- 1Department of Integrated Chinese and Western Medicine, Zhongnan Hospital of Wuhan University, Wuhan University, Wuhan, China
- 2Department of Oncology, Zhongnan Hospital of Wuhan University, Wuhan University, Wuhan, China
- 3Department of Spinal Surgery, The First Affiliated Hospital of Guangzhou University of Chinese Medicine, Guangzhou, China
- 4Department of Oncology, Wuhan Hospital of Traditional Chinese Medicine, Wuhan, China
- 5Peking University Shenzhen Hospital Hua Wei Clinic, Shenzhen, China
Multiple myeloma (MM) is a hematological malignancy characterized by clonal expansion of plasma cells in bone marrow, leading to the overproduction of monoclonal immunoglobulins. The clinical manifestations resulting from monoclonal proteins and malignant cells include signs of end-organ damage, such as hypercalcemia, renal failure, anemia, and bone lesions. Despite improvement in the survival of MM patients with use of myeloma-targeted and immunomodulatory therapies, MM remains an incurable disease. Moreover, patients with relapsed or refractory MM show poor survival outcomes. In recent years, there has been a growing interest in the use of traditional Chinese medicinal materials (TCMMs) for management of a wide spectrum of diseases. The bioactive ingredients derived from TCMMs hold great potential for the development of anticancer drugs. Here we summarize the evidence of the pharmacological effects of the active components in TCMMs on MM, including curcumin, resveratrol, baicalein, berberine, bufalin, cinobufagin, gambogic acid, ginsenoside, icariin, daidzin, formononetin, polysaccharides extracts from Hedyotis difus, and scutellarein. Available evidence indicates that the anti-MM effects of these bioactive ingredients are mediated via regulation of proliferation, apoptosis, autophagy, cell cycle, osteogenic differentiation, and drug resistance. In the future, the underlying mechanisms of the anti-MM effects of these components should be further investigated. Large-scale and well-designed clinical trials are also required to validate the efficacy of these bioactive constituents for MM.
Introduction
Multiple myeloma (MM) is the second most commonly diagnosed hematologic malignancy, accounting for nearly 10% of all hematological malignancies (Kumar et al., 2017). Environmental and occupational exposure (Georgakopoulou et al., 2021), genetic factors, and epigenetic alterations (Dimopoulos et al., 2014), race (Marinac et al., 2020) have been implicated in the causation of MM(Rajkumar, 2020). The disease is characterized by progressive monoclonal proliferation in the bone marrow, which leads to the overproduction of nonfunctional intact immunoglobulins (also known as M protein or monoclonal protein) (González et al., 2007; Joshua et al., 2019) or immunoglobulin chains in 15–20% of patients (Figure 1) (Drayson et al., 2001). Accumulation of these immunoglobulins and interaction of the aberrant monoclonal plasma cells with other cells in the bone marrow results in various serious complications including hypercalcemia, renal failure, anemia and bone lesions which are collectively referred to as CRAB features (Rajkumar et al., 2014; Rajkumar, 2020). Pathologically, MM is preceded by a premalignant phase termed monoclonal gammopathy of undetermined significance (MGUS), which is typically characterized by lower concentration of M proteins than that in MM (Ninkovic and Quach, 2020). MGUS is often asymptomatic, with or without an identified intervening stage, referred to as smoldering multiple myeloma (SMM). Most MGUS cases are clinically stable with a 1% annual risk of progression to MM (Kyle et al., 2018). Currently, there is a paucity of drugs that are able to recondition MM. Some new drugs, such as proteasome inhibitors bortezomib and carfilzomib as well as the immunomodulatory drugs (thalidomide, lenalidomide, and pomalidomide) have helped improve the treatment landscape for MM patients. However, the majority of patients ultimately develop recurrence. Therefore, development of novel therapeutic approaches for MM is a key imperative.
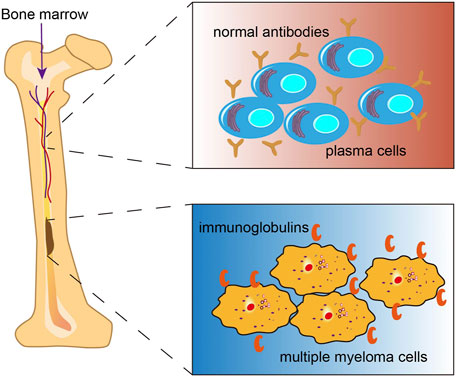
FIGURE 1. Multiple myeloma (MM). MM is a malignancy of plasma cells originating from the bone marrow, which produces excess monoclonal immunglobulins.
Natural products often constitute the basis for the identification of effective drugs. Many widely used drugs are of natural origin (Newman and Cragg, 2020). In other words, refinement, processing, and standardization of the active ingredients in natural products is still a common approach for the development of novel therapeutics. A recent study systematically reviewed anti-myeloma plant natural products including alkaloids, phenolics and terpenes. In addition, Traditional Chinese medicinal materials (TCMMs), as natural products, are also an attractive source of novel drugs for MM treatment. In recent years, a considerable body of scientific evidence has underlined the promising potential of bioactive ingredients derived from TCMMs for treatment of MM. To the best of our knowledge, there is no systematic summary of the anti-MM effects and clinical applications of these commonly utilized bioactive components extracted from TCMMs that are different from the natural products reviewed by Jöhrer et al.(Jöhrer and Ҫiҫek, 2021). The objective of this review was to systematically summarize the available evidence of the anti-MM effects of the bioactive components isolated from TCMMs and to identify future research priorities for the development of novel agents for MM.
Active Components Derived From TCMMS for Multiple Myeloma
Curcumin
Curcumin, a polyphenol extracted from Curcuma longa (also known as turmeric) is widely used in medicine and as a dietary constituent worldwide. It has gained increasing attention for its greater bioactivity compared to the other bioactive compounds isolated from turmeric. Curcumin belongs to a chemical class of polyphenols with a chemical formula of C21H20O6 (Figure 2A). Curcumin exhibits a wide spectrum of pharmacological effects including anti-microbial, anti-inflammatory, anti-oxidant, anti-cancer, anti-viral, and neuroprotective effects. The mechanisms of action of curcumin against various cancers and other disorders have been intensively reviewed, such as breast cancer (Hu et al., 2019), colorectal cancer (Pricci et al., 2020), lung cancer (Wan Mohd Tajuddin et al., 2019), cerebral ischemia (Subedi and Gaire, 2021), neurodegenerative diseases (Monroy et al., 2013), and diabetes (Pivari et al., 2019). An increasing body of evidence from clinical and experimental studies has shown the great potential of curcumin as anti-MM therapy. In a study by Golombick et al., oral curcumin treatment (at a dose of 4 g daily) for 3 months led to a decrease in paraprotein load and bone resorption in patients with MGUS. However, only patients with a paraprotein of >20 g/L responded to curcumin (Golombick et al..,2009). Subsequently, results from a randomized, double-blind placebo-controlled trial suggested that curcumin treatment (4 and 8 g daily) may potentially slow down the disease progression in patients with smoldering MM (Golombick et al., 2012). In a recent clinical trial, patients with MM who received adjuvant curcumin treatment (at a dose of 3–4 g daily), as a replacement of dexamethasone due to intolerance to dexamethasone, showed decreased paraprotein load and plasmacytosis by 38 and 59%, respectively, when administered in combination with other anti-myeloma therapies. These findings indicated that curcumin may slow disease progression without inducing the adverse effects associated with steroid use (Ramakrishna et al., 2020). Besides, curcumin was shown to inhibit STAT3 phosphorylation in U266 MM cells in a dose- and time-dependent manner. Curcumin-induced inhibition of STAT3 phosphorylation not only suppressed the growth of myeloma cells, but also sensitized MM cells to dexamethasone (Bharti et al., 2003). A curcumin analog, FLLL332, was also found to specifically inhibit STAT3 phosphorylation and DNA binding activity, which resulted in inhibition of downstream target genes involved in cell proliferation including cyclin D1, Bcl-2, survivin, thus inducing apoptosis in MM cells (Lin et al., 2010). Another water-soluble curcumin analog, curcumin #12, was found to increase the sensitivity of MM cells to proteasome inhibitor bortezomib, thus inducing a considerable increase in caspase activity (Mujtaba et al., 2012). Curcumin was also shown to reverse the resistance to melphalan chemotherapy in vitro possibly via inactivation of the Fanconi anemia/BRCA pathway (Xiao et al., 2010). Allegra et al. (2018) reported that curcumin inhibited NF-κB pathway, upregulated expression levels of p53 and p21 that were implicated in the regulation of apoptotic pathways and cell cycle in U266 cells. A combination of curcumin and carfilzomib exerted stronger proapoptotic effects than curcumin or carfilzomib alone. Consistently, in several studies, curcumin was found to ameliorate chemoresistance and to sensitize MM cells to bortezomib via inhibiting Notch1 pathway (Ge et al., 2019) and NF-κB pathway (Sung et al., 2009), leading to the downregulation of cyclin D1, survivin, Bcl-xL, cIAP-1, XIAP, Bcl-2, TRAF1, and VEGF, which are associated with proliferation, apoptosis, and angiogenesis (Sung et al., 2009; Zhang et al., 2011). Anti-angiogenesis effects of curcumin were also confirmed by Wang et al.(Wang et al., 2006). They observed that curcumin inhibited angiogenesis via interrupting the interaction between MM cells and endothelial cells by decreasing TrkB expression in endothelial cells and suppressing BDNF production in MM cells. In addition, curcumin-induced epigenetic regulation on MM has also been investigated. Chen et al. (2019) found that curcumin induced apoptosis and suppressed the proliferation of MM cells via hypermethylating the promoter of mTOR in CpG sites, which in turn lead to an upregulation of mTOR signaling involved in the regulation of apoptosis and autophagy in MM.
To summarize, the available evidence indicates multiple mechanisms of the anti-MM effects of curcumin, including induction of apoptosis, and inhibition of angiogenesis and proliferation (Figure 3).
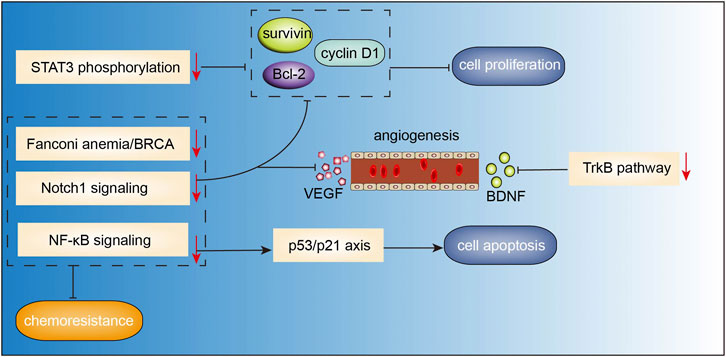
FIGURE 3. Action pathways involved in the anti-MM effects of curcumin.
Resveratrol
Resveratrol (3,4 ′,5-trihydroxy-trans-stilbene, Figure 2B) was first isolated from the roots of white hellebore in 1940s, and since then it has been extracted from a variety of other plant species. It was also abundantly found in the active ingredients isolated from several Chinese herbal medicines, such as Polygonum cuspidatum (Hu Zhang), Semen cassia (Jue Ming Zi), and Veratrum nigrum (Li Lu). Resveratrol has a broad spectrum of beneficial health effects including anti-aging, anti-oxidant, anti-inflammatory, neuroprotective, cardioprotective, anti-microbial, and immune-regulatory effects. Studies have shown that resveratrol may also exhibit significant anti-cancer activity against a wide range of solid tumors and hematological malignancies. Recently, the anti-MM effects of resveratrol have been gaining growing attention. In a study, resveratrol was found to suppress angiogenesis in RPMI 8226 cells by inhibiting the expressions of VEGF, bFGF, MMP-2, and MMP-9 (Hu et al., 2007), which was consistent with the results of a previous study (Sun et al., 2006b). In the study by Jin et al.(Jin et al., 2018), combination therapy with resveratrol and rapamycin exerted stronger effects in inhibiting proliferation and inducing apoptosis of MM cells, indicating that resveratrol may have an inhibitory effect on mTOR signaling. In another study, resveratrol was found to induce apoptosis of MM cells via mitochondrial apoptotic pathway and the recruitment of Fas/CD95 death receptor, and downstream signaling molecules into lipid rafts (Reis-Sobreiro et al., 2009). Wang et al.(Wang et al., 2011) demonstrated that resveratrol can induce endoplasmic reticulum stress response via activating IRE1α/XBP1 pathway, leading to a pro-apoptotic effects on MM cells. Additionally, it also suppressed pro-survival XBP1 signaling. Besides, inhibition of NEAT-1-mediated Wnt/β-catenin pathway (Geng et al., 2018), IL-6/STAT3 pathway (Bhardwaj et al., 2007), NF-κB signaling (Sun et al., 2006a; Bhardwaj et al., 2007), cyclin D1 and Bcl-xL (Sun et al., 2006a; Bhardwaj et al., 2007), ERK1/2 and JNK pathways (Xie et al., 2016) may also be involved in the resveratrol-induced suppression of cell proliferation and invasion, cell-cycle arrest, and apoptosis induction in MM. Other than the pro-apoptosis effects, resveratrol also showed pro-autophagic activities. Ma et al. (2021) found that resveratrol caused dose-dependent upregulation of the levels of LC3 and Beclin1 in several MM cell lines; further investigation demonstrated that resveratrol-induced autophagic flux was mediated by increasing the phosphorylation of AMPK at Thr172 site and decreasing the phosphorylation of mTOR (Ser2448), p70S6K(Thr389), and 4EBP1(Thr37/46). In addition, resveratrol not only sensitized the proteasome inhibitor carfilzomib-induced apoptosis via increasing the production of reactive oxygen species (ROS) in multiple kinds of MM cell lines, but also induced autophagy when administered in combination with low-dose carfilzomib, as evidenced by increased levels of LC3-II and p62/SQSTM1 (Li et al., 2018). Increased osteoclast formation and bone resorption and absence of bone formation are the serious consequences of MM (Terpos et al., 2018). In a study, resveratrol treatment was shown to promote osteogenic differentiation of bone marrow mesenchymal stem cells from patients with multiple myeloma via activating the SIRT1/RUNX2 pathway (Pan et al., 2021). Moreover, resveratrol inhibited osteoclastogenesis via receptor activator of nuclear factor-κB (NF-κB) ligand (RANKL)-induced osteoclast differentiation, which was associated with downregulation of RANK expression and decrease in NFATc1 stimulation and NF-κB nuclear translocation; these findings suggested that resveratrol may be an efficient osteoclast inhibitor in MM. Besides, resveratrol also induced the expressions of osteoblast markers osteocalcin and osteopontin in human bone marrow mesenchymal stem cells and sensitized their response to 1,25(OH)2 vitamin D3 (Boissy et al., 2005). Collectively, these studies demonstrate that the anti-tumor effects of resveratrol in MM cell lines include inhibition of cell proliferation, upregulation of apoptosis and autophagy, induction of oxidative stress, cell cycle arrest, and suppression of osteoclastogenesis (Figure 4). However, results from a phase-two study of resveratrol plus bortezomib for patients with relapsed and or refractory MM indicated minimal efficacy of resveratrol treatment; in addition, resveratrol caused severe adverse events such as nausea, vomiting, and even unexpected renal failure (Popat et al., 2013). Till date, there have been few clinical trials of resveratrol therapy in MM patients. Whether resveratrol is a potential therapeutic intervention for MM patients or not warrants further investigation.
Baicalein
Baicalein (4H-1-benzopyran-4-one,5,6,7-trihy-droxy-2- phenyl-C15H10O5, Figure 2C) is a major bioactive flavone derived from the roots of Scutellaria baicalensis georgi (Hunag Qin). Studies have demonstrated the anticancer effects of baicalein in the context of various cancers, including breast cancer (Yan et al., 2018), lung cancer (Zhang et al., 2020b), colorectal cancer (Phan et al., 2020), and hepatocellular carcinoma (Bie et al., 2017). Baicalein was shown to induce DNA damage without causing serious chromosomal instabilities or mutagenesis that may lead to severe side effects during chemotherapy (Fox et al., 2012). Recent studies have demonstrated the anti-MM effects of baicalein mediated via inhibition of proliferation and migration, and induction of apoptosis. In the study by Liu et al. (2018), baicalein suppressed the growth and promoted apoptosis of myeloma U266 cells via downregulating IKZF1 and IKZF3 (two essential lymphoid transcription factors in MM). Similarly, baicalein not only inhibited myeloma cell proliferation and induced apoptosis through downregulating IL-6 (Ma et al., 2005), but also abrogated IL-6-mediated signaling cascades including JAK, STAT3, MAPK, and Akt pathways associated with the proliferation and survival of MM cells (Liu et al., 2010). In addition, baicalein inhibited the proliferation and migration of RPMI 8226 and U266 MM cell lines via inhibition of Wnt/β-catenin pathway, c-myc, cyclin D1 and integrin β7, which are involved in cell proliferation (Xu et al., 2012). Otsuyama et al. (2007) found that combination of baicalein and dexamethasone can suppress the growth of U266 MM cells via activating PPARβ and glucocorticoid receptors, thus inhibiting the transcriptional activity of NF-κB followed by decreased levels of IL-6 and IKBα. Besides, baicalein can increase the sensitivity of MM cells to immunomodulatory drugs (IMiDs) by upregulating the CRBN which is also the target protein of IMiDs (Zhang et al., 2013). Side population cells show proliferation and differentiation potential similar to cancer stem cells. Gu et al. (2014) reported that baicalein decreased the proportion of side population cells via inhibition of ATP-binding cassette, subfamily G, isoform two protein (ABCG2) which is responsible for drug resistance in human MM cell line RPMI 8226. These findings suggest that baicalein may potentially target cancer stem cells of MM, indicating its potential use for the treatment of MM (Figure 5).
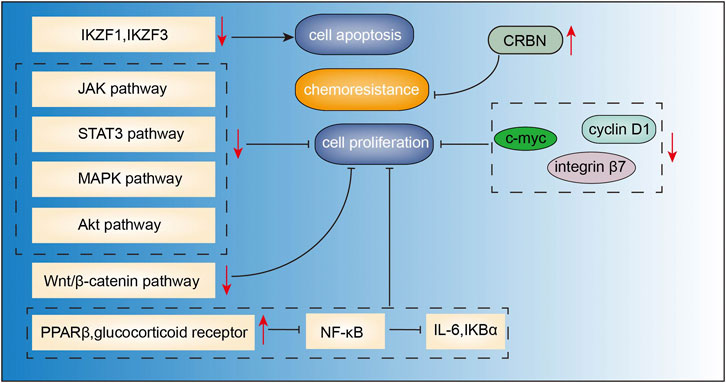
FIGURE 5. Action pathways involved in the anti-MM effects of baicalein.
Berberine
Berberine (Figure 2D) is the main active component of Optidis rhizome (Huang Lian). Several studies have demonstrated the anti-inflammatory, anti-oxidative, anti-microbial, anti-tumor, and neuroprotective effects of berberine. Recent experimental studies have also suggested the potential benefits of berberine in the treatment of MM (Figure 6). In the study by Tian et al., berberine administered in combination with bortezomib significantly increased the expressions of casepase-3, casepase-8, and casepase -9 in U266 cell line, and showed stronger pro-apoptotic effect than berberine or bortezomib alone (Tian et al., 2016). In a study by Qing et al.(Qing et al., 2014), berberine decreased the p53 DNA CpG methylation level via suppressing DNA methyltransferases DNMT1 and DNMT3B, and affected mRNA levels of several primary apoptosis-related proteins, thus inducing apoptosis and cell cycle arrest in U266 MM cells. Berberine can also suppress MM cells via possibly downregulating miRNA clusters including miR-99a∼125b, miR-17–92 and miR-106–25, which possibly affected MAPK, ErbB, and TP53 signaling pathways (Feng et al., 2015). Further studies validated that miR-106b/25 cluster encoding miR-106b, miR-93, and miR-25 (Gu et al., 2017), and miR-19a/92a cluster (Yin et al., 2018) were involved in berberine-induced inhibition of MM cells. Luo et al. (2014) found that berberine treatment suppressed MM cell growth via inhibiting IL6/STAT3/miR-21 pathway, which led to increased expression of PDCD4 and inhibition of p53 signaling. Similarly, berberine inhibited NF-κB translocation via Set9-mediated lysine methylation, resulting in a decrease in miR-21 level followed by decrease in Bcl-2 level, thereby triggering ROS generation and apoptosis in U266 MM cells (Hu et al., 2013). Lastly, Gu et al. (2020) also demonstrated that berberine treatment inhibited the growth of several MM cell lines via targeting UHRF1 (ubiquitin-like with PHD and RING Finger domains 1). Further molecular docking and surface plasmon resonance analysis confirmed UHRF1 as a berberine-binding protein and revealed that berberine binded UHRF1 in the tandem tudor domain and plant homeodomain.
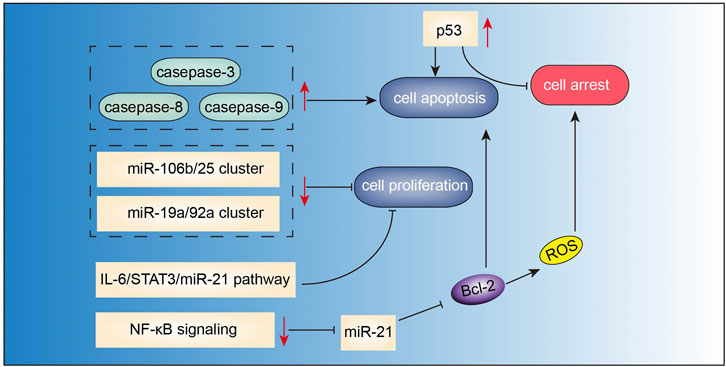
FIGURE 6. Action pathways involved in the anti-MM effects of berberine.
Chansu
Chansu, also known as toad venom, is an expensive TCMM extracted from the skin or parotid venom glands of Bufo gargarizans Cantor (Chen et al., 2018). Bufadienolides (including bufalin, cinobufagin, and resibufogenin) are considered as the main bioactive ingredients of Chansu. Among these, bufalin and cinobufagin are the main agents contributing to the antitumor effects (Zhang et al., 2020a). Studies have shown that Chansu can induce apoptosis and inhibit proliferation of MM cells. In the study by Wu et al. (2018), both bufalin and BF211 (a derivative of bufalin) were shown to suppress the progression of MM by inhibiting the IL-6/JAK2/STAT3 pathway in vivo and in vitro. Inhibition of IL-6/JAK2/STAT3 signaling pathway by BF211 led to increased levels of caspase-3, caspase-8, suppression of the expressions of Bcl-2 and Mcl-1, and showed stronger pro-apoptosis effects than bufalin. In addition, the cytocidal effect of bufalin in different MM cell lines was also mediated via inhibition of Akt/mTOR (Xiang et al., 2017). Results from Huang et al. (2013) revealed that the active site of bufalin interacted with the catalytic domain of ploy (ADP-ribose) polymerase1 (PARP1), triggering decreased activity of PARP1 and cell apoptosis as well as G2-M phase cell cycle arrest in various MM cell lines. While PARP1 overexpression reversed bufalin-induced cell apoptosis. Besides, cinobufagin was shown to exhibit pro-apoptotic effects in U266 cells through activating ERK, JNK and p38MAPK pathways (Baek et al., 2015). It should be noted that Chansu has a complex chemical composition, and its bioactive ingredients are highly toxic and may cause serious adverse events such as cardiac dysarythmia, tissue ischemia, and hypoxia (Li et al., 2020). Whether Chansu is suitable for the treatment of MM warrants further investigations.
Gambogic Acid
Gambogic acid (Figure 2E) is the major active component of gamboge derived from the Garcinia hanburryi tree. In TCM, gamboge (Teng Huang) has been used for relieving trauma–induced swelling and pain for thousands of years. It has been reported to exhibit potent anticancer activity against certain solid tumors. Recent studies have indicated the therapeutic potential of gambogic acid for the treatment of MM (Figure 7). Yang et al. (2012) found that gambogic acid can induce apoptosis of RPMI-8226 cells via ROS accumulation followed by caspase-3 activation, PARP cleavage, and SIRT1 downregulation. Hypoxic conditions in the bone marrow microenvironment have been implicated in the progression of angiogenesis, and chemotherapeutic resistance in MM (Ikeda and Tagawa, 2021). This hypoxic condition may induce adaptive cellular responses mediated via hypoxia-inducible transcription factors (HIF) (Martin et al., 2011; Borsi et al., 2015). In the study by Wang et al. (2014), gambogic acid treatment suppressed MM progression and angiogenesis through inhibition of HIF1α/VEGF expression, which was associated with the inhibition of PI3K/Akt/mTOR pathway. Osteoporosis and lytic lesions are also common in patients with MM (Andrews et al., 2021). Stromal cell-derived factor 1α (SDF-1α)/CXC chemokine receptor 4 (CXCR4) signaling has been shown to be associated with osteoclastogenesis (Dong et al., 2016). Gambogic acid was shown to inhibit SDF-1α-induced chemotaxis of MM cells and downstream signaling of CXCR4. Further study demonstrated that the gambogic acid-induced inhibition of CXCR4 was caused by inhibiting the binding of NF-κB to the CXCR4 prompter, which in turn caused inhibition of IL-6 expression in MM cells. Moreover, gambogic acid also inhibited expression of IL-6 in macrophages, thus inhibiting osteoclastogenesis (Pandey et al., 2014). Besides, gambogic acid and bortezomib at non-toxic concentration loaded with nanoparticles exhibited significant inhibitory effects by inducing G2/M phase cell cycle arrest and apoptosis via increasing expression of proapoptotic Bax, Caspase-3, and inhibiting anti-apoptotic PI3K/Akt pathway and Bcl-2 (Zhang et al., 2015).
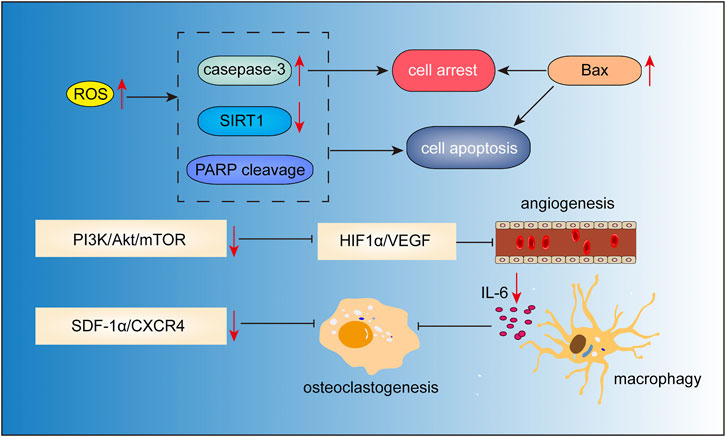
FIGURE 7. Action pathways involved in the anti-MM effects of gambogic acid.
Ginsenoside
Ginseng (Ren Shen) is a famous traditional Chinese medicine herb used in China for more than two thousand years. The medicinal value of Ginseng has been recognized globally. Rg3, one of the pharmacologically active ginsenoside saponins isolated from ginseng has shown anti-aging, anti-inflammatory, anti-tumor, cardioprotective and neuroprotective properties. Ginsenoside Rg3 has been studied for the different efficacy of two chemical forms, namely 20(R)-ginsenoside Rg3 (Figure 2F) and 20(S)-ginsenoside Rg3 (Figure 2G). Recent studies have shown the therapeutic potential of ginsenoside Rg3 in MM. Song et al.(Song et al., 2014) reported that 20(S)-ginsenoside Rg3 suppressed the proliferation of U266 cells via partly inducing G1 phase cell cycle arrest and apoptosis, as evidenced by increased levels of caspase-3, caspase-8, and caspase-9. In addition, the VEGF secretion by U266 cells was also downregulated. Li et al.(Li et al., 2016) demonstrated that the inhibitory effects of Rg3 on the proliferation of MM cells were associated with inhibition of the IGF-1/Akt/mTOR signaling pathway. Besides, ginseng, compound K, a metabolite of the ginsenoside was also shown to induce apoptosis in U266 cells via inhibiting the JAK1/STAT3 pathway (Park et al., 2011).
Other Bioactive Components
Other than the active ingredients discussed above, there are other bioactive components derived from TCMMs which have not been studied intensively, but could still provide new therapeutic options for MM patients. Icariin, a highly-potent active ingredient extracted from Epimedium (Ying Yang Huo), is a promising lead compound that has shown high efficiency in the treatment of various cancers. Jung et al.(Jung et al., 2018) recently reported that icariin significantly potentiated the apoptotic effects of bortezomib via inhibiting JAK/STAT3 signaling pathway, and downregulating the downstream targets including Bcl-2, Bcl-xl, survivin, IAP-1/2, COX-2, VEGF, and MMP-9. Additionally, daidzin, extracted from Pueraria lobate (Ge Gen) showed inhibitory effects on STAT3 signaling cascade in MM cells; this agent is frequently used to treat a broad spectrum of disorders including pain, diabetes, neurodegenerative diseases, gastrointestinal diseases, cerebrovascular disorders, and cardiac dysfunction (Wang et al., 2020). Formononetin, another naturally-occurring isoflavone derived from Pueraria lobata and Astragalus membranaceus (Huang Qi), can also suppress the DNA binding capacity and nuclear translocation of STAT3 and STAT5, thus triggering cell cycle arrest, inhibition of angiogenesis and proliferation, and induction of apoptosis in U266 and RPMI 8226 cells (Kim et al., 2018). Hedyotis diflfus (Bai Hua She She Cao) is a common ingredient in Chinese herbal medicine formulas used for cancer treatment. Lin et al.(Lin et al., 2013) found that polysaccharides extracts from Hedyotis difus significantly suppressed the proliferation and induced apoptosis in RPMI 8226 cells, at least, partly via downregulating Akt and NF-κB signaling cascades. Scutellarein is a flavonoid derived from Scutellaria barbata (Ban Zhi Lian), a famous and expensive anti-cancer traditional Chinese herbal medicine. It was shown to induce mitochondrial-mediated intrinsic apoptosis in a variety of MM cell lines, and to greatly reduce MM xenograft tumor burden in nude mice (Shi et al., 2019).
Summary, Conclusion, and Future Perspective
Currently, bioactive components derived from TCMMs have been gradually drawing attention, and their cytotoxic effects on MM have been intensively validated. Collectively, the available evidence supports that curcumin, resveratrol, baicalein, berberine, bufadienolides, gambogic acid, ginsenoside, and other less investigated ingredients exhibit significant anti-MM effects via mainly suppressing angiogenesis and proliferation, by inducing cell apoptosis, cell cycle arrest and autophagy, as well as by inhibiting osteoclastogenesis. These findings indicate a promising role of bioactive components extracted from TCMMs as novel therapeutic agents for MM. However, several issues still need to be considered. First, studies that have investigated the cytotoxic effects of these ingredients on MM cell lines have largely been limited to assessing the effects on cell proliferation, apoptosis, or autophagy, while there is a paucity of investigations on the immune system or multiple myeloma bone marrow microenvironment. In addition, epigenetic modifications induced by these ingredients should also be studied since epigenetics modulation are risk factors including environmental and occupational exposures, as well as aging (Dimopoulos et al., 2014). Moreover, theses bioactive components may have a cytotoxic effect on normal cells, while most studies have been performed on MM cells. Whether and how these ingredients discriminate between normal and MM cells remains unknown. Secondly, the targeting proteins, metabolites, and signaling pathways involved in the regulation of angiogenesis, proliferation, apoptosis should be studied by utilizing multi-omics technologies, including genomics, transcriptomics, proteomics, and metabolomics. This approach may provide more in-depth characterization of the mechanisms of actions of these bioactive components. Besides, network pharmacology and molecular docking analysis on molecular targets should also be considered to shed light on the underlying mechanisms of TCMMs. Additionally, the anti-MM impact of promising ingredients, such as curcumin and resveratrol, in the clinical context is still quite vague. Few clinical studies have evaluated treatment with these ingredients alone or in combination with other drugs in MM patients. Thus, further well-designed clinical trials with follow-up and in-vivo examinations should be performed. Third, many of these ingredients exhibit poor bioavailability and are rapidly metabolized, which may undermine their pharmacological activity. Therefore, discovery and utilization of drug delivery systems, such as nanoparticles and liposomes, as well as the novel synthetic analogs can enhance drug stability, prolong drug action, and enable a sustained and slow-release rate while reducing the side effects.
Author Contributions
C-CY and YL contributed equally to this work. C-CY and YL conceived the main ideas and wrote this paper. Z-JC, XW, and WM helped design the framework and illustrate figures. Y-WZ helped revise the manuscript. All authors contributed to the article and approved the submitted version.
FUNDING
This work was supported by the Key Research & Development Project funded by the Department of Science and Technology of Hubei of China (No. 2020BCB006); the National Famous and Experienced Chinese Medicine Experts’ Academic Inheritance Project funded by State Administration of Traditional Chinese Medicine (No. 29,2017); Traditional Chinese Medicine Research Project of Hubei Provincial Health Commission (No. ZY2021Q016).
Conflict of Interest
The authors declare that the research was conducted in the absence of any commercial or financial relationships that could be construed as a potential conflict of interest.
Publisher’s Note
All claims expressed in this article are solely those of the authors and do not necessarily represent those of their affiliated organizations, or those of the publisher, the editors and the reviewers. Any product that may be evaluated in this article, or claim that may be made by its manufacturer, is not guaranteed or endorsed by the publisher.
References
Allegra, A., Speciale, A., Molonia, M. S., Guglielmo, L., Musolino, C., Ferlazzo, G., et al. (2018). Curcumin Ameliorates the In Vitro Efficacy of Carfilzomib in Human Multiple Myeloma U266 Cells Targeting P53 and NF-Κb Pathways. Toxicol. Vitro 47, 186–194. doi:10.1016/j.tiv.2017.12.001
Andrews, R. E., Brown, J. E., Lawson, M. A., and Chantry, A. D. (2021). Myeloma Bone Disease: The Osteoblast in the Spotlight. J. Clin. Med. 10 (17). doi:10.3390/jcm10173973
Baek, S. H., Kim, C., Lee, J. H., Nam, D., Lee, J., Lee, S. G., et al. (2015). Cinobufagin Exerts Anti-proliferative and Pro-apoptotic Effects through the Modulation ROS-Mediated MAPKs Signaling Pathway. Immunopharmacol Immunotoxicol 37 (3), 265–273. doi:10.3109/08923973.2015.1027916
Bhardwaj, A., Sethi, G., Vadhan-Raj, S., Bueso-Ramos, C., Takada, Y., Gaur, U., et al. (2007). Resveratrol Inhibits Proliferation, Induces Apoptosis, and Overcomes Chemoresistance through Down-Regulation of STAT3 and Nuclear Factor-kappaB-Regulated Antiapoptotic and Cell Survival Gene Products in Human Multiple Myeloma Cells. Blood 109 (6), 2293–2302. doi:10.1182/blood-2006-02-003988
Bharti, A. C., Donato, N., and Aggarwal, B. B. (2003). Curcumin (Diferuloylmethane) Inhibits Constitutive and IL-6-inducible STAT3 Phosphorylation in Human Multiple Myeloma Cells. J. Immunol. 171 (7), 3863–3871. doi:10.4049/jimmunol.171.7.3863
Bie, B., Sun, J., Guo, Y., Li, J., Jiang, W., Yang, J., et al. (2017). Baicalein: A Review of its Anti-cancer Effects and Mechanisms in Hepatocellular Carcinoma. Biomed. Pharmacother. 93, 1285–1291. doi:10.1016/j.biopha.2017.07.068
Boissy, P., Andersen, T. L., Abdallah, B. M., Kassem, M., Plesner, T., and Delaissé, J. M. (2005). Resveratrol Inhibits Myeloma Cell Growth, Prevents Osteoclast Formation, and Promotes Osteoblast Differentiation. Cancer Res. 65 (21), 9943–9952. doi:10.1158/0008-5472.can-05-0651
Borsi, E., Terragna, C., Brioli, A., Tacchetti, P., Martello, M., and Cavo, M. (2015). Therapeutic Targeting of Hypoxia and Hypoxia-Inducible Factor 1 Alpha in Multiple Myeloma. Transl Res. 165 (6), 641–650. doi:10.1016/j.trsl.2014.12.001
Chen, J., Ying, Y., Zhu, H., Zhu, T., Qu, C., Jiang, J., et al. (2019). Curcumin-induced Promoter Hypermethylation of the Mammalian Target of Rapamycin Gene in Multiple Myeloma Cells. Oncol. Lett. 17 (1), 1108–1114. doi:10.3892/ol.2018.9662
Chen, Y. L., Bian, X. L., Guo, F. J., Wu, Y. C., and Li, Y. M. (2018). Two New 19-norbufadienolides with Cardiotonic Activity Isolated from the Venom of Bufo bufo Gargarizans. Fitoterapia 131, 215–220. doi:10.1016/j.fitote.2018.10.023
Dimopoulos, K., Gimsing, P., and Grønbæk, K. (2014). The Role of Epigenetics in the Biology of Multiple Myeloma. Blood Cancer J. 4 (5), e207. doi:10.1038/bcj.2014.29
Dong, Y., Liu, H., Zhang, X., Xu, F., Qin, L., Cheng, P., et al. (2016). Inhibition of SDF-1α/CXCR4 Signalling in Subchondral Bone Attenuates Post-Traumatic Osteoarthritis. Int. J. Mol. Sci. 17 (6). doi:10.3390/ijms17060943
Drayson, M., Tang, L. X., Drew, R., Mead, G. P., Carr-Smith, H., and Bradwell, A. R. (2001). Serum Free Light-Chain Measurements for Identifying and Monitoring Patients with Nonsecretory Multiple Myeloma. Blood 97 (9), 2900–2902. doi:10.1182/blood.v97.9.2900
Feng, M., Luo, X., Gu, C., Li, Y., Zhu, X., and Fei, J. (2015). Systematic Analysis of Berberine-Induced Signaling Pathway between miRNA Clusters and mRNAs and Identification of Mir-99a ∼ 125b Cluster Function by Seed-Targeting Inhibitors in Multiple Myeloma Cells. RNA Biol. 12 (1), 82–91. doi:10.1080/15476286.2015.1017219
Fox, J. T., Sakamuru, S., Huang, R., Teneva, N., Simmons, S. O., Xia, M., et al. (2012). High-throughput Genotoxicity Assay Identifies Antioxidants as Inducers of DNA Damage Response and Cell Death. Proc. Natl. Acad. Sci. U S A. 109 (14), 5423–5428. doi:10.1073/pnas.1114278109
Ge, X. P., Hong, Y. T., Li, Y. L., and Li, B. Z. (2019). [Curcumin Increases the Chemosensitivity of Multiple Myeloma to Bortezomib by Inhibiting the Notch1 Signaling Pathway]. Zhongguo Shi Yan Xue Ye Xue Za Zhi 27 (2), 464–471. doi:10.19746/j.cnki.issn.1009-2137.2019.02.025
Geng, W., Guo, X., Zhang, L., Ma, Y., Wang, L., Liu, Z., et al. (2018). Resveratrol Inhibits Proliferation, Migration and Invasion of Multiple Myeloma Cells via NEAT1-Mediated Wnt/β-Catenin Signaling Pathway. Biomed. Pharmacother. 107, 484–494. doi:10.1016/j.biopha.2018.08.003
Georgakopoulou, R., Fiste, O., Sergentanis, T. N., Andrikopoulou, A., Zagouri, F., Gavriatopoulou, M., et al. (2021). Occupational Exposure and Multiple Myeloma Risk: An Updated Review of Meta-Analyses. J. Clin. Med. 10 (18). doi:10.3390/jcm10184179
Golombick, T., Diamond, T. H., Badmaev, V., Manoharan, A., and Ramakrishna, R. (2009). The Potential Role of Curcumin in Patients with Monoclonal Gammopathy of Undefined Significance-Iits Effect on Paraproteinemia and the Urinary N-Telopeptide of Type I Collagen Bone Turnover Marker. Clin. Cancer Res. 15 (18), 5917–5922. doi:10.1158/1078-0432.ccr-08-2217
Golombick, T., Diamond, T. H., Manoharan, A., Ramakrishna, R., González, D., van der Burg, M., et al. (20127). Monoclonal Gammopathy of Undetermined Significance, Smoldering Multiple Myeloma, and Curcumin: a Randomized, Double-Blind Placebo-Controlled Cross-Over 4g Study and an Open-Label 8g Extension studyImmunoglobulin Gene Rearrangements and the Pathogenesis of Multiple Myeloma. Am. J. Hematolblood 87110 (59), 4553112–4603121. doi:10.1002/ajh.2315910.1182/blood-2007-02-069625
Gu, C., Li, T., Yin, Z., Chen, S., Fei, J., Shen, J., et al. (2017). Integrative Analysis of Signaling Pathways and Diseases Associated with the miR-106b/25 Cluster and Their Function Study In berberine-Induced Multiple Myeloma Cellerine-Induced Multiple Myeloma Cells. Funct. Integr. Genomics 17 (2-3), 253–262. doi:10.1007/s10142-016-0519-7
Gu, C., Yin, Z., Nie, H., Liu, Y., Yang, J., Huang, G., et al. (2020). Identification of Berberine as a Novel Drug for the Treatment of Multiple Myeloma via Targeting UHRF1. BMC Biol. 18 (1), 33. doi:10.1186/s12915-020-00766-8
Gu, Y. Y., Liu, L. P., Qin, J., Zhang, M., Chen, Y., Wang, D., et al. (2014). Baicalein Decreases Side Population Proportion via Inhibition of ABCG2 in Multiple Myeloma Cell Line RPMI 8226 In Vitro. Fitoterapia 94, 21–28. doi:10.1016/j.fitote.2014.01.019
Hu, C., Li, M., Guo, T., Wang, S., Huang, W., Yang, K., et al. (2019). Anti-metastasis Activity of Curcumin against Breast Cancer via the Inhibition of Stem Cell-like Properties and EMT. Phytomedicine 58, 152740. doi:10.1016/j.phymed.2018.11.001
Hu, H. Y., Li, K. P., Wang, X. J., Liu, Y., Lu, Z. G., Dong, R. H., et al. (2013). Set9, NF-Κb, and microRNA-21 Mediate Berberine-Induced Apoptosis of Human Multiple Myeloma Cells. Acta Pharmacol. Sin 34 (1), 157–166. doi:10.1038/aps.2012.161
Hu, Y., Sun, C. Y., Huang, J., Hong, L., Zhang, L., and Chu, Z. B. (2007). Antimyeloma Effects of Resveratrol through Inhibition of Angiogenesis. Chin. Med. J. (Engl) 120 (19), 1672–1677. doi:10.1097/00029330-200710010-00006
Huang, H., Cao, Y., Wei, W., Liu, W., Lu, S. Y., Chen, Y. B., et al. (2013). Targeting Poly (ADP-Ribose) Polymerase Partially Contributes to Bufalin-Induced Cell Death in Multiple Myeloma Cells. PLoS One 8 (6), e66130. doi:10.1371/journal.pone.0066130
Ikeda, S., and Tagawa, H. (2021). Impact of Hypoxia on the Pathogenesis and Therapy Resistance in Multiple Myeloma. Cancer Sci. 112 (10), 3995–4004. doi:10.1111/cas.15087
Jin, H. G., Wu, G. Z., Wu, G. H., and Bao, Y. G. (2018). Combining the Mammalian Target of Rapamycin Inhibitor, Rapamycin, with Resveratrol Has a Synergistic Effect in Multiple Myeloma. Oncol. Lett. 15 (5), 6257–6264. doi:10.3892/ol.2018.8178
Jöhrer, K., and Ҫiҫek, S. S. (2021). Multiple Myeloma Inhibitory Activity of Plant Natural Products. Cancers 13 (11), 2678. doi:10.3390/cancers13112678
Joshua, D. E., Bryant, C., Dix, C., Gibson, J., and Ho, J. (2019). Biology and Therapy of Multiple Myeloma. Med. J. Aust. 210 (8), 375–380. doi:10.5694/mja2.50129
Jung, Y. Y., Lee, J. H., Nam, D., Narula, A. S., Namjoshi, O. A., Blough, B. E., et al. (2018). Anti-myeloma Effects of Icariin Are Mediated through the Attenuation of JAK/STAT3-Dependent Signaling Cascade. Front. Pharmacol. 9, 531. doi:10.3389/fphar.2018.00531
Kim, C., Lee, S. G., Yang, W. M., Arfuso, F., Um, J. Y., Kumar, A. P., et al. (2018). Formononetin-induced Oxidative Stress Abrogates the Activation of STAT3/5 Signaling axis and Suppresses the Tumor Growth in Multiple Myeloma Preclinical Model. Cancer Lett. 431, 123–141. doi:10.1016/j.canlet.2018.05.038
Kumar, S. K., Rajkumar, V., Kyle, R. A., van Duin, M., Sonneveld, P., Mateos, M. V., et al. (2017). Multiple Myeloma. Nat. Rev. Dis. Primers 3, 17046. doi:10.1038/nrdp.2017.46
Kyle, R. A., Larson, D. R., Therneau, T. M., Dispenzieri, A., Kumar, S., Cerhan, J. R., et al. (2018). Long-Term Follow-Up of Monoclonal Gammopathy of Undetermined Significance. N. Engl. J. Med. 378 (3), 241–249. doi:10.1056/NEJMoa1709974
Li, M., Wang, X. J., Zhao, Q., Wang, J. X., Xing, H. Y., Zhang, Y. Z., et al. (2020). Bufalin-induced Cardiotoxicity: New Findings into Mechanisms. Chin. J. Nat. Med. 18 (7), 550–560. doi:10.1016/s1875-5364(20)30065-0
Li, Q., Yue, Y., Chen, L., Xu, C., Wang, Y., Du, L., et al. (2018). Resveratrol Sensitizes Carfilzomib-Induced Apoptosis via Promoting Oxidative Stress in Multiple Myeloma Cells. Front. Pharmacol. 9, 334. doi:10.3389/fphar.2018.00334
Li, Y., Yang, T., Li, J., Hao, H. L., Wang, S. Y., Yang, J., et al. (2016). Inhibition of Multiple Myeloma Cell Proliferation by Ginsenoside Rg3 via Reduction in the Secretion of IGF-1. Mol. Med. Rep. 14 (3), 2222–2230. doi:10.3892/mmr.2016.5475
Lin, L., Deangelis, S., Foust, E., Fuchs, J., Li, C., Li, P. K., et al. (2010). A Novel Small Molecule Inhibits STAT3 Phosphorylation and DNA Binding Activity and Exhibits Potent Growth Suppressive Activity in Human Cancer Cells. Mol. Cancer 9, 217. doi:10.1186/1476-4598-9-217
Lin, S. Y., Shen, C. Y., Jiang, J. P., Wu, L. Q., Dai, T. Y., Qian, W. B., et al. (2013). [Apoptosis of Multiple Myeloid Cells Induced by Polysaccharides Extracts from Hedyotis Diffusa and its Mechanism]. Zhonghua Xue Ye Xue Za Zhi 34 (4), 337–340. doi:10.3760/cma.j.issn.0253-2727.2013.04.019
Liu, S., Ma, Z., Cai, H., Li, Q., Rong, W., and Kawano, M. (2010). Inhibitory Effect of Baicalein on IL-6-mediated Signaling Cascades in Human Myeloma Cells. Eur. J. Haematol. 84 (2), 137–144. doi:10.1111/j.1600-0609.2009.01365.x
Liu, X. P., He, L., Zhang, Q. P., Zeng, X. T., and Liu, S. Q. (2018). Baicalein Inhibits Proliferation of Myeloma U266 Cells by Downregulating IKZF1 and IKZF3. Med. Sci. Monit. 24, 2809–2817. doi:10.12659/msm.907058
Luo, X., Gu, J., Zhu, R., Feng, M., Zhu, X., Li, Y., et al. (2014). Integrative Analysis of Differential miRNA and Functional Study of miR-21 by Seed-Targeting Inhibition in Multiple Myeloma Cells in Response to Berberine. BMC Syst. Biol. 8, 82. doi:10.1186/1752-0509-8-82
Ma, R., Yu, D., Peng, Y., Yi, H., Wang, Y., Cheng, T., et al. (2021). Resveratrol Induces AMPK and mTOR Signaling Inhibition-Mediated Autophagy and Apoptosis in Multiple Myeloma Cells. Acta Biochim. Biophys. Sin (Shanghai) 53 (6), 775–783. doi:10.1093/abbs/gmab042
Ma, Z., Otsuyama, K., Liu, S., Abroun, S., Ishikawa, H., Tsuyama, N., et al. (2005). Baicalein, a Component of Scutellaria Radix from Huang-Lian-Jie-Du-Tang (HLJDT), Leads to Suppression of Proliferation and Induction of Apoptosis in Human Myeloma Cells. Blood 105 (8), 3312–3318. doi:10.1182/blood-2004-10-3915
Marinac, C. R., Ghobrial, I. M., Birmann, B. M., Soiffer, J., and Rebbeck, T. R. (2020). Dissecting Racial Disparities in Multiple Myeloma. Blood Cancer J. 10 (2), 19. doi:10.1038/s41408-020-0284-7
Martin, S. K., Diamond, P., Gronthos, S., Peet, D. J., and Zannettino, A. C. (2011). The Emerging Role of Hypoxia, HIF-1 and HIF-2 in Multiple Myeloma. Leukemia 25 (10), 1533–1542. doi:10.1038/leu.2011.122
Monroy, A., Lithgow, G. J., and Alavez, S. (2013). Curcumin and Neurodegenerative Diseases. Biofactors 39 (1), 122–132. doi:10.1002/biof.1063
Mujtaba, T., Kanwar, J., Wan, S. B., Chan, T. H., and Dou, Q. P. (2012). Sensitizing Human Multiple Myeloma Cells to the Proteasome Inhibitor Bortezomib by Novel Curcumin Analogs. Int. J. Mol. Med. 29 (1), 102–106. doi:10.3892/ijmm.2011.814
Newman, D. J., and Cragg, G. M. (2020). Natural Products as Sources of New Drugs over the Nearly Four Decades from 01/1981 to 09/2019. J. Nat. Prod. 83 (3), 770–803. doi:10.1021/acs.jnatprod.9b01285
Ninkovic, S., and Quach, H. (2020). Shaping the Treatment Paradigm Based on the Current Understanding of the Pathobiology of Multiple Myeloma: An Overview. Cancers (Basel) 12 (11), 3488. doi:10.3390/cancers12113488
Otsuyama, K. I., Ma, Z., Abroun, S., Amin, J., Shamsasenjan, K., Asaoku, H., et al. (2007). PPARbeta-mediated Growth Suppression of Baicalein and Dexamethasone in Human Myeloma Cells. Leukemia 21 (1), 187–190. doi:10.1038/sj.leu.2404462
Pan, J., Tao, D. H., Ren, L., Su, C. Y., Chen, Z. L., Jiang, H. F., et al. (2021). [Resveratrol Promotes Osteogenic Differentiation of Multiple Myeloma Derived Bone Marrow Mesenchymal Stem Cells via Upregulating SIRT1/RUNX2]. Zhongguo Sheng Wu Hua Xue Yu Fen Zi Sheng Wu Xue Tong Bao 37 (03), 354–362. doi:10.13865/j.cnki.cjbmb.2021.01.1530
Pandey, M. K., Kale, V. P., Song, C., Sung, S. S., Sharma, A. K., Talamo, G., et al. (2014). Gambogic Acid Inhibits Multiple Myeloma Mediated Osteoclastogenesis through Suppression of Chemokine Receptor CXCR4 Signaling Pathways. Exp. Hematol. 42 (10), 883–896. doi:10.1016/j.exphem.2014.07.261
Park, S., Lee, H. J., Jeong, S. J., Song, H. S., Kim, M., Lee, H. J., et al. (2011). Inhibition of JAK1/STAT3 Signaling Mediates Compound K-Induced Apoptosis in Human Multiple Myeloma U266 Cells. Food Chem. Toxicol. 49 (6), 1367–1372. doi:10.1016/j.fct.2011.03.021
Phan, T., Nguyen, V. H., A'Lincourt Salazar, M., Wong, P., Diamond, D. J., Yim, J. H., et al. (2020). Inhibition of Autophagy Amplifies Baicalein-Induced Apoptosis in Human Colorectal Cancer. Mol. Ther. Oncolytics 19, 1–7. doi:10.1016/j.omto.2020.08.016
Pivari, F., Mingione, A., Brasacchio, C., and Soldati, L. (2019). Curcumin and Type 2 Diabetes Mellitus: Prevention and Treatment. Nutrients 11 (8). doi:10.3390/nu11081837
Popat, R., Plesner, T., Davies, F., Cook, G., Cook, M., Elliott, P., et al. (2013). A Phase 2 Study of SRT501 (Resveratrol) with Bortezomib for Patients with Relapsed and or Refractory Multiple Myeloma. Br. J. Haematol. 160 (5), 714–717. doi:10.1111/bjh.12154
Pricci, M., Girardi, B., Giorgio, F., Losurdo, G., Ierardi, E., and Di Leo, A. (2020). Curcumin and Colorectal Cancer: From Basic to Clinical Evidences. Int. J. Mol. Sci. 21 (7). doi:10.3390/ijms21072364
Qing, Y., Hu, H., Liu, Y., Feng, T., Meng, W., Jiang, L., et al. (2014). Berberine Induces Apoptosis in Human Multiple Myeloma Cell Line U266 through Hypomethylation of P53 Promoter. Cell Biol Int 38 (5), 563–570. doi:10.1002/cbin.10206
Rajkumar, S. V., Dimopoulos, M. A., Palumbo, A., Blade, J., Merlini, G., Mateos, M. V., et al. (2014). International Myeloma Working Group Updated Criteria for the Diagnosis of Multiple Myeloma. Lancet Oncol. 15 (12), e538–48. doi:10.1016/s1470-2045(14)70442-5
Rajkumar, S. V. (2020). Multiple Myeloma: 2020 Update on Diagnosis, Risk-Stratification and Management. Am. J. Hematol. 95 (5), 548–567. doi:10.1002/ajh.25791
Ramakrishna, R., Diamond, T. H., Alexander, W., Manoharan, A., and Golombick, T. (2020). Use of Curcumin in Multiple Myeloma Patients Intolerant of Steroid Therapy. Clin. Case Rep. 8 (4), 739–744. doi:10.1002/ccr3.2735
Reis-Sobreiro, M., Gajate, C., and Mollinedo, F. (2009). Involvement of Mitochondria and Recruitment of Fas/CD95 Signaling in Lipid Rafts in Resveratrol-Mediated Antimyeloma and Antileukemia Actions. Oncogene 28 (36), 3221–3234. doi:10.1038/onc.2009.183
Shi, L., Wu, Y., Lv, D. L., and Feng, L. (2019). Scutellarein Selectively Targets Multiple Myeloma Cells by Increasing Mitochondrial Superoxide Production and Activating Intrinsic Apoptosis Pathway. Biomed. Pharmacother. 109, 2109–2118. doi:10.1016/j.biopha.2018.09.024
Song, Y., Hou, J., Kang, L., and Gao, S. (2014). [Effect of 20 (S)-ginsenoside Rg3 on the Proliferation Inhibition and Secretion of Vascular Endothelial Growth Factor of Multiple Myeloma Cell Line U266]. Zhonghua Xue Ye Xue Za Zhi 35 (6), 519–523. doi:10.3760/cma.j.issn.0253-2727.2014.06.010
Subedi, L., and Gaire, B. P. (2021). Neuroprotective Effects of Curcumin in Cerebral Ischemia: Cellular and Molecular Mechanisms. ACS Chem. Neurosci. 12 (14), 2562–2572. doi:10.1021/acschemneuro.1c00153
Sun, C., Hu, Y., Liu, X., Wu, T., Wang, Y., He, W., et al. (2006a). Resveratrol Downregulates the Constitutional Activation of Nuclear Factor-kappaB in Multiple Myeloma Cells, Leading to Suppression of Proliferation and Invasion, Arrest of Cell Cycle, and Induction of Apoptosis. Cancer Genet. Cytogenet. 165 (1), 9–19. doi:10.1016/j.cancergencyto.2005.06.016
Sun, C. Y., Hu, Y., Guo, T., Wang, H. F., Zhang, X. P., He, W. J., et al. (2006b). Resveratrol as a Novel Agent for Treatment of Multiple Myeloma with Matrix Metalloproteinase Inhibitory Activity. Acta Pharmacol. Sin 27 (11), 1447–1452. doi:10.1111/j.1745-7254.2006.00343.x
Sung, B., Kunnumakkara, A. B., Sethi, G., Anand, P., Guha, S., and Aggarwal, B. B. (2009). Curcumin Circumvents Chemoresistance In Vitro and Potentiates the Effect of Thalidomide and Bortezomib against Human Multiple Myeloma in Nude Mice Model. Mol. Cancer Ther. 8 (4), 959–970. doi:10.1158/1535-7163.mct-08-0905
Terpos, E., Ntanasis-Stathopoulos, I., Gavriatopoulou, M., and Dimopoulos, M. A. (2018). Pathogenesis of Bone Disease in Multiple Myeloma: from Bench to Bedside. Blood Cancer J. 8 (1), 7. doi:10.1038/s41408-017-0037-4
Tian, Y., Li, L. H., Yang, G. Z., and Chen, W. M. (2016). [Study of the Effects In Vitro of Berberine Combined with Bortezomib on Multiple Myeloma Cell Line U266 Cells]. Zhonghua Xue Ye Xue Za Zhi 37 (11), 976–981. doi:10.3760/cma.j.issn.0253-2727.2016.11.010
Wan Mohd Tajuddin, W. N. B., Lajis, N. H., Abas, F., Othman, I., and Naidu, R. (2019). Mechanistic Understanding of Curcumin's Therapeutic Effects in Lung Cancer. Nutrients 11 (12). doi:10.3390/nu11122989
Wang, F., Zhang, W., Guo, L., Bao, W., Jin, N., Liu, R., et al. (2014). Gambogic Acid Suppresses Hypoxia-Induced Hypoxia-Inducible Factor-1α/vascular Endothelial Growth Factor Expression via Inhibiting Phosphatidylinositol 3-kinase/Akt/mammalian Target Protein of Rapamycin Pathway in Multiple Myeloma Cells. Cancer Sci. 105 (8), 1063–1070. doi:10.1111/cas.12458
Wang, F. M., Galson, D. L., Roodman, G. D., and Ouyang, H. (2011). Resveratrol Triggers the Pro-apoptotic Endoplasmic Reticulum Stress Response and Represses Pro-survival XBP1 Signaling in Human Multiple Myeloma Cells. Exp. Hematol. 39 (10), 999–1006. doi:10.1016/j.exphem.2011.06.007
Wang, S., Zhang, S., Wang, S., Gao, P., and Dai, L. (2020). A Comprehensive Review on Pueraria: Insights on its Chemistry and Medicinal Value. Biomed. Pharmacother. 131, 110734. doi:10.1016/j.biopha.2020.110734
Wang, Y. D., Hu, Y., and Sun, C. Y. (2006). Inhibitory Effect of Curcumin on Angiogenesis Induced by Brain Derived Neurotrophic Factor from Multiple Myeloma Cells. Zhongguo Shi Yan Xue Ye Xue Za Zhi 14 (1), 70–74. doi:10.3969/j.issn.1009-2137.2006.01.016
Wu, X. Y., Tian, F., Su, M. H., Wu, M., Huang, Y., Hu, L. H., et al. (2018). BF211, a Derivative of Bufalin, Enhances the Cytocidal Effects in Multiple Myeloma Cells by Inhibiting the IL-6/JAK2/STAT3 Pathway. Int. Immunopharmacol 64, 24–32. doi:10.1016/j.intimp.2018.08.016
Xiang, R. F., Wang, Y., Zhang, N., Xu, W. B., Cao, Y., Tong, J., et al. (2017). MK2206 Enhances the Cytocidal Effects of Bufalin in Multiple Myeloma by Inhibiting the AKT/mTOR Pathway. Cell Death Dis 8 (5), e2776. doi:10.1038/cddis.2017.188
Xiao, H., Xiao, Q., Zhang, K., Zuo, X., and Shrestha, U. K. (2010). Reversal of Multidrug Resistance by Curcumin through FA/BRCA Pathway in Multiple Myeloma Cell Line MOLP-2/R. Ann. Hematol. 89 (4), 399–404. doi:10.1007/s00277-009-0831-6
Xie, B., Xu, Z., Hu, L., Chen, G., Wei, R., Yang, G., et al. (2016). Pterostilbene Inhibits Human Multiple Myeloma Cells via ERK1/2 and JNK Pathway In Vitro and In Vivo. Int. J. Mol. Sci. 17 (11), 1927. doi:10.3390/ijms17111927
Xu, C. P., Cai, H. L., He, L., Ma, Z., and Liu, S. Q. (2012). [Effect of Baicalein on Proliferation and Migration in Multiple Myeloma Cell Lines RPMI 8226 and U266 Cells]. Zhonghua Xue Ye Xue Za Zhi 33 (11), 938–943. doi:10.3760/cma.j.issn.0253-2727.2012.11.011
Yan, W., Ma, X., Zhao, X., and Zhang, S. (2018). Baicalein Induces Apoptosis and Autophagy of Breast Cancer Cells via Inhibiting PI3K/AKT Pathway In Vivo and Vitro. Drug Des. Devel Ther. 12, 3961–3972. doi:10.2147/dddt.s181939
Yang, L. J., Chen, Y., He, J., Yi, S., Wen, L., Zhao, S., et al. (2012). Effects of Gambogic Acid on the Activation of Caspase-3 and Downregulation of SIRT1 in RPMI-8226 Multiple Myeloma Cells via the Accumulation of ROS. Oncol. Lett. 3 (5), 1159–1165. doi:10.3892/ol.2012.634
Yin, Z., Yang, J., Ning, R., Liu, Y., Feng, M., Gu, C., et al. (2018). Signal Pathways, Diseases, and Functions Associated with the miR-19a/92a Cluster and the Use of Berberine to Modulate the Expression of This Cluster in Multiple Myeloma Cells. J. Biochem. Mol. Toxicol. 32 (6), e22057. doi:10.1002/jbt.22057
Zhang, J., Hong, Y., Xie, P., Chen, Y., Jiang, L., Yang, Z., et al. (2020a). Spatial Lipidomics Reveals Anticancer Mechanisms of Bufalin in Combination with Cinobufagin in Tumor-Bearing Mice. Front. Pharmacol. 11, 593815. doi:10.3389/fphar.2020.593815
Zhang, R. B., He, L., Huang, Z., Ma, Z., and Liu, S. Q. (2013). [Synergistic Effect and Mechanism of Baicalein in Combination with Lenalidomide-Induced Apoptosis of Myeloma Cells]. Zhonghua Xue Ye Xue Za Zhi 34 (6), 546–547. doi:10.3760/cma.j.issn.0253-2727.2013.06.021
Zhang, W., Qiao, L., Wang, X., Senthilkumar, R., Wang, F., and Chen, B. (2015). Inducing Cell Cycle Arrest and Apoptosis by Dimercaptosuccinic Acid Modified Fe3O4 Magnetic Nanoparticles Combined with Nontoxic Concentration of Bortezomib and Gambogic Acid in RPMI-8226 Cells. Int. J. Nanomedicine 10, 3275–3289. doi:10.2147/ijn.s80795
Zhang, X., Ruan, Q., Zhai, Y., Lu, D., Li, C., Fu, Y., et al. (2020b). Baicalein Inhibits Non-small-cell Lung Cancer Invasion and Metastasis by Reducing Ezrin Tension in Inflammation Microenvironment. Cancer Sci. 111 (10), 3802–3812. doi:10.1111/cas.14577
Keywords: multiple myeloma, traditional Chinese medicinal materials, bioactive components, pharmacological effects, review
Citation: Yu C-C, Li Y, Cheng Z-J, Wang X, Mao W and Zhang Y-W (2022) Active Components of Traditional Chinese Medicinal Material for Multiple Myeloma: Current Evidence and Future Directions. Front. Pharmacol. 13:818179. doi: 10.3389/fphar.2022.818179
Received: 19 November 2021; Accepted: 10 January 2022;
Published: 27 January 2022.
Edited by:
Mark Thomas Shaw Williams, Glasgow Caledonian University, United KingdomReviewed by:
Sonam Mittal, Medical College of Wisconsin, United StatesXiaoxiao Huang, Shenyang Pharmaceutical University, China
Copyright © 2022 Yu, Li, Cheng, Wang, Mao and Zhang. This is an open-access article distributed under the terms of the Creative Commons Attribution License (CC BY). The use, distribution or reproduction in other forums is permitted, provided the original author(s) and the copyright owner(s) are credited and that the original publication in this journal is cited, in accordance with accepted academic practice. No use, distribution or reproduction is permitted which does not comply with these terms.
*Correspondence: Ying-Wen Zhang, aGhhbzM4MzhAc2luYS5jb20=