITC201911050
White, I. R., Barrett, J. K., Jackson, D., and Higgins, J. P. (2012). Consistency and Inconsistency in Network Meta-Analysis: Model Estimation Using Multivariate Meta-Regression. Res. Synth. Methods 3 (2), 111–125. doi:10.1002/jrsm.1045
White, I. R. (2009). Multivariate Random-Effects Meta-Analysis. Stata J. 9, 40–56. doi:10.1177/1536867x0900900103
White, I. R. (2011). Multivariate Random-Effects Meta-Regression: Updates to Mvmeta. Stata J. 11, 255–270. doi:10.1177/1536867x1101100206
Wilcox, T., De Block, C., Schwartzbard, A. Z., and Newman, J. D. (2020). Diabetic Agents, from Metformin to SGLT2 Inhibitors and GLP1 Receptor Agonists: JACC Focus Seminar. J. Am. Coll. Cardiol. 75 (16), 1956–1974. doi:10.1016/j.jacc.2020.02.056
Keywords: cardio-renal benefit, glucagon-like peptide-1 receptor agonist, network meta-analysis, semaglutide, type 2 diabetes
Citation: Zhuo C, Lin C, Zhou C, Gao X, Shao H, Fang T, Tian H, Ding L and Liu M (2021) Comparative Cardio-Renal Outcomes of Type 2 Diabetes Patients Administered Glucagon-Like Peptide-1 Receptor Agonists: A Network Meta-Analysis. Front. Pharmacol. 12:759262. doi: 10.3389/fphar.2021.759262
Received: 16 August 2021; Accepted: 18 November 2021;
Published: 24 December 2021.
Edited by:
Liberato Berrino, University of Campania Luigi Vanvitelli, ItalyReviewed by:
Celestino Sardu, University of Campania Luigi Vanvitelli, ItalyHaibo Wang, Peking University, China
Copyright © 2021 Zhuo, Lin, Zhou, Gao, Shao, Fang, Tian, Ding and Liu. This is an open-access article distributed under the terms of the Creative Commons Attribution License (CC BY). The use, distribution or reproduction in other forums is permitted, provided the original author(s) and the copyright owner(s) are credited and that the original publication in this journal is cited, in accordance with accepted academic practice. No use, distribution or reproduction is permitted which does not comply with these terms.
*Correspondence: Chuanjun Zhuo, Y2h1YW5qdW56aHVvdGptaEAxNjMuY29t, Y2h1YW5qdW56aHVvQG5hbmthaS5lZHUuY24=; Li Ding, ZGluZ2xpdG1sQHRtdS5lZHUuY24=; Ming Liu, bWluZ2xpdUB0bXUuZWR1LmNu
†These are the co-corresponding authors of this work