- 1Department of Biological Sciences, College of Science, King Faisal University, Al-Ahsa, Saudi Arabia
- 2Centre of Molecular Medicine and Diagnostics, Department of Bio-Chemistry, Saveetha Dental College and Hospitals, Saveetha Institute of Medical and Technical Sciences, Saveetha University, Chennai, Tamil Nadu, India
- 3Department of Basic Medical Sciences, Faculty of Medicine, Aqaba Medical Sciences University, Aqaba, Jordan
- 4Department of Zoology, Faculty of Science, Alexandria University Egypt, Alexandria, Egypt
- 5Laboratory of Molecular Physiology, Zoology Department, Faculty of Science, Assiut University, Assiut, Egypt
Background: Although aluminum (Al) is not biologically crucial to the human body, classical studies have demonstrated that excessive human exposure to Al can induce oxidative damage, neuroinflammatory conditions and neurotoxic manifestations implicated in Alzheimer’s disease (AD). Exposure to Al was reported to be associated with oxidative damage, neuroinflammation, and to enhance progressive multiregional neurodegeneration in animal models. Several plant-derived natural biomolecules have been recently used to reduce the toxic effects of Al through decreasing the oxidative stress and the associated diseases. A good candidate still to be tested is an active natural furanocoumarin, the isoimperatorin (IMP) that can be extracted from Lemon and lime oils and other plants. Here, we examined the neuroprotective effects of IMP on aluminum chloride (AlCl3)-induced neurotoxicity in albino mice.
Methods: Twenty-four male albino mice were used in this study. Mice were randomly devided into 5 groups. The first group was given distilled water as a control, the second group was given AlCl3 orally (10 mg/wt/day) starting from the 2nd week to the end of the 6th week, the third group received AlCl3 orally and IMP interperitoneally, i. p. (30 mg/wt/day) starting from week 2 till week 6 where IMP was supplement 1st and then 4 h later AlCl3 was given to mice. The fourth group received the control (IMP 30 mg/wt, i. p.) from the 2nd week till the end of the experiment. Rodent models of central nervous system (CNS) disorders were assessed using object location memory and Y-maze tests in 6th week began. Essential anti-inflammatory and oxidative stress indicators were evaluated, including interleukin-1 β (IL-1β), tumor necrosis factor α (TNF-α), malondialdehyde (MDA), total antioxidant capacity (TAC), and catalase activity (CAT). In addition, serum levels of brain neurotransmitters such as corticosterone, acetylcholine (ACh), dopamine and serotonin in brain homogenates were measured calorimetrically.
Results: The study results revealed that the daily treatment of AlCl3 upregulated the TNF-α and IL-1β levels, increased MDA accumulation, and decreased TAC and CAT activity. In addition, aluminum induced a reduction in concentrations of ACh, serotonin and dopamine in the brain. However, IMP significantly ameliorates the effect of AlCl3 through modulating the antioxidant and regulating the inflammatory response through targeting Nrf2 (NF-E2-related factor 2) and mitogen-activated protein kinase (MAPK).
Conclusion: Thus, IMP might be a promising treatment option for neurotoxicity and neurodegenerative diseases, such as Alzheimer’s disease and Parkinson’s disease, which are associated with neuro-inflammation and oxidative stress.
1 Introduction
Aluminum is one of the most abundant metals found in the earth crust. It is found in many products, making humans prone to daily exposure (Abbas et al., 2022; Hamdan et al., 2022; Turkez et al., 2022). The daily intake of aluminum depends up on the food type. Moreover, some vaccines contain Al salts as adjuvants, which boost the body’s response to the vaccine (Del Giudice et al., 2018; Nouchikian et al., 2018; Principi and Esposito, 2018; Shi et al., 2019; Goullé and Grangeot-Keros, 2020). In addition, drugs used to treat ulcers contain high percentages of Al (Mei and Yao, 1993; Dordevic et al., 2019). Moreover, studies have indicated that antacids contain various Al salts and other metals as active ingredients. Consequently, metal toxicity can be induced by antacid consumption or abuse (Shrivastava et al., 2018; Rahimzadeh et al., 2022). Al affects bone formation and remodeling. Increasing doses of Al slow down osteoblast and osteoclast activity and cause osteomalacia and adynamic bone disease in bone, both clinically and experimentally. Humans and animals’ levels of parathyroid hormone (PTH) are disrupted by Al. In Al-dependent osteotoxicity, altered PTH levels may play a major or even minor role. Al causes a microcytic anemia in hematopoietic tissue, which isn't reversible by iron (Jeffery et al., 1996). However, the blood-brain barrier is relatively highly permeable to Al, leading to its accumulation in the brain. Al accumulation in the brain results in central nervous system (CNS) dysfunction, leading to motor, cognitive and behavioral problems (Wang, 2018; Ishaq et al., 2021; Roe, 2021). On the other hand, patients doing kidney dialysis can experience neurobehavioral symptoms, seizures, CNS toxicity, and even die from Al exposure in the dialysis fluid (Chen et al., 2018; Inan-Eroglu and Ayaz, 2018; Ravat and Gosala, 2018; Lukiw et al., 2019).
In animal models, AlCl3, has been reported to stimulate inflammation, dysfunction in synaptic system and neurodegeneration in several areas of the brain and spinal cord. Mechanistic studies clarified that inflammation stimulates the IκB kinase protein to phosphorylate inhibitor kappa B (NF-кB), allowing the translocation of NF-кB dimers into the nucleus, where they bind to the promoter site of the target gene. This pathway is triggered by certain pro-inflammatory mediators, such as tumor necrosis factor α (TNF-α) and interleukin-1 β (IL-1β). Inflammatory mediators are also regulated by mitogen-activated protein kinases (MAPKs), including c-Jun NH2-terminal kinase (JNK), extracellular signal-regulated kinase (ERK), and p38 in mammals (Zhou et al., 2018; Ji et al., 2021; Zong et al., 2021; Mehrbeheshti et al., 2022). Related to that, the phosphorylation events caused by AlCl3 stimulation can activate JNK, ERK, and p38 (Mehrbeheshti et al., 2022; Tsai et al., 2022). Al exposure and it subsequent accumulation in the brain was shown to be associated with neurodegenerative disorders, such as Parkinson’s disease and Alzheimer’s disease (AD) (Dalla Torre et al., 2019; McLachlan et al., 2019; McLachlan et al., 2020; Bondy and Campbell, 2021; Hassan and Kadry, 2021; Kawahara et al., 2021; Zeng et al., 2021; Abbas et al., 2022). These disorders are characterized by changes in the levels of neurotransmitters, i.e., acetylcholine (Ach). Thus, this promotes neuroinflammation and neurodegeneration, resulting in Alzheimer’s disease. These toxic effects stimulate complex mechanisms to defend against toxicity, such as heme oxygenase 1 (HO-1), NAD(P)H: quinone oxidoreductase 1 (NQO-1), superoxide dismutase 1, glutathione peroxidase, thioredoxins, and glutathione S-transferase (Li et al., 2014), which are activated by NF-E2-related factor 2 (Nrf2) in the CNS (Loboda et al., 2016; Wang et al., 2019). In addition, HO-1 and NQO-1 were demonstrated to reduce oxidative stress and maintain mitochondrial integrity, thereby protecting neurons (Yang et al., 2015). In parallel with that, patients with dementia display altered levels of HO-1 and NQO-1 expression (Wang et al., 2000). However, overexpression of Nrf2 was found to provide sufficient protection against neurotoxicity (Wang et al., 2019). Thus, to reduce symptoms of oxidative stress and treating neurodegenerative diseases, stimulating the Nrf2 signaling pathway might be a valuable tool.
Several plant-derived antioxidants that protect against metal toxicity, inflammation and apoptosis-related neurodegenerative disorders have recently been identified (Cherrak et al., 2016; Yadav et al., 2016; Lakey-Beitia et al., 2021). Angelica dahurica, Rhizoma et Radix Notopterygii, and Radix Peucedani contain isoimperatorin (IMP), an active natural furanocoumarin (Lai et al., 2021). Lemon and lime oils also contain IMP. Among its pharmacological effects, IMP has anti-hypertensive (Hwang et al., 2017), anti-inflammatory (Wijerathne et al., 2017; Chen et al., 2021), anti-tumor (Yang et al., 2018a), antibacterial (de Souza et al., 2005; Pokharel et al., 2006), and liver protective (Pokharel et al., 2006) effects, including diastolic vasoactivity (Lai et al., 2021), among other effects (Wijerathne et al., 2017; Kiyonga et al., 2021; Lai et al., 2021; Chahardoli et al., 2022; Kim et al., 2022; Liu et al., 2022). Dietary furocoumarin IMP was shown to stimulate glucagon-like peptide secretion and reduces blood sugar in rats by activating the G protein-coupled bile acid receptor (Wang et al., 2017). In addition, IMP was found to enhance adipocyte differentiation in lipodystrophic patients. These patients had a triglyceride storage defects and severe insulin resistance because of ectopic lipids accumulate in adipose tissue. As a result of increased fat storage capacity in adipose tissue with low-fat mobilization, fat mass expands which is the most optimal method of storing lipids (Jiang et al., 2019). This study tested whether IMP protects albino mice against AlCl3-induced behavioral deficits, neurotransmitter abnormalities, and oxidative inflammatory damage.
2 Materials and methods
2.1 Chemicals
Sigma-Aldrich Co., (St. Louis, MO, United States) provided IMP and AlCl3. Stock solutions were prepared every week. The AlCl3 was dissolved in distilled water, while the IMP was dissolved in DMSO first, then diluted in normal saline to 0.1% DMSO. Aseptic conditions were used to prepare and inject the drugs.
2.2 Animal groups
Twenty-four male albino mice were used in this study. Each group included six mice (6–8 week old). Throughout the research, all methods were conducted according to relevant guidelines and regulations at King Faisal University. In a normal laboratory atmosphere, mice were kept at a temperature of 24°C and were subjected to a 12/12-h light/dark cycle. Mice were randomly divided into 5 groups. The first group was used as a control an received distilled water, the second group was given AlCl3 orally (10 mg/wt/day) starting from the 2nd week to the end of the 6th week, the third group received AlCl3 orally and IMP interperitoneally, i. p. (30 mg/wt/day) starting from week 2 till week 6 where IMP was supplement 1st and then 4 h later AlCl3 was given to mice. The fourth group received the control (IMP 30 mg/wt, i. p.) from the 2nd week till the end of the experiment. At the end of the experiment, mice were sedated with ether before being culled. To weigh the brain after excision, we used a Nimbus balance (MK, UK). In accordance with the protocol, the biochemical parameters were measured immediately after the brains were homogenized.
2.3 Object-location memory
6 weeks after beginning, an object recognition test was conducted. We put green and yellow color small plates Each mouse was adapted for 10 min in a 120 × 80 cm box. Intbox1 30 min’ intervals, they started training. 5 min were given to each mouse to practice object-location memory in a quadratic box (120 × 80 cm, 25 cm high). The apparatus was set up with two identical plastic objects 120 cm from the wall. There was a 1-day break between training and testing. The mice were tested with two identical objects 120 cm from the wall. Time spent looking at objects was used to measure animals’ behavior.
2.4 Y-Maze
6 weeks after beginning, an Y-Maze test was conducted. Studies have shown that excessive spontaneous alteration behavior is linked to enhanced cognitive performance. We followed Buried Food Test, the protocol have published by Mu Yang and Dr. Jacqueline N. Crawley. Simple Behavioral Assessment of Mouse Olfaction (Yang and Crawley, 2009). A Y-maze was used to analyze mice’s behavior. It was made of brown pointed sheets with three arms that were 60 cm long, 15 cm high, and 15 cm wide at the bottom and top. Mouse sessions lasted from three to 5 min. In the right arms of Y, there is food. The mouse capability to remember if food is located in one arm or the other (Limnios et al., 2022). By this way conditions could affect learning and memory and help to analyze the mice behavior. For the food identification, each mouse’s arm position was recorded manually 3 times in different days for 5 min each.
2.5 Evaluation of brain neurotransmitters
The acetylcholine levels were measured in brain supernatants using a colorimetric assay kit (BioVision Inc., Waltham, United States) at 570 nm (Contoli et al., 2017). Colorimetric measurements of serotonin (Chellammal et al., 2019) and dopamine (Chellammal et al., 2019) were also conducted (BioVision).
2.6 Quantification of markers of oxidative damage in brain tissue
A competitive ELISA kit (Cell Biolabs, San Diego, CA, United States) was used to measure malondialdehyde (MDA). ELISA immunoassay for MDA-protein adduct products was used for this assay. Moreover, total capacity of antioxidants was measured Capacity Assay Kit (Cell Biolabs) based on copper (II) being reduced to copper (I) by antioxidants. By reducing copper (I) ions, a coupling chromogen increases 490 nm absorbance. The net absorbance was compared to that of the standard curve based on known uric acid concentrations. An ELISA kit for mice catalase (CAT) (Cusabio, Wuhan, China) was used to measure catalase activity.
2.7 Western blot
Western blot was conducted according to a standard method. Isolated proteins were electrophoresed on SDS-PAGE gels and then transferred to PVDF membranes. The blots were probed overnight at 4°C using primary antibodies (Supplementary Table S1) after being blocked with 5% nonfat dry milk for 60 min. Blots were then incubated with the suitable HRP-conjugated secondary antibody (Supplementary Table S1) for 60 min at room temperature. To visualize the protein bands, we used Digit Blot (a 3,600-00-C-) Scanner. The untreated control band was normalized to 1 with Image Studio Lite software (Lincoln, NE, United States). We repeated each experiment three times.
2.8 Estimation of pro-inflammatory biomarkers
E-labscience (Beijing, China) ELISA Kits were used to determine IL-1β and TNF-α levels in the brain. The Sandwich-ELISA principle is used in this ELISA kit. A TNF-α and IL-1β specific antibody has been pre-coated on the micro ELISA plate in this kit. ELISA plates are filled with standards or samples and specific antibodies are added. A biotinylated detection antibody and Avidin-Horseradish Peroxidase (HRP) conjugate are added successively to each microplate well and incubated. Components that are not needed are washed away. The substrate solution is added to each well. Wells containing Mouse TNF-α and IL-1β, biotinylated detection antibody, and Avidin-HRP conjugate will be blue. When stop solution is added, the enzyme-substrate reaction stops and the color turns yellow. In spectrophotometry, optical density is measured at 450 nm ± 2 nm. A high OD value means more Mouse TNF-α and IL-1β. Using the standard curve, we can calculate the concentration of Mouse TNF in the samples. A Molecular Devices SpectraMaxTM Multimodal plate reader was used to get all the readings.
2.9 Histopathology
The brain tissues were embedded in paraffin and fixed in 10% formalin to prepare them for histopathology. Four-mm-wide sections of embedding brain tissues lobes stained with H&E. An optical microscope was used to study the prepared sections. A Leica D6000 microscope (Leica, Wetzlar, Germany) was used for measurements, and microscopy obtained images from cerebral cortex (×200 magnification).
2.10 Statistical analysis
Data analysis for behavioral, biochemical and histopathological evaluations was done using Prism 8 (GraphPad). Differences between groups were analyzed using ANOVA, followed by Tukey’s post hoc test. Data are presented as means ± SD. For in vivo experiments, at least three independent experiments were performed. The probability value of 0.05 was considered to be significant variation.
3 Results
3.1 Effect of IMP on AlCl3-induced body weight of experimental animals
First, we have compared the body weight (initial and end) of treated groups. According to the statistical analysis the end of body weight values show significant differences among the studied groups, (Figures 1A, B).
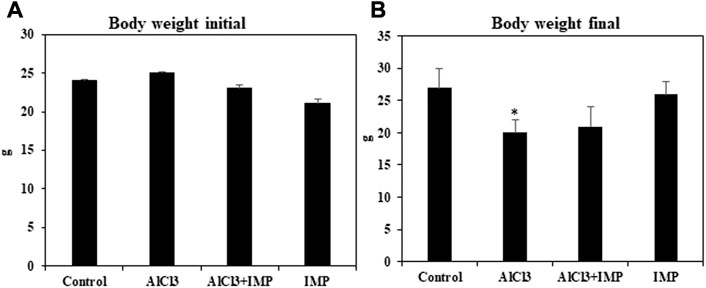
FIGURE 1. Effect of IMP on body and brain weight of experimental animals. (A) Body weight of animal starting date (g). (B) Body weight of the animal at the end of experimental date (g). The difference between the treatment and control groups was statistically significant; *p < 0.05, #p < 0.05 was considered statistically significant. Values are expressed as mean ± SD, n = 3. *p < 0.05 indicates significant differences between the AlCl3 alone group and the control group. #p < 0.05 shows significant differences between AlCl3 alone and IMP with AlCl3.
3.2 Effect of IMP on mice behavior
3.2.1 Object-location memory test
In rodent models of CNS disorders, object-location memory is used to assess cognition, and specifically spatial memory and discrimination. The effect of IMP treatment on novel object memory was also explored. A noticeable increase in the object memory index was found in the control group when the novel object-location memory test was compared to the training session. Treatment with AlCl3 also worsened amnesia (Figure 2A). However, pretreatment with IMP (30 mg/kg) prevented deficits in memory (p 0.05) caused by long-term exposure to AlCl3 (Supplementary Vedio S1). Therefore, IMP protects mice from the effects of AlCl3 on memory and object location.
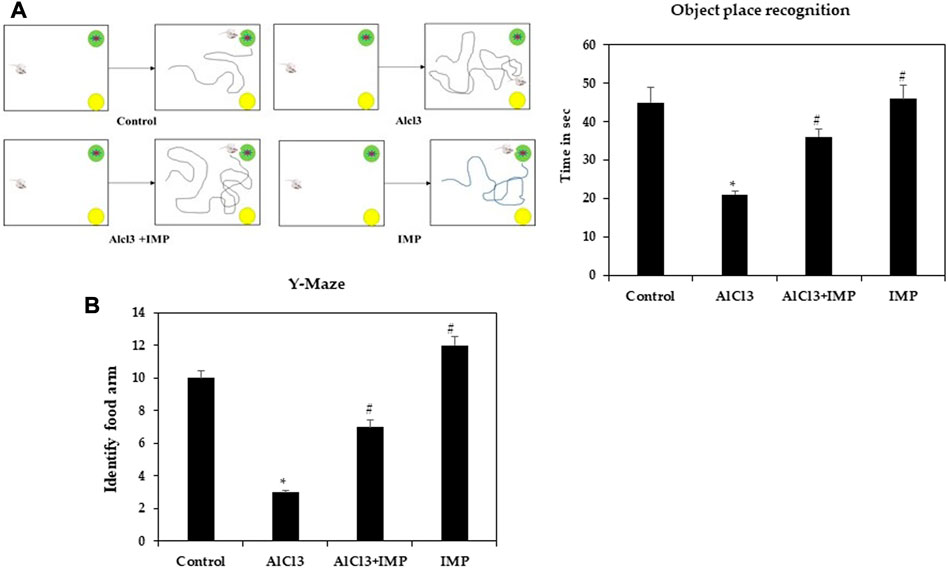
FIGURE 2. Spatial memory learning of mice. (A) Object place recognition test. (B) Y-maze test. The difference between the treatment and control groups was statistically significant; *p < 0.05, #p < 0.05 was considered statistically significant. Values are expressed as mean ± SD, n = 3. *p < 0.05 indicates that there are significant differences between the AlCl3 alone group and the control group. #p < 0.05 shows significant differences between AlCl3 alone and IMP with AlCl3.
3.2.2 IMP prevented memory damage
Spatial working memory was analyzed using a Y-maze task. Food rewards were placed around the arms of mice treated with AlCl3 were less likely to enter. When mice received IMP alone or in combination with AlCl3, altered behavior was significantly modulated (p < 0.05), indicating that IMP improved memory in AlCl3-treated mice (Figure 2B). There were no significant differences between group IV (Figure 2B) and the control group in response to the food rewards placed in the arms [(Supplementary Vedio S1)]. Then, IMP may improve the working memory.
3.3 IMP improved ach levels in mice with AlCl3-induced neurotoxicity
The acetylcholine, the first identified neurotransmitter, plays a significant role in hippocampal memory and AD pathogenesis. It is synthesized by choline acetyltransferase, AchE, an enzyme found in cerebrospinal fluid and plasma. The AchE is an enzyme that metabolizes Ach at synaptic clefts (Karami et al., 2021; Huang et al., 2022). To further test neuroprotective effects of IMP on brain damage, we examined cholinergic dysfunction, as indicated by a decreased Ach content (5.5 fold). Figure 3A shows that IMP reversed the reduction in Ach content (5 fold). The AlCl3 group had significantly lower dopamine and serotonin levels than the control group, and the AlCl3 + IMP group had significantly higher levels than the AlCl3 group (Figures 3B, C). Our findings suggest that IMP (30 mg/wt) helps alleviate AlCl3-induced neurotoxicity.
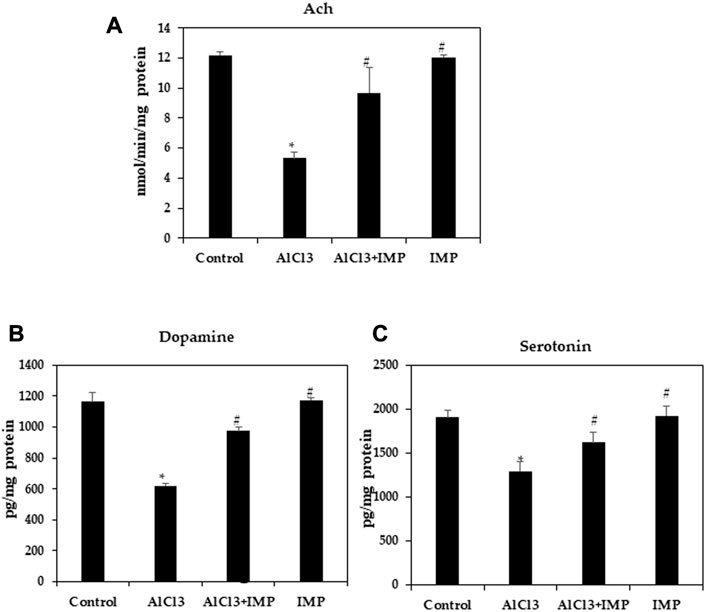
FIGURE 3. Effect of IMP on the level of Ach, Serotonin and Dopamine. (A) Level of acetylcholine concentration (nmol/min/mg protein). (B) Level of dopamine (pg/mg protein). (C) Level of serotonin (pg/mg protein). The difference between the treatment and control groups was statistically significant; *p < 0.05, #p < 0.05 was considered statistically significant. Values are expressed as mean ± SD, n = 3. *p < 0.05 indicates that there are significant differences between the AlCl3 alone group and the control group. #p < 0.05 shows significant differences between AlCl3 alone and IMP with AlCl3.
3.4 IMP downregulates pro-inflammatory markers
Neuroinflammation and monoamine neurotransmitters are closely related to AlCl3-induced neuronal damage (Stephenson et al., 2018; Ji et al., 2021). Enzyme-linked immunoassay was used to detect pro-inflammatory cytokine levels in mice. In Figures 4A, B, TNF-α and IL-1β levels in mouse brains were remarkably higher after AlCl3 treatment, but IMP (30 mg/wt) induced a significant decrease in these levels. Then, IMP reduced the inflammation caused by AlCl3.
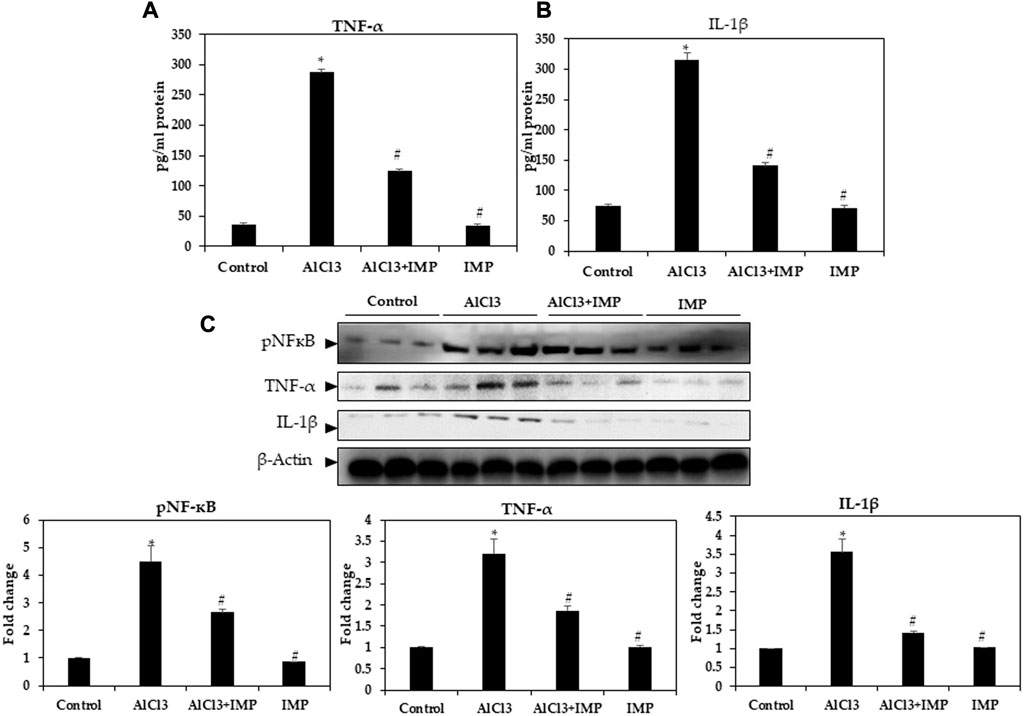
FIGURE 4. Level of pro-inflammatory cytokines in brain homogenates. (A) Level of TNF-α (pg/mg protein). (B) Level of IL-1β (pg/mg protein). (C) Western blot analysis of NF-кB and the pro-inflammatory cytokine (TNF-α and IL-1β) expression in brain homogenates. The difference between the treated groups and the control group was calculated; *p < 0.05, #p < 0.05 was considered statistically significant. Values are expressed as mean ± SD, n = 3. *p < 0.05 indicates significant differences between AlCl3 treated group and the control group. #p < 0.05 shows significant differences between AlCl3 alone and IMP with AlCl3.
3.5 IMP inhibits NF-кB activation and its associated inflammatory cytokines in AlCl3-induced mice
Innate and adaptive immune functions are controlled by NFκB (Mulero et al., 2019; Capece et al., 2022). It also mediates inflammation (Rajendran et al., 2018; Nichols et al., 2019; Peng et al., 2019). In IMP-pretreated and AlCl3-stimulated mouse brain tissues, we tested the efficacy of NFB in suppressing inflammation. Figure 3C illustrates that nuclear protein extracts from the brain tissue showed a remarkable increase in p65 levels after AlCl3 stimulation. AlCl3-induced p65 levels were significantly attenuated by IMP pretreatment. Next, we tested the effect of IMP on TNF-α and IL-1β by Western blotting. The Western blot results showed that AlCl3 stimulation overexpressed TNF-α and IL-1β. However, IMP suppressed this effect significantly, suggesting that IMP suppressed the activation of p65, which further suppressed the expression of inflammatory enzymes and cytokines (Figure 4C).
3.6 Effect of aluminum treatment and IMP on LPO and antioxidant enzymes
Various oxidant and antioxidant enzymes tightly regulate ROS production in cells as by-products of oxidative metabolism (Rajendran et al., 2014; Snezhkina et al., 2019). MDA is an indicator of oxidative stress caused by lipid peroxidation in cells and tissue (Fauziah et al., 2018; Subramanyam et al., 2018). Our first experiment was conducted to observe how IMP affected AlCl3-induced MDA levels. MDA levels significantly increased in mice exposed to 10 mg/kg AlCl3 for 6 weeks (Figure 5A). A consistent, significant decrease in Catalase and TAC content was caused by AlCl3 (Figures 5B, C). All of these pro-oxidative stress effects were potently inhibited by the co-administration of IMP. These results suggest that IMP protects the brain from oxidative damage caused by AlCl3.
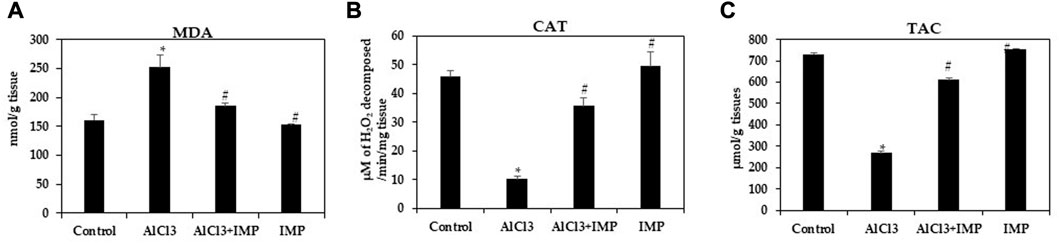
FIGURE 5. Activity of antioxidant enzymes. (A) Formation of MDA in brain homogenates (nmol/g tissue). (B) The activity of catalase (µM of H2O2 decomposed/min/mg tissue). (C) Total antioxidant capacity (µmol/g tissues). The difference between the treatment and control groups was statistically significant; *p < 0.05, #p < 0.05 was considered statistically significant. Values are expressed as mean ± SD, n = 3. *p < 0.05 indicates that there are significant differences between the AlCl3 alone group and the control group. #p < 0.05 shows significant differences between AlCl3 alone and IMP with AlCl3.
3.7 MAPK signaling pathway is affected by IMP
Inflammation and apoptosis are triggered by MAPKs, including JNK and p38 kinases (Kasuya et al., 2018; Aluko et al., 2021; Ijomone et al., 2021). As shown in Figure 6, AlCl3 increased the phosphorylation of the MAPKs p38 and JNK by AlCl3, while phosphorylation was inhibited by IMP treatment. IMP suppresses p38 and JNK activation. Thus, IMP attenuates inflammation by downregulating MAPK signaling through the inhibition of p38 and JNK activation in AlCl3-induced brain damage in mice.
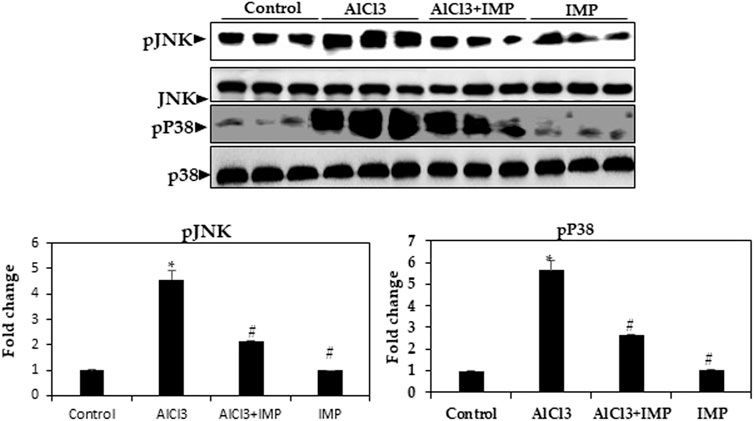
FIGURE 6. Expression of MAPK kinase in brain homogenates. Western blot analysis of brain homogenates determines the expression of pJNK and pP38—total JNK and total p38 as a loading control. The difference between the treatment and control groups was statistically significant; *p < 0.05, #p < 0.05 was considered statistically significant. Values are expressed as mean ± SD, n = 3. *p < 0.05 means that there are significant differences between the AlCl3-alone group and the control group. #p < 0.05 shows significant differences between AlCl3 alone and IMP with AlCl3.
3.8 AlCl3-stimulated ROS generation is attenuated by IMP
HO-1 and NQO are antioxidant enzymes that are regulated by the Nrf2 pathway (Wang et al., 2000; Li et al., 2014; Yang et al., 2018b). To understand the molecular mechanisms underlying the protective effects of IMP, we studied the Nrf2 pathway in mouse brain tissue. In mouse brains, exposure to AlCl3 for 6 weeks inhibited Nrf2 expression, which was profoundly restored by the co-administration of IMP (30 mg/wt) (Figure 7). We also evaluated Nrf2-dependent anti-oxidative and detoxifying enzymes. Compared with the AlCl3 group, the AlCl3 + IMP group demonstrated increased protein expression of HO-1 (Figure 6). NQO1 expression was partially rescued by IMP when AlCl3 decreased it. Overall, IMP treatment rescued AlCl3-induced oxidative damage by activating Nrf2.
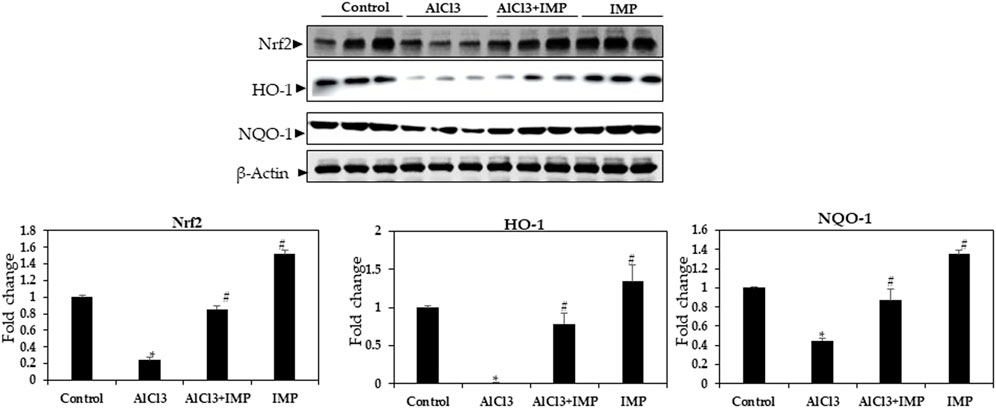
FIGURE 7. Expression of Nrf2 in brain homogenates. Western blot analysis of Nrf2, HO-1, and NQO-1 expression in control and experimental animals. The difference between the treatment and control groups was statistically significant; *p < 0.05, #p < 0.05 was considered statistically significant. Values are expressed as mean ± SD, n = 3. *p < 0.05 indicates significant differences between the AlCl3-alone group and the control group. #p < 0.05 shows significant differences between AlCl3 alone and IMP with AlCl3.
3.9 Histopathological evaluation of brain tissue
Figure 8 showed cerebral histopathological analysis. Control group showing normal cerebral cortex composed of six layers (I-VI). I- Molecular layer, II- External granular layer, III- External pyramidal layer, IV- Internal granular layer, V- Internal pyramidal layer, VI- Multiform layer. Pyramidal cells (P) have multipolar shape, vesicular nuclei, and basophilic cytoplasm while granular cells (G) have small nuclei and little cytoplasm. Smaller neuroglia cells appear scattered (N). AlCl3 group showing distortion and disappearance of normally arranged cortical layers and congested blood vessels (BV), neurophil vacuolation (black arrows), degenerating neurons with pyknotic nuclei in panel (red arrows) and vacuolation (*). AlCl3 + IMP Showing some disorganized layers, shrunken pyramidal cells (P black arrows) with loss of processes, dark cytoplasm and small darkly stained nucleus while granular cells (G) are surrounded by halos, degenerating neurons with pyknotic nuclei and dilated blood vessel (BV). IMP alone treated animals showing an improvement in histoarchitecture of organized layers, Pyramidal cells (P) and granular cells (G) appear more normal and few of degenerating neurons with pyknotic nuclei (red arrows). Together these data indicate that IMP partially reversed the effects of chronic AlCl3.
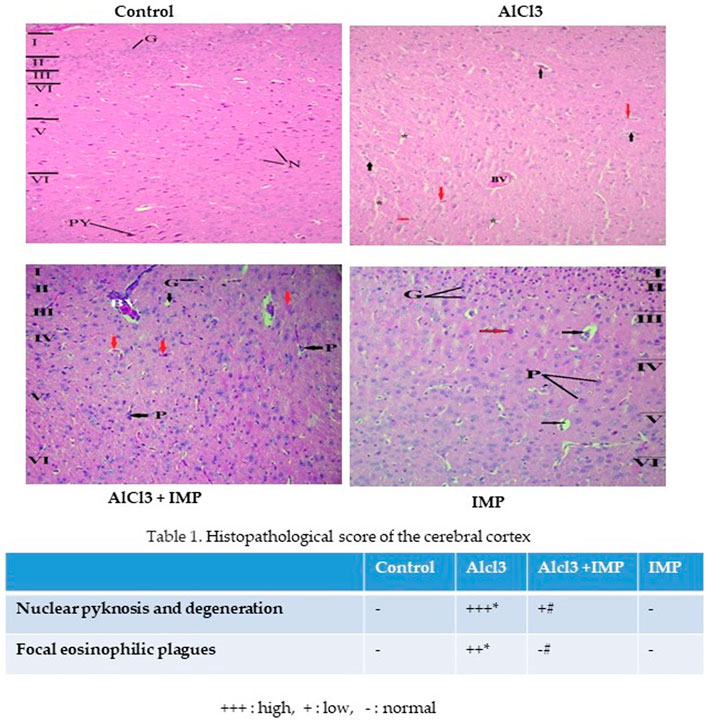
FIGURE 8. Histopathological analysis of brain tissue. The difference between the treatment and control groups was statistically significant; *p < 0.05, #p < 0.05 was considered statistically significant. Values are expressed as mean ± SD, n = 3. *p < 0.05 indicates significant differences between the AlCl3-alone group and the control group. #p < 0.05 shows significant differences between AlCl3 alone and IMP with AlCl3.
3 Discussion
In the present study, we show that IMP protects the brain from alunimium toxicity through modulating the levels of antioxidant and anti-inflammatory markers. In addition, it can partially reduce the effects of chronic AlCl3 exposure on object recognition index and spatial working memory.
Neurodegenerative disease progression has been extensively shown to be influenced by oxidative stress, inflammation, and apoptosis (Behl et al., 2021; Merelli et al., 2021; Zarneshan et al., 2022). In this regard, previous studies indicated that Al exposure can induce various neurological disorders (Aly et al., 2011; Hamdan et al., 2022; Turkez et al., 2022). Therefore, chronic exposure to Al in mouse models has frequently been used as an experimental model of neurodegenerative diseases. Supplemented AlCl3 is able pass through the blood-brain barriers and then induces neuroinflammation (Baydar et al., 2003; He et al., 2022). Moreover, AlCl3 is found to activate oxidative mediators (Abbas et al., 2022). Acute and chronic neurodegenerative diseases are closely related to excessive AlCl3 accumulation because they activate ROS production and oxidative neurotoxicity (Khalid et al., 2020; Li et al., 2020; Madi et al., 2020).
The current study results showed that IMP partially reversed the effects of chronic AlCl3 exposure on object recognition index and spatial working memory. During depression, the central serotonergic system is hypofunctional and the brain serotonin levels are lower than normal (Hashmi et al., 2018; Kaur et al., 2022). Consistent with that, AlCl3 reduced brain serotonin levels, while AlCl3/IMP combined treatment maintained adequate levels, which may explain the mitigation of depressive symptoms. In addition, exposure to AlCl3 reduced the ACh concentration in the brain. Suppressed cholinergic transmission was reported to activate the inflammatory system by increasing N-methyl-D-aspartate receptor expression, disrupting cognition, and increasing neurotoxicity (Haider et al., 2020; Amanzadeh Jajin et al., 2021; Firdaus et al., 2022). AlCl3 may cause neurodegeneration through multiple interlocking pathogenic pathways (Wang et al., 2019; Shunan et al., 2021; Hamdan et al., 2022). However, one of the reasons that IMP is effective against Al-induced neurotoxicity is probably due to its anti-inflammatory action on cytokines and its role in normalizing ACh levels in the brain.
Activation of microglia in brain disease was reported to be associated with the release of copious quantities of pro-inflammatory cytokines, which were believed to contribute to neuronal death and degeneration during neuroinflammation and brain disease (Ahmad et al., 2019). In the Nrf2/HO-1 axis of the AlCl3 group, NF-кb expression showed a significant increase, thereby increasing pro-inflammatory cytokines, including TNF-α and IL-1β, causing neuroinflammation in the brain (Hamdan et al., 2022). Natural flavonoids have been studied for their multiple neuroprotective properties, including their role in suppressing inflammation and neuronal apoptosis as well as in boosting neuronal survival and memory (Infante-Garcia and Garcia-Alloza, 2019). Our findings indicate that IMP suppressed the pro-inflammatory mediators induced by AlCl3 and consequently attenuated AlCl3-induced neuroinflammation in mice. MAPK pathway proteins such as p38, JNK, and ERK1/2 have been reported to contribute to NF-κB activation. The phosphorylation of MAPKs is essential for regulating cell division, proliferation, and differentiation. Ruther more, MAPKs can trigger apoptosis (Kahkhaie et al., 2019; Yu et al., 2019; Ijomone et al., 2021; Huang et al., 2022). Moreover, as shown above, IMP suppressed the activation of p38 and JNK caused by AlCl3. Based on that, IMP appers to exert anti-inflammatory effects in mice treated with AlCl3 through inhibiting the MAPK signaling pathway.
Neurodegenerative diseases are associated with an imbalance between free radicals (i.e., ROS) and antioxidants and antioxidant enzymes (Ajayi et al., 2021; Bardallo et al., 2022; Kısadere et al., 2022), leading to the induction of oxidative stress (Haider et al., 2020; Amanzadeh Jajin et al., 2021; Abdel-Salam, 2022; Mishra et al., 2022). The main antioxidant enzymes that protect against the harmful effects of ROS in cells are SOD and CAT, are among the brain’s most prominent antioxidant enzymes. Additionally, GSH-Px plays a crucial role in preventing membrane damage that may result from lipid peroxidation. In this regard, SOD, CAT, and GSH-px activity levels were found to be associated with the ability of the organism to eliminate free radicals from the environment (Stankovic et al., 2020). Related to that, we detected changes in these molecules, where the brain MDA levels in AlCl3 treated group showed a significant increase relative to the control group. Since dopamine and serotonergic signaling are tightly regulated, IMP sems to reverse these abnormalities by preserving serotonergic signaling by directly protecting serotonergic neurons or by maintaining dopamine levels.
In the current study, IMP increased Nrf2 activation and HO-1, NQO-1, and CAT expression in mouse brain tissue, thereby reducing AlCl3-induced ROS production. AlCl3-induced oxidative stress was attenuated by IMP by activating Nrf2, Nrf2, a critical signaling molecule for ROS detoxification in the brain, is essential for protecting the brain from oxidative stress (Bardallo et al., 2022). In addition, various cellular antioxidants are regulated by Nrf2 (Gureev and Popov, 2019). Phosphorylation of Keap1 causes Nrf2 to migrate to the nucleus when cells it is exposed to neurodegenerative disease-induced oxidative stress (Zhou et al., 2018; Ji et al., 2021; Zong et al., 2021; Mehrbeheshti et al., 2022). In parallel with that, Nrf2 signaling pathway is activated in HT22 cells by antioxidant enzymes such as catalase, NQO-1, and HO-1 (Lone et al., 2018). Thus, the activated Nrf2 as a result of IMP treatment protects the brain from the oxidative damage induced after exposure to AlCl3. In the present study when mice are treated with AlCl3, inflammation occurs, which increases expression of inflammatory markers such as TNF-, IL-1b, and NF-kB. TNF-1b, NF-kB, and MAPK activation are attenuated by supplementation with IMP. IMP supplementation attenuates Nrf2 and antioxidant enzymes activation during AlCl3 treatment. A representative shame to summarize that is shown in Figure 9.
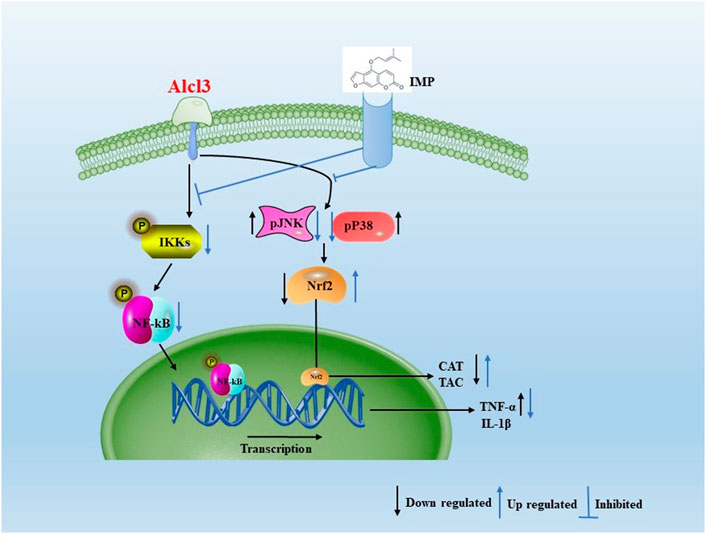
FIGURE 9. Aproposed scheme to summarizing the obtained results. Mechanistic interaction between signaling molecules is shown. In the present study when mice are treated with AlCl3, inflammation occurs, which increases expression of inflammatory markers such as TNF-α, IL-1β, and NF-kB. TNF-1β, NF-kB, and MAPK activation are attenuated by supplementation with IMP. IMP supplementation attenuates Nrf2 and antioxidant enzymes activation during AlCl3 treatment.
4 Conclusion
In the present study, systemic administration of AlCl3 to mice resulted in abnormal behavior, neurotransmitter deficits, and reduced Nrf2 expression in the brain, reflecting oxidative inflammatory stress. Due to its potential antioxidant and anti-inflammatory activities, IMP partially reversed the toxic effects of AlCl3. The results obtained in this work in the AlCl3 model lead us to suggest the IMP as an ideal candidate in diseases that involve processes involving inflammation, dysfunction in synaptic system and neurodegeneration in several areas of the brain and spinal cord.
Data availability statement
The original contributions presented in the study are included in the article/Supplementary Material, further inquiries can be directed to the corresponding authors.
Ethics statement
The animal study was reviewed and approved by Research Ethics Committee at King Faisal University Declaration of King Faisal University, and approved by the Institutional Review Board (KFU-REC/2021-04-22).
Author contributions
Conceptualization, DA and PR; methodology, PR; validation, PR, MB-I, and EA; formal analysis, MB-I and EA; investigation, DA and PR; resources, DA, PR, and EA data curation, MB-I and EA; writing—DA, PR, and EA; writing—review and editing, MB-I and EA; visualization, PR; supervision, DA; project administration, PR; funding acquisition, PR. All authors have read and agreed to the published version of the manuscript.
Funding
The authors extend their appreciation to the Deputyship for Research and Innovation, Ministry of Education in Saudi Arabia, for funding this research work through the Project number INST174.
Conflict of interest
The authors declare that the research was conducted in the absence of any commercial or financial relationships that could be construed as a potential conflict of interest.
Publisher’s note
All claims expressed in this article are solely those of the authors and do not necessarily represent those of their affiliated organizations, or those of the publisher, the editors and the reviewers. Any product that may be evaluated in this article, or claim that may be made by its manufacturer, is not guaranteed or endorsed by the publisher.
Supplementary material
The Supplementary Material for this article can be found online at: https://www.frontiersin.org/articles/10.3389/fphar.2023.1103940/full#supplementary-material
References
Abbas, F., Eladl, M. A., El-Sherbiny, M., Abozied, N., Nabil, A., Mahmoud, S. M., et al. (2022). Celastrol and thymoquinone alleviate aluminum chloride-induced neurotoxicity: Behavioral psychomotor performance, neurotransmitter level, oxidative-inflammatory markers, and BDNF expression in rat brain. Biomed. Pharmacother. 151, 113072. doi:10.1016/j.biopha.2022.113072
Abdel-Salam, O. M. (2022). The neurotoxic effects of cannabis on brain: Review of clinical and experimental data. J. Mol. Sci. Appl. 2022, 11–23.
Ahmad, M. H., Fatima, M., and Mondal, A. C. (2019). Influence of microglia and astrocyte activation in the neuroinflammatory pathogenesis of Alzheimer’s disease: Rational insights for the therapeutic approaches. J. Clin. Neurosci. 59, 6–11. doi:10.1016/j.jocn.2018.10.034
Ajayi, L. O., Ayeleso, A. O., and Oyedepo, T. A. (2021). Protective effect of hydroethanolic leaf extract of Parquetina nigrescens against D-galactose-induced neurotoxicity in male Wistar rats. Chem. Biol. Lett. 8, 79–87.
Aluko, O. M., Lawal, S. A., Ijomone, O. M., and Aschner, M. (2021). Perturbed MAPK signaling in ASD: Impact of metal neurotoxicity. Curr. Opin. Toxicol. 26, 1–7. doi:10.1016/j.cotox.2021.03.009
Aly, H. F., Metwally, F. M., and Ahmed, H. H. (2011). Neuroprotective effects of dehydroepiandrosterone (DHEA) in rat model of Alzheimer's disease. Acta Biochim. Pol. 58, 513–520. doi:10.18388/abp.2011_2218
Amanzadeh Jajin, E., Esmaeili, A., Rahgozar, S., and Noorbakhshnia, M. (2021). Quercetin-conjugated superparamagnetic iron oxide nanoparticles protect AlCl3-induced neurotoxicity in a rat model of Alzheimer’s disease via antioxidant genes, APP gene, and miRNA-101. Front. Neurosci. 14, 598617. doi:10.3389/fnins.2020.598617
Bardallo, G., Panisello-Roselló, A., Sanchez-Nuno, S., Alva, N., Roselló-Catafau, J., and Carbonell, T. (2022). Nrf2 and oxidative stress in liver ischemia/reperfusion injury. FEBS J. 289, 5463–5479. doi:10.1111/febs.16336
Baydar, T., Papp, A., Aydin, A., Nagymajtenyi, L., Schulz, H., Isimer, A., et al. (2003). Accumulation of aluminum in rat brain. Biol. trace Elem. Res. 92, 231–244. doi:10.1385/BTER:92:3:231
Behl, T., Makkar, R., Sehgal, A., Singh, S., Sharma, N., Zengin, G., et al. (2021). Current trends in neurodegeneration: Cross talks between oxidative stress, cell death, and inflammation. Int. J. Mol. Sci. 22, 7432. doi:10.3390/ijms22147432
Bondy, S. C., and Campbell, A. (2021). Aluminum and neurodegenerative disease. Handb. Neurotox., 1–21. doi:10.1007/978-3-030-71519-9_231-1
Capece, D., Verzella, D., Flati, I., Arboretto, P., Cornice, J., and Franzoso, G. (2022). NF-κB: Blending metabolism, immunity, and inflammation. Trends Immunol. 43, 757–775. doi:10.1016/j.it.2022.07.004
Chahardoli, A., Qalekhani, F., Shokoohinia, Y., and Fattahi, A. (2022). Isoimperatorin-mediated green-synthesized silver nanoparticles: Antibacterial, antioxidant, cytotoxicity, hemolytic and coagulation effects. Bull. Mater. Sci. 45, 88–97. doi:10.1007/s12034-022-02665-w
Chellammal, H. S. J., Veerachamy, A., Ramachandran, D., Gummadi, S. B., Manan, M. M., and Yellu, N. R. (2019). Neuroprotective effects of 1`δ-1`-acetoxyeugenol acetate on Aβ(25-35) induced cognitive dysfunction in mice. Biomed. Pharmacother. 109, 1454–1461. doi:10.1016/j.biopha.2018.10.189
Chen, G., Liu, Y., Xu, Y., Zhang, M., Guo, S., and Zhang, G. (2021). Isoimperatorin exerts anti-inflammatory activity by targeting the LPS-TLR4/MD-2-NF-κB pathway. Eur. J. Inflamm. 19, 205873922110005. doi:10.1177/20587392211000573
Chen, Y., Tian, X., and Wang, X. (2018). Advances in dialysis encephalopathy research: A review. Neurol. Sci. 39, 1151–1159. doi:10.1007/s10072-018-3426-y
Cherrak, S. A., Mokhtari-Soulimane, N., Berroukeche, F., Bensenane, B., Cherbonnel, A., Merzouk, H., et al. (2016). In vitro antioxidant versus metal ion chelating properties of flavonoids: A structure-activity investigation. PloS one 11, e0165575. doi:10.1371/journal.pone.0165575
Contoli, M., Marcellini, A., Caramori, G., and Papi, A. (2017). “Up-regulation of the cholinergic system in A model of ex-vivo virus-induced copd exacerbation-role of tiotropium bromide,” in C73. New mechanisms and therapies in copd (American Thoracic Society), A6288.
Dalla Torre, G., Mujika, J. I., Lachowicz, J. I., Ramos, M. J., and Lopez, X. (2019). The interaction of aluminum with catecholamine-based neurotransmitters: Can the Formation of these species be considered a potential risk factor for neurodegenerative diseases? Dalton Trans. 48, 6003–6018. doi:10.1039/c8dt04216k
de Souza, S. M., Delle Monache, F., and Smânia, A. (2005). Antibacterial activity of coumarins. Z. fuer Naturforsch. C 60, 693–700. doi:10.1515/znc-2005-9-1006
Del Giudice, G., Rappuoli, R., and Didierlaurent, A. M. (2018). “Correlates of adjuvanticity: A review on adjuvants in licensed vaccines,” in Proceedings of the seminars in immunology, 14–21.
Dordevic, D., Buchtova, H., Jancikova, S., Macharackova, B., Jarosova, M., Vitez, T., et al. (2019). Aluminum contamination of food during culinary preparation: Case study with aluminum foil and consumers’ preferences. Food Sci. Nutr. 7, 3349–3360. doi:10.1002/fsn3.1204
Fauziah, P. N., Maskoen, A. M., Yuliati, T., and Widiarsih, E. (2018). Optimized steps in determination of malondialdehyde (MDA) standards on diagnostic of lipid peroxidation. Padjadjaran J. Dent. 30, 136–139. doi:10.24198/pjd.vol30no2.18329
Firdaus, Z., Kumar, D., Singh, S. K., and Singh, T. D. (2022). Centella asiatica alleviates AlCl3-induced cognitive impairment, oxidative stress, and neurodegeneration by modulating cholinergic activity and oxidative burden in rat brain. Biol. Trace Elem. Res. 200, 5115–5126. doi:10.1007/s12011-021-03083-5
Goullé, J.-P., and Grangeot-Keros, L. (2020). Aluminum and vaccines: Current state of knowledge. Med. maladies Infect. 50, 16–21. doi:10.1016/j.medmal.2019.09.012
Gureev, A. P., and Popov, V. N. (2019). Nrf2/ARE pathway as a therapeutic target for the treatment of Parkinson diseases. Neurochem. Res. 44, 2273–2279. doi:10.1007/s11064-018-02711-2
Haider, S., Liaquat, L., Ahmad, S., Batool, Z., Siddiqui, R. A., Tabassum, S., et al. (2020). Naringenin protects AlCl3/D-galactose induced neurotoxicity in rat model of AD via attenuation of acetylcholinesterase levels and inhibition of oxidative stress. PloS one 15, e0227631. doi:10.1371/journal.pone.0227631
Hamdan, A. M. E., Alharthi, F. H. J., Alanazi, A. H., El-Emam, S. Z., Zaghlool, S. S., Metwally, K., et al. (2022). Neuroprotective effects of phytochemicals against aluminum chloride-induced Alzheimer’s disease through ApoE4/LRP1, wnt3/β-catenin/gsk3β, and TLR4/NLRP3 pathways with physical and mental activities in a rat model. Pharmaceuticals 15, 1008. doi:10.3390/ph15081008
Hashmi, W. J., Ismail, H., Mehmood, F., and Mirza, B. (2018). Neuroprotective, antidiabetic and antioxidant effect of Hedera nepalensis and lupeol against STZ+ AlCl3 induced rats model. DARU J. Pharm. Sci. 26, 179–190. doi:10.1007/s40199-018-0223-3
Hassan, S. A., and Kadry, M. O. (2021). Neurodegenerative and hepatorenal disorders induced via aluminum chloride in murine system: Impact of β-secretase, MAPK, and KIM. Biol. trace Elem. Res. 199, 227–236. doi:10.1007/s12011-020-02132-9
He, X., Yan, C., Zhao, S., Zhao, Y., Huang, R., and Li, Y. (2022). The preventive effects of probiotic Akkermansia muciniphila on D-galactose/AlCl3 mediated Alzheimer's disease-like rats. Exp. Gerontol. 170, 111959. doi:10.1016/j.exger.2022.111959
Huang, Q., Zhang, C., Qu, S., Dong, S., Ma, Q., Hao, Y., et al. (2022). Chinese herbal extracts exert neuroprotective effect in Alzheimer’s disease mouse through the dopaminergic synapse/apoptosis signaling pathway. Front. Pharmacol. 13, 817213. doi:10.3389/fphar.2022.817213
Hwang, Y.-H., Yang, H. J., and Ma, J. Y. (2017). Simultaneous determination of three furanocoumarins by UPLC/MS/MS: Application to pharmacokinetic study of Angelica dahurica radix after oral administration to normal and experimental colitis-induced rats. Mol. (Basel, Switz. 22, 416. doi:10.3390/molecules22030416
Ijomone, O. M., Iroegbu, J. D., Aschner, M., and Bornhorst, J. (2021). Impact of environmental toxicants on p38-and ERK-MAPK signaling pathways in the central nervous system. Neurotoxicology 86, 166–171. doi:10.1016/j.neuro.2021.08.005
Inan-Eroglu, E., and Ayaz, A. (2018). Is aluminum exposure a risk factor for neurological disorders? J. Res. Med. Sci. 23, 51. The official journal of Isfahan University of Medical Sciences.
Infante-Garcia, C., and Garcia-Alloza, M. (2019). Review of the effect of natural compounds and extracts on neurodegeneration in animal models of diabetes mellitus. Int. J. Mol. Sci. 20, 2533. doi:10.3390/ijms20102533
Ishaq, S., Liaqat, A., Hameed, A., and Ahmed, T. (2021). Biochemical mechanisms of aluminium and other metals exposure, their brain entry mechanisms, effects on blood brain barrier and important pharmacokinetic parameters in neurological disorders. Biochem. Mech. Alum. Induc. Neurological Disord. 1.
Jeffery, E. H., Abreo, K., Burgess, E., Cannata, J., and Greger, J. L. (1996). Systemic aluminum toxicity: Effects on bone, hematopoietic tissue, and kidney. J. Toxicol. Environ. Health Part A 48, 649–665. doi:10.1080/009841096161122
Ji, Y.-J., Kim, S., Kim, J.-J., Jang, G. Y., Moon, M., and Kim, H. D. (2021). Crude saponin from platycodon grandiflorum attenuates aβ-induced neurotoxicity via antioxidant, anti-inflammatory and anti-apoptotic signaling pathways. Antioxidants 10, 1968. doi:10.3390/antiox10121968
Jiang, T., Shi, X., Yan, Z., Wang, X., and Gun, S. (2019). Isoimperatorin enhances 3T3-L1 preadipocyte differentiation by regulating PPARγ and C/EBPα through the Akt signaling pathway. Exp. Ther. Med. 18, 2160–2166. doi:10.3892/etm.2019.7820
Kahkhaie, K. R., Mirhosseini, A., Aliabadi, A., Mohammadi, A., Mousavi, M. J., Haftcheshmeh, S. M., et al. (2019). Curcumin: A modulator of inflammatory signaling pathways in the immune system. Inflammopharmacology 27, 885–900. doi:10.1007/s10787-019-00607-3
Karami, A., Darreh-Shori, T., Schultzberg, M., and Eriksdotter, M. (2021). CSF and plasma cholinergic markers in patients with cognitive impairment. Front. Aging Neurosci. 13, 704583. doi:10.3389/fnagi.2021.704583
Kasuya, Y., Umezawa, H., and Hatano, M. (2018). Stress-activated protein kinases in spinal cord injury: Focus on roles of p38. Int. J. Mol. Sci. 19, 867. doi:10.3390/ijms19030867
Kaur, K., Narang, R., and Singh, S. (2022). AlCl3 induced learning and memory deficit in zebrafish. NeuroToxicology 92, 67–76. doi:10.1016/j.neuro.2022.07.004
Kawahara, M., Tanaka, K.-i., and Kato-Negishi, M. (2021). Neurotoxicity of aluminum and its link to neurodegenerative diseases. Metallomics Res. 1. rev-47-rev-65.
Khalid, A., Abbasi, U. A., Amber, S., Mirza, F. J., Asif, M., Javed, A., et al. (2020). Methylphenidate and Rosmarinus officinalis improves cognition and regulates inflammation and synaptic gene expression in AlCl3-induced neurotoxicity mouse model. Mol. Biol. Rep. 47, 7861–7870. doi:10.1007/s11033-020-05864-y
Kim, N. Y., Jung, Y. Y., Yang, M. H., Um, J.-Y., Sethi, G., and Ahn, K. S. (2022). Isoimperatorin down-regulates epithelial mesenchymal transition through modulating NF-κB signaling and CXCR4 expression in colorectal and hepatocellular carcinoma cells. Cell. Signal. 99, 110433. doi:10.1016/j.cellsig.2022.110433
Kısadere, İ., Karaman, M., Aydın, M. F., Donmez, N., and Usta, M. (2022). The protective effects of chitosan oligosaccharide (COS) on cadmium-induced neurotoxicity in Wistar rats. Archives Environ. Occup. Health 77, 755–763. doi:10.1080/19338244.2021.2008852
Kiyonga, A. N., Park, G. H., Kim, H. S., Suh, Y.-G., Kim, T. K., and Jung, K. (2021). An efficient ionic liquid-mediated extraction and enrichment of isoimperatorin from ostericum koreanum (max.) kitagawa. Molecules 26, 6555. doi:10.3390/molecules26216555
Lai, Y., Han, T., Zhan, S., Jiang, Y., Liu, X., and Li, G. (2021). Antiviral activity of isoimperatorin against influenza a virus in vitro and its inhibition of neuraminidase. Front. Pharmacol. 12, 657826. doi:10.3389/fphar.2021.657826
Lakey-Beitia, J., Burillo, A. M., La Penna, G., Hegde, M. L., and Rao, K. (2021). Polyphenols as potential metal chelation compounds against Alzheimer’s disease. J. Alzheimer's Dis. 82, S335–S357. doi:10.3233/JAD-200185
Li, L., Dong, H., Song, E., Xu, X., Liu, L., and Song, Y. (2014). Nrf2/ARE pathway activation, HO-1 and NQO1 induction by polychlorinated biphenyl quinone is associated with reactive oxygen species and PI3K/AKT signaling. Chemico-biological Interact. 209, 56–67. doi:10.1016/j.cbi.2013.12.005
Li, W., Yang, S., Xie, Z., Lu, H., Ling, J., and Cheng, W. (2020). Neuroprotective effects of salidroside against beta-amyloid-induced cognitive impairment in Alzheimer’s disease mice through the PKC/p38MAPK pathway. J. Biomaterials Tissue Eng. 10, 212–217. doi:10.1166/jbt.2020.2223
Limnios, A., Adamopoulou, C., Carretero, M. A., and Pafilis, P. (2022). Invasive Italian wall lizards outcompete native congeneric species in finding food in a Y-maze. acta ethologica. 25, 43–55. doi:10.1007/s10211-021-00385-8
Liu, Y., Tan, X., Zhang, Y., Ling, F., Liu, T., and Wang, G. I. (2022). Isoimperatorin: A promising anti gyrodactylus kobayashii natural compound from angelica dahurica. Aquaculture 560, 738552. doi:10.1016/j.aquaculture.2022.738552
Loboda, A., Damulewicz, M., Pyza, E., Jozkowicz, A., and Dulak, J. (2016). Role of Nrf2/HO-1 system in development, oxidative stress response and diseases: An evolutionarily conserved mechanism. Cell. Mol. life Sci. 73, 3221–3247. doi:10.1007/s00018-016-2223-0
Lone, A., Harris, R. A., Singh, O., Betts, D. H., and Cumming, R. C. (2018). p66Shc activation promotes increased oxidative phosphorylation and renders CNS cells more vulnerable to amyloid beta toxicity. Sci. Rep. 8(1), 1–17. doi:10.1038/s41598-018-35114-y
Lukiw, W. J., Kruck, T. P., Percy, M. E., Pogue, A. I., Alexandrov, P. N., Walsh, W. J., et al. (2019). Aluminum in neurological disease–a 36 year multicenter study. J. Alzheimer's Dis. Park. 8, 457. doi:10.4172/2161-0460.1000457
Madi, Y. F., Choucry, M. A., El-Marasy, S. A., Meselhy, M. R., and El-Kashoury, E.-S. A. (2020). UPLC-Orbitrap HRMS metabolic profiling of Cymbopogon citratus cultivated in Egypt; neuroprotective effect against AlCl3-induced neurotoxicity in rats. J. Ethnopharmacol. 259, 112930. doi:10.1016/j.jep.2020.112930
McLachlan, D. R., Bergeron, C., Alexandrov, P. N., Walsh, W. J., Pogue, A. I., Percy, M. E., et al. (2019). Retracted article: Aluminum in neurological and neurodegenerative disease. Mol. Neurobiol. 56, 1531–1538. doi:10.1007/s12035-018-1441-x
McLachlan, D. R., Bergeron, C., Alexandrov, P. N., Walsh, W. J., Pogue, A. I., Percy, M. E., et al. (2020). Retraction note: Aluminum in neurological and neurodegenerative disease. Mol. Neurobiol. 57, 1779. doi:10.1007/s12035-020-01883-9
Mehrbeheshti, N., Esmaili, Z., Ahmadi, M., and Moosavi, M. (2022). A dose response effect of oral aluminum nanoparticle on novel object recognition memory, hippocampal caspase-3 and MAPKs signaling in mice. Behav. Brain Res. 417, 113615. doi:10.1016/j.bbr.2021.113615
Mei, L., and Yao, T. (1993). Aluminum contamination of food from using aluminumware. Int. J. Environ. Anal. Chem. 50, 1–8. doi:10.1080/03067319308027577
Merelli, A., Repetto, M., Lazarowski, A., and Auzmendi, J. (2021). Hypoxia, oxidative stress, and inflammation: Three faces of neurodegenerative diseases. J. Alzheimer's Dis. 82, S109–S126. doi:10.3233/JAD-201074
Mishra, D. K., Awasthi, H., Srivastava, D., and Fatima, Z. (2022). Phytochemical: A treatment option for heavy metal induced neurotoxicity. J. Complementary Integr. Med. 19, 513–530. doi:10.1515/jcim-2020-0325
Mulero, M. C., Huxford, T., and Ghosh, G. (2019). NF-κB, IκB, and IKK: Integral components of immune system signaling. Struct. Immunol. 1172, 207–226. doi:10.1007/978-981-13-9367-9_10
Nichols, M. R., St-Pierre, M. K., Wendeln, A. C., Makoni, N. J., Gouwens, L. K., Garrad, E. C., et al. (2019). Inflammatory mechanisms in neurodegeneration. J. Neurochem. 149, 562–581. doi:10.1111/jnc.14674
Nouchikian, L., Roque, C., Song, J. Y., Rahman, N., and Ausar, S. F. (2018). An intrinsic fluorescence method for the determination of protein concentration in vaccines containing aluminum salt adjuvants. Vaccine 36, 5738–5746. doi:10.1016/j.vaccine.2018.08.005
Peng, X., Wang, Y., Li, H., Fan, J., Shen, J., Yu, X., et al. (2019). ATG5-mediated autophagy suppresses NF-κB signaling to limit epithelial inflammatory response to kidney injury. Cell death Dis. 10, 253–316. doi:10.1038/s41419-019-1483-7
Pokharel, Y. R., Han, E. H., Kim, J. Y., Oh, S. J., Kim, S. K., Woo, E.-R., et al. (2006). Potent protective effect of isoimperatorin against aflatoxin B 1-inducible cytotoxicity in H4IIE cells: Bifunctional effects on glutathione S-transferase and CYP1A. carcinogenesis 27, 2483–2490. doi:10.1093/carcin/bgl118
Principi, N., and Esposito, S. (2018). Aluminum in vaccines: Does it create a safety problem? Vaccine 36, 5825–5831. doi:10.1016/j.vaccine.2018.08.036
Rahimzadeh, M. R., Rahimzadeh, M. R., Kazemi, S., Amiri, R. J., Pirzadeh, M., and Moghadamnia, A. A. (2022). Aluminum poisoning with emphasis on its mechanism and treatment of intoxication. Emerg. Med. Int. 2022, 1480553. doi:10.1155/2022/1480553
Rajendran, P., Chen, Y. F., Chen, Y. F., Chung, L. C., Tamilselvi, S., Shen, C. Y., et al. (2018). The multifaceted link between inflammation and human diseases. J. Cell. physiology 233, 6458–6471. doi:10.1002/jcp.26479
Rajendran, P., Nandakumar, N., Rengarajan, T., Palaniswami, R., Gnanadhas, E. N., Lakshminarasaiah, U., et al. (2014). Antioxidants and human diseases. Clin. Chim. acta 436, 332–347. doi:10.1016/j.cca.2014.06.004
Roe, K. (2021). An alternative explanation for Alzheimer’s disease and Parkinson’s disease initiation from specific antibiotics, gut microbiota dysbiosis and neurotoxins. Neurochem. Res. 47, 517–530. doi:10.1007/s11064-021-03467-y
Shi, S., Zhu, H., Xia, X., Liang, Z., Ma, X., and Sun, B. (2019). Vaccine adjuvants: Understanding the structure and mechanism of adjuvanticity. Vaccine 37, 3167–3178. doi:10.1016/j.vaccine.2019.04.055
Shrivastava, B., Dipak, N. K., Karajagi, A., and Dosi, R. (2018). Severe neonatal hypercalcemia secondary to aluminium toxicity. Int. J. Contemp. Pediatr. 5, 254. doi:10.18203/2349-3291.ijcp20175595
Shunan, D., Yu, M., Guan, H., and Zhou, Y. (2021). Neuroprotective effect of Betalain against AlCl3-induced Alzheimer's disease in Sprague Dawley Rats via putative modulation of oxidative stress and nuclear factor kappa B (NF-κB) signaling pathway. Biomed. Pharmacother. 137, 111369. doi:10.1016/j.biopha.2021.111369
Snezhkina, A. V., Kudryavtseva, A. V., Kardymon, O. L., Savvateeva, M. V., Melnikova, N. V., Krasnov, G. S., et al. (2019). ROS generation and antioxidant defense systems in normal and malignant cells. Oxidative Med. Cell. Longev., 6175804.
Stankovic, J. S. K., Selakovic, D., Mihailovic, V., and Rosic, G. (2020). Antioxidant supplementation in the treatment of neurotoxicity induced by platinum-based chemotherapeutics—A review. Int. J. Mol. Sci. 21, 7753. doi:10.3390/ijms21207753
Stephenson, J., Nutma, E., van der Valk, P., and Amor, S. (2018). Inflammation in CNS neurodegenerative diseases. Immunology 154, 204–219. doi:10.1111/imm.12922
Subramanyam, D., Gurunathan, D., Gaayathri, R., and Priya, V. V. (2018). Comparative evaluation of salivary malondialdehyde levels as a marker of lipid peroxidation in early childhood caries. Eur. J. Dent. 12, 067–070. doi:10.4103/ejd.ejd_266_17
Tsai, Y.-T., Kao, S.-T., and Cheng, C.-Y. (2022). Medicinal herbs and their derived ingredients protect against cognitive decline in in vivo models of Alzheimer’s disease. Int. J. Mol. Sci. 23, 11311. doi:10.3390/ijms231911311
Turkez, H., Yıldırım, S., Sahin, E., Arslan, M. E., Emsen, B., Tozlu, O. O., et al. (2022). Boron compounds exhibit protective effects against aluminum-induced neurotoxicity and genotoxicity: In vitro and in vivo study. Toxics 10, 428. doi:10.3390/toxics10080428
Wang, L.-Y., Cheng, K.-C., Li, Y., Niu, C.-S., Cheng, J.-T., and Niu, H.-S. (2017). The dietary furocoumarin imperatorin increases plasma GLP-1 levels in type 1-like diabetic rats. Nutrients 9, 1192. doi:10.3390/nu9111192
Wang, L. (2018). “Entry and deposit of aluminum in the brain,” in Neurotoxicity of aluminum (Springer), 39–51.
Wang, Y.-J., Wang, X.-Y., Hao, X.-Y., Yan, Y.-M., Hong, M., Wei, S.-F., et al. (2019), Ethanol extract of centipeda minima exerts antioxidant and neuroprotective effects via activation of the Nrf2 signaling pathway. Oxidative Med. Cell. Longev. 2019, 9421037, doi:10.1155/2019/9421037
Wang, Y., Santa–Cruz, K., DeCarli, C., and Johnson, J. A. (2000). Nad (P) H: Quinone oxidoreductase activity is increased in hippocampal pyramidal neurons of patients with Alzheimer’s disease. Neurobiol. aging 21, 525–530. doi:10.1006/bbrc.2000.3707
Wijerathne, C. U., Seo, C.-S., Song, J.-W., Park, H.-S., Moon, O.-S., Won, Y.-S., et al. (2017). Isoimperatorin attenuates airway inflammation and mucus hypersecretion in an ovalbumin-induced murine model of asthma. Int. Immunopharmacol. 49, 67–76. doi:10.1016/j.intimp.2017.05.012
Yadav, P., Kaur, R., Kohli, S. K., Sirhindi, G., and Bhardwaj, R. (2016). Castasterone assisted accumulation of polyphenols and antioxidant to increase tolerance of B. juncea plants towards copper toxicity. Cogent Food & Agric. 2, 1276821. doi:10.1080/23311932.2016.1276821
Yang, H.-L., Lee, C.-L., Korivi, M., Liao, J.-W., Rajendran, P., Wu, J.-J., et al. (2018). Zerumbone protects human skin keratinocytes against UVA-irradiated damages through Nrf2 induction. Biochem. Pharmacol. 148, 130–146. doi:10.1016/j.bcp.2017.12.014
Yang, H. B., Gao, H. R., Ren, Y. J., Fang, F. X., Tian, H. T., Gao, Z. J., et al. (2018). Effects of isoimperatorin on proliferation and apoptosis of human gastric carcinoma cells. Oncol. Lett. 15, 7993–7998. doi:10.3892/ol.2018.8303
Yang, M., and Crawley, J. N. Simple behavioral assessment of mouse olfaction. Curr. Protoc. Neurosci. 2009, 48, Unit 8.24.24. 21–28.24. 12. doi:10.1002/0471142301.ns0824s48
Yang, Y., Jiang, S., Yan, J., Li, Y., Xin, Z., Lin, Y., et al. (2015). An overview of the molecular mechanisms and novel roles of Nrf2 in neurodegenerative disorders. Cytokine & growth factor Rev. 26, 47–57. doi:10.1016/j.cytogfr.2014.09.002
Yu, X., Zhang, F., and Shi, J. (2019). Effect of sevoflurane treatment on microglia activation, NF-kB and MAPK activities. Immunobiology 224, 638–644. doi:10.1016/j.imbio.2019.07.004
Zarneshan, S. N., Fakhri, S., and Khan, H. (2022). Targeting akt/CREB/BDNF signaling pathway by ginsenosides in neurodegenerative diseases: A mechanistic approach. Pharmacol. Res. 177, 106099. doi:10.1016/j.phrs.2022.106099
Zeng, X., MacLeod, J., Berriault, C., DeBono, N. L., Arrandale, V. H., Harris, A. M., et al. (2021). Aluminum dust exposure and risk of neurodegenerative diseases in a cohort of male miners in Ontario, Canada. Scand. J. work, Environ. health 47, 531–539. doi:10.5271/sjweh.3974
Zhou, P., Xie, W., Luo, Y., Lu, S., Dai, Z., Wang, R., et al. (2018). Protective effects of total saponins of Aralia elata (Miq.) on endothelial cell injury induced by TNF-α via modulation of the PI3K/Akt and NF-κB signalling pathways. Int. J. Mol. Sci. 20, 36. doi:10.3390/ijms20010036
Keywords: neurotoxicity, ALCL, inflammation, oxidative stress, isoimperatorin
Citation: Rajendran P, Althumairy D, Bani-Ismail M, Bekhet GM and Ahmed EA (2023) Isoimperatorin therapeutic effect against aluminum induced neurotoxicity in albino mice. Front. Pharmacol. 14:1103940. doi: 10.3389/fphar.2023.1103940
Received: 21 November 2022; Accepted: 05 April 2023;
Published: 18 April 2023.
Edited by:
Daniel Junqueira Dorta, University of São Paulo, Ribeirão Preto, BrazilReviewed by:
Francisca Pérez-Severiano, Instituto Nacional de Neurología y Neurocirugía MVS y, MexicoVenkataraman Magesh, Chung-Ang University, Republic of Korea
Copyright © 2023 Rajendran, Althumairy, Bani-Ismail, Bekhet and Ahmed. This is an open-access article distributed under the terms of the Creative Commons Attribution License (CC BY). The use, distribution or reproduction in other forums is permitted, provided the original author(s) and the copyright owner(s) are credited and that the original publication in this journal is cited, in accordance with accepted academic practice. No use, distribution or reproduction is permitted which does not comply with these terms.
*Correspondence: Peramaiyan Rajendran, cHJhamVuZHJhbkBrZnUuZWR1LnNh; Duaa Althumairy, ZGFsdGh1bWFpcnlAa2Z1LmVkdS5zYQ==; Emad A. Ahmed, ZWFhaG1lZEBrZnUuZWR1LnNh