- 1Key Laboratory of Medical Science and Laboratory Medicine of Jiangsu Province, School of Medicine, Jiangsu University, Zhenjiang, Jiangsu, China
- 2Nanjing Jiangning Hospital, Nanjing, Jiangsu, China
- 3School of Medical Technology, Zhenjiang College, Zhenjiang, Jiangsu, China
- 4Directorate of University Health Services, University of Cape Coast, Cape Coast, Ghana
Farnesoid-X receptor (FXR), as a nuclear receptor activated by bile acids, is a vital molecule involved in bile acid metabolism. Due to its expression in immune cells, FXR has a significant effect on the function of immune cells and the release of chemokines when immune cells sense changes in bile acids. In addition to its regulation by ligands, FXR is also controlled by post-translational modification (PTM) activities such as acetylation, SUMOylation, and methylation. Due to the high expression of FXR in the liver and intestine, it significantly influences intestinal homeostasis under the action of enterohepatic circulation. Thus, FXR protects the intestinal barrier, resists bacterial infection, reduces oxidative stress, inhibits inflammatory reactions, and also acts as a tumor suppressor to impair the multiplication and invasion of tumor cells. These potentials provide new perspectives on the treatment of intestinal conditions, including inflammatory bowel disease (IBD) and its associated colorectal cancer (CRC). Moreover, FXR agonists on the market have certain organizational heterogeneity and may be used in combination with other drugs to achieve a greater therapeutic effect. This review summarizes current data on the role of FXR in bile acid metabolism, regulation of immune cells, and effects of the PTM of FXR. The functions of FXR in intestinal homeostasis and potential application in the treatment of IBD and CRC are discussed.
Introduction
IBD includes Crohn’s disease (CD) and ulcerative colitis (UC), which over time could progress to CRC (Nadeem et al., 2020). IBD has advanced into a worldwide disease and the prevalence is still increasing, adversely affecting the quality of life of patients (Kaplan, 2015). The pathogenesis involves intestinal ecological environment disorders and autoimmune disorders, and there is no clearly defined etiology. At present, there are many treatment choices, including surgery, medication therapy, fecal microbiota transplantation, etc., but there are still many limitations. For example, fecal microbiota transplantation as a treatment is aimed at Clostridium difficile, and the intestinal microbiota is complex, which is easy to cause the recurrence of IBD (Ghouri et al., 2020). Therefore, there is an urgent hope for enhanced beneficial therapeutic methods that can adapt to the complex composition and microbial environment of the intestinal tract.
Different bile acid receptors are activated in the process of bile acid metabolism, including FXR, vitamin D receptor (VDR), pregnane X receptor (PXR), and G protein-coupled bile acid receptor 1 (GPBAR1). FXR is mainly activated by primary bile acids while GPBAR1 is mainly activated by secondary bile acids, and FXR controls the synthesis and secretion of bile acids along with the absorption of bile acids by the gut and liver (Chiang, 2013; Fiorucci et al., 2022). The activation of FXR directly affects bile acid synthesis and the size of the bile acid pool, and its ability to regulate bile acid composition indirectly affects gut microbial diversity, immune response, and the activity of other bile acid receptors (Nie et al., 2015; Fiorucci et al., 2021a). The nuclear FXR was first named in the 1990s due to its activation by the farnesol metabolite. FXR exists in many organs, but primarily in the liver and intestine, and is involved in the regulation of metabolisms. FXR is also expressed in many immune cells such as natural killer T (NKT) cells, mast cells, monocytes, and macrophages (Li et al., 2019; Fiorucci et al., 2022). In response to changes in bile acids, immune cells trigger a series of intracellular FXR activities, therefore, the function of FXR in the immune system is of great value in the study of inflammation and tumor. However, current research mainly focuses on the interaction between FXR and immune cells on liver inflammation and liver cancer. As a vital regulatory receptor of bile acids in the enterohepatic circulation, the interaction between FXR and immune cells in the progression of intestinal inflammation and tumor has not been well explored.
Bile acids could function as endogenous ligands for FXR activation and agonists for FXR have been developed to treat diseases (Anderson and Gayer, 2021). Recently, researchers have turned their attention to the PTM of FXR. An example is the glucose non-oxidant hexosamine biosynthesis pathway that regulates FXR, where the amino-terminal independent transcriptional activation domain 1 (AF1) of FXR interacts with O-GlcNAc transferase and is O-GlcNAcylated. Increased FXR O-GlcNAcylation can subsequently up-regulate FXR gene expression and improve protein stability (Berrabah et al., 2014). Similarly, hepatic FXR phosphorylation at a single residue Tyr-67 is crucial for preventing atherosclerosis in mice (Byun et al., 2019). These indicate that the dysregulation of PTM is closely related to metabolic disorders.
Extensive studies have found that FXR is closely linked to intestinal homeostasis. Abnormal bile acid metabolism gives rise to increased intestinal permeability, causing bacterial translocation (BTL), and FXR protects the intestinal mucosal barrier and prevents bacterial invasion (Verbeke et al., 2015). In addition, FXR is involved in the regulation of oxidative stress and inflammatory response and is connected with cancer-related WNT/β-catenin signaling. Thus, modulating FXR activity has broad therapeutic promise in IBD and related CRC (Yu et al., 2020a; Hua et al., 2021; Miyazaki et al., 2021). Meanwhile, a number of drugs have also been found to regulate FXR and may be used in combination with FXR agonists to compensate for their limitations. This article reviews the structural characteristics of FXR, its metabolic regulation and PTMs, its association with immune cells, the regulation of intestinal homeostasis, and the research progress in IBD and CRC.
Structural features of FXR
FXR is a type of nuclear receptor activated by bile acid and encoded by the NR1H4 (nuclear receptor subfamily 1, group H, member 4) gene (Keitel et al., 2019; Ramos Pittol et al., 2020). It is distributed in many organs, among which the liver and intestines are most abundant in expression (Wang et al., 2008a). FXR has a typical nuclear receptor structure, including AF1, DNA binding domain (DBD), hinge region, ligand-binding domain (LBD), and carboxy-terminal ligand-dependent transcriptional activation domain 2 (AF2) (Jiang et al., 2021). AF1 and AF2 are the regions of FXR molecules responsible for interacting with regulatory proteins. Ligand-activated FXR connects to the FXR response elements (FXREs) in target genes and can act heterodimers of retinoid X receptor (RXR) or monomers to regulate metabolisms (Stojancevic et al., 2012). FXR commonly exists in the form of FXRα and FXRβ in mammals. FXRα is conserved in humans, encoding four subtypes of FXRα (FXRα 1, FXRα 2, FXRα 3, and FXRα 4), which is the result of diverse uses of promoters and variable splicing of RNA. The expression of FXR is tissue-dependent, with the liver expressing FXRα1/2 at a similar level to FXRα3/4, while the intestine mainly expresses the FXRα3/4 subtypes. Differently, FXRβ is a pseudogene in humans but encodes an available receptor in other species (Wang et al., 2018; Jiang et al., 2021). Due to its characteristics as a ligand-activated transcription factor, explorations of FXR agonists have achieved good results in recent years.
FXR in bile acid and cholesterol metabolism
It is well known that bile acids are produced by the liver and are reabsorbed in the small intestine through the action of enterohepatic circulation, with approximately 95% of bile acids being reabsorbed in the small intestine (Ocvirk and O'Keefe, 2021; Hofmann, 1984). FXR is the main regulatory factor of bile acid metabolism in the body. Bile acids work as endogenous ligands of FXR, with different capacities of activation, such as chenodeoxycholic acid (CDCA) > deoxycholic acid (DCA) > lithocholic acid (LCA) > cholic acid (CA) (Fiorucci et al., 2021b). FXR controls the size of bile acid pools by changing the vitality of cholesterol 7 alpha-hydroxylase (CYP7A1) to ensure the influx and outflow of bile acids. Moreover, FXR has certain detoxification functions (Kundu et al., 2015). FXR regulates the metabolic homeostasis of bile acids mainly through the hepatic FXR/small heterodimer partner (SHP) axis and the ileal fibroblast growth factor 15/19 (FGF15/19)/hepatic FGF receptor 4 (FGFR4) axis (Fiorucci et al., 2020), which inhibit CYP7A1 activity and thereby inhibit bile acid synthesis (Manley and Ding, 2015; Ticho et al., 2019).
In a study by Kong et al., the effect of FXR deficiency on alcoholic liver disease (ALD) was investigated, reporting that alcohol feeding changed serum and liver bile acid profiles of FXR knockout (FXR-KO) mice. FXR deficiency led to alcoholic hepatitis, increased bile acid synthesis and level, changed bile acid pool composition, and worsened hepatotoxicity (Kong et al., 2019). Since bile acids are made from cholesterol, FXR has been linked to cholesterol metabolism (Makishima, 2005; Chiang, 2013). Moreover, CYP7A1 converts cholesterol into bile acids and is the main way to eliminate cholesterol in the body (Niesor et al., 2001). FXR can control the expression of the CYP7A1 (Cai et al., 2010; Xiao-Rong et al., 2021). It is also reported that there is excessive accumulation of cholesterol in the arterial wall with dysregulation of ceramide metabolism in patients with atherosclerosis (Chambers et al., 2019; Zhang et al., 2019), and sphingomyelin phosphodiesterase 3 (SMPD3) serves as a key enzyme that promotes the sphingomyelin to hydrolyze into ceramide (Liang et al., 2021). Interestingly, SMPD3 has been identified as a target gene for FXR. Intestinal FXR is activated in high-fat-fed mice and the use of intestinal FXR inhibitors could reduce ceramide production and control hypercholesterolemia, leading to new directions in the treatment of atherosclerosis (Wu et al., 2021).
It has been suggested that hepatic and intestinal FXR have different functions in regulating bile acid homeostasis. Intestinal FXR mainly inhibits CYP7A1, while the hepatic FXR mainly inhibits sterol 12 alpha-hydroxylase (CYP8B1), and the inhibitory effect on CYP7A1 is far less than that of intestinal FXR. The main reason is the effect of intestinal FXR-FGF15 signal. Under the action of FXR agonist GW4064, FGF15 is almost not induced in the ileum of mice with intestine-specific FXR deficiency, but the expression of FGF15 is higher in the ileum of mice with liver-specific FXR deficiency. GW4064 significantly inhibited CYP7A1 expression in liver FXR-specific knockout mice but had no significant effect on intestine FXR-specific knockout mice. At the same time, the loss of FGF15 caused GW4064 to significantly inhibit the level of CYP8B1 but had no significant effect on the level of CYP7A1. The fecal DCA content of mice with intestinal FXR-specific deficiency was also much higher than that of mice with liver-specific deficiency of FXR (Kim et al., 2007). Similarly, Modica et al. also found that selective activation of intestinal FXR could induce FXR-FGF15 signaling, and then reduce the total bile acid pool, which is sufficient to relieve the symptoms of cholecystitis and intestinal mucosal damage (Modica et al., 2012). To some extent, hepatic FXR and intestinal FXR have complementary effects on the inhibition of bile acid synthesis. The effects of hepatic FXR are mainly focused on the protection of cholesterol homeostasis and associated lipid accumulation levels. Mice with liver-specific FXR deficiency have higher serum triglyceride and cholesterol levels than mice with intestine-specific FXR deficiency. Moreover, there is more lipid accumulation in liver tissue sections, and specific deficiency of liver FXR induced high levels of the liver X receptor (LXR), however, this effect was not related to intestinal FXR-FGF15 signaling (Schmitt et al., 2015).
FXR and immune cells
Not only can FXR take part in the regulation of metabolism, but also the modulation of immune cells. Changes in bile acid metabolism affect the transformation of immune cytokines and the impacts on immune cells. Therefore, as a key regulatory receptor of bile acid metabolism, FXR plays a crucial regulatory role in the immune system. Studies in this area have focused on both direct effects due to the activation of FXR and indirect effects that are related to changes in bile acid pool composition.
T cells and NKT cells
While changes in bile acid metabolism affect T cells, T cells can also directly affect changes in the bile acid spectrum. Cholangitis induced by the adoptive infusion of CD8+T cells into the liver inhibits the production of unbound bile acids, and upregulates the level of bound bile acids, but does not lead to hepatocyte apoptosis. Therefore, to some extent, T cells do not produce harmful bile acid metabolism. However, this process requires the involvement of the proinflammatory factors tumor necrosis factor (TNF) and interferon-γ (IFN-γ), both of which depend on changes in hepatic FXR levels (Glaser et al., 2019). Except for the uncommon NKT cells, there is currently no evidence that T cells themselves express FXR (Fiorucci et al., 2022). An abnormal bile acid signal is also a promoter of tumorigenesis. As one of the components of bile acid, Nor Cholic acid (NorCA) can promote the immune escape of liver cancer cells and obviously increase Programmed Cell Death-1 (PD-1) on the surface of CD4+ T cells by destroying the FXR/SHP signal axis, accompanied by upregulation of Programmed Cell Death Ligand-1 (PD-L1) in hepatocellular carcinoma (HCC) cells and their secreted exosomes. It is well documented that PD-1/PD-L1 compromises T cell activity, which is related to the immune escape of tumors. However, under the treatment with the FXR agonist GW4064, the levels of PD-1 and PD-L1 are significantly inhibited. Gong et al. used a combination of FXR agonist and anti-PD-1 antibody to highlight insights into the use of the tumor immune microenvironment against HCC for the treatment of other tumors (Gong et al., 2021). Under inflammatory conditions, effector T cells can be recruited to the intestinal tract through the α4β7-Madcam1 axis to promote the inflammatory process. Under the action of obeticholic acid (OCA), the expression of chemokine Madcam1 can be significantly inhibited, thereby reducing the aggregation of effector T cells to the intestinal tract, while regulatory T cells (Tregs) rely on the CCL25-CCR9 axis to home to the intestine to play their role in inhibiting inflammation (Massafra et al., 2016). In another study, CDCA promoted the expression of FXR in an acute murine asthma model and inhibited the production of T-helper (Th) 2 cytokines, mainly interleukin (IL)-4, IL-5, and IL-13, and decreased the level of immunoglobulin E (IgE) in the lung. Thus, FXR induces the function of T cytokines and is also a lifesaving mechanism against respiratory inflammatory diseases (Shaik et al., 2015).
NKT cells are a special subset of T cells that express both NK cell receptors and T cell receptors (Cui and Wan, 2019). Triptolide (TP), an immunosuppressant, stimulates invariant Natural Killer T (iNKT) cells to secrete Th 2 cytokines, and at the same time, interacts with type 2 NKT cells to inhibit their protective effect on the liver. FXR expression is inhibited by TP at both the transcriptional and translational levels. However, the knockdown of iNKT cells alleviates liver injury and up-regulates FXR expression. Therefore, the combination of TP and FXR agonists may alleviate liver injury caused by TP (Zou et al., 2020). Considering the potential of FXR agonists, scholars are exploring how to efficiently absorb them during their application. Ji et al. created OCA-nanoemulsion (OCA-NE) to better attach OCA to the liver, which more accurately promotes the secretion of CXC chemokine ligand 16 (CXCL16) by liver sinusoidal endothelial cells (LSECs) compared with the traditional oral OCA. In turn, NKT cells are triggered to accumulate in the tumor site to exert an anti-tumor immune effect (Ji et al., 2020). Moreover, NKT cells themselves also express FXR. High expression of osteopontin (OPN) in NKT cells induced by Concanavalin A (ConA) can promote the expression of glycosylated protein OPN in liver inflammation. However, under the action of 6alpha-ethyl-chenodeoxycholic acid (6-ECDCA), also known as INT-747 and OCA (Pellicciari et al., 2002; Fiorucci et al., 2022), the FXR/SHP signaling axis is effectively activated and the development of inflammation is inhibited (Mencarelli et al., 2009).
Macrophages and monocytes
Macrophages play a momentous role in various immune diseases, M1 and M2 are different subtypes that adapt to environmental changes. M1 macrophage plays a role in promoting inflammation and its main markers include CD80, CD86, and inducible nitric oxide synthase (iNOS), while M2 type plays an antagonistic role in inflammation, and its main markers are arginase 1 (Arg1), CD206, and CD163 (Kadomoto et al., 2021). Yao et al. found that the activation of FXR with GW4064 alleviates liver injury caused by lipopolysaccharide (LPS) by disturbing the release of inflammatory cytokines from macrophages in the non-alcoholic fatty liver disease (NAFLD) mice model. In addition, the apoptosis of liver cells was observably reduced and the infiltration of macrophages with the F4/80 marker in liver tissue was also suppressed (Yao et al., 2014). Interestingly, Cao et al. reached similar conclusions in chronic periaortitis, an autoimmune disease distinguished by fibrosis and increased inflammatory response. CDCA, as a natural agonist of FXR and a component of bile acids, has a defensive increase in chronic periaortitis patients to promote the nuclear translocation of FXR in macrophages and inhibit the production of IL-6, a pro-inflammatory and pro-fibrotic factor, although CDCA does not add to the overall expression of FXR in macrophages (Cao et al., 2021). Macrophages are the main source of monocyte chemoattractant protein-1 (MCP-1) which promotes the infiltration and invasion of macrophages to the inflammatory site, while CDCA can inhibit the expression of MCP-1. Mechanistically, CDCA promotes FXR to stick to a DR4 component in the promoter region of MCP-1, thereby inhibiting the expression of MCP-1 (Li et al., 2015). Therefore, it can be speculated that the CDCA-FXR axis works as a good modulator of the anti-inflammatory function of macrophages.
In the LPS-induced model of macrophage inflammation, SUMOylation of FXR in macrophages is inhibited and INT-747 could promote SUMOylation of FXR and stabilize the nuclear co-repressor (NCoR) at the promoters of iNOS and IL-1β, effectively inhibiting inflammation (Vavassori et al., 2009). In the study of Hao et al., it was found that bile acid metabolism was abnormal in patients with cholestasis. High levels of bile acids could act as damage-associated molecular patterns (DAMPs) to promote NOD-like receptor thermal protein domain associated protein 3 (NLRP3) inflammasome activation in macrophages, while FXR inhibits NLRP3 inflammasome activity. Primary macrophages isolated from FXR-KO mice also showed spontaneous NLRP3 activation compared with wild-type (WT) mice. The main reason is that FXR can directly interact with NLRP3 or caspase 1 physically, without the bridge of promoting FXR transcription in a ligand-dependent manner, so the presence of FXR plays an important anti-inflammatory role in macrophages (Hao et al., 2017). However, FXR can also inhibit the activation of NLRP3 inflammasome through an SHP-dependent pathway. In the study of Yang et al., SHP in macrophages can directly act on NLRP3 to inhibit the formation of NLRP3 and apoptosis-associated speck-like protein containing a caspase recruit domain (ASC) complex, thereby inhibiting the activation of NLRP3 inflammasome and reducing mitochondrial damage to relieve inflammation (Yang et al., 2015).
Monocytes include two subsets, Ly6Clow and Ly6Chigh. Ly6Clow monocytes suppress inflammation, while Ly6Chigh monocytes promote inflammation. There is evidence of the ability of monocytes to express FXR (Fiorucci et al., 2022). Dual FXR/GPBAR1 agonist (INT-767) significantly inhibits the development of liver inflammation and fibrosis in NAFLD mice, and the mechanism is that INT-767 promotes the increase of Ly6Clow monocytes and decrease of Ly6Chigh monocytes by directly targeting Ly6C levels. INT-767 also promotes the differentiation of monocytes into M2 macrophages (McMahan et al., 2013). These promising observations require that the specific molecular mechanism of INT-767 regulating FXR in monocytes be further elaborated in future studies. Chronic inflammation in conditions such as HIV/AIDS continues to be of major concern. Data indicates that the expression of nuclear receptors such as FXR, PXR, and RXR in monocytes of patients with HIV infection is significantly decreased. The highly active antiretroviral therapy (HAART) can only inhibit the replication of the virus but cannot restore the expression of nuclear receptors, and the development of chronic inflammation still persists. The combination of HAART and FXR agonists may inhibit both inflammatory responses and viral replication (Renga et al., 2012).
Mast cells
Mast cells (MCs), which are important innate immune cells, degranulate in response to stimuli (Espinosa and Valitutti, 2018). After degranulation, the bioactive ingredients produced by MCs affect inflammation, fibrosis, and even tumorigenesis (Mukai et al., 2018). In diarrhea irritable bowel syndrome (IBS-D) patients with high levels of total bile acids in the colonic mucosa that leads to visceral hypersensitivity (VH), Li et al. found that under the stimulation of bile acids, mucosal mast cells (MMCs) secrete nerve growth factor (NGF), a mediator that can damage colonic tight junctions. It is through the NGF/transient receptor potential vanillin (TRPV)1 axis that VH is produced. However, there is no bile acids-induced overexpression of NGF in FXR-KO mice. At the same time, FXR is expressed in MCs and regulates the level of NGF expressed by MMCs through regulating its downstream P38 mitogen-activated protein kinases (MAPK)/nuclear factor κB (NF-κB) signaling pathway (Li et al., 2019). The progress of primary sclerosing cholangitis (PSC) is often accompanied by the emergence of IBD. There is increased infiltration of hepatic MCs in cholestasis and the high level of MCs-FXR crosstalk with intestinal FXR/FGF15 signal induces the infiltration of intestinal MCs, which leads to the increase of inflammatory response and promotes the transduction of serum histamine (HA) signal. The inhibition of MC-FXR significantly improves the level of total bile acids and inhibits inflammation. Contrary to these observations, previous studies reported that high levels of FXR in MCs can lead to a harmful outcome, which may be related to the different effects of FXR on different types of immune cells (Meadows et al., 2021) (Figure 1).
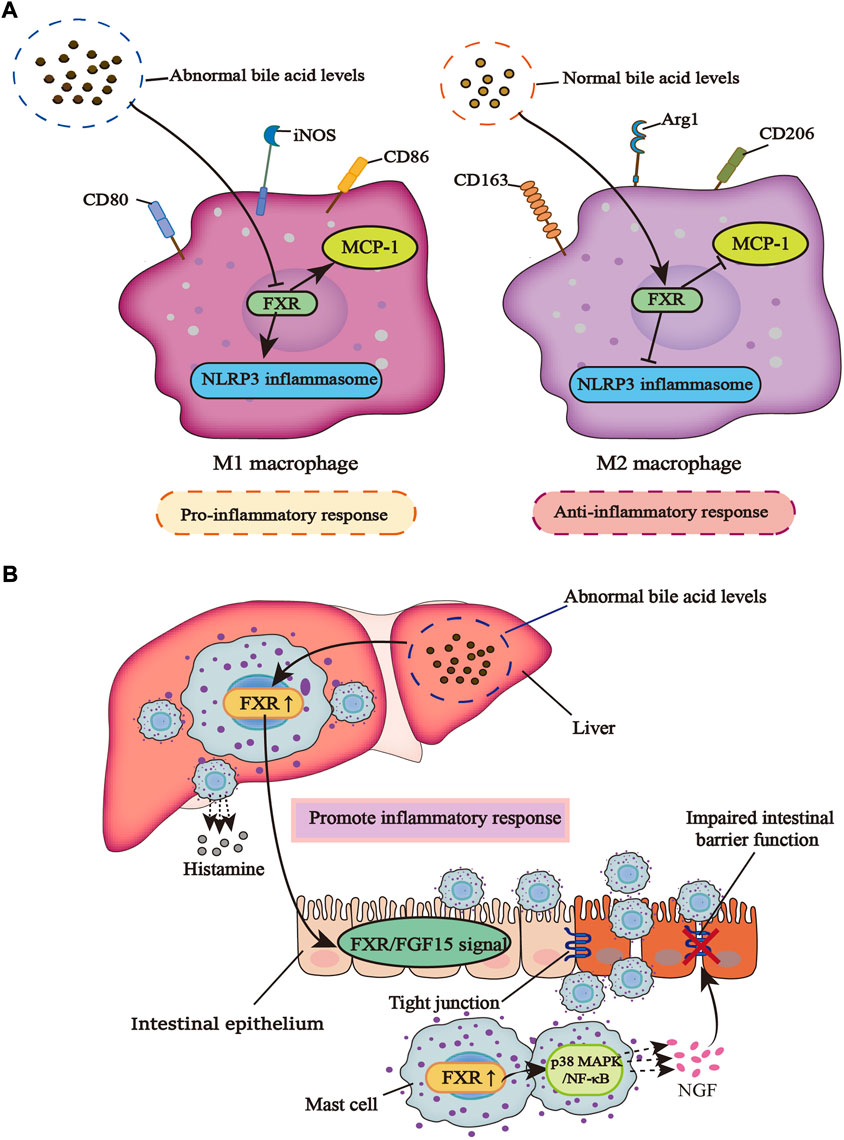
FIGURE 1. The different effects of FXR on macrophages and mast cells in an inflammatory response. (A) When bile acid metabolism is abnormal, macrophages are induced to the M1 type, which can promote inflammation and inhibit the regulation of FXR on NLRP3 inflammasome and MCP-1. However, in a normal bile acid metabolism, macrophages are polarized into the M2 type, which can inhibit inflammation and promote the regulation of FXR on NLRP3 inflammasome and MCP-1. (B) When bile acid metabolism is dysregulated, the infiltration of liver mast cells increases along with the increased release of histamine, which can promote the activity of FXR in liver mast cells. This leads to the activation of intestinal FXR/FGF15 signal under the action of enterohepatic circulation, and the infiltration of intestinal mast cells also increases. Activated FXR promotes the P38 MAPK/NF-κB signaling pathway, causing MCs to release more NGF, a mediator that can damage tight junctions in the gut, leading to a breakdown of the intestinal barrier and increased inflammatory response.
FXR agonists are now being considered as a treatment option for many diseases, and it is worth noting that their effects on immune cells vary between diseases (Table 1). And the FXR agonist, OCA, was originally discovered by Pellicciari and colleagues reported as 6-ECDCA (Pellicciari et al., 2002), and then christened by the same group as INT-747 and later renamed as OCA (Fiorucci et al., 2022). These different names have been used in different studies exploring the effects of FXR in different models of diseases.
Post-translational modifications of FXR
FXR can affect a myriad of physiological processes, including homeostasis and metabolism. Many studies prove that PTMs of FXR have an impact on the mechanism of action of diseases. PTM is crucial in regulating protein folding, activity, and function, allowing proteins to have multiple structures, perform more complex functions, and perform different tasks with greater precision, such as acetylation, SUMOylation, and methylation (He et al., 2018). Previous studies on the epigenetic modification of FXR could provide innovative ideas about diseases (Anbalagan et al., 2012; Wan and Sheng, 2018).
Acetylation
Using tandem mass spectrometry (MS/MS), Kim et al. found that the FXR hinge region, lysine (K)217 is the main acetylation site and also observed the acetylation of the DNA binding region K157. P300 and sirtuin 1(SIRT1) strictly adjust the acetylation of FXR, and P300 makes FXR acetylation. By employing molecular, cellular, and animal studies, it was confirmed that FXR is the regulatory target of SIRT1, SIRT1 has direct interaction with FXR and carries out FXR deacetylation, a process capable of destroying the stability of FXR and rendering it more easily degraded. When FXR is acetylated, it decreases the expression of FXR, impairs the heterodimerization of FXR/RXR with DNA, and reduces the trans-activation ability of FXR. Some scholars report that the levels of FXR acetylation are evidently higher in mice fed with a western-style fat diet for a long time than in mice with a normal diet. Thus, excessive acetylation of FXR results in harmful metabolism, which in turn harms health (Kemper et al., 2009). Resveratrol (RSV), a natural phenolic compound produced by plants, is a SIRT1 activator in nature (Deng et al., 2021), and reduces oxidative stress and prevents the production of pro-inflammatory factors by improving the antioxidant defense system. It was found that the reduced expressions of SIRT1, LXR, and FXR were associated with increased levels of lipid, alanine transaminase (ALT), alkaline phosphatase (ALP), and aspartate transaminase (AST) in rats with NAFLD, along with a significantly increased percentage of hepatocyte apoptosis (Hajighasem et al., 2013). The administration of RSV activates SIRT1, LXR, and FXR, and effectively improves abnormal lipid levels and hepatocyte apoptosis. As SIRT1 is an NAD+ histone deacetylase (HDAC)-dependent enzyme, it is strongly associated with gene regulation, apoptosis, autophagy, inflammation, and tumor occurrence (Alves-Fernandes and Jasiulionis, 2019). Therefore, making good use of SIRT1-FXR as a signal axis can widely regulate a variety of inflammatory and metabolic diseases (Kulkarni et al., 2016; Sun et al., 2020; Ma et al., 2021).
SUMOylation
There are three subtypes of small ubiquitin-like modifiers (SUMO) protein in mammals: SUMO-1, SUMO-2, and SUMO-3. SUMO-1 primarily embellishes physiological state proteins, while SUMO-2 embellishes stress proteins. SUMOylation is essential for the regulation of gene expression and the control of intracellular signal transduction since this pathway exists in almost all eukaryotes (Han et al., 2018). In their in vitro and in vivo experiments, Luo et al. found that FXR could be SUMOylated and therefore predicted that FXR SUMOylation might play a role in regulating liver function. There are several classic SUMO consensus sites in the AF-1 and LBD of FXR, and the sequential chromatin immunoprecipitation (ChIP-reChIP) confirmed the simultaneous binding of FXR and SUMO-1 to the bile salt export pump (BSEP) and SHP promoters, which are target genes of FXR. The overexpression of SUMO-1 significantly reduces the connection of FXR to SHP and BSEP promoters. Similarly, in HepG2 cells, small interfering RNA (siRNA) knockout of SUMO-1 increases the trans-activation of BSEP and SHP promoters. At the same time, the main attachment sites for SUMO-1 are K122 and 275 of FXR since K122 and 275 constrict SUMOylation consensus sites when mutated, eliminating FXR SUMOylation. It is worth noting that FXR SUMOylation is specific, reversible, and highly dynamic (Balasubramaniyan et al., 2013). Proteomic studies have shown that diet-induced acetylation of FXR at K217 occurs in mice. For example, 8–12 weeks of a high-fat diet (HFD) augmented the acetylation level of FXR but lessened the SUMO-2 level of FXR, causing a significantly activated expression of inflammatory genes. Interestingly, the SUMOylation of FXR promoted by GW4064 enhanced its connection with NF-κB. Selective restraint of inflammatory genes instead of influencing the expression of target genes of FXR/RXR demonstrates the anti-inflammatory effect of SUMO-FXR. Dysregulation of the FXR acetyl/SUMO switch may be the general mechanism by which anti-inflammatory responses of other transcriptional regulators are reduced, and may supply potential salutary and diagnostic methods for IBD and some inflammatory-related metabolic illnesses (Kim et al., 2015). Importantly, as early as 2009, Vavassori et al. found that FXR SUMOylation decreased in the model of macrophage inflammation induced by LPS, while FXR agonist INT-747 significantly promoted FXR SUMOylation and effectively inhibited the development of inflammation (Vavassori et al., 2009).
Interestingly, Gao et al. demonstrated that myocardial ischemia/reperfusion (MI/R) injury leads to massive apoptosis and that the levels of FXR SUMOylation play a role in regulating the apoptosis of cardiomyocytes. The SUMOylation of FXR occurs in normal heart tissues, and the SUMOylation of MI/R decreases, activating the mitochondrial apoptosis pathway (Gao et al., 2018). These results make clear that FXR SUMOylation also has great significance in apoptosis.
Methylation
Methylation is a vital modification of protein and nucleic acid, which goes hand in hand with many diseases such as cancer, senile dementia, and aging. The most common methylation modifications are DNA methylation and histone methylation. Intrahepatic cholestasis of pregnancy (ICP) is known as harmful bile acid metabolism during pregnancy, but symptoms quickly resolve after the end of pregnancy (Keitel et al., 2016). Therefore, some scholars believe that ICP pathogenesis may be deeply regulated by epigenetics. Based on samples from 88 ICP patients and 173 normal pregnant women in the third trimester, researchers showed that CpG dinucleotide of promoters of nuclear receptor subfamily 1 gene and adenosine triphosphate (ATP) binding box transporters were highly methylated during normal pregnancy, while methylation levels were decreased in ICP patients, and the methylation levels also influenced bile acid profiles. At the same time, the methylation of the distal and proximal promoters of the nuclear receptor FXR/NR1H4 had different effects. Since the distal promoters bind to transcription factors in a methylation-dependent manner and transcriptional regulatory elements can act as enhancers, they are more related to gene expression in the whole genome (Cabrerizo et al., 2014). Ann M. Bailey et al. found reduced FXR expression in early colon cancer owing to DNA methylation of the FXR promoter and enhanced Kirsten rat sarcoma viral oncogene (KRAS) signaling. DNA methylation is one mechanism that results in FXR silencing in colon cancer (Bailey et al., 2014). In addition, since BSEP is the target gene of FXR, FXR has histone methyltransferase activity at its locus, which is specific to arginine 17 of histone H3 and regulates BSEP expression (Ananthanarayanan et al., 2004).
In addition, the role of FXR phosphorylation and glycosylation has also been reported (Berrabah et al., 2014; Byun et al., 2019). The activity of FXR is mainly controlled by the ligand it binds to, but the PTM of FXR is another important factor, and PTM may be more influential than bile acid flux during feeding and fasting cycles (Panzitt and Wagner, 2021) (Table 2). At present, there is still little and limited research on the regulation of FXR and its target genes from the perspective of epigenetic modification. Perhaps, more discoveries can be made by focusing on this foothold.
FXR and intestinal homeostasis
FXR is the main regulatory factor of the entire hepatoenteric axis. Previous research mainly concentrated on the role of FXR and FXR agonists in liver diseases, but with the deepening of research, it has been found that FXR also shines in intestinal homeostasis, effectively protecting the intestinal mucosa, and plays a certain antibacterial role. The reports of a real-time quantitative PCR (RTQ-PCR) analysis indicated that FXR is mainly expressed in the ileum, colon, duodenum, and jejunum. Besides, FXR is not only highly expressed in the gut, but also has relative target genes that regulate it, including the SHP, ileal bile acid-binding protein (IBABP), and FGF15/19. However, there are many unknowns concerning how target genes are regulated and the mechanisms of action involved in intestinal disease (Inagaki et al., 2006). Under normal circumstances, there are a lot of bacteria in the intestinal tract, which restrict and depend on each other, forming a complex ecosystem. A study found that the number of aerobic bacteria in mesenteric lymph nodes of FXR-KO mice was 10 times more than that in WT mice (Inagaki et al., 2006). The excessive accumulation of the bile acids caused intestinal mucosal damage and bacterial proliferation, generating intestinal bacterial translocation, which is the transfer of bacteria from the intestinal lumen to lymph nodes of the mesentery and subsequently to external parts such as the peritoneum. In several cases, it can cause multiple organ failures and even death, while at the same time, the intestinal permeability can be increased and the intestinal epithelial barrier can be destroyed. In effect, FXR deficiency is associated with increased intestinal permeability, which then causes bacterial translocation. Moreover, the inactivation of intestinal FXR occurs in the rat’s model of cholestasis. The use of FXR agonist INT-747 restores intestinal permeability, promotes the expression of tight junction proteins claudin-1 and occludin in the ileum of rats, and promotes intestinal and systemic anti-inflammatory responses (Verbeke et al., 2015; Miyazaki et al., 2021).
Inflammasome activation plays a vital role in the immune defense of hosts against bacterial infection and is an important part of the natural immune system. Inflammasomes can discern pathogen-associated molecular patterns (PAMPs) or DAMPs to recruit and activate the pro-inflammatory protease caspase-1, where caspase-1 subsequently cleaves the precursors of IL-1β and IL-18, promoting the secretion of mature cytokines IL-1β and IL-18, a process valuable to intestinal homeostasis (Zhen and Zhang, 2019). Kang et al. demonstrated that the abnormal inflammasome activation in FXR-deficient mouse primary macrophage (BMDM) is associated with reduced IL-1β expression. After infection with Listeria monocytogenes or Escherichia coli, FXR-deficient BMDM showed decreased caspase-1 (P20) and caspase-11 (P30) activity compared to WT cells. The release of IL-1β was also observably lower than WT cells by enzyme-linked immunosorbent assay (ELISA). FXR-deficient mice were unable to effectively eliminate bacteria and had higher mortality and bacterial load than WT mice. Treatment with 6-ECDCA reduced bacterial load in vitro and increased survival in vivo. However, an interesting fact is that NLRP3 deficiency did not eliminate 6-ECDCA-induced bacterial clearance. Regardless, FXR could promote the inflammasome-mediated antibacterial response to a certain extent and may become a new target of antibacterial therapy (Kang et al., 2021). In a similar study, Zahiri et al. found that taurodeoxycholate (TDCA) supplementation promotes cell renewal to assist the maintenance of mucosal epithelium integrity and increases the expression of c-Myc, a key regulator of cell propagation, by increasing the expression of FXR. In addition, the intestinal villi length of mice treated with endotoxin was significantly shortened, but after TDCA supplementation, functional FXR maintained intestinal villi height compared with FXR-KO mice (Zahiri et al., 2011; Luo et al., 2019). Therefore, many FXR agonists have been developed to improve the activity of FXR and have been used to better execute the anti-inflammatory and antioxidant effects of FXR.
On the other hand, overactivation of FXR can sometimes lead to a negative outcome. Paneth cells are key elements of the intestinal stem cell (ISC) microenvironment (Schoenborn et al., 2019; Hou et al., 2020), generating antimicrobial peptides to protect the intestinal barrier (Battistini et al., 2020), and are usually found in the cecum and ascending colon (Yu et al., 2020b). Surprisingly, in western-diet-fed mice, overactivation of FXR was found to lead to defects in Paneth cells due to increased secondary bile acids (specifically DCA) in the ileum. Possible reasons for this observation include FXR overactivation directly affecting Paneth cells and FXR activation increasing the production of type I interferon in intestinal myeloid cells, resulting in abnormal Paneth cells (Liu et al., 2021). It can be seen that the regulatory mechanism of FXR in the intestinal tract is complicated to some extent, but in general, improving the activity of FXR must be more beneficial than harmful to intestinal diseases. In the regulation of intestinal homeostasis, FXR is still a new star worth exploring and full of potential.
The progress of FXR in IBD
The bile acid signal in IBD patients is significantly different from that of healthy people. The serum and fecal secondary bile acid levels are decreased while fecal-bound bile acid excretion is increased (Baars et al., 2015). This is closely related to the complex intestinal microbiota environment, as primary bile acids are modified into secondary bile acids by the intestinal microbiota. Studies indicate that the diversity of intestinal microbiota in IBD patients is decreased, with a characteristic reduction in firmicutes and elevation of proteobacteria. Since firmicutes are the main force of bile acid modification, their significant reduction in IBD patients affects the bile acid pattern. The protective effect of secondary bile acids on the gut decreases while primary bile acids increase (Franzosa et al., 2019; Li et al., 2021). In addition, a study found that the abundance of firmicutes and their bile salt biotransformation genes (BSBGs) were dominant in normal human and IBD samples in bioinformatics analysis of secondary bile acid metabolites, and the reduction of firmicutes in IBD was related to the reduced capacity of biliary salt transformation (Das et al., 2019). Since the ileum is primarily responsible for the reabsorption of conjugated bile acid, patients with CD often have bile acid malabsorption due to ileum dysfunction, which leads to a smaller pool of bile acids (Pavlidis et al., 2015; Vítek, 2015). The dysregulation of bile acid metabolism damages intestinal mucosa and promotes the inflammatory response. FXR serves as a key receptor regulating bile acid synthesis and plays an important role in IBD.
Bile acids pass through the intestine of the healthy person 6–10 times a day in response to the enterohepatic circulation. Repeated stimulation of the intestinal mucosa by hydrophobic bile acids can trigger intestinal inflammation, leading to cell death and apoptosis, and FXR can strictly control the homeostasis of bile acids in the human body to prevent their concentration from reaching cytotoxic levels (Ibrahim et al., 2019; Engin, 2021). Decreased intestinal FXR activity has been found in IBD patients and FXR mRNA expression is also reduced in inflamed colonic mucosa (Baars et al., 2015; Wilson et al., 2020). In our recent studies, we reported downregulated expression of FXR in both IBD patients and dextran sodium sulfate (DSS)-induced IBD mice model, which was accompanied by altered primary bile acid biosynthesis. Treatment of the animal model with mesenchymal stem cell-derived exosomes significantly restored colonic FXR alongside improved gut microbiota metagenomics and metabolomics, resulting in relieving macroscopic and microscopic features of IBD (Ocansey et al., 2022; Xu et al., 2022). In the mice model of colitis, the intestines of FXR-deficient mice show a more severe proinflammatory and profibrotic state, accompanied by immune dysfunction. INT-747 treatment could inhibit colitis effectively in WT mice, but not in FXR-deficient mice (Vavassori et al., 2009). The FXR agonist INT-747 also has been found to significantly reduce weight loss and prevent colon shortening in WT mice, meanwhile, FXR activation partially reduces goblet cell loss, protects the intestinal barrier, and inhibits inflammatory response (Gadaleta et al., 2011a) (Figure 2). In patients with IBD, high promotion of NF-κB is a key link to the inflammatory response, giving rise to strongly increased generation of pro-inflammatory cytokines (TNF-α, IL-8, and IL-6) and nitric oxide (NO) production. This is due to excessive activation of NF-κB subunits P50 and P65, which leads to FXR inhibition (Gadaleta et al., 2011b). In addition, target genes of FXR such as SHP, IBABP, and FGF15/19 are suppressed through reduced FXR (Wang et al., 2008b; Liu et al., 2016; Guo et al., 2021). Simultaneously, conservative NF-κB binding sites are also found in the promoter of the FXR target gene (Balasubramaniyan et al., 2016).
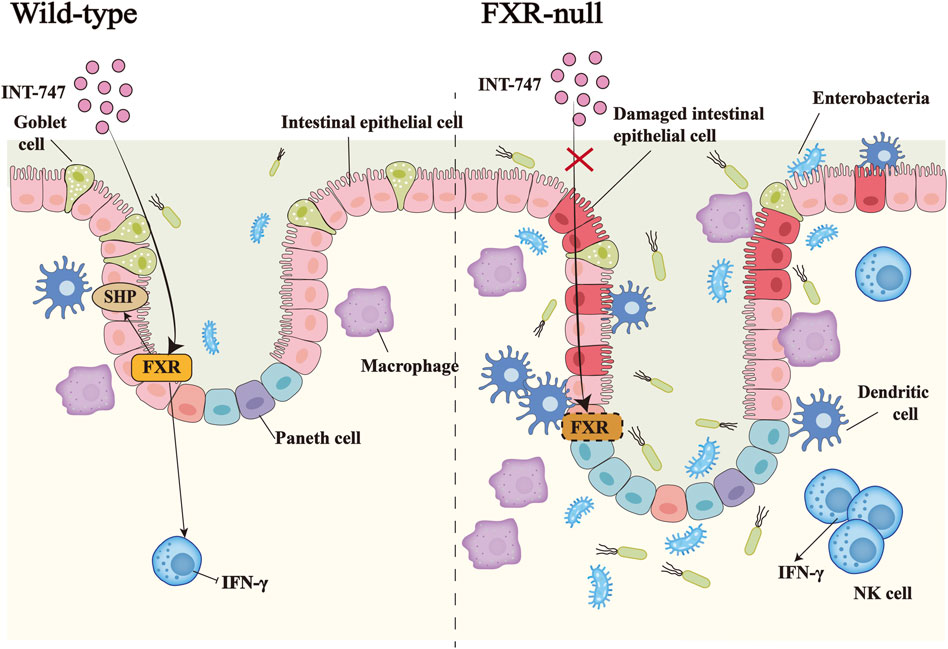
FIGURE 2. The importance of FXR in inflammatory bowel disease. In DSS-induced IBD, the administration of the FXR agonist INT-747 to FXR-KO mice and WT mice, activates the expression of FXR in the intestinal tract of WT mice, thereby promoting the upregulation of the target gene SHP of FXR and inhibiting the release of the pro-inflammatory factor IFN-γ by NK cells. INT-747 reduced intestinal permeability in WT mice, while FXR-KO mice could not have the same phenomenon due to the loss of the FXR gene. Meanwhile, FXR-KO mice had more damaged intestinal epithelial cells, fewer goblet cells, and increased bacterial invasion. The number of dendritic cells, macrophages, NK cells, and other immune cells also increased, which led to susceptibility to bacterial translocations.
Liu et al. constructed a model of IBD in WT and FXR KO mice by injecting LPS. Results showed that GW4064 inhibited LPS-induced toll-like receptor 4 (TLR4)/myeloid differentiation primary response 88 (MyD88) pathway and increased the levels of FXR protein and SHP mRNA. This relieved the breakdown of tight junction function caused by LPS and reduced macrophage infiltration in WT mice but had less impact in FXR KO mice. In other words, LPS resulted in a dramatically increased expression of TLR4 and MYD88 in WT and FXR KO mice, while treatment with GW4064 significantly reduced TLR4 and MYD88 protein levels in WT mice but did not significantly change in FXR KO mice. Similarly, LPS induced a reduced expression of zonula occludens-1 and claudin-1 in FXR KO mice compared to WT mice, while GW4064 markedly elevated their expression in WT mice, with little effect on FXR KO mice. By analyzing the markers F4/80 and CD11b of the ileum lamina proper macrophages, LPS upregulated F4/80 and CD11b in WT mice, while GW4064 treatment completely inhibited this effect. The markers F4/80 and CD11b in FXR KO mice were more highly expressed than in WT mice and showed an upward trend, however, GW4064 treatment could not reverse this outcome in FXR KO mice (Liu et al., 2017).
Some studies have reported that since the intestine and liver are connected through portal vein circulation, the regulatory function of bile acid is mainly mediated by bile acid receptors, and they can reduce intestinal and liver inflammation. By studying the liver of a 3% DSS-induced colitis model of mice, it was found that FXR and PXR were significantly down-regulated simultaneously in the intestinal tract and liver of mice. Elevated expression of pro-inflammatory cytokines IL-6 and IL-1β were found in both inflamed colon tissues and the liver. Since intestinal inflammation is usually associated with bacterial dysregulation, the adherent invasive Escherichia coli (AIEC) strain prototype LF82 was used as an inflammatory agent. The results of co-culture with intestinal cells and liver cells were also analyzed, where FXR expression was decreased but returned to normal after LF82 removal. These observations imply that the expression of bile acid receptors reinforces the relationship between the gut and the liver (Negroni et al., 2020). Similarly, Baitouweng Tang, a traditional Chinese herbal medicine, promoted the levels of hepatic FXR and GPBAR1 to improve the relative abundance of intestinal microorganisms such as Escherichia coli and Proteus and inhibited the activity of NF-κB to treat UC mice (Hua et al., 2021).
There is an imbalance in the intestinal fungal community in IBD and the abundance of Candida spp is high in stool cultures of IBD patients. After a large-scale analysis, it was found that the human intestinal fungus Candida metapsilosis M2006B and its metabolites could specifically activate FXR, which has a good therapeutic effect on colitis and has been verified in mouse models. Therefore, Candida metapsilosis M2006B may be a beneficial intestinal fungus for the treatment and prevention of IBD (Huo et al., 2022). In other studies, HFDs have been found to boost bile acid production and serve as a contributing factor to UC. For example, Zhao et al. demonstrated that HFD reduced FXR expression through the transforming growth factor-β (TGF-β) pathway, thereby increasing the incidence of UC. FexD, an FXR agonist, significantly increased TGF-β signaling, upregulated TGF-β receptor 1 (TGF-β R1) and Smad2, and effectively improved disease activity index scores and weight loss in HFD and DSS-induced UC mice. On this basis, the use of TGF-β R1 inhibitor SB431542 counteracts the effects of FexD, therefore, the activation of FXR may be considered an effective target for the treatment of UC (Zhao et al., 2020).
The occurrence of IBD is closely related to the destruction of the epithelial barrier. Studies have shown that the existence of intestinal bile acids has a certain relationship with the intestinal barrier. Since primary bile acids are transformed into secondary bile acids when transported to the intestinal lumen, Mroz et al. studied DCA and UDCA-two of the most common colon bile acids, in relation to the intestinal barrier. It was found that DCA as low as 25 μM increased the expression of FXR, but the increase of FXR also inhibited the repair of colon epithelial T84 cells. Boyden chamber was employed to observe the migration ability of cells and it was found that the migration ability was reduced after DCA treatment. Similar to DCA, upregulation of FXR by GW4064 significantly reduced wound closure and T84 cell migration in the Boyden chamber. Although both activate FXR, GW4064 and DCA do not superimpose but inhibit the activity of cystic fibrosis transmembrane conductance regulator (CFTR), which is essential for colon epithelial repair, thus inhibiting wound closure and reducing migration of intestinal epithelial cells. In contrast, UDCA treatment promoted colon epithelial repair in both cell and animal models, and prevented the harmful effects of DCA, suggesting that UDCA has a beneficial effect on IBD treatment. However, the specific mechanism is not yet clear (Mroz et al., 2018). Therefore, although FXR agonists are continuously being developed and applied, their treatment of diseases may not always bring good outcomes. In other words, the regulatory activity of FXR brings different effects, therefore, it is necessary to use FXR agonists with caution.
Therapeutic effects of FXR in colorectal cancer
As the third most general malignant tumor in the world, CRC owns an extremely high death rate (Bray et al., 2018). Risk factors include HFDs, abnormal bile acid metabolism, and disrupted gut microbiota (Miyazaki et al., 2021; Ocvirk and O'Keefe, 2021; Ocvirk et al., 2020). In human colon cancer samples, immunohistochemical (IHC) results show that there are fewer FXR markers in stage II adenocarcinoma compared to the normal colon. Moreover, mRNA levels of FXR are reduced several times in stages I, II, and III of colorectal adenocarcinoma, and are down-regulated in a stage-dependent manner, signifying that the silencing starts at the transcriptional level (Bailey et al., 2014). In several rodent models of tumorigenesis, FXR deficiency generates an obvious elevation in the size and number of colon tumors. Thus, FXR could provide targets for the prevention and treatment of CRC (Miyazaki et al., 2021).
The activation of epithelial-mesenchymal transformation (EMT) is a pivotal step in the transformation of colon cancer cells. At this stage, epithelial cells gain mesenchymal cell traits, enhancing the motility and migration of cancer cells. A primary identity of EMT is the downregulation of E-cadherin, which is associated with a poor prognosis of CRC (Vu and Datta, 2017). Yu et al. found that the number of lung metastasis nodules in HT-29-shFXR and Caco-2-shFXR was higher than the controls, along with decreased E-cadherin in HT-29-shFXR and Caco-2-shFXR cells but increased vimentin levels compared to control cells. The same observation was made in lung metastases in FXR KO BALB/C mice. Meanwhile, WNT signaling is involved in the development of EMT and FXR inhibits the WNT/β-catenin signaling activity of colon cancer cells. Activation of the WNT signaling gives rise to the increased expression of β-catenin in the cells, while overexpression of FXR can form a complex with β-catenin that disrupts the stability of the β-catenin/transcription factor 4 (TCF4) complex, thereby suppressing the transcriptional activity of WNT-related target genes. Conversely, it also antagonizes the FXR/RXRα complex and its transcriptional activity. SHP has also been shown to play a partial tumor-suppressive role, because FXR-mediated transcriptional activation of SHP inhibits the expression of cyclin D1 and C-C motif chemokine ligand 2 (CCL2), reducing the proliferation and invasion of tumor cells (Yu et al., 2020a).
As an agonist of FXR, OCA is nearly one hundred times more potent than the natural ligand of FXR, CDCA. OCA inhibits the activity and growth of HT-29 and Caco-2, delaying the proliferation of colon cancer cells by inhibiting G1/S transition and inducing apoptosis. EMT is also inhibited during the same process. The activation of FXR in an OCA-treated group restrained the development of xenograft tumors in nude mice in comparison to a dimethyl sulfoxide (DMSO) group. Immunohistochemical staining also revealed markers in xenograft tumors, weak Ki67 expression, but a strong caspase-3 expression. It has been shown that the activity of the Janus tyrosine kinases/signal and activator of transcription (JAK/STAT) pathway is strongly associated with colon cancer, where the suppressor of cytokine signaling-3 (SOCS3) negatively regulates the JAK/STAT pathway. OCA activation of FXR upregulates SOCS3 expression at the mRNA and protein levels and inhibits the JAK2/STAT3 pathway (Figure 3) (Li et al., 2020).
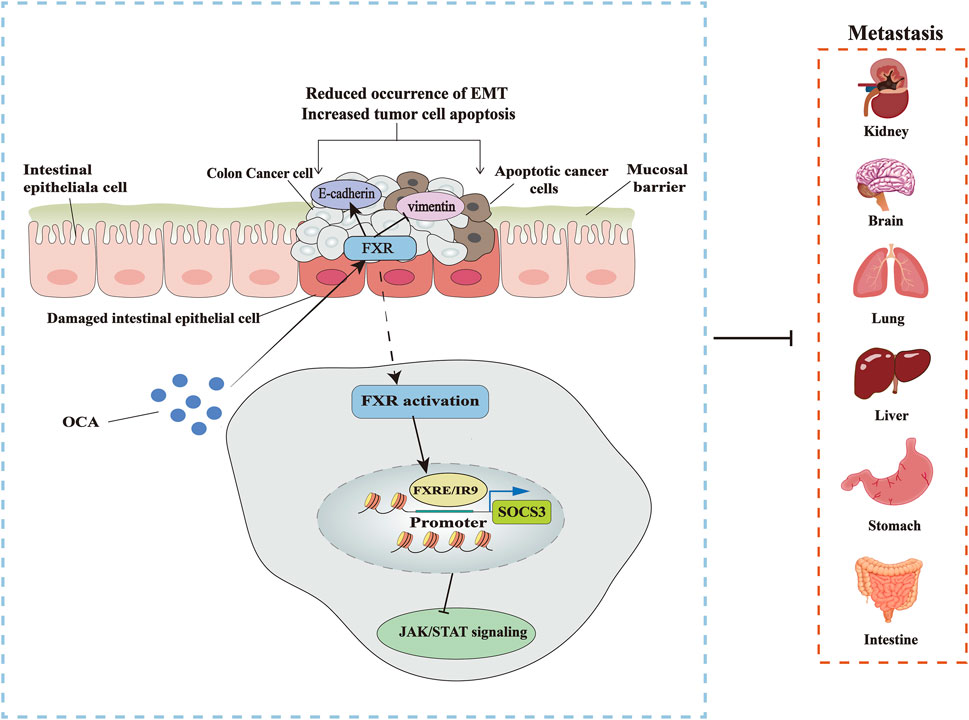
FIGURE 3. Targeting FXR to treat CRC. OCA inhibits the occurrence of EMT by activating FXR, thus blocking the metastasis of colon cancer cells and promoting the apoptosis of cancer cells, increasing the expression of EMT marker gene E-cadherin, and decreasing the level of vimentin. The specific mechanism is that OCA promotes the binding of FXR to SOCS3 promoter FXRE/IR9, inhibiting the JAK2/STAT3 pathway associated with colon cancer.
However, OCA is mainly used as a treatment for liver cancer and cholangiocarcinoma in previous studies. Due to the heterogeneity of tumors, its role in CRC may not have a good tumor inhibition effect. Recent studies have found that β-catenin interacts with FXR to affect the anti-tumor effect of OCA on CRC cells. Although OCA can increase the level of FXR in CRC cells, only RKO and HCT116 cells are the most sensitive to OCA, with a significant nuclear localization of FXR, while SW403, SW480, DLD-1, and HT-29 cells exhibit medium or mild nuclear localization. The expression of β-catenin in these four cells was also markedly higher than those in RKO and HCT116. By removing β-catenin expression, nuclear FXR was significantly elevated at 2 h after OCA treatment, whereas similar levels were seen at 6 h in control cells, leading to enhanced binding of FXR to SHP promoter. It is the level of β-catenin rather than its transcriptional activity that influences the antitumor effect of OCA. OCA was used to treat CRC in combination with nitazoxanide (NTZ), an antiparasitic agent that eliminates β-catenin expression. The average combination index (CI) showed strong synergistic inhibition of β-catenin in SW403, SW480, DLD-1, and HT-29 cells to better prevent the growth of cancer cells. Compared with single drug use, the combination of the two drugs elevated the apoptosis rate and the percentage of G0/G1 phase cells but reduced the percentage of S phase cells, and also impaired the invasion ability of the cancer cells. In vivo experiments also demonstrated that xenograft tumors in nude mice grew more slowly, smaller, and lighter under the influence of the combination of OCA and NTZ. In summary, the combined use of OCA and NTZ is more effective against CRC. NTZ also makes up for the deficiency of OCA to some extent (Yu et al., 2021).
Downstream targets of FXR include miRNAs that have a strong influence on the development of colon cancer. MiR-135A1 is highly produced in colon cancer specimens along with cell lines, while the level of the clinical significance of cyclin G2 (CCNG2), which can inhibit cell proliferation and promote apoptosis, is negatively correlated with miR-135A1 in human CRC tissues. Bioinformatics methods predicted and verified that CCNG2 is a downstream gene of miR-135A1. Since FXR is downregulated in CRC, the activation of FXR by GW4064 induces CCNG2 expression in a miR-135A1-dependent manner in vitro to hinder cell cycle progression and growth of colon cancer cells. Thus, the FXR/miR-135A1/CCNG2 axis could be a crucial treatment target for CRC (Qiao et al., 2018). Oxidative stress is the result of the imbalance between antioxidant and oxidant systems in the body, and the imbalance between the two has been linked to colon cancer (Basak et al., 2020). FXR can reduce reactive oxygen species (ROS) levels and act as an antioxidant (Dong et al., 2021). Nelumal A, a novel FXR agonist, attenuates oxidative stress by upregulating local antioxidant enzymes (including catalase, glutathione peroxidase, and superoxide dismutase) to inhibit azoxymethane (AOM)/DSS-induced CRC (Miyazaki et al., 2021).
Because cancer cells are prone to relapse and traditional chemotherapy has bad effects, innovative treatments are imminently needed to prolong survival rates for CRC patients. A large number of studies have shown that FXR is a potent inhibitor of tumor development and many FXR agonists can improve the activity of FXR, Table 3 summarizes the current studies about FXR agonists on CRC and IBD. Perhaps, starting from the molecular mechanism of its involvement in CRC pathogenesis could produce a good therapeutic outcome.
Conclusions and future perspectives
As a receptor of bile acid metabolism, FXR has been mainly focused on liver diseases in the past, but its role in the intestine has begun to be uncovered on account of the action of enterohepatic circulation. FXR exists in immune cells, therefore, FXR has a key regulatory role in the immune system although such studies regarding IBD and CRC are very scarce. When intestinal inflammation or tumor occurs, bile acid metabolism is disturbed and immune cells increase in infiltration, thus, research on FXR and immune cells in intestinal diseases is worth further exploration. In future studies, attention should be given to the relationship between the changes in intestinal FXR level and immune cells in the course of IBD and CRC, exploring the specific mechanisms involved. At the same time, it is necessary to consider whether changes in FXR levels expressed by immune cells themselves are related to intestinal inflammation and tumorigenesis. Although the role of FXR in the intestinal tract has been emphasized due to the influence of bile acids through enterohepatic circulation, previous studies have mainly focused on the treatment of intestinal diseases with FXR agonists. There is still a large gap in the study of IBD and related CRC regarding the regulation of FXR activity by PTMs. It would be interesting to investigate whether the PTM of FXR changes in intestinal diseases. Since there are many types of PTMs, the development of drugs targeting the significant PTM changes in FXR during the development of diseases may play a more precise therapeutic effect. It is also important to note that FXR agonists are not all good due to their tissue heterogeneity and have different effects on different diseases (Girisa et al., 2021). Currently, many drugs have been found to up-regulate FXR (Lu et al., 2021), therefore, it is worth exploring whether they can be used in combination with FXR agonists to enhance the therapeutic effects of FXR agonists on IBD and CRC, or whether they are not superimposable.
At present, the use of FXR agonists in clinical trials in patients with IBD and CRC is rare, although more promising results have been documented in cell and animal studies. However, since PSC is often associated with the development of IBD and has a high risk of progressing to CRC (Indriolo and Ravelli, 2014), results of clinical trials of FXR agonists in PSC could produce therapeutic ideas for IBD and CRC. Trauner et al. conducted a 12-week double-blind randomized phase II study of the nonsteroidal FXR agonist cilofexor for the treatment of PSC patients, where 60% of the screened PSC were associated with IBD. The results showed that cilofexor had certain level of safety and tolerability. Compared with the placebo group, the patients receiving 100 mg cilofexor had significantly lower levels of ALP, ALT, AST, and gamma-glutamyltransferase (GGT), and inhibited the activity of CYP7A1 to reduce bile acid synthesis. Both profibrotic and inflammatory factors were suppressed, but itch continued to develop in 36% of patients. Importantly, none of the patients who underwent the 12-week study had worsening IBD symptoms and no new IBD patients or symptoms were developed. Regardless, the risk of cardiovascular associated conditions remains to be explored (Trauner et al., 2019). In a phase II study of OCA, more than 50% of the PSC patients had IBD symptoms. OCA had a dose-dependent ALP reduction with or without UDCA but was associated with the occurrence of severe pruritus symptoms, where patients who received OCA 5–10 mg had significantly worse pruritus symptoms (Kowdley et al., 2020). In addition, during a 14-day treatment with 60 µg/day of the FXR agonist tropifexor, patients with primary bile acid diarrhea had increased expression of FGF19, inhibited bile acid synthesis, and slower overall colonic transport without causing pruritus (Camilleri et al., 2020). These observations indicate the clinical potential of FXR and bile acids in inflammatory-associated conditions; however, several challenges, including adverse events such as pruritis remain to be overcome. The dose-dependent pruritus associated with OCA may be related to the promiscuous activation of GPBAR1 by OCA (Sepe et al., 2018), as the activation of GPBAR1 can promote the excitation of sensory neurons that transmit itch and pain (Alemi et al., 2013).
Among the bile acid receptor family, FXR and GPBAR1 are the most characterized. GPBAR1 is also expressed in the liver and intestine and has certain anti-inflammatory effects. The use of a dual FXR/GPBAR1 ligand can be somewhat superior to the use of a single agonist (Fiorucci et al., 2022). The main dual FXR/GPBAR1 ligands studied so far are BAR502 and INT-767. BAR502, which primarily prioritized GPBAR1, significantly reduces liver fibrosis and improves metabolism by promoting fat browning in preclinical studies in non-alcoholic steatohepatitis (NASH) model mice. BAR502 induces an increase in the levels of FGF15, SHP, and glucagon-like peptide 1 (GLP-1) in the intestine, effectively inhibits the synthesis of bile acids and increases insulin sensitivity in mice (Carino et al., 2017). INT-767 mainly focuses on activating FXR, particularly, hepatic FXR but not the intestinal FXR. Compared with OCA, a lower dose of INT-767 shows more effect on liver steatosis, inflammation, and fibrosis in NASH model mice. However, INT-767 has a weaker ability to activate FGF15 in the ileum compared with OCA (Roth et al., 2018). In addition, GPBAR1 is also expressed in monocytes and macrophages. A study showed that INT-767 mainly focuses on activating GPBAR1 in macrophages to inhibit LPS-induced inflammation rather than activating FXR (Miyazaki-Anzai et al., 2014). The presence of GPBAR1 in macrophages can also inhibit the activation of NLRP3 inflammasome and promote the polarization of M2 macrophages (Shi et al., 2020). However, dual FXR/GPBAR1 ligands have been rarely studied in IBD and CRC, and are of great value in future exploration.
Author contributions
Conceptualization, MZ, DW, and XL; funding acquisition, DW and CY; project administration, FM; software, DO; visualization, YC; writing—original draft, MZ, DW, and YZ; writing—review and editing, DO. All authors have read and agreed to the published version of the manuscript.
Funding
This work was supported by the project of Zhenjiang key research and development plan (social development) (SH2021066), “169 Project” Scientific Research Project of Zhenjiang City, the open project of clinical medical research center of Gynecology and Traditional Chinese Medicine of Zhenjiang (Grant no. SS202204-KFC01), the 2022 Jiangsu Excellent postdoctoral program (Grant no. 2022ZB634), and the 20th batch of students' scientific research project approved by Jiangsu University (Grant no. 20A356).
Conflict of interest
The authors declare that the research was conducted in the absence of any commercial or financial relationships that could be construed as a potential conflict of interest.
Publisher’s note
All claims expressed in this article are solely those of the authors and do not necessarily represent those of their affiliated organizations, or those of the publisher, the editors and the reviewers. Any product that may be evaluated in this article, or claim that may be made by its manufacturer, is not guaranteed or endorsed by the publisher.
References
Alemi, F., Kwon, E., Poole, D. P., Lieu, T., Lyo, V., Cattaruzza, F., et al. (2013). The TGR5 receptor mediates bile acid-induced itch and analgesia. J. Clin. Invest. 123 (4), 1513–1530. doi:10.1172/JCI64551
Alves-Fernandes, D. K., and Jasiulionis, M. G. (2019). The role of SIRT1 on DNA damage response and epigenetic alterations in cancer. Int. J. Mol. Sci. 20 (13), E3153. doi:10.3390/ijms20133153
Ananthanarayanan, M., Li, S., Balasubramaniyan, N., Suchy, F. J., and Walsh, M. J. (2004). Ligand-dependent activation of the farnesoid X-receptor directs arginine methylation of histone H3 by CARM1. J. Biol. Chem. 279 (52), 54348–54357. doi:10.1074/jbc.M410021200
Anbalagan, M., Huderson, B., Murphy, L., and Rowan, B. G. (2012). Post-translational modifications of nuclear receptors and human disease. Nucl. Recept. Signal. 10, e001. doi:10.1621/nrs.10001
Anderson, K. M., and Gayer, C. P. (2021). The pathophysiology of farnesoid X receptor (FXR) in the GI tract: Inflammation, barrier function and innate immunity. Cells 10 (11), 3206. doi:10.3390/cells10113206
Appelman, M. D., van der Veen, S. W., and van Mil, S. W. C. (2021). Post-translational modifications of FXR; implications for cholestasis and obesity-related disorders. Front. Endocrinol. 12, 729828. doi:10.3389/fendo.2021.729828
Baars, A., Oosting, A., Knol, J., Garssen, J., and van Bergenhenegouwen, J. (2015). The gut microbiota as a therapeutic target in IBD and metabolic disease: A role for the bile acid receptors FXR and TGR5. Microorganisms 3 (4), 641–666. doi:10.3390/microorganisms3040641
Bailey, A. M., Zhan, L., Maru, D., Shureiqi, I., Pickering, C. R., Kiriakova, G., et al. (2014). FXR silencing in human colon cancer by DNA methylation and KRAS signaling. Am. J. Physiol. Gastrointest. Liver Physiol. 306 (1), G48–G58. doi:10.1152/ajpgi.00234.2013
Balasubramaniyan, N., Ananthanarayanan, M., and Suchy, F. J. (2012). Direct methylation of FXR by Set7/9, a lysine methyltransferase, regulates the expression of FXR target genes. Am. J. Physiol. Gastrointest. Liver Physiol. 302 (9), G937–G947. doi:10.1152/ajpgi.00441.2011
Balasubramaniyan, N., Ananthanarayanan, M., and Suchy, F. J. (2016). Nuclear factor-κB regulates the expression of multiple genes encoding liver transport proteins. Am. J. Physiol. Gastrointest. Liver Physiol. 310 (8), G618–G628. doi:10.1152/ajpgi.00363.2015
Balasubramaniyan, N., Luo, Y., Sun, A-Q., and Suchy, F. J. (2013). SUMOylation of the farnesoid X receptor (FXR) regulates the expression of FXR target genes. J. Biol. Chem. 288 (19), 13850–13862. doi:10.1074/jbc.M112.443937
Basak, D., Uddin, M. N., and Hancock, J. (2020). The role of oxidative stress and its counteractive utility in colorectal cancer (CRC). Cancers (Basel) 12 (11), E3336. doi:10.3390/cancers12113336
Battistini, C., Ballan, R., Herkenhoff, M. E., Saad, S. M. I., and Sun, J. (2020). Vitamin D modulates intestinal microbiota in inflammatory bowel diseases. Int. J. Mol. Sci. 22 (1), E362. doi:10.3390/ijms22010362
Benhamed, F., Filhoulaud, G., Caron, S., Lefebvre, P., Staels, B., and Postic, C. (2014). O-GlcNAcylation links ChREBP and FXR to glucose-sensing. Front. Endocrinol. 5, 230. doi:10.3389/fendo.2014.00230
Berrabah, W., Aumercier, P., Gheeraert, C., Dehondt, H., Bouchaert, E., Alexandre, J., et al. (2014). Glucose sensing O-GlcNAcylation pathway regulates the nuclear bile acid receptor farnesoid X receptor (FXR). Hepatol. Baltim. Md) 59 (5), 2022–2033. doi:10.1002/hep.26710
Bray, F., Ferlay, J., Soerjomataram, I., Siegel, R. L., Torre, L. A., and Jemal, A. (2018). Global cancer statistics 2018: GLOBOCAN estimates of incidence and mortality worldwide for 36 cancers in 185 countries. Ca. Cancer J. Clin. 68 (6), 394–424. doi:10.3322/caac.21492
Byun, S., Jung, H., Chen, J., Kim, Y-C., Kim, D-H., Kong, B., et al. (2019). Phosphorylation of hepatic farnesoid X receptor by FGF19 signaling-activated Src maintains cholesterol levels and protects from atherosclerosis. J. Biol. Chem. 294 (22), 8732–8744. doi:10.1074/jbc.RA119.008360
Cabrerizo, R., Castano, G. O., Burgueno, A. L., Fernandez Gianotti, T., Gonzalez Lopez Ledesma, M. M., Flichman, D., et al. (2014). Promoter DNA methylation of farnesoid X receptor and pregnane X receptor modulates the intrahepatic cholestasis of pregnancy phenotype. PLoS One 9 (1), e87697. doi:10.1371/journal.pone.0087697
Cai, S-Y., He, H., Nguyen, T., Mennone, A., and Boyer, J. L. (2010). Retinoic acid represses CYP7A1 expression in human hepatocytes and HepG2 cells by FXR/RXR-dependent and independent mechanisms. J. Lipid Res. 51 (8), 2265–2274. doi:10.1194/jlr.M005546
Camilleri, M., Nord, S. L., Burton, D., Oduyebo, I., Zhang, Y., Chen, J., et al. (2020). Randomised clinical trial: Significant biochemical and colonic transit effects of the farnesoid X receptor agonist tropifexor in patients with primary bile acid diarrhoea. Aliment. Pharmacol. Ther. 52 (5), 808–820. doi:10.1111/apt.15967
Cao, S., Meng, X., Li, Y., Sun, L., Jiang, L., Xuan, H., et al. (2021). Bile acids elevated in chronic periaortitis could activate farnesoid-X-receptor to suppress IL-6 production by macrophages. Front. Immunol. 12, 632864. doi:10.3389/fimmu.2021.632864
Carino, A., Cipriani, S., Marchianò, S., Biagioli, M., Santorelli, C., Donini, A., et al. (2017). BAR502, a dual FXR and GPBAR1 agonist, promotes browning of white adipose tissue and reverses liver steatosis and fibrosis. Sci. Rep. 7, 42801. doi:10.1038/srep42801
Chambers, K. F., Day, P. E., Aboufarrag, H. T., and Kroon, P. A. (2019). Polyphenol effects on cholesterol metabolism via bile acid biosynthesis, CYP7A1: A review. Nutrients 11 (11), E2588. doi:10.3390/nu11112588
Cui, Y., and Wan, Q. (2019). NKT cells in neurological diseases. Front. Cell. Neurosci. 13, 245. doi:10.3389/fncel.2019.00245
Das, P., Marcišauskas, S., Ji, B., and Nielsen, J. (2019). Metagenomic analysis of bile salt biotransformation in the human gut microbiome. BMC Genomics 20 (1), 517. doi:10.1186/s12864-019-5899-3
Deng, Z., Sun, M., Wu, J., Fang, H., Cai, S., An, S., et al. (2021). SIRT1 attenuates sepsis-induced acute kidney injury via Beclin1 deacetylation-mediated autophagy activation. Cell Death Dis. 12 (2), 217. doi:10.1038/s41419-021-03508-y
Dong, L., Yin, L., Li, R., Xu, L., Xu, Y., Han, X., et al. (2021). Dioscin alleviates lung ischemia/reperfusion injury by regulating FXR-mediated oxidative stress, apoptosis, and inflammation. Eur. J. Pharmacol. 908, 174321. doi:10.1016/j.ejphar.2021.174321
Engin, A. (2021). Bile acid toxicity and protein kinases. Adv. Exp. Med. Biol. 1275, 229–258. doi:10.1007/978-3-030-49844-3_9
Espinosa, E., and Valitutti, S. (2018). New roles and controls of mast cells. Curr. Opin. Immunol. 50, 39–47. doi:10.1016/j.coi.2017.10.012
Fiorucci, S., Baldoni, M., Ricci, P., Zampella, A., Distrutti, E., and Biagioli, M. (2020). Bile acid-activated receptors and the regulation of macrophages function in metabolic disorders. Curr. Opin. Pharmacol. 53, 45–54. doi:10.1016/j.coph.2020.04.008
Fiorucci, S., Carino, A., Baldoni, M., Santucci, L., Costanzi, E., Graziosi, L., et al. (2021). Bile acid signaling in inflammatory bowel diseases. Dig. Dis. Sci. 66 (3), 674–693. doi:10.1007/s10620-020-06715-3
Fiorucci, S., Distrutti, E., Carino, A., Zampella, A., and Biagioli, M. (2021). Bile acids and their receptors in metabolic disorders. Prog. Lipid Res. 82, 101094. doi:10.1016/j.plipres.2021.101094
Fiorucci, S., Zampella, A., Ricci, P., Distrutti, E., and Biagioli, M. (2022). Immunomodulatory functions of FXR. Mol. Cell. Endocrinol. 551, 111650. doi:10.1016/j.mce.2022.111650
Franzosa, E. A., Sirota-Madi, A., Avila-Pacheco, J., Fornelos, N., Haiser, H. J., Reinker, S., et al. (2019). Gut microbiome structure and metabolic activity in inflammatory bowel disease. Nat. Microbiol. 4 (2), 293–305. doi:10.1038/s41564-018-0306-4
Gadaleta, R. M., Oldenburg, B., Willemsen, E. C., Spit, M., Murzilli, S., Salvatore, L., et al. (2011). Activation of bile salt nuclear receptor FXR is repressed by pro-inflammatory cytokines activating NF-κB signaling in the intestine. Biochim. Biophys. Acta 1812 (8), 851–858. doi:10.1016/j.bbadis.2011.04.005
Gadaleta, R. M., van Erpecum, K. J., Oldenburg, B., Willemsen, E. C., Renooij, W., Murzilli, S., et al. (2011). Farnesoid X receptor activation inhibits inflammation and preserves the intestinal barrier in inflammatory bowel disease. Gut 60 (4), 463–472. doi:10.1136/gut.2010.212159
Gao, Y., Zhao, Y., Yuan, A., Xu, L., Huang, X., Su, Y., et al. (2018). Effects of farnesoid-X-receptor SUMOylation mutation on myocardial ischemia/reperfusion injury in mice. Exp. Cell Res. 371 (2), 301–310. doi:10.1016/j.yexcr.2018.07.004
Ghouri, Y. A., Tahan, V., and Shen, B. (2020). Secondary causes of inflammatory bowel diseases. World J. Gastroenterol. 26 (28), 3998–4017. doi:10.3748/wjg.v26.i28.3998
Girisa, S., Henamayee, S., Parama, D., Rana, V., Dutta, U., and Kunnumakkara, A. B. (2021). Targeting Farnesoid X receptor (FXR) for developing novel therapeutics against cancer. Mol. Biomed. 2 (1), 21. doi:10.1186/s43556-021-00035-2
Glaser, F., John, C., Engel, B., Höh, B., Weidemann, S., Dieckhoff, J., et al. (2019). Liver infiltrating T cells regulate bile acid metabolism in experimental cholangitis. J. Hepatol. 71 (4), 783–792. doi:10.1016/j.jhep.2019.05.030
Gong, Y., Li, K., Qin, Y., Zeng, K., Liu, J., Huang, S., et al. (2021). Norcholic acid promotes tumor progression and immune escape by regulating farnesoid X receptor in hepatocellular carcinoma. Front. Oncol. 11, 711448. doi:10.3389/fonc.2021.711448
Guo, D., He, L., Gao, Y., Jin, C., Lin, H., Zhang, L., et al. (2021). Obeticholic acid derivative, T-2054 suppresses osteoarthritis via inhibiting NF-κB-Signaling pathway. Int. J. Mol. Sci. 22 (8), 3807. doi:10.3390/ijms22083807
Hajighasem, A., Farzanegi, P., Mazaheri, Z., Naghizadeh, M., and Salehi, G. (2018). Effects of resveratrol, exercises and their combination on Farnesoid X receptor, Liver X receptor and Sirtuin 1 gene expression and apoptosis in the liver of elderly rats with nonalcoholic fatty liver. PeerJ 6, e5522 doi:10.7717/peerj.5522
Han, Z. J., Feng, Y. H., Gu, B. H., Li, Y. M., and Chen, H. (2018). The post-translational modification, SUMOylation, and cancer (Review). Int. J. Oncol. 52 (4), 1081–1094. doi:10.3892/ijo.2018.4280
Hao, H., Cao, L., Jiang, C., Che, Y., Zhang, S., Takahashi, S., et al. (2017). Farnesoid X receptor regulation of the NLRP3 inflammasome underlies cholestasis-associated sepsis. Cell Metab. 25 (4), 856–867. doi:10.1016/j.cmet.2017.03.007
He, W., Wei, L., and Zou, Q. (2018). Research progress in protein posttranslational modification site prediction. Brief. Funct. Genomics 18 (4), 220–229. doi:10.1093/bfgp/ely039
Hofmann, A. F. (1984). Chemistry and enterohepatic circulation of bile acids. Hepatol. Baltim. Md) 4 (5), 4S–14S. doi:10.1002/hep.1840040803
Hou, Q., Huang, J., Ayansola, H., Masatoshi, H., and Zhang, B. (2020). Intestinal stem cells and immune cell relationships: Potential therapeutic targets for inflammatory bowel diseases. Front. Immunol. 11, 623691. doi:10.3389/fimmu.2020.623691
Hua, Y-L., Jia, Y-Q., Zhang, X-S., Yuan, Z-W., Ji, P., Hu, J-J., et al. (2021). Baitouweng Tang ameliorates DSS-induced ulcerative colitis through the regulation of the gut microbiota and bile acids via pathways involving FXR and TGR5. Biomed. Pharmacother. = Biomedecine Pharmacother. 137, 111320. doi:10.1016/j.biopha.2021.111320
Hucke, S., Herold, M., Liebmann, M., Freise, N., Lindner, M., Fleck, A-K., et al. (2016). The farnesoid-X-receptor in myeloid cells controls CNS autoimmunity in an IL-10-dependent fashion. Acta Neuropathol. 132 (3), 413–431. doi:10.1007/s00401-016-1593-6
Huo, X., Li, D., Wu, F., Li, S., Qiao, Y., Wang, C., et al. (2022). Cultivated human intestinal fungus Candida metapsilosis M2006B attenuates colitis by secreting acyclic sesquiterpenoids as FXR agonists. Gut 2021, 325413. doi:10.1136/gutjnl-2021-325413
Ibrahim, S., Dayoub, R., Krautbauer, S., Liebisch, G., Wege, A. K., Melter, M., et al. (2019). Bile acid-induced apoptosis and bile acid synthesis are reduced by over-expression of Augmenter of Liver Regeneration (ALR) in a STAT3-dependent mechanism. Exp. Cell Res. 374 (1), 189–197. doi:10.1016/j.yexcr.2018.11.023
Inagaki, T., Moschetta, A., Lee, Y. K., Peng, L., Zhao, G., Downes, M., et al. (2006). Regulation of antibacterial defense in the small intestine by the nuclear bile acid receptor. Proc. Natl. Acad. Sci. U. S. A. 103 (10), 3920–3925. doi:10.1073/pnas.0509592103
Indriolo, A., and Ravelli, P. (2014). Clinical management of inflammatory bowel disease in the organ recipient. World J. Gastroenterol. 20 (13), 3525–3533. doi:10.3748/wjg.v20.i13.3525
Ji, G., Ma, L., Yao, H., Ma, S., Si, X., Wang, Y., et al. (2020). Precise delivery of obeticholic acid via nanoapproach for triggering natural killer T cell-mediated liver cancer immunotherapy. Acta Pharm. Sin. B 10 (11), 2171–2182. doi:10.1016/j.apsb.2020.09.004
Jiang, L., Zhang, H., Xiao, D., Wei, H., and Chen, Y. (2021). Farnesoid X receptor (FXR): Structures and ligands. Comput. Struct. Biotechnol. J. 19, 2148–2159. doi:10.1016/j.csbj.2021.04.029
Kadomoto, S., Izumi, K., and Mizokami, A. (2021). Macrophage polarity and disease control. Int. J. Mol. Sci. 23 (1), 144. doi:10.3390/ijms23010144
Kang, J. H., Kim, M., and Yim, M. (2021). FXR/TGR5 mediates inflammasome activation and host resistance to bacterial infection. Biochem. Biophys. Rep. 27, 101051. doi:10.1016/j.bbrep.2021.101051
Kaplan, G. G. (2015). The global burden of IBD: From 2015 to 2025. Nat. Rev. Gastroenterol. Hepatol. 12 (12), 720–727. doi:10.1038/nrgastro.2015.150
Keitel, V., Droge, C., and Haussinger, D. (2019). Targeting FXR in cholestasis. Handb. Exp. Pharmacol. 256, 299–324. doi:10.1007/164_2019_231
Keitel, V., Droge, C., Stepanow, S., Fehm, T., Mayatepek, E., Kohrer, K., et al. (2016). Intrahepatic cholestasis of pregnancy (ICP): Case report and review of the literature. Z. Gastroenterol. 54 (12), 1327–1333. doi:10.1055/s-0042-118388
Kemper, J. K., Xiao, Z., Ponugoti, B., Miao, J., Fang, S., Kanamaluru, D., et al. (2009). FXR acetylation is normally dynamically regulated by p300 and SIRT1 but constitutively elevated in metabolic disease states. Cell Metab. 10 (5), 392–404. doi:10.1016/j.cmet.2009.09.009
Kim, D-H., Xiao, Z., Kwon, S., Sun, X., Ryerson, D., Tkac, D., et al. (2015). A dysregulated acetyl/SUMO switch of FXR promotes hepatic inflammation in obesity. EMBO J. 34 (2), 184–199. doi:10.15252/embj.201489527
Kim, I., Ahn, S-H., Inagaki, T., Choi, M., Ito, S., Guo, G. L., et al. (2007). Differential regulation of bile acid homeostasis by the farnesoid X receptor in liver and intestine. J. Lipid Res. 48 (12), 2664–2672. doi:10.1194/jlr.M700330-JLR200
Kong, B., Zhang, M., Huang, M., Rizzolo, D., Armstrong, L. E., Schumacher, J. D., et al. (2019). FXR deficiency alters bile acid pool composition and exacerbates chronic alcohol induced liver injury. Dig. Liver Dis. 51 (4), 570–576. doi:10.1016/j.dld.2018.12.026
Kowdley, K. V., Vuppalanchi, R., Levy, C., Floreani, A., Andreone, P., LaRusso, N. F., et al. (2020). A randomized, placebo-controlled, phase II study of obeticholic acid for primary sclerosing cholangitis. J. Hepatol. 73 (1), 94–101. doi:10.1016/j.jhep.2020.02.033
Kulkarni, S. R., Soroka, C. J., Hagey, L. R., and Boyer, J. L. (2016). Sirtuin 1 activation alleviates cholestatic liver injury in a cholic acid-fed mouse model of cholestasis. Hepatology 64 (6), 2151–2164. doi:10.1002/hep.28826
Kundu, S., Kumar, S., and Bajaj, A. (2015). Cross-talk between bile acids and gastrointestinal tract for progression and development of cancer and its therapeutic implications. IUBMB Life 67 (7), 514–523. doi:10.1002/iub.1399
Li, L., Zhang, Q., Peng, J., Jiang, C., Zhang, Y., Shen, L., et al. (2015). Activation of farnesoid X receptor downregulates monocyte chemoattractant protein-1 in murine macrophage. Biochem. Biophys. Res. Commun. 467 (4), 841–846. doi:10.1016/j.bbrc.2015.10.056
Li, N., Zhan, S., Tian, Z., Liu, C., Xie, Z., Zhang, S., et al. (2021). Alterations in bile acid metabolism associated with inflammatory bowel disease. Inflamm. Bowel Dis. 27 (9), 1525–1540. doi:10.1093/ibd/izaa342
Li, S., Xu, Z., Guo, J., Zheng, J., Sun, X., and Yu, J. (2020). Farnesoid X receptor activation induces antitumour activity in colorectal cancer by suppressing JAK2/STAT3 signalling via transactivation of SOCS3 gene. J. Cell. Mol. Med. 24 (24), 14549–14560. doi:10.1111/jcmm.16083
Li, W-T., Luo, Q-Q., Wang, B., Chen, X., Yan, X-J., Qiu, H-Y., et al. (2019). Bile acids induce visceral hypersensitivity via mucosal mast cell-to-nociceptor signaling that involves the farnesoid X receptor/nerve growth factor/transient receptor potential vanilloid 1 axis. FASEB J. 33 (2), 2435–2450. doi:10.1096/fj.201800935RR
Liang, Y., Wang, Y., Wang, W., Zhao, J., Xu, M., and Zheng, M. (2021). SMPD3-ALK: A novel alk fusion gene in lung adenocarcinoma. Clin. Genet. 99 (3), 488–489. doi:10.1111/cge.13891
Liu, H., Pathak, P., Boehme, S., and Chiang, J. L. (2016). Cholesterol 7α-hydroxylase protects the liver from inflammation and fibrosis by maintaining cholesterol homeostasis. J. Lipid Res. 57 (10), 1831–1844. doi:10.1194/jlr.M069807
Liu, H-M., Liao, J-F., and Lee, T-Y. (2017). Farnesoid X receptor agonist GW4064 ameliorates lipopolysaccharide-induced ileocolitis through TLR4/MyD88 pathway related mitochondrial dysfunction in mice. Biochem. Biophys. Res. Commun. 490 (3), 841–848. doi:10.1016/j.bbrc.2017.06.129
Liu, T-C., Kern, J. T., Jain, U., Sonnek, N. M., Xiong, S., Simpson, K. F., et al. (2021). Western diet induces Paneth cell defects through microbiome alterations and farnesoid X receptor and type I interferon activation. Cell Host Microbe 29 (6), 988–1001.e6. doi:10.1016/j.chom.2021.04.004
Lu, Q., Wu, X., Han, W., Zhang, W., Wang, Y., Kong, D., et al. (2021). Effect of Glycyrrhiza uralensis against ulcerative colitis through regulating the signaling pathway of FXR/P-gp. Am. J. Transl. Res. 13 (8), 9296–9305.
Luo, W., Chen, J., Li, L., Ren, X., Cheng, T., Lu, S., et al. (2019). c-Myc inhibits myoblast differentiation and promotes myoblast proliferation and muscle fibre hypertrophy by regulating the expression of its target genes, miRNAs and lincRNAs. Cell Death Differ. 26 (3), 426–442. doi:10.1038/s41418-018-0129-0
Ma, C., Xiang, J., Huang, G., Zhao, Y., Wang, X., Wu, H., et al. (2021). Pterostilbene alleviates cholestasis by promoting SIRT1 activity in hepatocytes and macrophages. Front. Pharmacol. 12, 785403. doi:10.3389/fphar.2021.785403
Makishima, M. (2005). Nuclear receptors as targets for drug development: Regulation of cholesterol and bile acid metabolism by nuclear receptors. J. Pharmacol. Sci. 97 (2), 177–183. doi:10.1254/jphs.fmj04008x4
Manley, S., and Ding, W. (2015). Role of farnesoid X receptor and bile acids in alcoholic liver disease. Acta Pharm. Sin. B 5 (2), 158–167. doi:10.1016/j.apsb.2014.12.011
Massafra, V., Ijssennagger, N., Plantinga, M., Milona, A., Ramos Pittol, J. M., Boes, M., et al. (2016). Splenic dendritic cell involvement in FXR-mediated amelioration of DSS colitis. Biochim. Biophys. Acta 1862 (2), 166–173. doi:10.1016/j.bbadis.2015.11.001
McMahan, R. H., Wang, X. X., Cheng, L. L., Krisko, T., Smith, M., El Kasmi, K., et al. (2013). Bile acid receptor activation modulates hepatic monocyte activity and improves nonalcoholic fatty liver disease. J. Biol. Chem. 288 (17), 11761–11770. doi:10.1074/jbc.M112.446575
Meadows, V., Kennedy, L., Ekser, B., Kyritsi, K., Kundu, D., Zhou, T., et al. (2021). Mast cells regulate ductular reaction and intestinal inflammation in cholestasis through farnesoid X receptor signaling. Hepatol. Baltim. Md) 74 (5), 2684–2698. doi:10.1002/hep.32028
Mencarelli, A., Renga, B., Migliorati, M., Cipriani, S., Distrutti, E., Santucci, L., et al. (2009). The bile acid sensor farnesoid X receptor is a modulator of liver immunity in a rodent model of acute hepatitis. J. Immunol. 183 (10), 6657–6666. doi:10.4049/jimmunol.0901347
Miyazaki, T., Shirakami, Y., Mizutani, T., Maruta, A., Ideta, T., Kubota, M., et al. (2021). Novel FXR agonist nelumal A suppresses colitis and inflammation-related colorectal carcinogenesis. Sci. Rep. 11 (1), 492. doi:10.1038/s41598-020-79916-5
Miyazaki-Anzai, S., Masuda, M., Levi, M., Keenan, A. L., and Miyazaki, M. (2014). Dual activation of the bile acid nuclear receptor FXR and G-protein-coupled receptor TGR5 protects mice against atherosclerosis. PloS One 9 (9), e108270. doi:10.1371/journal.pone.0108270
Modica, S., Petruzzelli, M., Bellafante, E., Murzilli, S., Salvatore, L., Celli, N., et al. (2012). Selective activation of nuclear bile acid receptor FXR in the intestine protects mice against cholestasis. Gastroenterology 142 (2), 355–365. doi:10.1053/j.gastro.2011.10.028
Mroz, M. S., Lajczak, N. K., Goggins, B. J., Keely, S., and Keely, S. J. (2018). The bile acids, deoxycholic acid and ursodeoxycholic acid, regulate colonic epithelial wound healing. Am. J. Physiol. Gastrointest. Liver Physiol. 314 (3), G378–G87. doi:10.1152/ajpgi.00435.2016
Mukai, K., Tsai, M., Saito, H., and Galli, S. J. (2018). Mast cells as sources of cytokines, chemokines, and growth factors. Immunol. Rev. 282 (1), 121–150. doi:10.1111/imr.12634
Nadeem, M. S., Kumar, V., Al-Abbasi, F. A., Kamal, M. A., and Anwar, F. (2020). Risk of colorectal cancer in inflammatory bowel diseases. Semin. Cancer Biol. 64, 51–60. doi:10.1016/j.semcancer.2019.05.001
Negroni, A., Fiaschini, N., Palone, F., Vitali, R., Colantoni, E., Laudadio, I., et al. (2020). Intestinal inflammation alters the expression of hepatic bile acid receptors causing liver impairment. J. Pediatr. Gastroenterol. Nutr. 71 (2), 189–196. doi:10.1097/MPG.0000000000002759
Nie, Y-F., Hu, J., and Yan, X-H. (2015). Cross-talk between bile acids and intestinal microbiota in host metabolism and health. J. Zhejiang Univ. Sci. B 16 (6), 436–446. doi:10.1631/jzus.B1400327
Niesor, E. J., Flach, J., Lopes-Antoni, I., Perez, A., and Bentzen, C. L. (2001). The nuclear receptors FXR and LXRalpha: Potential targets for the development of drugs affecting lipid metabolism and neoplastic diseases. Curr. Pharm. Des. 7 (4), 231–259. doi:10.2174/1381612013398185
Ocansey, D. K. W., Zhang, Z., Xu, X., Liu, L., Amoah, S., Chen, X., et al. (2022). Mesenchymal stem cell-derived exosome mitigates colitis the modulation of the gut metagenomics-metabolomics-farnesoid X receptor axis. Biomater. Sci. 1, 1.
Ocvirk, S., and O'Keefe, S. J. D. (2021). Dietary fat, bile acid metabolism and colorectal cancer. Semin. Cancer Biol. 73, 347–355. doi:10.1016/j.semcancer.2020.10.003
Ocvirk, S., Wilson, A. S., Posma, J. M., Li, J. V., Koller, K. R., Day, G. M., et al. (2020). A prospective cohort analysis of gut microbial co-metabolism in Alaska Native and rural African people at high and low risk of colorectal cancer. Am. J. Clin. Nutr. 111 (2), 406–419. doi:10.1093/ajcn/nqz301
Panzitt, K., and Wagner, M. (2021). FXR in liver physiology: Multiple faces to regulate liver metabolism. Biochim. Biophys. Acta. Mol. Basis Dis. 1867 (7), 166133. doi:10.1016/j.bbadis.2021.166133
Pavlidis, P., Powell, N., Vincent, R. P., Ehrlich, D., Bjarnason, I., and Hayee, B. (2015). Systematic review: Bile acids and intestinal inflammation-luminal aggressors or regulators of mucosal defence? Aliment. Pharmacol. Ther. 42 (7), 802–817. doi:10.1111/apt.13333
Pellicciari, R., Fiorucci, S., Camaioni, E., Clerici, C., Costantino, G., Maloney, P. R., et al. (2002). 6alpha-ethyl-chenodeoxycholic acid (6-ECDCA), a potent and selective FXR agonist endowed with anticholestatic activity. J. Med. Chem. 45 (17), 3569–3572. doi:10.1021/jm025529g
Qiao, P., Li, S., Zhang, H., Yao, L., and Wang, F. (2018). Farnesoid X receptor inhibits proliferation of human colorectal cancer cells via the miR135A1/CCNG2 signaling pathway. Oncol. Rep. 40 (4), 2067–2078. doi:10.3892/or.2018.6636
Ramos Pittol, J. M., Milona, A., Morris, I., Willemsen, E. C. L., van der Veen, S. W., Kalkhoven, E., et al. (2020). FXR isoforms control different metabolic functions in liver cells via binding to specific DNA motifs. Gastroenterology 159 (5), 1853–1865. doi:10.1053/j.gastro.2020.07.036
Renga, B., Francisci, D., D'Amore, C., Schiaroli, E., Carino, A., Baldelli, F., et al. (2012). HIV-1 infection is associated with changes in nuclear receptor transcriptome, pro-inflammatory and lipid profile of monocytes. BMC Infect. Dis. 12, 274. doi:10.1186/1471-2334-12-274
Roth, J. D., Feigh, M., Veidal, S. S., Fensholdt, L. K., Rigbolt, K. T., Hansen, H. H., et al. (2018). INT-767 improves histopathological features in a diet-induced ob/ob mouse model of biopsy-confirmed non-alcoholic steatohepatitis. World J. Gastroenterol. 24 (2), 195–210. doi:10.3748/wjg.v24.i2.195
Schmitt, J., Kong, B., Stieger, B., Tschopp, O., Schultze, S. M., Rau, M., et al. (2015). Protective effects of farnesoid X receptor (FXR) on hepatic lipid accumulation are mediated by hepatic FXR and independent of intestinal FGF15 signal. Liver Int. 35 (4), 1133–1144. doi:10.1111/liv.12456
Schoenborn, A. A., von Furstenberg, R. J., Valsaraj, S., Hussain, F. S., Stein, M., Shanahan, M. T., et al. (2019). The enteric microbiota regulates jejunal Paneth cell number and function without impacting intestinal stem cells. Gut Microbes 10 (1), 45–58. doi:10.1080/19490976.2018.1474321
Sepe, V., Distrutti, E., Fiorucci, S., and Zampella, A. (2018). Farnesoid X receptor modulators 2014-present: A patent review. Expert Opin. Ther. Pat. 28 (5), 351–364. doi:10.1080/13543776.2018.1459569
Shaik, F. B., Panati, K., Narasimha, V. R., and Narala, V. R. (2015). Chenodeoxycholic acid attenuates ovalbumin-induced airway inflammation in murine model of asthma by inhibiting the T(H)2 cytokines. Biochem. Biophys. Res. Commun. 463 (4), 600–605. doi:10.1016/j.bbrc.2015.05.104
Shi, Y., Su, W., Zhang, L., Shi, C., Zhou, J., Wang, P., et al. (2020). TGR5 regulates macrophage inflammation in nonalcoholic steatohepatitis by modulating NLRP3 inflammasome activation. Front. Immunol. 11, 609060. doi:10.3389/fimmu.2020.609060
Stojancevic, M., Stankov, K., and Mikov, M. (2012). The impact of farnesoid X receptor activation on intestinal permeability in inflammatory bowel disease. Can. J. Gastroenterol. 26 (9), 631–637. doi:10.1155/2012/538452
Sun, S., Zhao, B., Qi, M., Yao, Y., Xu, L., Ji, R., et al. (2020). TUDCA ameliorates liver injury via activation of SIRT1-FXR signaling in a rat hemorrhagic shock model. Shock 53 (2), 217–222. doi:10.1097/SHK.0000000000001351
Ticho, A. L., Malhotra, P., Dudeja, P. K., Gill, R. K., and Alrefai, W. A. (2019). Intestinal absorption of bile acids in health and disease. Compr. Physiol. 10 (1), 21–56. doi:10.1002/cphy.c190007
Trauner, M., Gulamhusein, A., Hameed, B., Caldwell, S., Shiffman, M. L., Landis, C., et al. (2019). The nonsteroidal farnesoid X receptor agonist cilofexor (GS-9674) improves markers of cholestasis and liver injury in patients with primary sclerosing cholangitis. Hepatol. Baltim. Md) 70 (3), 788–801. doi:10.1002/hep.30509
Vavassori, P., Mencarelli, A., Renga, B., Distrutti, E., and Fiorucci, S. (2009). The bile acid receptor FXR is a modulator of intestinal innate immunity. J. Immunol. 183 (10), 6251–6261. doi:10.4049/jimmunol.0803978
Verbeke, L., Farre, R., Verbinnen, B., Covens, K., Vanuytsel, T., Verhaegen, J., et al. (2015). The FXR agonist obeticholic acid prevents gut barrier dysfunction and bacterial translocation in cholestatic rats. Am. J. Pathol. 185 (2), 409–419. doi:10.1016/j.ajpath.2014.10.009
Vítek, L. (2015). Bile acid malabsorption in inflammatory bowel disease. Inflamm. Bowel Dis. 21 (2), 476–483. doi:10.1097/MIB.0000000000000193
Vu, T., and Datta, P. K. (2017). Regulation of EMT in colorectal cancer: A culprit in metastasis. Cancers (Basel) 9 (12), E171. doi:10.3390/cancers9120171
Wan, Y-J. Y., and Sheng, L. (2018). Regulation of bile acid receptor activity. Liver Res. 2 (4), 180–185. doi:10.1016/j.livres.2018.09.008
Wang, H., He, Q., Wang, G., Xu, X., and Hao, H. (2018). FXR modulators for enterohepatic and metabolic diseases. Expert Opin. Ther. Pat. 28 (11), 765–782. doi:10.1080/13543776.2018.1527906
Wang, Y. D., Chen, W. D., Moore, D. D., and Huang, W. (2008). Fxr: A metabolic regulator and cell protector. Cell Res. 18 (11), 1087–1095. doi:10.1038/cr.2008.289
Wang, Y. D., Chen, W. D., Wang, M., Yu, D., Forman, B. M., and Huang, W. (2008). Farnesoid X receptor antagonizes nuclear factor kappaB in hepatic inflammatory response. Hepatology 48 (5), 1632–1643. doi:10.1002/hep.22519
Wilson, A., Almousa, A., Teft, W. A., and Kim, R. B. (2020). Attenuation of bile acid-mediated FXR and PXR activation in patients with Crohn's disease. Sci. Rep. 10 (1), 1866. doi:10.1038/s41598-020-58644-w
Wu, Q., Sun, L., Hu, X., Wang, X., Xu, F., Chen, B., et al. (2021). Suppressing the intestinal farnesoid X receptor/sphingomyelin phosphodiesterase 3 axis decreases atherosclerosis. J. Clin. Invest. 131 (9), 142865. doi:10.1172/JCI142865
Xiao-Rong, L., Ning, M., Xi-Wang, L., Shi-Hong, L., Zhe, Q., Li-Xia, B., et al. (2021). Untargeted and targeted metabolomics reveal the underlying mechanism of aspirin eugenol ester ameliorating rat hyperlipidemia via inhibiting FXR to induce CYP7A1. Front. Pharmacol. 12, 733789. doi:10.3389/fphar.2021.733789
Xu, M., Shen, Y., Cen, M., Zhu, Y., Cheng, F., Tang, L., et al. (2021). Modulation of the gut microbiota-farnesoid X receptor Axis improves deoxycholic acid-induced intestinal inflammation in mice. J. Crohns Colitis 15 (7), 1197–1210. doi:10.1093/ecco-jcc/jjab003
Xu, X., Ocansey, D. K. W., Hang, S., Wang, B., Amoah, S., Yi, C., et al. (2022). The gut metagenomics and metabolomics signature in patients with inflammatory bowel disease. Gut Pathog. 14 (1), 26. doi:10.1186/s13099-022-00499-9
Yang, C-S., Kim, J-J., Kim, T. S., Lee, P. Y., Kim, S. Y., Lee, H-M., et al. (2015). Small heterodimer partner interacts with NLRP3 and negatively regulates activation of the NLRP3 inflammasome. Nat. Commun. 6, 6115. doi:10.1038/ncomms7115
Yao, J., Zhou, C-S., Ma, X., Fu, B-Q., Tao, L-S., Chen, M., et al. (2014). FXR agonist GW4064 alleviates endotoxin-induced hepatic inflammation by repressing macrophage activation. World J. Gastroenterol. 20 (39), 14430–14441. doi:10.3748/wjg.v20.i39.14430
Yu, J., Li, S., Guo, J., Xu, Z., Zheng, J., and Sun, X. (2020). Farnesoid X receptor antagonizes Wnt/β-catenin signaling in colorectal tumorigenesis. Cell Death Dis. 11 (8), 640. doi:10.1038/s41419-020-02819-w
Yu, J., Yang, K., Zheng, J., Zhao, W., and Sun, X. (2021). Synergistic tumor inhibition of colon cancer cells by nitazoxanide and obeticholic acid, a farnesoid X receptor ligand. Cancer Gene Ther. 28 (6), 590–601. doi:10.1038/s41417-020-00239-8
Yu, S., Balasubramanian, I., Laubitz, D., Tong, K., Bandyopadhyay, S., Lin, X., et al. (2020). Paneth cell-derived lysozyme defines the composition of mucolytic microbiota and the inflammatory tone of the intestine. Immunity 53 (2), 398–416. doi:10.1016/j.immuni.2020.07.010
Zahiri, H. R., Perrone, E. E., and Strauch, E. D. (2011). Bile salt supplementation acts via the farnesoid X receptor to alleviate lipopolysaccharide-induced intestinal injury. Surgery 150 (3), 480–489. doi:10.1016/j.surg.2011.07.008
Zhang, H., Liu, Y., Bian, Z., Huang, S., Han, X., You, Z., et al. (2014). The critical role of myeloid-derived suppressor cells and FXR activation in immune-mediated liver injury. J. Autoimmun. 53, 55–66. doi:10.1016/j.jaut.2014.02.010
Zhang, X., Zhang, Y., Wang, P., Zhang, S-Y., Dong, Y., Zeng, G., et al. (2019). Adipocyte hypoxia-inducible factor 2α suppresses atherosclerosis by promoting adipose ceramide catabolism. Cell Metab. 30 (5), 937–951. doi:10.1016/j.cmet.2019.09.016
Zhao, D., Cai, C., Chen, Q., Jin, S., Yang, B., and Li, N. (2020). High-fat diet promotes DSS-induced ulcerative colitis by downregulated FXR expression through the TGFB pathway. Biomed. Res. Int. 2020, 3516128. doi:10.1155/2020/3516128
Zhen, Y., and Zhang, H. (2019). NLRP3 inflammasome and inflammatory bowel disease. Front. Immunol. 10, 276. doi:10.3389/fimmu.2019.00276
Keywords: Farnesoid X Receptor, immune cells, post-translational modification, inflammatory bowel disease, colorectal cancer, therapy
Citation: Zhou M, Wang D, Li X, Cao Y, Yi C, Wiredu Ocansey DK, Zhou Y and Mao F (2022) Farnesoid-X receptor as a therapeutic target for inflammatory bowel disease and colorectal cancer. Front. Pharmacol. 13:1016836. doi: 10.3389/fphar.2022.1016836
Received: 12 August 2022; Accepted: 20 September 2022;
Published: 06 October 2022.
Edited by:
Stefano Fiorucci, University of Perugia, ItalyReviewed by:
Valentina Sepe, University of Naples Federico II, ItalyLili Ding, Shanghai University of Traditional Chinese Medicine, China
Copyright © 2022 Zhou, Wang, Li, Cao, Yi, Wiredu Ocansey, Zhou and Mao. This is an open-access article distributed under the terms of the Creative Commons Attribution License (CC BY). The use, distribution or reproduction in other forums is permitted, provided the original author(s) and the copyright owner(s) are credited and that the original publication in this journal is cited, in accordance with accepted academic practice. No use, distribution or reproduction is permitted which does not comply with these terms.
*Correspondence: Yuling Zhou, MTM5MDUxNDE0MjdAMTM5LmNvbQ==; Fei Mao, bWFvZmVpMjAwM0B1anMuZWR1LmNu
†These authors have contributed equally to this work