- 1Department of Medical Ultrasonics, Zibo Central Hospital, Zibo, China
- 2Department of Cardiology, Zibo Central Hospital, Zibo, China
- 3Department of Infectious Disease, Zibo Infectious Disease Hospital, Zibo, China
- 4Laboratory Department, Zibo Central Hospital, Zibo, China
- 5Department of Cardiology, Qilu Hospital of Shandong University, Jinan, China
- 6Department of Cardiology, Central Hospital Affiliated to Shandong First Medical University, Jinan, Shandong, China
Background and Purpose: The aim of this study was to explore the effect of half a year of evolocumab plus moderate-intensity statin treatment on carotid intraplaque neovascularization (IPN) and blood lipid levels.
Methods: A total of 31 patients with 33 carotid plaques who received evolocumab plus statin treatment were included. Blood lipid levels, B-mode ultrasound and contrast-enhanced ultrasonography (CEUS) at baseline and after half a year of evolocumab plus statin therapy were collected. The area under the curve (AUC) reflected the total amount of acoustic developer entering the plaque or lumen within the 180 s measurement period. The enhanced intensity reflected the peak blood flow intensity during the monitoring period, and the contrast agent area reflected the area of vessels in the plaques.
Results: Except for high-density lipoprotein cholesterol (HDL-c), all other lipid indices decreased. Compared with baseline, low-density lipoprotein cholesterol (LDL-c) decreased by approximately 57% (p < 0.001); total cholesterol (TC) decreased by approximately 34% (p < 0.001); small dense low-density lipoprotein (sd-LDL) decreased by approximately 52% (p < 0.001); and HDL-c increased by approximately 20% (p < 0.001). B-mode ultrasonography showed that the length and thickness of the plaque and the hypoechoic area ratio were reduced (p < 0.05). The plaque area, calcified area ratio, and lumen cross-sectional area changed little (p > 0.05). CEUS revealed that the area under the curve of plaque/lumen [AUC (P/L)] decreased from 0.27 ± 0.13 to 0.19 ± 0.11 (p < 0.001). The enhanced intensity ratio of plaque/lumen [intensity ratio (P/L)] decreased from 0.37 ± 0.16 to 0.31 ± 0.14 (p = 0.009). The contrast agent area in plaque/area of plaque decreased from 19.20 ± 13.23 to 12.66 ± 9.59 (p = 0.003). The neovascularization score decreased from 2.64 ± 0.54 to 2.06 ± 0.86 (p < 0.001). Subgroup analysis based on statin duration (<6 months and ≥6 months) showed that there was no significant difference in the AUC (P/L) or intensity ratio (P/L) at baseline or after half a year of evolocumab treatment.
Conclusion: This study found that evolocumab combined with moderate-intensity statins significantly improved the blood lipid profile and reduced carotid IPN.
Clinical Trial Registration: https://www.clinicaltrials.gov; identifier: NCT04423406.
Introduction
Evolocumab is a novel lipid-lowering drug that can specifically inhibit proprotein convertase subtilisin-kexin type 9 (PCSK9), an enzyme that regulates serum low-density lipoprotein cholesterol (LDL-c) levels through the degradation of the LDL receptor (Yurtseven et al., 2020; Mercep et al., 2022). According to recent studies, blocking PCSK9 with specific inhibitors causes a significant decrease in LDL-c levels with or without statin cotreatment (Landmesser et al., 2017; Reyes-Soffer et al., 2017; Sabatine et al., 2017; Diaz et al., 2021; Sever et al., 2021). The FOURIER trial (Further Cardiovascular Outcomes Research with PCSK9 Inhibition in Subjects with Elevated Risk) showed that evolocumab lowered LDL-c levels to a median of 0.78 mmol/L and reduced the risk of cardiovascular events by 15% (Sabatine et al., 2017). Another study showed that lower LDL-c was related to more stable plaque (Kataoka et al., 2015). The Global Assessment of Plaque Regression with a PCSK9 Antibody as Measured by Intravascular Ultrasound (GLAGOV) study found that if LDL-c is low enough, it can even reverse atherosclerotic plaque (Nicholls et al., 2016). Based on these findings, we hypothesized that carotid plaques would become more stable with evolocumab treatment.
Plaque stability depends on several factors, including lipid content, thickness of the fibrous cap, and inflammatory reactions (Hafiane, 2019). In addition, angiogenesis plays a crucial role in determining plaque development and vulnerability. Several in vivo studies have demonstrated that increased microvessels are associated with plaque rupture (Dunmore et al., 2007; Kodama et al., 2012). Furthermore, vessel density was found to be two times greater in vulnerable plaques than in stable plaques and four times greater in ruptured plaques than in stable plaques (Chen et al., 2013). Therefore, identifying intraplaque neovascularization (IPN) has great significance for identifying vulnerable plaques. Magnetic resonance angiography (MRA) is the current gold standard to identify IPN in plaques with high resolution and sensitivity, but its high cost and long processing time limit its use. Traditional ultrasonography is a cost-effective carotid plaque screening imaging modality used in China, but the resolution of color/power Doppler is not sufficient for identifying IPN. However, contrast-enhanced ultrasonography (CEUS) may allow increased dynamic visualization of IPN distribution and density, providing a comprehensive assessment of plaque stability (Cui et al., 2021; Huang et al., 2021; Lyu et al., 2021).
To our knowledge, this is the first study to use CEUS for carotid IPN detection and carotid plaque stability evaluation after evolocumab treatment combined with statin therapy.
Patients and methods
Patients
This prospective, single-center, open-label, single-arm, comparative study was approved by our Research Ethics Committee. The study was explained to the participants by the investigator, and all participants signed written informed consent forms. From August 2020 to July 2021, 32 patients who met all of the following criteria were recruited: ① there was at least one carotid atherosclerotic plaque on ultrasound imaging, defined according to the Mannheim consensus as the presence of focal structures encroaching into the arterial lumen by > 0.5 mm, by 50% of the thickness of the surrounding intima-media complex, or by the thickness of the intima-media layer if this was >1.5 mm (Touboul et al., 2012); ② plaques thicker than 1.5 mm and uniformly or predominantly echolucent as determined by B-mode ultrasonography; ③ plaques had IPN determined by CEUS; ④ patients had been treated with statins for at least 1 month before enrollment, with LDL-c higher than 1.8 mmol/L and triglyceride (TG) lower than 4.5 mmol/L. Patients were excluded if they had known allergies to albumin or SonoVue; were pregnant; had severe liver, kidney or thyroid dysfunction; had severe arrhythmia, heart failure or hypertension; had poor blood glucose control; or had used evolocumab before.
Among the 32 patients, 31 completed the follow-up and were included in the analysis; one patient was lost to follow-up. Figure 1 shows the flow chart of this study.
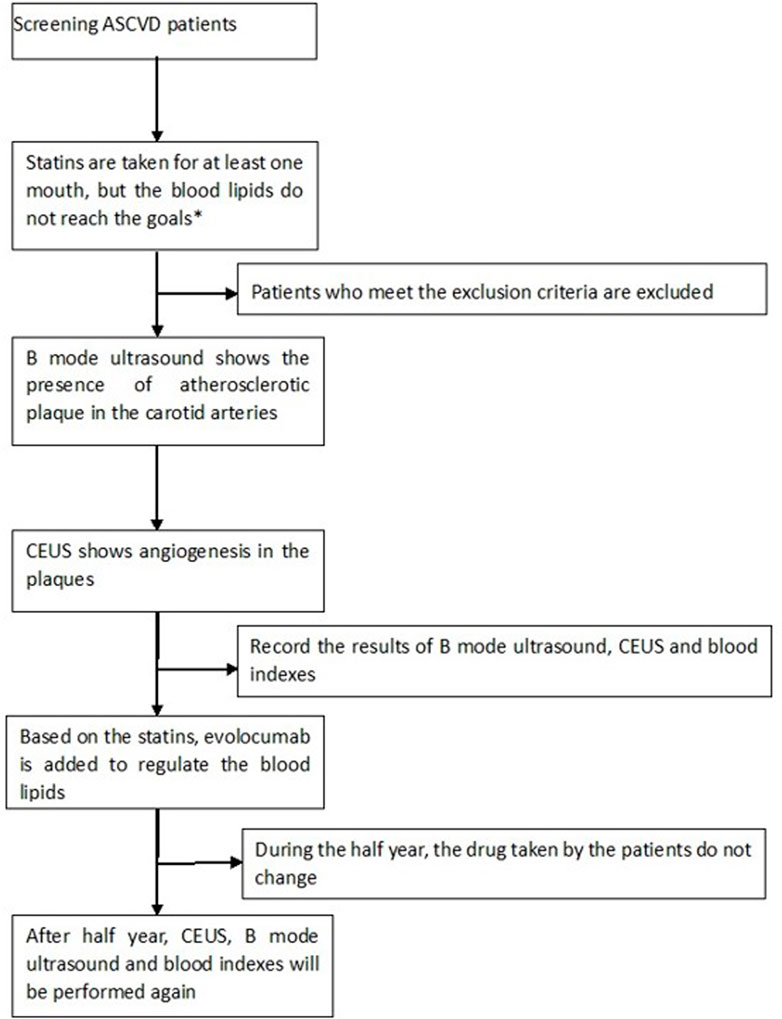
FIGURE 1. Our target goal for blood lipids was LDL-c<1.8 mmol/L; CEUS: contrast-enhanced ultrasound; ASCVD: arteriosclerotic cardiovascular disease; exclusion criteria: allergies to albumin or SonoVue; pregnancy; severe liver, kidney or thyroid dysfunction; severe arrhythmia, heart failure or hypertension; and diabetes patients with poor control of blood glucose who had used evolocumab before.
All included patients first underwent moderate-intensity statin therapy: rosuvastatin (10 mg qd po) or an equivalent dose of another statin for at least 1 month, followed by evolocumab (140 mg/2 weeks, ih). Table 1 shows the patients’ demographic characteristics, including sex, age, and comorbidities.
Analysis of blood lipid levels
A total of 5 ml of fasting blood was drawn using BD Vacationer vacuum blood collection tubes (Suzhou BD Medical Devices Co., Ltd.). The following blood lipid parameters were measured using an analyzer (Roche Diagnostics Co., Ltd. (Shanghai)) at baseline and after half a year of evolocumab plus statin therapy: total cholesterol (TC), TG, high-density lipoprotein cholesterol (HDL-c), LDL-c, small dense low-density lipoprotein (sd-LDL), and 1ipoprotein(a) (LP(a)).
US imaging acquisition
B-mode ultrasonography and CEUS were performed at baseline and after half a year of evolocumab plus statin therapy using a GE Logic E9 ultrasound system (GE Medical Systems Ultrasound and Primary Care Diagnostics; Wauwatosa, WI, USA) equipped with a 9-L probe at a transmission frequency of 9–11 MHz. All procedures were performed by a vascular radiologist (Ju Chen) with 15 years of experience who was blinded to the medical history and blood results of the participants.
Each patient was requested to lie in the supine position and fully expand the neck. The left and right common carotid arteries, extracranial segments of the internal carotid artery and external carotid artery were examined in the transverse and longitudinal views on B-mode images. Plaque was classified as follows: hyperechoic plaque was defined as a ratio of calcified area to total plaque area of greater than 90%; homogeneous echolucent plaque was defined as a plaque with an echogenicity less than that of the surrounding adventitia in >80% of the area, without acoustic shadowing; mixed plaque was defined as plaque containing <90% of the circumferential calcification or with associated echo-dense and anechoic regions that occupied <80% of the plaque area (Staub et al., 2011). Echolucent and mixed plaques were analyzed.
CEUS imaging was performed after the administration of SonoVue (Bracco SpA, Milan, Italy). A 1.6 ml bolus was injected via the antecubital vein within 2–3 s, followed by a 5 ml saline flush. Since it has a mean diameter of 2.5 µm (Kern et al., 2004), SonoVue can freely flow in tiny capillaries but does not pass through the vascular bed into the tissue space, making it an ideal intravascular tracer (Ferrara et al., 2000). The image setting was adjusted to maximize the visualization of SonoVue, with a machine index of 0.11–0.12, focusing below the region of interest (ROI), and with maximum signal gain and minimum noise. Cine clips were recorded immediately after injection and lasted for 3 min. All clips were stored for further analysis. The patients were observed for at least 30 min in case any complications occurred.
US image analysis
The maximal plaque thickness, calcified area and hypoechoic area were measured on the thickest cross-section of plaque. Maximal plaque thickness was measured from the media-adventitia to intima-lumen boundaries. The calcified area and hypoechoic area of the plaque were measured by B-mode ultrasound imaging on the maximum section of the long axis of the plaque by ImageJ software.
Plaques thicker than 1.5 mm were further analyzed by CEUS. First, CEUS imaging was quantitatively evaluated using time-signal intensity curve analysis software (TIC Analysis; TomTec, Germany) on the GE Logic E9. ROIs of similar size and depth were drawn around the margin of the plaque and in the surrounding lumen to obtain the following specific parameters: background intensity (BI), peak intensity (PI), enhanced intensity and area under the curve (AUC) of the plaque and lumen. The AUC reflected the total amount of acoustic developer entering the plaque or lumen within the measured 180 s; the enhanced intensity reflected the peak blood flow intensity during the monitoring period according to the formula: enhanced intensity = PI-BI. To avoid error caused by the background signal during each measurement, we used the standard method for the AUC and enhanced intensity values: AUC (P/L) = AUC in plaque/AUC in lumen; enhanced intensity (P/L) = (PI-BI) in plaque/(PI-BI) in lumen. The contrast agent area reflected the area of vessels in the plaques. We then used ImageJ to calculate the area ratio of contrast agent in the plaque, which was expressed as its ratio to the plaque area.
For each plaque, the neovascularization score was used to semiquantitatively categorize the degree of enhancement as follows: 0 points: no contrast medium in the plaque; 1 point: punctate contrast medium in the plaque; 2 points: between 1 and 3 points; 3 points: linear contrast medium in the plaque (Shah et al., 2007).
Two radiologists each with 10 years of experience who were blinded to the participants’ information independently analyzed the TIC parameter. If any disagreements occurred, another senior radiologist took part in the interpretation until a consensus was reached.
Statistical analysis
Values are reported as the mean ± standard deviation, where appropriate. The data analysis was performed using SPSS 26.0 (SPSS, Inc., Chicago, IL, USA). The t test of paired samples was used to compare parameters at baseline and after half a year of evolocumab plus statin therapy. Unpaired t tests were used for the subgroup analyses. p < 0.05 was considered to indicate a statistically significant difference.
Results
Baseline characteristics
During the study period, 31 subjects were enrolled for analysis: 20 males with a mean age of 56.95 ± 10.23 years and 11 females with a mean age of 62.00 ± 5.58 years. The number of patients with different risk factors was as follows: 11 patients had diabetes mellitus, 20 patients had hypertension, 6 patients had an active smoking history, 28 patients had coronary artery disease, 3 patients had experienced stroke and 2 patients had peripheral arterial disease. All subjects were diagnosed with atherosclerotic cardiovascular disease (ASCVD), and many types of drugs had been used, such as β-blockers and aspirin. The 31 patients included in the study presented with a total of 33 carotid plaques. The baseline characteristics of the included patients are shown in Table 1. Table 2 shows the changes in heart rate, blood pressure, blood lipid profiles and biochemistry indices pre- and posttherapy.
Effect of evolocumab plus statin therapy on blood lipid levels
Consistent with several other large studies, the lipid profiles changed significantly after evolocumab plus statin treatment. Compared with baseline, LDL-c decreased by approximately 57% (2.23 ± 0.75 mmol/L vs. 0.95 ± 0.63 mmol/L, p < 0.001); TC decreased by approximately 34% (3.84 ± 0.99 mmol/L vs. 2.55 ± 0.72 mmol/L, p < 0.001); TG decreased by approximately 11% (1.66 ± 0.83 mmol/L vs. 1.48 ± 1.63 mmol/L, p = 0.560); lipoprotein(a) [LP(a)] decreased by approximately 27% (202.26 ± 347.42 mg/L vs. 147.17 ± 243.67 mg/L, p = 0.274); sd-LDL decreased by approximately 52% (0.69 ± 0.32 mmol/L vs. 0.33 ± 0.25 mmol/L, p < 0.001); and HDL-c increased by approximately 20% (1.08 ± 0.34 mmol/L vs. 1.30 ± 0.26 mmol/L, p < 0.001) (Table 2).
Effect of evolocumab plus statin therapy on B-mode US features
The most commonly used evaluation indicators for plaques are the maximum length, thickness and cross-sectional area of the plaque’s long axis. Compared with baseline, the length of the plaques decreased from 16.97 ± 10.75 mm to 16.80 ± 10.77 mm (p = 0.002), and the thickness of the plaques decreased from 2.81 ± 1.08 mm to 2.73 ± 1.08 mm (p = 0.002). These changes were statistically significant.
The hypoechoic area of the plaque/area of plaque was reduced (from 0.037 ± 0.062 to 0.028 ± 0.052; p = 0.015). The plaque area, calcified plaque area/plaque area, and lumen cross-sectional area changed little (p = 0.222, p = 0.264 and p = 0.700, respectively) (Table 3).
Effect of evolocumab plus statin therapy on CEUS parameters
The CEUS results demonstrated that AUC (P/L), which represents the total amount of contrast agent in the intraplaque microvessels during 180 s of monitoring, was significantly reduced from 0.27 ± 0.13 at baseline to 0.19 ± 0.11 after half a year of evolocumab plus statin therapy (p < 0.001). The intensity ratio (P/L), which represents the peak flow velocity of contrast agent into the intraplaque microvessels, was reduced from 0.37 ± 0.16 at baseline to 0.31 ± 0.14 after half a year of therapy (p = 0.009). The contrast agent area in plaque/area of plaque, which represents the density of microvessels in a plaque, also decreased, from 19.20 ± 13.23 at baseline to 12.66 ± 9.59 after half a year of therapy (p = 0.003). The neovascularization score was also significantly reduced from 2.64 ± 0.54 to 2.06 ± 0.86 (p < 0.001) (Table 3 and Figure 2).
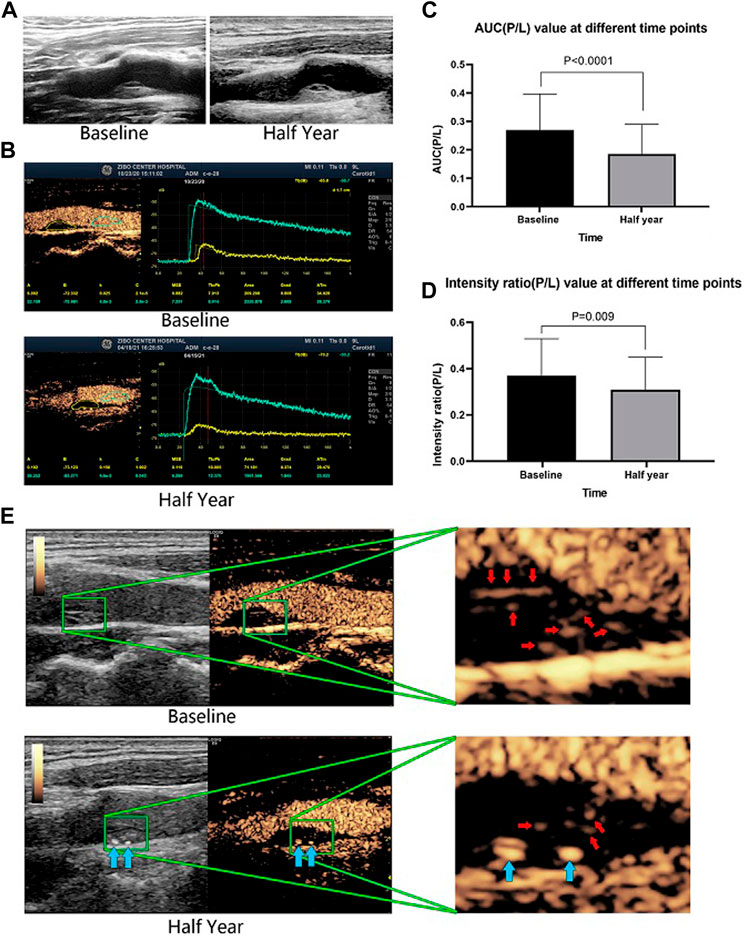
FIGURE 2. A representative patient who had a large plaque in the common carotid artery that was identified by B-mode ultrasound. The plaque morphology changed little between baseline and after half a year of therapy (A). CEUS revealed that the contrast agent area and microvessel density were obviously reduced after half a year of evolocumab plus statin treatment (B). The AUC (P/L) and the enhanced intensity (P/L) decreased significantly after half a year of treatment (E). Red arrows indicate vessels in the plaque, and blue arrows indicate calcified plaques. The changes in AUC (P/L) and enhanced intensity (P/L) of all included patients before and after half a year of treatment (C,D).
Figure 2 shows a representative patient who had a large plaque in the common carotid artery that was identified by B-mode ultrasound. Although the plaque morphology changed little (Figure 2A), CEUS revealed that the contrast agent area and microvessel density were obviously reduced (Figure 2B), and the AUC (P/L) and the intensity ratio (P/L) had decreased significantly (Figure 2C) after half a year of evolocumab plus statin treatment.
Influence of statins on the endpoints
As a single-arm study, the largest limitation of this study was that there was no statin control group. However, statins are certain to have an impact on the outcomes. To limit the effect of statins as confounding factors, the patients were initially asked to start statin therapy for at least 1 month. We then evaluated the influence of different durations of statin use on the outcomes. Subgroup analysis was performed according to the duration of statin administration before the addition of evolocumab (<6 months and ≥6 months). The AUC and intensity ratio values of different durations of statin administration were compared at baseline and 6 months, respectively. However, they were not significantly different (p > 0.05) Table 4.
Discussion
In this study, evolocumab plus statins significantly reduced the LDL-c levels in patients. Furthermore, the decreased CEUS parameters indicated that the plaques were stabilized by inhibiting IPN, which might reduce the occurrence of cardiovascular events.
Previous clinical studies (Scandinavian Simvastatin Survival Study Group, 1994; Colhoun et al., 2004; Heart Protection Study Collaborative Group, 2002; Long-Term Intervention with Pravastatin in Ischaemic Disease (LIPID) Study Group, 1998) have confirmed that statins can significantly reduce cardiovascular mortality and all-cause mortality in patients with atherosclerosis; therefore, statins have been seen as the cornerstone treatment for atherosclerotic diseases. However, statins have their own limitations. First, if the statin dose is doubled, its lipid-lowering effect is increased by only 6% (Zhao et al., 2014), which makes it difficult for many patients to achieve the LDL-c goal with statins alone. Second, some patients cannot tolerate statins (Reiner, 2018); this has been particularly identified in Chinese patients who are administered high-dose statins (Group et al., 2014).
Evolocumab provides a new regimen for these patients. It can reduce LDL-c levels by specifically binding PCSK9. The GAUSS-3 study (Goal Achievement after Utilizing an anti-PCK9 antibody in Statin Intolerant Subjects-3) confirmed that evolocumab was an effective alternative to reduce LDL-c in patients at high risk of ASCVD who had statin intolerance or familial hypercholesterolemia (Nissen et al., 2016). The FOURIER study proved that the addition of evolocumab to statin therapy could further reduce LDL-c by 59% and reduced the relative risk of major cardiovascular events by 15–20%. Further analysis showed that there was no lower limit for the reduction of LDL-c by evolocumab (Sabatine et al., 2017).
In our study, compared with baseline, the LDL-c level decreased by approximately 57% after a half year of evolocumab treatment. The change trends of other blood lipid indices, such as TC and HDL-c, were similar to the results of the FOURIER study. Sd-LDL is a subtype of LDL-c, and its metabolic pathway is the same as that of LDL-c. When LDL-c was reduced by evolocumab, the sd-LDL level also decreased. A previous study confirmed that sd-LDL was the most atherogenic lipoprotein parameter (Ikezaki et al., 2021). Theoretically, evolocumab can prevent, delay and even reverse the development of plaques.
One important feature of plaque vulnerability is IPN. Cui et al. (Cui et al., 2021) demonstrated that carotid IPN was an independent predictor of future vascular events in patients with recent ischemic stroke. Song et al. (Song et al., 2020) also showed that IPN was a risk factor for the occurrence of the clinical symptoms of stroke. Recent clinical studies have shown that statins can inhibit IPN (Xu et al., 2018; Du et al., 2019). In our study, the AUC (P/L), intensity ratio (P/L), agent area in plaque/area of plaque, and neovascularization score indicated that the amount of IPN had decreased. Our observations indicate that evolocumab inhibits IPN, similar to statins, and this occurs for several reasons. First, the GLAGOV study confirmed that compared with placebo, evolocumab reversed atherosclerotic plaque and reduced the plaque volume (Nicholls et al., 2016). This meant that there was a corresponding reduction in the amount of nutrients and oxygen required by the plaque, so the number of new blood vessels in the plaque was correspondingly reduced. Second, an important mechanism of IPN is vascular inflammation (Chistiakov et al., 2017). Current studies have demonstrated that ecolocumab has anti-inflammatory effects, so it might also reduce IPN in this way (Hoogeveen et al., 2019; Momtazi-Borojeni et al., 2019; Vlachopoulos et al., 2019). Third, evolocumab improves the blood lipid profile by reducing TC, LDL-c, sd-LDL, LP(a), and TG and increasing HDL-c. Improvement of the blood lipid profile itself will also inhibit IPN (Wang et al., 2021).
Other than CEUS, the MRA, positron emission tomography (PET) and computerized tomography angiography (CTA) techniques can also be used to assess angiogenesis within plaques. However, CEUS has some advantages; it is non-invasive, inexpensive, and has high temporal and spatial resolution. Most importantly, it has been confirmed that its results are well correlated with histopathological results (Saito et al., 2014; Huang et al., 2016) and it can be used predict future vascular events in patients (Song et al., 2020; Cui et al., 2021). Using CEUS to assess the stability of carotid atherosclerotic plaques and guide medication decision-making will help to reduce the incidence of ischemic stroke and improve patients’ health. Therefore, the clinical use of this technique should be promoted.
An important limitation of the present study was that it was a single-arm study with no control group. To minimize the confounding influence of background statins on the study results, we performed a subgroup analysis. The subgroup analysis indicated that there was no significant difference in the indicators of neovascularization in the plaque between patients who had used statins for less than 6 months before enrollment and patients who had used statins for more than 6 months. Xu et al. reported that IPN changes could occur after 1 year of statin treatment (Xu et al., 2018), while Du et al. found IPN changes after 3 months of rosuvastatin treatment (Du et al., 2019). Therefore, our study demonstrated that even in patients with a well-documented statin background, the use of evolocumab could further reduce IPN. Nevertheless, larger randomized controlled trials are needed to confirm the relationship between evolocumab and IPN.
In conclusion, our findings suggest that evolocumab effectively reduced LDL-c and improved the blood lipid profile. Evolocumab therapy significantly reduced IPN and thereby stabilized plaques.
Data availability statement
The original contributions presented in the study are included in the article/supplementary material, further inquiries can be directed to the corresponding authors.
Ethics statement
The studies involving human participants were reviewed and approved by Zibo Central Hospital Research Ethics Committee. The patients/participants provided their written informed consent to participate in this study.
Author contributions
All authors listed have made a substantial, direct, and intellectual contribution to the work and approved it for publication.
Funding
This study was supported by the Natural Science Foundation of China (No. 81700321). Clinical Medicine Science and Technology Innovation Program of Jinan (No. 202019045). Key Research and Design Plan of Zibo (No. 2020ZC010063, No. 2020ZC010020).
Conflict of interest
The authors declare that the research was conducted in the absence of any commercial or financial relationships that could be construed as a potential conflict of interest.
Publisher’s note
All claims expressed in this article are solely those of the authors and do not necessarily represent those of their affiliated organizations, or those of the publisher, the editors and the reviewers. Any product that may be evaluated in this article, or claim that may be made by its manufacturer, is not guaranteed or endorsed by the publisher.
References
Chen, S., Guo, L., Chen, B., Sun, L., and Cui, M. (2013). Association of serum angiopoietin-1, angiopoietin-2 and angiopoietin-2 to angiopoietin-1 ratio with heart failure in patients with acute myocardial infarction. Exp. Ther. Med. 5, 937–941. doi:10.3892/etm.2013.893
Chistiakov, D. A., Melnichenko, A. A., Myasoedova, V. A., Grechko, A. V., and Orekhov, A. N. (2017). Role of lipids and intraplaque hypoxia in the formation of neovascularization in atherosclerosis. Ann. Med. 49, 661–677. doi:10.1080/07853890.2017.1366041
Colhoun, H. M., Betteridge, D. J., Durrington, P. N., Hitman, G. A., W Neil Ha, , Livingstone, S. J., et al. (2004). Primary prevention of cardiovascular disease with atorvastatin in type 2 diabetes in the collaborative atorvastatin diabetes study (cards): Multicentre randomised placebo-controlled trial. Lancet 364, 685–696. doi:10.1016/S0140-6736(04)16895-5
Cui, L., Xing, Y., Zhou, Y., Wang, L., Liu, K., Zhang, D., et al. (2021). Carotid intraplaque neovascularisation as a predictive factor for future vascular events in patients with mild and moderate carotid stenosis: An observational prospective study. Ther. Adv. Neurol. Disord. 14, 17562864211023992. doi:10.1177/17562864211023992
Diaz, R., Li, Q. H., Bhatt, D. L., Bittner, V. A., Baccara-Dinet, M. T., Goodman, S. G., et al. (2021). Intensity of statin treatment after acute coronary syndrome, residual risk, and its modification by alirocumab: Insights from the odyssey outcomes trial. Eur. J. Prev. Cardiol. 28, 33–43. doi:10.1177/2047487320941987
Du, R., Cai, J., Cui, B., Wu, H., Zhao, X. Q., and Ye, P. (2019). Rapid improvement in carotid adventitial angiogenesis and plaque neovascularization after rosuvastatin therapy in statin treatment-naive subjects. J. Clin. Lipidol. 13, 847–853. doi:10.1016/j.jacl.2019.07.008
Dunmore, B. J., McCarthy, M. J., Naylor, A. R., and Brindle, N. P. (2007). Carotid plaque instability and ischemic symptoms are linked to immaturity of microvessels within plaques. J. Vasc. Surg. 45, 155–159. doi:10.1016/j.jvs.2006.08.072
Ferrara, K. W., Merritt, C. R., Burns, P. N., Foster, F. S., Mattrey, R. F., and Wickline, S. A. (2000). Evaluation of tumor angiogenesis with us: Imaging, Doppler, and contrast agents. Acad. Radiol. 7, 824–839. doi:10.1016/s1076-6332(00)80631-5
Group, H. T. C., Landray, M. J., Haynes, R., Hopewell, J. C., Parish, S., Aung, T., et al. (2014). Effects of extended-release niacin with laropiprant in high-risk patients. N. Engl. J. Med. 371, 203–212. doi:10.1056/NEJMoa1300955
Hafiane, A. (2019). Vulnerable plaque, characteristics, detection, and potential therapies. J. Cardiovasc Dev. Dis. 6, 26. doi:10.3390/jcdd6030026
Heart Protection Study Collaborative Group (2002). Mrc/bhf heart protection study of cholesterol lowering with simvastatin in 20 536 high-risk individuals: A randomised placebocontrolled trial. Lancet 360, 7–22. doi:10.1016/S0140-6736(02)09327-3
Hoogeveen, R. M., Opstal, T. S. J., Kaiser, Y., Stiekema, L. C. A., Kroon, J., Knol, R. J. J., et al. (2019). Pcsk9 antibody alirocumab attenuates arterial wall inflammation without changes in circulating inflammatory markers. JACC Cardiovasc Imaging 12, 2571–2573. doi:10.1016/j.jcmg.2019.06.022
Huang, R., Abdelmoneim, S. S., Ball, C. A., Nhola, L. F., Farrell, A. M., Feinstein, S., et al. (2016). Detection of carotid atherosclerotic plaque neovascularization using contrast enhanced ultrasound: A systematic review and meta-analysis of diagnostic accuracy studies. J. Am. Soc. Echocardiogr. 29, 491–502. doi:10.1016/j.echo.2016.02.012
Huang, S., Wu, X., Zhang, L., Wu, J., He, Y., Lai, M., et al. (2021). Assessment of carotid plaque stability using contrast-enhanced ultrasound and its correlation with the expression of cd147 and mmp-9 in the plaque. Front. Comput. Neurosci. 15, 778946. doi:10.3389/fncom.2021.778946
Ikezaki, H., Lim, E., Cupples, L. A., Liu, C. T., Asztalos, B. F., and Schaefer, E. J. (2021). Small dense low-density lipoprotein cholesterol is the most atherogenic lipoprotein parameter in the prospective framingham offspring study. J. Am. Heart Assoc. 10, e019140. doi:10.1161/JAHA.120.019140
Kataoka, Y., Hammadah, M., Puri, R., Duggal, B., Uno, K., Kapadia, S. R., et al. (2015). Plaque microstructures in patients with coronary artery disease who achieved very low low-density lipoprotein cholesterol levels. Atherosclerosis 242, 490–495. doi:10.1016/j.atherosclerosis.2015.08.005
Kern, R., Szabo, K., Hennerici, M., and Meairs, S. (2004). Characterization of carotid artery plaques using real-time compound b-mode ultrasound. Stroke 35, 870–875. doi:10.1161/01.STR.0000120728.72958.4A
Kodama, T., Narula, N., Agozzino, M., and Arbustini, E. (2012). Pathology of plaque haemorrhage and neovascularization of coronary artery. J. Cardiovasc Med. Hagerst. 13, 620–627. doi:10.2459/JCM.0b013e328356a5f2
Landmesser, U., Chapman, M. J., Farnier, M., Gencer, B., Gielen, S., Hovingh, G. K., et al. (2017). European society of cardiology/European atherosclerosis society task force consensus statement on proprotein convertase subtilisin/kexin type 9 inhibitors: Practical guidance for use in patients at very high cardiovascular risk. Eur. Heart J. 38, 2245–2255. doi:10.1093/eurheartj/ehw480
Long-Term Intervention with Pravastatin in Ischaemic Disease (LIPID) Study Group (1998). Prevention of cardiovascular events and death with pravastatin in patients with coronary heart disease and a broad range of initial cholesterol levels. N. Engl. J. Med. 338, 9. doi:10.1056/NEJM199811053391902
Lyu, Q., Tian, X., Ding, Y., Yan, Y., Huang, Y., Zhou, P., et al. (2021). Evaluation of carotid plaque rupture and neovascularization by contrast-enhanced ultrasound imaging: An exploratory study based on histopathology. Transl. Stroke Res. 12, 49–56. doi:10.1007/s12975-020-00825-w
Mercep, I., Strikic, D., Sliskovic, A. M., and Reiner, Z. (2022). New therapeutic approaches in treatment of dyslipidaemia-a narrative review. Pharm. (Basel) 15, 839. doi:10.3390/ph15070839
Momtazi-Borojeni, A. A., Sabouri-Rad, S., Gotto, A. M., Pirro, M., Banach, M., Awan, Z., et al. (2019). Pcsk9 and inflammation: A review of experimental and clinical evidence. Eur. Heart J. Cardiovasc Pharmacother. 5, 237–245. doi:10.1093/ehjcvp/pvz022
Nicholls, S. J., Puri, R., Anderson, T., Ballantyne, C. M., Cho, L., Kastelein, J. J., et al. (2016). Effect of evolocumab on progression of coronary disease in statin-treated patients: The glagov randomized clinical trial. JAMA 316, 2373–2384. doi:10.1001/jama.2016.16951
Nissen, S. E., Stroes, E., Dent-Acosta, R. E., Rosenson, R. S., Lehman, S. J., Sattar, N., et al. (2016). Efficacy and tolerability of evolocumab vs ezetimibe in patients with muscle-related statin intolerance: The gauss-3 randomized clinical trial. JAMA 315, 1580–1590. doi:10.1001/jama.2016.3608
Scandinavian Simvastatin Survival Study Group (1994). Randomised trial of cholesterol lowering in 4444 patients with coronary heart disease: The scandinavian simvastatin survival study (4s). Lancet 344, 1383–1389. doi:10.1016/s0140-6736(94)90566-5
Reiner, Z. (2018). Pcsk9 inhibitors in clinical practice: Expectations and reality. Atherosclerosis 270, 187–188. doi:10.1016/j.atherosclerosis.2018.01.001
Reyes-Soffer, G., Pavlyha, M., Ngai, C., Thomas, T., Holleran, S., Ramakrishnan, R., et al. (2017). Effects of pcsk9 inhibition with alirocumab on lipoprotein metabolism in healthy humans. Circulation 135, 352–362. doi:10.1161/CIRCULATIONAHA.116.025253
Sabatine, M. S., Giugliano, R. P., Keech, A. C., Honarpour, N., Wiviott, S. D., Murphy, S. A., et al. (2017). Evolocumab and clinical outcomes in patients with cardiovascular disease. N. Engl. J. Med. 376, 1713–1722. doi:10.1056/NEJMoa1615664
Saito, K., Nagatsuka, K., Ishibashi-Ueda, H., Watanabe, A., Kannki, H., and Iihara, K. (2014). Contrast-enhanced ultrasound for the evaluation of neovascularization in atherosclerotic carotid artery plaques. Stroke 45, 3073–3075. doi:10.1161/STROKEAHA.114.006483
Sever, P., Gouni-Berthold, I., Keech, A., Giugliano, R., Pedersen, T. R., Im, K., et al. (2021). Ldl-cholesterol lowering with evolocumab, and outcomes according to age and sex in patients in the Fourier trial. Eur. J. Prev. Cardiol. 28, 805–812. doi:10.1177/2047487320902750
Shah, F., Balan, P., Weinberg, M., Reddy, V., Neems, R., Feinstein, M., et al. (2007). Contrast-enhanced ultrasound imaging of atherosclerotic carotid plaque neovascularization: A new surrogate marker of atherosclerosis? Vasc. Med. 12, 291–297. doi:10.1177/1358863X07083363
Song, Y., Dang, Y., Dang, L. L., Zhao, C., Zheng, J., Feng, J., et al. (2020). Association between intraplaque neovascularization assessed by contrast-enhanced ultrasound and the risk of stroke. Clin. Radiol. 75, 70–75. doi:10.1016/j.crad.2019.08.019
Staub, D., Partovi, S., Schinkel, A. F., Coll, B., Uthoff, H., Aschwanden, M., et al. (2011). Correlation of carotid artery atherosclerotic lesion echogenicity and severity at standard us with intraplaque neovascularization detected at contrast-enhanced us. Radiology 258, 618–626. doi:10.1148/radiol.10101008
Touboul, P. J., Hennerici, M. G., Meairs, S., Adams, H., Amarenco, P., Bornstein, N., et al. (2012). Mannheim carotid intima-media thickness and plaque consensus (2004-2006-2011). An update on behalf of the advisory board of the 3rd, 4th and 5th watching the risk symposia, at the 13th, 15th and 20th European stroke conferences, mannheim, Germany, 2004, brussels, Belgium, 2006, and hamburg, Germany, 2011. Cerebrovasc. Dis. 34, 290–296. doi:10.1159/000343145
Vlachopoulos, C., Koutagiar, I., Skoumas, I., Terentes-Printzios, D., Zacharis, E., Kolovou, G., et al. (2019). Long-term administration of proprotein convertase subtilisin/kexin type 9 inhibitors reduces arterial fdg uptake. JACC Cardiovasc Imaging 12, 2573–2574. doi:10.1016/j.jcmg.2019.09.024
Wang, Y., Yao, M., Zou, M., Ge, Z., Cai, S., Hong, Y., et al. (2021). Relationship between serum lipid profiles and carotid intraplaque neovascularization in a high-stroke-risk population: A cross-sectional study in China. J. Am. Heart Assoc. 10, e021545. doi:10.1161/JAHA.121.021545
Xu, B., Xing, J., Wu, W., Zhang, W. J., Zhu, Q. Q., Zhang, D., et al. (2018). Improved plaque neovascularization following 2-year atorvastatin therapy based on contrast-enhanced ultrasonography: A pilot study. Exp. Ther. Med. 15, 4491–4497. doi:10.3892/etm.2018.5926
Yurtseven, E., Ural, D., Baysal, K., and Tokgozoglu, L. (2020). An update on the role of pcsk9 in atherosclerosis. J. Atheroscler. Thromb. 27, 909–918. doi:10.5551/jat.55400
Keywords: proprotein convertase subtilisin-kexin type 9, evolocumab, atherosclerotic cardiovascular disease, intra-plaque neovascularization, contrast-enhanced ultrasonography
Citation: Chen J, Zhao F, Lei C, Qi T, Xue X, Meng Y, Zhang W, Zhang H, Wang J, Zhu H, Cheng C, Wang Q, Bi C, Song B, Jin C, Niu Q, An F, Li B, Huo X, Zhao Y and Li B (2023) Effect of evolocumab on the progression of intraplaque neovascularization of the carotid based on contrast-enhanced ultrasonography (EPIC study): A prospective single-arm, open-label study. Front. Pharmacol. 13:999224. doi: 10.3389/fphar.2022.999224
Received: 20 July 2022; Accepted: 08 December 2022;
Published: 04 January 2023.
Edited by:
Konstantinos Tziomalos, Aristotle University of Thessaloniki, GreeceReviewed by:
Aleksey M. Chaulin, Samara State Medical University, RussiaDanel Staub, University Children’s Hospital Basel, Switzerland
Federica Marzano, University of Naples Federico II, Italy
Copyright © 2023 Chen, Zhao, Lei, Qi, Xue, Meng, Zhang, Zhang, Wang, Zhu, Cheng, Wang, Bi, Song, Jin, Niu, An, Li, Huo, Zhao and Li. This is an open-access article distributed under the terms of the Creative Commons Attribution License (CC BY). The use, distribution or reproduction in other forums is permitted, provided the original author(s) and the copyright owner(s) are credited and that the original publication in this journal is cited, in accordance with accepted academic practice. No use, distribution or reproduction is permitted which does not comply with these terms.
*Correspondence: Xiaoguang Huo, MTg2NTMzOTk5MTlAMTYzLmNvbQ==; Yunhe Zhao, MjgxMTkzMTgxQHFxLmNvbQ==; Bo Li, bGlib3N1Ym1pdEAxNjMuY29t
†These authors have contributed equally to this work