- 1Division of Infectious Diseases, Rocky Mountain Regional Veterans Affairs Medical Center, Aurora, CO, United States
- 2Division of Infectious Diseases, Department of Medicine, University of Colorado Anschutz Medical Campus, Aurora, CO, United States
- 3Hospital Infantil de México, Federico Gomez, Mexico City, Mexico
- 4Department of Internal Medicine, Yale University, Waterbury, CT, United States
Sepsis is infection sufficient to cause illness in the infected host, and more severe forms of sepsis can result in organ malfunction or death. Severe forms of Coronavirus disease-2019 (COVID-19), or disease following infection with severe acute respiratory syndrome coronavirus 2 (SARS-CoV-2) are examples of sepsis. Following infection, sepsis is thought to result from excessive inflammation generated in the infected host, also referred to as a cytokine storm. Sepsis can result in organ malfunction or death. Since COVID-19 is an example of sepsis, the hyperinflammation concept has influenced scientific investigation and treatment approaches to COVID-19. However, decades of laboratory study and more than 100 clinical trials designed to quell inflammation have failed to reduce sepsis mortality. We examine theoretical support underlying widespread belief that hyperinflammation or cytokine storm causes sepsis. Our analysis shows substantial weakness of the hyperinflammation approach to sepsis that includes conceptual confusion and failure to establish a cause-and-effect relationship between hyperinflammation and sepsis. We conclude that anti-inflammation approaches to sepsis therapy have little chance of future success. Therefore, anti-inflammation approaches to treat COVID-19 are likewise at high risk for failure. We find persistence of the cytokine storm concept in sepsis perplexing. Although treatment approaches based on the hyperinflammation concept of pathogenesis have failed, the concept has shown remarkable resilience and appears to be unfalsifiable. An approach to understanding this resilience is to consider the hyperinflammation or cytokine storm concept an example of a scientific paradigm. Thomas Kuhn developed the idea that paradigms generate rules of investigation that both shape and restrict scientific progress. Intrinsic features of scientific paradigms include resistance to falsification in the face of contradictory data and inability of experimentation to generate alternatives to a failing paradigm. We call for rejection of the concept that hyperinflammation or cytokine storm causes sepsis. Using the hyperinflammation or cytokine storm paradigm to guide COVID-19 treatments is likewise unlikely to provide progress. Resources should be redirected to more promising avenues of investigation and treatment.
1 Introduction
Sepsis refers to disease caused by infection that can manifest a spectrum of illness ranging from influenza-like discomfort to organ malfunction or death. The standard of care to treat sepsis includes controlling the infectious source, rapid administration of antimicrobial drugs, and supportive measures like volume infusion, oxygen and ventilatory support and use of vasopressors to augment blood pressure (Evans et al., 2021). However, despite advances in standard care, substantial mortality persists in sepsis with overall fatality of 25% and a 40–50% death rate for septic shock (Cavaillon et al., 2020). There is intense clinical interest in lowering this residual mortality. Investigation designed to accomplish this has focused on targeting the pathogen-triggered host inflammatory response to infection. By blocking inflammation as an adjunct therapy to antimicrobials and supportive care, it is hoped sepsis mortality can be lowered. Rationale for pursuing anti-inflammation adjunct therapies focuses on the notion that severe disease following infection is due to an overexuberant host inflammatory reaction. Since pro-inflammatory cytokines are thought to cause inflammation, infection-induced hyperinflammation is often referred to as a cytokine storm (Fajgenbaum and June 2020). According to the hyperinflammation concept, inflammation is a kind of two-edged sword (Chaudhry et al., 2013). A small or appropriate amount of inflammatory response following infection benefits the host by eliminating infection, whereas excessive inflammatory response to infection is detrimental and can result in organ malfunction or death. Interventions designed to suppress host inflammation as a sepsis treatment date to at least 1954 (Spink and Anderson, 1954; Vincent and Abraham, 2006), and a 2021 overview dates the cytokine storm concept back 116 years (Yongzhi, 2021). We believe the hyperinflammation concept of sepsis pathogenesis is mistaken. Recent world events call for urgent re-evaluation of the hyperinflammation concept due to substantial influence this approach has on clinical and research approaches to Coronavirus disease-2019 (COVID-19), the disease following infection with the severe acute respirology syndrome coronavirus 2 (SARS-CoV-2). GOOGLE entries for “inflammation causes COVID-19” reveals 75,500,000 entries and for PubMed this phrase yields 7,649 entries.
This theoretical report brings to light underappreciated problems with the hyperinflammation concept. According to this concept, pro-inflammatory cytokines are produced by host cells after recognizing microbial components and pro-inflammatory cytokines enter the circulation and spread inflammation systemically. Unregulated excessive production of these cytokines is assumed to cause sepsis as an undesired consequence of inflammation run amok. On close inspection, however, we uncover defects with this concept. Two defects we call attention to are conceptual confusion and failure to establish a cause-and-effect relationship between cytokine storm and sepsis. Conceptual confusion refers to a lack of precise understanding of concepts including inflammation, cytokine storm, and sepsis. These ideas are too vague to be used as optimal guides for research or treatment. Lack of clear understanding what these concepts refer to creates challenges in linking investigation and clinical study to sepsis. For example, no consensus defines which cytokines are pro-inflammatory and this complicates interpretation of studies associating specific cytokines with sepsis. These associations may represent true causes of sepsis, non-causal effects of sepsis, or unrelated chance associations with sepsis. A specific understanding of which cytokines are in fact pro-inflammatory would assist interpretation of studies associating specific cytokines with sepsis. Similarly, lack of adequate concepts of inflammation and cytokine storm complicate understanding of sepsis since sepsis pathogenesis depends on these background concepts. An overview of the concepts inflammation, cytokine storm, and sepsis indicates confusion that we believe weakens the relevance of research results to sepsis. We also assessed evidence supporting a cause-effect relationship between cytokine storm and sepsis. Not only is rationale supporting existence of this relationship weak, but available data suggest such a relationship cannot exist.
Finally, we are struck by resistance of the cytokine storm concept of sepsis to yield to falsifying clinical observation. There has been repeated and near exceptionless failure of studies designed to suppress hyperinflammation to treat sepsis. This record has generated little introspection about the viability of the hyperinflammation concept. Cytokine storm appears to be unfalsifiable. Substantial efforts to understand and treat COVID-19 as a manifestation of cytokine storm underscores continued dominance of this concept and establishes it is alive and flourishing in 2022. We believe the remarkable resilience of cytokine storm needs explanation, and we attempted to understand reasons for persistence. The strategy we chose was to consider the hyperinflammation concept of sepsis as a scientific paradigm as described by Kuhn (1962). This strategy not only explains persistence of the cytokine storm concept, but also suggests reasons the concept will likely continue without abandonment in the future. Finally, we propose a way forward that has potential to solve conceptual and practical problems with sepsis.
2 Conceptual Confusion
We believe the concept that hyperinflammation is the cause of sepsis is the dominant account of this disease. We therefore assessed pivotal notions associated with this account.
2.1 Inflammation
This most central concept in the current approach to sepsis pathogenesis has no established definition and disarray in the sepsis literature has ensued. Discussions of inflammation still emphasize the bedside descriptive characteristics of rubor (redness), tumor (swelling), calor (heat), and dolor (pain). This description is attributed to Celsus in the first century AD. In the 19th century Virchow added loss of function or functio laesa to this list. While much factual knowledge was accrued regarding clinical (bedside), histological, and molecular components of the inflammatory response, there remains no established unifying concept relating these components. No concise definition of inflammation has been established (Kushner, 1998; Groopman, 2015; Antonelli and Kushner, 2017). In addition to uncovering what regularities lie at the heart of inflammation, there is need to unify notions of local and systemic inflammation so they can be understood as manifestations of a single process. This point is crucial since the definition of sepsis is predicated on understanding systemic inflammation. Prior to the sepsis 3 definition of sepsis, a systemic inflammatory response syndrome (SIRS) caused by infection was defined as sepsis (Gul et al., 2017). Sepsis 3 characterizes sepsis as a dysregulated immune response to infection with organ malfunction. A dysregulation of immune response is unhelpful as a description of how the disease is caused, and there is no principled way to understand how this characterization differs from overactive systemic inflammation. Regardless, sepsis 3 focuses on infection-associated organ pathology with de-emphasis on underlying cause.
Confusion about inflammation has been noted and reviewed by Groopman (2015), in the FASEB Journal by Weissmann (2010) and later by Antonelli and Kushner (2017). There seems to be little agreement on what comprises inflammation other than association with innate immune antimicrobial activity, where innate immunity refers to host antipathogen function that does not require prior exposure to pathogen. Inflammation is thought to relate to innate immunity, and it is often implied some components of innate immunity are separate from inflammation. There is need for a definition of inflammation that captures its role in innate immunity, relates local and systemic inflammation, and unifies manifestations at the bedside, in histological specimens, and at the molecular level. A desirable quality of a novel understanding and definition would be a description that permits precise demarcation between inflammation and non-inflammatory phenomena like acquired immunity and autoimmunity. Our group is devising a novel definition of inflammation that we believe can accomplish many of these goals (manuscript in preparation).
2.2 Cytokine Storm
This is a nebulous term with an uncertain definition. Use of “cytokine storm” dates to at least 1993 in a description of graft vs host disease (Ferrara et al., 1993). A recent review summarized current thinking on this concept and includes discussion of the related cytokine release syndrome (Fajgenbaum and June 2020). Cytokine storm refers to overexuberant cytokine synthesis in response to a pathogen or other stimulus that causes adverse clinical manifestations that can include constitutional symptoms (including fever), organ malfunction, coagulopathy, epithelial barrier incompetence, or death (Fajgenbaum and June 2020). An explosion of cytokine research has uncovered over 100 genes coding cytokine-like activities, including at least 52 chemokines and 43 interleukins (Dinarello, 2007; Turner et al., 2014). Some cytokines are described as possessing inflammatory activities whereas others are described as possessing anti-inflammatory function. This has caused further confusion since there is no adequate definition of inflammation that permits a principled classification of cytokines as pro-inflammatory or anti-inflammatory. Recent technological advances have produced multiplex assay platforms that can determine numerous cytokines and other molecules in single biological (often blood) samples rapidly and at low cost. These technological achievements combined with absence of an applicable definition of inflammation has resulted in a chaotic literature. Identical molecules are sometimes described as pro-inflammatory or anti-inflammatory in different reports. A prototype example is interleukin (IL)-6, which is described as pro-inflammatory in reports of its role in COVID-19 (see below) despite reports showing IL-6 inhibits induction of the prototype pro-inflammatory cytokine tumor necrosis factor alpha (TNF) (Aderka et al., 1989; Schindler et al., 1990; Dinarello, 1991a). A sample of publications reporting circulating cytokine levels in COVID-19 shows measurement of circulating cytokines numbering 27 (Huang et al., 2020), 48 (Chi et al., 2020), 48 (Tjan et al., 2021), 35 (Mudd et al., 2020), and 53 (Herr et al., 2021). The GOOGLE entry “Cytokine levels in COVID-19” reveals 11, 400,000 entries and for PubMed 2,885 publications. Some studies identify molecules as cytokines that are not commonly considered cytokines like complement, tumor markers, ferritin, and CRP or procalcitonin. Perhaps no two reports describing cytokine levels in COVID-19 measure the same menu of cytokines, and no two reports disclose the same ensemble of significantly elevated cytokines. In the absence of an accepted definition of inflammation that precisely defines which cytokines are pro-inflammatory, it is understandable there is little agreement which cytokines are pro-inflammatory. Multiplex assays used to measure cytokines or other molecules in blood that show significant differences between COVID-19 patients and a comparator group (often healthy controls or COVID-19 patients with different severities) generates more opacity than clarity if used to uncover pathogenesis or devise therapeutic targets. Confusion associated with the concept of cytokine storm motivated us to devise a novel characterization of inflammation that includes structured criteria defining cytokines as pro-inflammatory or anti-inflammatory (manuscript in preparation).
2.3 Sepsis
Sepsis describes significant clinical disease following infection of any kind. The global significance of this disease is underscored by a report revealing 48.8 million sepsis cases and 11.0 million sepsis deaths that accounted for 18.7% of global deaths in 2017 (Rudd et al., 2020). In 2017 the World Health Organization designated sepsis a global health priority (Reinhart et al., 2017). The concept of pathogenesis that has dominated thinking and clinical study in sepsis is the role of hyperinflammation as cause (Dinarello, 1991b; Cross et al., 1993; Hotchkiss and Karl, 2003; Martin et al., 2003; Vincent and Abraham, 2006; Rittirsch et al., 2007; Marshall, 2014; Tsirigotis et al., 2016; Cavaillon et al., 2020; De Stefano et al., 2020; Jarczak et al., 2021). The focus on inflammation is clear when examining accepted criteria or definitions of sepsis (Mayr et al., 2014; Gul et al., 2017). The 1991 sepsis 1 definition formulated by the American College of Chest Physicians and Society of Critical Care Medicine (SCCM) defined sepsis as infection-associated systemic inflammatory response syndrome or SIRS. The SIRS criteria required 2 or more clinical findings from a list that included: fever or hypothermia, tachycardia, tachypnea or hypocapnia, and leukocytosis or leukopenia. The sepsis 1 definition suffered low specificity since non-infectious conditions could satisfy SIRS criteria, including post-surgery illness, drug effects, pancreatitis, trauma, burns, ischemia-reperfusion, and others. The 2001 sepsis 2 definition characterized sepsis as infection associated with expanded menus of general, inflammatory, hemodynamic, organ malfunction, and tissue perfusion anomalies. However, the diagnostic criteria were unaltered between sepsis 1 and sepsis 2. The sepsis 3 definition published 2016 (task force derived from the Society of Critical Care Medicine and the European Society of Intensive Care Medicine) eliminated SIRS criteria due to low sensitivity and specificity (Singer et al., 2016). Sepsis 3 focused on established or presumed infection and associated organ malfunction defined as increase in 2 or more points in the sequential organ failure assessment (SOFA) score. The sepsis 3 criteria marked a conceptual break with sepsis 1 and sepsis 2 definitions since evidence of underlying systemic inflammation was eliminated. Inadequate sensitivity and specificity motivated elimination of systemic inflammation criteria from sepsis 3. However, sepsis 3 broadly describes sepsis as a dysregulated immune response to infection. While we do not believe this phrase has a clear meaning, it suggests sepsis 3 retains reference to underlying excessive inflammation. This characterization permeates all three sepsis definitions. However, if sepsis is in fact not a disease caused by inflammation, attempts to link organ malfunction or death to inflammation will fail. We believe sepsis is not caused by any kind of hyperinflammation (Section 3). In retrospect, sepsis definitions have included paradoxical criteria including either elevated or reduced temperature and either increased or decreased white blood cell counts. Although inclusion of these peculiar criteria in sepsis 1 and sepsis 2 definitions were invoked to capture patients suffering disease due to infection-induced hyperinflammation, they call into question the conceptual underpinnings of sepsis.
2.4 Two-Edged Sword
This is an analogy invoked to describe the role of inflammation in host-pathogen interaction (Chaudhry et al., 2013). Properly regulated inflammation generated at appropriate levels and at the appropriate time benefits the host in eliminating pathogens. However, if inflammation is excessive or somehow dysregulated, host-derived inflammation becomes detrimental and can lead to organ malfunction or death. Conceptual difficulties are evident. If the inflammatory response is characterized as dysregulated (as in the sepsis 3 definition), little is gained other than asserting something has gone wrong. How a balance between pro-inflammatory and anti-inflammatory molecules relates to sepsis is undefined. A related defect in the two-edged sword analogy concerns quantification of inflammation mediators. The sword analogy clearly implies increased counterproductive inflammation exists in sepsis patients. However, no one has characterized which cytokines (or other molecules) participate as mediators of inflammation. Moreover, mediator concentrations that separate adaptive beneficial amounts from excessive detrimental levels are not specified in the literature. Poor characterization of identity and concentrations of cytokines that cause sepsis is a substantial conceptual defect. In response to this lacuna in the literature, our group has conducted a systematic review and meta-analysis that reports concentration of pro-inflammatory cytokines in the circulation of sepsis patients (manuscript in review) (Gharamti et al., 2021).
3 Cause Analysis; Hyperinflammation Does Not Cause Sepsis
The concept host inflammation causes organ damage or death following infection appears to date to two discoveries (Marshall, 2014). One was characterization of endogenous pyrogens or host-derived fever-inducing molecules that included the cytokine TNF (Dinarello, 1999). Since bedside observation associated fever with inflammation, characterization of endogenous pyrogen molecules suggested inflammation originated from host substances produced during infection. Attention focused on a host role in clinical manifestations of infection. The second discovery was characterization of special mice that resisted lethality in endotoxin models of sepsis (Sultzer, 1972; Lehmann et al., 1987). Injection of endotoxin surface components of gram-negative bacteria induced inflammation and physiological responses that resembled sepsis (Raetz and Whitfield, 2002; Rittirsch et al., 2007). Parenteral endotoxin administered at levels that killed normal mice showed no lethality in special endotoxin resistant mice. Therefore, mice that did not succumb to endotoxin infusion suggested host factors of some sort were interposed between endotoxin injection and sepsis mortality. Elegant experiments showed transfer of bone marrow cells from normal endotoxin-sensitive mice into special endotoxin-resistant mice converted resistant mice into endotoxin sensitive mice (Michalek et al., 1980). Therefore, some characteristic of white blood cells from special endotoxin resistant mice differed from normal endotoxin-sensitive mice. Since endotoxin injection was thought to mimic sepsis, it was believed components of host white blood cells were somehow mediating sepsis lethality. Here again, attention was called to a host role in infection. Moreover, characteristics of host white blood cells seemed to account for host lethality in the endotoxin sepsis model. It appears a role for microbial pathogens as direct causes of sepsis was concomitantly de-emphasized. A concept seemed to emerge that pathogens did not directly kill sepsis patients, but the response to pathogens caused sepsis patients to kill themselves. It was later discovered these special endotoxin unresponsive mice harbored a loss of function mutation in the pattern recognition receptor that identifies endotoxin (Qureshi et al., 1999). A link between the endotoxin sepsis model and white blood cell biology was demonstrated when specific blockade of TNF lowered mortality in an endotoxin mouse model of sepsis (Beutler et al., 1985). Since white blood cells are considered a primary source of TNF during sepsis, the combination of observations described above suggested infections supplied an endotoxin source that induced white blood cells to produce excessive TNF that in turn caused sepsis organ malfunction and mortality (Bradley, 2008). Importantly, it appeared blockade of the host cytokine TNF induced by bacterial endotoxin could lower mortality in sepsis.
TNF does not directly damage tissues, but TNF initiates biological activities that can culminate in tissue damage. Proposed mechanisms invoked to link TNF excess to toxicity in sepsis include polymorphonuclear neutrophil (PMN) chemotaxis directed into tissues (Smart and Casale, 1994; Vieira et al., 2009). Tumor necrosis factor can also activate PMNs. Activated PMNs can secrete reactive oxygen species, neutrophil elastase, and neutrophil extracellular traps, which may individually or in concert damage tissues (Bajaj et al., 1992; Ferrante, 1992; Keshari et al., 2012). Other mechanisms invoked to explain TNF-mediated tissue damage include activation of complement or coagulation cascades (van der Poll et al., 1990; Page et al., 2018; Gao et al., 2019). Sepsis-associated complement deposition on host cells can in principle cause cell damage, and coagulation activation may induce disseminated thromboses that may result in tissue ischemia.
3.1 Evidence That Inflammation Causes Sepsis
To evaluate the claim hyperinflammation or cytokine storm causes sepsis, we focus on the strength of evidence for TNF as cause for five reasons. First, we believe sound theoretical reasons support TNF as a pivotal cause of inflammation (manuscript in preparation). Tumor necrosis factor has been labeled the “master regulator” of inflammatory cytokine production (Parameswaran and Patial, 2010), and referred to as “the prime mediator of the inflammatory response seen in sepsis and septic shock” (Spooner et al., 1992). Second, TNF injection into animals results in altered physiology thought to mimic sepsis (Tracey et al., 1986; Bauss et al., 1987; Spooner et al., 1992), Third, neutralization of TNF activity has been widely studied as a therapy in animal sepsis models (Beutler et al., 1985; Tracey et al., 1986; Tracey et al., 1987; Mathison et al., 1988; Fong et al., 1989; Bajaj et al., 1992; Mohler et al., 1993; Mullen et al., 1994; Remick et al., 1995; Beutler et al., 2008; Bojalil et al., 2013). Fourth, the history of anti-inflammation strategies employed to treat human sepsis has emphasized specific inhibition of TNF as a sepsis treatment. (Table 1). Fifth, more studies are available that can be used to assess sepsis causality for TNF than for other molecules. These considerations support a focus on TNF as the prototype cytokine thought to cause the hyperinflammation of sepsis (Bradley, 2008). We believe three criteria can be used to conclude excessive inflammation causes sepsis. These include 1) showing pro-inflammatory cytokines are necessary for sepsis, 2) showing pro-inflammatory cytokines are sufficient for sepsis, and 3) showing pro-inflammatory cytokines satisfy an interventionist account of causality.
3.2 Necessary Condition
Evidence that TNF is necessary for sepsis amounts to showing TNF is elevated in sepsis patients compared to healthy controls. Remarkably, no report comprehensively quantifies circulating concentrations of TNF in healthy persons and in patients with sepsis. Therefore, we conducted a systematic review and meta-analysis that addressees this lacuna in the literature and submitted our manuscript that is currently being subjected to peer review. This manuscript is based on a prospectively published research plan (Gharamti et al., 2021), and the manuscript is available in an open-access repository (PROSPERO registration number CRD42020179800) and see https://www.medrxiv.org/content/10.1101/2021.12.13.21267720v1.full.pdf. We found healthy persons have approximately 5.5 pg/ml TNF in the circulation, and in sepsis patients TNF concentration increases to approximately 60 pg/ml. Therefore, the case for a necessary increase in cytokines in sepsis is reasonable with levels approximately 10-fold elevated compared to healthy persons. This criterion of causality establishes a consistent relationship between sepsis and increased pro-inflammatory cytokines.
3.3 Sufficient Condition
To show TNF is sufficient for sepsis, TNF should be capable of producing sepsis. The best available data derive from studies reporting injecting humans with TNF and observing for sepsis manifestations. Recombinant TNF has been injected intravenously into volunteers, usually as attempts to treat cancer (Chapman et al., 1987; Starnes et al., 1988). In general, injection with recombinant TNF can produce fever, tachycardia, and occasional fluid-responsive hypotension at high injection doses; no severe organ dysfunction or death has been reported. However, circulating TNF concentrations following recombinant TNF intravenous infusions were several orders of magnitude larger than amounts in sepsis patients. Serum or plasma TNF levels in human infusion studies were calculated based on dose or directly measured. We calculated TNF serum levels of approximately 100,000 pg/ml—200,000 pg/ml in studies that did not measure TNF, and studies that measured TNF found about 10,000—80,000 pg/ml in circulation (Blick et al., 1987; Chapman et al., 1987; Starnes et al., 1988). We believe available reports cannot support the criterion that pro-inflammatory cytokines are sufficient for sepsis, since injected amounts of TNF vastly exceeded levels that we determined to be present in natural sepsis (approximately 60 pg/ml). Even spectacularly large amounts of infused TNF fail to produce the severe detrimental effects (including death) of natural sepsis. It seems highly unlikely that infusion of TNF at amounts designed to produce circulating levels corresponding to those in natural sepsis will result in clinical effects exceeding influenza-like illness.
3.4 Interventionist Condition
If deliberately altering a putative cause by changing its magnitude or eliminating its presence entirely results in consistent alterations in the putative effect, then a cause-effect relationship is detected. Intuitively, if you “wiggle” the cause, you should “jiggle” the effect. This comprises the interventionist or manipulationist account of causality. This interventionist criterion comprises a powerful method for establishing cause-effect relationships and is useful in explaining how bioscience researchers establish causality in the laboratory or in clinical investigation. James Woodward is a champion of this concept which he describes as “counterfactual invariance under intervention” (Woodward, 2003). This is an epistemic or detection concept of causality, meaning it is a rule or guide that permits one to recognize cause and effect relationships when such relationships exist. Discovering that hyperinflammation or cytokine storm is a cause of sepsis would be satisfied by showing deliberate variation (intervention) in cytokines results in alteration in sepsis. Translating this account of causality into experimental design has focused on sepsis diminution (effect) following blockade (manipulation) of cytokines (cause). Sepsis diminution usually comprises reducing sepsis mortality at 28 days after diagnosis. Since an important goal of showing cytokine storm causes sepsis is to benefit patients, choosing mortality as outcome selects the ultimate benefit. Anti-inflammation interventions designed to treat sepsis are intended for use as adjunct therapies in addition to standard of care measures like antimicrobial drugs, fluid administration, pressor support, mechanical ventilation, vasopressors, and other measures (Evans et al., 2021). Unfortunately, all attempts to satisfy the manipulation criterion to show hyperinflammation causes sepsis have failed. No intervention designed to suppress inflammation or cytokine storm has demonstrated proven clinical benefit, where benefit refers to 28–30 days mortality as outcome. Table 1 shows a non-exhaustive collection of clinical studies that have failed to show beneficial effect for inflammation-blocking interventions. We present this table to underscore the many kinds of inflammation-defeating measures that have been tested. The magnitude of this effort demonstrates failure has not been due to lack of enthusiasm for the concept, lack of resources, or lack of imagination designing novel ways to block inflammation. Reviews of the status of immunomodulator therapies tested for sepsis treatments are typified by this 2014 comment in Opal et al. (2014): “Hundreds of millions of dollars have been expended enrolling over 30,000 patients in clinical trials to test and develop new immunomodulating agents, anti-inflammatory agents, and antiendotoxin agents. Yet, not a single agent has convincingly proven to be consistently efficacious in clinical trials. There are no new drugs on the market to show for all this effort.” Marshall wrote in 2014 “There have been more than 100 Phase II and Phase III clinical rials of strategies to modify the systemic inflammatory response by selectively or nonselective targeting its endogenous mediator molecules” (Marshall, 2014). Marik et al emphasized and expanded on this observation in 2017: “Over the last 3 decades, more than 100 phase 2 and phase 3 clinical trials have been performed testing various novel pharmacologic agents and therapeutic interventions in an attempt to improve the outcome of patients with severe sepsis and septic shock; all of these efforts ultimately failed to produce a novel pharmacologic agent that improved the outcome of sepsis” (Marik et al., 2017). Clearly, the hyperinflammation or cytokine storm concept has failed the interventionist criterion for sepsis causality.
4 Why Hyperinflammation Does Not Cause Sepsis
We do not believe hyperinflammation or cytokine storm is a cause of sepsis. Several lines of evidence support our contention. First, all attempts to block inflammation by numerous approaches have failed to reduce sepsis mortality (Table 1). In fact, we are not aware of any clinical study or case report definitively showing hyperinflammation caused organ malfunction of death. Second, quantification of circulating TNF in natural sepsis revealed concentrations inadequate to cause organ damage or death. In fact, our study determined counterintuitively low TNF levels in sepsis patients https://www.medrxiv.org/content/10.1101/2021.12.13.21267720v1.full.pdf. The circulating TNF levels of approximately 60 pg/ml in sepsis patients is orders of magnitude below concentrations easily tolerated by humans following injection of recombinant TNF (Section 3). Third, concentrations of TNF in sepsis are comparable to levels observed in common non-lethal diseases like rheumatoid arthritis, inflammatory bowel disease, and Streptococcal pharyngitis, casting further doubt that sepsis-associated inflammation causes sepsis (manuscript in preparation).
5 COVID-19
Since sepsis entails linkage between infection and clinical illness, COVID-19 patients can satisfy criteria for sepsis, severe sepsis, or septic shock (Lin et al., 2018; Gu et al., 2020). Importantly, since sepsis is presumed to be caused by hyperinflammation, COVID-19 sepsis adopts the view hyperinflammation or cytokine storm causes illness. Coronavirus disease 2019 has called attention of the hyperinflammation/cytokine storm concept like no previous disease. Remarkably, GOOGLE entries for “Inflammation causes COVID-19” numbers 82,800,000. Articles relating cytokine storm to COVID-19 have appeared in at least 8 of the 10 top US newspapers with largest circulations: The Wall Street Journal, USA Today, The New York Times, New York Daily News, The Washington Post, Minneapolis Star Tribune, Chicago Tribune, and Los Angeles times. The hyperinflammation concept has clearly influenced activities of The Coronavirus Treatment Acceleration Program (CTAP) that was created by the United States Food and Drug Administration (FDA) to rapidly assess and promote potential COVID-19 treatments (https://www.fda.gov/drugs/coronavirus-covid-19-drugs/coronavirus-treatment-acceleration-program-CTAP). The CTAP dashboard lists types of treatments under consideration with “safe to proceed investigational new drugs”. As of 10 March 2022, CTAP listed (excluding vaccines) more than 120 immunomodulator (mostly anti-inflammation) therapies, followed by “other” treatments with more than 110, neutralizing antibodies or cell and gene therapies with over 60 each, and antiviral approaches with more than 50 entries. This snapshot reflects the degree to which inflammation suppressing approaches influence research priorities in developing COVID-19 therapies. Anti-inflammation drugs to treat COVID-19 were investigated early during the pandemic. Following rapid conduct of clinical studies to treat COVID-19, accepted cytokine-suppressing treatments that reportedly lower mortality include drugs that block IL-6 biological activity and the immunosuppressive corticosteroid dexamethasone (Agarwal et al., 2020). Focusing on IL-6 inhibition, comprehensive meta-analyses of randomized controlled trials in hospitalized COVID-19 patients reported mortality benefit for the anti-IL-6 receptor antibody tocilizumab (Tharmarajah et al., 2021; WHO Rapid Evidence Appraisal for COVID-19 Therapies (REACT) Working Group, 2021). However, the history of failed immune-suppressing or cytokine-inhibiting treatments to treat sepsis (Table 1) suggests caution in accepting this conclusion. As elaborated above (Section 3), we add theoretical considerations suggesting inflammation is not the cause of sepsis as reason to closely scrutinize claims to the contrary. In fact, at least three reasons suggest rationale for IL-6 inhibitor COVID-19 benefit is suspect. Reason one is discrepant mortality results for IL-6-blocking drugs with similar mechanisms of biological activity. One meta-analysis showed reported benefit for IL-6 inhibitor therapy restricted to the anti-IL-6 receptor antibody preparation tocilizumab (WHO Rapid Evidence Appraisal for COVID-19 Therapies (REACT) Working Group, 2021). In contrast, no mortality benefit was observed in studies using the IL-6 blocker sarilumab, which has an identical biological mechanism of action as tocilizumab (antibody that binds IL-6 receptor) (WHO Rapid Evidence Appraisal for COVID-19 Therapies (REACT) Working Group, 2021). Reason two involves restriction of tocilizumab mortality benefit to studies employing weaker study design. Randomized controlled studies employing double-blinding and placebo were compared to open-label randomized controlled trials with neither double-blinding nor placebo (WHO Rapid Evidence Appraisal for COVID-19 Therapies (REACT) Working Group, 2021). Mortality benefit was restricted to open label unblinded studies without placebo. No mortality benefit was observed in studies employing double-blinding and placebo. A separate meta-analysis reported a similar pattern of findings with tocilizumab mortality benefit restricted to studies that did not include double-blinding or placebo (Tharmarajah et al., 2021). In fact, in this analysis all three tocilizumab studies with double-blinding and placebo showed nonsignificant increase in mortality in subjects given tocilizumab (Tharmarajah et al., 2021). We have previously expressed skepticism for rationale to use IL-6 inhibition to treat COVID-19 (Scherger et al., 2020). Therefore, absence of tocilizumab mortality benefit in trials with superior study design was anticipated, and we believe using this therapy in COVID-19 patients is dubious. Analysis of the mechanism of the discrepancy behind these two kinds of studies is presented below. Reason three is the underappreciated weight of evidence that must be ignored in order to accept a sepsis benefit due to inflammation-suppressing strategies like IL-6 blockade (Table 1). Benefit for tocilizumab to treat COVID-19 sepsis contradicts the history of failure of cytokine-inhibition therapy to treat sepsis in general. George Santayana reportedly said, “those who cannot remember the past are condemned to repeat it”.
6 COVID-19 and Corticosteroids; Déjà Vu all Over Again
The above quote attributed to the American baseball player Yogi Berra (1925–2015) refers to the tendency for history to repeat itself. Since COVID-19 is an example of sepsis (SARS-CoV-2 infection associated with advanced symptoms or signs, organ damage or death), hyperinflammation as the cause of disease was therefore implied (Lin et al., 2018). The Randomized Evaluation of COVID-19 Therapy (RECOVERY) trial concluded corticosteroid use in selected patients with COVID-19 lowered mortality, and dexamethasone treatment has been incorporated into COVID-19 treatment guidelines (RECOVERY Collaborative Group, 2021). This appears to represent a counterexample to failure of previous trials using corticosteroid therapy to lower sepsis mortality. There has been little discussion pointing out the striking outlier nature of the RECOVERY result. The established history of unsuccessful inflammation-suppressing treatments used for sepsis should urge caution in adopting this kind of COVID-19 therapy (Table 1). Two recent comprehensive reviews of corticosteroids in sepsis assessed 42 and 50 randomized controlled trials and reported no significant benefit in 28 days or short-term mortality (Rochwerg et al., 2018; Liang et al., 2021). Given historical precedent, we are dubious dexamethasone efficacy reported in RECOVERY indicates true corticosteroid efficacy in reducing COVID-19 mortality. Our concerns extend beyond historical steroid failure in sepsis. The ongoing RECOVERY trial uses an adaptive clinical trial design that employs randomization to one of several treatments and includes a usual or standard care arm as control. However, RECOVERY is neither blinded nor placebo controlled. Published assessments suggest these design omissions can bias trial results in favor of intervention (Roberts et al., 1993; Schulz et al., 1995; Hrobjartsson et al., 2012; Anthon et al., 2018; Saltaji et al., 2018). This concern is highlighted when examining a study similar to RECOVERY (Methylprednisolone as adjunctive therapy for patients hospitalized with Coronavirus Disease 2019 or Metcovid) that assessed the corticosteroid methylprednisolone for COVID-19 adjunct therapy (Jeronimo et al., 2021). Unlike RECOVERY, Metcovid employed double-blinding and placebo control. With inclusion of these safeguards against experimental bias, Metcovid did not demonstrate mortality benefit for corticosteroid use. Explanations that protect positive results in RECOVERY from discrepant mortality outcomes in Metcovid can be offered. Differences include use of alternative corticosteroid drugs (dexamethasone vs methylprednisolone), different patient populations and study designs, and smaller number of subjects in Metcovid. However, considering the remarkable history of failure of the hyperinflammation concept, we remain concerned the dexamethasone RECOVERY results are tenuous. Finally, we point out an historical precedent with striking parallels to RECOVERY dexamethasone results that raise additional cause for skepticism. A prior study by Fisher et al investigated the efficacy of adjunctive intravenous IL-1 receptor antagonist (IL-1ra) as a treatment for patients with severe sepsis or septic shock (Fisher et al., 1994b). This study enrolled 99 patients in a randomized, placebo controlled open label study that included three different doses of IL-1ra. Similar to RECOVERY, this trial was unblinded or open label, but unlike RECOVERY employed a placebo and explored a dose-response for IL-1ra. This study demonstrated significant mortality benefit using IL-1ra that also showed dose-responsive mortality reduction. However, two subsequent randomized, double blind, placebo controlled studies could not replicate the Fisher results and showed no mortality benefit for IL-1ra in sepsis (Fisher et al., 1994a; Opal et al., 1997). It is assumed the subsequent large phase 3 trials using strong experimental design revealed a true lack of benefit for IL-1ra in sepsis. This sequence of events is notable since the confirmatory studies employing double-blinding and placebo controls revealed the positive Fisher trial showing IL-1ra mortality benefit was incorrect. Although studying substantially fewer patients than RECOVERY, the positive Fisher study employed placebo control and conducted dose-response assessment of IL-1ra. These represent superior experimental design compared to RECOVERY. We see parallels between RECOVERY and the positive Fisher study and raise concern that subsequent definitive studies using superior methods will fail to replicate corticosteroid benefit in COVID-19. We point out the large RECOVERY trial also showed significant mortality benefit for tocilizumab in COVID-19. As discussed above, there are good reasons to question this conclusion as well.
7 Explaining Persistence of the Cytokine Storm Concept of Sepsis
Despite the troubled record of clinical applicability, the idea hyperinflammation is the cause of sepsis is rarely questioned. In fact, one may wonder if any amount of conflicting observation can disconfirm the cytokine storm concept. This pessimism is supported by sepsis reviews that acknowledge the weak track record of clinical progress in sepsis yet suggest future pathways that continue to follow the same hyperinflammation approach (Cross et al., 1993; Dinarello and Abraham, 2002; Vincent and Abraham, 2006; Rittirsch et al., 2007; Marshall, 2014; Tsirigotis et al., 2016; Cavaillon et al., 2020; De Stefano et al., 2020). Suggestions for future study run a bewildering gamut that includes blocking different cytokines or non-cytokine immune mediators, blocking inflammatory molecule combinations, suppressing inflammation at different times during the course of sepsis, inhibiting inflammation for different durations, altering doses of inflammation inhibitors, inventing biomarker instruments that identify optimal conditions of intervention, investing in genomic or proteomic research to determine the right targets, expand studies to target coagulation or complement, identifying special patient subsets that may benefit from anti-inflammation measures (Shakoory et al., 2016), bolstering inflammation by administration of pro-inflammatory mediators, devising novel sepsis diagnostic criteria, changing clinical endpoints like longer term mortality or disability, and altering design of clinical studies to more efficiently assess novel agents (Talisa et al., 2018). These suggestions prescribe altering almost everything except the hyperinflammation concept itself. We believe resistance of the hyperinflammation concept to contradicting results is striking and needs to be explained. A search for such an explanation led us to characterize the cytokine storm concept of sepsis as a scientific paradigm as conceived by Thomas Kuhn. The trajectory of scientific progress has been extensively studied by Thomas Kuhn (a physicist and philosopher of science) who popularized the terms “paradigm” and “paradigm shift” in his book titled: “The Structure of Scientific Revolutions” (Kuhn, 1962). Kuhn proposed science transitions through cycles that include discontinuous radical changes in scientific direction referred to as revolutionary science or paradigm shifts (Figure 1). Such shifts disrupt intervening periods of smooth accumulative scientific progress that he characterized as “normal” science or science conducted under direction of an existing paradigm. We believe applying Kuhn’s ideas about scientific progress provide the best available explanation for some peculiarities of the cytokine storm sepsis concept. If we cast the cytokine storm concept as the current paradigm directing investigation in a period of normal science, surprising persistence of the hyperinflammation approach becomes an anticipated phenomenon. We adapt features of Kuhn’s view of science to explain characteristics of the field of sepsis investigation that are otherwise puzzling. The two elements we find most interesting are the near-inexhaustible tolerance for repeated disconfirming clinical investigation (Table 1) and the lack of progress in enhancing patient care despite expenditure of massive resources to develop adjuvant immunomodulator sepsis therapies (conservatively estimated at >10 billion US dollars) (Marshall, 2014). Applying Kuhn’s view of scientific development to provide insight to understand the trajectory of sepsis investigation has been noticed previously. Artenstein et al invoke Kuhn’s thoughts on scientific progress to describe the course of anti-inflammation research in sepsis (Artenstein et al., 2013). Artenstein et al focus on supposed paradigm shifts in describing innovations in sepsis therapeutics (Artenstein et al., 2013). However, we believe the focus in Artenstein et al on Kuhn’s notion of paradigm shifts overlooks the most relevant contribution of Kuhn’s ideas to sepsis research. We believe characteristics of normal science or science conducted within an existing paradigm is most relevant for understanding peculiarities of the history of sepsis investigation.
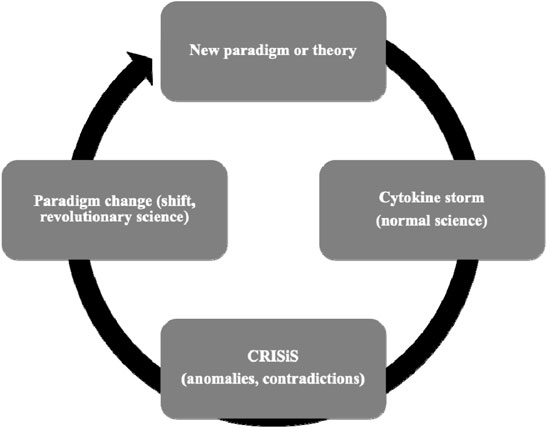
FIGURE 1. Adapted Kuhn cycle of scientific advancement using the cytokine storm paradigm as example.
7.1 Scientific Paradigm and Normal Science
The term “paradigm” is notoriously imprecise and detailed characterization exceeds the bounds of this report (Lakatos and Musgrave, 1970; Buchwald and Smith, 1997; Kindi and Arabatzis, 2012; Franklin, 2015; Orman, 2016). Kuhn’s view of paradigms evolved into the view that a paradigm is a disciplinary matrix (Kindi and Arabatzis, 2012). Due to extensive use and familiarity with the term “paradigm”, we use disciplinary matrix and paradigm interchangeably. We also select Kuhn concepts that readily apply to sepsis. A paradigm has two primary components that include scientific theory and exemplars. The theory component of a paradigm includes objects in nature (cytokines and other molecules, cells with various assigned functions, signaling molecules, receptors, drugs/therapies), defined patterns of interactions of the objects (innate immunity, inflammation, signaling pathways, cytokine storm, sepsis), and defined cause-effect relationships (inflammation causes organ malfunction or death, some molecules cause inflammation while other molecules suppress inflammation). Exemplars are concrete solved problems in science that serve as templates for teaching and research. They serve as guides for conduct of future investigation. They are paragons or especially clear solved problems that appear in textbooks and serve as precedents that are like legal precedent in case law. Effective exemplars demonstrate patterns of reasoning within a paradigm that demonstrate the pathway to future discoveries. A possible cytokine storm exemplar would be injection of endotoxin into animals with mortality reduction following administration of a TNF or IL-1 antagonist (Beutler et al., 1985; Ohlsson et al., 1990). A pivotal analogy formulated by Masterman compares paradigms to puzzle solutions (Lakatos and Musgrave, 1970). According to Masterman, normal science proceeds with the final experimental outcome known beforehand, but the pieces of the puzzle and how they fit together is specific to each of many experimental solutions to the puzzle. Given this analogy, we can characterize exemplars as especially transparent examples of how to solve puzzles and arrive at the correct answer repeatedly. By “correct” we refer to experimental results that support outcomes specified by the paradigm. According to Kuhn, the conduct of normal science is like puzzle solving and the goal of normal science is to solve as many interesting puzzles as possible. Importantly, the puzzle always looks the same, but the pieces keep changing shapes. The paradigm (final puzzle picture) is always the same even though the pieces used to make the puzzle can differ (different experiments). Applied to sepsis research, the puzzle pieces can differ according to different experimental designs, but the fnal picture defined by the pieces always shows cytokine storm. During the period of normal science, experimentation is conducted in service of an existing paradigm.
We consider current investigation in sepsis to be conducted under direction of the hyperinflammation or cytokine storm paradigm. Therefore, the field of sepsis research is operating within a period of normal sciences. Importantly, the paradigm defines which problems to pursue, what methodologies are used to pursue them, and what counts as a successful explanation for any problem under investigation (Kindi and Arabatzis, 2012). According to Kuhn, paradigms comprise the background conceptual scheme that directs the conduct and interpretation of research. The paradigm itself is never questioned. An interesting component of Kuhn’s concept of a paradigm is the evolution of a social structure including professional groups and institutions designed to project investigation into the future and includes funding organizations, professional journals and peer reviewers, guidelines for teaching, and standardized criteria for experimental methods. The social structure is designed to extend investigation into the future. It will also defend and augment the existing paradigm. Now let us apply Kuhn’s views on science to sepsis as an attempt to shed light on two peculiarities of sepsis study.
7.2 Life Within Normal Science: Paradigm Maintenance and Tolerance for Disconfirming Anomalies
In Kuhn’s view, normal science is highly resistant to anomalous or disconfirming observations, and data that do not fit an existing paradigm are ignored, altered in such a way as to be incorporated into the existing paradigm, or explained away by seeking reasons for experimental deviation from the existing paradigm. The paradigm itself is preserved at (nearly) all costs. A quote from Structure of Scientific revolutions is telling in this regard where Kuhn writes “it is a poor workman who blames his tools for a bad outcome” (Kuhn, 1962). Kuhn refers to pressure experienced by scientists to produce results that conform with the existing paradigm. Challenging the paradigm itself is not an option. Using the Masterman puzzle solving analogy of normal science, no matter what scientific question is posed in sepsis investigation, the answer is always hyperinflammation. The task for investigators is to construct an experimental path that solves the problem in a way that comports with hyperinflammation. As an example, consider research effort designed to understand COVID-19. Emergence of this disease presented many new challenges (puzzles) for investigators. Since current researchers operate within the hyperinflammation paradigm, these scientific puzzles were approached in a way that ensures cytokine storm is somehow characterized as the cause of disease. This accounts for the abundance of COVID-19 investigation that begins or concludes with elements of cytokine storm or hyperinflammation. It is no exaggeration to state reviews of COVID-19 pathogenesis always call forth the hyperinflammation paradigm.
Mechanisms that explain maintenance of the sepsis cytokine storm paradigm likely include two characteristics of paradigms. First, Kuhn’s emphasis on the role of a social structure that accompany paradigms is of special importance. Institutions enable and promote modern bioscience and they are heavily biased for care and maintenance of the current paradigm. Most scientists and physicians are educated to understand sepsis as a manifestation of cytokine storm and textbooks actively maintain the cytokine storm concept by depicting exemplars that encapsulate the contemporary approach to solving problems in infectious diseases. Journals favor manuscripts that offer experimental results that favor the hyperinflammation concept, and even lay press publications largely support the concept. Most importantly, funding agencies are populated with proponents of cytokine storm since adherence to this concept defines expertise in this field. A successful career in the modern era of bioscience requires large sums of funding to conduct research. Millions of US dollars are required to support even modest careers. Given these considerations, outlier scientists skeptical of cytokine storm face substantial difficulties obtaining resources with which to pursue such a controversial line of investigation. In fact, it is doubtful adherents to the current paradigm would view the work of anyone challenging that paradigm as conducting legitimate research. Funding agencies are unlikely to expend limited resources to support “speculative” experiments with a perceived small possibility of scientific return. Committing one’s career to being an outlier or “lone wolf” presents high risk for failure and such individuals are likely to receive little institutional, emotional, or financial support during career development. Second, conceptual confusion in the sepsis field is characterized by imprecision and weak definitions (Section 2). This can insulate the cytokine storm paradigm from falsification. Due to conceptual confusion, data of almost any sort can be interpreted as satisfying the paradigm. For example, clinical investigations often quantify multiple cytokines using multiplex assay platforms. Retrospective statistical analysis can usually generate association(s) between one or more cytokines and some clinical outcome or other. Since the concept of inflammation poorly characterizes which cytokines are pro-inflammatory, association between nearly every cytokine that can be measured with any of several clinical outcomes (mortality, use of oxygen, admission to intensive care unit, need for pressor support, etc) can be interpreted as a confirming instance of the cytokine storm sepsis paradigm. Imprecise sepsis-related concepts have led to retrospective re-examination of failed clinical trials to identify subgroups of patients that appeared to benefit from anti-inflammation interventions. Since concepts like inflammation or cytokine storm lack precision, these subgroups cannot be excluded as unlikely to benefit from anti-inflammation therapy. Examples of such subgroups include sepsis in a specific race (Bernard et al., 1997), bacterial etiology subgroups (Greenman et al., 1991; Ziegler et al., 1991; Fein et al., 1997), level of coagulopathy (Abraham et al., 2001; Abraham et al., 2003b), severity of sepsis at enrollment (Fisher et al., 1994a), and subgroups defined by circulating cytokine concentrations (Ferrara et al., 1993; Reinhart et al., 1996; Reinhart et al., 2001). No subgroup defined retrospectively led to successful therapy when subsequently studied prospectively. The cytokine storm paradigm is too imprecise to restrict expansion of subgroup analyses that serve up additional interventions based on cytokine storm.
Summarizing the points above, at least two characteristics of the cytokine storm paradigm foster persistence of this concept despite inability to deliver clinical treatment. First, social institutions formed around the cytokine storm paradigm direct the flow of many elements of science into this paradigm. A sepsis investigator encounters an educational history, funding agencies, and publishing organizations that channels the conduct of science to support the existing paradigm. Second, conceptual confusions in the sepsis field provide escape mechanisms that stymie falsification. Contradictory results are explained by failure of experimental design or conduct instead of paradigm failure. According to Kuhn, during normal science one possibility not to be considered is that investigation is conducted under direction of a faulty paradigm. Kuhn’s model of scientific progress appears to provide an understanding of how the current sepsis paradigm has survived despite apparent pressures to be discarded.
7.3 You Cannot Experiment Your Way out of a Failing Paradigm
Elements of theory within a paradigm establishes the menu of tools available for experimentation. This is a component of the theory-laden or theory-infection quality of experimentation (Franklin, 2015). Since we equate paradigm with a theory and associated exemplars, we refer to this idea as paradigm-laden or paradigm infection of experiment. As an example, sepsis experiments often use specific cell lines or animals, special stimuli like endotoxin or mitogens, selected culture conditions that specify atmospheric composition and temperature, outcomes that include cytokine quantification or other inflammation-related molecules. At every stage of experimentation from conception to design to available materials and protocols followed to interpretation of results, there is influence on experimental conduct exerted by cytokine storm orthodoxy. For a clear and striking example of how experiment is directed by paradigm or theory, consider the recent discovery of gravitational waves (Miller and Yunes, 2019). Existence of gravitational waves was inconceivable prior to the advent of Einstein’s theory of relativity since no prior paradigm contained a way to imagine gravitational waves. The relativity paradigm was used to design a unique experimental device called the Laser interferometer gravitation-wave observatory (LIGO) that did, in fact, detect gravitational waves in 2016. Note that LIGO was designed, engineered, and operated in accord with Einstein’s paradigm, demonstrating that a paradigm determines what is looked for in science and how one designs experiments that can find what you are looking for. Prior to Einstein, no amount of experimentation could possibly have discovered gravitational waves. Similarly, we are convinced experiments and clinical studies conducted within the cytokine storm paradigm are unlikely to lead to effective sepsis treatments. Decades of investment in experimentation under the guidance of cytokine storm has produced little therapeutic payoff. The pathway to advancement may not be through experimentation alone.
8 A Road Forward
If further experimentation is unlikely to provide meaningful advancement in sepsis therapeutics, we need to entertain alternative approaches. When applied to Kuhn’ model, the cytokine storm sepsis paradigm is in a state of normal science crisis. According to Kuhn, a scientific crisis is characterized by accumulation of anomalies that cannot be accommodated by an existing paradigm. Over time, accumulation of contradicting data can reach a point where the paradigm fails to function as a driver of scientific advancement and fails to solve puzzles. Normal science anomalies include contradictory observations (Table 1) and an inability to explain, predict, and control the empirical world. The criterion of control for cytokine storm focuses on delivering treatments based on suppressing inflammation. It is clear the cytokine storm paradigm cannot solve puzzles it should be able to solve. Figure 1 depicts a modified version of the Kuhn cycle of scientific progress.
8.1 Paradigm Shift
If we accept the verdict that the hyperinflammation sepsis paradigm is in crisis, resolution of the crisis will likely require a “paradigm shift.” Two impediments pose challenges for altering the current paradigm. The first impediment is Kuhn’s observation that the presence of crisis in science does not entail a search for paradigm alteration. In fact, shedding an existing paradigm is often the solution of last resort if it is considered at all. History shows anomalies or contradicting observations can be tolerated for prolonged periods of time and mechanisms are generated within the existing paradigm to explain anomalies (Kuhn, 1962).
As pointed out above, examination of commentaries acknowledging the presence of anomalies or failure of sepsis research to produce clinical benefit confirms this. The response to anomalies has been to invoke numerous suggestions for tweaking or rehabilitating the existing hyperinflammation sepsis paradigm. However, there has been no call to abandon the hyperinflammation or cytokine storm concept (Dinarello, 1991a; Cross et al., 1993; Vincent and Abraham, 2006; Rittirsch et al., 2007; Marshall, 2014; Tsirigotis et al., 2016; Cavaillon et al., 2020; De Stefano et al., 2020). This corresponds closely to Kuhn’s view that paradigm challenge is not an option that is entertained when things go wrong. The second impediment to sepsis paradigm change is pivotal. The Kuhn view includes the observation that an existing paradigm cannot be changed unless a viable alternative paradigm is presented as a replacement. Paradigms cannot be repealed until they are replaced, as stated explicitly in page 18 in Kindi and Arabatzis (Kindi and Arabatzis, 2012). Another way of stating this observation is to note paradigms are never falsified, they are replaced. Given the vital role a paradigm serves for conducting scientific research, it stands to reason an existing paradigm cannot be jettisoned without a replacement. In our view, lack of a replacement sepsis paradigm is the largest obstacle to generating sepsis therapies that will improve outcomes. Since it is unlikely a replacement paradigm can originate from experimentation, a replacement is more likely to emerge from investigators who function as medical theoreticians conversant with the basic research, clinical, and philosophical challenges associated with constructing a radically different view of sepsis. We are not aware of a sepsis paradigm alternative to cytokine storm in the public domain. However, our group has, in fact, devised a complete and consistent novel sepsis paradigm that has potential to replace cytokine storm. Discussing specific contents exceeds the focus of this report. However, some properties of this novel sepsis theory are noteworthy. It dispenses with any notion of hyperinflammation, explains why sepsis therapies have failed in the past, and proposes novel therapeutic approaches to treating sepsis.
9 Discussion
Standard of care for patients with sepsis includes rapid administration of antimicrobial drugs and supportive measures. However, despite advances in these areas, persistent substantial mortality is observed in sepsis. For this reason, attention has focused on adjunctive therapies targeting the pathogen-triggered host inflammatory response to infection. This approach follows a line of reasoning that posits hyperinflammation as the final common cause of organ malfunction or death. The COVID-19 pandemic has thrust hyperinflammation or cytokine storm to the forefront of discussion by scientific investigators, clinical trialists, and the lay public. Associating COVID-19 with cytokine storm was expected since severe COVID-19 is an example of sepsis, or infection sufficient to cause organ malfunction or death. Since sepsis is thought to be caused by hyperinflammation, a link between COVID-19 and hyperinflammation was forged. A substantial research tradition identifies pro-inflammatory cytokines as pivotal mediators of inflammation and are thus implicated as potentially reversible causes of host inflammation in response to infection. This accounts for use of the term “cytokine storm” to characterize sepsis hyperinflammation. Unfortunately, despite expenditure of ample resources to materially affect sepsis mortality by blocking inflammation, we have been unable to affect sepsis mortality using this concept. There is no indication we are on the verge of reversing this trend.
We believe the hyperinflammation or cytokine storm approach to sepsis is an imperiled concept. In this report we identify three elements that we believe are at the heart of difficulties with this concept. The three elements include 1) confusion and imprecision in descriptions of the hyperinflammation concept, 2) weak evidence supporting the idea hyperinflammation is the cause of sepsis, and 3) persistence of the hyperinflammation concept despite inability of therapies based on this concept to treat sepsis. First, we analyzed concepts that underly the hyperinflammation approach of sepsis. We found a surprising degree of conceptual confusion in pivotal ideas. The terms “inflammation” and “cytokine storm” are vague and imprecise. Considering inflammation, no explanation or definition precisely characterizes the systemic inflammation that presumedly causes sepsis. In fact, contemporary understanding of inflammation continues to refer to ideas present since antiquity. Prior sepsis definitions emphasized fever or hypothermia, tachypnea, tachycardia, and leukocytosis or leukopenia. How these patient data relate to inflammation is unclear. Inflammation manifests differently in separate individuals, and it can present in different ways in the same individual. An adequate account of inflammation must derive from an understanding of regularities present in all manifestations of inflammation. We believe such an account of inflammation must originate from a theory-driven characterization that directs selection of clinical or laboratory indicators that can identify and quantify inflammation. Relatedly, the term “cytokine storm” is vague and imprecise. We assume cytokine storm refers to excessive production of molecules that cause overexuberant inflammation and therefore sepsis. However, there is no consensus on which cytokines are relevant for production of inflammation. Moreover, there is no concept of what cytokine concentrations constitutes an excessive amount. Conceptual confusion has adverse scientific and clinical consequences. Given suppression of inflammation as a goal of sepsis therapy, we need to identify the right molecular targets to suppress. We believe progress in sepsis requires re-thinking concepts of inflammation and cytokine storm. Therefore, we have developed a novel conception of inflammation that attempts to demystify these concepts (manuscript in preparation).
Second, we analyzed the status of evidence supporting a cause-effect relationship between hyperinflammation and sepsis. Using criteria of necessity, sufficiency, and a pivotal interventionist criterion for causality, hyperinflammation or cytokine storm fails to satisfy criteria as a cause of sepsis.
Third, we have been struck by remarkable resilience of the hyperinflammation concept to contradicting clinical assessment. The concept persists with apparent indifference to a remarkable record of repeated inability to deliver clinical success (Table 1). It appears the concept is unfalsifiable by any amount of contradicting data. We believe Thomas Kuhn’s portrayal of scientific progress best explains this resilience. Kuhn’s description of the characteristics of normal science, or science conducted from within an established paradigm, is most applicable. It is reasonable to characterize hyperinflammation or cytokine storm as the current sepsis paradigm, where paradigm is the conceptual scheme that guides a scientific field. Paradigm components emphasize background theory and exemplars (Section 7). Defects we believe plague the cytokine storm paradigm include background knowledge (theory) that contains imprecise and poorly characterized concepts like inflammation and cytokine storm, mischaracterization of molecules/substances as pro- or anti-inflammatory, incorrect cause-effect relationships, and absent characterization of regularities relevant for an adequate theoretical description of sepsis. Exemplars are specific solved problems that serve as templates or patterns that guide future investigation. Objects and relations depicted in exemplars derive from theory and act as practical specific examples of how theory is supposed to apply to scientific observations. We fear currently employed exemplars are misguided and will direct research in unfruitful directions.
Kuhn refers to normal science as science conducted under the direction of a monpolistic paradigm. The paradigm is not questioned, and anomalous or contradictory observations are explained by errors of experimental design or experimental conduct. The underlying paradigm remains immune to disconfirmation and is insulated from experimental assessment. The Kuhn account of scientific progress anticipates problems that can originate from a faulty paradigm. The lack of precision or conceptual vagueness in the cytokine storm paradigm enables contradicting observations to be interpreted as consistent with hyperinflammation and cannot be used as falsifying examples. Failed clinical sepsis trails are nearly always viewed as failures of experimental design or conduct and are not used as reasons to challenge the underlying paradigm (Table 1). This accounts for suggestions to press on or “double down” by blocking different cytokines or combination blockade, treating different patient sub-populations, altering the dose or timing of anti-inflammation drugs, changing the outcome from mortality, and on and on ad infinitum. The one thing never questioned is the underlying hyperinflammation paradigm. A key implication is that paradigm change does not originate from experimentation alone. Indeed, the Kuhn concepts of scientific progress provides an account that explains resilience of the hyperinflammation or cytokine storm paradigm of sepsis despite substantial disconfirming data (Table 1). In accord with the Kuhn view of science, we believe a paradigm shift is long overdue in the field of sepsis. As Kuhn predicts, experimental triumph or failure is not the engine of paradigm change. The cytokine storm paradigm appears to represent a confirming example of Kuhn’s analysis of how science progresses. You cannot experiment your way out of a failing paradigm, and paradigms can be replaced but they cannot be falsified. We surmise the primary obstacle for paradigm shift in sepsis is unavailability of an alternative paradigm. Existing paradigms are never abandoned in the absence of a novel paradigm ready for adoption. Our group has developed such a novel sepsis paradigm that invokes no role for inflammation whatever. This will be the subject of a separate report. Finally, the ghost mentioned in our title refers to use of hyperinflammation or cytokine storm to understand and treat sepsis. We believe this paradigm is based on an illusion related to a reversal of cause-and-effect. Continued pursuit will be as unproductive as ghost hunting. We believe inflammation and sepsis are not causally related and inflammation has little, if anything, to do with sepsis. We have been chasing the nonexistent ghost of hyperinflammation in the belief it will somehow explain sepsis or provide guidance to improve patient care.
Data Availability Statement
The original contributions presented in the study are included in the article/Supplementary Material, further inquiries can be directed to the corresponding author.
Author Contributions
LS conceived general ideas and wrote the initial draft. SS, CF-P, AG, DF, and AFH-M contributed to concepts expressed in the manuscript, contributed to writing the manuscript, and reviewed and corrected the text.
Funding
LS is supported by The Emily Foundation for Medical Research, One Beacon Street, 15th Floor, Boston MA 02108, United States. This is a Foundation Grant for research investigating the role of inflammation in sepsis and infectious diseases. LS receives University of Colorado Royalty payments for work on an unrelated project. These funds can be used for open-access payments.
Conflict of Interest
The authors declare that the research was conducted in the absence of any commercial or financial relationships that could be construed as a potential conflict of interest.
Publisher’s Note
All claims expressed in this article are solely those of the authors and do not necessarily represent those of their affiliated organizations, or those of the publisher, the editors and the reviewers. Any product that may be evaluated in this article, or claim that may be made by its manufacturer, is not guaranteed or endorsed by the publisher.
Abbreviations
COVID, Coronavirus disease 2019; CTAP, Coronavirus treatment acceleration program; FDA, food and drug administration (United States); IL, interleukin; IL-1ra, IL-1 receptor antagonist; Metcovid, methylprednisolone as adjunctive therapy for patients hospitalized with Coronavirus Disease 2019; RECOVERY, randomized evaluation of COVID-19 therapy; SARS-CoV-2, severe acute respiratory syndrome Coronavirus 2; SCCM, society of critical care medicine; SIRS, systemic inflammatory response syndrome; SOFA, sequential organ failure assessment; TNF, tumor necrosis factor alpha.
References
Abraham, E., Anzueto, A., Gutierrez, G., Tessler, S., San Pedro, G., Wunderink, R., et al. (1998). Double-blind Randomised Controlled Trial of Monoclonal Antibody to Human Tumour Necrosis Factor in Treatment of Septic Shock. NORASEPT II Study Group. Lancet 351 (9107), 929–933. doi:10.1016/s0140-6736(05)60602-2
Abraham, E., Laterre, P. F., Garg, R., Levy, H., Talwar, D., Trzaskoma, B. L., et al. (2005). Drotrecogin Alfa (Activated) for Adults with Severe Sepsis and a Low Risk of Death. N. Engl. J. Med. 353 (13), 1332–1341. doi:10.1056/NEJMoa050935
Abraham, E., Naum, C., Bandi, V., Gervich, D., Lowry, S. F., Wunderink, R., et al. (2003a). Efficacy and Safety of LY315920Na/S-5920, a Selective Inhibitor of 14-kDa Group IIA Secretory Phospholipase A2, in Patients with Suspected Sepsis and Organ Failure. Crit. Care Med. 31 (3), 718–728. doi:10.1097/01.CCM.0000053648.42884.89
Abraham, E., Reinhart, K., Opal, S., Demeyer, I., Doig, C., Rodriguez, A. L., et al. (2003b). Efficacy and Safety of Tifacogin (Recombinant Tissue Factor Pathway Inhibitor) in Severe Sepsis: a Randomized Controlled Trial. JAMA 290 (2), 238–247. doi:10.1001/jama.290.2.238
Abraham, E., Reinhart, K., Svoboda, P., Seibert, A., Olthoff, D., Dal Nogare, A., et al. (2001). Assessment of the Safety of Recombinant Tissue Factor Pathway Inhibitor in Patients with Severe Sepsis: a Multicenter, Randomized, Placebo-Controlled, Single-Blind, Dose Escalation Study. Crit. Care Med. 29 (11), 2081–2089. doi:10.1097/00003246-200111000-00007
Abraham, E., Wunderink, R., Silverman, H., Perl, T. M., Nasraway, S., Levy, H., et al. (1995). Efficacy and Safety of Monoclonal Antibody to Human Tumor Necrosis Factor Alpha in Patients with Sepsis Syndrome. A Randomized, Controlled, Double-Blind, Multicenter Clinical Trial. TNF-Alpha MAb Sepsis Study Group. JAMA 273 (12), 934–941. doi:10.1001/jama.273.12.934
Aderka, D., Le, J. M., and Vilcek, J. (1989). IL-6 Inhibits Lipopolysaccharide-Induced Tumor Necrosis Factor Production in Cultured Human Monocytes, U937 Cells, and in Mice. J. Immunol. 143 (11), 3517–3523.
Annane, D., Sébille, V., Charpentier, C., Bollaert, P. E., François, B., Korach, J. M., et al. (2002). Effect of Treatment with Low Doses of Hydrocortisone and Fludrocortisone on Mortality in Patients with Septic Shock. JAMA 288 (7), 862–871. doi:10.1001/jama.288.7.862
Anthon, C. T., Granholm, A., Perner, A., Laake, J. H., and Møller, M. H. (2018). No Firm Evidence that Lack of Blinding Affects Estimates of Mortality in Randomized Clinical Trials of Intensive Care Interventions: a Systematic Review and Meta-Analysis. J. Clin. Epidemiol. 100, 71–81. doi:10.1016/j.jclinepi.2018.04.016
Antonelli, M., and Kushner, I. (2017). It's Time to Redefine Inflammation. FASEB J. 31 (5), 1787–1791. doi:10.1096/fj.201601326R
Artenstein, A. W., Higgins, T. L., and Opal, S. M. (2013). Sepsis and Scientific Revolutions. Crit. Care Med. 41 (12), 2770–2772. doi:10.1097/CCM.0b013e31829eb98f
Bajaj, M. S., Kew, R. R., Webster, R. O., and Hyers, T. M. (1992). Priming of Human Neutrophil Functions by Tumor Necrosis Factor: Enhancement of Superoxide Anion Generation, Degranulation, and Chemotaxis to Chemoattractants C5a and F-Met-Leu-Phe. Inflammation 16 (3), 241–250. doi:10.1007/BF00918813
Bauss, F., Dröge, W., and Männel, D. N. (1987). Tumor Necrosis Factor Mediates Endotoxic Effects in Mice. Infect. Immun. 55 (7), 1622–1625. doi:10.1128/iai.55.7.1622-1625.1987
Bernard, G. R., Vincent, J. L., Laterre, P. F., LaRosa, S. P., Dhainaut, J. F., Lopez-Rodriguez, A., et al. (2001). Efficacy and Safety of Recombinant Human Activated Protein C for Severe Sepsis. N. Engl. J. Med. 344 (10), 699–709. doi:10.1056/NEJM200103083441001
Bernard, G. R., Wheeler, A. P., Russell, J. A., Schein, R., Summer, W. R., Steinberg, K. P., et al. (1997). The Effects of Ibuprofen on the Physiology and Survival of Patients with Sepsis. The Ibuprofen in Sepsis Study Group. N. Engl. J. Med. 336 (13), 912–918. doi:10.1056/NEJM199703273361303
Beutler, B., Milsark, I. W., and Cerami, A. C. (1985). Passive Immunization against Cachectin/tumor Necrosis Factor Protects Mice from Lethal Effect of Endotoxin. Science 229 (4716), 869–871. doi:10.1126/science.3895437
Beutler, B., Milsark, I. W., and Cerami, A. C. (2008). Passive Immunization against Cachectin/tumor Necrosis Factor Protects Mice from Lethal Effect of Endotoxin. Science, 1985, 229(4716):869-871. Classical Article. J. Immunol. 181 (1), 7–9. doi:10.1126/science.3895437
Blick, M., Sherwin, S. A., Rosenblum, M., and Gutterman, J. (1987). Phase I Study of Recombinant Tumor Necrosis Factor in Cancer Patients. Cancer Res. 47 (11), 2986–2989.
Bojalil, R., Mata-González, M. T., Sánchez-Muñoz, F., Yee, Y., Argueta, I., Bolaños, L., et al. (2013). Anti-tumor Necrosis Factor VNAR Single Domains Reduce Lethality and Regulate Underlying Inflammatory Response in a Murine Model of Endotoxic Shock. BMC Immunol. 14, 17. doi:10.1186/1471-2172-14-17
Bone, R. C., Fisher, C. J., Clemmer, T. P., Slotman, G. J., Metz, C. A., and Balk, R. A. (1987). A Controlled Clinical Trial of High-Dose Methylprednisolone in the Treatment of Severe Sepsis and Septic Shock. N. Engl. J. Med. 317 (11), 653–658. doi:10.1056/NEJM198709103171101
Bradley, J. R. (2008). TNF-mediated Inflammatory Disease. J. Pathol. 214 (2), 149–160. doi:10.1002/path.2287
Buchwald, J. Z., and Smith, G. E. (1997). Thomas S. Kuhn, 1922-1996. Philos. Sci. 64 (2), 361–376. doi:10.1086/392557
Cavaillon, J. M., Singer, M., and Skirecki, T. (2020). Sepsis Therapies: Learning from 30 Years of Failure of Translational Research to Propose New Leads. EMBO Mol. Med. 12 (4), e10128. doi:10.15252/emmm.201810128
Chapman, P. B., Lester, T. J., Casper, E. S., Gabrilove, J. L., Wong, G. Y., Kempin, S. J., et al. (1987). Clinical Pharmacology of Recombinant Human Tumor Necrosis Factor in Patients with Advanced Cancer. J. Clin. Oncol. 5 (12), 1942–1951. doi:10.1200/JCO.1987.5.12.1942
Chaudhry, H., Zhou, J., Zhong, Y., Ali, M. M., McGuire, F., Nagarkatti, P. S., et al. (2013). Role of Cytokines as a Double-Edged Sword in Sepsis. Vivo 27 (6), 669–684.
Chi, Y., Ge, Y., Wu, B., Zhang, W., Wu, T., Wen, T., et al. (2020). Serum Cytokine and Chemokine Profile in Relation to the Severity of Coronavirus Disease 2019 in China. J. Infect. Dis. 222 (5), 746–754. doi:10.1093/infdis/jiaa363
Cicarelli, D. D., Vieira, J. E., and Benseñor, F. E. (2007). Early Dexamethasone Treatment for Septic Shock Patients: a Prospective Randomized Clinical Trial. Sao Paulo Med. J. 125 (4), 237–241. doi:10.1590/s1516-31802007000400009
Cohen, J., and Carlet, J. (1996). INTERSEPT: an International, Multicenter, Placebo-Controlled Trial of Monoclonal Antibody to Human Tumor Necrosis Factor-Alpha in Patients with Sepsis. International Sepsis Trial Study Group. Crit. Care Med. 24 (9), 1431–1440. doi:10.1097/00003246-199609000-00002
Cross, A. S., Opal, S. M., Sadoff, J. C., and Gemski, P. (1993). Choice of Bacteria in Animal Models of Sepsis. Infect. Immun. 61 (7), 2741–2747. doi:10.1128/iai.61.7.2741-2747.1993
Cruz, D. N., Antonelli, M., Fumagalli, R., Foltran, F., Brienza, N., Donati, A., et al. (2009). Early Use of Polymyxin B Hemoperfusion in Abdominal Septic Shock: the EUPHAS Randomized Controlled Trial. JAMA 301 (23), 2445–2452. doi:10.1001/jama.2009.856
De Stefano, L., Bobbio-Pallavicini, F., Manzo, A., Montecucco, C., and Bugatti, S. (2020). A "Window of Therapeutic Opportunity" for Anti-cytokine Therapy in Patients with Coronavirus Disease 2019. Front. Immunol. 11, 572635. doi:10.3389/fimmu.2020.572635
Dellinger, R. P., Bagshaw, S. M., Antonelli, M., Foster, D. M., Klein, D. J., Marshall, J. C., et al. (2018). Effect of Targeted Polymyxin B Hemoperfusion on 28-Day Mortality in Patients with Septic Shock and Elevated Endotoxin Level: The EUPHRATES Randomized Clinical Trial. JAMA 320 (14), 1455–1463. doi:10.1001/jama.2018.14618
Dellinger, R. P., Tomayko, J. F., Angus, D. C., Opal, S., Cupo, M. A., McDermott, S., et al. (2009). Efficacy and Safety of a Phospholipid Emulsion (GR270773) in Gram-Negative Severe Sepsis: Results of a Phase II Multicenter, Randomized, Placebo-Controlled, Dose-Finding Clinical Trial. Crit. Care Med. 37 (11), 2929–2938. doi:10.1097/CCM.0b013e3181b0266c
Derkx, B., Wittes, J., and McCloskey, R. (1999). Randomized, Placebo-Controlled Trial of HA-1A, a Human Monoclonal Antibody to Endotoxin, in Children with Meningococcal Septic Shock. European Pediatric Meningococcal Septic Shock Trial Study Group. Clin. Infect. Dis. 28 (4), 770–777. doi:10.1086/515184
Deshpande, A., Pasupuleti, V., and Rothberg, M. B. (2015). Statin Therapy and Mortality from Sepsis: a Meta-Analysis of Randomized Trials. Am. J. Med. 128 (4), 410–e1. e411. doi:10.1016/j.amjmed.2014.10.057
Dhainaut, J. F., Tenaillon, A., Hemmer, M., Damas, P., Le Tulzo, Y., Radermacher, P., et al. (1998). Confirmatory Platelet-Activating Factor Receptor Antagonist Trial in Patients with Severe Gram-Negative Bacterial Sepsis: a Phase III, Randomized, Double-Blind, Placebo-Controlled, Multicenter Trial. BN 52021 Sepsis Investigator Group. Crit. Care Med. 26 (12), 1963–1971. doi:10.1097/00003246-199812000-00021
Dhainaut, J. F., Tenaillon, A., Le Tulzo, Y., Schlemmer, B., Solet, J. P., Wolff, M., et al. (1994). Platelet-activating Factor Receptor Antagonist BN 52021 in the Treatment of Severe Sepsis: a Randomized, Double-Blind, Placebo-Controlled, Multicenter Clinical Trial. BN 52021 Sepsis Study Group. Crit. Care Med. 22 (11), 1720–1728. doi:10.1097/00003246-199422110-00005
Dinarello, C. A., and Abraham, E. (2002). Does Blocking Cytokines in Sepsis Work? Am. J. Respir. Crit. Care Med. 166 (9), 1156–1157. doi:10.1164/rccm.2208006
Dinarello, C. A. (1999). Cytokines as Endogenous Pyrogens. J. Infect. Dis. 179 (Suppl. 2), S294–S304. doi:10.1086/513856
Dinarello, C. A. (2007). Historical Insights into Cytokines. Eur. J. Immunol. 37 (Suppl. 1), S34–S45. doi:10.1002/eji.200737772
Dinarello, C. A. (1991a). Interleukin-1 and Interleukin-1 Antagonism. Blood 77 (8), 1627–1652. doi:10.1182/blood.v77.8.1627.1627
Dinarello, C. A. (1991b). The Proinflammatory Cytokines Interleukin-1 and Tumor Necrosis Factor and Treatment of the Septic Shock Syndrome. J. Infect. Dis. 163 (6), 1177–1184. doi:10.1093/infdis/163.6.1177
Evans, L., Rhodes, A., Alhazzani, W., Antonelli, M., Coopersmith, C. M., French, C., et al. (2021). Surviving Sepsis Campaign: International Guidelines for Management of Sepsis and Septic Shock 2021. Crit. Care Med. 49 (11), e1063–e1143. doi:10.1097/CCM.0000000000005337
Fajgenbaum, D. C., and June, C. H. (2020). Cytokine Storm. N. Engl. J. Med. 383 (23), 2255–2273. doi:10.1056/NEJMra2026131
Fein, A. M., Bernard, G. R., Criner, G. J., Fletcher, E. C., Good, J. T., Knaus, W. A., et al. (1997). Treatment of Severe Systemic Inflammatory Response Syndrome and Sepsis with a Novel Bradykinin Antagonist, Deltibant (CP-0127). Results of a Randomized, Double-Blind, Placebo-Controlled Trial. CP-0127 SIRS and Sepsis Study Group. JAMA 277 (6), 482–487. doi:10.1001/jama.277.6.482
Ferrante, A. (1992). Activation of Neutrophils by Interleukins-1 and -2 and Tumor Necrosis Factors. Immunol. Ser. 57, 417–436.
Ferrara, J. L., Abhyankar, S., and Gilliland, D. G. (1993). Cytokine Storm of Graft-Versus-Host Disease: a Critical Effector Role for Interleukin-1. Transpl. Proc. 25 (1 Pt 2), 1216–1217.
Fisher, C. J., Agosti, J. M., Opal, S. M., Lowry, S. F., Balk, R. A., Sadoff, J. C., et al. (1996). Treatment of Septic Shock with the Tumor Necrosis Factor receptor:Fc Fusion Protein. The Soluble TNF Receptor Sepsis Study Group. N. Engl. J. Med. 334 (26), 1697–1702. doi:10.1056/NEJM199606273342603
Fisher, C. J., Dhainaut, J. F., Opal, S. M., Pribble, J. P., Balk, R. A., Slotman, G. J., et al. (1994a). Recombinant Human Interleukin 1 Receptor Antagonist in the Treatment of Patients with Sepsis Syndrome. Results from a Randomized, Double-Blind, Placebo-Controlled Trial. Phase III rhIL-1ra Sepsis Syndrome Study Group. JAMA 271 (23), 1836–1843. doi:10.1001/jama.271.23.1836
Fisher, C. J., Opal, S. M., Dhainaut, J. F., Stephens, S., Zimmerman, J. L., Nightingale, P., et al. (1993). Influence of an Anti-tumor Necrosis Factor Monoclonal Antibody on Cytokine Levels in Patients with Sepsis. The CB0006 Sepsis Syndrome Study Group. Crit. Care Med. 21 (3), 318–327. doi:10.1097/00003246-199303000-00006
Fisher, C. J., Slotman, G. J., Opal, S. M., Pribble, J. P., Bone, R. C., Emmanuel, G., et al. (1994b). Initial Evaluation of Human Recombinant Interleukin-1 Receptor Antagonist in the Treatment of Sepsis Syndrome: a Randomized, Open-Label, Placebo-Controlled Multicenter Trial. Crit. Care Med. 22 (1), 12–21. doi:10.1097/00003246-199401000-00008
Fong, Y., Tracey, K. J., Moldawer, L. L., Hesse, D. G., Manogue, K. B., Kenney, J. S., et al. (1989). Antibodies to Cachectin/tumor Necrosis Factor Reduce Interleukin 1 Beta and Interleukin 6 Appearance during Lethal Bacteremia. J. Exp. Med. 170 (5), 1627–1633. doi:10.1084/jem.170.5.1627
Franklin, A. (2015). The Theory-Ladenness of Experiment. J. Gen. Philos. Sci. 46 (1), 155–166. doi:10.1007/510838-015-9285-910.1007/s10838-015-9285-9
Fujii, T., Luethi, N., Young, P. J., Frei, D. R., Eastwood, G. M., French, C. J., et al. (2020). Effect of Vitamin C, Hydrocortisone, and Thiamine vs Hydrocortisone Alone on Time Alive and Free of Vasopressor Support Among Patients with Septic Shock: The VITAMINS Randomized Clinical Trial. JAMA 323 (5), 423–431. doi:10.1001/jama.2019.22176
Gando, S., Saitoh, D., Ishikura, H., Ueyama, M., Otomo, Y., Oda, S., et al. (2013). A Randomized, Controlled, Multicenter Trial of the Effects of Antithrombin on Disseminated Intravascular Coagulation in Patients with Sepsis. Crit. Care 17 (6), R297. doi:10.1186/cc13163
Gao, H., Cao, M., Chen, P., Cooper, D. K. C., Zhao, Y., Wei, L., et al. (2019). TNF-α Promotes Human Antibody-Mediated Complement-dependent Cytotoxicity of Porcine Endothelial Cells through Downregulating P38-Mediated Occludin Expression. Cell. Commun. Signal 17 (1), 75. doi:10.1186/s12964-019-0386-7
Gharamti, A., Samara, O., Monzon, A., Scherger, S., DeSanto, K., Sillau, S., et al. (2021). Association between Cytokine Levels, Sepsis Severity and Clinical Outcomes in Sepsis: a Quantitative Systematic Review Protocol. BMJ Open 11 (8), e048476. doi:10.1136/bmjopen-2020-048476
Gordon, A. C., Mason, A. J., Thirunavukkarasu, N., Perkins, G. D., Cecconi, M., Cepkova, M., et al. (2016). Effect of Early Vasopressin vs Norepinephrine on Kidney Failure in Patients with Septic Shock: The VANISH Randomized Clinical Trial. JAMA 316 (5), 509–518. doi:10.1001/jama.2016.10485
Greenman, R. L., Schein, R. M., Martin, M. A., Wenzel, R. P., MacIntyre, N. R., Emmanuel, G., et al. (1991). A Controlled Clinical Trial of E5 Murine Monoclonal IgM Antibody to Endotoxin in the Treatment of Gram-Negative Sepsis. The XOMA Sepsis Study Group. JAMA 266 (8), 1097–1102. doi:10.1001/jama.266.8.1097
Groopman, J. (2015). Inflammed, the Debate over the Latest Cure-All Craze. The New Yorker.http://www.newyorker.com/magazine/2015/11/30/inflamed (Accessed November 30, 2015).1–13.
Gu, X., Zhou, F., Wang, Y., Fan, G., and Cao, B. (2020). Respiratory Viral Sepsis: Epidemiology, Pathophysiology, Diagnosis and Treatment. Eur. Respir. Rev. 29 (157), 200038. doi:10.1183/16000617.0038-2020
Gül, F., Arslantaş, M. K., Cinel, İ., and Kumar, A. (2017). Changing Definitions of Sepsis. Turk J. Anaesthesiol. Reanim. 45 (3), 129–138. doi:10.5152/TJAR.2017.93753
Haupt, M. T., Jastremski, M. S., Clemmer, T. P., Metz, C. A., and Goris, G. B. (1991). Effect of Ibuprofen in Patients with Severe Sepsis: a Randomized, Double-Blind, Multicenter Study. The Ibuprofen Study Group. Crit. Care Med. 19 (11), 1339–1347. doi:10.1097/00003246-199111000-00006
Herr, C., Mang, S., Mozafari, B., Guenther, K., Speer, T., Seibert, M., et al. (2021). Distinct Patterns of Blood Cytokines beyond a Cytokine Storm Predict Mortality in COVID-19. J. Inflamm. Res. 14, 4651–4667. doi:10.2147/JIR.S320685
RECOVERY Collaborative Group (2021). Dexamethasone in Hospitalized Patients with Covid-19. N. Engl. J. Med. 384 (8), 693–704. doi:10.1056/NEJMoa2021436
Hotchkiss, R. S., and Karl, I. E. (2003). The Pathophysiology and Treatment of Sepsis. N. Engl. J. Med. 348 (2), 138–150. doi:10.1056/NEJMra021333
Hróbjartsson, A., Thomsen, A. S., Emanuelsson, F., Tendal, B., Hilden, J., Boutron, I., et al. (2012). Observer Bias in Randomised Clinical Trials with Binary Outcomes: Systematic Review of Trials with Both Blinded and Non-blinded Outcome Assessors. BMJ 344, e1119. doi:10.1136/bmj.e1119
Huang, C., Wang, Y., Li, X., Ren, L., Zhao, J., Hu, Y., et al. (2020). Clinical Features of Patients Infected with 2019 Novel Coronavirus in Wuhan, China. Lancet 395 (10223), 497–506. doi:10.1016/S0140-6736(20)30183-5
Iwagami, M., Yasunaga, H., Doi, K., Horiguchi, H., Fushimi, K., Matsubara, T., et al. (2014). Postoperative Polymyxin B Hemoperfusion and Mortality in Patients with Abdominal Septic Shock: a Propensity-Matched Analysis. Crit. Care Med. 42 (5), 1187–1193. doi:10.1097/CCM.0000000000000150
Jaimes, F., De La Rosa, G., Morales, C., Fortich, F., Arango, C., Aguirre, D., et al. (2009). Unfractioned Heparin for Treatment of Sepsis: A Randomized Clinical Trial (The HETRASE Study). Crit. Care Med. 37 (4), 1185–1196. doi:10.1097/CCM.0b013e31819c06bc
Jarczak, D., Kluge, S., and Nierhaus, A. (2021). Sepsis-Pathophysiology and Therapeutic Concepts. Front. Med. (Lausanne) 8, 628302. doi:10.3389/fmed.2021.628302
Jeronimo, C. M. P., Farias, M. E. L., Val, F. F. A., Sampaio, V. S., Alexandre, M. A. A., Melo, G. C., et al. (2021). Methylprednisolone as Adjunctive Therapy for Patients Hospitalized with Coronavirus Disease 2019 (COVID-19; Metcovid): A Randomized, Double-Blind, Phase IIb, Placebo-Controlled Trial. Clin. Infect. Dis. 72 (9), e373–e381. doi:10.1093/cid/ciaa1177
Joannes-Boyau, O., Honoré, P. M., Perez, P., Bagshaw, S. M., Grand, H., Canivet, J. L., et al. (2013). High-volume versus Standard-Volume Haemofiltration for Septic Shock Patients with Acute Kidney Injury (IVOIRE Study): a Multicentre Randomized Controlled Trial. Intensive Care Med. 39 (9), 1535–1546. doi:10.1007/s00134-013-2967-z
Keh, D., Trips, E., Marx, G., Wirtz, S. P., Abduljawwad, E., Bercker, S., et al. (2016). Effect of Hydrocortisone on Development of Shock Among Patients with Severe Sepsis: The HYPRESS Randomized Clinical Trial. JAMA 316 (17), 1775–1785. doi:10.1001/jama.2016.14799
Keshari, R. S., Jyoti, A., Dubey, M., Kothari, N., Kohli, M., Bogra, J., et al. (2012). Cytokines Induced Neutrophil Extracellular Traps Formation: Implication for the Inflammatory Disease Condition. PLoS One 7 (10), e48111. doi:10.1371/journal.pone.0048111
Kindi, V., and Arabatzis, T. (2012). Kuhn’s the Structiure of Scientific Revolutions Revisited. Ney York, NY: Routledge.
Kor, D. J., Carter, R. E., Park, P. K., Festic, E., Banner-Goodspeed, V. M., Hinds, R., et al. (2016). Effect of Aspirin on Development of ARDS in At-Risk Patients Presenting to the Emergency Department: The LIPS-A Randomized Clinical Trial. JAMA 315 (22), 2406–2414. doi:10.1001/jama.2016.6330
Kruger, P., Bailey, M., Bellomo, R., Cooper, D. J., Harward, M., Higgins, A., et al. (2013). A Multicenter Randomized Trial of Atorvastatin Therapy in Intensive Care Patients with Severe Sepsis. Am. J. Respir. Crit. Care Med. 187 (7), 743–750. doi:10.1164/rccm.201209-1718OC
Kushner, I. (1998). Semantics, Inflammation, Cytokines and Common Sense. Cytokine Growth Factor Rev. 9 (3-4), 191–196. doi:10.1016/s1359-6101(98)00016-1
Lakatos, I., and Musgrave, A. (1970). Criticism and the Growth of Knowledge. Cambridge, United Kingdom: Cambridge University Press.
Lamontagne, F., Agoritsas, T., Macdonald, H., Leo, Y. S., Diaz, J., Agarwal, A., et al. (2020). A Living WHO Guideline on Drugs for Covid-19. BMJ 370, m3379. doi:10.1136/bmj.m3379
Lehmann, V., Freudenberg, M. A., and Galanos, C. (1987). Lethal Toxicity of Lipopolysaccharide and Tumor Necrosis Factor in Normal and D-Galactosamine-Treated Mice. J. Exp. Med. 165 (3), 657–663. doi:10.1084/jem.165.3.657
Levi, M., Levy, M., Williams, M. D., Douglas, I., Artigas, A., Antonelli, M., et al. (2007). Prophylactic Heparin in Patients with Severe Sepsis Treated with Drotrecogin Alfa (Activated). Am. J. Respir. Crit. Care Med. 176 (5), 483–490. doi:10.1164/rccm.200612-1803OC
Levin, M., Quint, P. A., Goldstein, B., Barton, P., Bradley, J. S., Shemie, S. D., et al. (2000). Recombinant Bactericidal/permeability-Increasing Protein (rBPI21) as Adjunctive Treatment for Children with Severe Meningococcal Sepsis: a Randomised Trial. rBPI21 Meningococcal Sepsis Study Group. Lancet 356 (9234), 961–967. doi:10.1016/s0140-6736(00)02712-4
Liang, H., Song, H., Zhai, R., Song, G., Li, H., Ding, X., et al. (2021). Corticosteroids for Treating Sepsis in Adult Patients: A Systematic Review and Meta-Analysis. Front. Immunol. 12, 709155. doi:10.3389/fimmu.2021.709155
Lin, G. L., McGinley, J. P., Drysdale, S. B., and Pollard, A. J. (2018). Epidemiology and Immune Pathogenesis of Viral Sepsis. Front. Immunol. 9, 2147. doi:10.3389/fimmu.2018.02147
Livigni, S., Bertolini, G., Rossi, C., Ferrari, F., Giardino, M., Pozzato, M., et al. (2014). Efficacy of Coupled Plasma Filtration Adsorption (CPFA) in Patients with Septic Shock: a Multicenter Randomised Controlled Clinical Trial. BMJ Open 4 (1), e003536. doi:10.1136/bmjopen-2013-003536
López, A., Lorente, J. A., Steingrub, J., Bakker, J., McLuckie, A., Willatts, S., et al. (2004). Multiple-center, Randomized, Placebo-Controlled, Double-Blind Study of the Nitric Oxide Synthase Inhibitor 546C88: Effect on Survival in Patients with Septic Shock. Crit. Care Med. 32 (1), 21–30. doi:10.1097/01.CCM.0000105581.01815.C6
Marik, P. E., Khangoora, V., Rivera, R., Hooper, M. H., and Catravas, J. (2017). Hydrocortisone, Vitamin C, and Thiamine for the Treatment of Severe Sepsis and Septic Shock: A Retrospective Before-After Study. Chest 151 (6), 1229–1238. doi:10.1016/j.chest.2016.11.036
Marshall, J. C. (2014). Why Have Clinical Trials in Sepsis Failed? Trends Mol. Med. 20 (4), 195–203. doi:10.1016/j.molmed.2014.01.007
Martin, G. S., Mannino, D. M., Eaton, S., and Moss, M. (2003). The Epidemiology of Sepsis in the United States from 1979 through 2000. N. Engl. J. Med. 348 (16), 1546–1554. doi:10.1056/NEJMoa022139
Mathison, J. C., Wolfson, E., and Ulevitch, R. J. (1988). Participation of Tumor Necrosis Factor in the Mediation of Gram Negative Bacterial Lipopolysaccharide-Induced Injury in Rabbits. J. Clin. Invest. 81 (6), 1925–1937. doi:10.1172/JCI113540
Mayr, F. B., Yende, S., and Angus, D. C. (2014). Epidemiology of Severe Sepsis. Virulence 5 (1), 4–11. doi:10.4161/viru.27372
McAuley, D. F., Laffey, J. G., O'Kane, C. M., Perkins, G. D., Mullan, B., Trinder, T. J., et al. (2014). Simvastatin in the Acute Respiratory Distress Syndrome. N. Engl. J. Med. 371 (18), 1695–1703. doi:10.1056/NEJMoa1403285
McCloskey, R. V., Straube, R. C., Sanders, C., Smith, S. M., and Smith, C. R. (1994). Treatment of Septic Shock with Human Monoclonal Antibody HA-1A. A Randomized, Double-Blind, Placebo-Controlled Trial. CHESS Trial Study Group. Ann. Intern Med. 121 (1), 1–5. doi:10.7326/0003-4819-121-1-199407010-00001
Michalek, S. M., Moore, R. N., McGhee, J. R., Rosenstreich, D. L., and Mergenhagen, S. E. (1980). The Primary Role of Lymphoreticular Cells in the Mediation of Host Responses to Bacterial Endotoxim. J. Infect. Dis. 141 (1), 55–63. doi:10.1093/infdis/141.1.55
Miller, M. C., and Yunes, N. (2019). The New Frontier of Gravitational Waves. Nature 568 (7753), 469–476. doi:10.1038/s41586-019-1129-z
Mitchell, A. B., Ryan, T. E., Gillion, A. R., Wells, L. D., and Muthiah, M. P. (2020). Vitamin C and Thiamine for Sepsis and Septic Shock. Am. J. Med. 133 (5), 635–638. doi:10.1016/j.amjmed.2019.07.054
Mohler, K. M., Torrance, D. S., Smith, C. A., Goodwin, R. G., Stremler, K. E., Fung, V. P., et al. (1993). Soluble Tumor Necrosis Factor (TNF) Receptors Are Effective Therapeutic Agents in Lethal Endotoxemia and Function Simultaneously as Both TNF Carriers and TNF Antagonists. J. Immunol. 151 (3), 1548–1561.
Mudd, P. A., Crawford, J. C., Turner, J. S., Souquette, A., Reynolds, D., Bender, D., et al. (2020). Distinct Inflammatory Profiles Distinguish COVID-19 from Influenza with Limited Contributions from Cytokine Storm. Sci. Adv. 6 (50). doi:10.1126/sciadv.abe3024
Mullen, P. G., Fisher, B. J., Walsh, C. J., Susskind, B. M., Leeper-Woodford, S. K., Jesmok, G. J., et al. (1994). Monoclonal Antibody to Tumor Necrosis Factor-Alpha Attenuates Plasma Interleukin-6 Levels in Porcine Gram-Negative Sepsis. J. Surg. Res. 57 (5), 625–631. doi:10.1006/jsre.1994.1192
Nadel, S., Goldstein, B., Williams, M. D., Dalton, H., Peters, M., Macias, W. L., et al. (2007). Drotrecogin Alfa (Activated) in Children with Severe Sepsis: a Multicentre Phase III Randomised Controlled Trial. Lancet 369 (9564), 836–843. doi:10.1016/S0140-6736(07)60411-5
Ohlsson, K., Björk, P., Bergenfeldt, M., Hageman, R., and Thompson, R. C. (1990). Interleukin-1 Receptor Antagonist Reduces Mortality from Endotoxin Shock. Nature 348 (6301), 550–552. doi:10.1038/348550a0
Opal, S., Laterre, P. F., Abraham, E., Francois, B., Wittebole, X., Lowry, S., et al. (2004). Recombinant Human Platelet-Activating Factor Acetylhydrolase for Treatment of Severe Sepsis: Results of a Phase III, Multicenter, Randomized, Double-Blind, Placebo-Controlled, Clinical Trial. Crit. Care Med. 32 (2), 332–341. doi:10.1097/01.CCM.0000108867.87890.6D
Opal, S. M., Dellinger, R. P., Vincent, J. L., Masur, H., and Angus, D. C. (2014). The Next Generation of Sepsis Clinical Trial Designs: what Is Next after the Demise of Recombinant Human Activated Protein C?*. Crit. Care Med. 42 (7), 1714–1721. doi:10.1097/CCM.0000000000000325
Opal, S. M., Fisher, C. J., Dhainaut, J. F., Vincent, J. L., Brase, R., Lowry, S. F., et al. (1997). Confirmatory Interleukin-1 Receptor Antagonist Trial in Severe Sepsis: a Phase III, Randomized, Double-Blind, Placebo-Controlled, Multicenter Trial. The Interleukin-1 Receptor Antagonist Sepsis Investigator Group. Crit. Care Med. 25 (7), 1115–1124. doi:10.1097/00003246-199707000-00010
Opal, S. M., Laterre, P. F., Francois, B., LaRosa, S. P., Angus, D. C., Mira, J. P., et al. (2013). Effect of Eritoran, an Antagonist of MD2-TLR4, on Mortality in Patients with Severe Sepsis: the ACCESS Randomized Trial. JAMA 309 (11), 1154–1162. doi:10.1001/jama.2013.2194
Orman, T. F. (2016). “Paradigm” as a Central Concept in Thomas Kuhn’s Thought. Int. J. Humanit. Soc. Sceinces 6 (10), 47–52.
Page, M. J., Bester, J., and Pretorius, E. (2018). The Inflammatory Effects of TNF-α and Complement Component 3 on Coagulation. Sci. Rep. 8 (1), 1812. doi:10.1038/s41598-018-20220-8
Panacek, E. A., Marshall, J. C., Albertson, T. E., Johnson, D. H., Johnson, S., MacArthur, R. D., et al. (2004). Efficacy and Safety of the Monoclonal Anti-tumor Necrosis Factor Antibody F(ab')2 Fragment Afelimomab in Patients with Severe Sepsis and Elevated Interleukin-6 Levels. Crit. Care Med. 32 (11), 2173–2182. doi:10.1097/01.ccm.0000145229.59014.6c
Parameswaran, N., and Patial, S. (2010). Tumor Necrosis Factor-α Signaling in Macrophages. Crit. Rev. Eukaryot. Gene Expr. 20 (2), 87–103. doi:10.1615/critreveukargeneexpr.v20.i2.10
Payen, D., Mateo, J., Cavaillon, J. M., Fraisse, F., Floriot, C., Vicaut, E., et al. (2009). Impact of Continuous Venovenous Hemofiltration on Organ Failure during the Early Phase of Severe Sepsis: a Randomized Controlled Trial. Crit. Care Med. 37 (3), 803–810. doi:10.1097/CCM.0b013e3181962316
Payen, D. M., Guilhot, J., Launey, Y., Lukaszewicz, A. C., Kaaki, M., Veber, B., et al. (2015). Early Use of Polymyxin B Hemoperfusion in Patients with Septic Shock Due to Peritonitis: a Multicenter Randomized Control Trial. Intensive Care Med. 41 (6), 975–984. doi:10.1007/s00134-015-3751-z
Poeze, M., Froon, A. H., Ramsay, G., Buurman, W. A., and Greve, J. W. (2000). Decreased Organ Failure in Patients with Severe SIRS and Septic Shock Treated with the Platelet-Activating Factor Antagonist TCV-309: a Prospective, Multicenter, Double-Blind, Randomized Phase II Trial. TCV-309 Septic Shock Study Group. Shock 14 (4), 421–428. doi:10.1097/00024382-200014040-00001
Quinn, M., Moody, C., Tunnicliffe, B., Khan, Z., Manji, M., Gudibande, S., et al. (2016). Systematic Review of Statins in Sepsis: There Is No Evidence of Dose Response. Indian J. Crit. Care Med. 20 (9), 534–541. doi:10.4103/0972-5229.190366
Qureshi, S. T., Larivière, L., Leveque, G., Clermont, S., Moore, K. J., Gros, P., et al. (1999). Endotoxin-tolerant Mice Have Mutations in Toll-like Receptor 4 (Tlr4). J. Exp. Med. 189 (4), 615–625. doi:10.1084/jem.189.4.615
Raetz, C. R., and Whitfield, C. (2002). Lipopolysaccharide Endotoxins. Annu. Rev. Biochem. 71, 635–700. doi:10.1146/annurev.biochem.71.110601.135414
Ranieri, V. M., Thompson, B. T., Barie, P. S., Dhainaut, J. F., Douglas, I. S., Finfer, S., et al. (2012). Drotrecogin Alfa (Activated) in Adults with Septic Shock. N. Engl. J. Med. 366 (22), 2055–2064. doi:10.1056/NEJMoa1202290
Reinhart, K., Daniels, R., Kissoon, N., Machado, F. R., Schachter, R. D., and Finfer, S. (2017). Recognizing Sepsis as a Global Health Priority - A WHO Resolution. N. Engl. J. Med. 377 (5), 414–417. doi:10.1056/NEJMp1707170
Reinhart, K., Menges, T., Gardlund, B., Harm Zwaveling, J., Smithes, M., Vincent, J. L., et al. (2001). Randomized, Placebo-Controlled Trial of the Anti-tumor Necrosis Factor Antibody Fragment Afelimomab in Hyperinflammatory Response during Severe Sepsis: The RAMSES Study. Crit. Care Med. 29 (4), 765–769. doi:10.1097/00003246-200104000-00015
Reinhart, K., Wiegand-Löhnert, C., Grimminger, F., Kaul, M., Withington, S., Treacher, D., et al. (1996). Assessment of the Safety and Efficacy of the Monoclonal Anti-tumor Necrosis Factor Antibody-Fragment, MAK 195F, in Patients with Sepsis and Septic Shock: a Multicenter, Randomized, Placebo-Controlled, Dose-Ranging Study. Crit. Care Med. 24 (5), 733–742. doi:10.1097/00003246-199605000-00003
Remick, D., Manohar, P., Bolgos, G., Rodriguez, J., Moldawer, L., and Wollenberg, G. (1995). Blockade of Tumor Necrosis Factor Reduces Lipopolysaccharide Lethality, but Not the Lethality of Cecal Ligation and Puncture. Shock 4 (2), 89–95. doi:10.1097/00024382-199508000-00002
Rice, T. W., Wheeler, A. P., Bernard, G. R., Vincent, J. L., Angus, D. C., Aikawa, N., et al. (2010). A Randomized, Double-Blind, Placebo-Controlled Trial of TAK-242 for the Treatment of Severe Sepsis. Crit. Care Med. 38 (8), 1685–1694. doi:10.1097/CCM.0b013e3181e7c5c9
Rice, T. W., Wheeler, A. P., Morris, P. E., Paz, H. L., Russell, J. A., Edens, T. R., et al. (2006). Safety and Efficacy of Affinity-Purified, Anti-tumor Necrosis Factor-Alpha, Ovine Fab for Injection (CytoFab) in Severe Sepsis. Crit. Care Med. 34 (9), 2271–2281. doi:10.1097/01.CCM.0000230385.82679.34
Rimmer, E., Houston, B. L., Kumar, A., Abou-Setta, A. M., Friesen, C., Marshall, J. C., et al. (2014). The Efficacy and Safety of Plasma Exchange in Patients with Sepsis and Septic Shock: a Systematic Review and Meta-Analysis. Crit. Care 18 (6), 699. doi:10.1186/s13054-014-0699-2
Rittirsch, D., Hoesel, L. M., and Ward, P. A. (2007). The Disconnect between Animal Models of Sepsis and Human Sepsis. J. Leukoc. Biol. 81 (1), 137–143. doi:10.1189/jlb.0806542
Roberts, A. H., Kewman, D. G., Mercier, L., and Hovell, M. (1993). The Power of Nonspecific Effects in Healing: Implications for Psychosocial and Biological Treatments. Clin. Psychol. Rev. 13 (5), 275–391. doi:10.1016/0272-7358(93)90010-j
Rochwerg, B., Oczkowski, S. J., Siemieniuk, R. A. C., Agoritsas, T., Belley-Cote, E., D'Aragon, F., et al. (2018). Corticosteroids in Sepsis: An Updated Systematic Review and Meta-Analysis. Crit. Care Med. 46 (9), 1411–1420. doi:10.1097/CCM.0000000000003262
Rudd, K. E., Johnson, S. C., Agesa, K. M., Shackelford, K. A., Tsoi, D., Kievlan, D. R., et al. (2020). Global, Regional, and National Sepsis Incidence and Mortality, 1990-2017: Analysis for the Global Burden of Disease Study. Lancet 395 (10219), 200–211. doi:10.1016/S0140-6736(19)32989-7
Saltaji, H., Armijo-Olivo, S., Cummings, G. G., Amin, M., da Costa, B. R., and Flores-Mir, C. (2018). Influence of Blinding on Treatment Effect Size Estimate in Randomized Controlled Trials of Oral Health Interventions. BMC Med. Res. Methodol. 18 (1), 42. doi:10.1186/s12874-018-0491-0
Schädler, D., Pausch, C., Heise, D., Meier-Hellmann, A., Brederlau, J., Weiler, N., et al. (2017). The Effect of a Novel Extracorporeal Cytokine Hemoadsorption Device on IL-6 Elimination in Septic Patients: A Randomized Controlled Trial. PLoS One 12 (10), e0187015. doi:10.1371/journal.pone.0187015
Scherger, S., Henao-Martínez, A., Franco-Paredes, C., and Shapiro, L. (2020). Rethinking Interleukin-6 Blockade for Treatment of COVID-19. Med. Hypotheses 144, 110053. doi:10.1016/j.mehy.2020.110053
Schindler, R., Mancilla, J., Endres, S., Ghorbani, R., Clark, S. C., and Dinarello, C. A. (1990). Correlations and Interactions in the Production of Interleukin-6 (IL-6), IL-1, and Tumor Necrosis Factor (TNF) in Human Blood Mononuclear Cells: IL-6 Suppresses IL-1 and TNF. Blood 75 (1), 40–47. doi:10.1182/blood.v75.1.40.bloodjournal75140
Schulz, K. F., Chalmers, I., Hayes, R. J., and Altman, D. G. (1995). Empirical Evidence of Bias. Dimensions of Methodological Quality Associated with Estimates of Treatment Effects in Controlled Trials. JAMA 273 (5), 408–412. doi:10.1001/jama.273.5.408
Shakoory, B., Carcillo, J. A., Chatham, W. W., Amdur, R. L., Zhao, H., Dinarello, C. A., et al. (2016). Interleukin-1 Receptor Blockade Is Associated with Reduced Mortality in Sepsis Patients with Features of Macrophage Activation Syndrome: Reanalysis of a Prior Phase III Trial. Crit. Care Med. 44 (2), 275–281. doi:10.1097/CCM.0000000000001402
WHO Rapid Evidence Appraisal for COVID-19 Therapies (REACT) Working Group (2021). Association between Administration of IL-6 Antagonists and Mortality Among Patients Hospitalized for COVID-19: A Meta-Analysis. JAMA 326 (6), 499–518. doi:10.1001/jama.2021.11330
Singer, M., Deutschman, C. S., Seymour, C. W., Shankar-Hari, M., Annane, D., Bauer, M., et al. (2016). The Third International Consensus Definitions for Sepsis and Septic Shock (Sepsis-3). JAMA 315 (8), 801–810. doi:10.1001/jama.2016.0287
Smart, S. J., and Casale, T. B. (1994). TNF-alpha-induced Transendothelial Neutrophil Migration Is IL-8 Dependent. Am. J. Physiol. 266 (3 Pt 1), L238–L245. doi:10.1152/ajplung.1994.266.3.L238
Spink, W. W., and Anderson, D. (1954). Experimental Studies on the Significance of Endotoxin in the Pathogenesis of Brucellosis. J. Clin. Invest. 33 (4), 540–548. doi:10.1172/JCI102924
Spooner, C. E., Markowitz, N. P., and Saravolatz, L. D. (1992). The Role of Tumor Necrosis Factor in Sepsis. Clin. Immunol. Immunopathol. 62 (1 Pt 2), S11–S17. doi:10.1016/0090-1229(92)90036-n
Sprung, C. L., Annane, D., Keh, D., Moreno, R., Singer, M., Freivogel, K., et al. (2008). Hydrocortisone Therapy for Patients with Septic Shock. N. Engl. J. Med. 358 (2), 111–124. doi:10.1056/NEJMoa071366
Sprung, C. L., Caralis, P. V., Marcial, E. H., Pierce, M., Gelbard, M. A., Long, W. M., et al. (1984). The Effects of High-Dose Corticosteroids in Patients with Septic Shock. A Prospective, Controlled Study. N. Engl. J. Med. 311 (18), 1137–1143. doi:10.1056/NEJM198411013111801
Starnes, H. F., Warren, R. S., Jeevanandam, M., Gabrilove, J. L., Larchian, W., Oettgen, H. F., et al. (1988). Tumor Necrosis Factor and the Acute Metabolic Response to Tissue Injury in Man. J. Clin. Invest. 82 (4), 1321–1325. doi:10.1172/JCI113733
Sultzer, B. M. (1972). Genetic Control of Host Responses to Endotoxin. Infect. Immun. 5 (1), 107–113. doi:10.1128/iai.5.1.107-113.1972
Suputtamongkol, Y., Intaranongpai, S., Smith, M. D., Angus, B., Chaowagul, W., Permpikul, C., et al. (2000). A Double-Blind Placebo-Controlled Study of an Infusion of Lexipafant (Platelet-Activating Factor Receptor Antagonist) in Patients with Severe Sepsis. Antimicrob. Agents Chemother. 44 (3), 693–696. doi:10.1128/AAC.44.3.693-696.2000
Talisa, V. B., Yende, S., Seymour, C. W., and Angus, D. C. (2018). Arguing for Adaptive Clinical Trials in Sepsis. Front. Immunol. 9, 1502. doi:10.3389/fimmu.2018.01502
Tharmarajah, E., Buazon, A., Patel, V., Hannah, J. R., Adas, M., Allen, V. B., et al. (2021). IL-6 Inhibition in the Treatment of COVID-19: A Meta-Analysis and Meta-Regression. J. Infect. 82 (5), 178–185. doi:10.1016/j.jinf.2021.03.008
Thomas, G., Hraiech, S., Loundou, A., Truwit, J., Kruger, P., McAuley, D. F., et al. (2015). Statin Therapy in Critically-Ill Patients with Severe Sepsis: a Review and Meta-Analysis of Randomized Clinical Trials. Minerva Anestesiol. 81 (8), 921–930.
Tjan, L. H., Furukawa, K., Nagano, T., Kiriu, T., Nishimura, M., Arii, J., et al. (2021). Early Differences in Cytokine Production by Severity of Coronavirus Disease 2019. J. Infect. Dis. 223 (7), 1145–1149. doi:10.1093/infdis/jiab005
Tracey, K. J., Beutler, B., Lowry, S. F., Merryweather, J., Wolpe, S., Milsark, I. W., et al. (1986). Shock and Tissue Injury Induced by Recombinant Human Cachectin. Science 234 (4775), 470–474. doi:10.1126/science.3764421
Tracey, K. J., Fong, Y., Hesse, D. G., Manogue, K. R., Lee, A. T., Kuo, G. C., et al. (1987). Anti-cachectin/TNF Monoclonal Antibodies Prevent Septic Shock during Lethal Bacteraemia. Nature 330 (6149), 662–664. doi:10.1038/330662a0
Tsirigotis, P., Chondropoulos, S., Gkirkas, K., Meletiadis, J., and Dimopoulou, I. (2016). Balanced Control of Both Hyper and Hypo-Inflammatory Phases as a New Treatment Paradigm in Sepsis. J. Thorac. Dis. 8 (5), E312–E316. doi:10.21037/jtd.2016.03.47
Turner, M. D., Nedjai, B., Hurst, T., and Pennington, D. J. (2014). Cytokines and Chemokines: At the Crossroads of Cell Signalling and Inflammatory Disease. Biochim. Biophys. Acta 1843 (11), 2563–2582. doi:10.1016/j.bbamcr.2014.05.014
van der Poll, T., Büller, H. R., ten Cate, H., Wortel, C. H., Bauer, K. A., van Deventer, S. J., et al. (1990). Activation of Coagulation after Administration of Tumor Necrosis Factor to Normal Subjects. N. Engl. J. Med. 322 (23), 1622–1627. doi:10.1056/NEJM199006073222302
Venkatesh, B., Finfer, S., Cohen, J., Rajbhandari, D., Arabi, Y., Bellomo, R., et al. (2018). Adjunctive Glucocorticoid Therapy in Patients with Septic Shock. N. Engl. J. Med. 378 (9), 797–808. doi:10.1056/NEJMoa1705835
Veterans Administration Systemic Sepsis Cooperative Study Group (1987). Effect of High-Dose Glucocorticoid Therapy on Mortality in Patients with Clinical Signs of Systemic Sepsis. N. Engl. J. Med. 317 (11), 659–665. doi:10.1056/NEJM198709103171102
Vieira, S. M., Lemos, H. P., Grespan, R., Napimoga, M. H., Dal-Secco, D., Freitas, A., et al. (2009). A Crucial Role for TNF-Alpha in Mediating Neutrophil Influx Induced by Endogenously Generated or Exogenous Chemokines, KC/CXCL1 and LIX/CXCL5. Br. J. Pharmacol. 158 (3), 779–789. doi:10.1111/j.1476-5381.2009.00367.x
Vincent, J. L., and Abraham, E. (2006). The Last 100 Years of Sepsis. Am. J. Respir. Crit. Care Med. 173 (3), 256–263. doi:10.1164/rccm.200510-1604OE
Vincent, J. L., Francois, B., Zabolotskikh, I., Daga, M. K., Lascarrou, J. B., Kirov, M. Y., et al. (2019). Effect of a Recombinant Human Soluble Thrombomodulin on Mortality in Patients with Sepsis-Associated Coagulopathy: The SCARLET Randomized Clinical Trial. JAMA 321 (20), 1993–2002. doi:10.1001/jama.2019.5358
Vincent, J. L., Ramesh, M. K., Ernest, D., LaRosa, S. P., Pachl, J., Aikawa, N., et al. (2013). A Randomized, Double-Blind, Placebo-Controlled, Phase 2b Study to Evaluate the Safety and Efficacy of Recombinant Human Soluble Thrombomodulin, ART-123, in Patients with Sepsis and Suspected Disseminated Intravascular Coagulation. Crit. Care Med. 41 (9), 2069–2079. doi:10.1097/CCM.0b013e31828e9b03
Vincent, J. L., Spapen, H., Bakker, J., Webster, N. R., and Curtis, L. (2000). Phase II Multicenter Clinical Study of the Platelet-Activating Factor Receptor Antagonist BB-882 in the Treatment of Sepsis. Crit. Care Med. 28 (3), 638–642. doi:10.1097/00003246-200003000-00006
Warren, B. L., Eid, A., Singer, P., Pillay, S. S., Carl, P., Novak, I., et al. (2001). Caring for the Critically Ill Patient. High-Dose Antithrombin III in Severe Sepsis: a Randomized Controlled Trial. JAMA 286 (15), 1869–1878. doi:10.1001/jama.286.15.1869
Weissmann, G. (2010). It's Complicated: Inflammation from Metchnikoff to Meryl Streep. FASEB J. 24 (11), 4129–4132. doi:10.1096/fj.10-1101ufm
Woodward, J. F. (2003). Making Things Happen : A Theory of Causal Explanation. New York: Oxford University Press.
Yamakawa, K., Aihara, M., Ogura, H., Yuhara, H., Hamasaki, T., and Shimazu, T. (2015). Recombinant Human Soluble Thrombomodulin in Severe Sepsis: a Systematic Review and Meta-Analysis. J. Thromb. Haemost. 13 (4), 508–519. doi:10.1111/jth.12841
Yang, Y. L., Liu, D. W., Wang, X. T., Long, Y., Zhou, X., and Chai, W. Z. (2013). Body Temperature Control in Patients with Refractory Septic Shock: Too Much May Be Harmful. Chin. Med. J. Engl. 126 (10), 1809–1813. doi:10.3760/cma.j.issn.0366-6999.20121880
Yin, F., Zhang, F., Liu, S., and Ning, B. (2020). The Therapeutic Effect of High-Volume Hemofiltration on Sepsis: a Systematic Review and Meta-Analysis. Ann. Transl. Med. 8 (7), 488. doi:10.21037/atm.2020.03.48
Yongzhi, X. (2021). COVID-19-associated Cytokine Storm Syndrome and Diagnostic Principles: an Old and New Issue. Emerg. Microbes Infect. 10 (1), 266–276. doi:10.1080/22221751.2021.1884503
Young, P., Saxena, M., Bellomo, R., Freebairn, R., Hammond, N., van Haren, F., et al. (2015). Acetaminophen for Fever in Critically Ill Patients with Suspected Infection. N. Engl. J. Med. 373 (23), 2215–2224. doi:10.1056/NEJMoa1508375
Zarychanski, R., Abou-Setta, A. M., Kanji, S., Turgeon, A. F., Kumar, A., Houston, D. S., et al. (2015). The Efficacy and Safety of Heparin in Patients with Sepsis: a Systematic Review and Metaanalysis. Crit. Care Med. 43 (3), 511–518. doi:10.1097/CCM.0000000000000763
Zeiher, B. G., Artigas, A., Vincent, J. L., Dmitrienko, A., Jackson, K., Thompson, B. T., et al. (2004). Neutrophil Elastase Inhibition in Acute Lung Injury: Results of the STRIVE Study. Crit. Care Med. 32 (8), 1695–1702. doi:10.1097/01.ccm.0000133332.48386.85
Ziegler, E. J., Fisher, C. J., Sprung, C. L., Straube, R. C., Sadoff, J. C., Foulke, G. E., et al. (1991). Treatment of Gram-Negative Bacteremia and Septic Shock with HA-1A Human Monoclonal Antibody against Endotoxin. A Randomized, Double-Blind, Placebo-Controlled Trial. The HA-1A Sepsis Study Group. N. Engl. J. Med. 324 (7), 429–436. doi:10.1056/NEJM199102143240701
Keywords: sepsis, inflammation, cytokine storm, COVID-19, tumor necrosis factor, paradigm, Kuhn
Citation: Shapiro L, Scherger S, Franco-Paredes C, Gharamti AA, Fraulino D and Henao-Martinez AF (2022) Chasing the Ghost: Hyperinflammation Does Not Cause Sepsis. Front. Pharmacol. 13:910516. doi: 10.3389/fphar.2022.910516
Received: 05 April 2022; Accepted: 23 May 2022;
Published: 23 June 2022.
Edited by:
Fulvio D'Acquisto, University of Roehampton London, United KingdomReviewed by:
Song Hui Jia, St. Michael’s Hospital, CanadaPier Maria Fornasari, Rizzoli Orthopedic Institute (IRCCS), Italy
Copyright © 2022 Shapiro, Scherger, Franco-Paredes, Gharamti, Fraulino and Henao-Martinez. This is an open-access article distributed under the terms of the Creative Commons Attribution License (CC BY). The use, distribution or reproduction in other forums is permitted, provided the original author(s) and the copyright owner(s) are credited and that the original publication in this journal is cited, in accordance with accepted academic practice. No use, distribution or reproduction is permitted which does not comply with these terms.
*Correspondence: Leland Shapiro, bGVsYW5kLnNoYXBpcm9AY3VhbnNjaHV0ei5lZHU=